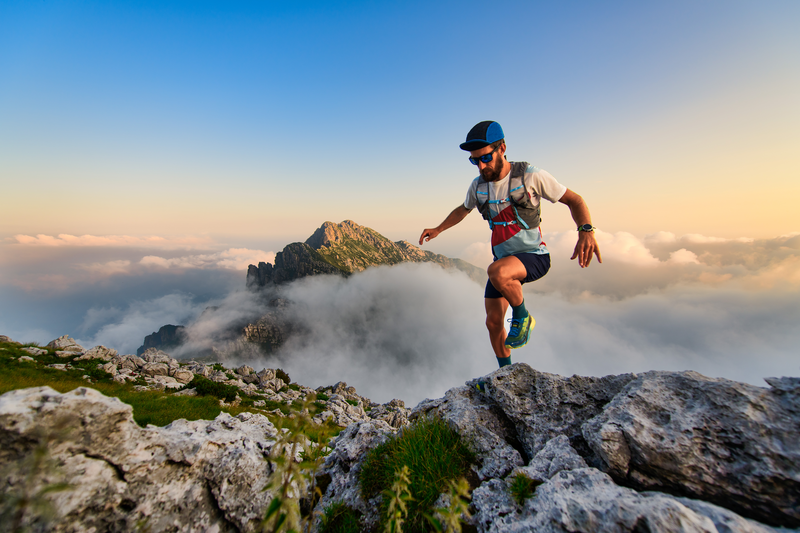
95% of researchers rate our articles as excellent or good
Learn more about the work of our research integrity team to safeguard the quality of each article we publish.
Find out more
MINI REVIEW article
Front. Syst. Neurosci. , 29 June 2020
Volume 14 - 2020 | https://doi.org/10.3389/fnsys.2020.00041
This article is part of the Research Topic Non-Invasive Brain Stimulation (NIBS) Techniques in Neurological and Neuropsychiatric Disorders: Physiological and Molecular Evidence View all 6 articles
Neural oscillations represent a fundamental mechanism that enables coordinated action during normal brain functioning. Auditory steady-state responses (ASSRs) are used to test the ability to generate gamma-range activity. Different non-invasive brain stimulation (NIBS) techniques have the potential to modulate neural activation patterns that are aberrant in a variety of neuropsychiatric disorders. Here, we summarize the current state of knowledge on how different methods of NIBS (transcranial altering current stimulation—tACS, transcranial direct current stimulation—tDCS, transcranial random noise stimulation—tRNS, paired associative stimulation—PAS, repetitive transcranial magnetic stimulation—rTMS) affect the gamma-range ASSRs in both healthy and clinical populations. We show that the current research has been far from systematic and methodologically heterogeneous. Nevertheless, some brain stimulation techniques, especially tACS and rTMS show strong potential for further exploration. We outline the main findings and provide directions for further research into neuromodulation of ASSRs as a promising biomarker of different psychopathological conditions such as schizophrenia, bipolar disorder, attention deficit hyperactivity disorder (ADHD), autism.
Neural oscillations in the gamma band (30–80 Hz) are thought to play a crucial role for information processing in cortical networks (Uhlhaas et al., 2009, 2011; Bosman et al., 2014) as well as are affected in a variety of neuropsychiatric disorders (for review see Herrmann and Demiralp, 2005) and associated with clinical symptoms (McNally and McCarley, 2016; Grent-’t-Jong et al., 2018), cognitive deficits (Bosman et al., 2014) and therapeutic outcomes of pharmaco-treatment (Khalid et al., 2016; Minzenberg et al., 2016; Arikan et al., 2018; Nugent et al., 2019).
Evaluation of brain responses to rhythmically repeated or modulated stimuli allows experimental assessment of gamma activity (Brenner et al., 2009). The largest auditory steady-state responses (ASSRs) recorded with EEG and/or MEG are observed at around 40 Hz (Galambos et al., 1981) with the current views on ASSRs reflecting both the aspect of superposition of middle-latency responses (Presacco et al., 2010) and a periodic activity related to the resonance within the activated system (Santarelli et al., 1995; Ross et al., 2005) These responses, though being distinct from transient evoked gamma (Ross et al., 2005), originate in auditory cortical and subcortical regions (Herdman et al., 2002) with a potential contribution from frontal, motor, parietal and occipital lobes (Farahani et al., 2017, 2019). ASSRs reflect the integrity of neural circuits and excitation/inhibition balance (for details refer to Tada et al., 2019) supported by N-methyl-D-aspartate (NMDA) and γ-aminobutyric acid (GABA) systems (Vohs et al., 2010; Sullivan et al., 2015; Sivarao et al., 2016; Koshiyama et al., 2018b). Accordingly, the alterations of 40-Hz ASSRs were consistently shown in schizophrenia (for review see Thuné et al., 2016) where both preclinical impairment (Tada et al., 2016) and potential to predict treatment outcome (Koshiyama et al., 2018a) were reported, making ASSRs a promising biomarker (O’Donnell et al., 2013). ASSRs were also viewed as indexing for typical and atypical cortical development (Edgar et al., 2016), with variable responses throughout the lifetime (Griskova-Bulanova et al., 2013).
