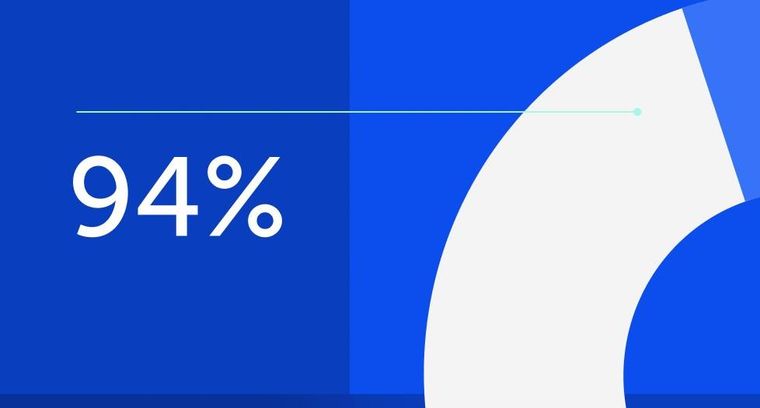
94% of researchers rate our articles as excellent or good
Learn more about the work of our research integrity team to safeguard the quality of each article we publish.
Find out more
ORIGINAL RESEARCH article
Front. Nucl. Eng., 20 November 2024
Sec. Radiation Measurements
Volume 3 - 2024 | https://doi.org/10.3389/fnuen.2024.1457737
This article is part of the Research TopicRadiation Detection and Measurement in Neutron Capture TherapyView all 5 articles
Introduction: Boron Neutron Capture Therapy (BNCT) is a promising cancer therapy. At present, development of accelerator based neutron source (ABNS) is underway to be utilized as a neutron source instead of nuclear reactor. However, it is known that the neutron field formed with accelerators have different characteristics depending on kinds of accelerators. We thus have to characterize the field before practical use.
Method: In the authors’ group, various neutronics characterization devices have been developed for our p-Li based BNCT machine named CSePT. In this paper, three neutron intensity monitor foils with an isomer production reaction for several tens to 800 keV of the p-Li neutrons were proposed, i.e., 107Ag, 115In and 189Os.
Result and Discussion: From the experimental test results, two activation foils of 107Ag and 115In were confirmed to be a possible candidate as the monitor. However, the isomer production cross sections of them should be examined for practical use.
Boron Neutron Capture Therapy (BNCT) is a promising cancer therapy in future. Accumulating 10B by administering a 10B compound in tumor cells, neutrons are then bombarded in a neutron source facility to induce a neutron nuclear reaction with 10B as Equation 1:
Emitted two charged particles have energies higher than 1 MeV. The range in a human body is around 10 μm, which is mostly the same as the size of a human body cell. If 10B would be accumulated only in tumor cells, only the tumor cells could be killed. BNCT is thus thought to be an excellent therapy which can treat tumor cells selectively with charged particles, sparing normal tissues.
BNCT has been carried out in nuclear reactors for more than 10 years as a clinical test in Japan. In recent years, epi-thermal neutrons (0.5eV–10 keV) have been begun to be used instead of thermal neutrons. Since then BNCT is recognized as a non-invasive therapy among cancer therapies. On the other hand, there is a critical problem, i.e., BNCT requires an intense epi-thermal neutron source. In the past, BNCT was performed at nuclear reactors of KUR, Kyoto Univ. (Kobayashi et al., 1990). and JRR-4, JAEA (Nakamura et al., 2011). However, especially in Japan it is not possible to construct a nuclear reactor in or close to a hospital. Under these circumstances, Japan is aiming at development and establishment of BNCT with an accelerator based neutron source (ABNS) instead of the nuclear reactor, because ABNS is safer and cheaper than nuclear reactor and can be constructed in hospitals. However, because the radiation field varies depending on kinds of accelerator and moderator, we have to conduct radiation field characterization of ABNS before practical use. As for the previous studies for this problem, basic approaches and considerations are summarized in Sauerwein’s text book for BNCT (Sauerwein et al., 2012). The more recent related researches are found in the ICNCT Conference Proceedings (Koivunoro et al., 2015).
