- 1Department of Pharmacognosy, Faculty of Pharmacy, Badr University in Cairo (BUC), Badr City, Cairo, Egypt
- 2Badr University in Cairo Research Center, Badr University in Cairo, Badr City, Cairo, Egypt
- 3Nanotechnology Research Center (NTRC), The British University in Egypt (BUE), El-Sherouk City, Cairo, Egypt
- 4Department of Pharmacognosy, Faculty of Pharmacy, Ain-Shams University, Abassia, Cairo, Egypt
- 5Research Center for Chinese Herbal Medicine and Graduate Institute of Health Industry Technology, College of Human Ecology, Chang Gung University of Science and Technology, Taoyuan, Taiwan
- 6Department of Chemical Engineering, Ming Chi University of Technology, New Taipei City, Taiwan
- 7Department of Anesthesiology, Chang Gung Memorial Hospital, Taoyuan, Taiwan
Bone metabolism is characterized by an interplay between the deposition of bone matrix and mineralization and the resorption process. Osteoporosis is a form of systemic metabolic bone condition that causes bone density to decline and its microarchitecture to deteriorate, increasing the risk of fracture owing to fragility. The underlying cause of this clinical disease lies in the imbalance in bone remodeling, in which bone resorption by osteoclasts predominates over bone creation by osteoblasts. Natural remedies have long been used to cure and prevent osteoporosis. Genus Sophora of the Fabaceae family comprises about 69 species that showed many pharmacological effects, including bone health preservation. The activity of Sophora sp. in maintaining bone health was attributed to its antioxidant, regenerative, and anti-inflammatory qualities. In this review, we focused on the therapeutic properties of the extracts and isolated compounds from the genus Sophora in maintaining bone health, with special emphasis on the management of osteoporosis.
1 Introduction
The genus Sophora, subfamily Faboideae, family Fabaceae, contains around 69 species according to World Flora Online Data 2022 (https://powo.science.kew.org/) accessed in November 2023. These plants of this genus are distributed in tropical and temperate regions (Aly et al., 2019a). The abundant chemical constituents and potent pharmacological properties of Sophora sp. contribute to its diverse medicinal properties (Abd-Alla et al., 2014; Aly et al., 2019b; Boozari et al., 2019). Sophora sp. exhibited many biological activities, including anti-osteoporosis activity (Aly et al., 2020a; 2020b; 2021a; Chen Y. et al., 2023b).
The most common type of bone metabolism disorder is osteoporosis, characterized by chronic loss of trabecular bone and heightened vulnerability to fractures (Föger-Samwald et al., 2020). Osteoporosis is classified into two main categories, namely, primary and secondary osteoporosis, based on its underlying causes. Primary osteoporosis primarily results from age-related factors and is commonly observed in individuals aged 50 and above. The most prevalent form of primary osteoporosis is postmenopausal osteoporosis (PMOP). Postmenopausal osteoporosis arises due to decreased estrogen production following menopause, leading to reduced bone mineral density (BMD). On the other hand, secondary osteoporosis is triggered by specific medications and medical conditions that result in a decline in BMD (Compston et al., 2019; Song et al., 2022).
The equilibrium between bone resorption, carried out by osteoclasts, and the formation of bone matrix, facilitated by osteoblasts, is meticulously regulated through a series of intricate and tightly controlled processes. These processes are crucial in maintaining mineral homeostasis and preserving bone mass (Kim et al., 2020). The imbalance between the formation and resorption of bones causes osteoporosis (Lei et al., 2023). Multiple transcription factors, including runt-related transcription factor 2 (Runx2) and osterix (Osx), along with essential developmental signals such as the Wingless-INT (WNT) signals, are recognized for their role in regulating osteoblast differentiation and function (Long, 2011). The differentiation and activity of osteoclasts are regulated by many factors, including cytokines, αVβ3 integrins, macrophage colony-stimulating factor (M-CSF), receptor activator of nuclear factor-κΒ ligand (RANKL), and its receptor RANK (Matsumoto and Endo, 2021; Song et al., 2022). A schema summarizing the cellular biology of osteoporosis is illustrated in Figure 1.
Natural alkaloids are currently used as anti-osteoporosis medications due to their high efficacy and low toxicity (Lin et al., 2022). Sophora alkaloids, including oxymatrine, matrine, sophocarpine, sophoridine, and aloperine, exhibited anti-osteoporosis effects through various pathways, particularly on the RANKL pathway. On the other hand, plant-derived flavonoids demonstrated protective effects against osteoporosis by promoting the survival, proliferation, and differentiation of cells involved in maintaining bone homeostasis (Bellavia et al., 2021). Sophora flavonoids and isoflavonoids, especially genistein, sophoricoside, sophorabioside, (2s)-2′-methoxykurarinone, 8-prenyl kaempferol, formononetin, maackiain, sophoraflavonoloside, nicotiflorin, and rutin showed anti-osteoporosis effects through several pathways. This review summarized Sophora’s isolated compound and prepared extracts from several species, which showed potential anti-osteoporosis efficacy. The discussed species of the genus Sophora include S. japonica L. (syn. Styphnolobium japonicum), S. flavescens var. flavescens, S. alopecuroides L., S. davidi var. davidi (Franch.) Skeels, S. secundiflora (Ortega) Lag. ex DC. (syn. Dermatophyllum secundiflorum) and S. tonkinensis var. tonkinensis Gagnep.
The literature covered in this review spanned over the time range of 2010–2023. Several sources such as PubMed (http://www.ncbi.nlm.nih.gov/pubmed/), SciFinder (), Google Scholar (http://scholar.google.com/), and Science Direct (http://www.sciencedirect.com/) were consulted to retrieve relevant information. Keywords in this study are Sophora extract, Sophora compounds, Osteoporosis, Bone, and treatment.
2 Reported isolated compounds from Sophora sp. with potential effect on bone health
2.1 Flavonoids and isoflavonoids
Flavonoids and isoflavonoids are naturally occurring polyphenolic compounds extracted from higher plants. They are essential to the human diet (El-Nashar et al., 2022). They provide a wide range of physiological and ecological activities (Wang et al., 2020). They are usually present as glycosides, which accumulate in plant cell vacuoles. Flavonoids are produced via the phenylpropanoid metabolic pathway and consist of three rings (C6–C3–C6) identified as A, B, and C, with a total of 15 carbon atoms (Santos et al., 2022). They are divided into seven subclasses based on their structural differences, including anthocyanidins, flavanones, flavones, isoflavones, flavonols, flavones, and chalcones (Manzoor et al., 2020). This classification is based on the degree of oxidation in the central heterocycle (Shen et al., 2022; Chen S. et al., 2023). Flavonoid glycosides can undergo various modifications, including glycosylation and acylation, at the sites where methyl and hydroxy groups link to the other two rings (Shen et al., 2022). The biosynthetic pathway of flavonoids occurs at the point of convergence of the shikimate pathway and the acetate pathway. The first can produce p-coumaroyl-CoA, whereas the second pathway is responsible for regulating the elongation of C2-chains (Li M. et al., 2022). They exhibit a wide range of pharmacological activities due to their anti-inflammatory, antioxidant, and anti-cancer effects, as well as their ability to stimulate the production of bones (Ramesh et al., 2021).
