- Department of Anatomy and Cell Biology, University of Kansas Medical Center, Kansas City, KS, United States
Pain is the most reported and troublesome symptom of nearly all functional disorders affecting the genitourinary and gastrointestinal organs. Patients with irritable bowel syndrome (IBS), interstitial cystitis/painful bladder syndrome (IC/PBS), vulvodynia, and/or chronic prostatitis/chronic pelvic pain syndrome (CP/CPPS; collectively termed chronic pelvic pain syndromes) report pain severe enough to impact quality of life and often suffer from symptoms of or are diagnosed with more than one of these syndromes. This increased comorbidity between chronic pelvic pain syndromes, and with pain disorders of disparate body regions, as well as with mood disorders, can be influenced by disruptions in the hypothalamic-pituitary-adrenal (HPA) axis, which regulates the response to stress and influences the perception of pain. Experiencing trauma, neglect, or abuse in early life can permanently affect the functioning of the HPA axis. As such, a significant proportion of patients suffering from comorbid chronic pelvic pain syndromes report a history of early life stress or trauma. Here we will report on how these early life experiences influence chronic pelvic pain in patients. We will also discuss various rodent models that have been developed to study this phenomenon to understand the mechanisms underlying HPA axis dysfunction, as well as potential underlying mechanisms connecting these syndromes to one another.
Introduction
The International Association for the Study of Pain (IASP) defines “pain” as an “unpleasant sensory and emotional experience associated with actual or potential tissue damage or described in terms of such damage” (Loeser et al., 1994). A particularly vexing type of pain for both patients and healthcare providers is chronic urogenital or pelvic pain: pain that is localized to the lower abdomen and the pelvic and perineal regions. Chronic pelvic and urogenital pain is common and debilitating, and is the foremost complaint of patients suffering from functional gastrointestinal disorders (e.g., irritable bowel syndrome; IBS) and genitourinary disorders (e.g., interstitial cystitis/painful bladder syndrome; IC/PBS); moreover, this type of pain is idiopathic, meaning the etiologies of these painful disorders are unknown and are not associated with identifiable infectious, anatomical, metabolic, or other organic pathologies. The mechanisms of chronic visceral pain are poorly understood, in part due to its diffuse and poorly localized nature that often involves two or more visceral organs. Additionally, the diverse nature of visceral pain is compounded by multiple factors, including psychosocial stress, sexual dimorphism, and genetic and/or environmental predisposition. These multiple contributing factors make treatment and research efforts, especially the development and study of relevant animal models, challenging.
Despite its multifaceted nature, visceral hypersensitivity has been recognized to occur due to: (1) sensitization of primary sensory afferents innervating the viscera (peripheral sensitization); (2) hyper-excitability of ascending spinal neurons receiving synaptic input from the viscera (central sensitization); and (3) dysregulation of descending pathways that modulate spinal nociceptive transmission (Sengupta, 2009). In this review, we will focus on the influence of early life stress on the descending pathway and feedback loop of the hypothalamic-pituitary-adrenal (HPA) axis, as well as its contribution to chronic pelvic pain of patients suffering from IBS, IC/PBS, vulvodynia, and/or chronic prostatitis/chronic pelvic pain syndrome (CP/CPPS; collectively termed chronic pelvic pain syndromes).
Hypothalamic-Pituitary-Adrenal Axis
Central Regulation
Stressful events experienced early in life can dramatically alter the functioning of the HPA axis, which regulates the stress response and influences the perception of pain (Heim et al., 1998, 2001; Rao et al., 2008; Tyrka et al., 2008; Mayson and Teichman, 2009; Videlock et al., 2009; schematically shown in Figure 1). Corticotropin-releasing factor (CRF) is the primary initiator of the stress response and, in the brain, is primarily expressed in the paraventricular nucleus (PVN) of the hypothalamus, central nucleus of the amygdala (Bale and Vale, 2004), and Barrington’s nucleus (BN), the pontine micturition center (Imaki et al., 1991; Pavcovich and Valentino, 1995). Under stressful conditions, CRF and arginine vasopressin are secreted from the PVN of the hypothalamus and travel through the hypophysical portal veins to reach the anterior pituitary corticotrophs and induce the release of adrenocorticotropic hormone (ACTH). Systemic circulation of ACTH stimulates the production and release of glucocorticoids (GC, cortisol in humans and corticosterone in rodents) from the adrenal cortex (Herman et al., 2005; Ulrich-Lai and Herman, 2009), which, under normal conditions, initiate an overall immune suppression and decrease CRF and ACTH production through a negative feedback loop (Kageyama and Suda, 2009; Tasker and Herman, 2011).
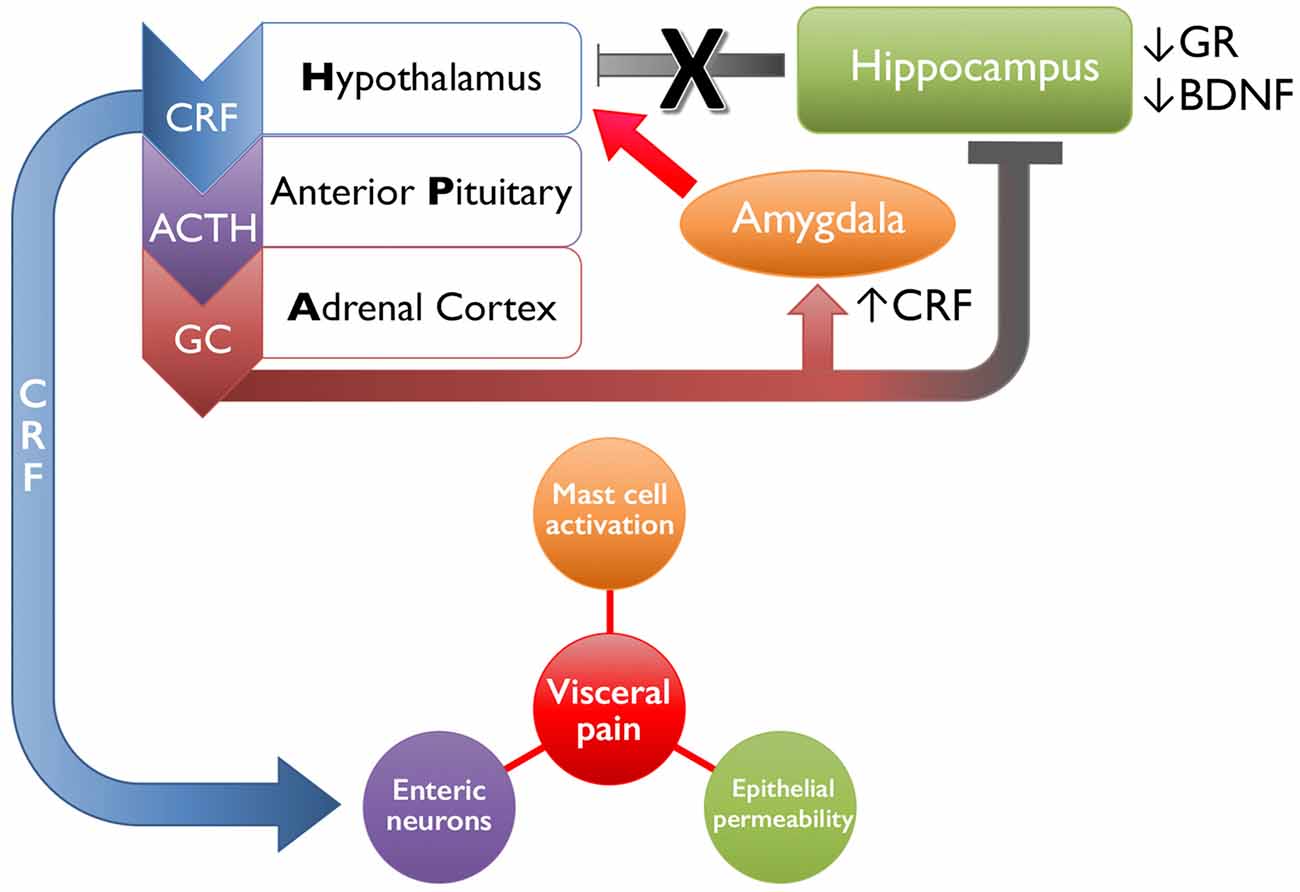
Figure 1. Schematic representation of early life stress-induced changes in limbic regulation of and downstream targets of the hypothalamic-pituitary-adrenal (HPA) axis. Following exposure to an acute stressor, the hypothalamus will release corticotropin-releasing factor (CRF), which signals the anterior pituitary to release adrenocorticotropic hormone (ACTH). The systemic circulation of ACTH initiates the adrenal cortex to release glucocorticoids (GCs, cortisol in humans, corticosterone in rodents). Both GC and CRF will bind to receptors expressed by higher structures within the HPA axis and by limbic structures, including the amygdala and hippocampus, to reduce HPA axis activity and restore homeostasis upon cessation of the stressor. Early life stress disrupts this system by increasing the release of CRF from the hypothalamus and amygdala, as well as decreasing glucocorticoid receptor (GR) and brain-derived neurotrophic factor (BDNF) in the hippocampus, which has a combined effect of increasing positive feedback onto the HPA axis and driving activation. Downstream actions of CRF include increasing mast cell activation and inducing local inflammatory effects, binding onto enteric neurons that can increase colonic motility, and increasing epithelial permeability by disrupting tight junctions. Together these mechanisms drive increased visceral pain in organs affected in irritable bowel syndrome (IBS), interstitial cystitis/painful bladder syndrome (IC/PBS), chronic prostatitis/chronic pelvic pain syndrome (CP/CPPS), and/or vulvodynia.
In addition to CRF, its family members, the urocortins (Ucn1–3), are also produced in stress-related brain regions. Ucn2 and Ucn3 are found in the PVN (Reyes et al., 2001; Venihaki et al., 2004) and Ucn1 is largely expressed in Edinger-Westphal, superior lateral olive, and supraoptic nuclei (Vaughan et al., 1995; Bittencourt et al., 1999). It has been hypothesized that CRF and Ucn1 comprise two separate and functionally intertwined stress-responsive neuronal circuits, as Ucn1 mRNA levels are upregulated in the Edinger-Westphal nucleus following acute pain and/or restraint stress, but on a delayed and longer time scale than CRF is increased in the PVN (Kozicz, 2007). Two G-protein coupled receptors, CRF1 and CRF2, bind CRF and Ucn ligands with varying affinity. CRF binds CRF1 with a 10-fold higher affinity than CRF2, Ucn1 binds CRF1 and CRF2 with equal affinity, and Ucn2 and Ucn3 both preferentially bind CRF2 (Bale and Vale, 2004). Opposing roles of CRF1 and CRF2 in stress-related behaviors have been defined through gene deletion and pharmacological studies. Disruption of CRF1 signaling is largely anxiolytic and results in a significant decrease in anxiety-like behaviors (Smith et al., 1998). In contrast, CRF2 deletion or blockade increases behavioral indicators of anxiety and prevents homeostatic resolution within HPA axis following a stressful event (Bale et al., 2000, 2002). Stress exposure also promotes CRF release in the central amygdala, a limbic structure involved in memory processing, decision-making, and emotional reactions (Cook, 2004). Chronic GC exposure increases expression of CRF mRNA in the amygdala (Makino et al., 1994, 1999), suggesting that sensitization may be involved in the development of stress-related pathologies (Herman et al., 2012). Studies have also described central activation of CRF receptors mediating stress-related changes in GI function (Van Pett et al., 2000; Reyes et al., 2008). Additionally, studies in children exposed to severe deprivation, neglect, or abuse report lower baseline levels of GCs (Gunnar and Quevedo, 2008; Lupien et al., 2009). It has been hypothesized that this may be due to a downregulation of the HPA axis at the level of the pituitary in response to chronic drive of CRF from the hypothalamus (Fries et al., 2005), or target tissue hypersensitivity to GCs (Yehuda et al., 2006).
