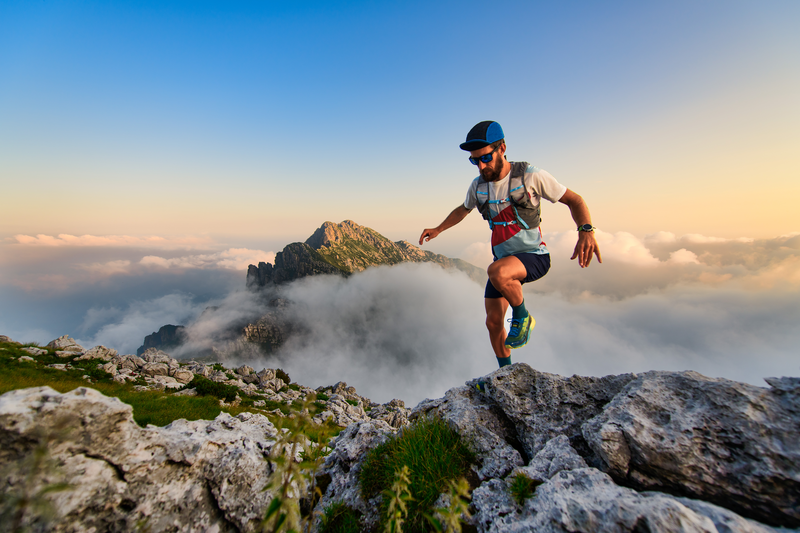
94% of researchers rate our articles as excellent or good
Learn more about the work of our research integrity team to safeguard the quality of each article we publish.
Find out more
FIELD GRAND CHALLENGE article
Front. Nucl. Eng. , 13 July 2022
Volume 1 - 2022 | https://doi.org/10.3389/fnuen.2022.945270
The 1992 United Nations Rio de Janeiro declaration (United Nations, 1992) states that “Human beings are at the centre of concerns for sustainable development. They are entitled to a healthy and productive life in harmony with nature.” This brief affirmation highlights the implications from the impact of growing human population on the environment (Cartledge, 1995; MacKay, 2009), manifested in the notion of climate emergency (Ripple et al., 2020). Nuclear power offers progressive options to mitigate global warming and other effects of climate change, with the International Energy Agency (IEA) suggesting that the nuclear energy generation currently eliminates between 1.3 and 2.6 giga-tonnes of CO2 emissions from the power sector each year, depending on whether it is assumed that it replaces gas- or coal-fired power plants.
The IEA’s 2015 Technology Roadmap (IEA, 2015) report noted that to meet the Paris Agreement target of global temperature not rising by more than 2°C, the world nuclear power generation capacity needs to increase to 930 GW in 2050. For comparison, the Smart Energy Europe analysis projected the European nuclear power generation capacity in 2050 to the level of 105 GW (Connolly et al., 2016), against the current levels of 61.3 GW in France, 9 GW in the United Kingdom, raising to 24 GW by 2050 (UK Government, 2022), and 4.3 GW in Germany, down from the 2021 level of 7.4 GW. Comparing these figures and the data for other geographical areas with the projected world total nuclear generation capacity, we observe that many new power plants are expected to be constructed in the countries where nuclear power generation technology and engineering have so far been largely unknown. This expected expansion will involve a broad range of engineering and technological challenges spanning the manufacturing of reactor components, the fabrication and extraction of fuel, the development of efficient coolant and heat transfer technology, the reactor assembly schedules, the establishment of supporting hot cell and waste processing facilities, and the technology for scheduled and unscheduled remote maintenance and operation, enabling the reactor systems to reliably function over long periods of time.
All the presently operating commercial nuclear reactors use fissile nuclear fuel, containing isotopes of uranium and other actinide elements. On the other hand, fusion power generation, an area of active development and innovation worldwide, aims to use light fusable chemical elements, for example the deuterium and tritium isotopes of hydrogen (Pearson and Takeda, 2020; Prager and Najmabadi, 2020). Fusion technology presents a range of scientific and engineering challenges that need to be addressed to enable the construction of a fusion power plant (Chapman and Walkden, 2020). These include the development of a reliable and safe tritium and deuterium extraction and handling technology, the integration of structural and functional materials in a power plant design, and the extensive use of remote handling and robotics in the maintenance of a power plant. But first and foremost, it is the development of robust means for controlling the high temperature plasma (Kodama et al., 2001; Hender et al., 2007), either in a magnetic confinement device or in a pulsed, for example a laser-driven, fusion system that presents an outstanding challenge to the fusion power plant engineering.
