- 1Department of Medical and Biological Engineering, Kyungpook National University, Daegu, Republic of Korea
- 2Department of Preventive Dentistry, School of Dentistry, Kyungpook National University, Daegu, Republic of Korea
- 3Institute for Translational Research in Dentistry, Kyungpook National University, Daegu, Republic of Korea
- 4Department of Physical Medicine and Rehabilitation, College of Medicine, Yeungnam University, Daegu, Republic of Korea
- 5GE HealthCare, Munich, Germany
- 6Department of Radiology and Radiological Sciences, Vanderbilt University Medical Center, Nashville, TN, United States
- 7Department of Radiology, Kyungpook National University Hospital, Daegu, Republic of Korea
- 8Department of Molecular Medicine, School of Medicine, Kyungpook National University, Daegu, Republic of Korea
Introduction: Chewing has been reported to enhance cognitive function through the increase in cerebral blood flow. However, the mechanisms linking cerebral blood flow increase to metabolic changes in the brain affecting cognition remain unclear. We hypothesized that glutathione (GSH) plays a pivotal role in these mechanisms. Therefore, this study aimed to investigate changes in brain GSH levels following chewing and their association with cognitive function in healthy young adults.
Methods: A total of 52 university students were recruited, and the Korean version of the Repeatable Battery for the Assessment of Neuropsychological Status was used for the neurocognitive evaluations. Brain GSH levels following chewing gum or wood blocks were measured using MEscher-GArwood Point RESolved Spectroscopy (MEGA-PRESS) sequence, and their relevance to neurocognitive evaluation results was investigated.
Results: Chewing significantly increased brain GSH concentration, particularly in the wood-chewing group compared to the gum-chewing group, as observed in the anterior cingulate cortex. Furthermore, the rise in GSH concentration in the wood-chewing group was positively correlated with memory function.
Conclusion: Chewing moderately hard material elevates brain antioxidant levels such as GSH, potentially influencing cognitive function.
Introduction
Recent evidence has suggested a potential link between mastication and cognitive function (Lin, 2018; Yokoyama et al., 2017; Onozuka et al., 2003; De Cicco et al., 2016; Fantozzi et al., 2021). This connection may stem from mastication’s ability to influence neuronal metabolism and regional cerebral blood flow across multiple brain areas, including the prefrontal cortex (PFC). For instance, chewing moderately hard food increases cerebral blood flow, while chewing soft and elastic food like gum of varying hardness levels significantly increases brain activity (Suzuki et al., 1994; Onozuka et al., 2002). High levels of blood oxygen have been observed in brain regions with heightened cerebral blood flow, such as the PFC and hippocampus, which are crucial for mastication and cognitive processes like learning and memory (Yokoyama et al., 2017). Clinical studies suggest that individuals experiencing chewing difficulties tend to exhibit lower cognitive function and greater cognitive impairment, whereas activation of cerebral blood appears to positively impact various cognitive functions (Tada and Miura, 2017). However, the precise metabolic changes in the brain associated with cognition following cerebral blood flow increase remain unclear.
Oxidative damage to the brain is believed to play a key role in the decline of cognitive function (Head, 2009; Collin, 2019). Studies on both normal and pathological brains, including those affected by neurodegenerative diseases and aging, have demonstrated higher levels of oxidative damage in aged brains compared to younger ones (Montine et al., 2002). The brain is highly vulnerable to oxidative damage for several reasons. First, human brain consumes approximately 20% of the body’s total oxygen and contains a high amount of polyunsaturated fatty acids, making it susceptible to peroxidation. Second, the accumulation of redox-active metals (such as iron, copper, and zinc) in the brain can catalyze the formation of reactive oxygen species (ROS). Several metabolic processes generate ROS, which can lead to accumulated oxidative damage in human brain (Baierle et al., 2015; Uysal et al., 2018).
To defend against ROS, the antioxidant system consists of several enzymes, such as catalase, superoxide dismutase, glutathione peroxidase, and numerous non-enzymatic endogenous antioxidants such as glutathione (GSH) (Mehta and Gowder, 2015). Among these, GSH is the most crucial antioxidant in the brain for defense against oxidative stress (Meister and Anderson, 1983). In normal adults, GSH concentration typically ranges from 1 to 3 mM (Sanaei Nezhad et al., 2017). Neurons in the brain require high levels of GSH to mitigate oxidative stress, maintain structural integrity, and support cognitive function (Uttara et al., 2009). Magnetic resonance spectroscopy (MRS) has been employed to examine cortical GSH levels. However, measuring GSH with MRS presents challenges due to the low GSH concentration, low signal-to-noise ratio of the brain spectra, and significant spectral overlap between metabolites with varying peak intensities (Lee and Kim, 2019). Consequently, various methods have been proposed to assess GSH concentration in vivo within the human brain (Lagopoulos et al., 2013; Mlynárik et al., 2006; Zhao et al., 2006; Mescher et al., 1998). Among these, the MEscher-GArwood Point RESolved Spectroscopy (MEGA-PRESS) sequence has been widely recommend (Rae and Williams, 2007; Terpstra et al., 2006). This spectral editing MRS method is extensively utilized to distinguish and quantify specific metabolites.
Despite the recognition of the crucial role of GSH, how GSH levels in the brain change with mastication remains unexplored. Additionally, the impact of chewing hardness on the correlation between GSH levels and mastication has not been previously studied. In this study, we utilized MEGA-PRESS to measure brain GSH levels in a healthy cohort and investigate their associations with mastication. The effects of chewing hardness on brain’s GSH levels were then examined using materials with different hardness, including gum and wood blocks. The anterior cingulate cortex (ACC), which is pivotal for cognitive control, was selected as the regions of interest. Subsequently, we explored the relationship between brain GSH levels and cognitive function. Our hypotheses were as follows: (1) brain GSH levels correlate with chewing hardness, and (2) chewing hardness influences the relationship between changes in GSH levels and cognitive function.