Glucocorticoid receptors (GRs) are also abundantly expressed in the hypothalamus and limbic structures and respond to systemic and diurnal release of GCs from the adrenal cortex. The high-affinity mineralocorticoid receptor (MR) can be bound at low circulating levels of GCs, and is thus thought to be important in ambient GC signaling (Dallman et al., 1989) and maintaining the diurnal tone of the HPA axis (Reul and de Kloet, 1985). Tonic feedback within the HPA axis via hippocampal MR is thought to occur by dampening HPA activity during the diurnal trough (van Haarst et al., 1997). The lower-affinity GR is bound during diurnal peaks and spikes in GCs following an acute stressor (Reul and de Kloet, 1985; De Kloet et al., 1998). GR is richly expressed throughout the hippocampus and the prefrontal cortex, limbic structures that are implicated in negative feedback regulation of the HPA axis (Herman, 1993; Herman et al., 2012). Through electrical and chemical stimulation, genetic manipulation, and lesion studies, these brain regions have been shown to be responsible for negative feedback inhibition of the stress response through GR binding, likely in parallel due to innervation of common subcortical targets (Radley and Sawchenko, 2011; Herman et al., 2012). Both MR and GR are highly expressed in hippocampal neurons that, when activated, shut down the HPA axis following the resolution of stressful stimuli by glutamatergic input to GABAergic PVN relays within the hypothalamus (Cho and Little, 1999; Kohara et al., 2001; Numakawa et al., 2009). Negative feedback inhibition of the HPA axis from limbic circuitry is programmed during development and can permanently alter HPA axis function, making the limbic system particularly vulnerable to adversity early in life (Perry and Pollard, 1998; Vázquez, 1998; Bremne and Vermetten, 2001; Heim and Nemeroff, 2002; Teicher et al., 2002, 2003; Rao et al., 2008; Rincón-Cortés and Sullivan, 2014).
Peripheral Targets
CRF1 and CRF2 are also widely distributed in the periphery, where ligand-binding activation influences evolutionarily conserved mammalian physiological mechanisms that mediate the return to homeostasis following a stressful event. In the rat colon, CRF1 immunoreactivity was observed in the mucosal layer, primarily in cells of an inflammatory nature, and in the myenteric plexus and submucosal plexus; whereas, CRF2 immunoreactivity was observed on the luminal surface of goblet cells and in blood vessels located in the submucosa, but not in the enteric innervation (Chatzaki et al., 2004). Expression of CRF1 has been confirmed in submucosal and myenteric neurons of the human colon and by macrophages in the lamina propria (Yuan et al., 2012). Exogenous application of CRF or plasma from IBS patients stimulated contractility of explanted rat colon, the latter of which could be blocked by CRF1, but not CRF2, antagonist pretreatment (Buckley et al., 2014). In the same study, blocking interleukin (IL)-6 or CRF alleviated the increased gastrointestinal motility and improved visceral pain thresholds in a Wistar-Kyoto rat model of IBS. Increased transepithelial resistance and mucosal-to-serosal flux was observed in porcine ileus explants following exposure to increasing amounts of CRF, which was blocked by a non-selective CRF1/2 antagonist or a mast cell stabilizer (Overman et al., 2012). Feline urothelial cells also express functionally-active CRF1 and CRF2, along with their intrinsic ligands CRF and Ucn1 (Hanna-Mitchell et al., 2014). Samples from cats with feline interstitial cystitis, widely accepted as the most relevant preclinical model of IC, demonstrated alterations in CRF receptor expression and functioning, suggesting an etiological role for CRF signaling in this disorder. In human peripheral tissues, CRF2 is expressed in high levels in the skin and muscles (skeletal, smooth and cardiac), whereas CRF1 is expressed in tissues such as adrenal, adipose tissue, the gonads, endometrium, myometrium, placenta, skin, spleen, and specific cells of the immune system (Hillhouse and Grammatopoulos, 2006).
One downstream target of the HPA axis that has been implicated in nearly all chronic pelvic pain syndromes is the mast cell. Mast cells are multifunctional immune cells that express high-affinity immunoglobulin E receptors and release potent inflammatory mediators including, but not limited to, leukotrienes, cytokines, serotonin, histamine, and proteases, such as tryptase (Ren et al., 1998). Involved in the innate immune response, hematopoietic progenitors of mast cells develop in the bone marrow and are recruited to the peripheral tissues, primarily those interfacing with the environment (e.g., the respiratory-, gastrointestinal-, and genitourinary tracts), where they take up residence and undergo maturation following a complex network of signaling and transcription factors (Tore et al., 2001; Beghdadi et al., 2011). Mast cells are highly responsive to activation of the HPA axis, as they express five isoforms of the CRF1 receptor, a single isoform of the CRF2 receptor, and contain one of the largest peripheral stores of CRF (Theoharides et al., 2004). The hallmark form of mast cell activation is through degranulation and release of their intracellular stores; however, stress can induce release of cytokines and growth factors in the absence of partial or complete degranulation (Theoharides et al., 2004; Anand et al., 2012). As mast cells are often found in close proximity to nerves and their contents are known to act on nociceptive fibers, it is strongly asserted that mast cells play a key role in peripheral sensitization in chronic pain. Biopsies of affected tissues from patients with IBS (Barbara et al., 2004), IC/PBS (Anand et al., 2012), vulvodynia (Goetsch et al., 2010), and CP/CPPS (Done et al., 2012) have all shown increased mast cell infiltration, degranulation, and/or exuded contents.
Impact of Early Life Stress or Insult on the HPA Axis
Exposure to early life stress or trauma is a significant risk factor for developing HPA abnormalities and associated chronic pain syndromes (Anand, 1998). The national rate of child maltreatment in the United States has consistently risen over the past decade (U.S. Department of Health and Human Services, Administration for Children and Families, and Administration on Children, Youth and Families, Children’s Bureau, 2012) and exposure to adverse childhood experiences (ACEs) significantly increases the risk of developing chronic disease and disability later in life (Gilbert et al., 2015). Although medical advancements have allowed for prematurely-born babies to survive at increasingly earlier gestational stages, prolonged stays in the neonatal intensive care unit (NICU) provide chronic exposure to numerous stressors, including repeated invasive procedures and prolonged periods of maternal separation (Simons et al., 2003). A preterm neonate in the NICU is subjected to approximately 16 interventional procedures per day, 10 of which are considered to be painful (Carbajal et al., 2008). The likelihood of NICU admission is higher for full-term babies born to women aged 40–44, an age group whose birth rate rose 4% in 2015 (Martin et al., 2017). If current trends continue, increasing rates of childhood adversity and stress will add extra layers of complexity onto already prevalent and hard-to-treat chronic pain disorders.
Rodent models incorporating early life stress or peripheral insult have demonstrated disrupted functioning of the HPA axis. Neonatal maternal separation (NMS) in rodents has been used for several decades as a model of early life stress that significantly impacts the functioning of the HPA axis (McIntosh et al., 1999; Romeo et al., 2003; Daniels et al., 2004; Millstein and Holmes, 2007). In rats, the neonatal period between postnatal day 1 (P1) through P14 is critical for neurological development. In humans, this period begins prenatally and lasts until age 5 (Perry and Pollard, 1998); the nervous system is exceptionally pliant to nurturing and adverse events during this critical window of development (Teicher et al., 2002). Maternal deprivation in rats lowered GR expression in the hippocampus and cortex, resulting in protracted responses to acute stress and deficient GC feedback in rats (Ladd et al., 2004). Altered hypothalamic and limbic CRF receptor and GR expression has also been reported (Ladd et al., 2004; Plotsky et al., 2005; Aisa et al., 2008; O’Malley et al., 2011a). These animals also had increased corticosterone levels (O’Mahony et al., 2009) and prolonged ACTH release following stressful events (Romeo et al., 2003; Ladd et al., 2004; Plotsky et al., 2005; Aisa et al., 2008); induced depressive behaviors including heightened anxiety behaviors in an open field area (McIntosh et al., 1999; Daniels et al., 2004; Millstein and Holmes, 2007; O’Malley et al., 2010); and increased visceromotor response (VMR) to colorectal balloon distention (CRD; Coutinho et al., 2002; Zhang et al., 2009; O’Malley et al., 2010; Moloney et al., 2012). The hyperalgesia was exacerbated following exposure to water avoidance stress (WAS) and was prevented by a preemptive administration of a selective CRF1 antagonist (Schwetz et al., 2005), indicating that CRF may play a critical role in the development of visceral hypersensitivity. Altered functioning of the HPA axis likely drove the heightened sensitivity to acute stressors and anxiety-provoking situations in NMS rats (Taché et al., 2001; O’Malley et al., 2011a), as CRF mRNA and CRF1 immunoreactivity were both elevated following NMS in PVN, amygdala, and locus coeruleus (Plotsky et al., 2005). Moreover, NMS rats displayed significantly higher levels of c-Fos expression in the cingulate cortex and superficial and deeper laminae of the spinal cord in response to colorectal distention than did naïve rats (Chung et al., 2007; Ren et al., 2007), suggesting that early life stress likely induced functional changes in the central descending modulatory system that may have contributed to hyperalgesia (Sengupta, 2009).
Rodent models of peripheral inflammation or insult during neonatal development also demonstrate altered HPA axis functioning in adulthood. Rat pups that were subjected to gastric suctioning from postnatal day 2–12 developed colorectal sensitivity as adults that could be mitigated by CRF1 antagonist treatment prior to suctioning (Smith et al., 2007). Our lab demonstrated that neonatal vaginal irritation (NVI) in mice resulted in vaginal and colorectal hypersensitivity that could also be prevented by CRF1 antagonist treatment at the time of insult (Pierce et al., 2015). Many additional studies have used neonatal peripheral inflammation or insult in rodents as preclinical models of chronic pelvic pain disorders with or without effects on multiple organ systems. These include neonatal bladder irritation with zymosan (Randich et al., 2006; Miranda et al., 2011) or neonatal colon irritation with mustard oil (Al-Chaer et al., 2000; Christianson et al., 2010), acetic acid (Winston et al., 2007), or colorectal distension (Al-Chaer et al., 2000; Lin and Al-Chaer, 2003; Wang et al., 2008). All of these studies reported increased visceral sensitivity with alterations in central and/or peripheral nociceptive processing; however, none directly interrogated the potential role of altered HPA axis regulation or output.