The fundamental considerations involved in the assessment of nuclear power are its economic competitiveness against the power sources using coal and gas, or the renewable sources like solar and wind power (Alonso et al., 2016), and its environmental impact (Pigford, 1974; Danish et al., 2022). The economic factors have until recently been driving a gradual decline of the global share of nuclear electricity generation from 17.5% in 1996 to 10% in 2019 (Ramana, 2021). However, the increasing cost of fossil fuels and the global warming resulting from the present unsustainable level of CO2 emissions, and the intermittent nature of solar and wind power generation as opposed to the firm energy delivered by the nuclear or hydroelectric power plants at a high level of stability of supply, appear to be shifting the balance, making nuclear power generation increasingly more attractive.
Among the environmental effects of nuclear power generation, the management, storage, and eventual disposal of nuclear waste–a significant part of which can be referred to as not fully used nuclear fuel–is guided by the internationally accepted standards (Kim et al., 2011). Annually, a 1 GW nuclear power plant generates several cubic metres of high-level radioactive waste requiring active cooling, and 500–1,000 cubic metres of low-level waste, and this waste must be disposed of in such a way that it imposes minimal burden of care on later generations. As a part of the disposal process, a proof beyond reasonable doubt is required that the increase in radiation due to the deposited material is a small fraction of the natural background level (Roberts, 1990). Fusion power plants are also expected to produce radioactive materials, requiring reprocessing or disposal, with a notable difference that fusion power, unlike fission, does not involve the use of actinides. A suitable choice of structural materials can further reduce the waste burden. Inventory calculations, included in the fusion power plant design process, can predict the evolution of chemical composition, activation, the decay heat of materials exposed to fusion neutrons, as well as the gamma-dose and neutron shielding requirements, maintenance schedules, aiding the recycling and disposal prospects (Gilbert et al., 2017).
Large light water reactors (LWRs) have been selected by the utility companies around the world as their primary choice of nuclear power plants because of their reliability, the economy of scale, and the fact that the construction of an LWR involves commonly available materials such as water, concrete, and stainless steel, offering the advantage of extensive know-how and enabling the rapid adaptation of existing technologies to the manufacturing of reactor components (Murakami, 2021).
The less well established nuclear power generating options presently attracting interest are the Small Modular Reactors (SMR) (Locatelli et al., 2014; Schaffrath et al., 2021), Fast Reactors (FRs) (Merk et al., 2015), especially the sodium-cooled FRs that have been developed and operated since the 1970s, and the high-temperature gas-cooled reactors that could drive hydrogen production (Jaszczur et al., 2016) or water desalination (Al-Othman et al., 2019). SMRs can replace the coal-fired power plants and be integrated with renewable sources into an electricity grid, ensuring the stability of supply and balancing the fluctuating wind and solar power generation (Liu et al., 2022). A sodium-cooled fast reactor system, a front-runner among the Generation IV reactors (Ramana, 2021), involves a fast-neutron-spectrum reactor and closed fuel recycling technology, enabling the improved use of nuclear fuel, management of high-level nuclear waste and, in particular, the utilization of plutonium and other actinides (Aoto et al., 2014). As recent practical steps, in February 2021 the BN-800 sodium-cooled fast reactor unit at the Beloyarsk nuclear power plant was connected to the grid, operating solely with uranium-plutonium fuel (BN-800, 2021) and, in December 2021, the world’s first high-temperature pebble-bed Generation IV reactor was launched at the Shidaowan nuclear power plant (CNNC, 2021).