Methods
Participants
The study involved 52 healthy university students from Daegu, Korea. The sample size was determined using G-power software with an effect size (Cohen’s d) of 0.8, significance level of 0.05, and power of 0.8 (Poldrack et al., 2011). Participants were voluntarily participated in the study through recruitment notice, and individuals with neurological disease, psychiatric illness, temporomandibular joint disorders, or contraindications to magnetic resonance imaging were excluded. The participants were divided into two groups, including the gum-chewing (n = 27) and wood-chewing (n = 25) group, ensuring similarity in age, sex, and education level between the groups. Sociodemographic information, including age, sex, education, and general health status, were collected via a self-reported questionnaire. This study was approved by the Institutional Review Board of Kyungpook National University Dental Hospital (KNUDH-2021-06-09-02), and all participants provided informed consent prior to participation.
Neuropsychological measurement
The Korean Repeatable Battery for the Assessment of Neuropsychological State (K-RBANS) was administered by a clinical psychologist. The K-RBANS neuropsychological test assesses five indices within 30-min timeframe, covering immediate and delayed memory, visuospatial capacity, verbal ability, and attention (Park et al., 2021). The results of the K-RBANS are reported as scores for the five indices, scores for the 12 subtests, and a total scale score. This format enables a comprehensive evaluation of the participant’s cognitive function as well as an assessment of specific cognitive domains.
Chewing task
Participants were positioned supine with their heads stabilized using a fixation device and instructed to chew either paraffin wax gum (CAT 21 Buf Test, Morita Co., Japan) or a wood stick (Tongue Depressors, Daehan medical, Korea) for 5 min. To ensure consistent movement, participants chewed on the right molar region at a frequency of 1 Hz, alternating between 30 s of chewing and 30 s of rest.
MRS data measurement
All MRS data were acquired using a 3.0 T GE SIGNA Architect MR scanner with a 48-channel head coil before (MAS_before) and after mastication (MAS_after). MRS data were obtained using a GSH-edited MEGA-PRESS sequence on a single voxel of 2 × 2 × 2 cm3 located at the ACC (repetition time = 2,000 ms, echo time = 79 ms, number of averaging = 128, total sampling points = 4,096, and spectral width = 5 kHz). A 20-ms Gaussian 180° RF pulse (bandwidth = 60.4 Hz), serving as an editing pulse, was applied before and after the second 180° slice-selective RF pulse at 7 ppm in the editing-off (Edit-OFF) scan and at 4.56 ppm coupled with GSH spin resonance at 2.95 ppm in the editing-on (Edit-ON) scan within the MEGA-PRESS sequence. Individual Edit-ON and Edit-OFF resonance spectra were alternately acquired. Vender-supplied tools were used for B1 calibration, water suppression (water linewidth = 8.1 ± 1.7 Hz for MAS_before, 8.0 ± 1.5 Hz for MAS_after), and B0 shim (zero, first, and second order). At the beginning of each scan, an unsuppressed reference water signal was acquired for eddy-current correction and multichannel combination. Foam pads were utilized inside the head coil to minimize head movement.
MRS data processing
Individual MR spectra were obtained using apodization with a 1.5-Hz exponential function and underwent frequency and phase-shift correction. The Edit-ON and Edit-OFF spectra were aligned by minimizing the difference signals of N-acetyl-aspartate (NAA) at 2.01 ppm, choline at 3.21 ppm, and creatine at 3.03 ppm to determine the GSH-edited difference spectra. To estimate the levels of metabolites in the GSH-edited difference and Edit-OFF spectra, the LCModel1 was applied. The basis set for spectral fitting of GSH-edited difference spectra included GSH, NAA, N-acetylaspartateglutamate (NAAG), aspartate, lactate, myo-inositol (MI), phosphocholine (PCh), glycerophosphocholine (GPC), threonine, phosphoethanolamine, and glucose. The basis set for spectral fitting of Edit-OFF spectra additionally included glutamine, glutamate, creatine (Cr), phosphocreatine (PCr), glycine, alanine, acetate, ethanolamine, ascorbic acid, taurine, and serine, with LCModel built-in macromolecular and lipid basis signals. The metabolite basis set was determined using a simulation tool incorporating the MEGA-PRESS sequence diagram, spectral and slice-selective RF, and gradient pulse strength from a published simulation algorithm (Choi et al., 2012). After GSH estimates were normalized to the unsuppressed water, millimolar concentrations were calculated by assuming a mean total creatine concentration of 8 mM (Mekle et al., 2009; Tkac et al., 2009). The quality of spectral fitting was assessed using the Cramér–Rao lower bounds (CRLB) as the error estimate of the concentration measurement, with each GSH CRLB result being <20%, indicating reliable measurement (Mandal et al., 2015; Mandal et al., 2023). The fitting of GSH using the MEGA-PRESS sequence is illustrated in Figure 1.
