- School of Veterinary Medicine, University of Wisconsin-Madison, Madison, WI, United States
Bladder-related pain is one of the most common forms of visceral pain, and visceral pain is among the most common complaints for which patients seek physician consultation. Despite extensive studies of visceral innervation and treatment of visceral pain, opioids remain a mainstay for management of bladder pain. Side effects associated with opioid therapy can profoundly diminish quality of life, and improved options for treatment of bladder pain remain a high priority. Endocannabinoids, primarily anandamide (AEA) and 2-arachidonoylglycerol (2-AG), are endogenously-produced fatty acid ethanolamides with that induce analgesia. Animal experiments have demonstrated that inhibition of enzymes that degrade AEA or 2-AG have the potential to prevent development of visceral and somatic pain. Although experimental results in animal models have been promising, clinical application of this approach has proven difficult. In addition to fatty acid amide hydrolase (FAAH; degrades AEA) and monacylglycerol lipase (MAGL; degrades 2-AG), cyclooxygenase (COX) acts to metabolize endocannabinoids. Another potential limitation of this strategy is that AEA activates pro-nociceptive transient receptor potential vanilloid 1 (TRPV1) channels. Dual inhibitors of FAAH and TRPV1 or FAAH and COX have been synthesized and are currently undergoing preclinical testing for efficacy in providing analgesia. Local inhibition of FAAH or MAGL within the bladder may be viable options to reduce pain associated with cystitis with fewer systemic side effects, but this has not been explored. Further investigation is required before manipulation of the endocannabinoid system can be proven as an efficacious alternative for management of bladder pain.
Introduction
Despite the exceedingly common occurrence of visceral pain, far less is known about the anatomy and physiology that underlie visceral pain relative to that associated with somatic pain. An excellent recent review of the physiology of visceral pain pointed out that afferent innervation of viscera consists of “either vagal and spinal nerves or two anatomically distinct sets of spinal nerves” (Gebhart and Bielefeldt, 2016). The diffuse and somewhat sparse nature of afferent visceral innervation results in poorly localized discomfort that is often perceived as pain referred to somatic structures, possibly as a result of cross-communication between afferent visceral and somatic nerves as they comingle in peripheral ganglia, dorsal root ganglia, the spinal cord, or higher centers (Pierau et al., 1984; Arendt-Nielsen et al., 2000; Craig, 2003; Farrell et al., 2014; Luz et al., 2015; Lovick, 2016).
Patients with visceral pain thought to arise from specific organs, such as the bladder or bowel are treated with a variety of analgesics, including opioids, but failure to respond, alterations in pain sensitivity, decreased bowel motility and addiction are unfortunately common in these patients (Quang-Cantagrel et al., 2000; Brock et al., 2012; Wang et al., 2017; Weber et al., 2017). Alternative therapies such as electrostimulation of nerves, immunotherapy and homeopathic remedies have been used with mixed, but typically poor or transient, results in these patients (Farhadi et al., 2001; John et al., 2003; Capodice et al., 2005; Brock et al., 2008; Mykoniatis et al., 2017).
Treatment of visceral pain thought to arise specifically from the bladder has included instillation of compounds into the bladder or distention of the bladder. The efficacy of various intravesical treatments was recently reviewed (Zhang et al., 2017). This report observed that botulinum toxin A, bacillus Calmette-Guerin, and pentosan polysulfate showed the greatest promise. Distention of the bladder provides transient relief in some patients, but the mechanism for this remains unknown. Data in support of the efficacy of this treatment are relatively weak, and duration of positive effects are relatively short-lived (Erickson et al., 2007; Hoke et al., 2017; Olson et al., 2018). Translation of experimental findings generated in rodent models of acute or chronic bladder inflammation to clinical practice has proven difficult in patients with persistent bladder pain of long duration.
Recent developments in legalization of cannabis or cannabinoid products has increased interest in these compounds as an alternative therapy for pain. Systemic administration of exogenous cannabinoids to control pain appears to be most efficacious in patients with cancer-related pain (Tateo, 2017). The capacity of cannabinoids to decrease nausea and pain in cancer patients has been described by multiple authors, albeit often in the presence of side effects relating to altered mentation (Johnson et al., 2010; Abrams and Guzman, 2015; Davis, 2016). A recent meta-analysis found that pre-clinical studies using animal models of pain strongly supported the capacity of cannabinoids to reduce opioid doses, but clinical trials to date have failed to support this observation (Nielsen et al., 2017). Similarly, a meta-analysis of studies revealed that relief of non-cancer pain by cannabinoids was extremely weak and accompanied by significant side effects in these patients (Allende-Salazar and Rada, 2017). Short-term adverse side effects of smoked cannabis include anxiety, agitation, illusions, feelings of depersonalization, hallucinations, paranoid ideation, temporal slowing, impaired judgment/attention, red eyes, dryness of the mouth, tachycardia and increased appetite (Zhang and Ho, 2015), and occasionally, hyperemesis and intestinal perforation (Buyukbese Sarsu, 2016; Dezieck et al., 2017). An alternative to management of bladder pain by administration of exogenous cannabinoids is manipulation of endocannabinoids.
Endocannabinoid Metabolism
As the name implies, endocannabinoids are endogenously synthesized fatty acids with chemical structures similar to those of biologically active exogenous cannabinoids. Endocannabinoids are hydrophobic, neutral lipids that are not stored within cells but rather are produced on demand (Marsicano et al., 2003). Post-synaptic neurons rapidly synthesize endocannabinoids that bind to cannabinoid 1 (CB1) and 2 (CB2) receptors on presynaptic neurons to inhibit signaling; endocannabinoids are then metabolized by enzymes within presynaptic neurons (Piomelli et al., 1998; Alhouayek and Muccioli, 2012; Kohnz and Nomura, 2014). N-arachidonoyl ethanolamine (anandamide or AEA) and 2-arachidonoylglycerol (2-AG) are the most widely recognized and best characterized endocannabinoids, and 2-AG is the most abundant endocannabinoid identified to date (Palmer et al., 2002; Di Marzo et al., 2004; Kogan and Mechoulam, 2006). The presence and activity of endocannabinoids are tightly controlled by enzymatic degradation (Ahn et al., 2008); 2-AG is primarily degraded by monoacylglycerol lipase (MAGL) and AEA by fatty acid amide hydrolase (FAAH; Bisogno et al., 1997; Thomas et al., 1997; Goparaju et al., 1999). Strategies for testing management of visceral pain (and other types of pain) by endocannabinoids have primarily focused on inhibition of degradation of AEA or 2-AG by administration of compounds that inhibit the function of FAAH and MAGL or generation of mice lacking functional FAAH or MAGL.
Cyclooxygenase 1 and 2 (COX1 and COX2), but primarily COX2, also participate in degradation of AEA and 2-AG by oxygenation of these compounds to the corresponding PGH2 analogs (Kozak et al., 2000, 2001; Weber et al., 2004; Goodman et al., 2018). The primary function of COX is conversion of arachidonic acid to prostaglandins that promote inflammation and pain, and inhibition of COX has become standard first line therapy for acute inflammatory pain (Smith et al., 2000; Fleckenstein et al., 2016). However, COX antagonists alone do not adequately control visceral pain (Fleckenstein et al., 2016). Interestingly, it has been reported that nonsteroidal drugs that inhibit COX activity may also suppress the function of FAAH (Fowler et al., 1997, 1999), and intrathecal administration of COX inhibitors suppressed in vivo pain induced by subcutaneous formalin injection in the rat (Guhring et al., 2002; Ates et al., 2003), as well as release of calcitonin gene-related peptide (pro-algesic neuropeptide) in response to in vitro exposure of rat spinal cord to capsaicin (Seidel et al., 2003). The salient effects of COX antagonists in both studies were inhibited by administration of an antagonist to CB1. A dual inhibitor of FAAH and both isoforms of COX suppressed experimentally-induced gastrointestinal inflammation, as well as pain and edema due to subcutaneous injection of carrageenan in mice (Sasso et al., 2015). This compound is particularly interesting, because its chemical structure is such that it also has high efficacy in inhibition of degradation of 2-AG by COX (Goodman et al., 2018).
