- 1Karlsruhe Institute of Technology (KIT), Institute for Nuclear Waste Disposal (INE), Karlsruhe, Germany
- 2German Cancer Research Center (DKFZ), Service Unit for Radiopharmaceuticals and Preclinical Trials, Im Neuenheimer Feld 280, Heidelberg, Germany
- 3German Cancer Research Center (DKFZ), Research Group Molecular Biology of Systemic Radiotherapy, Heidelberg, Germany
- 4TrisKem International, Bruz, France
Targeted alpha therapy (TαT) represents an emerging and cutting-edge treatment option for patients dealing with highly challenging metastatic cancer diseases. Critically, the limited supply of alpha-particle-emitting radionuclides, so-called alpha in vivo nanogenerators, hampers wider utilization of TαT in clinical settings. This could effectively be circumvented by alternative production routes, including straightforward purification and reformulation strategies. Radionuclide generators offering great potential in simple and robust elution strategies can be provided that still adhere to high radioisotopic, radionuclidic, and radiochemical purity criteria. This study takes a first step towards novel separation strategies by providing additional sources of alpha in vivo nanogenerators for TαT through experiments with various metal surrogates. With different systems, 232Th/natBa was used as a radionuclide generator analogue to 227Th/223Ra, and 232Th/natBa/natLa was used as a triplet analogue to 229Th/225Ra/225Ac. Three selective resins (UTEVA, TEVA, DGA-N) were evaluated for the 232Th/natBa system. Two perturbations of the best-performing resin were further evaluated using a larger diameter column and 1 week of equilibration. For the 232Th/natBa/natLa separation system, a combined column with two selective resins (TK200, TK101) was employed and evaluated. The results thus obtained pave the way for alternative separation strategies in radioactive proof-of-concept validation in the near future.
1 Introduction
Targeted alpha therapy (TαT) is a treatment method that systematically addresses cancer types that include advanced prostate and neuroendocrine malignancies (Kratochwil et al., 2017; Lindén et al., 2021; Yadav et al., 2022). TαT uses alpha-particle-emitting radionuclides (α-emitters), in contrast to targeted radionuclide therapy (TRNT) that commonly applies beta-minus-particle-emitting radionuclides (β–-emitters) (Kim and Brechbiel, 2012). Both TαT and TRNT selectively deliver cytotoxic radiation to cancer cells. However, TαT demonstrates overall higher treatment efficiencies due to the higher linear energy transfer (LET) and smaller tissue penetration observed for α particles (Kratochwil et al., 2018). Within this concept, the most promising α-emitters are represented by actinium-225 (225Ac), thorium-227 (227Th), and radium-223 (223Ra).
The radionuclide 225Ac decays with a half-life of 9.9 days while generating 4α + 2β–emissions with one branching ratio for 213Bi. Currently, 225Ac represents the most promising alpha in vivo nanogenerator for the clinical management of various cancer types (Królicki et al., 2020; Ma et al., 2022; Yadav et al., 2022) and can be produced:
i) via the cyclotron bombardment of 226Ra (Parker et al., 2018; Higashi et al., 2022), e.g.,
ii) via photonuclear reaction on 226Ra within the 225Ra/225Ac generator principle (Maslov et al., 2006; Diamond and Ross, 2021), e.g.,
iii) or in a nuclear reactor within the 229Th/225Ra/225Ac generator principle (Robertson et al., 2018), e.g.,
The radionuclide 227Th decays with a half-life of 18.7 days while generating 5α + 2β–emissions with one branching ratio for 211Bi. 227Th is pre-clinically tested for application in several solid as well as hematologic malignancies (Hagemann et al., 2019; Frantellizzi et al., 2020; Karlsson et al., 2023). 227Th can be produced via a neutron activation of 226Ra within the 227Ra/227Ac/227Th generator principle (Henriksen et al., 2001; Larsen et al., 2007), e.g.,:
The radionuclide 223Ra with a half-life of 11.4 days practically shares the decay chain of its mother radionuclide 227Th. Radium is an alkaline earth metal ion and accumulates in bones through an analogical mechanism such as calcium. Based on this property, 223RaCl2 (Xofigo®, Bayer Healthcare) found its main clinical application in the treatment of bone metastases. 223Ra is usually gained within the 227Ac/227Th/223Ra generator principle (Abou et al., 2017), e.g.,:
Based on limiting challenges of, for example, high costs, regulations, and the scarce availability of these radionuclides, the optimization, research, and broader availability of radionuclide generators are essential for prospective application in cancer therapies (Kim and Brechbiel, 2012; Elgqvist et al., 2014; Parker et al., 2018).
In general, three main principles for radionuclide generator build-up are known: i) sublimation generator, ii) extraction generator, and, most versatile, iii) column generator (Rösch and Knapp, 2011). Furthermore, a rapid and uncomplicated chemical separation should be ensured to enable seamless implementation, such as in hospitals. The desired daughter radionuclide should be eluted with high radioisotopic and radionuclidic purity, crucial for not only medical applications but also for subsequent radiolabeling of various pharmaceuticals.
The working principle of the radionuclide generator is based on a “genetic relationship” between mother and (grand)daughter radionuclide(s) and can be “milked” several times after ingrowth of the (grand)daughter radionuclide(s). In contrast to expensive cyclotron and/or nuclear reactors procedures, the radionuclide generator offers an affordable alternative that is easier to maintain and operate. Currently, the number of generator concepts is limited, however, the demand for α-emitters in the pre-clinical and clinical settings is increasing. Additionally, present political strains are critically impacting 225Ac availability for the wider application of α-emitters in cancer treatment (van Cleve et al., 2019; Causey et al., 2020). Therefore, it is essential to research, evaluate, and produce alternate radionuclides which are suitable for TαT and subsequent (pre-)clinical purposes. In this study, we systematically validated separation strategies for two thorium-based generator concepts using extraction chromatographic resins and corresponding metal surrogates. These surrogates were chosen on chemical similarity; ideally, chemically identical isotopic surrogates are chosen. In this study, this was the case for the surrogate 232Th for 227/229Th. If such surrogates are unavailable, chemically similar elements were chosen. Here, 223/225Ra was surrogated by natBa and 225Ac by natLa, although these are not ideal since there are slight differences in their documented chemical behavior. A fully developed generator system should be tested with isotopic surrogates or the desired radionuclides itself.