Genistein is a phytoestrogen that belongs to isoflavones. It possesses various pharmacological properties, including anti-inflammatory, anti-apoptotic, and anti-angiogenic effects (Nazari-Khanamiri and Ghasemnejad-Berenji, 2021). It is a tyrosine kinase inhibitor, which was reported to be dysregulated in the pathological development of certain pathways in osteoporosis, osteoarthritis, and intervertebral disc degeneration (IDD). Numerous signaling pathways, including MAPK, NF-κB, and NRF2/HO-1, contribute to genistein’s regulatory role in preventing bone and cartilage disorders (Wu and Liu, 2022). Genistein isolated from S. japonica exhibited an anti-osteoporosis effect similar to soybean genistein. The study showed that the administration of a large amount of genistein from S. japonica to OVX rats at a dose of 4.5 or 9 mg/kg could prevent osteoporosis through many aspects, including improving bone density, bone mineral components like calcium, phosphorus, and magnesium, trabecular thickness, trabecular area percentage, and trabecular number (Wang et al., 2006).
An isoflavone called sophoricoside (genistein-4′-β-D-glucoside) was extracted from S. japonica (Kim and Lee, 2021a; 2021b). It exhibited many pharmacological properties, including estrogenic activity, anti-inflammatory, antioxidant, anti-diabetic, and immunomodulatory effects (Patel et al., 2020). A study was conducted to evaluate the estrogenic proliferative activities of seven isolated compounds including genistin, sophoricoside, sophorabioside, sophoraflavonoloside, genistein 7,4ʹ-di-O-β-D-glucopyransoide, kaempferol 3-O-α–L-rhamnopyranosyl(1→6)β-D-glucopyranosyl(1→2)β-D-glucopyranoside, and rutin in the estrogen-dependent MCF-7 cell line. The findings of this study according to the profile of cytotoxicity, impact on membrane integrity, and estrogenic proliferative activity revealed that the most potent estrogenic compound was sophoricoside. Consequently, sophoricoside was chosen for additional in vivo study to investigate its anti-osteoporosis effect in comparison with estradiol as a positive control in ovariectomized rats in vivo at doses of 15 mg/kg and 30 mg/kg for 45 days. The results showed that sophoricoside increased the mechanical strength of bones. Additionally, it increased osteogenic biochemical markers such as serum alkaline phosphatase (ALP) and osteocalcin (OCN) levels and decreased acid phosphatase levels (Abdallah et al., 2014).
Kurarinone is a natural flavanone found in various plants. It exhibited several pharmacological properties, including chemoprevention, anticancer, antifungal, antibacterial, anti-corona virus, neuroprotective, antioxidant, and anti-inflammatory properties (Kumar et al., 2021). It is an active component of S. flavescens (Li Z. et al., 2022). Kim et al. isolated (2S)-2′-methoxykurarinone (MK) from S. flavescens roots, at a concentration of 20 μM. MK prevented mature osteoclastic bone resorption and RANKL-induced osteoclastogenesis of bone marrow macrophages (BMMs) in an in vitro study. The therapeutic effect was linked to the downregulation of Akt, p38, and JNK, as well as c-Fos and NFATc1, which decreased TRAP and OSCAR expression (Kim et al., 2014).
8-Prenylkaempferol (8-PK), a prenylated flavonoid extracted from S. flavescens, a Chinese herb with anti-inflammatory and antiviral effects (Chiou et al., 2011a). In MC3T3-E1 cells, 8-PK at different concentrations ranging from 1 to 20 μM promoted osteoblast development and maturation. The impact of 8-PK on cell maturation was mediated by boosting bone morphogenetic protein (BMP)-2 expression, phosphorylating Smad1/5/8 and p38, and promoting Runx2 nuclear translocation and transcription. 8-PK also increased ALP activity and bone nodule formation and upregulated the mRNA expressions of OCN, osteopontin (OPN), and type 1 collagen (Coll 1). As a result, 8-PK may be beneficial in boosting osteogenic activity, which is necessary for bone formation, however further in vivo and clinical studies are needed to evaluate the effect of this compound (Chiou et al., 2011b).
Formononetin (FORM) is a phytoestrogen isoflavone that demonstrated a wide range of physiological activities that benefit health via estrogen-dependent and independent pathways (Machado Dutra et al., 2021). FORM was isolated from S. secundiflora and S. flavescens (Jiang et al., 2019; Aly et al., 2021b). An in vivo study compared the therapeutic potency of three phytoestrogens, including genistein, daidzein, and FORM. In Sprague Dawley rats, they reported that FORM at doses of 1 and 10 mg/kg/day enhanced trabecular bone areas (TBAs) like genistein and 17ꞵ-estradiol (E2) by increasing the activity of ALP (Ha et al., 2010). The formononetin estrogenic efficacy may be attributed to equol the end product of the metabolic process of formononetin, which is characterized by a ketone group at C2 and a single bond between C1 and C8 that allows its higher binding affinity to ER. Also, the in vitro study utilizing ALP activity assays in Saos-2 cells showed that FORM significantly raised ALP activity to 33.0% ± 5.8% of the control group (p < 0.05) at a dosage of 1 × 10−4 mg/mL, indicating its potential to promote osteoblast proliferation (Ha et al., 2010). It’s worth noting that FORM LD50 value equals to 103.6 mg/kg/BW (Pingale and Gupta, 2023).
Maackiain is a naturally occurring isoflavonoid derived from S. flavescens that exhibits several pharmacological properties, including anti-adipogenic, anti-allergic, anti-tumor, and immunostimulant activities (Huh et al., 2020; Liu et al., 2020; Mladenova et al., 2022). It showed activity in osteoclast-related conditions at in vitro study using concentrations of 5 μmol/L to 40 μmol/L. It disrupted the F-actin belt structures in mature osteoclasts and inhibited RANKL-stimulated protein levels and nuclear factor of activated T cells 1 (NFATc1) transcriptional activity. Moreover, it downregulated bone resorption-related genes, including c-Fos, CTSK, Acp5, MMP9, and integrin β3. It also inhibited Ca2+ oscillation levels, which suppressed gene expression related to osteoclasts (Liu et al., 2020) Table 1; Figure 2. The chemical structures of the flavonoids and isoflavonoids of Sophora sp. with potential effects on bone health are illustrated in Figure 3.