Cannabinoid Receptors
Endocannabinoids inhibit nociception primarily by binding to G-protein coupled receptors, CB1 and CB2, in peripheral tissue, spinal cord and brain. CB1 and CB2 have also been identified in the bladders of mice, rats, monkey and humans, primarily within the urothelium and afferent and cholinergic nerves within the bladder wall (Hayn et al., 2008; Gratzke et al., 2009, 2010; Tyagi et al., 2009; Merriam et al., 2011; Bakali et al., 2013, 2016; Wang et al., 2013). The function of CB1 and CB1 located on nerves has been extensively investigated, but the functional purpose of expression of cannabinoid receptors by non-neuronal tissue remains largely unknown. A summary of studies reporting localization of cannabinoid receptors in the bladder has been published (Hedlund, 2014).
CB1 is particularly abundant in the brain and plays a significant role in modulation of nociceptive signaling in the central and peripheral nervous systems (Wilson and Nicoll, 2002; Agarwal et al., 2007; Lau et al., 2014). A majority of somatic and visceral afferent nerve fibers express CB1 (Hohmann and Herkenham, 1999; Ahluwalia et al., 2000; Agarwal et al., 2007), and genetic deletion of CB1 from afferent nerves renders mice sensitive to subthreshold stimuli (allodynia) and enhances response to noxious stimuli (hyperalgesia; Agarwal et al., 2007). We demonstrated that CB1 receptors have the capacity to suppress the sensitizing effect of nerve growth factor (NGF) on the response of mouse afferent neurons to capsaicin in vitro (Wang et al., 2015a). Interestingly, we also showed that intrathecal administration of the CB1 agonist arachidonyl-2’-chloroethylamide (ACEA) blocked referred mechanical hypersensitivity induced by inflammatory cystitis in rats (Jones et al., 2015).
CB2 receptors are present on immune cells, as well as within the brain, spinal cord and peripheral afferent nerves (Galiegue et al., 1995; Ibrahim et al., 2003; Wotherspoon et al., 2005; Anand et al., 2009; Graham et al., 2010). Multiple studies have demonstrated analgesic effects of activation of CB2 in neuropathic or inflammatory pain models (Ibrahim et al., 2003; Nackley et al., 2004; Gutierrez et al., 2007; Anand et al., 2008). We have previously reported inhibition of increased referred mechanical sensitivity by systemic treatment of mice with CB2 agonists administered prior to Wang et al. (2013) or after Wang et al. (2014) induction of bladder inflammation. Thus, our prior work has demonstrated the capacity of activation of CB1 and/or CB2 receptors to block increased peripheral mechanical sensitivity accompanying inflammatory cystitis, and further that this may in part be due to suppression of the sensitizing effects of NGF on afferent nerve signaling.
Inhibition of FAAH
Suppression of FAAH activity by chemical inhibitors increases abundance of AEA in humans (Li et al., 2012) or mice (Ahn et al., 2011), and genetic deletion of functional FAAH in mice also increases concentrations of AEA (Cravatt et al., 2001; Lichtman et al., 2004). We observed increased AEA in bladders of FAAH-deficient (knock out or KO) mice, but abundance of 2-AG within the bladders of these mice was not affected in controls or by bladder inflammation (Wang et al., 2015b; Figure 1). Preclinical studies have demonstrated that increased AEA suppresses nociception in models of somatic and visceral pain when initiated prior to onset of pain (Cravatt et al., 2001; Lichtman et al., 2004; Merriam et al., 2011; Aizawa et al., 2016). We reported that severity of cyclophosphamide-induced bladder inflammation and associated referred pain was decreased in mice lacking FAAH (Wang et al., 2015b; Figure 2). We also found that systemic treatment of rats with established cystitis with a FAAH inhibitor given 1 h prior to testing diminished referred mechanical hypersensitivity (Merriam et al., 2011).
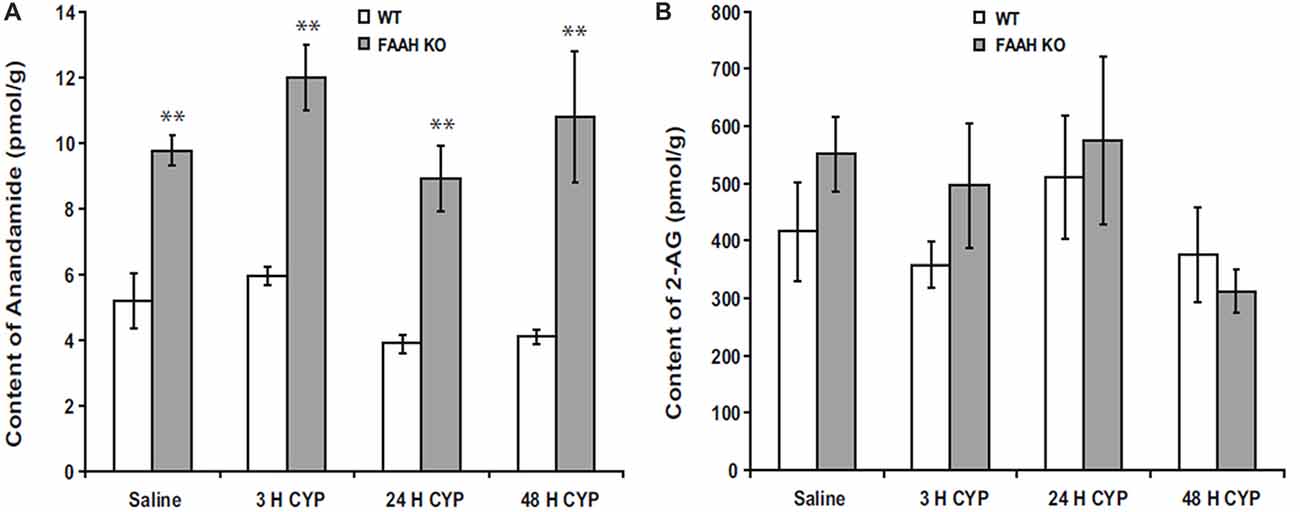
Figure 1. Bladder content of anandamide (AEA) (A) were consistently greater in fatty acid amide hydrolase (FAAH) knock out (KO) mice than wild-type (WT) mice treated with saline (controls) or cyclophosphamide (CYP; 150 mg/kg) given intraperitoneally 3 h prior to sacrifice. Bladder content of 2-arachidonoylglycerol (2-AG; B) was similar in both KO and WT and was unaffected by treatment. Mean ± SEM. **p < 0.01 KO vs. WT; n = 4 for each group. Reprinted by permission from the publisher of Journal of Molecular Neuroscience, Nature/Springer/Palgrave; (Wang et al., 2015b).
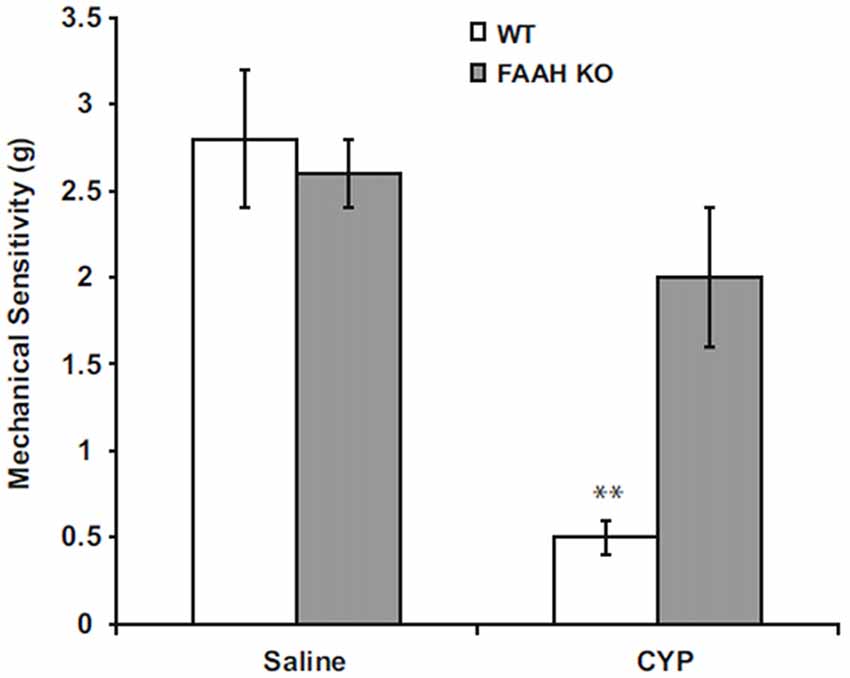
Figure 2. Peripheral mechanical sensitivity determined by application of von Frey monofilaments to hind paws 24 h after treatment with intraperitoneal saline (controls) or CYP (150 mg/kg). Mean ± SEM. **p < 0.01; vs. saline treated; n = 6–8. Reprinted by permission from the publisher of Journal of Molecular Neuroscience, Nature/Springer/Palgrave; (Wang et al., 2015b).