2 Materials and methods
Milli-Q water (18.2 MΩ cm; Milli-Q Plus, Merck Millipore, Darmstadt, Germany) and ultra-pure-grade acids (VWR International GmbH, Darmstadt, Germany) were used. A column (Figure 1) made from a 1 mL-syringe (Omnifix B. Braun, Melsungen, Germany) with a filter plate (TrisKem International, Bruz, France) was filled with 0.3 mL resin which approximately corresponds to 100 mg. The resins used in this work were UTEVA, TEVA, DGA-N, TK101, and TK200 (TrisKem International, Bruz, France). The resin was covered by another filter plate and connected to a 5 mL syringe (Omnifix B. Braun, Melsungen, Germany) and an outlet tube via connectors (Altmann Analytik GmbH & Co. KG, Munich Germany).
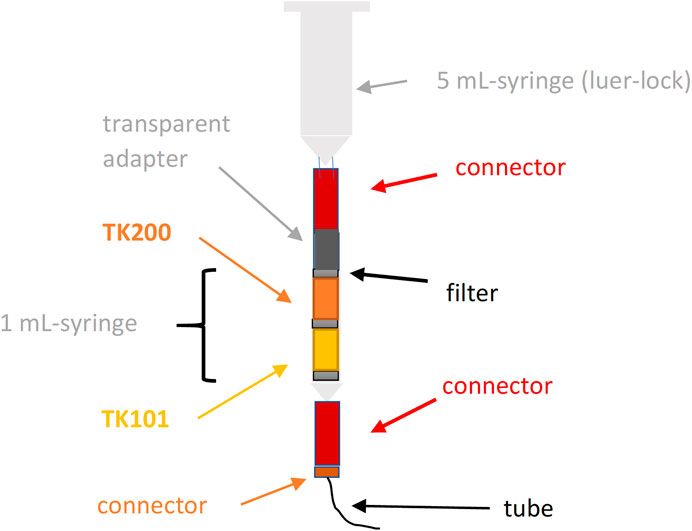
Figure 1. General elution set-up shown for examined generator systems. For the binary generator system, i.e. 227Th/223Ra, only a single resin (UTEVA, TEVA or DGA-N) was used. All columns were pre-conditioned with 3 mL 3 mol/L HNO3; the eluate was collected as ten fractions of 0.3 mL each in a screw-cap vessel (polypropylene (PP), Zinsser Analytic, Fürstenfeldbruck, Germany).
For the 227Th/223Ra system, the columns with a single resin (UTEVA, TEVA, DGA-N) were loaded with 56 µL of 0.001 mg/mL 232Th solution (56 ng Th) and 90 µL of 0.001 mg/mL natBa solution (90 ng Ba), both solutions in 0.5 mol/L HNO3. The liquid was carefully and slowly pushed onto the resin with a 5 mL syringe and allowed to equilibrate over 2.5 h. The collected eluate was labeled “loading fraction”.
Successively for the elution of Ba, 3 mol/L HNO3 was applied and collected in five fractions of 0.5 mL in individual screw cap vessels (PE, Sarstedt Ag & Co. KG, Nümbrecht, Germany). To avoid cross-contamination in the small fractions, 7.5 mL air was flushed through the resin. Finally, the eluent was changed to 0.05 mol/L HCl for Th elution and five fractions of 0.2 mL each were collected before flushing 7.5 mL air through the resin again.
Both a “blind column” and a “spiked blind column” were prepared for each resin set-up. Blind columns were not loaded with corresponding elements, while the washing and elution procedure remained unchanged. This enabled the evaluation of contaminants potentially present in applied resins or solvents. To verify the selected analytical method, the respective amount of evaluated elements (232Th, natBa) was added after elution to each of the “spiked blind column” eluent fractions, serving as a positive control for the sensitivity and quantification of examined elements.
For the 229Th/225Ra/225Ac system, the combined column (TK200/TK101) was washed with ten times its volume (6 mL 0.5 mol/L HNO3). Subsequently, it was loaded with 90 µL 0.001 g/L natBa solution (0.66 nmol Ba) and 56 µL 0.001 g/L 232Th solution (0.24 nmol Th). In addition, 36 µL of 0.01 g/L concentrated natLa solution (2.6 nmol La) was loaded onto the column and allowed to equilibrate over 1.5 h. The first five eluted fractions were chosen to be of 0.2 mL H2O each to evaluate whether La could be eluted without acidic conditions.
In the next step, 0.05 mol/L HNO3 was chosen to enable proper elution of La. To avoid cross-contamination in the small fractions, 7.5 mL air was flushed through the resin. Ba was then eluted with 6 mol/L HNO3 (5 × 0.2 mL fractions), and, in a final step, Th was eluted with 0.1 mol/L HCl in 5 × 0.2 mL fractions. To evaluate potential retarding effects of the TK200 resin on the Ba elution profile, this procedure was first repeated with a column of the combined resins (TK200+TK101) and then with a column containing only 0.3 mL of TK101 resin afterwards.