Currently available pharmaco-treatments are relatively ineffective for remediation of cognitive impairments in neuropsychiatric conditions (Goff et al., 2011; Pan et al., 2017; Robbins, 2019). NIBS techniques were proposed as an alternative for pharmaco-treatments and as a cognitive enhancement approach (Cinel et al., 2019; Ishii et al., 2019). The NIBS induces changes in the excitability of target brain areas potentially leading to normalization of altered activation patterns and consequently—to clinically beneficial outcomes (Holtzheimer et al., 2012). Different NIBS techniques use either electrical or magnetic fields to modulate brain activity (for review see Lewis et al., 2016). rTMS uses a magnetic field which is generated by brief current pulses in the coil placed on the scalp, while PAS combines sensory stimulation (e.g., electrical stimulation on the wrist extensor muscle, or auditory stimuli) and magnetic cortical stimulation to induce changes in cortical excitability. Electrical currents (usually up to 2 mA) are used to modulate brain activity between the two electrodes of the opposing polarity in constant (tDCS), altering (tACS) or noise waveform (tRNS) manner. Although the application of these methods has reportedly contributed to the positive outcomes in clinical states (e.g., see Lefaucheur et al., 2020), the effects induced in the brain are not fully understood. According to the growing number of meta-analyses, the efficacy of brain stimulation methods is inconsistent across different conditions, e.g., depression (Razza et al., 2020; Sonmez et al., 2019; Moffa et al., 2020), mild cognitive impairments and dementia (Vacas et al., 2019; Chou et al., 2020; Wang et al., 2020), anxiety and post-traumatic stress (Cirillo et al., 2019), autism spectrum disorders (Barahona-Corrêa et al., 2018), ADHD (Salehinejad et al., 2019), positive and negative symptoms of schizophrenia (Aleman et al., 2018; Kim et al., 2019; Yang et al., 2019). Further research could benefit from the combination of NIBS with different neurophysiological measures like ASSR.
Considering a growing interest in ASSR as a biomarker of neuropsychiatric disorders, it is important to evaluate the effects that NIBS induces on gamma-range ASSRs. Despite its promise for clinical application, this line of research has been pursued sporadically so far. Due to a small number of studies, a comprehensive systematic review is not possible at this time. We attempt to summarize the existing research on the neuromodulation of ASSRs, describe the common patterns found, and define further directions for research on neuromodulation of gamma-range ASSRs.
Online-searches were performed in PubMed and ScienceDirect databases for the keywords “auditory steady-state response,” “auditory steady-state evoked potential,” “auditory entrainment” in combination with “neurostimulation,” “neuromodulation,” “tDCS,” “tACS,” “TES” (transcranial electrical stimulation), “ECT” (electroconvulsive therapy), “TMS.” Potentially relevant articles were also identified by a manual search among the reference lists of included articles. Titles and abstracts were scanned by authors (KS and IG-B) to meet the selection criteria. When the abstract provided insufficient information, the “Materials and Methods” section of the article was reviewed. When a disagreement on the inclusion arose, the last author’s (JB) opinion was sought.
The following inclusion criteria were used: (1) EEG/MEG with gamma-range (30–80 Hz) auditory stimulation; (2) statistical comparison of ASSR measures pre- to post-stimulation; (3) original research articles. Articles published in non-English languages and animal studies were excluded. When articles were not accessible as a full text or lacking necessary information, efforts were made to retrieve missing data by contacting the authors.
From each study, we extracted: (1) sample (type, size, age, gender composition); (2) brain stimulation technique and relevant parameters (electrode/coil position, settings, duration, number of sessions); (3) control group/condition; (4) auditory stimulation settings (stimulation frequencies, stimulation type, number of repetitions, duration); and (5) EEG assessment (site, measures evaluated).
In total nine articles were included in the review (Table 1, study numbers are further used as references). The majority of studies assessed basic mechanisms and were conducted on young healthy subjects. Only three studies included patient samples—schizophrenia patients were studied in one tACS/tDCS research (St1), and tinnitus patients—in two rTMS studies (St6-7). Overall, both tACS (St1-2, 5) and tDCS (St8-9) were used in three studies each [tDCS acted as a control condition for tACS in one study (St1)]; in addition, one study employed tRNS (St3). The remaining three studies (St4, 6–7) used a variety of TMS-based protocols. The sham condition was used as a control in all except one (St2) of included articles. Additionally, three studies (St1-2, 7) included a different stimulation approach to serve as an active control condition for the specific hypothesis testing. A healthy control group was used only in one (St6) out of three studies in clinical populations. The clinically/behaviorally relevant domains were assessed in four articles, i.e. hallucination prevalence (St1), tinnitus-related impairment (St6-7), and gap detection performance (St2).
Table 1. Summary of brain stimulation effects on auditory steady-state responses and methodological descriptions for the included studies.
Five studies (St5-9) evaluated MEG as an outcome measure. 40-Hz ASSR was assessed in five reports (St1, 3, 4, 7–9); three studies utilized near 40 Hz stimulation (individual gamma frequencies around 50 Hz (St1); 41 (St5) or 37 Hz (St6)); additionally, responses to 20 Hz (St1, 3–4) and 10, 30 and 80 Hz (St1) stimulation were evaluated. Monaural presentation of sounds was used only in two tinnitus-oriented reports (St6-7); remaining studies employed binaural stimulation. The duration of auditory stimuli varied from 180 ms to the continuous sound presentation and only three studies (St1, 5, 8) utilized stimulation with click trains. All included studies focused on either amplitude or power measures of the ASSRs; three studies (St1, 8–9) additionally evaluated phase-locking properties of the response (inter-trial phase coherence, ITPC). Activity at the source level was assessed in three MEG-based works (St5-6, 9); remaining articles reported the measures at the region-of-interest basis. The effects induced by the course of stimulation (5 daily sessions) with tACS (St1) and TMS (St6) were estimated in two studies; single or double daily stimulation was performed in the remaining reports.
Of the reviewed articles, two tACS reports (St1-2) and one tRNS-based report (St3) observed 40-Hz ASSR increase after the stimulation, and one study (St5) reported decreased 41-Hz ASSR after tACS. Among the included studies, two utilized tACS at the alpha frequency (10 Hz (St1) and 12 Hz (St5)); however, both reports produced contradictory results on 40-Hz ASSR. Three studies did not find a tDCS effect on the power of 40-Hz ASSR (St1, 8–9); however, in one study a drop of phase-locking after stimulation was reported (St9). TMS-based protocols resulted in a decrease of ASSRs at 20 (St4), 37 (St6), and 40 Hz (St7).