Clinical Evidence of Early Life Stress-Related Visceral Pain Syndromes
Experience of early life adverse childhood events has been linked to maladjusted stress response in adulthood, and can serve as a risk factor for developing mood and functional pain disorders later in life (Grunau et al., 1994; Anand, 1998; Whitfield and Grunau, 2000; Mayson and Teichman, 2009; O’Malley et al., 2011a,b; Maniam et al., 2014). Accordingly, patients suffering from chronic pelvic pain syndromes commonly report having endured early life stress and often experience stress-related symptom onset or increased severity. Symptoms of more than one syndrome, as well as comorbid mood disorders, are often experienced in chronic pelvic pain patients (Green et al., 2010; Warren et al., 2011b; Potts and Payne, 2012; Bullones Rodríguez et al., 2013; Suskind et al., 2013), complicating already less-than-ideal treatment strategies and compounding the negative impact on quality of life. In this section, we will discuss evidence of early life-induced symptomology and the potential role of altered HPA axis signaling in four common chronic pelvic pain syndromes.
Irritable Bowel Syndrome
IBS is the most commonly diagnosed chronic pelvic pain disorder, as well as functional gastrointestinal disorder. IBS is characterized by chronic or recurrent abdominal pain or discomfort and altered bowel habits. Symptom onset or exacerbation is often triggered by stress in patients suffering from IBS (Mayer et al., 2001; Blanchard et al., 2008; Dufton et al., 2008; Stasi et al., 2012; Fond et al., 2014). A reported history of early life stress, such as premature birth, neglect, abuse, loss of a parent, or parental discord, is a significant risk factor for developing IBS in adulthood (Barreau et al., 2007; Chitkara et al., 2008; Videlock et al., 2009). Premature birth or low birth weight increases the risk of developing IBS, in part due to a developmentally immature gastrointestinal system at birth (Berseth, 1989; Bengtson et al., 2006). Gastric suctioning shortly after birth can also increase the risk of developing functional gastrointestinal disorders (Anand et al., 2004).
Improper functioning of the HPA axis, particularly over-activity, has been noted in sub-populations of IBS sufferers. In a study that investigated the impact of childhood trauma on HPA axis responsiveness in IBS patients, subjects that reported early adverse life events had significantly higher salivary cortisol levels following sigmoidoscopy than control patients (Videlock et al., 2009). In those patients with IBS, a faster resolution of stress-induced cortisol correlated with lower symptom severity and higher quality of life ratings. Furthermore, over-activation of the HPA axis was more related to a history of early life adverse events than to the presence of IBS, suggesting that exposure to early life stress alone may not be sufficient for the manifestation of IBS. In a separate study that did not interrogate the role of early life stress exposure, female IBS patients had significantly lower basal ACTH levels, but higher basal and stimulated plasma cortisol levels. They also displayed a positive correlation between plasma cortisol and reported anxiety levels prior to sigmoidoscopy (Chang et al., 2009). Based on preclinical rodent studies, increased CRF/CRF1 signaling in both the brain and colon has been proposed to contribute towards comorbid anxiety/depression in female diarrhea-predominant IBS patients (Taché et al., 2005). Clinical studies looking at the effectiveness of CRF1 antagonist treatment have had mixed success in patients. An initial study reported no effect of CRF1 antagonist on influencing colonic transit time, stool frequency, or consistency in female diarrhea-predominant IBS patients (Sweetser et al., 2009). Reported anxiety and depression scores were not different between placebo and treatment groups; however, the authors state that 7/30 patients reported anxiety scores that indicate significant anxiety. Considering the role of comorbidity in HPA axis over-activity, it would have been informative to determine whether the CRF1 antagonist was more or less effective in these highly anxious patients. A later study addressed this relationship by measuring the effectiveness of a CRF1 antagonist on mediating the functional connectivity of the emotional-arousal circuit using fMRI prior to the expectation of abdominal pain (Hubbard et al., 2011). In this study, the CRF1 antagonist, relative to placebo, significantly reduced the blood oxygen level-dependent signal in the hypothalamus in IBS patients with average or high levels of anxiety during expectation of abdominal pain. The authors suggested that their findings, when compared to previous, negative outcomes with CRF1 antagonists, support the idea that targeting the CRF/CRF1 signaling pathway may only be beneficial in a subset of patients who also display stress sensitivity, anxiety, and hyper-responsiveness of the HPA axis. These studies emphasize the etiologic heterogeneity underlying IBS, despite similar symptomology. Further understanding of IBS and comorbidities will be essential in designing appropriate, personalized treatment strategies.
Due to the significant impact of early life stress on IBS development, NMS is a frequently used preclinical model for understanding the mechanisms underlying early life stress-induced gastrointestinal hypersensitivity and dysfunction. Adult rodents that were exposed to NMS exhibit many of the same colorectal sensitivities and functional and neuroimmune abnormalities observed in human cases. These include increased growth factor and cytokine expression, such as NGF, IL-6, IL-1β, IL-2, IL-4, IL-10, and interferon (IFN)-γ, as well as infiltration of mast cells, in the distal colon, all of which can sensitize peripheral nociceptors and enhance visceral perception (Barreau et al., 2004a,b; Daniels et al., 2009; van den Wijngaard et al., 2009, 2012; O’Malley et al., 2011a,b; Lennon et al., 2013). While these studies have all been performed in rat models, studies from our lab using mice have revealed little to no effect of NMS on colorectal sensitivity, cytokine expression, or mast cell infiltration, with the exception of increasing susceptibility to trinitrobenzene sulfonic acid (TNBS)-induced colitis in male mice (Fuentes et al., 2016; Pierce et al., 2016). One other study on NMS in mice reported an increase in colonic hypersensitivity; however, this model also incorporated unpredictable maternal stress with unpredictable NMS, which likely altered the outcomes of this study, as it relates to our findings (Moloney et al., 2012). Other recent studies have highlighted the influence that the gut microbiota has on stress-related gastrointestinal disorders (Moloney et al., 2016), which can be impacted by age, sex, diet, genotype, and environment (Laukens et al., 2016), making it difficult to compare the outcomes of similar studies even in the same strain of mice. It is likely that contributing variables outside of species, sex, strain, and stressor-type will emerge as this field continues to move forward.
Interstitial Cystitis/Painful Bladder Syndrome
The chief complaints of IC/PBS patients include idiopathic pelviperineal pain and increased urinary urgency and frequency. Diagnosis of IC/PBS is largely symptom-based, particularly irritative voiding and referred lower urinary tract pain, after excluding other pathologies that mimic symptoms of IC/PBS (e.g., urinary tract infection; Hanno et al., 2011). As with other functional pain disorders, IC/PBS patients are more likely to have a history of early life stress or adversity. In a study of 87 female patients with IC/PBS, just over half reported a history of abuse, the majority of which occurred during childhood or adolescence (Peters et al., 2008). In another study of 207 female IC/PBS patients, 24% reported sexual abuse prior to the age of 17, compared to 15% in the control group. Those IC/PBS patients with a history of abuse had greater comorbid depression, anxiety, and mental functioning, as well as greater pain, but not functional symptoms, than those who did not report abuse (Nickel et al., 2011). Patients with IC/PBS who reported childhood trauma perpetuated by someone with whom they had a close relationship had a greater degree of comorbid anxiety, despite similar urogenital symptom severity, compared to IC/PBS patients without a history of abuse (Chiu et al., 2017). Multiple studies have reported that patients with IC/PBS have a higher incidence of childhood bladder problems, including recurrent urinary tract infections (Jones and Nyberg, 1997; Peters et al., 2008).
Similar to the aforementioned fMRI study illustrating increased functional connectivity in the emotional-arousal circuit of IBS patients, multiple studies have investigated changes in brain connectivity in patients with IC/PBS. Patients with more widespread pain, affecting multiple and disparate parts of the body outside of the pelvis, had greater increases in gray matter volume and functional connectivity of sensorimotor and insular cortices (Kutch et al., 2017). These changes also correlated with decreased physical and mental function. A separate study also correlated widespread pain with increased comorbid anxiety and depression and a lesser quality of life (Lai et al., 2017). While these studies do not directly address the role of the HPA axis or early life stress, the evidence linking these occurrences with comorbidity of mood disorder suggest that these patients may have a higher likelihood of early adverse events and/or increased activation of the HPA axis. In support of the latter, molecular and histological evidence of increased CRF signaling is present in tissue samples, serum, and/or urine from IC/PBS patients. Tissue biopsies from IC/PBS patients have revealed increased mast cell infiltration (Kastrup et al., 1983; Christmas and Rode, 1991; Spanos et al., 1997; Peeker et al., 2000; Tomaszewski et al., 2001; Larsen et al., 2008) and close proximity to densely-populated substance P (SP)-immunopositive nerve fibers (Pang et al., 1995). Mast cell tryptase was elevated in IC/PBS urine (Boucher et al., 1995; Okragly et al., 1999) and elevated NGF levels in seminal plasma have been demonstrated to be directly correlated with pain severity (Miller et al., 2002; Watanabe et al., 2011). Elevated concentrations of NGF, histamine, and pro-inflammatory cytokines have also been observed in IC/PBS patients’ serum (Jiang et al., 2013) and urine (Yun et al., 1992; Lotz et al., 1994; Jacobs et al., 2010; Corcoran et al., 2013).
CRF is not only involved in inflammation and pain signaling in the context of IC/PBS, BN, located in the dorsolateral pontine tegmentum, is central to the micturition pathway and uses CRF as a neurotransmitter within this relay (Vincent and Satoh, 1984; Sakanaka et al., 1987; Valentino et al., 1995). Neurons from the BN project to the lumbosacral parasympathetic nucleus, where preganglionic neurons innervate the pelvic viscera, and send collateral branches to the locus coeruleus (Sasaki, 2005; Valentino et al., 2011). Information relayed by bladder afferents from both the spinal cord, as well as the periaqueductal gray region (Blok et al., 1995; Ding et al., 1997; Rouzade-Dominguez et al., 2003b), stimulates BN neurons to induce increases in bladder pressure, cause bladder contraction, inhibit the external urethral sphincter, and relax the urethra (Sasaki, 2005). Activation of the locus coeruleus increases arousal and shifts the mode of attention to modify behavior to best coordinate visceral functions, such as voiding, through dense projections throughout the cortex (Berridge and Waterhouse, 2003; Aston-Jones and Cohen, 2005). Neurons in the BN have been shown to be sympathetically linked to and able to influence the distal colon, genitals, and bladder, allowing for co-regulation of multiple organ systems under certain conditions, such as stress (Marson, 1995; Marson and McKenna, 1996; Rouzade-Dominguez et al., 2003a). This organization may contribute towards the comorbidity of multiple pelvic visceral symptoms as CRF may play a role in regulating bladder or colonic motility as a component of the stress response (Valentino et al., 1999). Repeated social defeat stress in adult rats has been shown to increase CRF expression within BN (Kiddoo et al., 2006; Wood et al., 2009) and promote urine retention (Wood et al., 2009). Excitatory effects of CRF on colonic motoneurons may contribute to stress-related increases in colonic motility and symptoms of IBS (Pavcovich et al., 1998; Valentino et al., 1999); however, in vivo cystometry studies have suggested both excitatory and inhibitory effects of CRF on micturition by evaluating the effects of CRF and CRF antagonists administered systemically or intrathecally (Klausner and Steers, 2004; Klausner et al., 2005; Kiddoo et al., 2006). Treatment with CRF1 antagonist reduced both bladder filling and micturition volumes that were initially increased due to intrathecal administration of CRF or Ucn2 (Kiddoo et al., 2006). Klausner et al. (2005), however, observed the opposite effect. CRF administration decreased micturition volume in normal Wistar rats, and intrathecal administration of astressin, a non-selective CRF1/CRF2 antagonist, increased void volumes of high-anxiety Wistar-Kyoto rats (Klausner et al., 2005). The exact role of CRF expression in BN in humans, particularly as it relates to IC/PBS, has yet to be elucidated, however these studies and others suggest it may play a pivotal role in stress-related symptomology.
