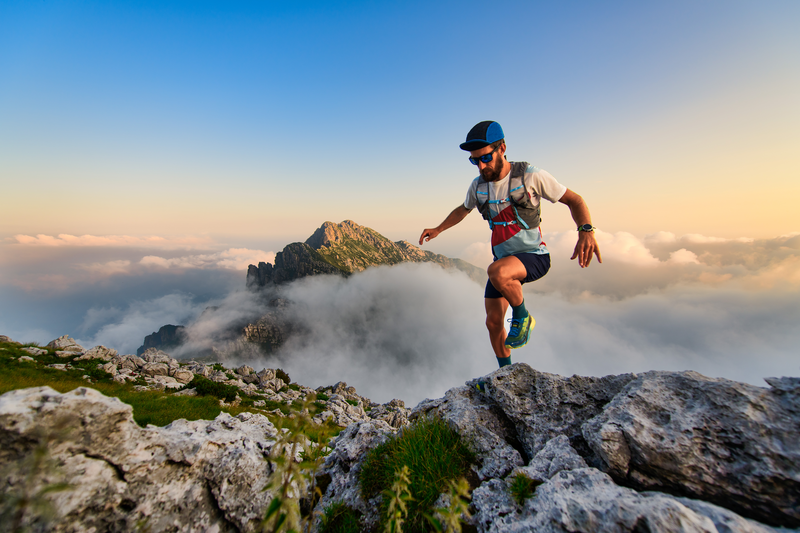
95% of researchers rate our articles as excellent or good
Learn more about the work of our research integrity team to safeguard the quality of each article we publish.
Find out more
MINI REVIEW article
Front. Nat. Prod. , 26 August 2024
Sec. Biological Activities of Natural Products
Volume 3 - 2024 | https://doi.org/10.3389/fntpr.2024.1456361
Natural products have been used since ancient times to treat various ailments and have been recognized for many years as a source of therapeutic agents and structural diversity. Plant-derived products have thus served as dietary components but also to maintain a state of wellbeing and health by preventing different diseases both of inflammatory and infective nature. Pentacyclic triterpenoids, particularly ursolic acid (UA) and oleanolic acid (OA), are well-studied natural products endowed with complex biological profiles. In this mini-review, we summarized the most advanced results on extraction methodologies and antimicrobial activity of UA and OA, focusing on their potential role as antimicrobic adjuvants, bacterial biofilm inhibitors and related mechanisms of action. This offers a theoretical basis and inspiration for further studies on their bioactivity mechanism.
The emergence of new pathogens is a significant global issue, presenting unique challenges for public health. For decades, scientists have warned that microorganisms are rapidly evolving resistance to antibiotics, complicating the effective treatment of many infectious diseases, but antimicrobial resistance has nevertheless become a global pandemic threatening human health, life expectancy, and food production. The increasing antibiotic resistance and the void in the new class of antibiotics discovery have given a renewed impulse to search for natural sources and new antibacterial strategies (Elmaidomy et al., 2022; Atanasov et al., 2021). New bioactive natural products (NPs) and combinations of NPs have shown the potential to contrast the rising incidence of drug-resistant pathogens (Vaou et al., 2021) and examples of potential next-generation therapeutics include bacteriophages (Luong et al., 2020) antibacterial peptide produced by microorganism (Xuan et al., 2023) and plant-derived compounds such as polyphenols and flavonoids (Manso et al., 2021), terpenoids (Sycz et al., 2022), plant essential oils (López-Hortas et al., 2018), and alkaloids (Othman et al., 2019). Triterpenoids represent the most abundant group of terpenoids found in dicotyledons serving as chemical defenses against competing plants, pathogens and herbivores (Wrońska et al., 2022). They exhibit antioxidant, antimicrobial, antiallergic, antidiabetic, fungicidal (Becker et al., 2005) and antiparasitic (Jaki et al., 2008) properties. Additional studies have also reported their anti-inflammatory (Andre et al., 2012), analgesic (Hussain et al., 2017; G; Liu et al., 2023), cardiotonic and sedative (Do Nascimento et al., 2014) properties. Particularly, two triterpenoids, namely, ursolic acid (UA, Figure 1, 1) and oleanolic acid (OA, Figure 1, 2) and their derivatives are renewed for their antibacterial properties against Gram-positive (Park et al., 2015) and Gram-negative bacteria (Sycz et al., 2022). This mini-review aims to summarize recent updates concerning the extraction methodologies and bioactivity of UA and OA with a focus on the mode of action of antibacterial and anti-biofilm activities.