Before ICP-MS measurements, all wash, loading, and Ba elution fractions were diluted with 4.5 mL deionized water, whereas the Th elution fractions were diluted with 4.8 mL 2% HNO3. The eluents were measured by HR-ICP-MS (ELEMENT XR by Thermo Fisher Scientific, Dreich/Bremen, Germany) and with Q-ICP-MS (Nexion-5000 by Perkin Elmer) while assessing essential limits of detection (LOD) for all three tested elements (Table 1; Supplementary Table S8).
3 Results
3.1 227Th/223Ra separation system surrogated by 232Th/137Ba
The first system investigated the separation of 227Th/223Ra surrogated by 232Th/natBa. Considering a prospective application of the depicted separation processes, we readily implemented the possible impact of nanogram tracer amounts into this proof-of-concept. The results of the 227Th/223Ra separation are shown in Figure 2.
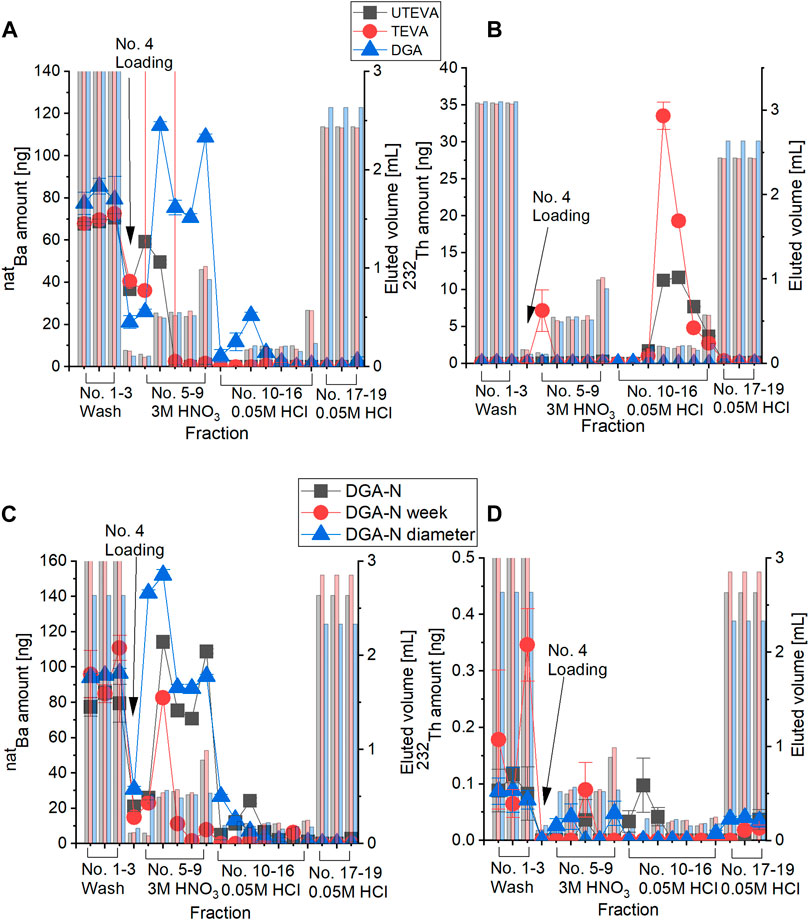
Figure 2. Eluted amount per fraction for Ba (A, C) and Th (B, D) with the following resins: (A, B) UTEVA (black), TEVA (red), and DGA-N (blue). (C, D) Comparison of the unperturbed DGA-N column results with equilibration times of 1 week and at an increased column diameter of 13 mm. Elution volume profiles are shown in mL as bars on the right y-axis. The errors were calculated by error propagation.
It is noteworthy that the wash fraction (No. 1–3) of all resins indicated Ba impurities much higher than the detection limit (Figure 2A), effectively hindering the quantification of non-retained Ba. In spite of these background values, clear differences are apparent in qualitative comparisons between resins such as TEVA and UTEVA, which show only a negligible retention of Ba in contrast to DGA-N. For TEVA and UTEVA, Ba is already washed out with the loading fraction (No. 4) and up to fraction No. 6 the elution is complete, while the DGA-N resin enables the Ba elution to be separated from the loading in fractions No. 6 to No. 12 (Figure 2A).
The elution profile of Th (Figure 2B) clearly shows low cross-contaminations of Th in Ba fractions, which is highly desirable for a system with a Th mother radionuclide. However, overall yields of Th remain low with a DGA-N resin, possibly due to the high affinity of Th to the DGA-N according to known k’ values (TrisKem International, 2015a; TrisKem International, 2015b; TrisKem International, 2015c; TrisKem International, 2023a; TrisKem International, 2023b). Furthermore, experiments at increased acidic concentrations have shown improved Th-retention on UTEVA and TEVA resins. Nevertheless, Ba target fractions should preferentially be eluted in diluted acids to ensure more efficient radiolabeling of prospective pharmaceuticals.
The DGA-N resin showed ideal separation results through high Th-retention and favorable Ba-elution behavior in the presence of less concentrated acids (Roesch, 2012; International Atomic Energy Agency, 2016). Based on these results, the DGA-N resin was chosen for further tests under variable conditions.
Several Th/Ra separation approaches have been reported, ranging from extraction-based approaches (Shishkin et al., 2011; Havelka, 2016) to methods using ion exchange resin like Dowex-1/Dowex-50 (Mokhodoeva et al., 2015; Abou et al., 2017) or Dipex-2 and AG50W-X12 for the separation of 223Ra (Henriksen et al., 2001). However, Henriksen et al. (2001) used 223Ra-stripping concentrations of 8 mol/L HNO3 which, compared to 3 mol/L HNO3 applied in this study, are unfavorable for the subsequent radiolabeling within the intended radiopharmaceutical approach. In comparison, Mastren et al. (2017) have reported on successful Ra isotope separation on a larger scale with radiochemical purities of 99.99%—very similar to the resins tested in this study.