Preclinical models incorporating early life stress and bladder inflammation have been used to investigate possible mechanisms underlying IC/PBS. Rats treated with intravesicular zymosan, an irritant component of the yeast cell wall, on postnatal days 14 and 16 displayed increased micturition frequency and heightened VMR and arterial blood pressure during urinary bladder distension (UBD) following reinflammation with zymosan as adults (Randich et al., 2006). Later investigations into this model revealed disruption of descending inhibitory pathways (DeBerry et al., 2007) and increased plasma extravasation and neuropeptide release after intravesicular mustard oil application (DeBerry et al., 2010). Studies from our laboratory showed that NMS in female mice increases the VMR during UBD both at baseline and following an acute exposure to WAS (Pierce et al., 2016). Bladder mast cell degranulation and pro-inflammatory gene expression profiles were significantly increased in female NMS mice, along with molecular evidence of altered hippocampal input onto the HPA axis. Stress-induced bladder hypersensitivity, vascular permeability, and upregulated peripheral expression of TNF-α and IL-10 in adult rat models have been described as being driven by a CRF2 rather than a CRF1-mediated mechanism (Robbins and Ness, 2008; Huang et al., 2009; Boucher et al., 2010; Novembri et al., 2011). Intrathecal administration of a CRF2 antagonist prior to UBD attenuated unpredictable footshock-induced urinary bladder hypersensitivity as measured by VMR (Robbins and Ness, 2008).
Chronic Prostatitis/Chronic Pelvic Pain Syndrome
CP/CPPS is characterized by chronic, idiopathic pain in the lower abdomen, rectum, perineum, prostate, penis, and/or testicles, with or without urinary symptoms (Duloy et al., 2007; Murphy et al., 2009), and is diagnosed symptomatically due to the lack of associated pathology (Pontari and Ruggieri, 2004). CP/CPPS has detrimental effects on quality of life, comparable to myocardial infarction, angina, Crohn’s disease, and diabetes (Murphy et al., 2009; Strauss and Dimitrakov, 2010). Little is known regarding the influence of early life stress or adversity on the later development of CP/CPPS; however, the significant overlap with IC/PBS and susceptibility to stress suggests a potential early life component to the disorder. Additionally, because male patients have been reported to experience significant psychological disturbances (McNaughton Collins et al., 2001; Tripp et al., 2006; Anderson et al., 2008, 2009; Nickel et al., 2008; Shoskes et al., 2009; Ahn et al., 2012; Riegel et al., 2014) similar to female patients that have experienced early life stress (Heim et al., 1998, 2002; Mayson and Teichman, 2009; Nickel et al., 2010; Warren et al., 2011a, b), it has been hypothesized that men with CP/CPPS may have similar histories. Findings from the Multi-Disciplinary Approach to the Study of Chronic Pelvic Pain (MAPP) Research Network reported that both male and female patients suffering from urologic chronic pelvic pain syndromes (UCPPS; specifically, CP/CPPS in males and IC/PBS in females) exhibit greater psychological distress, poorer coping, higher levels of current and lifetime stress, and more widespread pain symptoms that contribute to poorer self-reported quality of life than sex- and education-matched healthy controls. The study goes on to report that individuals living with UCPPS experience greater incidences of early life and adult trauma compared to healthy controls (though women more significantly than men; Naliboff et al., 2015). To our knowledge, only one study has looked explicitly at the prevalence of early childhood trauma and developing CP/CPPS in adulthood: the Boston Area Community Health (BACH) survey examined the relationship between sexual, physical, or emotional abuse and symptoms suggestive of CP/CPPS. Hu et al. (2007) concluded that among the 2301 men recruited, reporting abuse increased the odds of also presenting symptoms suggestive of CP/CPPS, though greater frequency of both childhood and adult abuse were associated with increased prevalence of CP/CPPS symptomology. Furthermore, if a subject reported more than one type of abuse, he was at increased odds of reporting both increased pain and urinary scores of the NIH chronic prostatitis symptom index (CPSI), suggesting a cumulative effect (Hu et al., 2007).
As with the other chronic pelvic pain syndromes, the etiology underlying CP/CPPS is largely unknown, although stress has been shown to worsen or bring on symptoms. Evidence of altered HPA axis functioning has been demonstrated primarily by increased mast cell activation in the affected tissue. Though studies have reported varied degrees of mast cell degranulation and activation, CP/CPPS biopsies were observed having altered granular structure (Theoharides et al., 1990) and decreased numbers of intact mast cells (Amir et al., 1998), suggesting that mast cell activation without complete degranulation and increased rate of complete degranulation could occur in CP/CPPS. Concentrations of tryptase and carboxypeptidase A (CPA3), a marker of mast cell activation, were increased in urine samples from CP/CPPS patients (Roman et al., 2014). Mast cell tryptase and NGF levels were also increased in expressed prostatic secretions from patients (Done et al., 2012). Furthermore, Anderson et al. (2008, 2009) have reported that men with CP/CPPS, compared to healthy controls, have a greater slope of waking cortisol response (Anderson et al., 2008) and delayed ACTH release correlating with significant psychological disturbances (phobic anxiety, perceived stress, depression, etc.) in response to an acute stress (Anderson et al., 2009), suggesting altered HPA axis function.
The majority of published rodent models of CP/CPPS are centered around experimental infection; injection of exogenous antigens, androgens, or irritants (Pierce and Christianson, 2015); or invasive surgery (Vykhovanets et al., 2007) and, as such, are largely undefined in their mechanism of CP/CPPS development. We have recently begun investigating the use of NMS in mice as a model of CP/CPPS. Adult male NMS mice display significant perigenital mechanical hypersensitivity, as well as significantly increased mast degranulation in the prostate and bladder (Fuentes et al., 2015; current issue). This model also displays increased micturition frequency and output and molecular evidence of altered HPA functioning (current issue). Further investigation of NMS in mice will hopefully provide a more clinically relevant rodent model of CP/CPPS for investigation of potential therapies and interventions.
Vulvodynia
Vulvodynia presents clinically as vulvar discomfort, most often as a burning pain, with no identifiable pathological or neurological evidence of disease (Moyal-Barracco and Lynch, 2004). Although there is variability in clinical presentation, the hallmark symptom is allodynia and/or burning, stinging, or itching of the vulva, vestibule, or vaginal canal (McKay, 1988; Edwards, 2004). Early life adverse events are strongly linked with an increased likelihood of vulvodynia in adulthood and are attributed to dysfunctional regulation of the HPA axis. Vulvodynia was strongly associated with a sense of danger or abuse, particularly by a primary family member, during childhood (Harlow and Stewart, 2005). A separate study did not find an increase in childhood abuse; however, parental divorce was more common among women with vulvodynia when compared to controls (Plante and Kamm, 2008). In a survey of women diagnosed with IC/PBS, 63% reported vulvar pain and 29% reported a history of abuse (Carrico et al., 2009). Women with vulvodynia have blunted serum cortisol cycles (Ehrström et al., 2009) and, like with other chronic pelvic pain syndromes, symptom severity is increased following acute stress exposure (Gordon et al., 2003). Defective regulation of the downstream inflammatory response is also observed as increased mast cell degranulation and infiltration within vestibular biopsies when compared to controls (Bornstein et al., 2008). Mast cell-derived heparanase is increased in the vestibule of vulvodynia patients (Bornstein et al., 2008), where it serves to degrade the heparin sulfate component of basement membranes and the extracellular matrix as needed for leukocyte infiltration (Goldberg et al., 2013). Age-related changes have been observed, as mast cell activation increases in post- compared to pre-menopausal vulvodynia patients, despite similar expression levels of estrogen receptor α and evidence of neural hyperplasia (Leclair et al., 2013). Increases in proinflammatory cytokines IL-1 and TNF-α have also been reported (Foster and Hasday, 1997; Jeremias et al., 2000), both of which have been demonstrated to be produced by activated mast cells (Galli et al., 2005). Moreover, decreased IL-1 receptor antagonist (IL-1RA) activity has been observed in vulvodynia patients, and has been associated with increased IL-1 beta activity, which has been observed in tissues sampled from patients (Foster et al., 2007). Inflammatory challenge in patients resulted in blunted IL-1RA response (Gerber et al., 2002). The prevention of stress-induced elevations in IL-1β and associated increase of HPA axis activity by pretreatment with IL-1RA (Gądek-Michalska et al., 2011), suggests that peripheral cytokines can influence central perceptions of pain through limbic control.
Despite the clinical prevalence of vulvodynia, few animal models have been published investigating preclinical mechanisms possibly underlying this disorder. Animal models have exploited both the hormonal and allodynic characteristics of vulvodynia by observing peripheral neuron sprouting under low estrogenic conditions (Bhattacherjee et al., 2013), vulvar allodynia following repeated vulvovaginal fungal infection (Farmer et al., 2011), and oxazolone-induced delayed-type contact hypersensitivity of the vulva (Martinov et al., 2013). We have developed two separate preclinical models of vulvodynia using NMS (Pierce et al., 2014) and NVI with zymosan (Pierce et al., 2015). Both models develop significant vaginal hypersensitivity in adulthood with associated increases in HPA axis gene expression levels and/or output. As mentioned above, the vaginal hypersensitivity in the NVI model was prevented by pretreating with CRF1 antagonist prior to zymosan treatment, verifying the involvement of the HPA axis in the development of vaginal hypersensitivity (Pierce et al., 2015).