Since pharmacologically active compounds are usually present in low concentrations in vegetable matrices, optimal extraction conditions are always needed for their recovery and purification (Tostes et al., 2016). Many studies have been published concerning the extraction of UA and OA from plant materials using methods such as maceration with ethanol (Ludeña Huaman et al., 2021) or sunflower oil (Maisto et al., 2023) as well as ultrasound-assisted extraction (Fan et al., 2016). Interestingly, in recent years a great attention has been directed to more environmentally friendly and low toxic solvents and extraction techniques. Natural deep eutectic solvents (NADES) are a class of solvents that are formed by the combination of a hydrogen bond donor and a hydrogen bond acceptor, which results in a eutectic mixture (Villa et al., 2024). While most NADES are hydrophilic, hydrophobic deep eutectic solvents (HDES) have been recently discovered. HDES can selectively dissolve non-polar compounds, offering an alternative to traditional organic solvents (Cao and Su, 2021). Silva et al. (2020) published a study in which the extraction of triterpene acids from Eucalyptus globules biomass was evaluated by using HDES. The solubility of UA in ethanol was approximately 5 mg/g and in n-hexane it was 13 mg/g. However, using the series of HDES, the solubility significantly increased ranging from 28 up to 50 mg/g. Furthermore, UA’s solubility was highest in HDES with higher thymol ratio at 60°C leading to a solubility of 66 mg/g. Overall, the results were positive for the recovery of triterpene acids while reinforcing the potential of DES as potential solvents to be applied in extraction processes. In 2023, Li et al. (2023) published a paper concerning the extraction of UA with HDES corroborating previous results. Fifteen different HDES were prepared and used to extract UA from apple. The results shown that the solubility of UA in HDES can be nine times higher than traditional solvent such as ethanol. The highest UA solubility was observed in HDES composed of menthol and thymol with a molar ratio 1:6 and an optimization temperature of 49°C. The same chemical considerations can also be made concerning OA extraction. In the same year, Petrochenko et al. (2023), successfully extracted twenty-one different triterpene saponins (derivatives of UA and OA) from Aralia elata var. mandshurica using seven acid-based NADES. Hydrophilic NADES were found to be more effective solvents for extracting the triterpenes saponins compared to water and ethanol. Specifically, NADES formulated with choline chloride and malic acid (1:1), as well as sorbitol and malic acid (1:1), demonstrated the highest solubility for the target compounds. Besides the numerous publications available concerning UA and OA extraction, there are also different patented sequences for extraction and purification of UA and OA, but to our knowledge, these patented technologies use organic and more toxic solvents making these technologies only appreciable in the laboratory scale research (Hussain et al., 2017).
The threat posed by antibiotic resistance has motivated the quest for novel strategies to deal with the new generations of multidrug resistant bacteria. Triterpenoids, and UA and OA in particular, show high prospective in the context. Thus, while the antibacterial actions of UA and OA in the planktonic state is known for decades, the last years have seen new and unexpected modalities of action be deciphered (Table 1). The antibacterial actions of triterpenoids are reported to be associated with morphological changes in the bacterial cells (Sycz et al., 2022), with UA and OA stimulating chemotaxis genes in host defense and affecting bacterial gene expression related to biofilm formation, peptidoglycan turnover, and cell autolysis (Wolska et al., 2010). In addition, OA and UA along with their derivatives, have shown strong antimutagenic effects (Lira et al., 2008). In 2003, a study was published regarding the sensitization by triterpenoids of S.aureus and Escherichia coli to antibiotics by Brehm-Stecher & Johnson, (2003). Due to their inherent lipophilicities, terpenoids show an affinity for and partition within biological membranes, where their accumulation may have an impact on the structural and functional properties of these membranes. Starting from this concept, it was discovered that triterpenoids and sesquiterpenoids can disrupt the normal barrier function of the bacterial cell membrane, allowing the permeation into the cell of exogenous solutes such as ethidium bromide and different antibiotics. In 2023, Sekandi et al. (2023) investigated the antibacterial and antifungal effects of Spermacoce princeae (K. schum), a medicinal plant used in the African traditional medicine to treat skin infections. They isolated and purified different active compounds, including UA, and results highlighted that UA was active against S. aureus and E. coli with inhibition zones of 20.0 ± 0.1 mm and 18.0 ± 0.1 mm, respectively and fungi C. albicans and A. flavus, with inhibition zones of 12.0 ± 0.1 mm and 20.5 ± 0.3. Recently, Du et al. (2024) published a