The analgesic effects of increased systemic AEA may be due to actions within the central nervous system (CNS) or peripheral tissues. Intravenous treatment of rats with URB937, a FAAH inhibitor with minimal penetration of the CNS decreased activity of bladder-specific afferent nerve in response to controlled filling of the bladder (Aizawa et al., 2014), and similar results were obtained by administration of URB937 prior to induction of cystitis by intravesical instillation of prostaglandin E2 (Aizawa et al., 2016).
AEA has been shown to be an agonist of the TRPV1 pro-nociceptive channel (Tognetto et al., 2001). The degree to which AEA activates TRPV1 varies among tissues and models of pain, but this effect has decreased enthusiasm for analgesic strategies entailing increased abundance of AEA alone. Simultaneous administration of inhibitors of FAAH and TRPV1, or compounds that act as antagonists against both, has produced promising, but mixed, results (Costa et al., 2010; de Novellis et al., 2011; Bashashati et al., 2017). This may be due in part to the fact that the relationship between CB1 and TRPV1 is far more complex than previously appreciated, and this interaction may be critically altered by tissue-specific metabolic factors (Fioravanti et al., 2008; Kim et al., 2008; De Petrocellis and Di Marzo, 2009).
Clinical trials of FAAH inhibitors failed to alleviate pain associated with osteoarthritis (Huggins et al., 2012). Of greater concern is a recent report that described serious adverse effects in a phase 1 trial of a FAAH inhibitor in humans, including coma and death in one subject and hospitalization of five other subjects, two with serious neurological symptoms (Mallet et al., 2016).
Inhibition of Monoacylglycerol Lipase (MAGL)
Although 2-AG is far more abundant than AEA, less work has been done investigating the therapeutic potential of inhibition of MAGL to ameliorate visceral pain. In a mouse chronic neurogenic pain model, MAGL inhibition alleviated neuropathic pain (Kinsey et al., 2009; Ignatowska-Jankowska et al., 2015), and MAGL inhibition displayed reduced cannabimimetic effects compared to the CB1 receptor agonists (Ignatowska-Jankowska et al., 2015). Interestingly, combining COX and MAGL inhibition has shown a promising result of reducing neuropathic pain with minimal side effects (Crowe et al., 2015). MAGL inhibition increased paw skin 2-AG content and suppressed pain and inflammation subsequent to injection of formalin in the rat paw (Guindon et al., 2011; Ghosh et al., 2013). These data have clearly demonstrated that 2-AG is capable of inhibiting neuropathic and inflammatory pain.
A major obstacle to management of pain by inhibiting MAGL is the observation that increased systemic 2-AG actually results in enhanced response to painful stimuli due to desensitization of CB1 (Schlosburg et al., 2010; Kinsey et al., 2013; Ignatowska-Jankowska et al., 2014). CB1 receptors play a key role in the analgesic effects of endocannabinoids (Wilson and Nicoll, 2002; Agarwal et al., 2007; Lau et al., 2014), and chronic exposure to high concentrations of 2-AG results in CB1 desensitization and loss of CB1 analgesic input (Chanda et al., 2010; Lichtman et al., 2010; Ignatowska-Jankowska et al., 2014). There is differential expression of 2-AG among various organs, and it is possible that the degree of CB1 desensitization is proportionate to 2-AG concentrations, being most profoundly apparent in the brain (Lichtman et al., 2010). The observation of lower concentrations of 2-AG in peripheral structures is intriguing, because this raises the possibility of tissue-specific regulation of MAGL function to achieve local increases in 2-AG without inducing CB1 desensitization. Further, it has been reported that the anti-nociceptive and anti-inflammatory effects of chemical inhibitors of MAGL are preserved when these compounds are given chronically at low doses (Schlosburg et al., 2010; Ignatowska-Jankowska et al., 2014) in the absence of CB1 desensitization (Ghosh et al., 2013).
Endocannabinoids and Inflammation
Endocannabinoids have been demonstrated to suppress inflammation in bladder (Wang et al., 2015b), joints (Barrie and Manolios, 2017), gut (Lee et al., 2016), skin (Lichtman et al., 2004) and CNS (Krishnan and Chatterjee, 2014). Our laboratory (Merriam et al., 2011) and others (Dinis et al., 2004) have reported increased AEA in inflamed bladders of rats, but we did not observe this in bladder inflammation in wild-type (WT) or FAAH KO mice (Figure 1; Wang et al., 2015b), and whether or not inflammation increases local synthesis of endocannabinoids remains unclear.
Mechanisms of suppression of inflammation by endocannabinoids are not completely understood, but AEA has been shown to inhibit mitogen-induced T- and B-cell lymphocyte proliferation by increasing apoptosis (Schwarz et al., 1994). AEA inhibited lipopolysaccharide (LPS)-mediated activation of NFκB (Nakajima et al., 2006) and has also been shown to block TNFα-induced activation of NFκB by suppression of I-κB kinase, the enzyme that mediates NFκB activation (Sancho et al., 2003). We observed decreased inflammation in bladders of FAAH-deficient (knock out or KO) mice with cyclophosphamide-induced cystitis (Wang et al., 2015b). Message COX2 and NGF were decreased in inflamed bladders of FAAH KO mice relative to those of wild type mice (Figure 3).
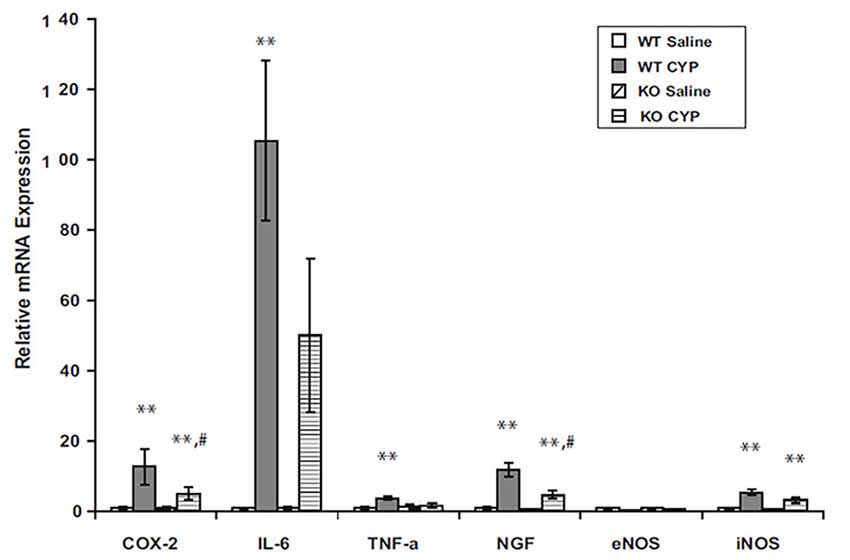
Figure 3. Bladder content of mRNA for inflammatory mediators in male WT and FAAH KO mice treated with saline (controls) or CYP (150 mg/kg) given intraperitoneally 3 h prior to sacrifice. Total bladder RNA was extracted, RT-PCR was performed, and results were normalized to signal for L19, a ribosomal protein. **p < 0.05 CYP vs. saline for each genotype; #p < 0.05 CYP treated KO vs. CYP treated WT; n = 6–8 for each genotype and treatment. Reprinted by permission from the publisher of Journal of Molecular Neuroscience, Nature/Springer/Palgrave; (Wang et al., 2015b).