This paper presents the development of an activation detector for measuring the source term of a neutron source used in BNCT. The key objective of this research is to experimentally verify the reliability of the evaluated nuclear data for the isotopes used, specifically validating the cross-sections listed in JENDL (JNDC, 1991). The experimental results presented here confirm the feasibility of this approach and benchmark the nuclear data of the monitor material. The method, which directly measures the source term of neutrons generated by the p-Li reaction at several hundred keV, has rarely been reported in BNCT-related studies. Therefore, this technique would be useful for other p-Li based ABNS-BNCT facilities.
The ABNS-BNCT, CSePT, utilizes the following p-Li reaction as Equation 2 (Murata et al., 2015):
In the p-Li based BNCT, ∼2.5 MeV protons are usually bombarded to a metal lithium target to produce neutrons. Figure 1 shows the neutron energy spectra (n/MeV/sr/μC) for bombarded proton energy Ep = 2.5 MeV as a function of emission angle (Drosg, 2005). The neutron energy spectrum structure is complex, i.e., the energy is lower in backward and higher in forward angles. In CSePT, an easy and convenient way to measure the absolute intensity is thus under development. The energy of neutrons to measure ranges from several tens to ∼800 keV. However, it is generally known to be difficult to measure these neutrons. In this study, the foil activation method was employed to apply to convenient characterization of an intense neutron field. However, for the neutron energy range, very few activation foils are known to be available. If the energy is lower than this energy range, that is, around eV region or lower, neutron capture reaction is available. If it is in MeV or higher region, threshold reactions like (n,p), (n,α) reactions are suitable. Several tens to 800 keV are in-between capture and threshold reactions. So, we focused on isomer production reaction induced by neutron inelastic scattering. If a certain excited state of a nuclide has its own half-life, it may play a role of activation foil. Now, assuming the excited energy is Eex and if the incident neutron energy is larger than Eex, the nuclide may have a sensitivity to make itself excited to be an isomer. In addition, if the isomer decays by emitting a gamma-ray, the energy is also Eex. If the Eex is within several tens to 800 keV, that can be used for p-Li reaction, the nuclide can be used and the emitted gamma-rays can be measured with a germanium semiconductor detector easily. According to Table of Isotopes (Firestone and Shirley, 1996), there exist over 20 candidate nuclides which can create an isomer by p-Li neutrons as listed in the following:
60Co, 77Se, 79Se, 87Sr, 94Nb, 96Tc, 99Tc, 101Rh, 103Rh, 107Pd, 107Ag, 111Cd, 113In, 115In, 117Sn, 133Ba, 134Cs, 135Ba, 154Eu, 158Tb, 163Ho, 167Er, 183W, 189Os, 193Pt.
Among them, taking into account their half-lives, threshold energies, reaction cross sections and energy dependence, we finally selected three candidate nuclides of 107Ag, 115In, 189Os. Table 1 summarizes their basic information (JNDC, 1991).
The cross sections of them are described in Figure 2 (JNDC, 1991). The energies we have to take into account are from several tens to 800 keV. From the figure 189Os has sensitivity in lower energy region and the absolute cross section value is higher than others. The threshold energy of 115In is the highest, because the first excited state energy is the highest. And 107Ag is in-between the two. The cross section value of 115In is as a result the lowest among the three and thus 107Ag is in the middle of the three. The low threshold energy means that the cross section goes up from the lower energy region as in the case of 189Os, and the absolute cross section value shows commonly higher compared with other two. However, it means that the emitted gamma-ray energy is lower (30.8 keV for 189Os), because it is the same as the threshold energy. Consequently, 189Os seems to be the best, because the cross section shows the largest among the three. However, its emitted gamma-ray energy is critically low to measure, which is a serious problem for practical use.
Figure 2. Isomer production cross sections of 107Ag, 115In and 189Os (JNDC, 1991).
In any case, the selected three foils can be utilized for the absolute intensity monitor for p-Li neutron source, because they have sensitivity in energies of interest. As mentioned above, however, each has its own feature, i.e., we have to consider their measuring purposes when we apply them to the real BNCT scene. Theoretically, they have the following characteristics for possible applications.
107Ag: Because of its short half-life, 107Ag is the most convenient monitor for an intense neutron source of the real p-Li based ABNS-BNCT machine. However, we have to notice the activity decreases very rapidly after irradiation.