Moreover, we chose to investigate two crucial parameters in the DGA-N experiments. Firstly, larger column diameters can result in less interaction between stationary and mobile phases, which can result in different resolution profiles of the evaluated elements. To examine this, we chose to increase the column diameter from 5 mm to 13 mm while retaining the volume of the applied resin. Secondly, equilibration times on the column can affect the durability, potential degradation, or other reactions of applied resins and nuclides. Therefore, we chose to elute a week after loading the column.
While the diameter variation revealed no difference to the elution profiles (Figures 2C, D), the equilibration time seems to impact the retrievability of Ba. Considering the background of Ba in the resin, Figure 2C shows similar amounts of eluted Ba in the wash fractions (No. 1–3). In contrast, the elution of Ba from the one-week equilibrated column was generally lower and ended five fractions earlier than in the directly eluted columns. This is clearly disadvantageous when considering that this component is applied in generator set-ups with contact times of up to a whole year. To counteract this lower retrievability, tests were performed in which the eluent was heated to 60 °C (Supplementary Figure S1; Supplementary Tables S1, S2). In general, this resulted in a higher retrievability of Ba but also in higher backgrounds in the wash fraction, making a quantitative comparability impossible. The implementation of radioisotope 133Ba could potentially circumvent this problem, as it should be possible to determine the elution profile via gamma spectroscopy.
Very strong adhesion of Th was found for all columns, with detected values being close to the ICP-MS detection limit. In general, repeated experiments verified trends and profiles (Supplementary Figure S2; Supplementary Tables S3, S4), albeit with different Ba backgrounds. As indicated by our results, the elution of Ba is strongly sensitive to temperature. It is possible that these results might have been affected by fluctuating laboratory temperatures or different ICP-MS devices.
3.2 229Th/225Ra/225Ac separation system surrogated by 232Th/137Ba/139La
The second system investigated the separation of 229Th/225Ra/225Ac surrogated by 232Th/natBa/natLa. We compared the combination of TK200 and TK101 resins to the elution profile of sole TK200 resin retaining only Th.
In this system, the elution profile of La (Figure 3A) indicates an efficient La retention on the column and no elution with the loading fraction. Additionally, we evaluated the necessity of acidic elution conditions by applying deionized water on the combined TK200/TK101 set-up. The water-eluted amount of La corresponds to 18% of the initially loaded La onto the column. In contrast, 57% ± 3% of the initially loaded La was successfully eluted from the TK200 column using 0.05 mol/L HNO3. Comparing the profiles of the TK200 (Figure 3B) and TK200/TK101 columns (Figure 3A), it is evident that an elution using water is ideally possible when only TK200 is applied. Thus, it can be hypothesized that La was partially retained on TK101 during the elution with deionized water, which is in congruence with KD values of La on the TK101 resin. The KD values are about 3 mL/g at 10−2 mol/L HNO3 and decrease for 0.1 mol/L HNO3 (TrisKem International, 2023b).
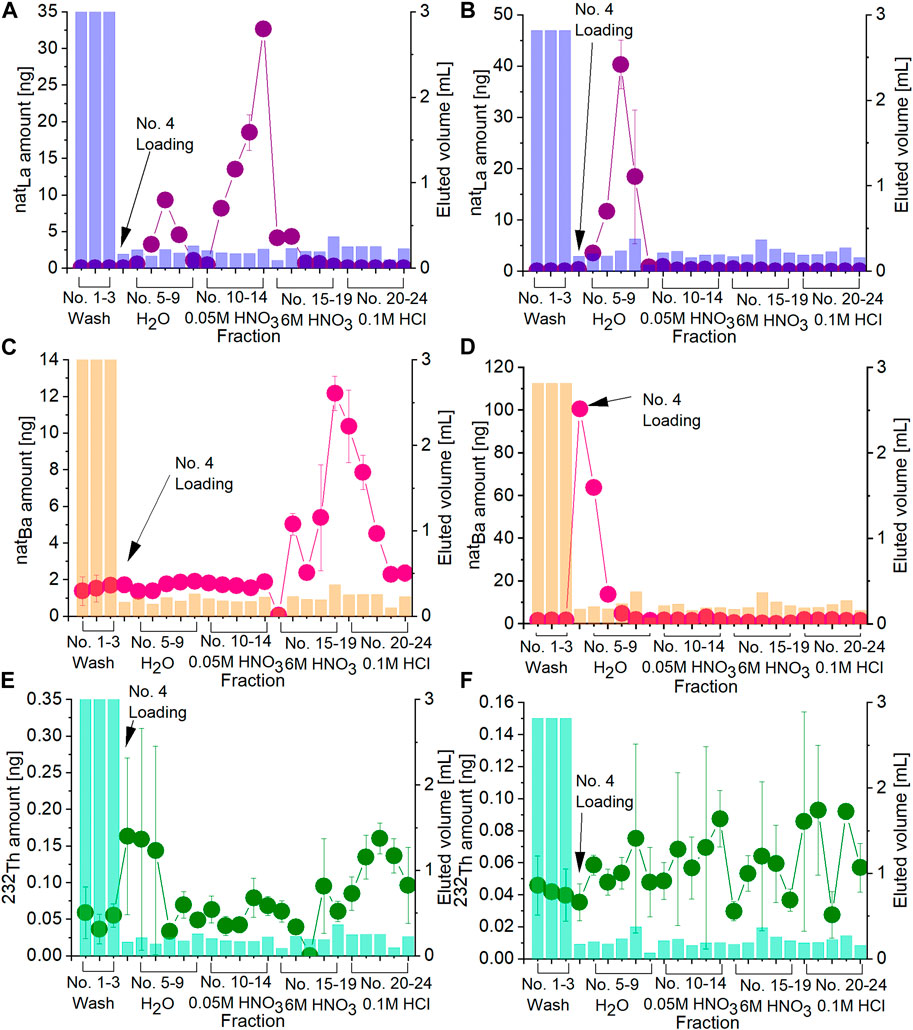
Figure 3. Eluted amount per fraction for La (A, B), Ba (C, D), and Th (E, F). (A, C, E) show separation using the combination of two resins (TK200+TK101) while (B, D, F) show the separation using only the TK200 resin. Additionally, elution volume profiles are shown in mL as bars on the right y-axis. The errors were calculated by error propagation.