Binding of 2-AG to CB1 inhibits cyclooxygenase-2 in nerves resulting in suppression of MAPK/NFκB signaling (Zhang and Chen, 2008). 2-AG also prevented in vitro damage to hippocampal slices exposed to β-amyloid by a process mediated by CB1 binding that resulted in diminished ERK 1/2 phosphorylation, decreased NFκB activation, and reduced COX-2 expression (Chen et al., 2011). It has also been reported that AEA has the capacity to diminish Th-17 cell-mediated delayed-type hypersensitivity through increased IL-10 synthesis and subsequent microRNA production (Jackson et al., 2014). As research in this area continues, it is highly probable that other pathways by which endocannabinoids suppress inflammation will be discovered.
Inflammation plays a key role in release of substances that modulate nociception. Thus, it is highly likely that the analgesic effects of endocannabinoids may in part be due to their anti-inflammatory effects.
Endocannabinoids and Bladder Pain
Evaluation of bladder pain in rodent models can be difficult, and the presence of bladder pain is most often inferred by evaluating referred mechanical sensitivity of the hind paws or abdominal wall or activity of bladder afferent nerves (Sadler et al., 2013; Stemler et al., 2013; Aizawa et al., 2014, 2016; Wang et al., 2014, 2015a,b; Girard et al., 2016). Inhibition of FAAH to increase AEA by systemic treatment with FAAH inhibitors (Merriam et al., 2011; Aizawa et al., 2014, 2016) or genetic disruption of function FAAH (Wang et al., 2015b) has been shown to suppress surrogates measurements of pain associated with bladder inflammation in rodents. However, it is unclear whether or not strategies applied systemically would be effective if adapted to tissue-specific (e.g., bladder) application.
Bladders and associated afferent nerves were isolated from mice treated in vivo with cyclophosphamide to induce inflammatory cystitis (Walczak et al., 2009; Walczak and Cervero, 2011). Afferent nerve activity was recorded during increasing intravesical pressure in inflamed bladders ex vivo in the presence or absence of intravesical cannabinoid agonists, and it was determined that intravesical cannabinoids suppressed afferent nerve fiber firing in inflamed bladders via CB1 activation (Walczak et al., 2009; Walczak and Cervero, 2011). We have also observed that intravesical administration of a CB1 agonist inhibited bladder responses to subsequent instillation of NGF (Wang et al., 2015a). These studies support the concept that intravesical manipulation of the endocannabinoid system may have the capacity to alter nociceptive signaling.
Conclusion
Manipulation of the endocannabinoid system has emerged as an appealing alternative to opioids for management of severe bladder pain. However, the potential for undesirable side effects or lack of efficacy remain significant obstacles to advancement of this therapy. Emergence of dual inhibitors of endocannabinoid degradation and either activation of TRPV1 or COX may address many of the limitations of this approach. Information on the activity of endocannabinoids synthesized in peripheral tissues remains extremely limited. It remains unclear whether or not strategies to address organ-specific pain by manipulation of endocannabinoids is a viable option, but this is an intriguing alternative that has the potential to provide effective analgesia with minimal systemic side effects.
Ethics Statement
All studies were approved by the University of Wisconsin Animal Care and Use Committee prior to performance. Procedures were consistent with guidelines provided by the Guide for the Care and Use of Laboratory Animals, 8th Edition, The National Academies Press, Washington, DC, USA.
Author Contributions
DB and ZW participated in the concept experimental design, analysis and writing. ZW was directly involved in performing experiments.
Funding
The funding for this work was provided by National Institutes of Health (NIH) R01DK088806 and NIH U54 DK104310 (DB).
Conflict of Interest Statement
The authors declare that the research was conducted in the absence of any commercial or financial relationships that could be construed as a potential conflict of interest.
References
Abrams, D. I., and Guzman, M. (2015). Cannabis in cancer care. Clin. Pharmacol. Ther. 97, 575–586. doi: 10.1002/cpt.108
Agarwal, N., Pacher, P., Tegeder, I., Amaya, F., Constantin, C. E., Brenner, G. J., et al. (2007). Cannabinoids mediate analgesia largely via peripheral type 1 cannabinoid receptors in nociceptors. Nat. Neurosci. 10, 870–879. doi: 10.1038/nn1916
Ahluwalia, J., Urban, L., Capogna, M., Bevan, S., and Nagy, I. (2000). Cannabinoid 1 receptors are expressed in nociceptive primary sensory neurons. Neuroscience 100, 685–688. doi: 10.1016/s0306-4522(00)00389-4
Ahn, K., McKinney, M. K., and Cravatt, B. F. (2008). Enzymatic pathways that regulate endocannabinoid signaling in the nervous system. Chem. Rev. 108, 1687–1707. doi: 10.1021/cr0782067
Ahn, K., Smith, S. E., Liimatta, M. B., Beidler, D., Sadagopan, N., Dudley, D. T., et al. (2011). Mechanistic and pharmacological characterization of PF-04457845: a highly potent and selective fatty acid amide hydrolase inhibitor that reduces inflammatory and noninflammatory pain. J. Pharmacol. Exp. Ther. 338, 114–124. doi: 10.1124/jpet.111.180257
Aizawa, N., Gandaglia, G., Hedlund, P., Fujimura, T., Fukuhara, H., Montorsi, F., et al. (2016). URB937, a peripherally restricted inhibitor for fatty acid amide hydrolase, reduces prostaglandin E2-induced bladder overactivity and hyperactivity of bladder mechano-afferent nerve fibres in rats. BJU Int. 117, 821–828. doi: 10.1111/bju.13223
Aizawa, N., Hedlund, P., Fullhase, C., Ito, H., Homma, Y., and Igawa, Y. (2014). Inhibition of peripheral FAAH depresses activities of bladder mechanosensitive nerve fibers of the rat. J. Urol. 192, 956–963. doi: 10.1016/j.juro.2014.04.008
Alhouayek, M., and Muccioli, G. G. (2012). The endocannabinoid system in inflammatory bowel diseases: from pathophysiology to therapeutic opportunity. Trends Mol. Med. 18, 615–625. doi: 10.1016/j.molmed.2012.07.009
Allende-Salazar, R. F., and Rada, G. (2017). Are cannabinoids an effective treatment for chronic non-cancer pain? Medwave 17:e6972. doi: 10.5867/medwave.2017.6972
Anand, U., Otto, W. R., Sanchez-Herrera, D., Facer, P., Yiangou, Y., Korchev, Y., et al. (2008). Cannabinoid receptor CB2 localisation and agonist-mediated inhibition of capsaicin responses in human sensory neurons. Pain 138, 667–680. doi: 10.1016/j.pain.2008.06.007
Anand, P., Whiteside, G., Fowler, C. J., and Hohmann, A. G. (2009). Targeting CB2 receptors and the endocannabinoid system for the treatment of pain. Brain Res. Rev. 60, 255–266. doi: 10.1016/j.brainresrev.2008.12.003
Arendt-Nielsen, L., Laursen, R. J., and Drewes, A. M. (2000). Referred pain as an indicator for neural plasticity. Prog. Brain Res. 129, 343–356. doi: 10.1016/s0079-6123(00)29026-2