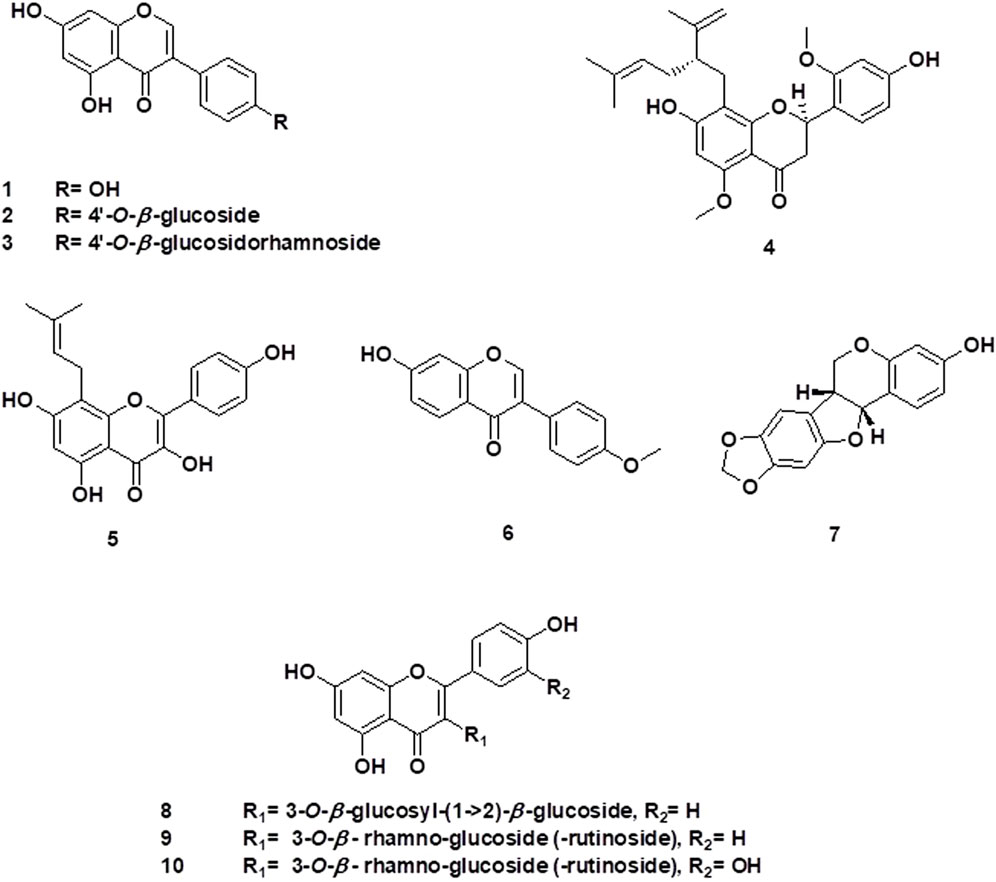
FIGURE 3. Chemical structures of the flavonoids and isoflavonoids of Sophora sp. with potential effects on bone health.
2.2 Alkaloids
Alkaloids are secondary metabolites that have been mainly found in plants. However, they have also been found in fungi and animals. This chemical class has more than 12,000 different structures (Schläger and Dräger, 2016). The essential characteristic of an alkaloid is the presence of a basic nitrogen atom (excluding nitrogen from an amide bond or peptide) in any molecular position (Gutiérrez-Grijalva et al., 2020). They are found in plants as powerful bioactive substances (Wang et al., 2022). They specifically have a variety of pharmacological properties, including anesthesia, cardioprotective, and anti-inflammatory effects. Morphine, strychnine, quinine, ephedrine, and nicotine are well-known alkaloids used in clinical practice (Heinrich et al., 2021). They support the development of mesenchymal stem cells, boost osteoblast proliferation, encourage osteoblast autophagy, and inhibit the growth of osteoclasts. Alkaloids could control several signaling pathways, including blocking the interaction of tumor necrosis factor receptor-associated factor 6 (TRAF6) and receptor activator of nuclear factor κb (RANK), blocking the nuclear factor kappa B (NF-κB) pathway in osteoclasts, activating the p38 mitogen-activated protein kinases pathway in osteoblasts, and initiating the wingless and int-1 pathways in mesenchymal stem cells (Lin et al., 2022). Quinolizidine alkaloids are the main active compounds of S. alopecuroides (Zhang et al., 2022). They are generally synthesized through a biosynthetic pathway involving lysine amino acid (Bunsupa et al., 2012a) as represented by lupinine-type, cytisine-type, sparteine-type, and marine-type alkaloids (Wang et al., 2019). Previous investigations provided evidence indicating that the starting point in the production of quinolizidine alkaloids involves the decarboxylation of L-lysine into cadaverine, catalyzed by the enzyme lysine decarboxylase followed by cyclization (Golebiewski and Spenser, 1988). Various structurally related alkaloids can be produced by alterations in the main skeleton of quinolizidine alkaloids through selective processes such as dehydrogenation, oxygenation, or esterification (Bunsupa et al., 2012b). Lu et al. reported the impact of total alkaloids derived from S. alopecuroides on the growth of osteosarcoma cells. They found that the S. alopecuroides alkaloids at dose of 1.5, 3, and 4.5 g/kg were able to restrict the growth of human osteosarcoma OS732 cells by inhibiting their growth rate 18.4%, 27.4% and 52.8%, respectively using MTT assay (Lu et al., 2014).
Oxymatrine (OMT) is a quinolizidine alkaloid isolated from Sophora medicinal plants. It belongs to the matrine-type alkaloids. Previous research articles showed that OMT exhibited various pharmacological properties, including anti-inflammatory, anti-viral, anti-cancer, and anti-diabetic properties. It showed protective effects on the skin, bone, renal, vascular, gastrointestinal, liver, heart, and lung organs (Huan et al., 2023). OMT extracted from S. flavescens in an in vitro study using at concentrations of 0, 100, 200, and 400 μM suppressed sterol regulatory element-binding protein 2 (SREBP2) activation and the expression of downstream NFATc1 during osteoclastogenesis by lowering ROS levelsIt also suppressed RANKL-induced osteoclast-specific gene expression, which includes the c-fos, Nfatc1, Ctsk, Trap, Atp6v0d2, and Mmp9 genes. While OMT treatment suppressed the expression of these genes, RANKL upregulated them. OMT also stopped ovariectomy (OVX)-induced osteoporosis in vivo at dose of 10 mg/kg. Therefore, OMT may eventually prove to be a valuable medication for the treatment of osteoporosis (Jiang et al., 2021).
Matrine is a quinolizidine alkaloid extracted from S. alopecuroides, S. tonkinensis var. tonkinensis, and S. flavescens (Dai et al., 2021; Liu N. et al., 2022). It showed various pharmacological effects, including anti-inflammatory, anti-tumor, and antiviral activities (Zhang et al., 2019; Luo et al., 2021; Liu J. et al., 2022). It demonstrated anti-fibrotic action by inhibiting the TGF-β/Smad pathway in liver, cardiac, and pancreatic fibrosis (Tan et al., 2023). Additionally, Mao et al. showed that inhibiting the TGF-β/Smad pathway is a possible treatment for heterotopic ossification (HO) by inducing mesenchymal stem cell (MSC) migration and osteogenic differentiation (Mao et al., 2020). Matrine may act as a novel osteoclastogenesis inhibitor at a dose of 150 mg/kg/d by suppressing numerous signaling pathways. It can suppress RANKL-induced activation of NF-κB, MAPK, and AKT pathways and NFATc1 expression in osteoclasts. As a result, it suppressed ERK, JNK, P38, and C-fos phosphorylation in the MAPK pathway, as well as inhibited AKT activation, resulting in lower expression of osteoclastogenesis-related markers such as MMP-9, TRAP, C-Src, and cathepsin K. It reduced serum levels of TRAcp5b, TNF-α, and IL-6, which suppressed osteoclastogenesis and prevented ovariectomy-induced bone loss. These effects indicated that matrine reduced bone loss in OVX mice by reducing osteoclastogenesis rather than increasing osteogenesis (Chen et al., 2017).