What are the key scientific, technological and engineering challenges associated with the current state of development of nuclear power? The generic new features of advanced nuclear fission reactors were identified in a recent review by the Office of Nuclear Energy of the US Department of Energy. They include the requirement of no or minimal operator intervention in the event of an accident, the reduction of the amount of spent fuel requiring disposal, and the development of reactor technologies that can re-use the spent nuclear fuel. Also, new reactors are expected to utilize the heat directly for industrial processes, including hydrogen production and water desalination, and to enable load following, to integrate them into the electricity grid to support the intermittent power sources like solar and wind. Finally, if the aim of capital cost reduction resulting from the economy of scale can be achieved, this should enable the broader deployment of reactors in a modular form. Fusion power plants are expected to satisfy similar requirements (Federici et al., 2021), with the added engineering challenge associated with designing, constructing and operating the largest ever superconducting magnets (Sgobba et al., 2022). A number of major fusion engineering challenges have been already addressed in connection with the design and construction of ITER (Merola et al., 2014), and the increasing focus on building demonstration fusion power plants of different design, supported by private and public investment worldwide, is expected to help identify and address the challenges that are still outstanding.
Frontiers in Nuclear Engineering is a multi-disciplinary, open-access scientific journal providing the platform dedicated to the publication of ideas, reports, methods, techniques and data that can help advance the broad field of nuclear engineering, and enable addressing the above challenges. The aim of the journal is to encourage information exchange and collaboration between scientists, stakeholders, and civil society to support the environmentally sustainable and safe use of nuclear power.
Whereas above we emphasized the use of nuclear energy as a carbon-free power generation option, this by no means defines the entire range of applications of nuclear engineering. Nuclear reactors and accelerator-driven systems produce specialized isotopes for medical applications. X-ray, magnetic resonance imaging (MRI) and positron emission tomography (PET) scanners have revolutionised modern medicine by providing the means for in situ high-resolution imaging of organs in a human body. The development of advanced medical devices is a timely noble challenge to nuclear engineering. An example of application of nuclear engineering on a very different length scale, the small radioisotope general-purpose heat sources powered the Voyager probes that have now reached beyond the boundaries of the Solar system, and enabled other space missions including the martian Curiosity rover.
The development of nuclear engineering is expected to stimulate advances and discoveries in the related scientific disciplines, including the remote handling and robotics essential for the maintenance and operation of nuclear reactors, the use of new specialized materials, and the development of new mathematical methods and algorithms for computer modelling, to improve the understanding of operating conditions and safety of nuclear reactors. The concept of a virtual reactor REVE (REacteur Virtuel d’Etudes) proposed at the turn of the century (Jumel et al., 2000) has now evolved into the notion of a digital twin, a virtual replica of a reactor integrating the sensors, data, computers, and mathematical models that is expected to enable operating a reactor at an unprecedented level of monitoring, control, training, supervision and security (Yadav et al., 2021).
An even greater challenge is associated with the effort to design an advanced fission or a fusion power plant, where the predictive assessment of operating conditions and reliability of reactor components necessarily requires the development and application of multiscale multi-physics computer simulations (Eyre and Matthews, 1993; Gaston et al., 2009, 2015) that include models for the effect of neutron irradiation on materials (Cui et al., 2018; Mason et al., 2021; Reali et al., 2022). To ensure the success of macroscopic finite element simulations, the underlying microscopic models must be able to interpolate and extrapolate over a broad range of environmental parameters and conditions. The challenge here involves optimising the balance between the generation of input data for the data-driven algorithms (Schmidt et al., 2019), which is a difficult issue in its own right as the expected conditions in a reactor are hard to reproduce in a laboratory, and the fundamental physical content, defining the quality and range of validity of a model (Finnis, 2003). A particular question concerns the digital interfaces between the various models involved in an integrated numerical representation of a reactor, where the verification, validation and uncertainty quantification of coupled neutron transport and thermo-hydraulic models in the context of LWR design and safety analysis were reviewed in (Ivanov and Avramova, 2007; Avramova and Ivanov, 2010; Avramova et al., 2021).
Nuclear engineering involves the invention and development of means for exploiting and controlling the energy confined in the atomic nuclei by one of the most fundamental natural phenomena, the strong force (Salam and Taylor, 1990). It is hardly a surprise that the subject proves challenging and demanding, since the sub-atomic origin of nuclear energy makes the problem inherently complex and multi-scale.
The starting point for nuclear engineering is the understanding of nuclear reactions, the transport of neutrons, other sub-atomic particles and electromagnetic radiation, and their interaction with materials and effect on reactor components. This raises the question of quantitative observation, linked with modelling and simulation of operating conditions in a nuclear engineering system. Given the statistical and stochastic nature of nuclear interactions, the probabilistic aspects of structural integrity of nuclear reactors emerge as one of the important areas in reliability and safety analysis (Chavoshi et al., 2021).
