- Science and Technology on Reactor System Design Technology Laboratory, Nuclear Power Institute of China, Chengdu, China
As one of the forth-generation nuclear energy system reactor types, lead fast reactor has good safety and economical properties due to the stable chemical properties of the coolant and the proliferation characteristics of the fuel, and modular nuclear power faster reactor designed for nuclear plant can further improve the economics of the reactor. In this article, the conceptual design of the lead-based modular power reactors with different power levels loaded with uranium alloy fuel is found to be found that when reactor core size increased to a certain level, the proliferation performance is too high due to the increase of the reactor core size under a specific core life such as 2000EFPD, so at the end of core life, the reactor core still has a large remaining reactivity. The proliferation advantage of the core cannot be fully released during the current core life time. Based on this phenomenon, in this article, we optimized the conceptual design of lead-based modular nuclear power reactor core loaded with uranium alloy fuel, and proposed to choose the appropriate rod to diameter ratio and effective density of fuel based on the power level and life time of the core. By adjusting the amount of uranium and 235U per unit volume, the proliferation performance of the core can be changed to match the power level and life time of the core. So the reactivity of core during the life period does not change, which not only reduce the difficulty of the reactivity control, but also make full use of the proliferation performance of the core. And at the same time, the reasonable rod to diameter ratio can provide safety and design margin for the analysis of thermal and hydraulic safety, and effectively improve the economy and safety of the core.
1 Introduction
Lead-based fast reactors (Zrodnikov et al., 2001; Zrodnikov et al., 2008; Zrodnikov et al., 2009), as one of the fourth-generation nuclear energy systems, mainly benefit from the chemical stability of lead-based coolants and the breeding performance of fast reactor fuels. Compared with active metals such as sodium, lead-based coolants hardly react with water and air, so they are safer than sodium-cooled fast reactors. The core energy spectrum of lead-based fast reactors is harder than that of the common thermal spectrum reactors such as Pressure Water Reactor (PWR), and the share of fast neutrons is high, which can convert fissionable nuclides such as 238U in the fuel into fissile nuclides such as 239Pu, etc., which can effectively improve the utilization rate of uranium resources. Therefore, it is more economical than the thermal spectrum core.
Modular nuclear power design is to use a certain number of single reactors with a specific power level to be combined to meet the power level requirements of nuclear power plants. The use of modular design can make the core design easier and make the power combination of nuclear power plants more flexible. The improved design also reduces design and production costs, further improving core economics.
U-10Zr alloy fuel and UO2 fuel are both commonly used fuel types in reactor design. Because U-10Zr alloy fuel does not contain oxygen atoms, it has weaker moderating effect on neutrons, and the core energy spectrum is relatively stiffer, which is more conducive to the proliferation effect of fuels in fast reactors. Meanwhile, U-10Zr alloy is more suitable for the dry reprocessing process of closed fuel in fast reactors. It can realize fuel recycling and improve the economy of core fuel. Therefore, the core design will be based on U-10Zr alloy fuel in this paper.
In this paper, uranium-zirconium alloy fuel is used to carry out the conceptual design of the modular nuclear power reactor core, and the basic characteristics of the core under different power levels are analyzed. For example, the end-of-life reactivity of 2000EFPD cannot be fully released, and the reactivity and breeding characteristics of the core cannot be effectively released and utilized in the current core power and lifetime due to excessive breeding characteristics. Based on this, this paper puts forward the optimization ideas and directions. The optimal design of the core with different power levels finally makes the change of the core reactivity basically match the core power and life, and effectively improves the safety and economy of the core.
2 Calculation program
The calculation in this paper is completed by RMC program. Reactor Monte Carlo code (RMC) is a three-dimensional transport Monte Carlo code independently developed by the Reactor Engineering Computational Analysis Laboratory (REAL Team), Institute of Nuclear Energy Science and Engineering Management, Department of Engineering Physics, Tsinghua University, for reactor core calculation and analysis. The RMC program is developed for the basic requirements of reactor calculation and analysis, and combined with the characteristics of flexible geometry, complex neutron energy spectrum, diverse material components, anisotropy and strong leakage in the design of the new concept reactor system. It can handle complex geometry and describe complex energy spectrum and materials by using continuous energy point cross sections. The eigenvalue of critical problem, eigenfunction calculation, system burn-up simulation and transient process analysis can be calculated according to the needs of practical problems. The continuous energy point cross section database in ACE format of CENDL1.0 is used for neutron transport calculation. The RMC program adopts a variety of parallel algorithms to improve computing efficiency, and currently has the function of kilo-core parallel computation on large servers. Functions such as parallel computing enable Monte Carlo methods to be used in nuclear design calculations of reactor cores.