Figure 3C demonstrates the high efficiency of combined resins in retaining the majority of applied Ba. However, the elution of Ba in 6 mol/L HNO3 continued into the Th target fractions and resulted in cross-contamination. Applying a larger volume of 6 mol/L HNO3 could potentially deter this problem but might lead to potential difficulties in subsequent radiolabeling procedures. In comparison to Ba, Ra is less strongly bound to TK101 at 6 mol/L HNO3 (TrisKem International, 2023a), and the feasibility of both Ra extraction and elution from environmental samples was proven with TK100, which is a direct derivative of the crown-ether-based TK101 resin (van Es et al., 2017). The elution profiles with TK200 (Figure 3D) conclude that Ba is present in all loading fractions, confirming that TOPO ligands in the applied resin offer subpar Ba-complexation properties (Ghose et al., 1975).
On a final note, Th was successfully retained on combined (TK200/TK101; Figure 3E) and single (TK200; Figure 3F) resin columns with a low recovery of only 2.9%, similar to Th on the DGA-N resin, which is congruent with conditions applied in practical radionuclide generator systems. During generator operation, Th, as the mother nuclide, should be retained and only washed from the column to protect the resin from undesired radiolytic effects in case a reloading strategy would prove feasible. These results of the previous elution profiles of the repeated experiments are nearly identical; however the total recovery of Ba is again lower.
In this study, the quantities of examined surrogates for potential generator settings were chosen in accordance with established ones to allow for comparisons with real radionuclide generator systems. For instance, 100 MBq of 229Th and 227Th respectively correspond to 13.7 mg and 87.9 ng, and 100 MBq of 225Ra and 223Ra to 69.4 ng and 52.6 ng.
A similar approach of combining two separate resins was performed by Lin et al. (2023). They chose different resins to combine, but their elution profiles strongly resemble ours. Our study thus reinforces the application of sequentially combined resins in a single column for multi-radionuclide separation purposes.
3.3 Purity and retrievable yields
For TαT purposes, the purity of the applied radionuclide is the decisive factor, and a specific purity limit must be ensured prior to application. As established in various radionuclide generators, subsequent purification steps are often attached to the radionuclide production procedure itself (Guseva and Dogadkin, 2010).
Since the 232Th/137Ba separation system is a surrogate for 227Th/223Ra, 223Ra represents the desired radionuclide. Consequently, the fraction with high radionuclidic purity is of utmost importance for the Ba fraction (cf. Table 2). While UTEVA can provide high nuclidic purities of 99.7% ± 0.1%, higher purities of 99.99% ± 0.01% are possible when using DGA-N. In the case of TEVA, a breakthrough of Th reduced the overall purities to 91% ± 6%. For all applied resins, retrievability remained impossible to quantify as Ba impurities remained present before loading (Figure 2A). Despite being a potential TαT nuclide (Frantellizzi et al., 2020; Karlsson et al., 2023), 227Th showed generally low retrievability, especially on DGA-N resin, which would prevent a dual use of the generator.
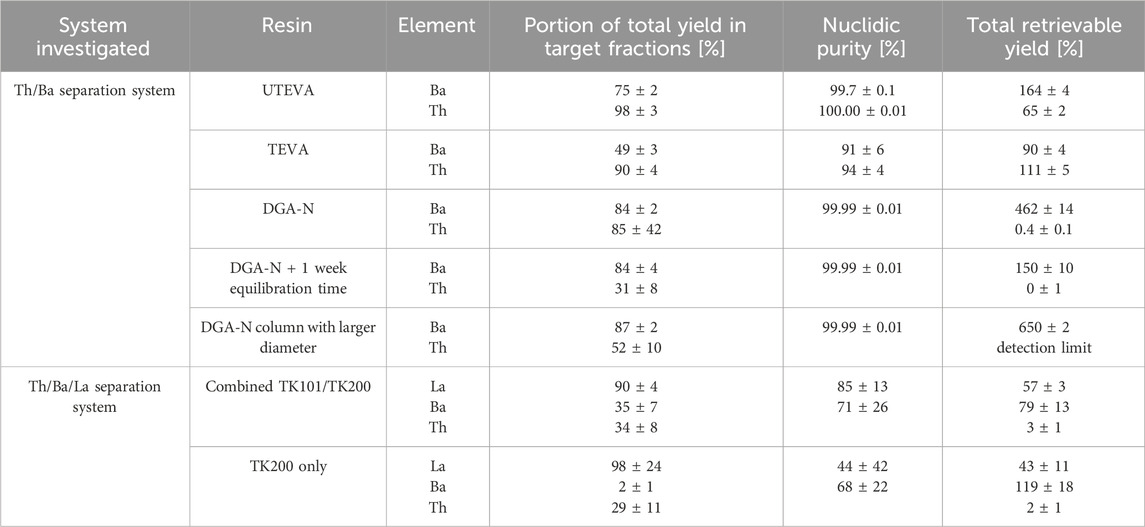
Table 2. Amounts of eluted nuclide in respective target fractions (defined in Figures 2 and 3 of the specific nuclide). Additionally, nuclidic purity is listed as the ratio, expressed as an atom percentage, of the desired nuclide amount to the total amount of nuclides in the target fraction. The retrievability in the target fractions is given as the percentage of the spike added. Due to the Ba impurities detected in all resins, the values exceed 100%.