Ates, M., Hamza, M., Seidel, K., Kotalla, C. E., Ledent, C., and Gühring, H. (2003). Intrathecally applied flurbiprofen produces an endocannabinoid-dependent antinociception in the rat formalin test. Eur. J. Neurosci. 17, 597–604. doi: 10.1046/j.1460-9568.2003.02470.x
Bakali, E., Elliott, R. A., Taylor, A. H., Willets, J., Konje, J. C., and Tincello, D. G. (2013). Distribution and function of the endocannabinoid system in the rat and human bladder. Int. Urogynecol. J. 24, 855–863. doi: 10.1007/s00192-012-1954-1
Bakali, E., McDonald, J., Elliott, R. A., Lambert, D. G., and Tincello, D. G. (2016). Cannabinoid receptor expression in the bladder is altered in detrusor overactivity. Int. Urogynecol. J. 27, 129–139. doi: 10.1007/s00192-015-2802-x
Barrie, N., and Manolios, N. (2017). The endocannabinoid system in pain and inflammation: its relevance to rheumatic disease. Eur. J. Rheumatol. 4, 210–218. doi: 10.5152/eurjrheum.2017.17025
Bashashati, M., Fichna, J., Piscitelli, F., Capasso, R., Izzo, A. A., Sibaev, A., et al. (2017). Targeting fatty acid amide hydrolase and transient receptor potential vanilloid-1 simultaneously to modulate colonic motility and visceral sensation in the mouse: a pharmacological intervention with N-arachidonoyl-serotonin (AA-5-HT). Neurogastroenterol. Motil. 29:e13148. doi: 10.1111/nmo.13148
Bisogno, T., Sepe, N., Melck, D., Maurelli, S., De Petrocellis, L., and Di Marzo, V. (1997). Biosynthesis, release and degradation of the novel endogenous cannabimimetic metabolite 2-arachidonoylglycerol in mouse neuroblastoma cells. Biochem. J. 322, 671–677. doi: 10.1042/bj3220671
Brock, C., Nissen, T. D., Gravesen, F. H., Frøkjaer, J. B., Omar, H., Gale, J., et al. (2008). Multimodal sensory testing of the rectum and rectosigmoid: development and reproducibility of a new method. Neurogastroenterol. Motil. 20, 908–918. doi: 10.1111/j.1365-2982.2008.01126.x
Brock, C., Olesen, S. S., Olesen, A. E., Frøkjaer, J. B., Andresen, T., and Drewes, A. M. (2012). Opioid-induced bowel dysfunction: pathophysiology and management. Drugs 72, 1847–1865. doi: 10.2165/11634970-000000000-00000
Buyukbese Sarsu, S. (2016). Unusual side effect of cannabis use: acute abdomen due to duodenal perforation. Int. J. Emerg. Med. 9:18. doi: 10.1186/s12245-016-0114-7
Capodice, J. L., Bemis, D. L., Buttyan, R., Kaplan, S. A., and Katz, A. E. (2005). Complementary and alternative medicine for chronic prostatitis/chronic pelvic pain syndrome. Evid. Based Complement. Alternat. Med. 2, 495–501. doi: 10.1093/ecam/neh128
Chanda, P. K., Gao, Y., Mark, L., Btesh, J., Strassle, B. W., Lu, P., et al. (2010). Monoacylglycerol lipase activity is a critical modulator of the tone and integrity of the endocannabinoid system. Mol. Pharmacol. 78, 996–1003. doi: 10.1124/mol.110.068304
Chen, X., Zhang, J., and Chen, C. (2011). Endocannabinoid 2-arachidonoylglycerol protects neurons against β-amyloid insults. Neuroscience 178, 159–168. doi: 10.1016/j.neuroscience.2011.01.024
Costa, B., Bettoni, I., Petrosino, S., Comelli, F., Giagnoni, G., and Di Marzo, V. (2010). The dual fatty acid amide hydrolase/TRPV1 blocker, N-arachidonoyl-serotonin, relieves carrageenan-induced inflammation and hyperalgesia in mice. Pharmacol. Res. 61, 537–546. doi: 10.1016/j.phrs.2010.02.001
Craig, A. D. (2003). Pain mechanisms: labeled lines versus convergence in central processing. Annu. Rev. Neurosci. 26, 1–30. doi: 10.1146/annurev.neuro.26.041002.131022
Cravatt, B. F., Demarest, K., Patricelli, M. P., Bracey, M. H., Giang, D. K., Martin, B. R., et al. (2001). Supersensitivity to anandamide and enhanced endogenous cannabinoid signaling in mice lacking fatty acid amide hydrolase. Proc. Natl. Acad. Sci. U S A 98, 9371–9376. doi: 10.1073/pnas.161191698
Crowe, M. S., Leishman, E., Banks, M. L., Gujjar, R., Mahadevan, A., Bradshaw, H. B., et al. (2015). Combined inhibition of monoacylglycerol lipase and cyclooxygenases synergistically reduces neuropathic pain in mice. Br. J. Pharmacol. 172, 1700–1712. doi: 10.1111/bph.13012
Davis, M. P. (2016). Cannabinoids for symptom management and cancer therapy: the evidence. J. Natl. Compr. Canc. Netw. 14, 915–922. doi: 10.6004/jnccn.2016.0094
de Novellis, V., Vita, D., Gatta, L., Luongo, L., Bellini, G., De Chiaro, M., et al. (2011). The blockade of the transient receptor potential vanilloid type 1 and fatty acid amide hydrolase decreases symptoms and central sequelae in the medial prefrontal cortex of neuropathic rats. Mol. pain 7:7. doi: 10.1186/1744-8069-7-7
De Petrocellis, L., and Di Marzo, V. (2009). Role of endocannabinoids and endovanilloids in Ca2+ signalling. Cell Calcium 45, 611–624. doi: 10.1016/j.ceca.2009.03.003
Dezieck, L., Hafez, Z., Conicella, A., Blohm, E., O’Connor, M. J., Schwarz, E. S., et al. (2017). Resolution of cannabis hyperemesis syndrome with topical capsaicin in the emergency department: a case series. Clin. Toxicol. (Phila) 55, 908–913. doi: 10.1080/15563650.2017.1324166
Di Marzo, V., Bifulco, M., and De Petrocellis, L. (2004). The endocannabinoid system and its therapeutic exploitation. Nat. Rev. Drug Discov. 3, 771–784. doi: 10.1038/nrd1495
Dinis, P., Charrua, A., Avelino, A., Yaqoob, M., Bevan, S., Nagy, I., et al. (2004). Anandamide-evoked activation of vanilloid receptor 1 contributes to the development of bladder hyperreflexia and nociceptive transmission to spinal dorsal horn neurons in cystitis. J. Neurosci. 24, 11253–11263. doi: 10.1523/JNEUROSCI.2657-04.2004
Erickson, D. R., Kunselman, A. R., Bentley, C. M., Peters, K. M., Rovner, E. S., Demers, L. M., et al. (2007). Changes in urine markers and symptoms after bladder distention for interstitial cystitis. J. Urol. 177, 556–560. doi: 10.1016/j.juro.2006.09.029
Farhadi, A., Bruninga, K., Fields, J., and Keshavarzian, A. (2001). Irritable bowel syndrome: an update on therapeutic modalities. Expert Opin. Investig. Drugs 10, 1211–1222. doi: 10.1517/13543784.10.7.1211
Farrell, K. E., Keely, S., Graham, B. A., Callister, R., and Callister, R. J. (2014). A systematic review of the evidence for central nervous system plasticity in animal models of inflammatory-mediated gastrointestinal pain. Inflamm. Bowel Dis. 20, 176–195. doi: 10.1097/01.MIB.0000437499.52922.b1
Fioravanti, B., De Felice, M., Stucky, C. L., Medler, K. A., Luo, M. C., Gardell, L. R., et al. (2008). Constitutive activity at the cannabinoid CB1 receptor is required for behavioral response to noxious chemical stimulation of TRPV1: antinociceptive actions of CB1 inverse agonists. J. Neurosci. 28, 11593–11602. doi: 10.1523/JNEUROSCI.3322-08.2008
Fleckenstein, J., Kohls, N., Evtouchenko, E., Lehmeyer, L., Kramer, S., Lang, P. M., et al. (2016). No effect of the cyclooxygenase-2 inhibitor etoricoxib on pre-emptive and post-operative analgesia in visceral surgery: results of a randomized controlled trial. Eur. J. Pain 20, 186–195. doi: 10.1002/ejp.699