study where they investigated the antibacterial mode of action of compounds isolated from Urtica dioica L. The antimicrobial activity against methicillin-resistant Staphylococcus aureus (MRSA) was explored based on bio-guided fractioning. Thus, nine pharmacologically active compounds were isolated (including UA, with MIC of 0.75 mg/mL against MRSA), all of them inhibiting bacterial division and growth during the logarithmic period. The inhibition was mediated by the inhibition of cell structure, leading to leakage of sugars, nucleic acids and proteins. Another possible mechanism of action of these triterpenoids is related to their moderate ability to interfere with efflux pumps, which could directly interfere with the viability of this species, as demonstrated in E.coli. (Martins et al., 2011). Triterpenoids from Rosmarinus officinalis and extracts from Levisticum officinale were reported to inhibit the multidrug efflux pump and thus potentiate respectively the susceptibility of S.aureus to erythromycin (Oluwatuyi et al., 2004) and P.aeruginosa to ciprofloxacin (Al-Qudah, 2024). OA and UA are associated with the induction of stress response (Grudniak et al., 2011; Park et al., 2015, studied the mechanism of action of OA and UA on S.mutans and the findings suggested that the antimicrobial activity is achieved by inhibiting glycolysis, fatty acids synthesis, amino acids synthesis, and peptidoglycan synthesis pathways. In 2012, a study published by Kurek et al. (2012) showed that the pentacyclic terpenoids UA and OA can modulate resistance to β-lactam antibiotics, ampicillin and oxacillin, in four bacterial pathogens (P.aeruginosa, S.aureus, S.epidermidis, L.monocytogenes). FICI (fractional inhibitory concentration index) estimation and the results of time-kill assays demonstrated that some combinations of the tested compounds produced synergistic antibacterial effects. OA and/or UA in combination with ampicillin have shown synergic effects on S. aureus, both in the planktonic and in biofilm states, and against S.epidermidis in the state of biofilm. OA and UA exhibited a synergistic effect with oxacillin on S.aureus, S.epidermidis, L.monocytogenes although in the case of S.aureus, only the combination with OA and not UA displayed significant synergy. The authors hypothesized two different mechanisms of action for the observed synergy, namely, the possibility that PBPs, the target of β-lactams, are also the target of these pentacyclic triterpenoids, and that triterpenoids interfere with β-lactamase translocation to the periplasm. However, as could have been expected the marked structural diversity between triterpenoids and β-lactams, none of the studied L.monocytetogenes PBPs was able to interact with radiolabeled OA, and OA failed to compete with penicillin for binding to PBPs. In addition, the synergy of the combination of triterpenoids and β-lactams in preventing the growth of non-pathogenic E.coli cannot be explained entirely by inhibition of β-lactamase translocation to the periplasm, since this was found to be diminished by only 25%. It has also been demonstrated that both OA and UA do not substantially increase the transport of β-lactam (nitrocefin) into E.coli cells. Thus, the synergistic action of plant-derived compounds and antibiotics is based on the interaction of the agents with bacterial resistance mechanisms, each of these may be influenced by plant-derived compounds. Some years later, Catteau et al. (2017) published an interesting study concerning the synergistic effect of these two triterpenoids with β-lactams on MRSA. In the study, they evaluated the direct and indirect antimicrobial activities of Vitellaria paradoxa leaves extract (containing known concentrations of UA and OA) in combination with β-lactams (ampicillin, oxacillin and nafcillin) on a panel of S.aureus reference strains as well as clinical MRSA isolates. A synergistic effect was obtained for concentrations of 4 and 8 mg/L of UA and OA (i.e., ¼ MIC), respectively with both antibiotics. The potential mechanism of action was investigated focusing on the two principal β-lactam resistance mechanisms of MRSA. Results were somehow inconsistent with those of Kurek et al. (2012), as both triterpene acids reverted β-lactam resistance mediated by PBP2A delocalization (effect of OA and UA on PBP2 recruitment to the septum) and inhibition of β-lactamase activity (nitrocefin test). Moreover, the synergistic effect in vivo was also investigated. UA in combination with nafcillin was tested in a murine model of subcutaneous infection of MRSA. Results demonstrated that the synergy also takes place in vivo by reducing the size of the lesion and the production of pro-inflammatory cytokines. For instance, in 2007, data published showed that farnesol (a natural sesquiterpene) can inhibit the recycling of C55 lipid carrier of the murein monomer precursor and reduce the secretion and activity of β-lactamase, thus contributing to increased susceptibility to β-lactams MRSA (Kuroda et al., 2007). The ability of triterpenoids to inhibit biofilm formation and/or disruption of preformed biofilm, is also an object of several