In the system 229Th/225Ra/225Ac, 225Ac is the desired radionuclide to separate for application in TαT. Therefore, it is preferable to obtain the La fraction in high nuclidic purity from the 232Th/natBa/natLa separation system. Our data indicate reasonably efficient separation strategies, with La purities of 85% ± 13% observed. Nevertheless, future research should focus on delivering not only higher nuclidic purities but also increased retrievability. In this study, only 57% ± 3% of the introduced La were recovered successfully, which is a range of 57%–71% compared to other proposed 223Ra-generator concepts (Henriksen et al., 2001; Abou et al., 2017).
4 Discussion and conclusion
This study acquired preliminary data towards separation strategies of TαT-suitable radionuclides from experimental series using cold metal surrogates.
In our study, two separation systems were set-up and analyzed: i) the binary 232Th/137Ba system representing the generator pair 227Th/223Ra and ii) the tertiary 232Th/137Ba/139La system representing the generator triplet 229Th/225Ra/225Ac.
In the Th/Ba separation system, the performances of TEVA, UTEVA, and DGA-N resins were thoroughly studied using analytes in nanogram amounts. All tested analyte contents were observed to be well below the capacities of evaluated extraction chromatographic resins for the examined elements, which is in the order of 15–50 mg/g of resin. The best-performing resin (DGA-N) was further evaluated under varied parameters at both increased column diameters (13 mm), heated eluents, and equilibration time (1 week). For the Th/Ba/La separation system, a column with a successive resin combination (TK200/TK101) and a sole resin (TK200) was evaluated and compared.
The DGA-N resin was found to be the most effective in separating Th from Ba. In the case of UTEVA, the lowest percentage of Ba and Th retrieval in the target fractions were obtained. Similarly, elution yields of Th decreased to 0.4%, possibly due to high affinities of Th to DGA-N. In prolonged equilibration times of 1 week, only 0.8% of the Th could be recovered. Essentially, the nanogram amounts of Th were strongly retained in all systems. While overall elution yields remained low, the strong retention of Th on the examined resin(s) can prospectively be of advantage in (227Th/223Ra) separation systems in which Th is utilized as a mother radionuclide.
In the Th/Ba/La system using a combination of TK101/TK200 resins, La was separated sufficiently and was almost exclusively collected in the target fractions. Ba was successfully retained by TK101 and only eluted with 6 mol/L HNO3 at the onset of the target fractions. Nevertheless, Ba contaminations leaked into subsequent fractions, which could potentially be counteracted by a larger volume of the eluent. As the Dw values of Ra on the resin are lower compared to Ba, this would most probably not be relevant to the same extend for the real generator system. As with the DGA-N resin, Th was strongly retained on TK200 and could hardly be eluted with the amounts used.
These results raise new questions that call for follow-up research in the near future. To study different factors, further resins could be implemented and tested at varied parameters analogous to our studies—for example, TK200 in Th/Ba separation systems. In addition, eluents and acids of different kinds and concentrations could be applied. Potential complexing agents might be tested, especially given the low retrievability of Th found by this research.
To further understand and develop additional separation systems, more surrogates, deriving from daughter radionuclides of 229Th and 227Th two-decay series, should be implemented in experiments. Finally, if practical approaches are desired, the surrogates should be replaced with the desired radionuclides to facilitate a transfer in research and medical application. To conclude, this research sheds light on radionuclide generator development for TαT and demonstrates that many existing and new questions remain to be investigated.
Data availability statement
The original contributions presented in the study are included in the article/Supplementary Material; further inquiries can be directed to the corresponding author.
Author contributions
BS: conceptualization, formal analysis, methodology, project administration, resources, supervision, validation, visualization, writing–original draft, writing–review and editing. KM: data curation, formal analysis, investigation, validation, visualization, writing–review and editing. MS: investigation, methodology, writing–review and editing. YR: investigation, methodology, writing–review and editing. FG: data curation, methodology, writing–review and editing. AF: data curation, methodology, writing–review and editing. SH: conceptualization, writing–review and editing. MB-S: conceptualization, methodology, supervision, validation, writing–review and editing.
Funding
The author(s) declare that financial support was received for the research, authorship, and/or publication of this article. It was funded by the Federal Ministry of Education and Research (BMBF) and the Baden-Württemberg Ministry of Science as part of the Excellence Strategy of the German Federal and State Governments. The authors acknowledge funding from the European Research Council (ERC) Consolidator Grant 2020 under the European Union’s Horizon 2020 research and innovation program (grant agreement no. 101003292). Co-funding of this project was made available through the joint strategic alliance between the German Cancer Research Center (DKFZ) and Bayer AG. The authors declare that this study received funding from Bayer AG. The funder was not involved in the study design, collection, analysis, interpretation of data, the writing of this article, or the decision to submit it for publication.
Acknowledgments
The authors sincerely acknowledge the support of Harun Taş from the German Cancer Research Center (DKFZ) in Heidelberg for his valuable and profound comments as well as thorough adjustment of the manuscript’s format, structure, and style.
Conflict of interest
Author SH is employed by TrisKem International.
The remaining authors declare that the research was conducted in the absence of any commercial or financial relationships that could be construed as a potential conflict of interest.
Publisher’s note
All claims expressed in this article are solely those of the authors and do not necessarily represent those of their affiliated organizations, or those of the publisher, the editors and the reviewers. Any product that may be evaluated in this article, or claim that may be made by its manufacturer, is not guaranteed or endorsed by the publisher.