We assessed available studies addressing the effects of neuromodulatory/brain stimulation approaches on the gamma-range auditory steady-state responses and summarized their results.
Among the most consistent results are two reports on the rTMS-induced reduction of 40-Hz ASSR in tinnitus (Lorenz et al., 2010; Li et al., 2019), where rTMS potentially causes long-term depression of synaptic transmission in the auditory cortex (Li et al., 2019). Lorenz et al. (2010) reported significantly attenuated ASSRs along with tinnitus loudness in patients after both 1 Hz rTMS and continuous theta burst rTMS (Lorenz et al., 2010). In support of this, a recent study of Li et al. (2019) provided evidence for the long-term effect of rTMS on both ASSRs and tinnitus reduction (Li et al., 2019). In healthy participants (Engel et al., 2017), no significant changes were observed for 40-Hz ASSRs after the PAS session where TMS and auditory stimuli were delivered 45 ms apart; however, 20-Hz ASSR was decreased pointing to spike-timing-dependent plasticity as a mechanism of action. Collectively, these findings suggest that rTMS might be potentially used in tinnitus where initial gamma-range ASSRs are elevated; yet, longitudinal studies are needed for more powerful evidence.
Spike-timing-dependent plasticity is proposed as a mode of action of tACS (Tavakoli and Yun, 2017). Although gamma-range tACS has been used to modulate various behavioral aspects in both visual and auditory domains (Rufener et al., 2016; Gonzalez-Perez et al., 2019), surprisingly, no studies published in full to date evaluated the effect of periodic 40 Hz electrical stimulation on the periodic 40 Hz brain responses. In the conference abstract by Knott et al. (2019) (not included in the review due to insufficient information), authors provide initial evidence that no effects of 40 Hz tACS (vs. sham tACS) on 40-Hz ASSRs could be observed (Knott et al., 2019). However, among reviewed studies, Baltus et al. (2018) reported an increase of the gamma-range responses at the stimulation frequencies around 50 Hz compared to individual estimated responses during the rest. Overall, increasing evidence suggests that tACS may modulate cross-frequency interactions (Herrmann et al., 2016). Accordingly, among the reviewed studies, two utilized tACS at lower frequencies—theta (6.5 Hz tACS) and alpha (10 and 12 Hz tACS)—to estimate the role of low-frequency oscillations in the modulation of the 40-Hz ASSRs (Hyvärinen et al., 2018; Ahn et al., 2019). Hyvärinen et al. (2018) suggested that tACS at alpha frequency could induce immediate inhibition of 40-Hz ASSRs. In line with this assumption, the authors observed reduced 40-Hz ASSRs in healthy subjects after a single session of bilateral 12 Hz tACS compared to sham condition. On the contrary, Ahn et al. (2019) expected 10 Hz tACS to enhance 40-Hz ASSRs in schizophrenia patients. The observed augmentation after the course of left-sided stimulation administered for 5 days was linked to the increase in functional connectivity and the reduction in auditory hallucinations. However, a course of five left-sided tACS sessions did not result in evident aftereffects at the 1-week and 1-month follow-up (Ahn et al., 2019), suggesting induced changes being short-lived. Importantly, no significant difference was found in ASSR under the bilateral 6.5 Hz tACS compared to sham in the study by Hyvärinen et al. (2018), and the difference in ASSR change between 12 Hz tACS and 6.5 Hz stimulation conditions was not significant. Nevertheless, in the preliminary report of Knott et al. (2019), bilateral 6 Hz tACS resulted in a significant reduction of amplitude and phase-locking of 40-Hz ASSRs (Knott et al., 2019). In contrast, a single study employing rRNS at 101–640 Hz, resulted in a significant increase of 40-Hz ASSRs when compared to the baseline (Van Doren et al., 2014). These observations suggest the potential for theta, alpha and high-frequency tACS protocols to modulate gamma-range ASSRs. The effect could be due to the direct action on underlying networks (Tavakoli and Yun, 2017) and/or modulation of attention towards auditory stimuli (Hopfinger et al., 2017; Wöstmann et al., 2018), as ASSRs are sensitive to variations in attention level (Skosnik et al., 2007; Griskova-Bulanova et al., 2011). However, the effects of tACS on attention are still unclear, thus this possibility should be addressed in future studies: experimental designs with attentional distraction or audio-visual conflict paradigm may provide insight into top-down vs. bottom-up effects of NIBS on ASSRs.
The effect of tDCS on gamma-range ASSRs, acting through long-term potentiation and long-term depression (LTP/LTD)-like neuroplasticity (Roche et al., 2015), was assessed in three recent studies (Miyagishi et al., 2018; Ahn et al., 2019; Pellegrino et al., 2019). Ahn et al. (2019) employed a left-sided tDCS as a control condition for tACS in schizophrenia patients, expecting no effect of tDCS on 40-Hz ASSRs; on the contrary, Miyagishi et al. (2018) and Pellegrino et al. (2019) anticipated modulation of 40-Hz ASSRs in healthy subjects. Both Ahn et al. (2019) and Miyagishi et al. (2018) did not find changes of ASSR after the stimulation, whereas Pellegrino et al. (2019) reported a decrease in phase-locking of 40-Hz ASSRs in the right temporal cortex without a significant influence on response power (Pellegrino et al., 2019) after a single session of tDCS over the central region. These findings point to the potential site-specific and measure-specific effects of tDCS.