Another quinolizidine alkaloid is sophoridine, which is found in many traditional Chinese plants such as S. davidi var. davidi, S. japonica L., S. flavescens Alt., and S. alopecuroides L. (Tang et al., 2022). It showed various pharmacological actions such as anticancer, hepatoprotective, myocardial protective, antiviral, and anti-inflammatory effects (Wang et al., 2022). It exhibited antiosteoporosis and anti-osteoclastogenic effects in vivo at a dose of 15 mg/kg. Its anti-osteoporosis was achieved through the inhibition of osteoclast production and the suppression of anti-osteoclastogenic effect by reducing RANKL-induced activation of ERK and c-Fos and subsequently decreasing the expression of NFATc1, the most crucial factor in controlling osteoclastogenesis. It also reduced the level of the osteoclastogenesis marker CTX-1 rather than the osteogenesis marker OCN, indicating that sophoridine only inhibited osteoclastogenesis to tackle osteoporosis (Zhao et al., 2017).
Aloperine is a quinolizidine alkaloid found in the leaves and seeds of the medicinal plant S. alopecuroides L. (Zhou et al., 2020). Due to its potent anti-inflammatory, antioxidant, antibacterial, and antiviral effects, it has been used as a herbal medicine in China for centuries. It was established that aloperine is a potent therapeutic agent for various pathological diseases, including viral infections, cardiovascular and inflammatory disorders, and cancer (Tahir et al., 2022). Without influencing the activity of BMMs, In an in vitro investigation, aloperine was found to inhibit osteoclast activity and formation induced by RANKL via decreasing the ERK, JNK, and NF-κB pathways. This effect was observed at various concentrations of 10, 20, 40, and 50 μM. Additionally, it inhibited the expression of genes related to osteoclasts, such as matrix metallopeptidase 9 (MMP9), cathepsin K (Ctsk), NFATc1, tartrate-resistant acid phosphatase (TRAcP), V-ATPase d2, and calcitonin receptor. It prevented the phosphorylation of P65, ERK, and JNK and IκBα degradation. At a dosage of 30 mg/kg in vivo, it effectively decreased osteoclast activity and mitigated bone loss in OVX mice (Hu et al., 2021).
Alkaloids from S. flavescens (ASF) showed potent anti-inflammatory and proliferative effects on Staphylococcus aureus infected rat calvarial osteoblasts (ROBs) treated or untreated with vancomycin through increased viability of ROBs. These results might be obtained by controlling the mRNA and protein expression of BMP2, Runx2, OPG, and RANKL, reducing the secretion of the inflammatory factor TNF-α, and boosting ALP activity. ASF also reduced TRAP activity and osteoclast viability. As a result, when combined with an antibiotic, ASF might be a potential supplementary herbal treatment for chronic osteomyelitis (Wang et al., 2018), as depicted in Table 2 and Figure 4.
Figure 5 illustrates the chemical structures of the alkaloids of Sophora sp. with potential effects on bone health.
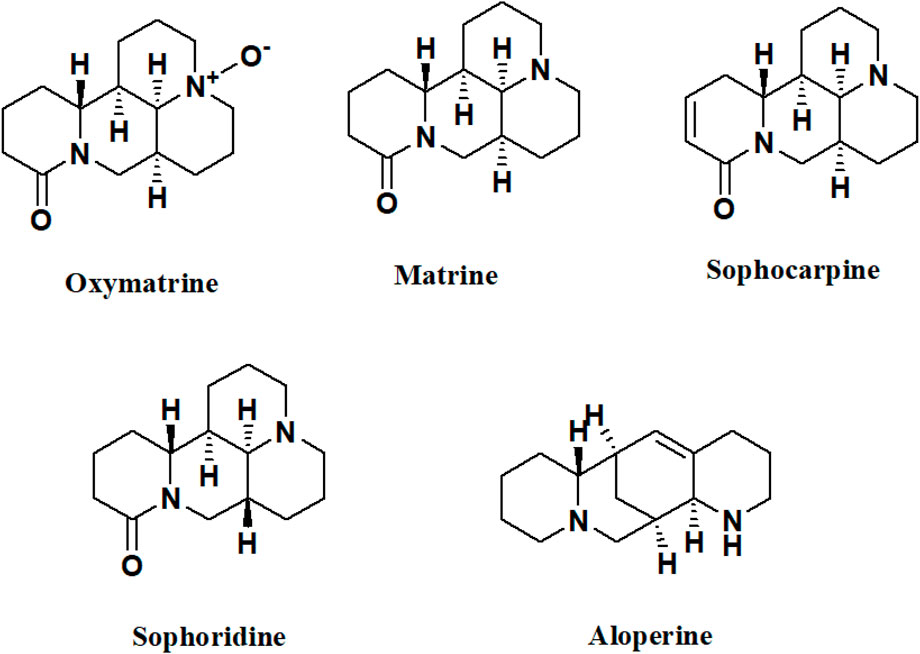
FIGURE 5. Chemical structures of the alkaloids of Sophora sp. with potential effects on bone health.
2.3 Sophora extracts
Sophora pachycarpa root extract (SPRE), acts as a stimulant for bone-formation in human adipose-derived mesenchymal stem cells. In vitro, SPRE demonstrated a significant increase in mineralization, ALP activity, and mRNA expression of bone gamma-carboxyglutamate protein (BGLAP), RUNX2, secreted phosphoprotein 1 (SPP1), and collagen type I alpha 1 (COL1A1) at different concentrations of 0.1, 1, 5, and 10 μg/mL (Mollazadeh et al., 2017).
The flowers and buds of S. japonica are called Sophorae Flos (SF) (Shi et al., 2023). The SF showed anti-obesity, anti-allergic, antiproliferative, and anti-inflammatory effects. Kim et al. found that SF extract showed inhibitory effects on IκBα phosphorylation in vitro, this resulted in a reduction of the NF-κB pathway activation induced by RANKL at different concentrations (0, 50, 100, 200 μg/mL). As a result, NFATc1 was downregulated, and the differentiation of osteoclasts brought on by RANKL was inhibited. These results demonstrated that SFE might be a potent candidate for managing inflammatory bone disorders, including osteoporosis, rheumatoid arthritis, and periodontitis (Kim et al., 2017).
The administration of Sophorae fructus extract at a dose of 0.556 g/kg/day in OVX rats decreased serum Ca and TBA and increased the levels of Dpd. These findings showed that this extract efficiently prevented bone loss and suggested that Sophorae fructus might be developed as a potential agent in preventing and treating osteoporosis (Shim et al., 2005).