Fowler, C. J., Janson, U., Johnson, R. M., Wahlström, G., Stenström, A., Norström, K., et al. (1999). Inhibition of anandamide hydrolysis by the enantiomers of ibuprofen, ketorolac, and flurbiprofen. Arch. Biochem. Biophys. 362, 191–196. doi: 10.1006/abbi.1998.1025
Fowler, C. J., Stenstrom, A., and Tiger, G. (1997). Ibuprofen inhibits the metabolism of the endogenous cannabimimetic agent anandamide. Pharmacol. Toxicol. 80, 103–107. doi: 10.1111/j.1600-0773.1997.tb00291.x
Galiegue, S., Mary, S., Marchand, J., Dussossoy, D., Carriere, D., Carayon, P., et al. (1995). Expression of central and peripheral cannabinoid receptors in human immune tissues and leukocyte subpopulations. Eur. J. Biochem. 232, 54–61. doi: 10.1111/j.1432-1033.1995.tb20780.x
Gebhart, G. F., and Bielefeldt, K. (2016). Physiology of visceral pain. Compr. Physiol. 6, 1609–1633. doi: 10.1002/cphy.c150049
Ghosh, S., Wise, L. E., Chen, Y., Gujjar, R., Mahadevan, A., Cravatt, B. F., et al. (2013). The monoacylglycerol lipase inhibitor JZL184 suppresses inflammatory pain in the mouse carrageenan model. Life Sci. 92, 498–505. doi: 10.1016/j.lfs.2012.06.020
Girard, B. M., Malley, S. E., Mathews, M. M., May, V., and Vizzard, M. A. (2016). Intravesical PAC1 receptor antagonist, PACAP(6–38), reduces urinary bladder frequency and pelvic sensitivity in NGF-OE mice. J. Mol. Neurosci. 59, 290–299. doi: 10.1007/s12031-016-0764-1
Goodman, M. C., Xu, S., Rouzer, C. A., Banerjee, S., Ghebreselasie, K., Migliore, M., et al. (2018). Dual cyclooxygenase-fatty acid amide hydrolase inhibitor exploits novel binding interactions in the cyclooxygenase active site. J. Biol. Chem. 293, 3028–3038. doi: 10.1074/jbc.M117.802058
Goparaju, S. K., Ueda, N., Taniguchi, K., and Yamamoto, S. (1999). Enzymes of porcine brain hydrolyzing 2-arachidonoylglycerol, an endogenous ligand of cannabinoid receptors. Biochem. Pharmacol. 57, 417–423. doi: 10.1016/s0090-6980(99)90443-4
Graham, E. S., Angel, C. E., Schwarcz, L. E., Dunbar, P. R., and Glass, M. (2010). Detailed characterisation of CB2 receptor protein expression in peripheral blood immune cells from healthy human volunteers using flow cytometry. Int. J. Immunopathol. Pharmacol. 23, 25–34. doi: 10.1177/039463201002300103
Gratzke, C., Streng, T., Park, A., Christ, G., Stief, C. G., Hedlund, P., et al. (2009). Distribution and function of cannabinoid receptors 1 and 2 in the rat, monkey and human bladder. J. Urol. 181, 1939–1948. doi: 10.1016/j.juro.2008.11.079
Gratzke, C., Streng, T., Stief, C. G., Downs, T. R., Alroy, I., Rosenbaum, J. S., et al. (2010). Effects of cannabinor, a novel selective cannabinoid 2 receptor agonist, on bladder function in normal rats. Eur. Urol. 57, 1093–1100. doi: 10.1016/j.eururo.2010.02.027
Guhring, H., Hamza, M., Sergejeva, M., Ates, M., Kotalla, C. E., Ledent, C., et al. (2002). A role for endocannabinoids in indomethacin-induced spinal antinociception. Eur. J. Pharmacol. 454, 153–163. doi: 10.1016/s0014-2999(02)02485-8
Guindon, J., Guijarro, A., Piomelli, D., and Hohmann, A. G. (2011). Peripheral antinociceptive effects of inhibitors of monoacylglycerol lipase in a rat model of inflammatory pain. Br. J. Pharmacol. 163, 1464–1478. doi: 10.1111/j.1476-5381.2010.01192.x
Gutierrez, T., Farthing, J. N., Zvonok, A. M., Makriyannis, A., and Hohmann, A. G. (2007). Activation of peripheral cannabinoid CB1 and CB2 receptors suppresses the maintenance of inflammatory nociception: a comparative analysis. Br. J. Pharmacol. 150, 153–163. doi: 10.1038/sj.bjp.0706984
Hayn, M. H., Ballesteros, I., de Miguel, F., Coyle, C. H., Tyagi, S., Yoshimura, N., et al. (2008). Functional and immunohistochemical characterization of CB1 and CB2 receptors in rat bladder. Urology 72, 1174–1178. doi: 10.1016/j.urology.2008.03.044
Hedlund, P. (2014). Cannabinoids and the endocannabinoid system in lower urinary tract function and dysfunction. Neurourol. Urodyn. 33, 46–53. doi: 10.1002/nau.22442
Hohmann, A. G., and Herkenham, M. (1999). Localization of central cannabinoid CB1 receptor messenger RNA in neuronal subpopulations of rat dorsal root ganglia: a double-label in situ hybridization study. Neuroscience 90, 923–931. doi: 10.1016/s0306-4522(98)00524-7
Hoke, T. P., Goldstein, H., Saks, E. K., and Vakili, B. (2017). Hydrodistention of the bladder for the treatment of bladder pain syndrome/interstitial cystitis (BPS/IC). Neurourol. Urodyn. 36, 784–786. doi: 10.1002/nau.23024
Huggins, J. P., Smart, T. S., Langman, S., Taylor, L., and Young, T. (2012). An efficient randomised, placebo-controlled clinical trial with the irreversible fatty acid amide hydrolase-1 inhibitor PF-04457845, which modulates endocannabinoids but fails to induce effective analgesia in patients with pain due to osteoarthritis of the knee. Pain 153, 1837–1846. doi: 10.1016/j.pain.2012.04.020
Ibrahim, M. M., Deng, H., Zvonok, A., Cockayne, D. A., Kwan, J., Mata, H. P., et al. (2003). Activation of CB2 cannabinoid receptors by AM1241 inhibits experimental neuropathic pain: pain inhibition by receptors not present in the CNS. Proc. Natl. Acad. Sci. U S A 100, 10529–10533. doi: 10.1073/pnas.1834309100
Ignatowska-Jankowska, B. M., Ghosh, S., Crowe, M. S., Kinsey, S. G., Niphakis, M. J., Abdullah, R. A., et al. (2014). In vivo characterization of the highly selective monoacylglycerol lipase inhibitor KML29: antinociceptive activity without cannabimimetic side effects. Br. J. Pharmacol. 171, 1392–1407. doi: 10.1111/bph.12298
Ignatowska-Jankowska, B. M., Wilkerson, J. L., Mustafa, M., Abdullah, R., Niphakis, M., Wiley, J. L., et al. (2015). Selective monoacylglycerol lipase inhibitors: antinociceptive versus cannabimimetic effects in mice. J. Pharmacol. Exp. Ther. 353, 424–432. doi: 10.1124/jpet.114.222315
Jackson, A. R., Nagarkatti, P., and Nagarkatti, M. (2014). Anandamide attenuates Th-17 cell-mediated delayed-type hypersensitivity response by triggering IL-10 production and consequent microRNA induction. PLoS One 9:e93954. doi: 10.1371/journal.pone.0093954
John, H., Rüedi, C., Kötting, S., Schmid, D. M., Fatzer, M., and Hauri, D. (2003). A new high frequency electrostimulation device to treat chronic prostatitis. J. Urol. 170, 1275–1277. doi: 10.1097/01.ju.0000085582.54511.de
Johnson, J. R., Burnell-Nugent, M., Lossignol, D., Ganae-Motan, E. D., Potts, R., and Fallon, M. T. (2010). Multicenter, double-blind, randomized, placebo-controlled, parallel-group study of the efficacy, safety, and tolerability of THC:CBD extract and THC extract in patients with intractable cancer-related pain. J. Pain Symptom Manage. 39, 167–179. doi: 10.1016/j.jpainsymman.2009.06.008
Jones, M. R., Wang, Z. Y., and Bjorling, D. E. (2015). Intrathecal cannabinoid-1 receptor agonist prevents referred hyperalgesia in acute acrolein-induced cystitis in rats. Am. J. Clin. Exp. Urol. 3, 28–35.