studies. Bacteria can form biofilms on living surfaces such as tissue and non-living surfaces like medical devices and implants which are of the utmost clinical relevance. Ren et al. (2005) found that UA inhibits biofilm formation and demonstrated that this compound affects the expression of genes in E.coli, particularly those involved in sulfur metabolism, stress responses and cysteine regulation. Hence, they demonstrated that the biofilm inhibition by UA does not seem to be restricted to specific species, strains or growth conditions. By studying the complete transcriptome, it was also shown that UA induces chemotaxis, motility, and heat shock genes in E.coli and represses genes related to sulfur metabolism, which suggests that UA may function as a signal that tells cells to remain too motile for adequate biofilm formation. UA was effective at low concentrations since 10 μg/mL removed 72% of the E.coli biofilm. Hence, the addition of UA as an adjuvant to destabilize biofilms is an alternative approach. UA may also be chemically modified to improve efficacy by using combinatorial chemistry and screening with the biofilm assay to identify even better biofilm inhibitors. Grudniak et al. (2011) observed that E.coli treated with OA and UA altered the synthesis of DnaK, inducing the heat-shock response in this species. The results demonstrated that the treatment of E.coli with OA or UA reduces the expression of three operons that are members of the cysteine regulation. In addition, OA is a weak inducer of DnaK synthesis, so this compound extends the list of known stress-inducing agents. Again, Y. Liu et al. (2021) in a study recently published, confirmed the antibiofilm activity of UA against S.mutans and further revealed the mechanism underlying the inhibitory effect of UA on glucosyltransferases (GTF) mediated synthesis of extracellular polysaccharides (EPSs), and so they provide UA as a potential antimicrobial agent that can be used to prevent and cure oral and other GTF-related diseases. To explore the potential of UA, they first performed an antimicrobial activity assay and then an XTT reduction assay to screen the viability effect of UA on biofilms, finally fluorescence staining was used to determine biofilm integrity. In the same study, MIC (0.25 mg/mL) and MBC (0.25 mg/mL) values of UA against S.mutans were evaluated and then the effect of UA on biofilm was evaluated through the XTT reduction method. The bacterial viability within the biofilm decreased as the concentration of UA increased. To observe the direct effects of UA on biofilms, the live/dead bacterial viability kit was used to examine the activity of UA against S.mutans. The results confirm that UA can significantly destroy biofilms by affecting bacterial survival and adhesion. Recently, G. Liu et al. (2024), copiously examined the mechanism of action of UA, at different purity levels, extracted from R. officinalis. The bacteriostatic properties were assessed by calculating MIC values, while the synergistic effects by measuring the FICI index. UA exhibited antibacterial activity against S.aureus (MIC 39 μg/mL), S.dysgalactiae (MIC 19.5 μg/mL), S.agalactiae (MIC 156 μg/mL), E.faecalis (MIC 19.5 μg/mL), and S.mutans (MIC 9.75 μg/mL). However, it did not affect E.coli in contrast with what was previously reported by Ren et al. (2005) and Martins et al. (2011). Furthermore, in order to identify the possible mode of action they studied the impact of UA on S.aureus growth and a suppression of stationary phase growth was highlighted. Levels of biofilm formation were quantified using crystal violet staining, revealing that biofilm levels declined with increasing UA concentration. At the above MIC, UA significantly reduced the biofilm levels. SEM and TEM analysis were adopted to study the morphological changes caused by extracts, surface sinking, slurring of the boundary and disturbance of the uniformity of cytosol were identified after exposure at different concentrations. While UA and OA hold potentials as antimicrobial agents, careful consideration of the associated risks is crucial. Like many antimicrobial agents, there is a potential for microorganism to develop resistance to UA and OA. Continuous exposure can lead to adaptive changes in microbial population, thus combination therapies, rotational use of antibiotics and dosage optimization can mitigate these effects (Álvarez-Martínez et al., 2020). UA and OA can also manifest cytotoxicity to cells (i.e., HepG2 cell line). Pure synthetic mixtures of UA and OA at concentration of 32 μmol/L produced a decrease of approximately 35% on the cell viability, while natural mixture of UA and OA from leaves of Plumeria obtusa L. var. sericifolia at same concentration did not produce significant changes in cell viability (A. M. Silva et al., 2019). This increased toxicity is attributed both to the highest purity of synthetic mixtures and to the presence of other isomers with lower activity in natural mixtures. Conducting comprehensive in vitro and in vivo toxicity studies to establish the safety margins and guidelines will help address challenges associated with these compounds.