The efficient use of nuclear fuel is not only an economic consideration but also an environmental challenge since it is the partially burnt fuel that produces the high level waste. The efficient use of fuel involves not only the optimal choice of its chemical composition and microstructure (Tonks et al., 2017) but also the selection of high-performance cladding materials and operating conditions. The development and qualification of cladding (Preuss, 2021) and advanced structural nuclear materials (Cabet et al., 2019; Zinkle et al., 2019; Rieth et al., 2021) is an area of research where Frontiers in Nuclear Engineering will help stimulate the interdisciplinary collaboration between materials scientists and engineers.
Finally, we would like to highlight an overarching challenge that has a bearing on all current and future endeavours in the field of nuclear engineering. The challenge is in its relation to society, through understanding, communication and education. Nuclear science and engineering is an advanced field, where applications are based on the detailed knowledge and appreciation of complex nuclear phenomena, often occurring in extreme conditions. The detailed rational explanation and communication of the concepts and data, the critical assessment of risks and benefits, and the advanced means for the visual and interactive communication of fundamental nuclear ideas and notions, bridging science and art, are among the essential topics that Frontiers in Nuclear Engineering aims to promote and inspire.
The author confirms being the sole contributor of this work and has approved it for publication.
This work received funding from the RCUK Energy Programme Grant No. EP/W006839/1 and MIDAS EPSRC Grant No. EP/S01702X/1, and was partially carried out within the framework of the EUROfusion Consortium, funded by the European Union via the Euratom Research and Training Programme (Grant Agreement No 101052200—EUROfusion). Views and opinions expressed are however those of the author only and do not necessarily reflect those of the European Union or the European Commission. Neither the European Union nor the European Commission can be held responsible for them.
The author declares that the research was conducted in the absence of any commercial or financial relationships that could be construed as a potential conflict of interest.
All claims expressed in this article are solely those of the authors and do not necessarily represent those of their affiliated organizations, or those of the publisher, the editors and the reviewers. Any product that may be evaluated in this article, or claim that may be made by its manufacturer, is not guaranteed or endorsed by the publisher.
The author is grateful to G. Aiello, R. Akers, M. Boleininger, M. R. Gilbert, P.-W. Ma, D. R. Mason, J. R. Matthews, M. O’Brien, M. Preuss and L. Reali for stimulating discussions.
Al-Othman, A., Darwish, N. N., Qasim, M., Tawalbeh, M., Darwish, N. A., and Hilal, N. (2019). Nuclear Desalination: A State-Of-The-Art Review. Desalination 457, 39–61. doi:10.1016/j.desal.2019.01.002
Alonso, G., Bilbao, S., and Valle, E. (2016). Economic Competitiveness of Small Modular Reactors versus Coal and Combined Cycle Plants. Energy 116, 867–879. doi:10.1016/j.energy.2016.10.030
Aoto, K., Dufour, P., Hongyi, Y., Glatz, J. P., Kim, Y.-i., Ashurko, Y., et al. (2014). A Summary of Sodium-Cooled Fast Reactor Development. Prog. Nucl. Energy 77, 247–265. doi:10.1016/j.pnucene.2014.05.008
Avramova, M., Abarca, A., Hou, J., and Ivanov, K. (2021). Innovations in Multi-Physics Methods Development, Validation, and Uncertainty Quantification. J. Nucl. Eng. 2, 44–56. doi:10.3390/jne2010005
Avramova, M. N., and Ivanov, K. N. (2010). Verification, Validation and Uncertainty Quantification in Multi-Physics Modeling for Nuclear Reactor Design and Safety Analysis. Prog. Nucl. Energy 52, 601–614. doi:10.1016/j.pnucene.2010.03.009
BN-800 (2021). BN-800 Fast Reactor Has First Full Refuelling with MOX Fuel (World Nuclear News). Available at: https://world–nuclear–news.org/Articles/BN–800–fast–reactor–fully–loaded–with–MOX–fuel.
Cabet, C., Dalle, F., Gaganidze, E., Henry, J., and Tanigawa, H. (2019). Ferritic-martensitic Steels for Fission and Fusion Applications. J. Nucl. Mater. 523, 510–537. doi:10.1016/j.jnucmat.2019.05.058
Cartledge, B. (1995). Population and the Environment: The Linacre Lectures 1993-4. Oxford, United Kingdom: Oxford University Press.