Altogether, it seems that different neuromodulatory techniques can produce physiologically relevant changes in ASSRs. However, this rather new line of research should adopt standardization of brain stimulation protocols (e.g., for TMS see Lefaucheur et al., 2020) to avoid some of the most common issues in NIBS research. First, the use of sham-controlled designs is essential; even though some sham-protocols were shown to produce effects beyond intended sensational masking (for discussion see Duecker and Sack, 2015; Fonteneau et al., 2019; Zis et al., 2020), the success in blinding procedures and any unexpected sham-effects should be reported. Second, the consistency of stimulation protocols applied is needed to increase reproducibility. So far, frontal (Miyagishi et al., 2018; Ahn et al., 2019), sensory-motor (Pellegrino et al., 2019) and temporal (Hyvärinen et al., 2018) areas were stimulated to achieve modulation of ASSRs; however, the primary modulation target for auditory responses should be the auditory cortex. As 40-Hz ASSRs are somewhat asymmetric across the hemispheres, with a right-hemispheric dominance (Ross et al., 2005; Draganova et al., 2008) in healthy subjects, and diminished left-right hemispheric asymmetry in schizophrenia and bipolar disorder (Teale et al., 2003; Reite et al., 2009; Tsuchimoto et al., 2011), the bipolar homolog positioning of the electrodes for TES and TMS coil navigation following MRI-based atlases (Jiang et al., 2020) could be an appropriate starting point. If targeting at temporal lobes is ineffective, other areas beyond auditory pathways should be considered (see Farahani et al., 2017, 2019). Third, sample sizes in the reviewed studies are relatively small (10–26 participants) with no a priori or a posteriori power estimations provided. A priori power analysis (e.g., using free tools such as G * Power; Faul et al., 2007) and, where appropriate, adoption of within-subject study design to control the interindividual differences (Li et al., 2015) should help to achieve higher reproducibility. Furthermore, it must be noted that only four studies (Lorenz et al., 2010; Baltus et al., 2018; Ahn et al., 2019; Li et al., 2019) evaluated behavior output alongside EEG/MEG assessment. The majority of neuromodulation studies use either physiological or behavioral outputs; however, the concurrent monitoring of neurophysiological and behavioral performance is necessary to gain a better understanding of NIBS effects (Abellaneda-Pérez et al., 2020). Moreover, even though tACS and TMS show a great promise, it remains unclear which NIBS technique most effectively modulates neural circuits underlying ASSRs; thus, future studies should comparatively assess the effects of multiple brain stimulation methods (Ahn et al., 2019). Finally, only two studies (Ahn et al., 2019; Li et al., 2019) assessed long-term changes after the stimulation course; future research should investigate longitudinal effects on the neural activity, their duration, and potential functional side-effects.
There is a limited amount of research analyzing the impact of neuromodulation on gamma-range ASSRs. It has been shown that ASSRs can be either enhanced, decreased, or stay unaffected following NIBS with inconsistent findings both within and across different stimulation approaches. The research on rTMS has shown promising results in regards to the reduction of ASSRs in tinnitus; however, future research should aim to provide more evidence on the long-term effects of rTMS. Among TES protocols, tACS at theta, alpha, and high frequencies showed the potential to modulate gamma-range ASSRs. However, future investigations are necessary to explore the mechanisms underlying the modulation of gamma-range ASSRs to develop the non-invasive brain stimulation-based treatments for abnormal auditory processing. A standardization of brain stimulation protocols and ASSR assessment approaches, more rigorous study designs (including adequate control condition, a priori power-based determination of sample sizes, minimizing and controlling for potential confounding factors, etc.) as well as inclusion of a wide range of outcome measures to account for unexpected findings, would be beneficial for this research field.
IG-B and JB designed the work. KS and IG-B analyzed the data. IG-B and KS drafted the manuscript. JB critically reviewed the manuscript. All authors approved the manuscript.
This article is based upon the work from COST Action CA18106 Neural architecture of consciousness, supported by COST (European Cooperation in Science and Technology). IG-B was supported by the Research Council of Lithuania (LMTLT, agreement No. S-LJB-20-1). JB receives institutional support from Ministry of Education, Science and Technological Development of the Republic of Serbia (contract: 451-03-68/2020-14/200015).
The authors declare that the research was conducted in the absence of any commercial or financial relationships that could be construed as a potential conflict of interest.
Abellaneda-Pérez, K., Vaqué-Alcázar, L., Perellón-Alfonso, R., Bargalló, N., Kuo, M.-F., Pascual-Leone, A., et al. (2020). Differential tDCS and tACS effects on working memory-related neural activity and resting-state connectivity. Front. Neurosci. 13:1440. doi: 10.3389/fnins.2019.01440