Supplementary material
The Supplementary Material for this article can be found online at: https://www.frontiersin.org/articles/10.3389/fnuen.2024.1379996/full#supplementary-material
References
Abou, D. S., Pickett, J., Mattson, J. E., and Thorek, D. L. (2017). A radium-223 microgenerator from cyclotron-produced trace actinium-227. Appl. Radiat. Isotopes 119 (1), 36–42. Available at:. doi:10.1016/j.apradiso.2016.10.015
Causey, P., Perron, R., and Gendron, D. (2020). Production of actinium-225 at the Canadian Nuclear Laboratories: operation of a thorium generator and quality control of Ac-225. J. Nucl. Med. 61 (Suppl. 1), 467.
Diamond, W. T., and Ross, C. K. (2021). Actinium-225 production with an electron accelerator. J. Appl. Phys. 129 (10). Available at:. doi:10.1063/5.0043509
Elgqvist, J., Frost, S., Pouget, J. P., and Albertsson, P. (2014). The potential and hurdles of targeted alpha therapy – clinical trials and beyond. Front. Oncol. 3, 324. Available at:. doi:10.3389/fonc.2013.00324
Frantellizzi, V., Cosma, L., Brunotti, G., Pani, A., Spanu, A., Nuvoli, S., et al. (2020). Targeted alpha therapy with thorium-227. Cancer Biotherapy Radiopharm. 35 (6), 437–445. Available at:. doi:10.1089/cbr.2019.3105
Ghose, A. K., Šebesta, F., and Starý, J. (1975). Synergistic extraction of radium and barium using 1-phenyl-3-methyl-4-benzoylpyrazol-5-one and trioctylphosphine oxide. J. Radioanalytical Chem. 24 (2), 345–351. Available at:. doi:10.1007/bf02518432
Guseva, L. I., and Dogadkin, N. N. (2010). A generator system for production of medical alpha-radionuclides Ac-225 and Bi-213. J. Radioanalytical Nucl. Chem. 285 (3), 667–673. Available at:. doi:10.1007/s10967-010-0593-6
Hagemann, U. B., Ellingsen, C., Schuhmacher, J., Kristian, A., Mobergslien, A., Cruciani, V., et al. (2019). Mesothelin-targeted thorium-227 conjugate (MSLN-TTC): Preclinical evaluation of a new targeted alpha therapy for mesothelin-positive cancers. Clin. Cancer Res. 25 (15), 4723–4734. doi:10.1158/1078-0432.ccr-18-3476
Havelka, M. (2016). Preparation of (228)Ra standard solution. Appl. Radiat. isotopes Incl. data, Instrum. methods use Agric. industry Med. 109, 222–225. Available at:. doi:10.1016/J.APRADISO.2015.11.062
Henriksen, G., Hoff, P., Alstad, J., and Larsen, R. H. (2001). 223Ra for endoradiotherapeutic applications prepared from an immobilized 227Ac/227Th source. Radiochim. Acta 89 (37165), 661–666. Available at:. doi:10.1524/ract.2001.89.10.661
Higashi, T., Nagatsu, K., Tsuji, A. B., and Zhang, M. R. (2022). Research and development for cyclotron production of 225Ac from 226Ra—the challenges in a country lacking natural resources for medical applications. Processes 10 (6), 1215. Available at:. doi:10.3390/pr10061215
International Atomic Energy Agency, (IAEA) (2016). Human health campus - decay properties for generators of short-lived radionuclides. Available at: https://humanhealth.iaea.org/HHW/Radiopharmacy/VirRad/Eluting_the_Generator/Generator_Module/Design_principles/Non_99mTc_generators/table.html (Accessed November 25, 2023).
Karlsson, J., Schatz, C. A., Wengner, A. M., Hammer, S., Scholz, A., Cuthbertson, A., et al. (2023). Targeted thorium-227 conjugates as treatment options in oncology. Front. Med. 9 (January), 1071086–1071089. Available at:. doi:10.3389/fmed.2022.1071086
Kim, Y. S., and Brechbiel, M. W. (2012). An overview of targeted alpha therapy. Tumor Biol. 33 (3), 573–590. Available at:. doi:10.1007/s13277-011-0286-y
Kratochwil, C., Bruchertseifer, F., Rathke, H., Bronzel, M., Apostolidis, C., Weichert, W., et al. (2017). Targeted α-therapy of metastatic castration-resistant prostate cancer with 225 Ac-PSMA-617: dosimetry estimate and empiric dose finding. J. Nucl. Med. 58 (10), 1624–1631. Available at:. doi:10.2967/jnumed.117.191395
Kratochwil, C., Bruchertseifer, F., Rathke, H., Hohenfellner, M., Giesel, F. L., Haberkorn, U., et al. (2018). Targeted a-therapy of metastatic castration-resistant prostate cancer with 225 Ac-PSMA-617: Swimmer-plot analysis suggests efficacy regarding duration of tumor control. J. Nucl. Med. 59 (5), 795–802. Available at:. doi:10.2967/jnumed.117.203539
Królicki, L., Kunikowska, J., Bruchertseifer, F., Koziara, H., Królicki, B., Jakuciński, M., et al. (2020). 225Ac- and 213Bi-substance P analogues for glioma therapy. Seminars Nucl. Med. 50 (2), 141–151. Available at:. doi:10.1053/j.semnuclmed.2019.11.004
Larsen, R. H., Borrebaek, J., Dahle, J., Melhus, K. B., Krogh, C., Valan, M. H., et al. (2007). Preparation of Th 227 -labeled radioimmunoconjugates, assessment of serum stability and antigen binding ability. Cancer Biotherapy Radiopharm. 22 (3), 431–437. Available at:. doi:10.1089/cbr.2006.321