3 Preliminary conceptual reactor design
3.1 Assembly design
The type of assemblies used in the core refer to the assembly design of the lead-based research reactor SLBR-50 (Small Lead-Based Reactor - 50 MWt) (Zhao et al., 2021) of Nuclear Power Institute of China (NPIC). The schematic diagram of the assemblies is shown in Figure 1. The main design parameters are shown in Table 1.
3.2 Core design
According to the above assembly structure design, U-10Zr fuel core is used for core design of different power levels, and the core life time meets 2000EFPD (Zrodnikov et al., 2009), main design parameters of each scheme is shown in Table 2.
Schematic diagrams of radial and axial arrangements of the five core schemes with different power levels are shown in Figures 2–6. The design parameters of each scheme of the core are shown in Table 3.
From the comparison of the parameters of each core scheme in Table 3 and Figure 7, it can be seen that the core design is carried out on the premise that the power density of the cores is not much different. When the core power level is 100 MWt and 300 MW, the core keff curve increases with the decrease of lifetime monotonically, but the decreasing rate of the keff curve of the 300 MWt core is smaller than that of the 100 MWt core. When the core power is 500 MW, the keff curve rises and then decreases in a very small range during the core life. Due to the extremely small amplitude, it can be considered that there is almost no change in keff during the life of the core. When the core power is 700 MWt and 1,000 MWt, the keff curve in the core life has an upward trend with the burnup, and the keff at the end of the life is larger than the beginning of the life. If the burnup is further deepened, the keff will show a downward trend to the end of the life. The core life has far exceeded the required 2000EFPD, which may not meet the core refueling cycle and fuel burnup limit requirements.
For the above-mentioned core schemes, the breeding performance of the 500 MWt core has a good match with the core power and life. The breeding characteristics of the core during the 2000EFPD life are effectively utilized, and the reactivity fluctuation during the core life is very small. It is beneficial to the control of the core reactivity, and the core economy and safety are relatively high. The breeding characteristics of the 100 MWt and 300 MWt core schemes are relatively weak, while the breeding characteristics of the 700 MWt and 1000 MWt cores are relatively strong, both of which are not conducive to the improvement of fuel utilization and the control of core reactivity. Therefore, it is necessary to optimize the design of the cores with different power levels, and the core schemes of other power levels can also achieve the reactivity variation characteristics of the 500 MWt core by changing the core parameters.
4 Optimization route analysis
From the calculation and analysis results of the above schemes, it can be seen that when the uranium-zirconium alloy fuel is designed with different powers under the given assembly structure, power density level and core life, and the core power level is proportional to the core size, and the breeding performance of the core is also proportional to the core power level and core size. At low power level, the core size is relatively small, the core breeding performance is weak, and the core reactivity curve shows a monotonically decreasing trend with burnup. And at high power level, the core size is larger and the core breeding performance is stronger, the core reactivity curve first increases and then decreases with burnup. When the core power level matches a given assembly structure, power density level and core lifetime, the core reactivity slightly increases firstly and then decreases throughout the lifetime. Due to the small fluctuation, it can be considered that the core reactivity hardly changes throughout the core life.
According to the above analysis, if it is necessary to design lead-based modular nuclear power reactor cores with different power levels in the project, it is necessary to design the assembly structure according to the core power level and lifetime, and at the same time, it is necessary to limit the volume power density level to meet the requirements of thermal safety analysis. This paper only qualitatively analyzes the power density level, focusing on the influence of the assembly structure on the physical performance of the core.
In addition to the size of the core, the breeding performance of the uranium-zirconium alloy fuel core is mainly affected by the uranium loading per unit volume. Therefore, the effective density of the uranium-zirconium alloy fuel core and rod to diameter ratio in the assembly can be changed as required. Two factors change the amount of uranium per unit volume.
Since the uranium-zirconium alloy fuel core needs to consider its radiation swelling effect, the effective density of 75% is generally used in the engineering design, that is, 15.92 g/cm3×0.75 = 11.94 g/cm3 above. The 75% effective density is achieved by reserving a hole in the central area of the fuel core to contain the fissionable gas and to buffer the increase in the volume of the fuel core due to swelling of the fuel core. When the porosity reaches 25%, the effective density of the fuel core is 75%. The pore size in the center of the fuel core can be further increased according to the need to further reduce the effective density of the fuel core.