Chapman, I. T., and Walkden, N. R. (2020). An Overview of Shared Technical Challenges for Magnetic and Inertial Fusion Power Plant Development. Phil. Trans. R. Soc. A 379, 20200019. doi:10.1098/rsta.2020.0019
Chavoshi, S. Z., Booker, J., Bradford, R., and Martin, M. (2021). A Review of Probabilistic Structural Integrity Assessment in the Nuclear Sector and Possible Future Directions. Fatigue Fract. Eng. Mater Struct. 44, 3227–3257. doi:10.1111/ffe.13572
CNNC (2021). World’s First HTR-PM Nuclear Power Plant Connected to Grid (China National Nuclear Corporation). Available at: https://en.cnnc.com.cn/2021-12/20/c_692103.htm.
Connolly, D., Lund, H., and Mathiesen, B. V. (2016). Smart Energy Europe: The Technical and Economic Impact of One Potential 100 % Renewable Energy Scenario for the European Union. Renew. Sustain. Energy Rev. 60, 1634–1653. doi:10.1016/j.rser.2016.02.025
Cui, Y., Po, G., and Ghoniem, N. M. (2018). A Coupled Dislocation Dynamics-Continuum Barrier Field Model with Application to Irradiated Materials. Int. J. Plasticity 104, 54–67. doi:10.1016/j.ijplas.2018.01.015
Danish, D., Ulucak, R., and Erdogan, S. (2022). The Effect of Nuclear Energy on the Environment in the Context of Globalization: Consumption vs Production-Based CO2 Emissions. Nucl. Eng. Technol. 54, 1312–1320. doi:10.1016/j.net.2021.10.030
Eyre, B. L., and Matthews, J. R. (1993). Technological Impact of Microstructural Evolution during Irradiation. J. Nucl. Mater. 205, 1–15. doi:10.1016/0022-3115(93)90066-8
Federici, G., Baylard, C., Beaumont, A., and Holden, J. (2021). The Plan Forward for EU DEMO. Fusion Eng. Des. 173, 112960. doi:10.1016/j.fusengdes.2021.112960
Finnis, M. (2003). Interatomic Forces in Condensed Matter. Oxford, United Kingdom: Oxford University Press.
Gaston, D., Newman, C., Hansen, G., and Lebrun-Grandié, D. (2009). MOOSE: A Parallel Computational Framework for Coupled Systems of Nonlinear Equations. Nucl. Eng. Des. 239, 1768–1778. doi:10.1016/j.nucengdes.2009.05.021
Gaston, D. R., Permann, C. J., Peterson, J. W., Slaughter, A. E., Andrš, D., Wang, Y., et al. (2015). Physics-Based Multiscale Coupling for Full Core Nuclear Reactor Simulation. Ann. Nucl. Energy 84, 45–54. doi:10.1016/j.anucene.2014.09.060
Gilbert, M. R., Eade, T., Bachmann, C., Fischer, U., and Taylor, N. P. (2017). Activation, Decay Heat, and Waste Classification Studies of the European DEMO Concept. Nucl. Fusion 57, 046015. doi:10.1088/1741-4326/aa5bd7
Hender, T. C., Wesley, J. C., Bialek, J., Bondeson, A., Boozer, A. H., Buttery, R. J., et al. (2007). Chapter 3: MHD Stability, Operational Limits and Disruptions. Nucl. Fusion 47, S128–S202. doi:10.1088/0029-5515/47/6/S03
Ivanov, K., and Avramova, M. (2007). Challenges in Coupled Thermal-Hydraulics and Neutronics Simulations for LWR Safety Analysis. Ann. Nucl. Energy 34, 501–513. doi:10.1016/j.anucene.2007.02.016
Jaszczur, M., Rosen, M. A., Śliwa, T., Dudek, M., and Pieńkowski, L. (2016). Hydrogen Production Using High Temperature Nuclear Reactors: Efficiency Analysis of a Combined Cycle. Int. J. Hydrogen Energy 41, 7861–7871. doi:10.1016/j.ijhydene.2015.11.190
Jumel, S., Domain, C., Ruste, J., Van Duysen, J. C., Becquart, C., Legris, A., et al. (2000). Simulation of the Irradiation Effects in Reactor Materials : The REVE Project. J. Phys. IV Fr. 10, Pr6–191. doi:10.1051/jp4:2000633
Kim, J.-S., Kwon, S.-K., Sanchez, M., and Cho, G.-C. (2011). Geological Storage of High Level Nuclear Waste. KSCE J. Civ. Eng. 15, 721–737. doi:10.1007/s12205-011-0012-8