In conclusion, the exploration of UA and OA as natural triterpenoids with biological activities presents a compelling avenue for further research. These compounds, abundantly found in various common plants such as apples, olives, and numerous aromatic and medicinal herbs, offer a sustainable approach to harnessing their bioactive properties. The accessibility of UA and OA from natural sources underscores their potential for therapeutic applications and promotes the use of renewable resources in drug development. However, it is not yet clear the mechanism of action involved in the antimicrobial activity of these compounds. Variability in reported activities may be due to differences in test methods, compounds purity, reproducibility, precision and bacterial strain purity as stated by Jaki et al. (2008). They explore the variability of biological responses of UA from the perspective of sample purity and introduces the concept of purity-activity relationship (PARs) in natural product research. Results highlights that there is no correlation between biological activity and sample purity. Nonetheless, UA and OA have demonstrated significant potential in various therapeutic areas beyond their antimicrobial properties, including anti-inflammatory, anticancer, and cardioprotective effects. Their ability to modulate multiple biological pathways suggests they could be valuable in treating complex diseases that involve multiple targets. This multifaceted potential adds to their appeal in drug development. Moreover, the integration of UA and OA in adjuvant strategies represents a promising approach to combat biofilm-related challenges, antibiotic resistance, and persistent infections. Their role as adjuvants enhance the efficacy of existing antibiotics, potentially reducing the required dosage and minimizing side effects. This synergy is crucial in the fight against multidrug-resistant bacterial strains, providing a complementary approach to traditional antibiotics. Furthermore, techniques such as gene expression analysis and proteomics studies can provide insights into the mechanism of actions and facilitate the development of targeted therapies. Additionally, exploring the synergistic effects of UA and OA with other natural or synthetic compounds can unlock new therapeutic combinations, enhancing their effectiveness and broadening their applicability. Moreover, advanced drug delivery systems, including lipid-based delivery systems and micro/nano emulsions or nanoparticles, can be employed to overcome challenges such as poor solubility and limited bioavailability (Wasim and Bergonzi, 2024). In summary, UA and OA represent a promising frontier in natural product research, with the potential to contribute significantly to modern medicine. Their sustainable sourcing and multifaceted bioactivities align well with current trends toward more natural and eco-friendly therapeutic options. By addressing the current knowledge gaps and optimizing their use, UA and OA could become integral components of future therapeutic strategies.
CS: Conceptualization, Investigation, Writing–original draft. GA: Formal Analysis, Writing–review and editing. GC: Writing–review and editing.
The author(s) declare that no financial support was received for the research, authorship, and/or publication of this article.
The authors declare that the research was conducted in the absence of any commercial or financial relationships that could be construed as a potential conflict of interest.
All claims expressed in this article are solely those of the authors and do not necessarily represent those of their affiliated organizations, or those of the publisher, the editors and the reviewers. Any product that may be evaluated in this article, or claim that may be made by its manufacturer, is not guaranteed or endorsed by the publisher.