The rod to diameter ratio is the ratio of the center-to-center distance between the two fuel rods in the assembly to the fuel rod diameter. In the above analysis, the fuel rod center-to-center distance is 1.09 cm, and the fuel rod diameter is 9.2 cm. In addition to the parameters in the assembly, the center distance of the assembly also affects the uranium content per unit volume. And the center distance of the assemblies is 9.35 cm. Since the center distance of the fuel rods in the assembly and the center distance of the assembly need to be changed adaptively, so in this paper it’s considered that the rod to diameter ratio as the main representative influencing factor.
5 Design optimization
This section will take the 700 MWt and 1000 MWt cores as the research objects. By optimizing the effective density of the uranium-zirconium alloy fuel core and the ratio of the pitch distance and the rod diameter, the corresponding optimized core schemes can be obtained respectively, so that the core reactivity can be improved. There is almost no change during the life cycle, so as to reduce the difficulty of controlling the reactivity of the core and make the breeding performance of the core match the power and life of the core, so as to improve the safety and economy of the core.
5.1 Assembly optimization
According to the above optimization ideas, the parameter optimization is mainly carried out from the assembly level. Through calculation and verification, the fuel core density and the ratio of the pitch distance and rod diameter used after optimization are shown in Table 4.
5.2 Core optimization
Firstly, the core optimization design is carried out according to the assembly parameters after the optimized the rod to diameter ratio. By adjusting the fuel enrichment, two optimization schemes are proposed for Scheme 4 and Scheme 5, namely Scheme 4-O1, Scheme 4-O2, Scheme 5-O1 and scheme 5-O2. The design parameters of each core scheme are shown in Table 5, and the variation curve of reactivity with life of each core scheme is shown in Figure 8.