Ahn, S., Mellin, J. M., Alagapan, S., Alexander, M. L., Gilmore, J. H., Jarskog, L. F., et al. (2019). Targeting reduced neural oscillations in patients with schizophrenia by transcranial alternating current stimulation. NeuroImage 186, 126–136. doi: 10.1016/j.neuroimage.2018.10.056
Aleman, A., Enriquez-Geppert, S., Knegtering, H., and Dlabac-de Lange, J. J. (2018). Moderate effects of noninvasive brain stimulation of the frontal cortex for improving negative symptoms in schizophrenia: meta-analysis of controlled trials. Neurosci. Biobehav. Rev. 89, 111–118. doi: 10.1016/j.neubiorev.2018.02.009
Arikan, M. K., Metin, B., and Tarhan, N. (2018). EEG gamma synchronization is associated with response to paroxetine treatment. J. Affect. Disord. 235, 114–116. doi: 10.1016/j.jad.2018.04.041
Baltus, A., Wagner, S., Wolters, C. H., and Herrmann, C. S. (2018). Optimized auditory transcranial alternating current stimulation improves individual auditory temporal resolution. Brain Stimulat. 11, 118–124. doi: 10.1016/j.brs.2017.10.008
Barahona-Corrêa, J. B., Velosa, A., Chainho, A., Lopes, R., and Oliveira-Maia, A. J. (2018). Repetitive transcranial magnetic stimulation for treatment of autism spectrum disorder: a systematic review and meta-analysis. Front. Integr. Neurosci. 12:27. doi: 10.3389/fnint.2018.00027
Bosman, C. A., Lansink, C. S., and Pennartz, C. M. A. (2014). Functions of gamma-band synchronization in cognition: from single circuits to functional diversity across cortical and subcortical systems. Eur. J. Neurosci. 39, 1982–1999. doi: 10.1111/ejn.12606
Brenner, C. A., Krishnan, G. P., Vohs, J. L., Ahn, W.-Y., Hetrick, W. P., Morzorati, S. L., et al. (2009). Steady state responses: electrophysiological assessment of sensory function in schizophrenia. Schizophr. Bull. 35, 1065–1077. doi: 10.1093/schbul/sbp091
Cinel, C., Valeriani, D., and Poli, R. (2019). Neurotechnologies for human cognitive augmentation: current state of the art and future prospects. Front. Hum. Neurosci. 13:13. doi: 10.3389/fnhum.2019.00013
Cirillo, P., Gold, A. K., Nardi, A. E., Ornelas, A. C., Nierenberg, A. A., Camprodon, J., et al. (2019). Transcranial magnetic stimulation in anxiety and trauma-related disorders: a systematic review and meta-analysis. Brain Behav. 9:e01284. doi: 10.1002/brb3.1284
Chou, Y., Ton That, V., and Sundman, M. (2020). A systematic review and meta-analysis of rTMS effects on cognitive enhancement in mild cognitive impairment and Alzheimer’s disease. Neurobiol. Aging 86, 1–10. doi: 10.1016/j.neurobiolaging.2019.08.020
Draganova, R., Ross, B., Wollbrink, A., and Pantev, C. (2008). Cortical steady-state responses to central and peripheral auditory beats. Cereb. Cortex N. Y. N 18, 1193–1200. doi: 10.1093/cercor/bhm153
Duecker, F., and Sack, A. T. (2015). Rethinking the role of sham TMS. Front. Psychol. 6:210. doi: 10.3389/fpsyg.2015.00210
Edgar, J. C., Fisk, C. L. IV., Liu, S., Pandey, J., Herrington, J. D., Schultz, R. T., et al. (2016). Translating adult electrophysiology findings to younger patient populations: difficulty measuring 40-Hz auditory steady-state responses in typically developing children and children with autism spectrum disorder. Dev. Neurosci. 38, 1–14. doi: 10.1159/000441943
Engel, S., Markewitz, R. D. H., Langguth, B., and Schecklmann, M. (2017). Paired associative stimulation of the temporal cortex: effects on the auditory steady-state response. Front. Psychiatry 8:227. doi: 10.3389/fpsyt.2017.00227
Farahani, E. D., Goossens, T., Wouters, J., and van Wieringen, A. (2017). Spatiotemporal reconstruction of auditory steady-state responses to acoustic amplitude modulations: potential sources beyond the auditory pathway. NeuroImage 148, 240–253. doi: 10.1016/j.neuroimage.2017.01.032
Farahani, E. D., Wouters, J., and van Wieringen, A. (2019). Contributions of non-primary cortical sources to auditory temporal processing. NeuroImage 191, 303–314. doi: 10.1016/j.neuroimage.2019.02.037
Faul, F., Erdfelder, E., Lang, A.-G., and Buchner, A. (2007). G*Power 3: a flexible statistical power analysis program for the social, behavioral and biomedical sciences. Behav. Res. Methods 39, 175–191. doi: 10.3758/bf03193146
Fonteneau, C., Mondino, M., Arns, M., Baeken, C., Bikson, M., Brunoni, A. R., et al. (2019). Sham tDCS: a hidden source of variability? Reflections for further blinded, controlled trials. Brain Stimulat. 12, 668–673. doi: 10.1016/j.brs.2018.12.977
Galambos, R., Makeig, S., and Talmachoff, P. J. (1981). A 40-Hz auditory potential recorded from the human scalp. Proc. Natl. Acad. Sci. U. S. A. 78, 2643–2647. doi: 10.1073/pnas.78.4.2643
Goff, D. C., Hill, M., and Barch, D. (2011). The treatment of cognitive impairment in schizophrenia. Pharmacol. Biochem. Behav. 99, 245–253. doi: 10.1016/j.pbb.2010.11.009
Gonzalez-Perez, M., Wakui, E., Thoma, V., Nitsche, M. A., and Rivolta, D. (2019). Transcranial alternating current stimulation (tACS) at 40 Hz enhances face and object perception. Neuropsychologia 135:107237. doi: 10.1016/j.neuropsychologia.2019.107237
Grent-’t-Jong, T., Gross, J., Goense, J., Wibral, M., Gajwani, R., Gumley, A. I., et al. (2018). Resting-state gamma-band power alterations in schizophrenia reveal E/I-balance abnormalities across illness-stages. eLife 7:e37799. doi: 10.7554/eLife.37799
Griskova-Bulanova, I., Dapsys, K., and Maciulis, V. (2013). Does brain ability to synchronize with 40 Hz auditory stimulation change with age? Acta Neurobiol. Exp. 73, 564–570.