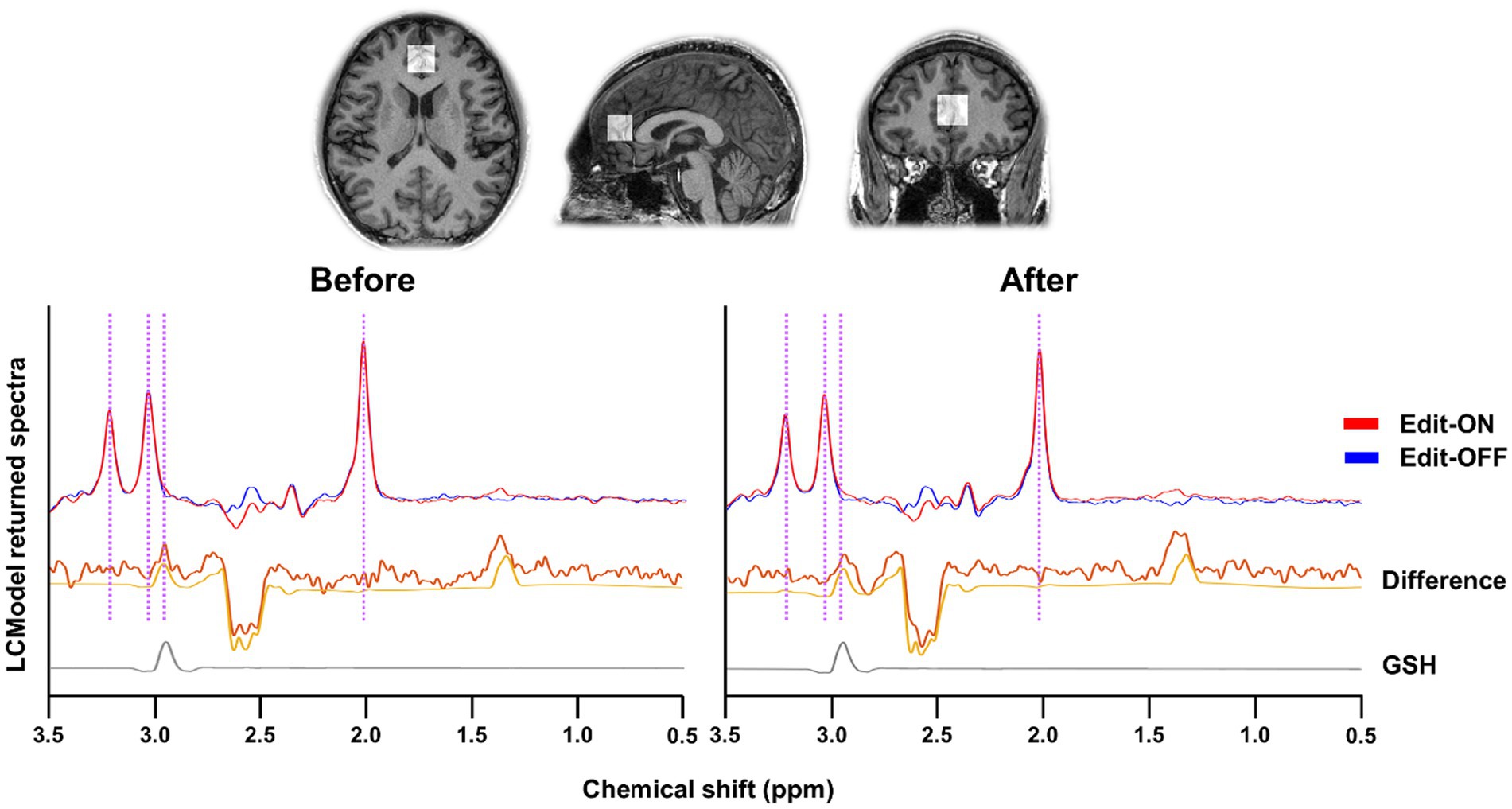
Figure 1. The representative GSH-edited spectra of mastication before and after from anterior cingulate cortex (ACC) by MEGA-PRESS method. Editing on (Red line) and off (Blue line) spectra were aligned and differenced. The difference spectra and spectral fitting results were described as the yellow thick and thin lines, respectively. Because GSH signal in the MR spectra is overlapped with other metabolite signals, the spectral editing technique with edit-on and edit-off MRS sequences was employed to give only GSH signal as shown in the bottom (gray line). The purple vertical lines indicate the peaks of NAA (2.01 ppm), GSH (2.95 ppm), Cr (3.03 ppm), Cho (3.21 ppm).
Statistical analysis
The Statistical Package for Social Sciences (SPSS 25; https://www.ibm.com/kr-ko/products/spss-statistics) was used for all statistical analyses. An independent samples t-test was conducted to compare the neuropsychological scores and GSH levels between participants in the gum-and wood-chewing groups. A paired t-test was performed to investigate changes in GSH levels before and after mastication. Pearson’s coefficients were calculated to examine correlations between GSH levels in ACC and neuropsychological scores.
Results
Demographic characteristics and neuropsychological analysis
The gum-chewing group consisted of 13 men and 14 women, and the wood-chewing group consisted of 11 men and 14 women. The average age of all participants was 21.81 ± 1.56 years, with the gum-chewing group averaging 22.00 ± 1.66 years and the wood-chewing group averaging 21.60 ± 1.44 years. Participants had an average education level of 14.63 ± 1.14 years, with the gum-chewing group averaging 14.74 ± 1.06 years and the wood-chewing group averaging 14.52 ± 1.23 years. No significant difference was found in the sociodemographic characteristics between the two groups (p > 0.05) (data not shown).
No statistically significant difference was observed in the K-RBANS assessment scores between the gum-and wood-chewing groups (p > 0.05) (Table 1).
Metabolites quantification by MRS using MEGA-PRESS
GSH levels in ACC (mean ± SD and CRLB) were 1.23 ± 0.24 mM (9% ± 2%) and 1.22 ± 0.18 mM (8% ± 2%) for MAS_before and 1.28 ± 0.33 mM (8% ± 3%) and 1.37 ± 0.23 mM (8% ± 2%) for MAS_after in the gum-and wood-chewing groups, respectively. According to a paired t-test, GSH levels in MAS_after were significantly increased compared to MAS_before in the wood-chewing group (t = 2.97, p = 0.007, effect size = 0.81). However, no significant increase in GSH level was observed in the gum-chewing group (t = 0.68, p = 0.505, effect size = 0.1) (Figure 2). In an independent samples t-test (p = 0.237, effect size = 0.33), there was no significant difference observed between the groups in terms of changes in GSH levels. Additionally, the concentration for other metabolites estimated from Edit-off spectra, such as tNAA (total NAA; NAA + NAAG), tCr (total creatine; Cr + PCr), tCho (total choline; PCh + GPC, and MI), were not different among the two groups. The metabolite concentration changes between MAS_before and MAS_after were also insignificant in each group. These results were provided in Supplementary Figure S1.