Kim, S. R., Bok, E., Chung, Y. C., Chung, E. S., and Jin, B. K. (2008). Interactions between CB1 receptors and TRPV1 channels mediated by 12-HPETE are cytotoxic to mesencephalic dopaminergic neurons. Br. J. Pharmacol. 155, 253–264. doi: 10.1038/bjp.2008.246
Kinsey, S. G., Long, J. Z., O’Neal, S. T., Abdullah, R. A., Poklis, J. L., Boger, D. L., et al. (2009). Blockade of endocannabinoid-degrading enzymes attenuates neuropathic pain. J. Pharmacol. Exp. Ther. 330, 902–910. doi: 10.1124/jpet.109.155465
Kinsey, S. G., Wise, L. E., Ramesh, D., Abdullah, R., Selley, D. E., Cravatt, B. F., et al. (2013). Repeated low-dose administration of the monoacylglycerol lipase inhibitor JZL184 retains cannabinoid receptor type 1-mediated antinociceptive and gastroprotective effects. J. Pharmacol. Exp. Ther. 345, 492–501. doi: 10.1124/jpet.112.201426
Kogan, N. M., and Mechoulam, R. (2006). The chemistry of endocannabinoids. J. Endocrinol. Invest. 29, 3–14.
Kohnz, R. A., and Nomura, D. K. (2014). Chemical approaches to therapeutically target the metabolism and signaling of the endocannabinoid 2-AG and eicosanoids. Chem. Soc. Rev. 43, 6859–6869. doi: 10.1039/c4cs00047a
Kozak, K. R., Crews, B. C., Ray, J. L., Tai, H. H., Morrow, J. D., and Marnett, L. J. (2001). Metabolism of prostaglandin glycerol esters and prostaglandin ethanolamides in vitro and in vivo. J. Biol. Chem. 276, 36993–36998. doi: 10.1074/jbc.M105854200
Kozak, K. R., Rowlinson, S. W., and Marnett, L. J. (2000). Oxygenation of the endocannabinoid, 2-arachidonylglycerol, to glyceryl prostaglandins by cyclooxygenase-2. J. Biol. Chem. 275, 33744–33749. doi: 10.1074/jbc.M007088200
Krishnan, G., and Chatterjee, N. (2014). Endocannabinoids affect innate immunity of Muller glia during HIV-1 Tat cytotoxicity. Mol. Cell. Neurosci. 59, 10–23. doi: 10.1016/j.mcn.2014.01.001
Lau, B. K., Drew, G. M., Mitchell, V. A., and Vaughan, C. W. (2014). Endocannabinoid modulation by FAAH and monoacylglycerol lipase within the analgesic circuitry of the periaqueductal grey. Br. J. Pharmacol. 171, 5225–5236. doi: 10.1111/bph.12839
Lee, Y., Jo, J., Chung, H. Y., Pothoulakis, C., and Im, E. (2016). Endocannabinoids in the gastrointestinal tract. Am. J. Physiol. Gastrointest. Liver Physiol. 311, G655–G666. doi: 10.1152/ajpgi.00294.2015
Li, G. L., Winter, H., Arends, R., Jay, G. W., Le, V., Young, T., et al. (2012). Assessment of the pharmacology and tolerability of PF-04457845, an irreversible inhibitor of fatty acid amide hydrolase-1, in healthy subjects. Br. J. Clin. Pharmacol. 73, 706–716. doi: 10.1111/j.1365-2125.2011.04137.x
Lichtman, A. H., Blankman, J. L., and Cravatt, B. F. (2010). Endocannabinoid overload. Mol. Pharmacol. 78, 993–995. doi: 10.1124/mol.110.069427
Lichtman, A. H., Shelton, C. C., Advani, T., and Cravatt, B. F. (2004). Mice lacking fatty acid amide hydrolase exhibit a cannabinoid receptor-mediated phenotypic hypoalgesia. Pain 109, 319–327. doi: 10.1016/s0304-3959(04)00054-5
Lovick, T. A. (2016). Central control of visceral pain and urinary tract function. Auton. Neurosci. 200, 35–42. doi: 10.1016/j.autneu.2016.02.001
Luz, L. L., Fernandes, E. C., Sivado, M., Kokai, E., Szucs, P., and Safronov, B. V. (2015). Monosynaptic convergence of somatic and visceral C-fiber afferents on projection and local circuit neurons in lamina I: a substrate for referred pain. Pain 156, 2042–2051. doi: 10.1097/j.pain.0000000000000267
Mallet, C., Dubray, C., and Duale, C. (2016). FAAH inhibitors in the limelight, but regrettably. Int. J. Clin. Pharmacol. Ther. 54, 498–501. doi: 10.5414/cp202687
Marsicano, G., Goodenough, S., Monory, K., Hermann, H., Eder, M., Cannich, A., et al. (2003). CB1 cannabinoid receptors and on-demand defense against excitotoxicity. Science 302, 84–88. doi: 10.1126/science.1088208
Merriam, F. V., Wang, Z. Y., Hillard, C. J., Stuhr, K. L., and Bjorling, D. E. (2011). Inhibition of fatty acid amide hydrolase suppresses referred hyperalgesia induced by bladder inflammation. BJU Int. 108, 1145–1149. doi: 10.1111/j.1464-410X.2010.09583.x
Mykoniatis, I., Katafigiotis, I., Sfoungaristos, S., and Yutkin, V. (2017). Immunotherapy options for painful bladder syndrome: what’s the potential? Expert Opin. Biol. Therapy 17, 1471–1480. doi: 10.1080/14712598.2017.1375094
Nackley, A. G., Zvonok, A. M., Makriyannis, A., and Hohmann, A. G. (2004). Activation of cannabinoid CB2 receptors suppresses C-fiber responses and windup in spinal wide dynamic range neurons in the absence and presence of inflammation. J. Neurophysiol. 92, 3562–3574. doi: 10.1152/jn.00886.2003
Nakajima, Y., Furuichi, Y., Biswas, K. K., Hashiguchi, T., Kawahara, K., Yamaji, K., et al. (2006). Endocannabinoid, anandamide in gingival tissue regulates the periodontal inflammation through NF-kappaB pathway inhibition. FEBS letters 580, 613–619. doi: 10.1016/j.febslet.2005.12.079
Nielsen, S., Sabioni, P., Trigo, J. M., Ware, M. A., Betz-Stablein, B. D., Murnion, B., et al. (2017). Opioid-sparing effect of cannabinoids: a systematic review and meta-analysis. Neuropsychopharmacology 42, 1752–1765. doi: 10.1038/npp.2017.51
Olson, L. E., Dyer, J. E., Haq, A., Ockrim, J., and Greenwell, T. J. (2018). A systematic review of the literature on cystodistension in bladder pain syndrome. Int. Urogynecol. J. 29, 251–257. doi: 10.1007/s00192-017-3355-y
Palmer, S. L., Thakur, G. A., and Makriyannis, A. (2002). Cannabinergic ligands. Chem. Phys. Lipids 121, 3–19. doi: 10.1016/S0009-3084(02)00143-3
Pierau, F. K., Fellmer, G., and Taylor, D. C. (1984). Somato-visceral convergence in cat dorsal root ganglion neurones demonstrated by double-labelling with fluorescent tracers. Brain Res. 321, 63–70. doi: 10.1016/0006-8993(84)90681-4
Piomelli, D., Beltramo, M., Giuffrida, A., and Stella, N. (1998). Endogenous cannabinoid signaling. Neurobiol. Dis. 5, 462–473. doi: 10.1006/nbdi.1998.0221
Quang-Cantagrel, N. D., Wallace, M. S., and Magnuson, S. K. (2000). Opioid substitution to improve the effectiveness of chronic noncancer pain control: a chart review. Anesth. Analg. 90, 933–937. doi: 10.1213/00000539-200004000-00029
Sadler, K. E., Stratton, J. M., DeBerry, J. J., and Kolber, B. J. (2013). Optimization of a pain model: effects of body temperature and anesthesia on bladder nociception in mice. PLoS One 8:e79617. doi: 10.1371/journal.pone.0079617
Sancho, R., Calzado, M. A., Di Marzo, V., Appendino, G., and Muáoz, E. (2003). Anandamide inhibits nuclear factor-kappaB activation through a cannabinoid receptor-independent pathway. Mol. Pharmacol. 63, 429–438. doi: 10.1124/mol.63.2.429