Griskova-Bulanova, I., Ruksenas, O., Dapsys, K., Maciulis, V., and Arnfred, S. M. H. (2011). Distraction task rather than focal attention modulates gamma activity associated with auditory steady-state responses (ASSRs). Clin. Neurophysiol. 122, 1541–1548. doi: 10.1016/j.clinph.2011.02.005
Herdman, A. T., Lins, O., Van Roon, P., Stapells, D. R., Scherg, M., and Picton, T. W. (2002). Intracerebral sources of human auditory steady-state responses. Brain Topogr. 15, 69–86. doi: 10.1023/a:1021470822922
Herrmann, C. S., and Demiralp, T. (2005). Human EEG γ oscillations in neuropsychiatric disorders. Clin. Neurophysiol. 116, 2719–2733. doi: 10.1016/j.clinph.2005.07.007
Herrmann, C. S., Strüber, D., Helfrich, R. F., and Engel, A. K. (2016). EEG oscillations: from correlation to causality. Int. J. Psychophysiol. 103, 12–21. doi: 10.1016/j.ijpsycho.2015.02.003
Holtzheimer, P. E., Kosel, M., and Schlaepfer, T. (2012). Brain stimulation therapies for neuropsychiatric disease. Handb. Clin. Neurol. 106, 681–695. doi: 10.1016/B978-0-444-52002-9.00041-3
Hopfinger, J. B., Parsons, J., and Fröhlich, F. (2017). Differential effects of 10-Hz and 40-Hz transcranial alternating current stimulation (tACS) on endogenous versus exogenous attention. Cogn. Neurosci. 8, 102–111. doi: 10.1080/17588928.2016.1194261
Hyvärinen, P., Choi, D., Demarchi, G., Aarnisalo, A. A., and Weisz, N. (2018). tACS-mediated modulation of the auditory steady-state response as seen with MEG. Hear. Res. 364, 90–95. doi: 10.1016/j.heares.2018.03.023
Ishii, R., Nishida, K., Youssef, N. A., Jann, K., and Takahashi, S. (2019). Editorial: neuromodulation in basic, translational and clinical research in psychiatry. Front. Hum. Neurosci. 13:438. doi: 10.3389/fnhum.2019.00438
Jiang, Y., Li, Z., Zhao, Y., Xiao, X., Zhang, W., Sun, P., et al. (2020). Targeting brain functions from the scalp: transcranial brain atlas based on large-scale fMRI data synthesis. NeuroImage 210:116550. doi: 10.1016/j.neuroimage.2020.116550
Khalid, A., Kim, B. S., Seo, B. A., Lee, S.-T., Jung, K.-H., Chu, K., et al. (2016). Gamma oscillation in functional brain networks is involved in the spontaneous remission of depressive behavior induced by chronic restraint stress in mice. BMC Neurosci. 17:4. doi: 10.1186/s12868-016-0239-x
Kim, J., Iwata, Y., Plitman, E., Caravaggio, F., Chung, J. K., Shah, P., et al. (2019). A meta-analysis of transcranial direct current stimulation for schizophrenia: “is more better?”. J. Psychiatr. Res. 110, 117–126. doi: 10.1016/j.jpsychires.2018.12.009
Knott, V., Payumo, M., Hyde, M., Nelson, R., Duncan, B., Devlin, M., et al. (2019). The effects of transcranial alternating current stimulation on the auditory steady-state response and its association with schizotypy. Brain Stimul. 12:506. doi: 10.1016/j.brs.2018.12.659
Koshiyama, D., Kirihara, K., Tada, M., Nagai, T., Fujioka, M., Ichikawa, E., et al. (2018a). Auditory gamma oscillations predict global symptomatic outcome in the early stages of psychosis: a longitudinal investigation. Clin. Neurophysiol. 129, 2268–2275. doi: 10.1016/j.clinph.2018.08.007
Koshiyama, D., Kirihara, K., Tada, M., Nagai, T., Fujioka, M., Ichikawa, E., et al. (2018b). Electrophysiological evidence for abnormal glutamate-GABA association following psychosis onset. Transl. Psychiatry 8:211. doi: 10.1038/s41398-018-0261-0
Lefaucheur, J.-P., Aleman, A., Baeken, C., Benninger, D. H., Brunelin, J., Di Lazzaro, V., et al. (2020). Evidence-based guidelines on the therapeutic use of repetitive transcranial magnetic stimulation (rTMS): an update (2014–2018). Clin. Neurophysiol. 131, 474–528. doi: 10.1016/j.clinph.2019.11.002
Lewis, P. M., Thomson, R. H., Rosenfeld, J. V., and Fitzgerald, P. B. (2016). Brain neuromodulation techniques: a review. Neuroscientist 22, 406–421. doi: 10.1177/1073858416646707
Li, L. P.-H., Shiao, A.-S., Li, C.-T., Lee, P.-L., Cheng, C.-M., Chou, C.-C., et al. (2019). Steady-state auditory evoked fields reflect long-term effects of repetitive transcranial magnetic stimulation in tinnitus. Clin. Neurophysiol. 130, 1665–1672. doi: 10.1016/j.clinph.2019.05.022
Li, L. M., Uehara, K., and Hanakawa, T. (2015). The contribution of interindividual factors to variability of response in transcranial direct current stimulation studies. Front. Cell. Neurosci. 9:181. doi: 10.3389/fncel.2015.00181
Lorenz, I., Müller, N., Schlee, W., Langguth, B., and Weisz, N. (2010). Short-term effects of single repetitive TMS sessions on auditory evoked activity in patients with chronic tinnitus. J. Neurophysiol. 104, 1497–1505. doi: 10.1152/jn.00370.2010
McNally, J. M., and McCarley, R. W. (2016). Gamma band oscillations: a key to understanding schizophrenia symptoms and neural circuit abnormalities. Curr. Opin. Psychiatry 29, 202–210. doi: 10.1097/yco.0000000000000244
Minzenberg, M. J., Yoon, J. H., Cheng, Y., and Carter, C. S. (2016). Sustained modafinil treatment effects on control-related gamma oscillatory power in schizophrenia. Neuropsychopharmacology 41, 1231–1240. doi: 10.1038/npp.2015.271