The mature fruit of S. japonica L. was extracted using ethanol, hexane, dichloromethane (DCM), ethyl acetate, and butanol, which were investigated for their effects on the differentiation of C3H10T1/2 cells into osteoblasts at 10, 25, 50, and 100 μg/mL. Among the studied extracts, the in vitro study revealed that the DCM extracts showed the most potent pro-osteogenic activity. The DCM extract significantly increased early osteoblast marker ALP activity compared with the tested extracts. The DCM fractions contained significant quantities of genistein. The expression of estrogen target genes was also altered similarly to the effect of genistein and DCM fractions, and both substances were active in transfection tests that assessed estrogen agonistic activity (Yoon et al., 2013). The impact of Sophora extracts on bone health is presented in Table 3.
3 Conclusion
This review focused on the genus Sophora derived secondary metabolites and extracts in managing bone diseases, especially osteoporosis. We highlighted the reported flavonoids, isoflavonoids, and alkaloids from different Sophora species with potential roles in bone repair and maintenance of bone health. We explored their mechanisms of action that would potentially demonstrate their clinical efficacy. The mechanism of Sophora flavonoids and isoflavonoids is based on enhancing the mechanical strength of bones and a significant increase in osteogenic biochemical markers such as serum ALP, OCN levels, and BMP-2 expression. Sophora species increased bone mineral components such as calcium, phosphorus, and magnesium. On the other hand, the reduction of Ca2+ oscillation levels further suppressed the expression of genes associated with osteoclasts. Sophora alkaloids facilitated the growth of mesenchymal stem cells, enhanced the proliferation of osteoblasts, promoted osteoblast autophagy, and hindered the proliferation of osteoclasts. These investigations can potentially drive research toward developing new bone health medications derived from the Sophora genus. Furthermore, synthesizing more potent derivatives and investigating their mechanisms of action, exploring their pharmacokinetics and clinical efficiency. There is a lack of clinical trials in the existing literature that investigate the effectiveness and pharmacological effects of Sophora in alleviating osteoporosis. A comprehensive research framework, including clinical trials and preclinical evaluations, is essential for validating the therapeutic efficacy of Sophora extracts and isolated compounds in the management of osteoporosis that can derive research in producing more potent therapeutic agents tackling bone diseases.
Author contributions
SA: Conceptualization, Data curation, Resources, Visualization, Writing–original draft. AE: Visualization, Investigation, Resources, Writing–original draft. ME-S: Project administration, Supervision, Validation, Writing–review and editing. T-LH: Project administration, Supervision, Validation, Writing–review and editing.
Funding
The author(s) declare that no financial support was received for the research, authorship, and/or publication of this article.
Conflict of interest
The authors declare that the research was conducted in the absence of any commercial or financial relationships that could be construed as a potential conflict of interest.
The author(s) declared that they were an editorial board member of Frontiers, at the time of submission. This had no impact on the peer review process and the final decision.
Publisher’s note
All claims expressed in this article are solely those of the authors and do not necessarily represent those of their affiliated organizations, or those of the publisher, the editors and the reviewers. Any product that may be evaluated in this article, or claim that may be made by its manufacturer, is not guaranteed or endorsed by the publisher.
References
Abdallah, H. M., Al-Abd, A. M., Asaad, G. F., Abdel-Naim, A. B., and El-halawany, A. M. (2014). Isolation of antiosteoporotic compounds from seeds of Sophora japonica. PLoS One 9, e98559. doi:10.1371/journal.pone.0098559
Aly, S., Elissawy, A., Eldahshan, O., Elshanawany, M., and Singab, A. N. (2019a). Morphological and genetic characteristics of Sophora secundiflora and Sophora tomentosa (Fabaceae) cultivated in Egypt. Taeckholmia 39, 103–129. doi:10.21608/taec.2020.20572.1010
Aly, S. H., Elissawy, A. M., Allam, A. E., Farag, S. M., Eldahshan, O. A., Elshanawany, M. A., et al. (2021a). New quinolizidine alkaloid and insecticidal activity of Sophora secundiflora and Sophora tomentosa against Culex pipiens (Diptera: Culicidae). Nat. Prod. Res. 36 (11), 2722–2734. doi:10.1080/14786419.2021.1919108
Aly, S. H., Elissawy, A. M., Eldahshan, O. A., Elshanawany, M. A., Nasser, A., and Singab, B. (2020a). Phytochemical investigation using GC/MS analysis and evaluation of antimicrobial and cytotoxic activities of the lipoidal matter of leaves of Sophora secundiflora and Sophora, Arch. Pharm. Sci. Ain shams univ. 4, 207–214. doi:10.21608/APS.2020.38371.1039
Aly, S. H., Elissawy, A. M., Eldahshan, O. A., Elshanawany, M. A., and Singab, A. N. B. (2020b). Variability of the chemical composition of the essential oils of flowers and the alkaloid contents of leaves of Sophora secundiflora and Sophora tomentosa. J. Essent. Oil-Bear. Plants 23 (3), 442–452. doi:10.1080/0972060X.2020.1750489
Aly, S. H., Elissawy, A. M., Fayez, A. M., Eldahshan, O. A., Elshanawany, M. A., and Singab, A. N. B. (2021b). Neuroprotective effects of Sophora secundiflora, Sophora tomentosa leaves and formononetin on scopolamine-induced dementia. Nat. Prod. Res. 35, 5848–5852. doi:10.1080/14786419.2020.1795853
Aly, S. H., Elissawy, A. M., Mahmoud, A. M. A., El-Tokhy, F. S., Mageed, S. S. A., Almahli, H., et al. (2023a). Synergistic effect of Sophora japonica and Glycyrrhiza glabra flavonoid-rich fractions on wound healing: in vivo and molecular docking studies. Molecules 28, 2994. doi:10.3390/molecules28072994