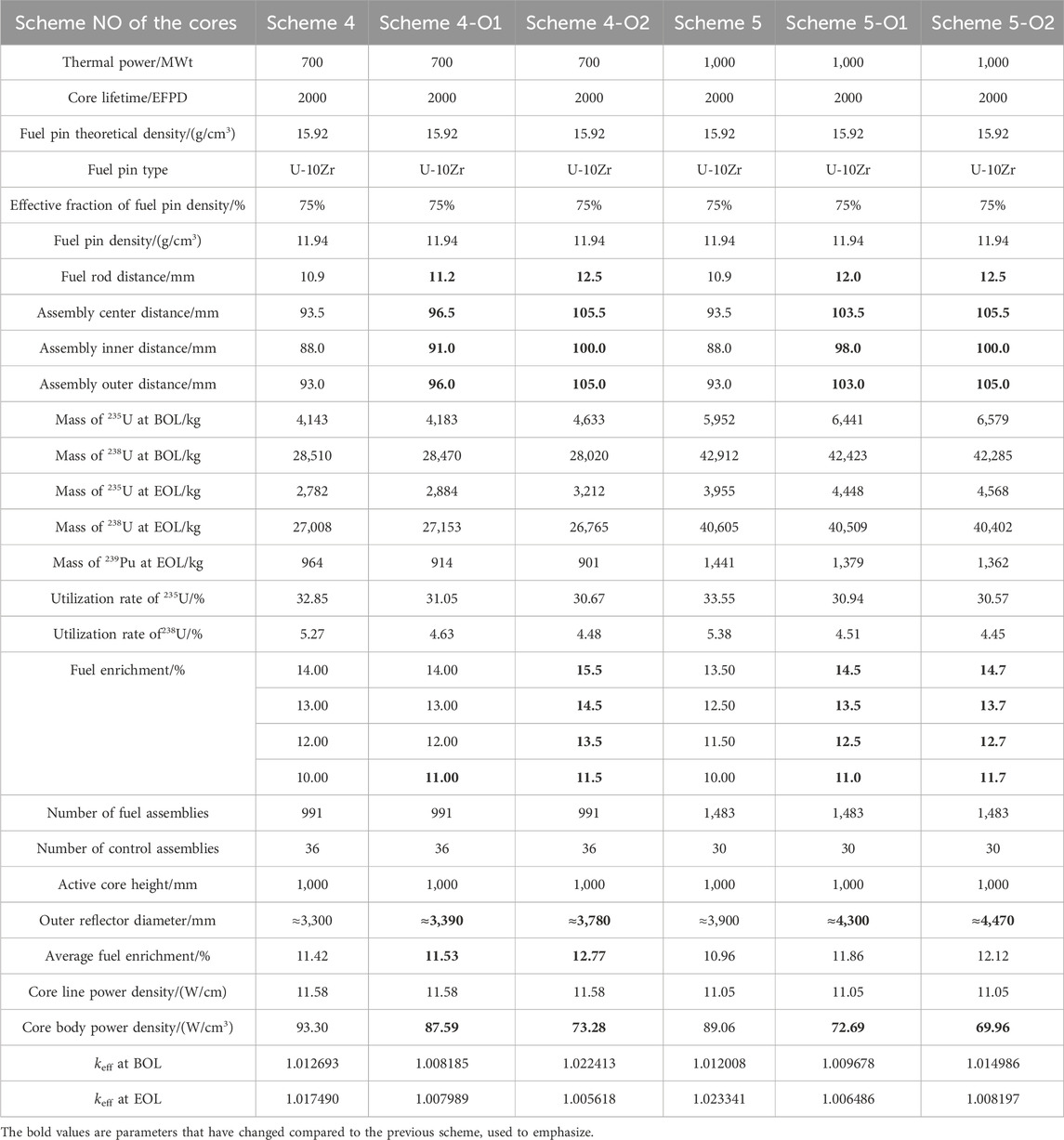
TABLE 5. Design parameters of each core scheme before and after optimization of the rod to diameter ratio.
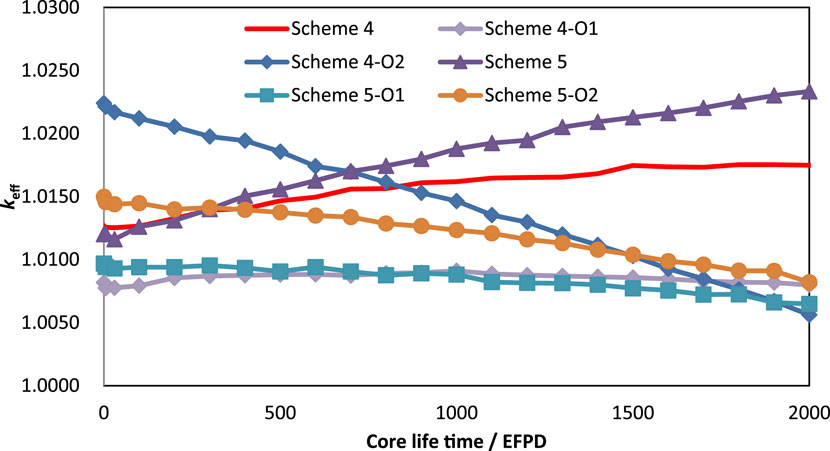
FIGURE 8. Reactivity curves along core life time of each core scheme before and after optimization of the rod to diameter ratio.
It can be seen from Table 5 that during the optimization of the scheme, by increasing the center distance of the fuel rods, the center distance of the assemblies, the inner-to-edge distance and the outer-to-edge distance of the assembly boxes are adjusted accordingly, and the average fuel enrichment of the core is appropriately increased. The diameter increases and the core power density decreases. This change is beneficial to thermal safety. The core power can be increased by appropriately increasing the core power density in theory. But in this article the core power is not changed to show the optimization effect brought by it.
It can be seen from Figure 8 that after optimizing the rod to diameter ratio of scheme 4-O1 and scheme 5-O1 match the core power and lifetime, and the reactivity of the core increases firstly and then increases during the lifetime. There is a downward trend, but the change range is very small, and it can be considered that the core reactivity hardly changes during the whole lifetime. Scheme 4-O2 and Scheme 5-O2 further increase the center distance of fuel rods on the basis of Scheme 4-O1 and Scheme 5-O1, and the reactivity curve shows a monotonically decreasing trend during the core lifetime, which is the similar to that of Scheme 1 and Scheme 2 with core powers of 100 MWt and 300 MWt in Figure 7, indicating that the breeding performance of the core is not enough to compensate for the reactivity loss caused by the core burnup.