Centralized Pain Phenotype
Obvious similarities are present between these previously discussed syndromes, including a lack of overt pathology (with the exception of IC) and disruption of proper functioning of the HPA axis. Patients diagnosed with one of these disorders are very often diagnosed with, or present with symptoms of, additional functional pain syndromes and/or mood disorders, such as anxiety, panic disorder, or depression (Bullones Rodríguez et al., 2013). This high rate of comorbidity, as well as similar symptomology, has led to the hypothesis that these syndromes are different manifestations of a centralized pain phenotype possibly resulting from early life trauma (Nicol et al., 2016). Recent work from the MAPP Research Network has shown that patients with IC/PBS are less likely to have pain restricted to the pelvis (25% of patients) than intermediate pain around the pelvis (37% of patients) or widespread pain throughout the body (38% of patients; Lai et al., 2017). Patients with a greater number of painful body sites also reported higher pain, anxiety, and depression scores, along with worse quality of life. Changes in functional connectivity between relevant brain structures, as well as increased gray matter, have also been correlated with worsened pain and mental function scores (Kutch et al., 2017). These observations support the need for fully phenotyping patients prior to prescribing pharmacological or other interventional treatments. While patients reporting pain only in the affected organ may respond to peripherally-restricted therapies, most patients have multiple comorbidities and may respond more effectively to therapies targeted to the central nervous system. Future work in our lab and others are exploring the role of the central nervous system, and interventions such as exercise, in preclinical models of chronic pelvic pain syndromes to evaluate their efficacy in preventing or alleviating symptomology similar to that seen in humans.
Conclusion
Patients with chronic pelvic pain disorders commonly report having experienced early life stress, trauma, or peripheral insult, including repeated infections. The strong influence that stress has on initiating and/or exacerbating symptoms associated with pelvic pain disorders further strengthens this relationship between stress and pain, likely due to the influence of the HPA axis. Development of clinical therapies and preclinical animal models should heed this important and influential relationship to improve treatment options and better understand the underlying etiology of these related disorders.
Author Contributions
IMF and JAC: equal contribution for the literature search and article preparation.
Funding
This work was supported by National Institutes of Health (NIH) grants R01 DK099611, R01 DK103872, T32 HD057850.
Conflict of Interest Statement
The authors declare that the research was conducted in the absence of any commercial or financial relationships that could be construed as a potential conflict of interest.
Acknowledgments
We acknowledge that part of this work appears in dissertation form.
References
Ahn, S. G., Kim, S. H., Chung, K. I., Park, K. S., Cho, S. Y., and Kim, H. W. (2012). Depression, anxiety, stress perception, and coping strategies in korean military patients with chronic prostatitis/chronic pelvic pain syndrome. Korean J. Urol. 53, 643–648. doi: 10.4111/kju.2012.53.9.643
Aisa, B., Tordera, R., Lasheras, B., Del Rio, J., and Ramirez, M. J. (2008). Effects of maternal separation on hypothalamic-pituitary-adrenal responses, cognition and vulnerability to stress in adult female rats. Neuroscience 154, 1218–1226. doi: 10.1016/j.neuroscience.2008.05.011
Al-Chaer, E. D., Kawasaki, M., and Pasricha, P. J. (2000). A new model of chronic visceral hypersensitivity in adult rats induced by colon irritation during postnatal development. Gastroenterology 119, 1276–1285. doi: 10.1053/gast.2000.19576
Amir, T., Pai, R. R., and Raghuveer, C. V. (1998). Mast cell profile in prostatic lesions. Indian J. Med. Sci. 52, 507–513.
Anand, K. J. (1998). Clinical importance of pain and stress in preterm neonates. Biol. Neonate 73, 1–9. doi: 10.1159/000013953
Anand, K. J., Runeson, B., and Jacobson, B. (2004). Gastric suction at birth associated with long-term risk for functional intestinal disorders in later life. J. Pediatr. 144, 449–454. doi: 10.1016/j.jpeds.2003.12.035
Anand, P., Singh, B., Jaggi, A. S., and Singh, N. (2012). Mast cells: an expanding pathophysiological role from allergy to other disorders. Naunyn Schmiedebergs Arch. Pharmacol. 385, 657–670. doi: 10.1007/s00210-012-0757-8
Anderson, R. U., Orenberg, E. K., Chan, C. A., Morey, A., and Flores, V. (2008). Psychometric profiles and hypothalamic-pituitary-adrenal axis function in men with chronic prostatitis/chronic pelvic pain syndrome. J. Urol. 179, 956–960. doi: 10.1016/j.juro.2007.10.084
Anderson, R. U., Orenberg, E. K., Morey, A., Chavez, N., and Chan, C. A. (2009). Stress induced hypothalamus-pituitary-adrenal axis responses and disturbances in psychological profiles in men with chronic prostatitis/chronic pelvic pain syndrome. J. Urol. 182, 2319–2324. doi: 10.1016/j.juro.2009.07.042
Aston-Jones, G., and Cohen, J. D. (2005). An integrative theory of locus coeruleus-norepinephrine function: adaptive gain and optimal performance. Annu. Rev. Neurosci. 28, 403–450. doi: 10.1146/annurev.neuro.28.061604.135709
Bale, T. L., Contarino, A., Smith, G. W., Chan, R., Gold, L. H., Sawchenko, P. E., et al. (2000). Mice deficient for corticotropin-releasing hormone receptor-2 display anxiety-like behaviour and are hypersensitive to stress. Nat. Genet. 24, 410–414. doi: 10.1038/74263
Bale, T. L., Picetti, R., Contarino, A., Koob, G. F., Vale, W. W., and Lee, K. F. (2002). Mice deficient for both corticotropin-releasing factor receptor 1 (CRFR1) and CRFR2 have an impaired stress response and display sexually dichotomous anxiety-like behavior. J. Neurosci. 22, 193–199.
Bale, T. L., and Vale, W. W. (2004). CRF and CRF receptors: role in stress responsivity and other behaviors. Annu. Rev. Pharmacol. Toxicol. 44, 525–557. doi: 10.1146/annurev.pharmtox.44.101802.121410
Barbara, G., Stanghellini, V., De Giorgio, R., Cremon, C., Cottrell, G. S., Santini, D., et al. (2004). Activated mast cells in proximity to colonic nerves correlate with abdominal pain in irritable bowel syndrome. Gastroenterology 126, 693–702. doi: 10.1053/j.gastro.2003.11.055
Barreau, F., Cartier, C., Ferrier, L., Fioramonti, J., and Bueno, L. (2004a). Nerve growth factor mediates alterations of colonic sensitivity and mucosal barrier induced by neonatal stress in rats. Gastroenterology 127, 524–534. doi: 10.1053/j.gastro.2004.05.019
Barreau, F., Ferrier, L., Fioramonti, J., and Bueno, L. (2004b). Neonatal maternal deprivation triggers long term alterations in colonic epithelial barrier and mucosal immunity in rats. Gut 53, 501–506. doi: 10.1136/gut.2003.024174
Barreau, F., Ferrier, L., Fioramonti, J., and Bueno, L. (2007). New insights in the etiology and pathophysiology of irritable bowel syndrome: contribution of neonatal stress models. Pediatr. Res. 62, 240–245. doi: 10.1203/pdr.0b013e3180db2949
Beghdadi, W., Madjene, L. C., Benhamou, M., Charles, N., Gautier, G., Launay, P., et al. (2011). Mast cells as cellular sensors in inflammation and immunity. Front. Immunol. 2:37. doi: 10.3389/fimmu.2011.00037
Bengtson, M. B., Rønning, T., Vatn, M. H., and Harris, J. R. (2006). Irritable bowel syndrome in twins: genes and environment. Gut 55, 1754–1759. doi: 10.1136/gut.2006.097287
Berridge, C. W., and Waterhouse, B. D. (2003). The locus coeruleus-noradrenergic system: modulation of behavioral state and state-dependent cognitive processes. Brain Res. Rev. 42, 33–84. doi: 10.1016/s0165-0173(03)00143-7
Berseth, C. L. (1989). Gestational evolution of small intestine motility in preterm and term infants. J. Pediatr. 115, 646–651. doi: 10.1016/s0022-3476(89)80302-6
Bhattacherjee, A., Rumi, M. A., Staecker, H., and Smith, P. G. (2013). Bone morphogenetic protein 4 mediates estrogen-regulated sensory axon plasticity in the adult female reproductive tract. J. Neurosci. 33, 1050–1061. doi: 10.1523/JNEUROSCI.1704-12.2013
Bittencourt, J. C., Vaughan, J., Arias, C., Rissman, R. A., Vale, W. W., and Sawchenko, P. E. (1999). Urocortin expression in rat brain: evidence against a pervasive relationship of urocortin-containing projections with targets bearing type 2 CRF receptors. J. Comp. Neurol. 415, 285–312. doi: 10.1002/(sici)1096-9861(19991220)415:3<285::aid-cne1>3.3.co;2-s
Blanchard, E. B., Lackner, J. M., Jaccard, J., Rowell, D., Carosella, A. M., Powell, C., et al. (2008). The role of stress in symptom exacerbation among IBS patients. J. Psychosom. Res. 64, 119–128. doi: 10.1016/j.jpsychores.2007.10.010
Blok, B. F., De Weerd, H., and Holstege, G. (1995). Ultrastructural evidence for a paucity of projections from the lumbosacral cord to the pontine micturition center or M-region in the cat: a new concept for the organization of the micturition reflex with the periaqueductal gray as central relay. J. Comp. Neurol. 359, 300–309. doi: 10.1002/cne.903590208
Bornstein, J., Cohen, Y., Zarfati, D., Sela, S., and Ophir, E. (2008). Involvement of heparanase in the pathogenesis of localized vulvodynia. Int. J. Gynecol. Pathol. 27, 136–141. doi: 10.1097/pgp.0b013e318140021b
Boucher, W., El-Mansoury, M., Pang, X., Sant, G. R., and Theoharides, T. C. (1995). Elevated mast cell tryptase in the urine of patients with interstitial cystitis. Br. J. Urol. 76, 94–100. doi: 10.1111/j.1464-410x.1995.tb07840.x
Boucher, W., Kempuraj, D., Michaelian, M., and Theoharides, T. C. (2010). Corticotropin-releasing hormone-receptor 2 is required for acute stress-induced bladder vascular permeability and release of vascular endothelial growth factor. BJU Int. 106, 1394–1399. doi: 10.1111/j.1464-410x.2010.09237.x
Bremne, J. D., and Vermetten, E. (2001). Stress and development: behavioral and biological consequences. Dev. Psychopathol. 13, 473–489. doi: 10.1017/s0954579401003042
Buckley, M. M., O’Halloran, K. D., Rae, M. G., Dinan, T. G., and O’Malley, D. (2014). Modulation of enteric neurons by interleukin-6 and corticotropin-releasing factor contributes to visceral hypersensitivity and altered colonic motility in a rat model of irritable bowel syndrome. J. Physiol. 592, 5235–5250. doi: 10.1113/jphysiol.2014.279968
Bullones Rodríguez, M. A., Afari, N., Buchwald, D. S., and National Institute of Diabetes and Digestive and Kidney Diseases Working Group on Urological Chronic Pelvic Pain. (2013). Evidence for overlap between urological and nonurological unexplained clinical conditions. J. Urol. 189, S66–S74. doi: 10.1016/j.juro.2012.11.019
Carbajal, R., Rousset, A., Danan, C., Coquery, S., Nolent, P., Ducrocq, S., et al. (2008). Epidemiology and treatment of painful procedures in neonates in intensive care units. JAMA 300, 60–70. doi: 10.1001/jama.300.1.60
Carrico, D. J., Sherer, K. L., and Peters, K. M. (2009). The relationship of interstitial cystitis/painful bladder syndrome to vulvodynia. Urol. Nurs. 29, 233–238.