Sasso, O., Migliore, M., Habrant, D., Armirotti, A., Albani, C., Summa, M., et al. (2015). Multitarget fatty acid amide hydrolase/cyclooxygenase blockade suppresses intestinal inflammation and protects against nonsteroidal anti-inflammatory drug-dependent gastrointestinal damage. FASEB J. 29, 2616–2627. doi: 10.1096/fj.15-270637
Schlosburg, J. E., Blankman, J. L., Long, J. Z., Nomura, D. K., Pan, B., Kinsey, S. G., et al. (2010). Chronic monoacylglycerol lipase blockade causes functional antagonism of the endocannabinoid system. Nat. Neurosci. 13, 1113–1119. doi: 10.1038/nn.2616
Schwarz, H., Blanco, F. J., and Lotz, M. (1994). Anadamide, an endogenous cannabinoid receptor agonist inhibits lymphocyte proliferation and induces apoptosis. J. Neuroimmunol. 55, 107–115. doi: 10.1016/0165-5728(94)90152-x
Seidel, K., Hamza, M., Ates, M., and Gühring, H. (2003). Flurbiprofen inhibits capsaicin induced calcitonin gene related peptide release from rat spinal cord via an endocannabinoid dependent mechanism. Neurosci. Lett. 338, 99–102. doi: 10.1016/s0304-3940(02)01366-6
Smith, W. L., DeWitt, D. L., and Garavito, R. M. (2000). Cyclooxygenases: structural, cellular, and molecular biology. Annu. Rev. Biochem. 69, 145–182. doi: 10.1146/annurev.biochem.69.1.145
Stemler, K. M., Crock, L. W., Lai, H. H., Mills, J. C., Gereau, R. W. IV., and Mysorekar, I. U. (2013). Protamine sulfate induced bladder injury protects from distention induced bladder pain. J. Urol. 189, 343–351. doi: 10.1016/j.juro.2012.08.189
Tateo, S. (2017). State of the evidence: cannabinoids and cancer pain-A systematic review. J. Am. Assoc. Nurse Pract. 29, 94–103. doi: 10.1002/2327-6924.12422
Thomas, E. A., Cravatt, B. F., Danielson, P. E., Gilula, N. B., and Sutcliffe, J. G. (1997). Fatty acid amide hydrolase, the degradative enzyme for anandamide and oleamide, has selective distribution in neurons within the rat central nervous system. J. Neurosci. Res. 50, 1047–1052. doi: 10.1002/(sici)1097-4547(19971215)50:6<1047::aid-jnr16>3.0.co;2-1
Tognetto, M., Amadesi, S., Harrison, S., Creminon, C., Trevisani, M., Carreras, M., et al. (2001). Anandamide excites central terminals of dorsal root ganglion neurons via vanilloid receptor-1 activation. J. Neurosci. 21, 1104–1109. doi: 10.1523/JNEUROSCI.21-04-01104.2001
Tyagi, V., Philips, B. J., Su, R., Smaldone, M. C., Erickson, V. L., Chancellor, M. B., et al. (2009). Differential expression of functional cannabinoid receptors in human bladder detrusor and urothelium. J. Urol. 181, 1932–1938. doi: 10.1016/j.juro.2008.11.078
Walczak, J. S., and Cervero, F. (2011). Local activation of cannabinoid CB1 receptors in the urinary bladder reduces the inflammation-induced sensitization of bladder afferents. Mol. Pain 7:31. doi: 10.1186/1744-8069-7-31
Walczak, J. S., Price, T. J., and Cervero, F. (2009). Cannabinoid CB1 receptors are expressed in the mouse urinary bladder and their activation modulates afferent bladder activity. Neuroscience 159, 1154–1163. doi: 10.1016/j.neuroscience.2009.01.050
Wang, J., Chen, Y., Chen, J., Zhang, G., and Wu, P. (2017). Sacral neuromodulation for refractory bladder pain syndrome/interstitial cystitis: a global systematic review and meta-analysis. Sci. Rep. 7:11031. doi: 10.1038/s41598-017-11062-x
Wang, Z. Y., Wang, P., and Bjorling, D. E. (2013). Activation of cannabinoid receptor 2 inhibits experimental cystitis. Am. J. Physiol. Regul. Integr. Comp. Physiol. 304, R846–R853. doi: 10.1152/ajpregu.00585.2012
Wang, Z. Y., Wang, P., and Bjorling, D. E. (2014). Treatment with a cannabinoid receptor 2 agonist decreases severity of established cystitis. J. Urol. 191, 1153–1158. doi: 10.1016/j.juro.2013.10.102
Wang, Z. Y., Wang, P., and Bjorling, D. E. (2015a). Activation of cannabinoid receptor 1 inhibits increased bladder activity induced by nerve growth factor. Neurosci. Lett. 589, 19–24. doi: 10.1016/j.neulet.2015.01.009
Wang, Z. Y., Wang, P., Hillard, C. J., and Bjorling, D. E. (2015b). Attenuation of cystitis and pain sensation in mice lacking fatty acid amide hydrolase. J. Mol. Neurosci. 55, 968–976. doi: 10.1007/s12031-014-0453-x
Weber, A., Ni, J., Ling, K. H., Acheampong, A., Tang-Liu, D. D., Burk, R., et al. (2004). Formation of prostamides from anandamide in FAAH knockout mice analyzed by HPLC with tandem mass spectrometry. J. Lipid Res. 45, 757–763. doi: 10.1194/jlr.m300475-jlr200
Weber, L., Yeomans, D. C., and Tzabazis, A. (2017). Opioid-induced hyperalgesia in clinical anesthesia practice: what has remained from theoretical concepts and experimental studies? Curr. Opin. Anaesthesiol. 30, 458–465. doi: 10.1097/ACO.0000000000000485
Wilson, R. I., and Nicoll, R. A. (2002). Endocannabinoid signaling in the brain. Science 296, 678–682. doi: 10.1126/science.1063545
Wotherspoon, G., Fox, A., McIntyre, P., Colley, S., Bevan, S., and Winter, J. (2005). Peripheral nerve injury induces cannabinoid receptor 2 protein expression in rat sensory neurons. Neuroscience 135, 235–245. doi: 10.1016/j.neuroscience.2005.06.009
Zhang, J., and Chen, C. (2008). Endocannabinoid 2-arachidonoylglycerol protects neurons by limiting COX-2 elevation. J. Biol. Chem. 283, 22601–22611. doi: 10.1074/jbc.M800524200
Zhang, W., Deng, X., Liu, C., and Wang, X. (2017). Intravesical treatment for interstitial cystitis/painful bladder syndrome: a network meta-analysis. Int. Urogynecol. J. 28, 515–525. doi: 10.1007/s00192-016-3079-4
Keywords: bladder pain, visceral pain, endocannabinoids, 2-arachidonoylglycerol, anandamide, fatty acid amide hydrolase
Citation: Bjorling DE and Wang Z (2018) Potential of Endocannabinoids to Control Bladder Pain. Front. Syst. Neurosci. 12:17. doi: 10.3389/fnsys.2018.00017
Received: 28 September 2017; Accepted: 24 April 2018;
Published: 15 May 2018.
Edited by:
Jyoti N. Sengupta, Medical College of Wisconsin, United StatesReviewed by:
Victor Manuel Pulgar, Wake Forest School of Medicine, United StatesLivio Luongo, Università degli Studi della Campania Luigi Vanvitelli, Italy
Copyright © 2018 Bjorling and Wang. This is an open-access article distributed under the terms of the Creative Commons Attribution License (CC BY). The use, distribution or reproduction in other forums is permitted, provided the original author(s) and the copyright owner are credited and that the original publication in this journal is cited, in accordance with accepted academic practice. No use, distribution or reproduction is permitted which does not comply with these terms.
*Correspondence: Dale E. Bjorling, ZGFsZS5iam9ybGluZ0B3aXNjLmVkdQ==