Al-Qudah, M. M. A. (2024). Antibacterial effect of Asphodelus fistulosus aqueous and ethanolic crude extracts on gram positive and gram negative bacteria. Braz. J. Biol. 84, e260029. doi:10.1590/1519-6984.260029
Álvarez-Martínez, F. J., Barrajón-Catalán, E., and Micol, V. (2020). Tackling antibiotic resistance with compounds of natural origin: a comprehensive review. Biomedicines 8 (10), 405. doi:10.3390/biomedicines8100405
Andre, C. M., Greenwood, J. M., Walker, E. G., Rassam, M., Sullivan, M., Evers, D., et al. (2012). Anti-inflammatory procyanidins and triterpenes in 109 apple varieties. J. Agric. Food Chem. 60 (42), 10546–10554. doi:10.1021/jf302809k
Atanasov, A. G., Zotchev, S. B., Dirsch, V. M., and Supuran, C. T. (2021). Natural products in drug discovery: advances and opportunities. Nat. Rev. Drug Discov. 20 (3), 200–216. doi:10.1038/s41573-020-00114-z
Becker, H., Scher, J. M., Speakman, J.-B., and Zapp, J. (2005). Bioactivity guided isolation of antimicrobial compounds from Lythrum salicaria. Fitoterapia 76 (6), 580–584. doi:10.1016/j.fitote.2005.04.011
Brehm-Stecher, B. F., and Johnson, E. A. (2003). Sensitization of Staphylococcus aureus and Escherichia coli to antibiotics by the sesquiterpenoids nerolidol, farnesol, bisabolol, and apritone. Antimicrob. Agents Chemother. 47 (10), 3357–3360. doi:10.1128/AAC.47.10.3357-3360.2003
Cao, J., and Su, E. (2021). Hydrophobic deep eutectic solvents: the new generation of green solvents for diversified and colorful applications in green chemistry. J. Clean. Prod. 314, 127965. doi:10.1016/j.jclepro.2021.127965
Catteau, L., Reichmann, N., Olson, J., Pinho, M., Nizet, V., Van Bambeke, F., et al. (2017). Synergy between ursolic and oleanolic acids from Vitellaria paradoxa leaf extract and β-lactams against methicillin-resistant Staphylococcus aureus: in vitro and in vivo activity and underlying mechanisms. Molecules 22 (12), 2245. doi:10.3390/molecules22122245
Do Nascimento, P., Lemos, T., Bizerra, A., Arriaga, Â., Ferreira, D., Santiago, G., et al. (2014). Antibacterial and antioxidant activities of ursolic acid and derivatives. Molecules 19 (1), 1317–1327. doi:10.3390/molecules19011317
Du, J., Fu, J., and Chen, T. (2024). Investigation of the antibacterial properties and mode of action of compounds from Urtica dioica L. Cureus 16, e52083. doi:10.7759/cureus.52083
Elmaidomy, A. H., Shady, N. H., Abdeljawad, K. M., Elzamkan, M. B., Helmy, H. H., Tarshan, E. A., et al. (2022). Antimicrobial potentials of natural products against multidrug resistance pathogens: a comprehensive review. RSC Adv. 12 (45), 29078–29102. doi:10.1039/D2RA04884A
Fan, J.-P., Liao, D.-D., and Zhang, X.-H. (2016). Ultrasonic assisted extraction of ursolic acid from apple pomace: a novel and facile technique. Sep. Sci. Technol. 51 (8), 1344–1350. doi:10.1080/01496395.2016.1165253
FooDB (2024). FooDB. Available at: https://foodb.ca/(Accessed May 9, 2024).
Grudniak, A. M., Kurek, A., Szarlak, J., and Wolska, K. I. (2011). Oleanolic and ursolic acids influence affect the expression of the cysteine regulon and the stress response in Escherichia coli. Curr. Microbiol. 62 (4), 1331–1336. doi:10.1007/s00284-010-9866-0
Hussain, H., Green, I. R., Ali, I., Khan, I. A., Ali, Z., Al-Sadi, A. M., et al. (2017). Ursolic acid derivatives for pharmaceutical use: a patent review (2012-2016). Expert Opin. Ther. Pat. 27 (9), 1061–1072. doi:10.1080/13543776.2017.1344219
Jaki, B. U., Franzblau, S. G., Chadwick, L. R., Lankin, D. C., Zhang, F., Wang, Y., et al. (2008). Purity−Activity relationships of natural products: the case of anti-TB active ursolic acid. J. Nat. Prod. 71 (10), 1742–1748. doi:10.1021/np800329j
Kurek, A., Nadkowska, P., Pliszka, S., and Wolska, K. I. (2012). Modulation of antibiotic resistance in bacterial pathogens by oleanolic acid and ursolic acid. Phytomedicine 19 (6), 515–519. doi:10.1016/j.phymed.2011.12.009
Kuroda, M., Nagasaki, S., and Ohta, T. (2007). Sesquiterpene farnesol inhibits recycling of the C55 lipid carrier of the murein monomer precursor contributing to increased susceptibility to β-lactams in methicillin-resistant Staphylococcus aureus. J. Antimicrob. Chemother. 59 (3), 425–432. doi:10.1093/jac/dkl519