Aly, S. H., Elissawy, A. M., Mahmoud, A. M. A., El-Tokhy, F. S., Mageed, S. S. A., Almahli, H., et al. (2023b). Synergistic effect of Sophora japonica and Glycyrrhiza glabra flavonoid-rich fractions on wound healing: in vivo and molecular docking studies. Molecules 28, 2994. doi:10.3390/molecules28072994
Bellavia, D., Dimarco, E., Costa, V., Carina, V., De Luca, A., Raimondi, L., et al. (2021). Flavonoids in bone erosive diseases: perspectives in osteoporosis treatment. Trends Endocrinol. Metab. 32, 76–94. doi:10.1016/J.TEM.2020.11.007
Boozari, M., Soltani, S., and Iranshahi, M. (2019). Biologically active prenylated flavonoids from the genus Sophora and their structure–activity relationship—a review. Phytother. Res. 33, 546–560. doi:10.1002/PTR.6265
Bunsupa, S., Katayama, K., Ikeura, E., Oikawa, A., Toyooka, K., Saito, K., et al. (2012a). Lysine decarboxylase catalyzes the first step of quinolizidine alkaloid biosynthesis and coevolved with alkaloid production in leguminosae. Plant Cell 24, 1202–1216. doi:10.1105/tpc.112.095885
Bunsupa, S., Yamazaki, M., and Saito, K. (2012b). Quinolizidine alkaloid biosynthesis: recent advances and future prospects. Front. Plant Sci. 3, 239. doi:10.3389/fpls.2012.00239
Chen, S., Wang, X., Cheng, Y., Gao, H., and Chen, X. (2023a). A review of classification, biosynthesis, biological activities and potential applications of flavonoids. Molecules 28, 4982. doi:10.3390/molecules28134982
Chen, X., Zhi, X., Pan, P., Cui, J., Cao, L., Weng, W., et al. (2017). Matrine prevents bone loss in ovariectomized mice by inhibiting RANKL-induced osteoclastogenesis. FASEB J. 31, 4855–4865. doi:10.1096/fj.201700316R
Chen, Y., Wang, X., Ye, D., Yang, Z., Shen, Q., Liu, X., et al. (2023b). Research progress of sophoridine’s pharmacological activities and its molecular mechanism: an updated review. Front. Pharmacol. 14, 1126636. doi:10.3389/fphar.2023.1126636
Chiou, W. F., Chen, C. C., and Wei, B. L. (2011a). 8-Prenylkaempferol suppresses influenza A virus-induced rantes production in A549 cells via blocking PI3K-mediated transcriptional activation of NF-κb and IRF3. Evid. Based Complement. Altern. Med. 920828, 1–10. doi:10.1093/ecam/nep066
Chiou, W. F., Lee, C. H., Liao, J. F., and Chen, C. C. (2011b). 8-Prenylkaempferol accelerates osteoblast maturation through bone morphogenetic protein-2/P38 pathway to activate Runx2 transcription. Life Sci. 88, 335–342. doi:10.1016/j.lfs.2010.12.009
Compston, J. E., McClung, M. R., and Leslie, W. D. (2019). Osteoporosis. Lancet. 393, 364–376. doi:10.1016/S0140-6736(18)32112-3
Dai, M., Chen, N., Li, J., Tan, L., Li, X., Wen, J., et al. (2021). In vitro and in vivo anti-metastatic effect of the alkaloid matrine from Sophora flavecens on hepatocellular carcinoma and its mechanisms. Phytomedicine 87, 153580. doi:10.1016/j.phymed.2021.153580
El-Nashar, H. A. S., Aly, S. H., Ahmadi, A., and El-Shazly, M. (2022). The impact of polyphenolics in the management of breast cancer: mechanistic aspects and recent patents. Recent Pat. Anticancer Drug Discov. 17, 358–379. doi:10.2174/1574892816666211213090623
Föger-Samwald, U., Dovjak, P., Azizi-Semrad, U., Kerschan-Schindl, K., and Pietschmann, P. (2020). Osteoporosis: pathophysiology and therapeutic options. EXCLI J. 19, 1017–1037. doi:10.17179/EXCLI2020-2591
Fu, Y., Zhang, H., Zhou, W., Lai, Z., and Dong, Y.-F. (2023). The protective effects of sophocarpine on sepsis-induced cardiomyopathy. Eur. J. Pharmacol. 950, 175745. doi:10.1016/j.ejphar.2023.175745
Golebiewski, W. M., and Spenser, I. D. (1988). Biosynthesis of the lupine alkaloids. II. Sparteine and lupanine. Can. J. Chem. 66, 1734–1748. doi:10.1139/v88-280
Gutiérrez-Grijalva, E. P., López-Martínez, L. X., Contreras-Angulo, L. A., Elizalde-Romero, C. A., and Heredia, J. B. (2020). “Plant alkaloids: structures and bioactive properties,” in Plant-derived bioactives: chemistry and mode of action. Editor M. K. Swamy (Singapore: Springer Singapore), 85–117. doi:10.1007/978-981-15-2361-8_5
Ha, H., Lee, H. Y., Lee, J. H., Jung, D., Choi, J., Song, K. Y., et al. (2010). Formononetin prevents ovariectomy-induced bone loss in rats. Arch. Pharm. Res. 33, 625–632. doi:10.1007/s12272-010-0418-8
Heinrich, M., Mah, J., and Amirkia, V. (2021). Alkaloids used as medicines: structural phytochemistry meets biodiversity—an update and forward look. Molecules 26, 1836. doi:10.3390/molecules26071836
Hu, R., Chen, L., Chen, X., Xie, Z., Xia, C., and Chen, Y. (2021). Aloperine improves osteoporosis in ovariectomized mice by inhibiting RANKL-induced NF-κb, ERK and JNK approaches. Int. Immunopharmacol. 97, 107720. doi:10.1016/j.intimp.2021.107720
Huan, D. Q., Hop, N. Q., and Son, N. T. (2023). Oxymatrine: a current overview of its health benefits. Fitoterapia 168, 105565. doi:10.1016/j.fitote.2023.105565
Huh, J., Lee, J., Jeon, E., Ryu, H. W., Oh, S., Ahn, K., et al. (2020). Maackiain, A compound derived from Sophora flavescens, increases IL-1β production by amplifying nigericin-mediated inflammasome activation. FEBS Open Bio 10, 1482–1491. doi:10.1002/2211-5463.12899
Jiang, C., Ma, Q., Wang, S., Shen, Y., Qin, A., Fan, S., et al. (2021). Oxymatrine attenuates osteoclastogenesis via modulation of ROS-mediated SREBP2 signaling and counteracts ovariectomy-induced osteoporosis. Front. Cell Dev. Biol. 9, 684007. doi:10.3389/fcell.2021.684007
Jiang, D., Rasul, A., Batool, R., Sarfraz, I., Hussain, G., Mateen Tahir, M., et al. (2019). Potential anticancer properties and mechanisms of action of formononetin. Biomed. Res. Int. 5854315, 1–11. doi:10.1155/2019/5854315
Kim, B. H., and Lee, S. (2021a). Sophoricoside from Sophora japonica ameliorates allergic asthma by preventing mast cell activation and CD4+ T cell differentiation in ovalbumin-induced mice. Biomed. Pharmacother. 133, 111029. doi:10.1016/j.biopha.2020.111029
Kim, B. H., and Lee, S. (2021b). Sophoricoside from Styphnolobium japonicum improves experimental atopic dermatitis in mice. Phytomedicine 82, 153463. doi:10.1016/j.phymed.2021.153463