115In: 115In can become the most accurate monitor, because the abundance is large and the emitted gamma-ray energy is fairly large compared to the other two
189Os: 189Os is the best monitor for measuring in the backward angle with respect to the proton beam, because the cross section in low energy region is large. However, there are two problems left, one of which is difficulty in preparing a thin sample. The other is that 189Os should be enriched because there exists stable 188Os having abundance of 13.2%.
Experimental tests were carried out before practical use for CSePT. We utilized a dynamitron accelerator of Tohoku University, Japan for the experiments. The details of the dynamitron accelerator is summarized in Table 2.
The neutron intensity is fairly low compared to the real BNCT machine. Nevertheless, by the preliminary analysis the activation is confirmed to be enough large for the present irradiation experiment. In the experiment, the proton energy was 3.5 MeV and beam current was around 3 μA. The target material is a thin 7LiF cooled by air to avoid distorting the source neutron spectrum. Figure 3 shows a photo of the experimental setup. A sample is placed at 7 cm from the target on the 0 deg. axis as in the figure. The absolute neutron intensity was determined by the activity of 7Be produced from 7Li(p,n)7Be reaction, because the number of produced 7Be is the same as the number of neutrons emitted. The number of neutrons in an experiment can be fixed by making difference of the activities measured before and after irradiation. The accuracy of this method is generally very high, because several hundred Bq can be obtained after only 1 h irradiation. The relative neutron intensity was monitored by a BF3 counter located behind the sample on the 0 deg. axis.
Experiments were carried out for 107Ag and 115In. For 189Os, it was not possible to prepare a thin sample. Irradiations were performed at 0 deg. and 90 deg. directions. Since the half-life of 107Ag* is short, we adopted 5 min irradiation, 1 min Cooling and 5 min Measurement. The reason of 1 min Cooling is that it takes time to remove the sample and set it in a germanium semiconductor detector. We repeated this irradiation and measurement 20 times. After the experiment, we decided to exclude the data of 90 deg., because of the low measurement accuracy. For 115In, the irradiation time was 19,000 s for 0 deg. and 27,600 s for 90 deg. Cooling of 116In was not considered because the contribution was found to be very small. The measurement started after ∼10 min After irradiation, and the measurement time was 48,000 s.
Neutron transport analysis of the experiment was carried out with MCNP5 (MCNP Team, 2005). In the analysis, JENDL-Activation Cross Section 96 (JENDL/A-96) (JNDC, 1991) and International Reactor Dosimetry and Fusion File (IRDFF) (Capote et al., 2012) were employed as activation cross section libraries. As for the neutron source term of p-Li reaction, that is, angle dependent neutron spectrum, DROSG-2000 was adopted. And the absolute neutron intensity was taken from the value determined by 7Be in the target described earlier. The calculation model was made to accurately simulate the experimental system in Figure 3, including lithium target, activation foil, styrene form and so on. To estimate activity of the foil, track length estimator, F4, was used in MCNP5, until the uncertainty reach to less than 1%.
Figure 4 shows an example of measured pulse height spectrum for Ag. This is the case for 0° after 20 times irradiation. A 93 keV peak is clearly observed. Other background gamma-rays from radioisotopes created by (n,γ) reaction do not affect the measurement as shown in the figure, because their energies are quite higher than 93 keV. Figure 5 shows the comparison result of 107Ag between experiment and calculation. The y-axis is the number of measured gamma-ray counts. JENDL shown in the figure means the calculated result with JENDL/A-96. The ratio of the Calculated counts to the Experimental counts (C/E) is 0.6 and fairly low, indicating the cross section shows large underestimation. Figure 6 describes the cross section curve of 107Ag inelastic scattering reaction. Similar to the result of Figure 5, JENDL/A-96 shows very small cross section value compared to those of other excited levels of third and fourth level excitations measured by Nishimura (Nishimura et al., 1965). If we use a similar cross section value of Nishimura’s data shown in a red curve in Figure 6, the calculation value shows almost an equal value to the experiment within 1% difference. In any case, it shows that the evaluation was confirmed to be substantially low and should be examined and revised.