Chang, L., Sundaresh, S., Elliott, J., Anton, P. A., Baldi, P., Licudine, A., et al. (2009). Dysregulation of the hypothalamic-pituitary-adrenal (HPA) axis in irritable bowel syndrome. Neurogastroenterol. Motil. 21, 149–159. doi: 10.1111/j.1365-2982.2008.01171.x
Chatzaki, E., Crowe, P. D., Wang, L., Million, M., Taché, Y., and Grigoriadis, D. E. (2004). CRF receptor type 1 and 2 expression and anatomical distribution in the rat colon. J. Neurochem. 90, 309–316. doi: 10.1111/j.1471-4159.2004.02490.x
Chitkara, D. K., van Tilburg, M. A., Blois-Martin, N., and Whitehead, W. E. (2008). Early life risk factors that contribute to irritable bowel syndrome in adults: a systematic review. Am. J. Gastroenterol. 103, 765–774; quiz 775. doi: 10.1111/j.1572-0241.2007.01722.x
Chiu, C.-D., Lee, M.-H., Chen, W.-C., Ho, H. L., and Wu, H.-C. (2017). Childhood trauma perpetrated by close others, psychiatric dysfunction, and urological symptoms in patients with interstitial cystitis/bladder pain syndrome. J. Psychosom. Res. 93, 90–95. doi: 10.1016/j.jpsychores.2016.12.014
Cho, K., and Little, H. J. (1999). Effects of corticosterone on excitatory amino acid responses in dopamine-sensitive neurons in the ventral tegmental area. Neuroscience 88, 837–845. doi: 10.1016/s0306-4522(98)00264-4
Christianson, J. A., Bielefeldt, K., Malin, S. A., and Davis, B. M. (2010). Neonatal colon insult alters growth factor expression and TRPA1 responses in adult mice. Pain 151, 540–549. doi: 10.1016/j.pain.2010.08.029
Christmas, T. J., and Rode, J. (1991). Characteristics of mast cells in normal bladder, bacterial cystitis and interstitial cystitis. Br. J. Urol. 68, 473–478. doi: 10.1111/j.1464-410x.1991.tb15388.x
Chung, E. K., Zhang, X., Li, Z., Zhang, H., Xu, H., and Bian, Z. (2007). Neonatal maternal separation enhances central sensitivity to noxious colorectal distention in rat. Brain Res. 1153, 68–77. doi: 10.1016/j.brainres.2007.03.047
Cook, C. J. (2004). Stress induces CRF release in the paraventricular nucleus, and both CRF and GABA release in the amygdala. Physiol. Behav. 82, 751–762. doi: 10.1016/j.physbeh.2004.06.013
Corcoran, A. T., Yoshimura, N., Tyagi, V., Jacobs, B., Leng, W., and Tyagi, P. (2013). Mapping the cytokine profile of painful bladder syndrome/interstitial cystitis in human bladder and urine specimens. World J. Urol. 31, 241–246. doi: 10.1007/s00345-012-0852-y
Coutinho, S. V., Plotsky, P. M., Sablad, M., Miller, J. C., Zhou, H., Bayati, A. I., et al. (2002). Neonatal maternal separation alters stress-induced responses to viscerosomatic nociceptive stimuli in rat. Am. J. Physiol. Gastrointest. Liver Physiol. 282, G307–G316. doi: 10.1152/ajpgi.00240.2001
Dallman, M. F., Levin, N., Cascio, C. S., Akana, S. F., Jacobson, L., and Kuhn, R. W. (1989). Pharmacological evidence that the inhibition of diurnal adrenocorticotropin secretion by corticosteroids is mediated via type I corticosterone-preferring receptors. Endocrinology 124, 2844–2850. doi: 10.1210/endo-124-6-2844
Daniels, W. M., Fairbairn, L. R., van Tilburg, G., McEvoy, C. R., Zigmond, M. J., Russell, V. A., et al. (2009). Maternal separation alters nerve growth factor and corticosterone levels but not the DNA methylation status of the exon 1(7) glucocorticoid receptor promoter region. Metab. Brain Dis. 24, 615–627. doi: 10.1007/s11011-009-9163-4
Daniels, W. M., Pietersen, C. Y., Carstens, M. E., and Stein, D. J. (2004). Maternal separation in rats leads to anxiety-like behavior and a blunted ACTH response and altered neurotransmitter levels in response to a subsequent stressor. Metab. Brain Dis. 19, 3–14. doi: 10.1023/b:mebr.0000027412.19664.b3
DeBerry, J., Ness, T. J., Robbins, M. T., and Randich, A. (2007). Inflammation-induced enhancement of the visceromotor reflex to urinary bladder distention: modulation by endogenous opioids and the effects of early-in-life experience with bladder inflammation. J. Pain 8, 914–923. doi: 10.1016/j.jpain.2007.06.011
DeBerry, J., Randich, A., Shaffer, A. D., Robbins, M. T., and Ness, T. J. (2010). Neonatal bladder inflammation produces functional changes and alters neuropeptide content in bladders of adult female rats. J. Pain 11, 247–255. doi: 10.1016/j.jpain.2009.07.010
De Kloet, E. R., Vreugdenhil, E., Oitzl, M. S., and Joels, M. (1998). Brain corticosteroid receptor balance in health and disease. Endocr. Rev. 19, 269–301. doi: 10.1210/edrv.19.3.0331
Ding, Y.-Q., Zheng, H.-X., Gong, L.-W., Lu, Y., Zhao, H., and Qin, B. Z. (1997). Direct projections from the lumbosacral spinal cord to Barrington’s nucleus in the rat: a special reference to micturition reflex. J. Comp. Neurol. 389, 149–160. doi: 10.1002/(sici)1096-9861(19971208)389:1<149::aid-cne11>3.0.co;2-g
Done, J. D., Rudick, C. N., Quick, M. L., Schaeffer, A. J., and Thumbikat, P. (2012). Role of mast cells in male chronic pelvic pain. J. Urol. 187, 1473–1482. doi: 10.1016/j.juro.2011.11.116
Dufton, L. M., Konik, B., Colletti, R., Stanger, C., Boyer, M., Morrow, S., et al. (2008). Effects of stress on pain threshold and tolerance in children with recurrent abdominal pain. Pain 136, 38–43. doi: 10.1016/j.pain.2007.06.012
Duloy, A. M., Calhoun, E. A., and Clemens, J. Q. (2007). Economic impact of chronic prostatitis. Curr. Urol. Rep. 8, 336–339. doi: 10.1007/s11934-007-0081-x
Edwards, L. (2004). Subsets of vulvodynia: overlapping characteristics. J. Reprod. Med. 49, 883–887.
Ehrström, S., Kornfeld, D., Rylander, E., and Bohm-Starke, N. (2009). Chronic stress in women with localised provoked vulvodynia. J. Psychosom. Obstet. Gynaecol. 30, 73–79. doi: 10.1080/01674820802604359
Farmer, M. A., Taylor, A. M., Bailey, A. L., Tuttle, A. H., MacIntyre, L. C., Milagrosa, Z. E., et al. (2011). Repeated vulvovaginal fungal infections cause persistent pain in a mouse model of vulvodynia. Sci. Transl. Med. 3:101ra91. doi: 10.1126/scitranslmed.3002613
Fond, G., Loundou, A., Hamdani, N., Boukouaci, W., Dargel, A., Oliveira, J., et al. (2014). Anxiety and depression comorbidities in irritable bowel syndrome (IBS): a systematic review and meta-analysis. Eur. Arch. Psychiatry Clin. Neurosci. 264, 651–660. doi: 10.1007/s00406-014-0502-z
Foster, D. C., and Hasday, J. D. (1997). Elevated tissue levels of interleukin-1 β and tumor necrosis factor-α in vulvar vestibulitis. Obstet. Gynecol. 89, 291–296. doi: 10.1016/s0029-7844(96)00447-4
Foster, D. C., Piekarz, K. H., Murant, T. I., LaPoint, R., Haidaris, C. G., and Phipps, R. P. (2007). Enhanced synthesis of proinflammatory cytokines by vulvar vestibular fibroblasts: implications for vulvar vestibulitis. Am. J. Obstet. Gynecol. 196, 346.e1–346.e8. doi: 10.1016/j.ajog.2006.12.038
Fries, E., Hesse, J., Hellhammer, J., and Hellhammer, D. H. (2005). A new view on hypocortisolism. Psychoneuroendocrinology 30, 1010–1016. doi: 10.1016/j.psyneuen.2005.04.006
Fuentes, I. M., Pierce, A. N., O’Neil, P. T., and Christianson, J. A. (2015). Assessment of perigenital sensitivity and prostatic mast cell activation in a mouse model of neonatal maternal separation. J. Vis. Exp. 102:e53181. doi: 10.3791/53181
Fuentes, I. M., Walker, N. K., Pierce, A. N., Holt, B. R., Di Silvestro, E. R., and Christianson, J. A. (2016). Neonatal maternal separation increases susceptibility to experimental colitis and acute stress exposure in male mice. IBRO Rep. 1, 10–18. doi: 10.1016/j.ibror.2016.07.001
Gądek-Michalska, A., Tadeusz, J., Rachwalska, P., Spyrka, J., and Bugajski, J. (2011). Effect of prior stress on interleukin-1β and HPA axis responses to acute stress. Pharmacol. Rep. 63, 1393–1403. doi: 10.1016/s1734-1140(11)70703-4
Galli, S. J., Nakae, S., and Tsai, M. (2005). Mast cells in the development of adaptive immune responses. Nat. Immunol. 6, 135–142. doi: 10.1038/ni1158
Gerber, S., Bongiovanni, A. M., Ledger, W. J., and Witkin, S. S. (2002). Defective regulation of the proinflammatory immune response in women with vulvar vestibulitis syndrome. Am. J. Obstet. Gynecol. 186, 696–700. doi: 10.1067/mob.2002.121869
Gilbert, L. K., Breiding, M. J., Merrick, M. T., Thompson, W. W., Ford, D. C., Dhingra, S. S., et al. (2015). Childhood adversity and adult chronic disease: an update from ten states and the District of Columbia, 2010. Am. J. Prev. Med. 48, 345–349. doi: 10.1016/j.amepre.2014.09.006
Goetsch, M. F., Morgan, T. K., Korcheva, V. B., Li, H., Peters, D., and Leclair, C. M. (2010). Histologic and receptor analysis of primary and secondary vestibulodynia and controls: a prospective study. Am. J. Obstet. Gynecol. 202, 614.e1–614.e8. doi: 10.1016/j.ajog.2010.01.028
Goldberg, R., Meirovitz, A., Hirshoren, N., Bulvik, R., Binder, A., Rubinstein, A. M., et al. (2013). Versatile role of heparanase in inflammation. Matrix Biol. 32, 234–240. doi: 10.1016/j.matbio.2013.02.008
Gordon, A. S., Panahian-Jand, M., McComb, F., Melegari, C., and Sharp, S. (2003). Characteristics of women with vulvar pain disorders: responses to a Web-based survey. J. Sex Marital Ther. 29, 45–58. doi: 10.1080/713847126
Green, J. G., McLaughlin, K. A., Berglund, P. A., Gruber, M. J., Sampson, N. A., Zaslavsky, A. M., et al. (2010). Childhood adversities and adult psychiatric disorders in the national comorbidity survey replication I: associations with first onset of DSM-IV disorders. Arch. Gen. Psychiatry 67, 113–123. doi: 10.1001/archgenpsychiatry.2009.186