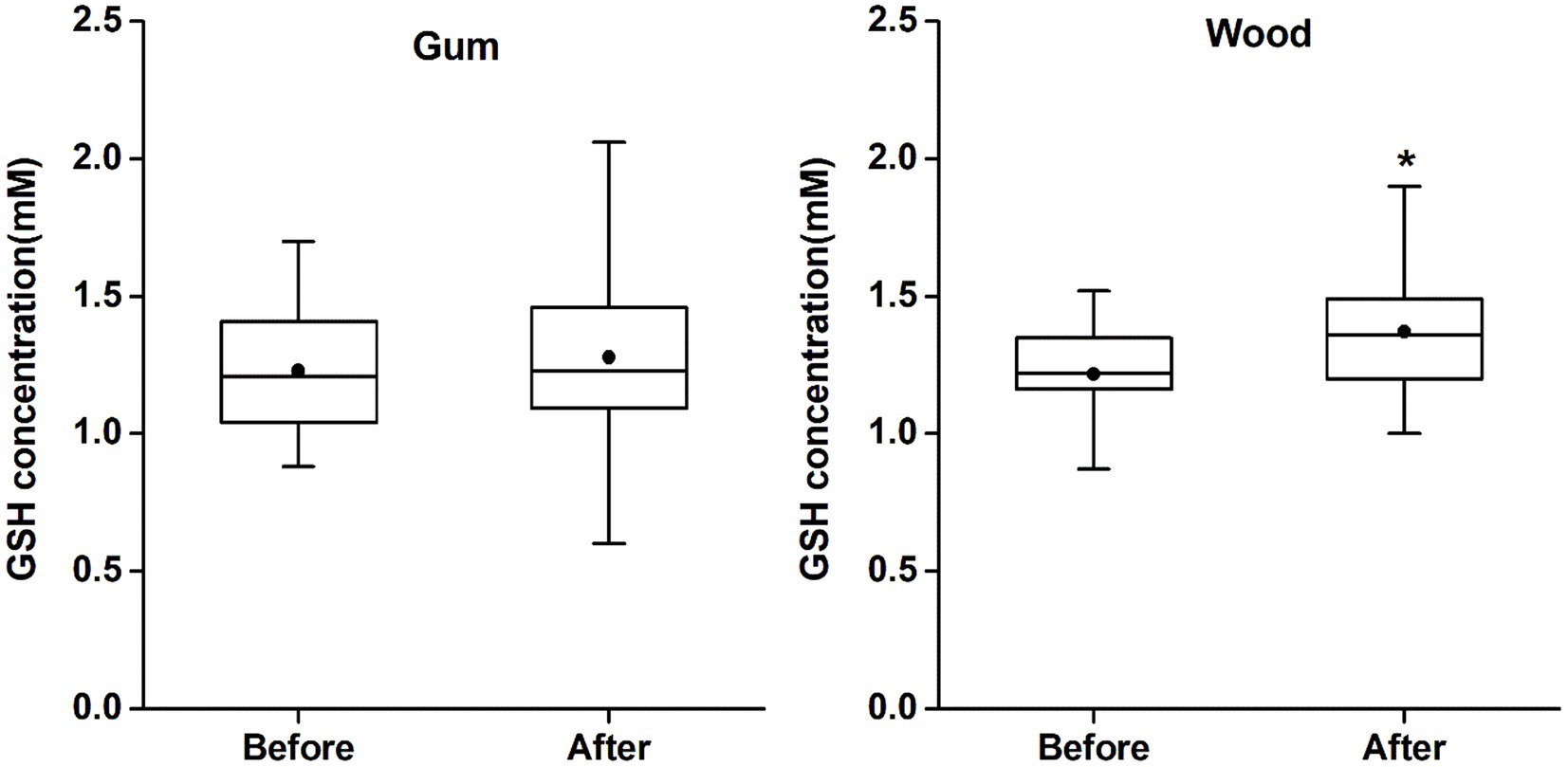
Figure 2. The changes of GSH concentrations in each group. The GSH concentrations (Cramér-Rao lower bound) were 1.23 mM (9%), 1.28 mM (8%) in the gum group, and 1.22 mM (8%), 1.37 mM (8%) in the wood group in mastication before and after, respectively. (*p < 0.01). The gum group showed no significant change before and after gum chewing but the wood group showed statistically significant increase in GSH concentration after wood chewing compared to GSH concentration before wood chewing.
Relationship between GSH levels and neuropsychological measurements
In the wood-chewing group, changes in GSH concentrations demonstrated a positive correlation with immediate memory (r = 0.520, p = 0.008) and story memory (r = 0.439, p = 0.028) scores (Figure 3). However, no significant correlations were observed in the gum-chewing group.