Kim, J. M., Lee, J. H., Lee, G. S., Noh, E., Song, H. K., Gu, D. R., et al. (2017). Sophorae Flos extract inhibits RANKL-induced osteoclast differentiation by suppressing the NF-Κb/Nfatc1 pathway in mouse bone marrow cells. BMC Complement. Altern. Med. 17, 164. doi:10.1186/s12906-016-1550-x
Kim, J. M., Lin, C., Stavre, Z., Greenblatt, M. B., and Shim, J. H. (2020). Osteoblast-osteoclast communication and bone homeostasis. Cells 9, 2073. doi:10.3390/cells9092073
Kim, J.-Y., Kim, J. Y., Kim, J. J., Oh, J., Kim, Y.-C., and Lee, M. S. (2014). (2S)-2′-Methoxykurarinone inhibits osteoclastogenesis and bone resorption through down-regulation of RANKL signaling. Biol. Pharm. Bull. 37, 255–261. doi:10.1248/bpb.b13-00695
Kumar, S., Prajapati, K. S., Shuaib, M., Kushwaha, P. P., Tuli, H. S., and Singh, A. K. (2021). Five-decade update on chemopreventive and other pharmacological potential of kurarinone: a natural flavanone. Front. Pharmacol. 12, 737137. doi:10.3389/fphar.2021.737137
Lei, C., Song, J., Li, S., Zhu, Y., Liu, M., Wan, M., et al. (2023). Advances in materials-based therapeutic strategies against osteoporosis. Biomater 296, 122066. doi:10.1016/J.biomaterials.2023.122066
Li, M., Guo, L., Wang, Y., Li, Y., Jiang, X., Liu, Y., et al. (2022a). Molecular and biochemical characterization of two 4-coumarate: coa ligase genes in tea plant (Camellia sinensis). Plant Mol. Biol. 109, 579–593. doi:10.1007/s11103-022-01269-6
Li, Z., Lin, M., Li, Y., Shao, J., Huang, R., Qiu, Y., et al. (2022b). Total flavonoids of Sophora flavescens and kurarinone ameliorated ulcerative colitis by regulating Th17/treg cell homeostasis. J. Ethnopharmacol. 297, 115500. doi:10.1016/j.jep.2022.115500
Lin, B., Xu, P., Zheng, J., Deng, X., Ye, Q., Huang, Z., et al. (2022). Effects and mechanisms of natural alkaloids for prevention and treatment of osteoporosis. Front. Pharmacol. 13, 1014173. doi:10.3389/fphar.2022.1014173
Liu, J., Chen, C., Du, H., Wang, D., Ma, H., Wang, G., et al. (2022a). The antiviral effect and potential mechanism of matrine against white spot syndrome virus infection in crayfish (Procambarus clarkii). Aquac 561, 738662. doi:10.1016/j.aquaculture.2022.738662
Liu, N., Yang, C., Yang, L., Li, T., Gong, M., Wang, H., et al. (2022b). Matrine induces autophagy in human neuroblastoma cells via blocking the AKT-mtor pathway. Med. Oncol. 39, 167. doi:10.1007/s12032-022-01762-4
Liu, Y., Zeng, W., Ma, C., Wang, Z., Wang, C., Li, S., et al. (2020). Maackiain dampens osteoclastogenesis via attenuating RANKL-stimulated NF-κb signalling pathway and Nfatc1 activity. J. Cell Mol. Med. 24, 12308–12317. doi:10.1111/jcmm.15647
Long, F. (2011). Building strong bones: molecular regulation of the osteoblast lineage. Nat. Rev. Mol. Cell Biol. 13 (1), 27–38. doi:10.1038/nrm3254
Lu, X., Lin, B., Tang, J. G., Cao, Z., and Hu, Y. (2014). Study on the inhibitory effect of total alkaloids of Sophora alopecuroides on osteosarcoma cell growth. Afr. J. Tradit. Complement. Altern. Med. 11, 172–175. doi:10.4314/ajtcam.v11i1.27
Luo, D., Chen, N. H., Wang, W. Z., Zhang, J. H., Li, C. J., Zhuo, X. F., et al. (2021). Structurally diverse matrine-based alkaloids with anti-inflammatory effects from Sophora alopecuroides. Chin. J. Chem. 39, 3339–3346. doi:10.1002/cjoc.202100526
Machado Dutra, J., Espitia, P. J. P., and Andrade Batista, R. (2021). Formononetin: biological effects and uses – a review. Food Chem. 359, 129975. doi:10.1016/j.foodchem.2021.129975
Manzoor, A., Dar, I. H., Bhat, S. A., and Ahmad, S. (2020). “Flavonoids: health benefits and their potential use in food systems,” in Functional food products and sustainable health. Editors S. Ahmad, and N. A. Al-Shabib (Singapore: Springer Singapore), 235–256. doi:10.1007/978-981-15-4716-4_15
Mao, D., Pan, X., Rui, Y., and Li, F. (2020). Matrine attenuates heterotopic ossification by suppressing TGF-Β induced mesenchymal stromal cell migration and osteogenic differentiation. Biomed. Pharmacother. 127, 110152. doi:10.1016/j.biopha.2020.110152
Matsumoto, T., and Endo, I. (2021). RANKL as a target for the treatment of osteoporosis. J. Bone Min. Metab. 39 (1), 91–105. doi:10.1007/s00774-020-01153-7
Mladenova, S. G., Savova, M. S., Marchev, A. S., Ferrante, C., Orlando, G., Wabitsch, M., et al. (2022). Anti-adipogenic activity of maackiain and ononin is mediated via inhibition of pparγ in human adipocytes. Biomed. Pharmacother. 149, 112908. doi:10.1016/j.biopha.2022.112908
Mollazadeh, S., Neshati, V., Fazly Bazzaz, B. S., Iranshahi, M., Mojarrad, M., Naderi-Meshkin, H., et al. (2017). Standardized Sophora pachycarpa root extract enhances osteogenic differentiation in adipose-derived human mesenchymal stem cells. Phytother. Res. 31, 792–800. doi:10.1002/ptr.5803
Nazari-Khanamiri, F., and Ghasemnejad-Berenji, M. (2021). Cellular and molecular mechanisms of genistein in prevention and treatment of diseases: an overview. J. Food Biochem. 45, e13972. doi:10.1111/jfbc.13972
Patel, K., Husain, G. M., Katiyar, D. K., Prasad, S. K., and Patel, D. K. (2020). Sophoricoside: bioactive compounds from Sophora japonica, their role in disease prevention and treatment. Curr. Tradit. Med. 7, 180–188. doi:10.2174/2215083806666200214114106
Pingale, T. D., and Gupta, G. L. (2023). Acute and sub-acute toxicity study reveals No dentrimental effect of formononetin in mice upon repeated ip dosing. Toxicol. Mech. Methods. 33 (8), 688–697. doi:10.1080/15376516.2023.2234026
Ramesh, P., Jagadeesan, R., Sekaran, S., Dhanasekaran, A., and Vimalraj, S. (2021). Flavonoids: classification, function, and molecular mechanisms involved in bone remodelling. Front. Endocrinol. (Lausanne) 12, 779638. doi:10.3389/fendo.2021.779638
Santos, dos, Silva, da, Velasco, G., Pereira do Nascimento, R., Lino dos Santos, B., Alves Oliveira Amparo, J., et al. (2022). Neuroimmunomodulatory properties of flavonoids and derivates: a potential action as adjuvants for the treatment of glioblastoma. Pharmaceutics 14 (1), 116. doi:10.3390/pharmaceutics14010116