Li, H., Liu, Y., Guo, S., Shi, M., Qin, S., and Zeng, C. (2023). Extraction of ursolic acid from apple peel with hydrophobic deep eutectic solvents: comparison between response surface methodology and artificial neural networks. Foods 12 (2), 310. doi:10.3390/foods12020310
Lira, W. D. M., Dos Santos, F. V., Sannomiya, M., Rodrigues, C. M., Vilegas, W., and Varanda, E. A. (2008). Modulatory effect of Byrsonima basiloba extracts on the mutagenicity of certain direct and indirect-acting mutagens in Salmonella typhimurium assays. J. Med. Food 11 (1), 111–119. doi:10.1089/jmf.2007.553
Liu, G., Qin, P., Cheng, X., Wu, L., Wang, R., and Gao, W. (2023). Ursolic acid: biological functions and application in animal husbandry. Front. Veterinary Sci. 10, 1251248. doi:10.3389/fvets.2023.1251248
Liu, G., Qin, P., Cheng, X., Wu, L., Zhao, W., and Gao, W. (2024). Evaluation of the mechanistic basis for the antibacterial activity of ursolic acid against Staphylococcus aureus. Front. Microbiol. 15, 1389242. doi:10.3389/fmicb.2024.1389242
Liu, Y., Huang, Y., Fan, C., Chi, Z., Bai, M., Sun, L., et al. (2021). Ursolic acid targets glucosyltransferase and inhibits its activity to prevent Streptococcus mutans biofilm formation. Front. Microbiol. 12, 743305. doi:10.3389/fmicb.2021.743305
López-Hortas, L., Pérez-Larrán, P., González-Muñoz, M. J., Falqué, E., and Domínguez, H. (2018). Recent developments on the extraction and application of ursolic acid. A review. Food Res. Int. 103, 130–149. doi:10.1016/j.foodres.2017.10.028
Ludeña Huaman, M. A., Tupa Quispe, A. L., Huamán Quispe, R. I., Serrano Flores, C. A., and Robles Caycho, J. (2021). A simple method to obtain ursolic acid. Results Chem. 3, 100144. doi:10.1016/j.rechem.2021.100144
Luong, T., Salabarria, A.-C., and Roach, D. R. (2020). Phage therapy in the resistance era: where do we stand and where are we going? Clin. Ther. 42 (9), 1659–1680. doi:10.1016/j.clinthera.2020.07.014
Maisto, M., Piccolo, V., Novellino, E., Schiano, E., Iannuzzo, F., Ciampaglia, R., et al. (2023). Optimization of ursolic acid extraction in oil from annurca apple to obtain oleolytes with potential cosmeceutical application. Antioxidants 12 (2), 224. doi:10.3390/antiox12020224
Manso, T., Lores, M., and De Miguel, T. (2021). Antimicrobial activity of polyphenols and natural polyphenolic extracts on clinical isolates. Antibiotics 11 (1), 46. doi:10.3390/antibiotics11010046
Martins, A., Vasas, A., Viveiros, M., Molnár, J., Hohmann, J., and Amaral, L. (2011). Antibacterial properties of compounds isolated from Carpobrotus edulis. Int. J. Antimicrob. Agents 37 (5), 438–444. doi:10.1016/j.ijantimicag.2011.01.016
Oluwatuyi, M., Kaatz, G., and Gibbons, S. (2004). Antibacterial and resistance modifying activity of. Phytochemistry 65 (24), 3249–3254. doi:10.1016/j.phytochem.2004.10.009
Othman, L., Sleiman, A., and Abdel-Massih, R. M. (2019). Antimicrobial activity of polyphenols and alkaloids in middle eastern plants. Front. Microbiol. 10, 911. doi:10.3389/fmicb.2019.00911
Park, S.-N., Ahn, S.-J., and Kook, J.-K. (2015). Oleanolic acid and ursolic acid inhibit peptidoglycan biosynthesis in Streptococcus mutans UA159. Braz. J. Microbiol. 46 (2), 613–617. doi:10.1590/S1517-838246246220130209
Petrochenko, A. A., Orlova, A., Frolova, N., Serebryakov, E. B., Soboleva, A., Flisyuk, E. V., et al. (2023). Natural deep eutectic solvents for the extraction of triterpene saponins from Aralia elata var. Mandshurica (rupr. and maxim.) J. Wen. Molecules 28 (8), 3614. doi:10.3390/molecules28083614
Ren, D., Zuo, R., González Barrios, A. F., Bedzyk, L. A., Eldridge, G. R., Pasmore, M. E., et al. (2005). Differential gene expression for investigation of Escherichia coli biofilm inhibition by plant extract ursolic acid. Appl. Environ. Microbiol. 71 (7), 4022–4034. doi:10.1128/AEM.71.7.4022-4034.2005