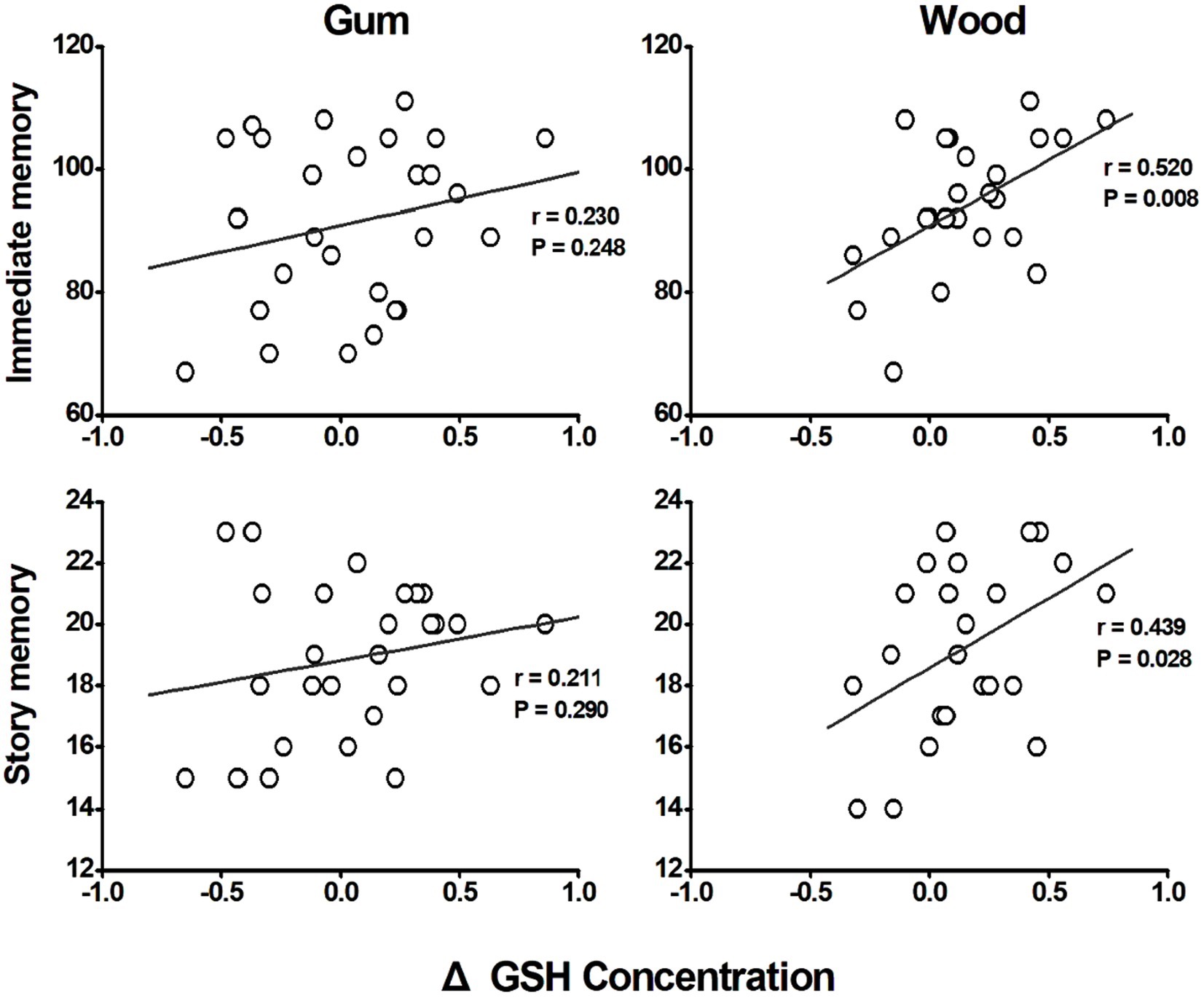
Figure 3. Correlation between the changes of GSH concentrations in anterior cingulate cortex (ACC) and cognitive functions. Both correlations with immediate memory and story memory as cognitive function measure revealed that the gum chewing group did not show any significant correlations between the changes of GSH concentrations and immediate memory and story memory (p = 0.248 and p = 0.290 respectively). The wood chewing group however showed the significant positive correlations between the changes of GSH concentrations and immediate memory and story memory (p = 0.008 and p = 0.028 respectively). These results therefore suggest that the increase of GSH concentration by wood chewing increase the cognitive functioning in wood chewing group.
Discussion
In the current study, GSH concentration measured by MRS was significantly higher in the ACC in the wood-chewing group compared to that in the gum-chewing group. Furthermore, the increase in GSH in the wood-chewing group was positively correlated with memory function items in neuropsychological measurements. To the best of our knowledge, this is the first report indicating that mastication can alter the level of antioxidants in the human brain, and that an increase in brain antioxidant levels is associated with cognitive function.
The results from the current study revealed that chewing moderately hard material leads to an increase in the GSH concentration in the brain. GSH is present in almost all cell types and is involved in numerous physiological functions, including detoxication of xenobiotics, intracellular redox homeostasis, cysteine carrier/storage, cell signaling, protein folding and function, gene expression, cell differentiation/proliferation, immune response, and antiviral (Cooper and Kristal, 1997; Pastore et al., 2003). Additionally, GSH acts as a “master antioxidant” across all tissues, particularly in the brain where the GSH plays a crucial role in antioxidant defense (Dringen, 2000). While low levels of ROS are necessary for maintaining normal cellular function, their accumulation leads to oxidative stress, resulting in lipid peroxidation, protein oxidation, and DNA damage that ultimately impair cellular function. The brain is especially vulnerable to oxidative stress. Therefore, the finding that chewing hard materials increases GSH concentration is intriguing and significant because it suggests that mastication may help protect the brain from oxidative stress. Based on these results, we hypothesized that the increase of GSH, brain antioxidant, by chewing hard material may effectively remove the reactive oxygen species in the brain and thus stimulate brain cells to improve the brain cognitive function. Furthermore, this finding may be particularly relevant for elderly individuals, as GSH deficiency contributes to oxidative stress, which is implicated in aging and the pathogenesis of many neurodegenerative diseases (Jenner, 1994; Jenner, 2003; Maher, 2005). An MRS study using MEGA-PRESS sequence provided in vivo evidence that the patients with Alzheimer’s disease exhibit reduced GSH concentrations in the frontal cortex compared to age-matched healthy controls (Mandal et al., 2015).
While the findings in this study strongly suggest that chewing relatively hard material is closely associated with an increase in GSH concentration in the brain, the exact cause of this GSH alteration remains to be elucidated. It is possible that the increase in GSH simply reflects an increase in cerebral blood flow (CBF). Several studies have shown that chewing moderately hard food leads to an increase in CBF, and chewing gum with varying degrees of hardness significantly enhances brain activity (Suzuki et al., 1994; Onozuka et al., 2002). Increased CBF has been linked to improved cognitive functions. According to previous reports, it is tempting to hypothesize that the increase CBF may supply sufficient nutrients to the brain to stimulate GSH synthesis. The correlation observed in the current study between the increase in GSH and cognitive measures found seems to support our hypothesis that cognitive function improves as GSH concentration increases.