Grunau, R. V., Whitfield, M. F., Petrie, J. H., and Fryer, E. L. (1994). Early pain experience, child and family factors, as precursors of somatization: a prospective study of extremely premature and fullterm children. Pain 56, 353–359. doi: 10.1016/0304-3959(94)90174-0
Gunnar, M. R., and Quevedo, K. M. (2008). Early care experiences and HPA axis regulation in children: a mechanism for later trauma vulnerability. Prog. Brain Res. 167, 137–149. doi: 10.1016/s0079-6123(07)67010-1
Hanna-Mitchell, A. T., Wolf-Johnston, A., Roppolo, J. R., Buffington, T. C., and Birder, L. A. (2014). Corticotropin-releasing factor family peptide signaling in feline bladder urothelial cells. J. Endocrinol. 222, 113–121. doi: 10.1530/joe-13-0422
Hanno, P. M., Burks, D. A., Clemens, J. Q., Dmochowski, R. R., Erickson, D., Fitzgerald, M. P., et al. (2011). AUA guideline for the diagnosis and treatment of interstitial cystitis/bladder pain syndrome. J. Urol. 185, 2162–2170. doi: 10.1016/j.juro.2011.03.064
Harlow, B. L., and Stewart, E. G. (2005). Adult-onset vulvodynia in relation to childhood violence victimization. Am. J. Epidemiol. 161, 871–880. doi: 10.1093/aje/kwi108
Heim, C., Ehlert, U., Hanker, J. P., and Hellhammer, D. H. (1998). Abuse-related posttraumatic stress disorder and alterations of the hypothalamic-pituitary-adrenal axis in women with chronic pelvic pain. Psychosom. Med. 60, 309–318. doi: 10.1097/00006842-199805000-00017
Heim, C., and Nemeroff, C. B. (2002). Neurobiology of early life stress: clinical studies. Semin. Clin. Neuropsychiatry 7, 147–159. doi: 10.1053/scnp.2002.33127
Heim, C., Newport, D. J., Bonsall, R., Miller, A. H., and Nemeroff, C. B. (2001). Altered pituitary-adrenal axis responses to provocative challenge tests in adult survivors of childhood abuse. Am. J. Psychiatry 158, 575–581. doi: 10.1176/appi.ajp.158.4.575
Heim, C., Newport, D. J., Wagner, D., Wilcox, M. M., Miller, A. H., and Nemeroff, C. B. (2002). The role of early adverse experience and adulthood stress in the prediction of neuroendocrine stress reactivity in women: a multiple regression analysis. Depress. Anxiety 15, 117–125. doi: 10.1002/da.10015
Herman, J. P. (1993). Regulation of adrenocorticosteroid receptor mRNA expression in the central nervous system. Cell. Mol. Neurobiol. 13, 349–372. doi: 10.1007/bf00711577
Herman, J. P., McKlveen, J. M., Solomon, M. B., Carvalho-Netto, E., and Myers, B. (2012). Neural regulation of the stress response: glucocorticoid feedback mechanisms. Braz. J. Med. Biol. Res. 45, 292–298. doi: 10.1590/s0100-879x2012007500041
Herman, J. P., Ostrander, M. M., Mueller, N. K., and Figueiredo, H. (2005). Limbic system mechanisms of stress regulation: hypothalamo-pituitary-adrenocortical axis. Prog. Neuropsychopharmacol. Biol. Psychiatry 29, 1201–1213. doi: 10.1016/j.pnpbp.2005.08.006
Hillhouse, E. W., and Grammatopoulos, D. K. (2006). The molecular mechanisms underlying the regulation of the biological activity of corticotropin-releasing hormone receptors: implications for physiology and pathophysiology. Endocr. Rev. 27, 260–286. doi: 10.1210/er.2005-0034
Hu, J. C., Link, C. L., McNaughton-Collins, M., Barry, M. J., and McKinlay, J. B. (2007). The association of abuse and symptoms suggestive of chronic prostatitis/chronic pelvic pain syndrome: results from the Boston Area Community Health survey. J. Gen. Intern. Med. 22, 1532–1537. doi: 10.1007/s11606-007-0341-y
Huang, M., Kempuraj, D., Papadopoulou, N., Kourelis, T., Donelan, J., Manola, A., et al. (2009). Urocortin induces interleukin-6 release from rat cardiomyocytes through p38 MAP kinase, ERK and NF-κB activation. J. Mol. Endocrinol. 42, 397–405. doi: 10.1677/jme-08-0120
Hubbard, C. S., Labus, J. S., Bueller, J., Stains, J., Suyenobu, B., Dukes, G. E., et al. (2011). Corticotropin-releasing factor receptor 1 antagonist alters regional activation and effective connectivity in an emotional-arousal circuit during expectation of abdominal pain. J. Neurosci. 31, 12491–12500. doi: 10.1523/jneurosci.1860-11.2011
Imaki, T., Nahan, J. L., Rivier, C., Sawchenko, P. E., and Vale, W. (1991). Differential regulation of corticotropin-releasing factor mRNA in rat brain regions by glucocorticoids and stress. J. Neurosci. 11, 585–599.
Jacobs, B. L., Smaldone, M. C., Tyagi, V., Philips, B. J., Jackman, S. V., Leng, W. W., et al. (2010). Increased nerve growth factor in neurogenic overactive bladder and interstitial cystitis patients. Can. J. Urol. 17, 4989–4994.
Jeremias, J., Ledger, W. J., and Witkin, S. S. (2000). Interleukin 1 receptor antagonist gene polymorphism in women with vulvar vestibulitis. Am. J. Obstet. Gynecol. 182, 283–285. doi: 10.1016/s0002-9378(00)70212-2
Jiang, Y. H., Peng, C. H., Liu, H. T., and Kuo, H. C. (2013). Increased pro-inflammatory cytokines, C-reactive protein and nerve growth factor expressions in serum of patients with interstitial cystitis/bladder pain syndrome. PLoS One 8:e76779. doi: 10.1371/journal.pone.0076779
Jones, C. A., and Nyberg, L. (1997). Epidemiology of interstitial cystitis. Urology 49, 2–9. doi: 10.1016/s0090-4295(99)80327-6
Kageyama, K., and Suda, T. (2009). Regulatory mechanisms underlying corticotropin-releasing factor gene expression in the hypothalamus. Endocr. J. 56, 335–344. doi: 10.1507/endocrj.k09e-075
Kastrup, J., Hald, T., Larsen, S., and Nielsen, V. G. (1983). Histamine content and mast cell count of detrusor muscle in patients with interstitial cystitis and other types of chronic cystitis. Br. J. Urol. 55, 495–500. doi: 10.1111/j.1464-410x.1983.tb03356.x
Kiddoo, D. A., Valentino, R. J., Zderic, S., Ganesh, A., Leiser, S. C., Hale, L., et al. (2006). Impact of state of arousal and stress neuropeptides on urodynamic function in freely moving rats. Am. J. Physiol. Regul. Integr. Comp. Physiol. 290, R1697–R1706. doi: 10.1152/ajpregu.00742.2005
Klausner, A. P., and Steers, W. D. (2004). Corticotropin releasing factor: a mediator of emotional influences on bladder function. J. Urol. 172, 2570–2573. doi: 10.1097/01.ju.0000144142.26242.f3
Klausner, A. P., Streng, T., Na, Y. G., Raju, J., Batts, T. W., Tuttle, J. B., et al. (2005). The role of corticotropin releasing factor and its antagonist, astressin, on micturition in the rat. Auton. Neurosci. 123, 26–35. doi: 10.1016/j.autneu.2005.08.003
Kohara, K., Kitamura, A., Morishima, M., and Tsumoto, T. (2001). Activity-dependent transfer of brain-derived neurotrophic factor to postsynaptic neurons. Science 291, 2419–2423. doi: 10.1126/science.1057415
Kozicz, T. (2007). On the role of urocortin 1 in the non-preganglionic Edinger-Westphal nucleus in stress adaptation. Gen. Comp. Endocrinol. 153, 235–240. doi: 10.1016/j.ygcen.2007.04.005
Kutch, J. J., Ichesco, E., Hampson, J. P., Labus, J. S., Farmer, M. A., Martucci, K. T., et al. (2017). Brain signature and functional impact of centralized pain: a multidisciplinary approach to the study of chronic pelvic pain (MAPP) network study. Pain 158, 1979–1991. doi: 10.1097/j.pain.0000000000001001
Ladd, C. O., Huot, R. L., Thrivikraman, K. V., Nemeroff, C. B., and Plotsky, P. M. (2004). Long-term adaptations in glucocorticoid receptor and mineralocorticoid receptor mRNA and negative feedback on the hypothalamo-pituitary-adrenal axis following neonatal maternal separation. Biol. Psychiatry 55, 367–375. doi: 10.1016/j.biopsych.2003.10.007
Lai, H. H., Jemielita, T., Sutcliffe, S., Bradley, C. S., Naliboff, B., Williams, D. A., et al. (2017). Characterization of whole body pain in urological Chronic Pelvic Pain Syndrome at baseline: a MAPP research network study. J. Urol. 198, 622–631. doi: 10.1016/j.juro.2017.03.132
Larsen, M. S., Mortensen, S., Nordling, J., and Horn, T. (2008). Quantifying mast cells in bladder pain syndrome by immunohistochemical analysis. BJU Int. 102, 204–207; discussion 207. doi: 10.1111/j.1464-410x.2008.07576.x
Laukens, D., Brinkman, B. M., Raes, J., De Vos, M., and Vandenabeele, P. (2016). Heterogeneity of the gut microbiome in mice: guidelines for optimizing experimental design. FEMS Microbiol. Rev. 40, 117–132. doi: 10.1093/femsre/fuv036
Leclair, C. M., Goetsch, M. F., Li, H., and Morgan, T. K. (2013). Histopathologic characteristics of menopausal vestibulodynia. Obstet. Gynecol. 122, 787–793. doi: 10.1097/aog.0b013e3182a5f25f
Lennon, E. M., Maharshak, N., Elloumi, H., Borst, L., Plevy, S. E., and Moeser, A. J. (2013). Early life stress triggers persistent colonic barrier dysfunction and exacerbates colitis in adult IL-10−/− mice. Inflamm. Bowel Dis. 19, 712–719. doi: 10.1097/mib.0b013e3182802a4e
Lin, C., and Al-Chaer, E. D. (2003). Long-term sensitization of primary afferents in adult rats exposed to neonatal colon pain. Brain Res. 971, 973–982. doi: 10.1016/s0006-8993(03)02358-8
Loeser, J. D., Arendt-Nielsen, L., Baron, R., Basbaum, A., Bond, M., Breivik, H., et al. (1994). “Part III: pain terms, a current list with definitions and notes on usage,” in Classification of Chronic Pain, 2nd Edn. eds H. Merskey and N. Bogduk (Seattle, WA: IASP Press), 209–214.