Figure 7 shows an example of 0° for In. A 336 keV peak is observed separately, though it is known that gamma-rays from 116In can normally be measured simultaneously. It means that the influence of 116In is acceptably small. In this measurement, the cooling time is not taken into account explicitly, i.e., around 10 min, suggesting 116In would not be a serious problem in real applications. The comparison results of 115In for 0 deg. and 90 deg. are shown in Figures 8, 9, respectively. For 0 deg., the agreement with the result of JENDL was better than that of IRDF-2002 and fitting curve based on experimental value from EXFOR, and was acceptable, that is, prediction of the source intensity can be made in 20% accuracy. For 90-degree direction, as shown in Figure 9, JENDL shows a significantly higher value than the experimental result. Unfortunately, the experimental accuracy is a little low for the following reason: as shown in Figure 1, the neutron energy at 90° in this experiment was theoretically below approximately 400 keV. However, as indicated in Figure 2, the cross section of 115 In at this energy level was very small, resulting in minimal activation of the experimental sample. Consequently, the statistical error in the measurement was relatively large. To address this issue, it would have been necessary to extend the irradiation time or perform repeated irradiations. Figure 10 describes the cross section of JENDL/A-96 together with experimental values cited from EXFOR (Otuka et al., 2014). As expected in Figure 9, JENDL/A-96 shows larger cross sections than the experimental values. Red plots in EXFOR data were measured results obtained by Lövestam (Lövestam et al., 2007), which were the latest experimental values. If we employ the red curve fitted from Lövestam’s data, the agreement is really improved as shown in Figure 9. It is thus suggested that the JENDL/A-96 data should be improved. In the figure the curve of IRDFF is also shown. The IRDFF is a little larger than Lövestam’s data, indicating the IRDFF might be a little too large suggested from the present experiment.
In Osaka University, a new p-Li based ABNS-BNCT (CSePT) project is underway, and various neutronics devices are being developed for characterization of CSePT. In the present paper, a source neutron intensity monitor for the p-Li neutron source was investigated. As a result, the following three foils could be applied for the monitor depending on measuring purposes in the real BNCT scene:
107Ag: Most convenient in a short measuring time.
115In: Highest measuring accuracy.
189Os: Only available for backward angle.
Also from the experimental tests, it was found that the activation reaction cross section data of JENDL-A-96 should be improved for 107Ag and 115In, while IRDFF was acceptable, for real applications of BNCT, because the accuracy of the performance check is quite important for real BNCT machines.
In the next step, we should check isomer production cross sections especially in lower energy region below 1 MeV for 107Ag and 115In. And it is necessary to investigate possibility of 189Os for backward angle measurement. Our BNCT machine (CSePT) will be constructed in near future and will be operated with our developing neutronics devices for clinical tests.
The raw data supporting the conclusions of this article will be made available by the authors, without undue reservation.
ST: Conceptualization, Investigation, Methodology, Validation, Visualization, Writing–original draft, Writing–review and editing. YO: Conceptualization, Investigation, Methodology, Validation, Visualization, Writing–original draft, Writing–review and editing. SK: Investigation, Writing–review and editing. FS: Methodology, Supervision, Validation, Writing–review and editing. IM: Conceptualization, Methodology, Supervision, Validation, Writing–review and editing.
The author(s) declare that no financial support was received for the research, authorship, and/or publication of this article.
We would like to express our deep gratitude to Shigeo Matsuyama and the staff members of the FNL at Tohoku University for their invaluable assistance in conducting the dynamitron accelerator experiments. Chat GPT 4 was used as generative AI technology to editing and revise the manuscript.
The authors declare that the research was conducted in the absence of any commercial or financial relationships that could be construed as a potential conflict of interest.
The author(s) declared that they were an editorial board member of Frontiers, at the time of submission. This had no impact on the peer review process and the final decision.
All claims expressed in this article are solely those of the authors and do not necessarily represent those of their affiliated organizations, or those of the publisher, the editors and the reviewers. Any product that may be evaluated in this article, or claim that may be made by its manufacturer, is not guaranteed or endorsed by the publisher.