The findings of the present study suggest important implications for a therapeutic strategies aimed at increasing neuronal GSH levels in the brain. While elevating brain GSH levels holds promises as a treatment for GSH-related neurodegenerative or neuropsychiatric diseases (Gilgun-Sherki et al., 2004; Pittenger et al., 2011), there are currently no therapeutic drugs available for this purpose. Orally administered GSH is rapidly degraded in the gut, and intravenously administered GSH is quickly oxidized to GSSG in the bloodstream with a half-life of 2–3 min (Ammon et al., 1986; Wendel and Cikryt, 1980). Therefore, the direct administration of GSH does not appear promising for treating GSH-related neurodegenerative or neuropsychiatric diseases. On the other hand, the current study demonstrated that chewing moderately hard materials such as wood blocks can increase brain GSH levels without degradation in the gut or oxidization in the blood. Hence, mastication, especially with moderately hard material, may offer a practical approach to enhancing GSH levels in the brain and is beneficial for maintaining or bolstering antioxidant defense in the brain.
This study has several limitations that should be addressed. Firstly, the participants in the present study were aged 20–30 years and were selected using convenience sampling. Due to this narrow age range, the findings need further validations across broader age groups, including both younger and older individuals. Secondly, the study population was limited in size, necessitating caution when generalizing the findings to a larger populations. Thirdly, our study focused specifically on the ACC, and thus, the results may not be applicable to other brain regions. Future research could explore GSH levels across a broader range of brain regions to gain a more comprehensive understanding of region-specific changes in GSH in response to chewing hardness. Fourthly, while our study limited chewing time to 5 min using relatively soft gum, longer chewing durations are possible with softer gums, potentially affecting brain activity. Moreover, chewing gum and wood can be differed not only in hardness but also in various other characteristics such as texture of the materials. Investigating changes in GSH concentrations that reflect real-world chewing scenarios will be necessary in future research. Fifthly, we were unable to objectively measure chewing hardness. Further research is needed to quantify chewing hardness and determine the optimal chewing force that can enhance cognitive function. These limitations highlight avenues for future studies to further elucidate the effects of chewing on GSH levels in the brain under diverse conditions and across different populations.
In summary, this study yielded two major findings. Firstly, the wood-chewing group experienced stimulated of brain GSH synthesis, leading to increased GSH levels in the brain. Secondly, correlation analysis indicated that the higher GSH levels in the wood-chewing group were associated with improved scores in cognitive measures. Since there are currently no drugs or established practices for boosting brain GSH levels, our findings suggest that chewing moderately hard material could serve as an effective practice for increasing GSH levels in the brain. Based on these results, consuming harder foods might prove more effective in enhancing brain antioxidant defenses through elevated GSH levels.
Data availability statement
The raw data supporting the conclusions of this article will be made available by the authors, without undue reservation.
Ethics statement
The studies involving humans were approved by Institutional Review Board of Kyungpook National University Dental Hospital. The studies were conducted in accordance with the local legislation and institutional requirements. The participants provided their written informed consent to participate in this study.
Author contributions
SK: Formal analysis, Investigation, Validation, Writing – original draft, Writing – review & editing, Visualization. J-HK: Formal analysis, Investigation, Validation, Visualization, Writing – original draft, Writing – review & editing, Data curation, Project administration. HL: Data curation, Formal analysis, Investigation, Visualization, Writing – review & editing. SJ: Data curation, Writing – review & editing, Conceptualization, Methodology, Validation. RN: Data curation, Methodology, Validation, Writing – review & editing. CC: Data curation, Methodology, Validation, Writing – review & editing. YC: Data curation, Methodology, Writing – review & editing, Conceptualization, Formal analysis, Supervision, Writing – original draft. Y-HC: Conceptualization, Data curation, Formal analysis, Methodology, Supervision, Writing – original draft, Writing – review & editing, Funding acquisition, Investigation, Project administration, Validation.
Funding
The author(s) declare that financial support was received for the research, authorship, and/or publication of this article. This work was supported by the National Research Foundation of Korea (NRF) grant funded by the Korea government (MSIT) under grant numbers NRF-2021R1A2C2003160 and RS-2024-00349360.
Acknowledgments
We thank clinical psychologists from the Department of Psychiatry, College of Medicine, Yeungnam University who conducted neuropsychological assessment.
Conflict of interest
RN was employed by GE HealthCare.
The remaining authors declare that the research was conducted in the absence of any commercial or financial relationships that could be construed as a potential conflict of interest.
Publisher’s note
All claims expressed in this article are solely those of the authors and do not necessarily represent those of their affiliated organizations, or those of the publisher, the editors and the reviewers. Any product that may be evaluated in this article, or claim that may be made by its manufacturer, is not guaranteed or endorsed by the publisher.
Supplementary material
The Supplementary material for this article can be found online at: https://www.frontiersin.org/articles/10.3389/fnsys.2024.1489919/full#supplementary-material
Abbreviations
ACC, Anterior cingulate cortex; CBF, Cerebral blood flow; Cr, Creatine; CRLB, Cramér–Rao lower bounds; Edit-OFF, Editing-off; Edit-ON, Editing-on; fMRI, Functional magnetic resonance imaging; GPC, Glycerophosphocholine; GSH, Glutathione; K-RBANS, Korean Repeatable Battery for the Assessment of Neuropsychological State; MAS_before, Before mastication; MAS_after, After mastication; MEGA-PRESS, Mescher-Garwood Point Resolved Spectroscopy; MI, Myo-inositol; MRS, Magnetic resonance spectroscopy; NAA, N-acetyl-aspartate; NAAG, N-acetylaspartateglutamate; PCh, Phosphocholine; PCr, Phosphocreatine; PFC, Prefrontal cortex; ROS, Reactive oxygen species; SD, Standard deviation; SPSS, Statistical Package for Social Sciences; tCho, Total choline; tCr, Total creatine; tNAA, Total N-acetyl-aspartate.