Lin, Y., Wang, J., Shao, K., Zhang, G., Wang, X., Liu, T., et al. (2023). Two-step separation of Th, La and Ba using combined chromatographic columns. J. Radioanalytical Nucl. Chem. 332 (4), 1245–1252. Available at:. doi:10.1007/s10967-023-08761-3
Lindén, O., Bates, A. T., Cunningham, D., Hindorf, C., Larsson, E., Cleton, A., et al. (2021). 227Th-Labeled anti-CD22 antibody (bay 1862864) in relapsed/refractory CD22-positive non-hodgkin lymphoma: a first-in-human, phase i study. Cancer Biotherapy Radiopharm. 36 (8), 672–681. Available at:. doi:10.1089/cbr.2020.4653
Ma, J., Li, L., Liao, T., Gong, W., and Zhang, C. (2022). Efficacy and safety of 225Ac-PSMA-617-Targeted alpha therapy in metastatic castration-resistant prostate cancer: a systematic review and meta-analysis. Front. Oncol. 12 (February), 796657. Available at:. doi:10.3389/fonc.2022.796657
Maslov, O. D., Sabel’nikov, A. V., and Dmitriev, S. N. (2006). Preparation of 225Ac by 226Ra(γ, n) photonuclear reaction on an electron accelerator, MT-25 microtron. Radiochemistry 48 (2), 195–197. doi:10.1134/S1066362206020184
Mastren, T., Radchenko, V., Owens, A., Copping, R., Boll, R., Griswold, J. R., et al. (2017) ‘Simultaneous separation of actinium and radium isotopes from a proton irradiated thorium matrix’, Sci. Rep. 7:1, pp. 8216–8217. doi:10.1038/s41598-017-08506-9
Mokhodoeva, O., Guseva, L., and Dogadkin, N. (2015). Isolation of generator-produced 223Ra in 0.9-% NaCl solutions containing EDTA for direct radiotherapeutic studies. J. Radioanalytical Nucl. Chem. 304 (1), 449–453. Available at:. doi:10.1007/s10967-014-3777-7
Parker, C., Lewington, V., Shore, N., Kratochwil, C., Levy, M., Lindén, O., et al. (2018). Targeted alpha therapy, an emerging class of cancer agents: a review. JAMA Oncol. 4 (12), 1765–1772. Available at:. doi:10.1001/jamaoncol.2018.4044
Robertson, A. K. H., Ramogida, C. F., Schaffer, P., and Radchenko, V. (2018). Development of 225Ac radiopharmaceuticals: TRIUMF perspectives and experiences. Curr. Radiopharm. 11 (3), 156–172. Available at:. doi:10.2174/1874471011666180416161908
Roesch, F. (2012). Maturation of a key resource – the germanium-68/gallium-68 generator: development and new insights. Curr. Radiopharm. 5 (3), 202–211. Available at:. doi:10.2174/1874471011205030202
Rösch, F., and Knapp, F. F. F. (2011). “Radionuclide generators,” in Handbook of nuclear chemistry. Editor A. Vertes (Boston, MA: Springer US), 1935–1976. Available at:. doi:10.1007/978-1-4419-0720-2_40
Shishkin, D. N., Krupitskii, S. V., and Kuznetsov, S. A. (2011). Extraction generator of 223Ra for nuclear medicine. Radiochemistry 53 (4), 404–406. Available at:. doi:10.1134/S1066362211040126
TrisKem International (2023a). Product sheet TK100/TK101 resins. Available at: www.triskem.com.
van Cleve, S., Boll, R., Benny, P., Dyke, T., Kehn, J., and Phillips, K. (2019). Thorium-229 generator production of actinium-225 at Oak Ridge National laboratory. J. Med. Imaging Radiat. Sci. 50 (1), S11–S12. Available at:. doi:10.1016/j.jmir.2019.03.037
van Es, E. M., Russell, B. C., Ivanov, P., García Miranda, M., Read, D., Dirks, C., et al. (2017). The behaviour of 226Ra in high-volume environmental water samples on TK100 resin. J. Radioanalytical Nucl. Chem. 312 (1), 105–110. Available at:. doi:10.1007/s10967-017-5203-4
Keywords: targeted alpha therapy, alpha in vivo nanogenerators, thorium, radium, radionuclide generators, radionuclide separation
Citation: Schacherl B, Maurer K, Schäfer M, Remde Y, Geyer F, Fried A, Happel SA and Benešová-Schäfer M (2024) Concept validation of separations for thorium-based radionuclide generator systems for medical application. Front. Nucl. Eng. 3:1379996. doi: 10.3389/fnuen.2024.1379996
Received: 31 January 2024; Accepted: 20 June 2024;
Published: 08 August 2024.
Edited by:
Anne Campbell, Oak Ridge National Laboratory (DOE), United StatesReviewed by:
Jean-Emmanuel Groetz, University of Franche-Comté, FranceFrankie White, Oak Ridge National Laboratory (DOE), United States
Copyright © 2024 Schacherl, Maurer, Schäfer, Remde, Geyer, Fried, Happel and Benešová-Schäfer. This is an open-access article distributed under the terms of the Creative Commons Attribution License (CC BY). The use, distribution or reproduction in other forums is permitted, provided the original author(s) and the copyright owner(s) are credited and that the original publication in this journal is cited, in accordance with accepted academic practice. No use, distribution or reproduction is permitted which does not comply with these terms.
*Correspondence: Bianca Schacherl, Bianca.schacherl@kit.edu; Martina Benešová-Schäfer, m.benesova@dkfz.de
†Present address: Chemical Sciences Division (CSD), Lawrence Berkeley National Laboratory (LBNL), Berkeley, CA, United States