Kodama, R., Norreys, P. A., Mima, K., Dangor, A. E., Evans, R. G., Fujita, H., et al. (2001). Fast Heating of Ultrahigh-Density Plasma as a Step Towards Laser Fusion Ignition. Nature 412, 798–802. doi:10.1038/35090525
Liu, Y., Huang, G., Chen, J., Zhang, X., Zheng, X., and Zhai, M. (2022). Development of an Optimization-Aided Small Modular Reactor Siting Model - A Case Study of Saskatchewan, Canada. Appl. Energy 305, 117867. doi:10.1016/j.apenergy.2021.1178610.1016/j.apenergy.2021.117867
Locatelli, G., Bingham, C., and Mancini, M. (2014). Small Modular Reactors: A Comprehensive Overview of Their Economics and Strategic Aspects. Prog. Nucl. Energy 73, 75–85. doi:10.1016/j.pnucene.2014.01.010
Mason, D. R., Granberg, F., Boleininger, M., Schwarz-Selinger, T., Nordlund, K., and Dudarev, S. L. (2021). Parameter-Free Quantitative Simulation of High-Dose Microstructure and Hydrogen Retention in Ion-Irradiated Tungsten. Phys. Rev. Mater. 5, 095403. doi:10.1103/PhysRevMaterials.5.095403
Merk, B., Stanculescu, A., Chellapandi, P., and Hill, R. (2015). Progress in Reliability of Fast Reactor Operation and New Trends to Increased Inherent Safety. Appl. Energy 147, 104–116. doi:10.1016/j.apenergy.2015.02.023
Merola, M., Escourbiac, F., Raffray, R., Chappuis, P., Hirai, T., and Martin, A. (2014). Overview and Status of ITER Internal Components. Fusion Eng. Des. 89, 890–895. doi:10.1016/j.fusengdes.2014.01.055
Murakami, T. (2021). A Historical Review and Analysis on the Selection of Nuclear Reactor Types and Implications to Development Programs for Advanced Reactors; A Japanese Study. Energy Rep. 7, 3428–3436. doi:10.1016/j.egyr.2021.05.049
Pearson, R. J., and Takeda, S. (2020). “Review of Approaches to Fusion Energy,” in Commercialising Fusion Energy. Editors W. J. Nuttall, S. Konishi, S. Takeda, and D. Webbe-Wood (Bristol: Institute of Physics). doi:10.1088/978-0-7503-2719-0ch2
Pigford, T. H. (1974). Environmental Aspects of Nuclear Energy Production. Annu. Rev. Nucl. Sci. 24, 515–560. doi:10.1146/annurev.ns.24.120174.002503
Prager, S. C., and Najmabadi, F. (2020). Fusion Reactor (Encyclopedia Britannica). Available at: https://www.britannica.com/technology/fusion–reactor.
Preuss, M. (2021). “A Review of Early Findings within the Collaborative Research Programme MUZIC-"Mechanistic Understanding of Zirconium Corrosion",” in Zirconium in the Nuclear Industry - 19th International Symposium, STP1622. Editors A. T. Motta, and S. K. Yagnik (West Conshohocken, USA: ASTM International), 44–65. doi:10.1520/STP162220190105
Ramana, M. V. (2021). Small Modular and Advanced Nuclear Reactors: a Reality Check. IEEE Access 9, 42090–42099. doi:10.1109/ACCESS.2021.3064948
Reali, L., Boleininger, M., Gilbert, M. R., and Dudarev, S. L. (2022). Macroscopic Elastic Stress and Strain Produced by Irradiation. Nucl. Fusion 62, 016002. doi:10.1088/1741-4326/ac35d4
Rieth, M., Simondon, E., Pintsuk, G., Aiello, G., Henry, J., Terentyev, D., et al. (2021). Technological Aspects in Blanket Design: Effects of Micro-Alloying and Thermo-Mechanical Treatments of EUROFER97 Type Steels after Neutron Irradiation. Fusion Eng. Des. 168, 112645. doi:10.1016/j.fusengdes.2021.112645
Ripple, W. J., Wolf, C., Newsome, T. M., Barnard, P., and Moomaw, W. R. (2020). World Scientists' Warning of a Climate Emergency. BioScience 70, 8–12. doi:10.1093/biosci/biz088
Roberts, L. E. J. (1990). Radioactive Waste Management. Annu. Rev. Nucl. Part. Sci. 40, 79–112. doi:10.1146/annurev.ns.40.120190.000455
Salam, A., and Taylor, J. C. (1990). Unification of Fundamental Forces: The First 1988 Dirac Memorial Lecture. Cambridge, United Kingdom: Cambridge University Press.