Footnotes
References
Ammon, H. P., Melien, M. C., and Verspohl, E. J. (1986). Pharmacokinetics of intravenously administered glutathione in the rat. J. Pharm. Pharmacol. 38, 721–725. doi: 10.1111/j.2042-7158.1986.tb04478.x
Baierle, M., Nascimento, S. N., Moro, A. M., Brucker, N., Freitas, F., Gauer, B., et al. (2015). Relationship between inflammation and oxidative stress and cognitive decline in the institutionalized elderly. Oxidative Med. Cell. Longev. 2015, 1–12. doi: 10.1155/2015/804198
Choi, C., Ganji, S. K., DeBerardinis, R. J., Hatanpaa, K. J., Rakheja, D., Kovacs, Z., et al. (2012). 2-hydroxyglutarate detection by magnetic resonance spectroscopy in subjects with IDH-mutated gliomas. Nat. Med. 18, 624–629. doi: 10.1038/nm.2682
Collin, F. (2019). Chemical basis of reactive oxygen species reactivity and involvement in neurodegenerative diseases. Int. J. Mol. Sci. 20:2407. doi: 10.3390/ijms20102407
Cooper, A. J., and Kristal, B. S. (1997). Multiple roles of glutathione in the central nervous system. Biol. Chem. 378, 793–802
De Cicco, V., Barresi, M., Fantozzi, M. P. T., Cataldo, E., Parisi, V., and Manzoni, D. (2016). Oral implant-prostheses: new teeth for a brighter brain. PLoS One 11:e0148715. doi: 10.1371/journal.pone.0148715
Dringen, R. (2000). Metabolism and functions of glutathione in brain. Prog. Neurobiol. 62, e649–e671. doi: 10.1016/s0301-0082(99)00060-x
Fantozzi, M. P. T., De Cicco, V., De Cicco, D., d'Ascanio, P., Cataldo, E., Bruschini, L., et al. (2021). Chewing and cognitive improvement: the side matters. Front. Syst. Neurosci. 15:749444. doi: 10.3389/fnsys.2021.749444
Gilgun-Sherki, Y., Melamed, E., and Offen, D. (2004). The role of oxidative stress in the pathogenesis of multiple sclerosis: the need for effective antioxidant therapy. J. Neurol. 251, 261–268. doi: 10.1007/s00415-004-0348-9
Head, E. (2009). Oxidative damage and cognitive dysfunction: antioxidant treatments to promote healthy brain aging. Neurochem. Res. 34, 670–678. doi: 10.1007/s11064-008-9808-4
Jenner, P. (1994). Oxidative damage in neurodegenerative disease. Lancet 344, 796–798. doi: 10.1016/S0140-6736(94)92347-7
Jenner, P. (2003). Oxidative stress in Parkinson’s disease. Ann. Neurol. 53, S26–S38. doi: 10.1002/ana.10483
Lagopoulos, J., Hermens, D. F., Tobias-Webb, J., Duffy, S., Naismith, S. L., White, D., et al. (2013). In vivo glutathione levels in young persons with bipolar disorder: a magnetic resonance spectroscopy study. J. Psychiatr. Res. 47, 412–417. doi: 10.1016/j.jpsychires.2012.12.006
Lee, H. H., and Kim, H. (2019). Intact metabolite spectrum mining by deep learning in proton magnetic resonance spectroscopy of the brain. Magn. Reson. Med. 82, 33–48. doi: 10.1002/mrm.27727
Lin, C. (2018). Revisiting the link between cognitive decline and masticatory dysfunction. BMC Geriatr. 18:5. doi: 10.1186/s12877-017-0693-z
Maher, P. (2005). The effects of stress and aging on glutathione metabolism. Ageing Res. Rev. 4, 288–314. doi: 10.1016/j.arr.2005.02.005
Mandal, P. K., Rimil, G. R., and Avinash, K. (2023). Distribution pattern of closed and extended forms of glutathione in the human brain: MR spectroscopic study. ACS Chem. Neurosci. 14, 270–276. doi: 10.1021/acschemneuro.2c00573
Mandal, P. K., Saharan, S., Tripathi, M., and Murari, G. (2015). Brain glutathione levels–a novel biomarker for mild cognitive impairment and Alzheimer’s disease. Biol. Psychiatry 78, 702–710. doi: 10.1016/j.biopsych.2015.04.005
Mehta, S. K., and Gowder, S. J. T. (2015). “Members of antioxidant machinery and their functions basic principles and clinical significance of oxidative stress” in Basic principles and clinical significance of oxidative stress. ed. S. J. T. Gowder (BoD-Book on Demand: United Kingdom), 55–59.