From the above analysis and calculation results, it can be seen that the breeding performance of the core can be changed by adjusting the rod to diameter ratio, and the power and life of the core can match with the appropriate rod to diameter ratio, and finally the reactivity of the core hardly changes with the life, or the reactivity of the core can be monotonically decreased with the lifetime by further increasing the rod to diameter ratio. Similarly, Schemes 1 and 2 with power levels of 100 MWt and 300 MWt should theoretically be able to make the core reactivity almost unchanged with life by reducing the rod to diameter ratio. However, it can be seen from Table 5 that the disadvantageous factor brought by increasing the rod to diameter ratio is that the average fuel enrichment of the core needs to be increased, thereby increasing the 235U inventory of the core, and it is not conducive to the breeding performance of the core, and ultimately reduce the core fuel utilization rate.
This section continues to use the method of optimizing the fuel core density above to optimize the core design. Taking Scheme 4 as an example, the design parameters of each core scheme before and after optimizing the fuel core density are shown in Table 6. The change curve of core reactivity with life is shown in Figure 9.
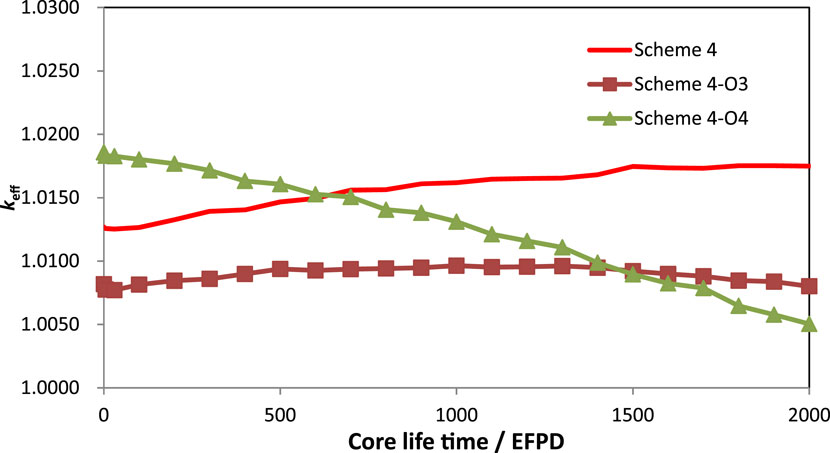
FIGURE 9. Reactivity curves along core life time of each core scheme before optimization of the density of fuel pin.
It can be seen from Table 6 that by reducing the effective density of the fuel from 75% to 70% and 65%, the required inventory of U and 235U of the core will be reduced, and the uranium resource utilization at the end of the core life will be appropriately improved.
It can be seen from Figure 9 that the reactivity of scheme 4-O3 hardly changes during the lifetime after the fuel core density is optimized, while the reactivity of the core of scheme 4-O4 decreases with the lifetime due to the further reduction of the effective density of the fuel core, and the reduction is a monotonous downward trend.
By adjusting the fuel core density, the change trend of the core reactivity with life can be adjusted. When the effective density of the fuel core matches with the core power and life, the core reactivity can hardly change during the life. At the same time, by adjusting the density of the fuel core, the inventory of U and 235U in the core at the beginning of the life can also be reduced, and the utilization rate of uranium resources can be appropriately improved.
6 Conclusion
In this paper, by studying the uranium-zirconium alloy fuel core scheme at different power levels, it is found that with the increase of the core power level and the increase of the core size, the reactivity of the core will increase firstly and then decrease with the life cycle, and at the end of the core life, the core reactivity cannot be effectively released. Based on this phenomenon, an optimization idea is proposed in this paper. By optimizing the rod to diameter ratio and the density of the fuel, the reactivity of the core hardly changes during the lifetime, or the reactivity of the core decreases monotonically with the lifetime. It can effectively improve the safety and economy of the core. The main conclusions of the above research are as follows:
(1) With the increase of the core size with uranium-zirconium alloy fuel, the breeding performance of the core gradually increases, and the reactivity of the core will increase firstly and then decrease with the life.
(2) By adjusting the rod to diameter ratio, the breeding performance of the core can be adjusted, so that the reactivity of the core hardly changes during the lifetime, but it will reduce core fuel utilization.
(3) By adjusting the density of the fuel, the breeding performance of the core can also be adjusted, and the reactivity of the core can also be almost unchanged during the life cycle, which can not only effectively reduce the inventory of U and 235U at the beginning of the life, but also it can improve the utilization rate of core fuel.