Capote, R., Zolotarev, K. I., Pronyaev, V. G., and Trkov, A. (2012). Updating and extending the IRDF-2002 Dosimetry library. J. ASTM Int. 9 (4), 1–9. doi:10.1520/jai104119
Nakajima, Y.JNDC (1991). JENDL activation cross section file. Proc. 1990 Symposium Nucl. Data, JAERI-M 91-032, 43.
Kobayashi, T., Kanda, K., Ujeno, Y., and Ishida, M. R. (1990). Biomedical irradiation system for boron neutron capture therapy at the Kyoto University Reactor. Basic Life Sci. 54, 321–339. doi:10.1007/978-1-4684-5802-2_25
H. Koivunoro, S. Green, I. Auterinen, and M. Kulvik (2015). Proc. The 16th international congress on neutron capture therapy (ICNCT-16) (Elsevier: Applied Radiation and Isotopes), 106, 1–264.
Lövestam, G., Hult, M., Fessler, A., Gamboni, T., Gasparro, J., Geerts, W., et al. (2007). Measurement of neutron excitation functions using wide energy neutron beams. Nucl. Instrum. Methods Phys. Res. Sect. A Accel. Spectrom. Detect. Assoc. Equip. 580 (3), 1400–1409. doi:10.1016/j.nima.2007.06.090
Murata, I., Otani, Y., and Sato, F. (2015). Neutron intensity monitor with activation foil for p-Li neutron source for BNCT - feasibility test of the concept. Appl. Radiat. Isotopes 106, 75–77. doi:10.1016/j.apradiso.2015.07.034
Murata, I. (2015). Research and development of p-liquid Li based boron neutron capture therapy machine, CSePT. ESI-NEWS 33 (6), 1–13. (in Japanese).
Nakamura, T., Horiguchi, H., Kishi, T., Motohashi, J., Sasajima, F., and Kumada, H. (2011). Resumption of JRR-4 and characteristics of neutron beam for BNCT. Appl. Radiat. Isot. 69, 1932–1935. doi:10.1016/j.apradiso.2011.05.015
Nishimura, K., Okano, K., and Kikuchi, S. (1965). Studies of excitation cross sections of (n,n’γ) reactions. Nucl. Phys. 70, 421–448. doi:10.1016/0029-5582(65)90538-9
Otuka, N., Dupont, E., Semkova, V., Pritychenko, B., Blokhin, A. I., Aikawa, M., et al. (2014). Towards a more complete and accurate experimental nuclear reaction data library (EXFOR): international collaboration between nuclear reaction data centres (NRDC). Nucl. Data Sheets 120, 272–276. doi:10.1016/j.nds.2014.07.065
Keywords: boron neutron capture therapy (BNCT), neutron intensity monitor, accelerator based neutron source, p-Li reaction, isomer/isomerization
Citation: Tamaki S, Ohtani Y, Kusaka S, Sato F and Murata I (2024) Experimental test of neutron intensity monitor with isomer production reaction for p-Li neutron source for boron neutron capture therapy. Front. Nucl. Eng. 3:1457737. doi: 10.3389/fnuen.2024.1457737
Received: 01 July 2024; Accepted: 05 November 2024;
Published: 20 November 2024.
Edited by:
Pedro Teles, University of Porto, PortugalReviewed by:
Hee Reyoung Kim, Ulsan National Institute of Science and Technology, Republic of KoreaCopyright © 2024 Tamaki, Ohtani, Kusaka, Sato and Murata. This is an open-access article distributed under the terms of the Creative Commons Attribution License (CC BY). The use, distribution or reproduction in other forums is permitted, provided the original author(s) and the copyright owner(s) are credited and that the original publication in this journal is cited, in accordance with accepted academic practice. No use, distribution or reproduction is permitted which does not comply with these terms.
*Correspondence: Shingo Tamaki, dGFtYWtpQHNlZS5lbmcub3Nha2EtdS5hYy5qcA==
Disclaimer: All claims expressed in this article are solely those of the authors and do not necessarily represent those of their affiliated organizations, or those of the publisher, the editors and the reviewers. Any product that may be evaluated in this article or claim that may be made by its manufacturer is not guaranteed or endorsed by the publisher.
Research integrity at Frontiers
Learn more about the work of our research integrity team to safeguard the quality of each article we publish.