Meister, A., and Anderson, M. E. (1983). Glutathione. Annu. Rev. Biochem. 52, 711–760. doi: 10.1146/annurev.bi.52.070183.003431
Mekle, R., Mlynarik, V., Gambarota, G., Hergt, M., and Krueger, G. (2009). MR spectroscopy of the human brain with enhanced signal intensity at ultrashort echo times on a clinical platform at 3T and 7T. Magn. Reson. Med. 61, 1279–1285. doi: 10.1002/mrm.21961
Mescher, M., Merkle, H., Kirsch, J., Garwood, M., and Gruetter, R. (1998). Simultaneous in vivo spectral editing and water suppression. NMR Biomed. 11, 266–272. doi: 10.1002/(SICI)1099-1492(199810)11:6<266::AID-NBM530>3.0.CO;2-J
Mlynárik, V., Gambarota, G., Frenkel, H., and Gruetter, R. (2006). Localized short-echo-time proton MR spectroscopy with full signal-intensity acquisition. Magn. Reson. Med. 56, 965–970. doi: 10.1002/mrm.21043
Montine, T. J., Neely, M. D., Quinn, J. F., Beal, M. F., Markesbery, W. R., Roberts, L. J., et al. (2002). Lipid peroxidation in aging brain and Alzheimer’s disease. Free Radic. Biol. Med. 33, 620–626. doi: 10.1016/S0891-5849(02)00807-9
Onozuka, M., Fujita, M., Watanabe, K., Hirano, H., Niwa, M., Nishiyama, K., et al. (2002). Mapping brain region activity during chewing: a functional magnetic resonance imaging study. J. Dent. Res. 81, 743–746. doi: 10.1177/0810743
Onozuka, M., Fujita, M., Watanabe, K., Hirano, H., Niwa, M., Nishiyama, K., et al. (2003). Age-related changes in brain regional activity during chewing: a functional magnetic resonance imaging study. J. Dent. Res. 82, 657–660. doi: 10.1177/154405910308200817
Park, J. O., Koo, B. H., Kim, J. Y., Bai, D. S., Chang, M. S., and Kim, O. L. (2021). The Korean repeatable battery for the assessment of neuropsychological status-update: psychiatric and neurosurgery patient sample validity. J. Korean Neurosurg. Soc. 64, 125–135. doi: 10.3340/jkns.2020.0090
Pastore, A., Federici, G., Bertini, E., and Piemonte, F. (2003). Analysis of glutathione: implication in redox and detoxification. Clin. Chim. Acta 333, 19–39. doi: 10.1016/S0009-8981(03)00200-6
Pittenger, C., Bloch, M. H., and Williams, K. (2011). Glutamate abnormalities in obsessive compulsive disorder: neurobiology, pathophysiology, and treatment. Pharmacol. Ther. 132, 314–332. doi: 10.1016/j.pharmthera.2011.09.006
Poldrack, R. A., Mumford, J. A., and Nichols, T. E. (2011). “The multiple testing problem and solutions” in Handbook of functional MRI data analysis. eds. R. A. Poldrack and K. R. Henson (Cambridge: Cambridge University Press).
Rae, C. D., and Williams, S. R. (2007). Glutathione in the human brain: review of its roles and measurement by magnetic resonance spectroscopy. Anal. Biochem. 529, 127–143. doi: 10.1016/j.ab.2016.12.022
Sanaei Nezhad, F., Anton, A., Parkes, L. M., Deakin, B., and Williams, S. R. (2017). Quantification of glutathione in the human brain by MR spectroscopy at 3 tesla: comparison of PRESS and MEGA-PRESS. Magn. Reson. Med. 78, 1257–1266. doi: 10.1002/mrm.26532
Suzuki, M., Ishiyama, I., Takiguchi, T., Ishikawa, H., Suzuki, Y., and Sato, Y. (1994). Effects of gum hardness on the response of common carotid blood flow volume, oxygen uptake, heart rate and blood pressure to gum-chewing. J. Mastica. Health. Sci. 4, 51–62. doi: 10.1016/j.neures.2009.03.008
Tada, A., and Miura, H. (2017). Association between mastication and cognitive status: a systematic review. Arch. Gerontol. Geriatr. 70, 44–53. doi: 10.1016/j.archger.2016.12.006
Terpstra, M., Marjanska, M., Henry, P. G., Tkáˇc, I., and Gruetter, R. (2006). Detection of an antioxidant profile in the human brain in vivo via double editing with mega-press. Magn. Reson. Med. 56, 1192–1199. doi: 10.1002/mrm.21086
Tkac, I., Oz, G., Adriany, G., Ugurbil, K., and Gruetter, R. (2009). In vivo 1H NMR spectroscopy of the human brain at high magnetic fields: metabolite quantification at 4T vs. 7T. Magn. Reson. Med. 62, 868–879. doi: 10.1002/mrm.22086
Uttara, B., Singh, A., Zamboni, P., and Mahajan, R. (2009). Oxidative stress and neurodegenerative diseases: a review of upstream and downstream antioxidant therapeutic options. Curr. Neuropharmacol. 7, 65–74. doi: 10.2174/157015909787602823
Uysal, S., Senkardes, I., Mollica, A., Zengin, G., Bulut, G., Dogan, A., et al. (2018). Biologically active compounds from two members of the Asteraceae family: Tragopogon dubius Scop. and Tussilago farfara L. J. Biomol. Struct. Dyn. 37, 1–13. doi: 10.1080/07391102.2018.1506361
Wendel, A., and Cikryt, P. (1980). The level and half-life of glutathione in human plasma. FEBS Lett. 120, 209–211. doi: 10.1016/0014-5793(80)80299-7
Yokoyama, T., Sato, M., Natsui, S., Kuboyama, N., Suzuki, K., Inaba, H., et al. (2017). Effect of gum chewing frequency on oxygenation of the prefrontal cortex. Percept. Mot. Skills 124, 58–71. doi: 10.1177/0031512516683074
Keywords: mastication, hardness, functional magnetic resonance imaging (fMRI), cognitive function, motor function, brain activation
Citation: Kim S, Kim J-H, Lee H, Jang SH, Noeske R, Choi C, Chang Y and Choi Y-H (2024) Effect of chewing hard material on boosting brain antioxidant levels and enhancing cognitive function. Front. Syst. Neurosci. 18:1489919. doi: 10.3389/fnsys.2024.1489919
Edited by:
Diego Manzoni, University of Pisa, ItalyReviewed by:
Zhiliang Wei, Johns Hopkins Medicine, United StatesDavide De Cicco, Istituto Stomatologico Italiano, Italy
Enrico Cataldo, University of Pisa, Italy
Copyright © 2024 Kim, Kim, Lee, Jang, Noeske, Choi, Chang and Choi. This is an open-access article distributed under the terms of the Creative Commons Attribution License (CC BY). The use, distribution or reproduction in other forums is permitted, provided the original author(s) and the copyright owner(s) are credited and that the original publication in this journal is cited, in accordance with accepted academic practice. No use, distribution or reproduction is permitted which does not comply with these terms.
*Correspondence: Youn-Hee Choi, Y3loMTAwMUBrbnUuYWMua3I=; Yongmin Chang, eWNoYW5nQGtudS5hYy5rcg==
†These authors have contributed equally to this work and share first authorship