Miyagishi, Y., Ikeda, T., Takahashi, T., Kudo, K., Morise, H., Minabe, Y., et al. (2018). Gamma-band auditory steady-state response after frontal tDCS: a double-blind, randomized, crossover study. PLoS One 13:e0193422. doi: 10.1371/journal.pone.0193422
Moffa, A. H., Martin, D., Alonzo, A., Bennabi, D., Blumberger, D. M., Benseñor, I. M., et al. (2020). Efficacy and acceptability of transcranial direct current stimulation (tDCS) for major depressive disorder: an individual patient data meta-analysis. Prog. Neuropsychopharmacol. Biol. Psychiatry 99:109836. doi: 10.1016/j.pnpbp.2019.109836
Nugent, A. C., Ballard, E. D., Gould, T. D., Park, L. T., Moaddel, R., Brutsche, N. E., et al. (2019). Ketamine has distinct electrophysiological and behavioral effects in depressed and healthy subjects. Mol. Psychiatry 24, 1040–1052. doi: 10.1038/s41380-018-0028-2
O’Donnell, B. F., Vohs, J. L., Krishnan, G. P., Rass, O., Hetrick, W. P., and Morzorati, S. L. (2013). The auditory steady-state response (ASSR): a translational biomarker for schizophrenia. Suppl. Clin. Neurophysiol. 62, 101–112. doi: 10.1016/b978-0-7020-5307-8.00006-5
Pan, Z., Grovu, R. C., Cha, D. S., Carmona, N. E., Subramaniapillai, M., Shekotikhina, M., et al. (2017). Pharmacological treatment of cognitive symptoms in major depressive disorder. CNS Neurol. Disord. Drug Targets 16, 891–899. doi: 10.2174/1871527316666170919115100
Pellegrino, G., Arcara, G., Pino, G. D., Turco, C., Maran, M., Weis, L., et al. (2019). Transcranial direct current stimulation over the sensory-motor regions inhibits gamma synchrony. Hum. Brain Mapp. 40, 2736–2746. doi: 10.1002/hbm.24556
Presacco, A., Bohórquez, J., Yavuz, E., and Özdamar, Ö. (2010). Auditory steady-state responses to 40-Hz click trains: relationship to middle latency, gamma band beta band responses studied with deconvolution. Clin. Neurophysiol. 121, 1540–1550. doi: 10.1016/j.clinph.2010.03.020
Razza, L. B., Palumbo, P., Moffa, A. H., Carvalho, A. F., Solmi, M., Loo, C. K., et al. (2020). A systematic review and meta-analysis on the effects of transcranial direct current stimulation in depressive episodes. Depress. Anxiety doi: 10.1002/da.23004 [Epub ahead of print].
Reite, M., Teale, P., Rojas, D. C., Reite, E., Asherin, R., Hernandez, O.. (2009). MEG auditory evoked fields suggest altered structural/functional asymmetry in primary but not secondary auditory cortex in bipolar disorder. Bipolar Disord. 11, 371–381. doi: 10.1111/j.1399-5618.2009.00701.x
Robbins, T. W. (2019). Pharmacological treatment of cognitive deficits in nondementing mental health disorders. Dialogues Clin. Neurosci. 21, 301–308. doi: 10.31887/dcns.2019.21.3/trobbins
Roche, N., Geiger, M., and Bussel, B. (2015). Mechanisms underlying transcranial direct current stimulation in rehabilitation. Ann. Phys. Rehabil. Med. 58, 214–219. doi: 10.1016/j.rehab.2015.04.009
Ross, B., Herdman, A. T., and Pantev, C. (2005). Stimulus induced desynchronization of human auditory 40-Hz steady-state responses. J. Neurophysiol. 94, 4082–4093. doi: 10.1152/jn.00469.2005
Rufener, K. S., Zaehle, T., Oechslin, M. S., and Meyer, M. (2016). 40 Hz-Transcranial alternating current stimulation (tACS) selectively modulates speech perception. Int. J. Psychophysiol. 101, 18–24. doi: 10.1016/j.ijpsycho.2016.01.002
Salehinejad, M. A., Wischnewski, M., Nejati, V., Vicario, C. M., and Nitsche, M. A. (2019). Transcranial direct current stimulation in attention-deficit hyperactivity disorder: a meta-analysis of neuropsychological deficits. PLoS One 14:e0215095. doi: 10.1371/journal.pone.0215095
Santarelli, R., Maurizi, M., Conti, G., Ottaviani, F., Paludetti, G., and Pettorossi, V. E. (1995). Generation of human auditory steady-state responses (SSRs). II: addition of responses to individual stimuli. Hear. Res. 83, 9–18. doi: 10.1016/0378-5955(94)00185-s
Sivarao, D. V., Chen, P., Senapati, A., Yang, Y., Fernandes, A., Benitex, Y., et al. (2016). 40 Hz auditory steady-state response is a pharmacodynamic biomarker for cortical NMDA receptors. Neuropsychopharmacology 41, 2232–2240. doi: 10.1038/npp.2016.17
Skosnik, P. D., Krishnan, G. P., and O’Donnell, B. F. (2007). The effect of selective attention on the gamma-band auditory steady-state response. Neurosci. Lett. 420, 223–228. doi: 10.1016/j.neulet.2007.04.072
Sonmez, A. I., Camsari, D. D., Nandakumar, A. L., Voort, J. L. V., Kung, S., Lewis, C. P., et al. (2019). Accelerated TMS for depression: a systematic review and meta-analysis. Psychiatry Res. 273, 770–781. doi: 10.1016/j.psychres.2018.12.041
Sullivan, E. M., Timi, P., Hong, L. E., and O’Donnell, P. (2015). Effects of NMDA and GABA-A receptor antagonism on auditory steady-state synchronization in awake behaving rats. Int. J. Neuropsychopharmacol. 18:pyu118. doi: 10.1093/ijnp/pyu118
Tada, M., Kirihara, K., Koshiyama, D., Fujioka, M., Usui, K., Uka, T., et al. (2019). Gamma-band auditory steady-state response as a neurophysiological marker for excitation and inhibition balance: a review for understanding schizophrenia and other neuropsychiatric disorders. Clin. EEG Neurosci. doi: 10.1177/1550059419868872 [Epub ahead of print].