Schläger, S., and Dräger, B. (2016). Exploiting plant alkaloids. Curr. Opin. Biotechnol. 37, 155–164. doi:10.1016/j.copbio.2015.12.003
Shen, N., Wang, T., Gan, Q., Liu, S., Wang, L., and Jin, B. (2022). Plant flavonoids: classification, distribution, biosynthesis, and antioxidant activity. Food Chem. 383, 132531. doi:10.1016/j.foodchem.2022.132531
Shi, P., Liao, J., Duan, T., Wu, Q., Huang, X., Pei, X., et al. (2023). Chemical composition and pharmacological properties of Flos Sophorae immaturus, Flos Sophorae and fructus Sophorae: a review. J. Future Foods 3, 330–339. doi:10.1016/j.jfutfo.2023.03.004
Shim, J. G., Yeom, H., Kim, H. J., Choi, Y. W., Lee, I., Song, K. Y., et al. (2005). Bone loss preventing effect of Sophorae fructus on ovariectomized rats. Arch. Pharm. Res. 28, 106–110. doi:10.1007/BF02975144
Song, S., Guo, Y., Yang, Y., and Fu, D. (2022). Advances in pathogenesis and therapeutic strategies for osteoporosis. Pharmacol. Ther. 237, 108168. doi:10.1016/J.PHARMTHERA.2022.108168
Tahir, M., Ali, S., Zhang, W., Lv, B., Qiu, W., and Wang, J. (2022). Aloperine: a potent modulator of crucial biological mechanisms in multiple diseases. Biomedicines 10 (4), 905. doi:10.3390/biomedicines10040905
Tan, X., Hao, Y., Ma, N., Yang, Y., Jin, W., Meng, Y., et al. (2023). M6P-Modified solid lipid nanoparticles loaded with matrine for the treatment of fibrotic liver. Drug Deliv. 30 (1), 2219432. doi:10.1080/10717544.2023.2219432
Tang, Q., Liu, Y., Peng, X., Wang, B., Luan, F., and Zeng, N. (2022). Research progress in the pharmacological activities, toxicities, and pharmacokinetics of sophoridine and its derivatives. Drug Des. devel. Ther. 16, 191–212. doi:10.2147/DDDT.S339555
Wang, H., Xia, C., Chen, L., Zhao, J., Tao, W., Zhang, X., et al. (2019). Phytochemical information and biological activities of quinolizidine alkaloids in Sophora: a comprehensive review. Curr. Drug Targets 20, 1572–1586. doi:10.2174/1389450120666190618125816
Wang, J. F., Liu, S. S., Song, Z. Q., Xu, T. C., Liu, C. S., Hou, Y. G., et al. (2020). Naturally occurring flavonoids and isoflavonoids and their microbial transformation: a review. Molecules 25 (21), 5112. doi:10.3390/molecules25215112
Wang, Q., Li, Y., Li, K.-W., and Zhou, C.-Z. (2022). Sophoridine: a review of its pharmacology, pharmacokinetics and toxicity. Phytomedicine 95, 153756. doi:10.1016/j.phymed.2021.153756
Wang, X., Zheng, R., Huang, X., Mao, Z., Wang, N., Li, H., et al. (2018). Effects of alkaloids from Sophora flavescens on osteoblasts infected with Staphylococcus aureus and osteoclasts. Phytother. Res. 32, 1354–1363. doi:10.1002/ptr.6069
Wang, Z. L., Sun, J. Y., Wang, D. N., Xie, Y. H., Wang, S. W., and Zhao, W. M. (2006). Pharmacological studies of the large-scaled purified genistein from huaijiao (Sophora japonica – leguminosae) on anti-osteoporosis. Phytomedicine 13, 718–723. doi:10.1016/j.phymed.2005.09.005
Wu, Z., and Liu, L. (2022). The protective activity of genistein against bone and cartilage diseases. Front. Pharmacol. 13, 1016981. doi:10.3389/fphar.2022.1016981
Yang, Y., Tian, Y., Zhang, Q., Li, X., Fu, Y., Pei, H., et al. (2020). Comparative effects of flavonoids from fructus Sophorae on rat osteoblasts in vitro. Rec. Nat. Prod. 14, 65–76. doi:10.25135/rnp.138.19.04.1262
Yoon, H. J., Seo, C. R., Kim, M., Kim, Y. J., Song, N. J., Jang, W. S., et al. (2013). Dichloromethane extracts of Sophora japonica L. Stimulate osteoblast differentiation in mesenchymal stem cells. Nutr. Res. 33, 1053–1062. doi:10.1016/j.nutres.2013.08.004
Zhang, R., Wang, R., Zhao, S., Chen, D., Hao, F., Wang, B., et al. (2022). Extraction, separation, antitumor effect, and mechanism of alkaloids in Sophora alopecuroides: a review. Separations 9, 380. doi:10.3390/separations9110380
Zhang, X., Hou, G., Liu, A., Xu, H., Guan, Y., Wu, Y., et al. (2019). Matrine inhibits the development and progression of ovarian cancer by repressing cancer associated phosphorylation signaling pathways. Cell Death Dis. 10, 770. doi:10.1038/s41419-019-2013-3
Zhao, X., Mei, L., Pei, J., Liu, Z., Shao, Y., Tao, Y., et al. (2017). Sophoridine from Sophora flower attenuates ovariectomy induced osteoporosis through the RANKL-ERK-NFAT pathway. J. Agric. Food Chem. 65, 9647–9654. doi:10.1021/acs.jafc.7b03666
Zhou, C., Shi, Z., Meng, J., Hu, B., Zhao, C., Yang, Y., et al. (2018). Sophocarpine attenuates wear particle-induced implant loosening by inhibiting osteoclastogenesis and bone resorption via suppression of the NF-κB signalling pathway in a rat model. Br. J. Pharmacol. 175, 859–876. doi:10.1111/bph.14092
Zhou, H., Li, J., Sun, F., Wang, F., Li, M., Dong, Y., et al. (2020). A review on recent advances in aloperine research: pharmacological activities and underlying biological mechanisms. Front. Pharmacol. 11, 538137. doi:10.3389/fphar.2020.538137
Glossary
Keywords: alkaloids, bone health, flavonoids, osteoporosis, Sophora
Citation: Aly SH, Elbadry AMM, El-Shazly M and Hwang T-L (2023) Exploring the potential role of genus Sophora in the management of osteoporosis: a phytochemical and biological review. Front. Nat. Produc. 2:1302371. doi: 10.3389/fntpr.2023.1302371
Received: 26 September 2023; Accepted: 07 December 2023;
Published: 21 December 2023.
Edited by:
Michał Tomczyk, Medical University of Bialystok, PolandReviewed by:
Alan Talevi, National University of La Plata, ArgentinaSergio Ortiz, Université de Strasbourg, France
Copyright © 2023 Aly, Elbadry, El-Shazly and Hwang. This is an open-access article distributed under the terms of the Creative Commons Attribution License (CC BY). The use, distribution or reproduction in other forums is permitted, provided the original author(s) and the copyright owner(s) are credited and that the original publication in this journal is cited, in accordance with accepted academic practice. No use, distribution or reproduction is permitted which does not comply with these terms.
*Correspondence: Mohamed El-Shazly, mohamed.elshazly@pharma.asu.edu.eg; Tsong-Long Hwang, htl@mail.cgu.edu.tw