Schaffrath, A., Wielenberg, A., Kilger, R., and Seubert, A. (2021). SMRs - Overview, International Developments, Safety Features and the GRS Simulation Chain. Front. Energy 15, 793–809. doi:10.1007/s11708-021-0751-2
Schmidt, J., Marques, M. R. G., Botti, S., and Marques, M. A. L. (2019). Recent Advances and Applications of Machine Learning in Solid-State Materials Science. npj Comput. Mater 5, 83–3257. doi:10.1038/s41524-019-0221-0
Sgobba, S., Aviles Santillana, I., Lourenco, S., Guinchard, M., De Frutos, O., Jong, C., et al. (2022). Examination and Characterization of Physical and Mechanical Properties of the ITER Central Solenoid Module Coils. IEEE Trans. Appl. Supercond. 32, 1–5. doi:10.1109/TASC.2022.3147732
Tonks, M. R., Andersson, D., Phillpot, S. R., Zhang, Y., Williamson, R., Stanek, C. R., et al. (2017). Mechanistic Materials Modeling for Nuclear Fuel Performance. Ann. Nucl. Energy 105, 11–24. doi:10.1016/j.anucene.2017.03.005
UK Government (2022). Major Acceleration of Homegrown Power in Britain’s Plan for Greater Energy Independence. London, United Kingdom: Department of Business, Energy and Industrial Strategy.
United Nations (1992). Report of the United Nations Conference on Environment and Development, Rio de Janeiro, 13-14 June, 1992. Rio de Janeiro, Brazil: United Nations General Assembly report. A/CONF.151/26.
Yadav, V., Agarwal, V., Gribok, A. V., Hays, R. D., Pluth, A. J., Ritter, C. S., et al. (2021). Technical Challenges and Gaps in Digital-Twin-Enabling Technologies for Nuclear Reactor Applications. Idaho Falls, ID: Idaho National Laboratory report. INL/EXT-21-65316; TLR/RES-DE-REB-2021-17).
Keywords: nuclear engineering, fusion and fission, nuclear fuel, waste disposal, nuclear materials, deuterium and tritium, radiation effects, computer modelling and simulation
Citation: Dudarev SL (2022) Grand Challenges in Nuclear Engineering. Front. Nucl. Eng. 1:945270. doi: 10.3389/fnuen.2022.945270
Received: 16 May 2022; Accepted: 13 June 2022;
Published: 13 July 2022.
Edited and reviewed by:
Edgar C. Buck, Pacific Northwest National Laboratory (DOE), United StatesCopyright © 2022 Dudarev. This is an open-access article distributed under the terms of the Creative Commons Attribution License (CC BY). The use, distribution or reproduction in other forums is permitted, provided the original author(s) and the copyright owner(s) are credited and that the original publication in this journal is cited, in accordance with accepted academic practice. No use, distribution or reproduction is permitted which does not comply with these terms.
*Correspondence: Sergei L. Dudarev, c2VyZ2VpLmR1ZGFyZXZAdWthZWEudWs=
Disclaimer: All claims expressed in this article are solely those of the authors and do not necessarily represent those of their affiliated organizations, or those of the publisher, the editors and the reviewers. Any product that may be evaluated in this article or claim that may be made by its manufacturer is not guaranteed or endorsed by the publisher.
Research integrity at Frontiers
Learn more about the work of our research integrity team to safeguard the quality of each article we publish.