Sekandi, P., Namukobe, J., Byamukama, R., Nagawa, C. B., Barbini, S., Bacher, M., et al. (2023). Antimicrobial, antioxidant, and sun protection potential of the isolated compounds from Spermacoce princeae (K. Schum). BMC Complementary Med. Ther. 23 (1), 201. doi:10.1186/s12906-023-04026-4
Silva, A. M., Alvarado, H. L., Abrego, G., Martins-Gomes, C., Garduño-Ramirez, M. L., García, M. L., et al. (2019). In vitro cytotoxicity of oleanolic/ursolic acids-loaded in PLGA nanoparticles in different cell lines. Pharmaceutics 11 (8), 362. doi:10.3390/pharmaceutics11080362
Silva, N. H. C. S., Morais, E. S., Freire, C. S. R., Freire, M. G., and Silvestre, A. J. D. (2020). Extraction of high value triterpenic acids from Eucalyptus globulus biomass using hydrophobic deep eutectic solvents. Molecules 25 (1), 210. doi:10.3390/molecules25010210
Sycz, Z., Tichaczek-Goska, D., and Wojnicz, D. (2022). Anti-planktonic and anti-biofilm properties of pentacyclic triterpenes—asiatic acid and ursolic acid as promising antibacterial future pharmaceuticals. Biomolecules 12 (1), 98. doi:10.3390/biom12010098
Tostes, J. B. D. F., Nakamura, M. J., De Saboya, C. G. F., Mazzei, J. L., and Siani, A. C. (2016). Efficient and selective method to separate triterpene acids by direct treatment of apple peels with alkaline ethanol. Sep. Sci. Technol. 51 (12), 1986–1993. doi:10.1080/01496395.2016.1200088
Vaou, N., Stavropoulou, E., Voidarou, C., Tsigalou, C., and Bezirtzoglou, E. (2021). Towards advances in medicinal plant antimicrobial activity: a review study on challenges and future perspectives. Microorganisms 9 (10), 2041. doi:10.3390/microorganisms9102041
Villa, C., Caviglia, D., Robustelli Della Cuna, F. S., Zuccari, G., and Russo, E. (2024). NaDES application in cosmetic and pharmaceutical fields: an overview. Gels (Basel, Switz.) 10 (2), 107. doi:10.3390/gels10020107
Wasim, M., and Bergonzi, M. C. (2024). Unlocking the potential of oleanolic acid: integrating pharmacological insights and advancements in delivery systems. Pharmaceutics 16 (6), 692. doi:10.3390/pharmaceutics16060692
Wolska, K., Grudniak, A., Fiecek, B., Kraczkiewicz-Dowjat, A., and Kurek, A. (2010). Antibacterial activity of oleanolic and ursolic acids and their derivatives. Open Life Sci. 5 (5), 543–553. doi:10.2478/s11535-010-0045-x
Wrońska, N., Szlaur, M., Zawadzka, K., and Lisowska, K. (2022). The synergistic effect of triterpenoids and flavonoids—new approaches for treating bacterial infections? Molecules 27 (3), 847. doi:10.3390/molecules27030847
Keywords: pentacyclic triterpenoids, natural products, bioactive compounds, antibacterials, adjuvant strategy
Citation: Spaggiari C, Annunziato G and Costantino G (2024) Ursolic and oleanolic acids: two natural triterpenoids targeting antibacterial multidrug tolerance and biofilm formation. Front. Nat. Produc. 3:1456361. doi: 10.3389/fntpr.2024.1456361
Received: 28 June 2024; Accepted: 12 August 2024;
Published: 26 August 2024.
Edited by:
Julius Liobikas, Lithuanian University of Health Sciences, LithuaniaReviewed by:
Zunlai Sheng, Northeast Agricultural University, ChinaCopyright © 2024 Spaggiari, Annunziato and Costantino. This is an open-access article distributed under the terms of the Creative Commons Attribution License (CC BY). The use, distribution or reproduction in other forums is permitted, provided the original author(s) and the copyright owner(s) are credited and that the original publication in this journal is cited, in accordance with accepted academic practice. No use, distribution or reproduction is permitted which does not comply with these terms.
*Correspondence: Chiara Spaggiari, Y2hpYXJhLnNwYWdnaWFyaUB1bmlwci5pdA==
Disclaimer: All claims expressed in this article are solely those of the authors and do not necessarily represent those of their affiliated organizations, or those of the publisher, the editors and the reviewers. Any product that may be evaluated in this article or claim that may be made by its manufacturer is not guaranteed or endorsed by the publisher.
Research integrity at Frontiers
Learn more about the work of our research integrity team to safeguard the quality of each article we publish.