(4) The thermal performance of the core will be changed by adjusting the rod to diameter ratio. The design optimization of increasing the rod to diameter ratio in this paper is conducive to thermal safety. In the subsequent consideration of the physical and thermal coupling, both the rod to diameter ratio and fuel density can be adjusted, which ultimately improves the safety and economy of the core (Wang et al., 2013).
Data availability statement
The raw data supporting the conclusion of this article will be made available by the authors, without undue reservation.
Author contributions
LL: Methodology, Software, Writing–original draft. LW: Conceptualization, Methodology, Writing–review and editing. XP: Conceptualization, Validation, Writing–review and editing. ZC: Data curation, Software, Visualization, Writing–review and editing. BZo: Conceptualization, Data curation, Writing–review and editing. ChZ: Data curation, Supervision, Writing – review and editing. BZa: Data curation, Methodology, Supervision, Writing–review and editing. MY: Conceptualization, Funding acquisition, Writing–review and editing. DM: Funding acquisition, Software, Writing–review and editing. XW: Methodology, Software, Writing–review and editing. ZZ: Validation, Visualization, Writing–review and editing. CeZ: Software, Validation, Writing–review and editing.
Funding
The author(s) declare financial support was received for the research, authorship, and/or publication of this article. This work is supported by China Association for Science and Technology (Young Elite Scientists Sponsorship Program 2019QNRC001) and the National Natural Science Foundation of China (Nos.12205283).
Conflict of interest
The authors declare that the research was conducted in the absence of any commercial or financial relationships that could be construed as a potential conflict of interest.
Publisher’s note
All claims expressed in this article are solely those of the authors and do not necessarily represent those of their affiliated organizations, or those of the publisher, the editors and the reviewers. Any product that may be evaluated in this article, or claim that may be made by its manufacturer, is not guaranteed or endorsed by the publisher.
References
Wang, K., Li, Z. G., She, D., Liang, J., Xu, Q., Qiu, A., et al. (2013). “RMC-A Monte Carlo code for reactor physics analysis,” in Joint International Conference on Supercomputing in Nuclear Applications and Monte Carlo, July 2013, Paris, France.
Zhao, C., Lou, L., Peng, X., Zhang, B., and Wang, L. (2021). Application of the spectral-shift effect in the small lead-based reactor SLBR-50. Front. Energy Res. 9. 756106, doi:10.3389/fenrg.2021.756106
Zrodnikov, A. V., et al. (2001). Nuclear power plants based on reactormodules with svbr-75/100. At. Energy 91 (6). 957-966. doi:10.1023/A:1014862701400
Zrodnikov, A. V., Chitaikin, V. I., Toshinskii, G. I., Grigor'ev, O. G., Dragunov, Y. G., Stepanov, V. S., et al. “Fuel cycle of reactor SVBR-100,” in Proceedings of Global 2009, 2009, Paris, France, September 2009.
Keywords: lead-based modular reactor, uranium alloy fuel, optimization of reactor core conceptual design, effective density of fuel pin, the rod to diameter ratio
Citation: Lou L, Wang L, Peng X, Chen Z, Zhou B, Zhao C, Zhang B, Yan M, Ma D, Wang X, Zhao Z and Zhang C (2024) Optimization of conceptual design on the lead-based modular nuclear power reactor core loaded with U-10Zr alloy fuel. Front. Nucl. Eng. 3:1328964. doi: 10.3389/fnuen.2024.1328964
Received: 01 November 2023; Accepted: 31 January 2024;
Published: 19 February 2024.
Edited by:
Songbai Cheng, Harbin Engineering University, ChinaReviewed by:
Hui He, Shanghai Jiao Tong University, ChinaYandong Hou, Northeast Electric Power University, China
Copyright © 2024 Lou, Wang, Peng, Chen, Zhou, Zhao, Zhang, Yan, Ma, Wang, Zhao and Zhang. This is an open-access article distributed under the terms of the Creative Commons Attribution License (CC BY). The use, distribution or reproduction in other forums is permitted, provided the original author(s) and the copyright owner(s) are credited and that the original publication in this journal is cited, in accordance with accepted academic practice. No use, distribution or reproduction is permitted which does not comply with these terms.
*Correspondence: Lei Lou, MzcxNjgyMDExQHFxLmNvbQ==; Lianjie Wang, bWNkMjI2NEAxMjYuY29t