Tada, M., Nagai, T., Kirihara, K., Koike, S., Suga, M., Araki, T., et al. (2016). Differential alterations of auditory gamma oscillatory responses between pre-onset high-risk individuals and first-episode schizophrenia. Cereb. Cortex 26, 1027–1035. doi: 10.1093/cercor/bhu278
Tavakoli, A. V., and Yun, K. (2017). Transcranial alternating current stimulation (tACS) mechanisms and protocols. Front. Cell. Neurosci. 11:214. doi: 10.3389/fncel.2017.00214
Teale, P., Carlson, J., Rojas, D., and Reite, M. (2003). Reduced laterality of the source locations for generators of the auditory steady-state field in schizophrenia. Biol. Psychiatry 54, 1149–1153. doi: 10.1016/s0006-3223(03)00411-6
Thuné, H., Recasens, M., and Uhlhaas, P. J. (2016). The 40-Hz auditory steady-state response in patients with schizophrenia: a meta-analysis. JAMA Psychiatry 73, 1145–1153. doi: 10.1001/jamapsychiatry.2016.2619
Tsuchimoto, R., Kanba, S., Hirano, S., Oribe, N., Ueno, T., Hirano, Y. et al. (2011). Reduced high and low frequency gamma synchronization in patients with chronic schizophrenia. Schizophr. Res. 133, 99–105. doi: 10.1016/j.schres.2011.07.020
Uhlhaas, P. J., Pipa, G., Neuenschwander, S., Wibral, M., and Singer, W. (2011). A new look at gamma? High- (>60 Hz) γ-band activity in cortical networks: function, mechanisms and impairment. Prog. Biophys. Mol. Biol. 105, 14–28. doi: 10.1016/j.pbiomolbio.2010.10.004
Uhlhaas, P., Pipa, G., Lima, B., Melloni, L., Neuenschwander, S., Nikolić, D., et al. (2009). Neural synchrony in cortical networks: history, concept and current status. Front. Integr. Neurosci. 3:17. doi: 10.3389/neuro.07.017.2009
Vacas, S. M., Stella, F., Loureiro, J. C., do Couto, F. S., Oliveira-Maia, A. J., and Forlenza, O. V. (2019). Noninvasive brain stimulation for behavioural and psychological symptoms of dementia: a systematic review and meta-analysis. Int. J. Geriatr. Psychiatry 34, 1336–1345. doi: 10.1002/gps.5003
Van Doren, J., Langguth, B., and Schecklmann, M. (2014). Electroencephalographic effects of transcranial random noise stimulation in the auditory cortex. Brain Stimulat. 7, 807–812. doi: 10.1016/j.brs.2014.08.007
Vohs, J. L., Chambers, R. A., Krishnan, G. P., O’Donnell, B. F., Berg, S., and Morzorati, S. L. (2010). GABAergic modulation of the 40 Hz auditory steady-state response in a rat model of schizophrenia. Int. J. Neuropsychopharmacol. 13, 487–497. doi: 10.1017/s1461145709990307
Wang, X., Mao, Z., and Yu, X. (2020). The role of noninvasive brain stimulation for behavioral and psychological symptoms of dementia: a systematic review and meta-analysis. Neurol. Sci. 41, 1063–1074. doi: 10.1007/s10072-020-04245-4
Wöstmann, M., Vosskuhl, J., Obleser, J., and Herrmann, C. S. (2018). Opposite effects of lateralised transcranial alpha versus gamma stimulation on auditory spatial attention. Brain Stimulat. 11, 752–758. doi: 10.1016/j.brs.2018.04.006
Yang, F., Fang, X., Tang, W., Hui, L., Chen, Y., Zhang, C., et al. (2019). Effects and potential mechanisms of transcranial direct current stimulation (tDCS) on auditory hallucinations: a meta-analysis. Psychiatry Res. 273, 343–349. doi: 10.1016/j.psychres.2019.01.059
Zis, P., Shafique, F., Hadjivassiliou, M., Blackburn, D., Venneri, A., Iliodromiti, S., et al. (2020). Safety, tolerability, and nocebo phenomena during transcranial magnetic stimulation: a systematic review and meta-analysis of placebo-controlled clinical trials. Neuromodulation Technol. Neural Interface 23, 291–300. doi: 10.1111/ner.12946
Keywords: auditory steady-state response (ASSR), gamma, brain stimulation, biomarker, neuropsychiatric disorders
Citation: Griskova-Bulanova I, Sveistyte K and Bjekic J (2020) Neuromodulation of Gamma-Range Auditory Steady-State Responses: A Scoping Review of Brain Stimulation Studies. Front. Syst. Neurosci. 14:41. doi: 10.3389/fnsys.2020.00041
Received: 02 April 2020; Accepted: 02 June 2020;
Published: 29 June 2020.
Edited by:
Michael Okun, University of Leicester, United KingdomReviewed by:
Robert A. Seymour, Macquarie University, AustraliaCopyright © 2020 Griskova-Bulanova, Sveistyte and Bjekic. This is an open-access article distributed under the terms of the Creative Commons Attribution License (CC BY). The use, distribution or reproduction in other forums is permitted, provided the original author(s) and the copyright owner(s) are credited and that the original publication in this journal is cited, in accordance with accepted academic practice. No use, distribution or reproduction is permitted which does not comply with these terms.
*Correspondence: Inga Griskova-Bulanova, aS5ncmlza292YUBnbWFpbC5jb20=; aW5nYS5ncmlza292YS1idWxhbm92YUBnZi52dS5sdA==
Disclaimer: All claims expressed in this article are solely those of the authors and do not necessarily represent those of their affiliated organizations, or those of the publisher, the editors and the reviewers. Any product that may be evaluated in this article or claim that may be made by its manufacturer is not guaranteed or endorsed by the publisher.
Research integrity at Frontiers
Learn more about the work of our research integrity team to safeguard the quality of each article we publish.