Lotz, M., Villiger, P., Hugli, T., Koziol, J., and Zuraw, B. L. (1994). Interleukin-6 and interstitial cystitis. J. Urol. 152, 869–873. doi: 10.1016/S0022-5347(17)32594-6
Lupien, S. J., McEwen, B. S., Gunnar, M. R., and Heim, C. (2009). Effects of stress throughout the lifespan on the brain, behaviour and cognition. Nat. Rev. Neurosci. 10, 434–445. doi: 10.1038/nrn2639
Makino, S., Gold, P. W., and Schulkin, J. (1994). Corticosterone effects on corticotropin-releasing hormone mRNA in the central nucleus of the amygdala and the parvocellular region of the paraventricular nucleus of the hypothalamus. Brain Res. 640, 105–112. doi: 10.1016/0006-8993(94)91862-7
Makino, S., Shibasaki, T., Yamauchi, N., Nishioka, T., Mimoto, T., Wakabayashi, I., et al. (1999). Psychological stress increased corticotropin-releasing hormone mRNA and content in the central nucleus of the amygdala but not in the hypothalamic paraventricular nucleus in the rat. Brain Res. 850, 136–143. doi: 10.1016/s0006-8993(99)02114-9
Maniam, J., Antoniadis, C., and Morris, M. J. (2014). Early-life stress, HPA axis adaptation, and mechanisms contributing to later health outcomes. Front. Endocrinol. 5:73. doi: 10.3389/fendo.2014.00073
Marson, L. (1995). Central nervous system neurons identified after injection of pseudorabies virus into the rat clitoris. Neurosci. Lett. 190, 41–44. doi: 10.1016/0304-3940(95)11495-i
Marson, L., and McKenna, K. E. (1996). CNS cell groups involved in the control of the ischiocavernosus and bulbospongiosus muscles: a transneuronal tracing study using pseudorabies virus. J. Comp. Neurol. 374, 161–179. doi: 10.1002/(SICI)1096-9861(19961014)374:2<161::AID-CNE1>3.0.CO;2-0
Martin, J. A., Hamilton, B. E., Osterman, M. J., Driscoll, A. K., and Mathews, T. J. (2017). Births: final data for 2015. Natl. Vital Stat. Rep. 66:1.
Martinov, T., Glenn-Finer, R., Burley, S., Tonc, E., Balsells, E., Ashbaugh, A., et al. (2013). Contact hypersensitivity to oxazolone provokes vulvar mechanical hyperalgesia in mice. PLoS One 8:e78673. doi: 10.1371/journal.pone.0078673
Mayer, E. A., Naliboff, B. D., Chang, L., and Coutinho, S. V. (2001). V. Stress and irritable bowel syndrome. Am. J. Physiol. Gastrointest. Liver Physiol. 280, G519–G524. doi: 10.1152/ajpgi.2001.280.4.G519
Mayson, B. E., and Teichman, J. M. (2009). The relationship between sexual abuse and interstitial cystitis/painful bladder syndrome. Curr. Urol. Rep. 10, 441–447. doi: 10.1007/s11934-009-0070-3
McIntosh, J., Anisman, H., and Merali, Z. (1999). Short- and long-periods of neonatal maternal separation differentially affect anxiety and feeding in adult rats: gender-dependent effects. Dev. Brain Res. 113, 97–106. doi: 10.1016/s0165-3806(99)00005-x
McNaughton Collins, M., Pontari, M. A., O’Leary, M. P., Calhoun, E. A., Santanna, J., Landis, J. R., et al. (2001). Quality of life is impaired in men with chronic prostatitis: the chronic prostatitis collaborative research network. J. Gen. Intern. Med. 16, 656–662. doi: 10.1111/j.1525-1497.2001.01223.x
Miller, L. J., Fischer, K. A., Goralnick, S. J., Litt, M., Burleson, J. A., Albertsen, P., et al. (2002). Nerve growth factor and chronic prostatitis/chronic pelvic pain syndrome. Urology 59, 603–608. doi: 10.1016/s0090-4295(01)01597-7
Millstein, R. A., and Holmes, A. (2007). Effects of repeated maternal separation on anxiety- and depression-related phenotypes in different mouse strains. Neurosci. Biobehav. Rev. 31, 3–17. doi: 10.1016/j.neubiorev.2006.05.003
Miranda, A., Mickle, A., Schmidt, J., Zhang, Z., Shaker, R., Banerjee, B., et al. (2011). Neonatal cystitis-induced colonic hypersensitivity in adult rats: a model of viscero-visceral convergence. Neurogastroenterol. Motil. 23:683–e281. doi: 10.1111/j.1365-2982.2011.01724.x
Moloney, R. D., Johnson, A. C., O’Mahony, S. M., Dinan, T. G., Greenwood-Van Meerveld, B., and Cryan, J. F. (2016). Stress and the microbiota-gut-brain axis in visceral pain: relevance to irritable bowel syndrome. CNS Neurosci. Ther. 22, 102–117. doi: 10.1111/cns.12490
Moloney, R. D., O’Leary, O. F., Felice, D., Bettler, B., Dinan, T. G., and Cryan, J. F. (2012). Early-life stress induces visceral hypersensitivity in mice. Neurosci. Lett. 512, 99–102. doi: 10.1016/j.neulet.2012.01.066
Moyal-Barracco, M., and Lynch, P. J. (2004). 2003 ISSVD terminology and classification of vulvodynia: a historical perspective. J. Reprod. Med. 49, 772–777.
Murphy, A. B., Macejko, A., Taylor, A., and Nadler, R. B. (2009). Chronic prostatitis: management strategies. Drugs 69, 71–84. doi: 10.2165/00003495-200969010-00005
Naliboff, B. D., Stephens, A. J., Afari, N., Lai, H., Krieger, J. N., Hong, B., et al. (2015). Widespread psychosocial difficulties in men and women with urologic chronic pelvic pain syndromes: case-control findings from the multidisciplinary approach to the study of chronic pelvic pain research network. Urology 85, 1319–1327. doi: 10.1016/j.urology.2015.02.047
Nickel, J. C., Tripp, D. A., Chuai, S., Litwin, M. S., McNaughton-Collins, M., Landis, J. R., et al. (2008). Psychosocial variables affect the quality of life of men diagnosed with chronic prostatitis/chronic pelvic pain syndrome. BJU Int. 101, 59–64. doi: 10.1111/j.1464-410x.2007.07196.x
Nickel, J. C., Tripp, D. A., Pontari, M., Moldwin, R., Mayer, R., Carr, L. K., et al. (2010). Psychosocial phenotyping in women with interstitial cystitis/painful bladder syndrome: a case control study. J. Urol. 183, 167–172. doi: 10.1016/j.juro.2009.08.133
Nickel, J. C., Tripp, D. A., Pontari, M., Moldwin, R., Mayer, R., Carr, L. K., et al. (2011). Childhood sexual trauma in women with interstitial cystitis/bladder pain syndrome: a case control study. Can. Urol. Assoc. J. 5, 410–415. doi: 10.5489/cuaj.11110
Nicol, A. L., Sieberg, C. B., Clauw, D. J., Hassett, A. L., Moser, S. E., and Brummett, C. M. (2016). The association between a history of lifetime traumatic events and pain severity, physical function, and affective distress in patients with chronic pain. J. Pain 17, 1334–1348. doi: 10.1016/j.jpain.2016.09.003
Novembri, R., Torricelli, M., Bloise, E., Conti, N., Galeazzi, L. R., Severi, F. M., et al. (2011). Effects of urocortin 2 and urocortin 3 on IL-10 and TNF-α expression and secretion from human trophoblast explants. Placenta 32, 969–974. doi: 10.1016/j.placenta.2011.09.013
Numakawa, T., Kumamaru, E., Adachi, N., Yagasaki, Y., Izumi, A., and Kunugi, H. (2009). Glucocorticoid receptor interaction with TrkB promotes BDNF-triggered PLC-γ signaling for glutamate release via a glutamate transporter. Proc. Natl. Acad. Sci. U S A 106, 647–652. doi: 10.1073/pnas.0800888106
Okragly, A. J., Niles, A. L., Saban, R., Schmidt, D., Hoffman, R. L., Warner, T. F., et al. (1999). Elevated tryptase, nerve growth factor, neurotrophin-3 and glial cell line-derived neurotrophic factor levels in the urine of interstitial cystitis and bladder cancer patients. J. Urol. 161, 438–441; discussion 441–432. doi: 10.1097/00005392-199902000-00021
O’Mahony, S. M., Marchesi, J. R., Scully, P., Codling, C., Ceolho, A. M., Quigley, E. M., et al. (2009). Early life stress alters behavior, immunity, and microbiota in rats: implications for irritable bowel syndrome and psychiatric illnesses. Biol. Psychiatry 65, 263–267. doi: 10.1016/j.biopsych.2008.06.026
O’Malley, D., Dinan, T. G., and Cryan, J. F. (2010). Alterations in colonic corticotropin-releasing factor receptors in the maternally separated rat model of irritable bowel syndrome: differential effects of acute psychological and physical stressors. Peptides 31, 662–670. doi: 10.1016/j.peptides.2010.01.004
O’Malley, D., Dinan, T. G., and Cryan, J. F. (2011a). Neonatal maternal separation in the rat impacts on the stress responsivity of central corticotropin-releasing factor receptors in adulthood. Psychopharmacology 214, 221–229. doi: 10.1007/s00213-010-1885-9
O’Malley, D., Liston, M., Hyland, N. P., Dinan, T. G., and Cryan, J. F. (2011b). Colonic soluble mediators from the maternal separation model of irritable bowel syndrome activate submucosal neurons via an interleukin-6-dependent mechanism. Am. J. Physiol. Gastrointest. Liver Physiol. 300, G241–G252. doi: 10.1152/ajpgi.00385.2010
Overman, E. L., Rivier, J. E., and Moeser, A. J. (2012). CRF induces intestinal epithelial barrier injury via the release of mast cell proteases and TNF-α. PLoS One 7:e39935. doi: 10.1371/journal.pone.0039935
Pang, X., Marchand, J., Sant, G. R., Kream, R. M., and Theoharides, T. C. (1995). Increased number of substance P positive nerve fibres in interstitial cystitis. Br. J. Urol. 75, 744–750. doi: 10.1111/j.1464-410x.1995.tb07384.x
Pavcovich, L. A., and Valentino, R. J. (1995). Central regulation of micturition in the rat the corticotropin-releasing hormone from Barrington’s nucleus. Neurosci. Lett. 196, 185–188. doi: 10.1016/0304-3940(95)11873-u
Pavcovich, L. A., Yang, M., Miselis, R. R., and Valentino, R. J. (1998). Novel role for the pontine micturition center, Barrington’s nucleus: evidence for coordination of colonic and forebrain activity. Brain Res. 784, 355–361. doi: 10.1016/s0006-8993(97)01178-5
Peeker, R., Enerbäck, L., Fall, M., and Aldenborg, F. (2000). Recruitment, distribution and phenotypes of mast cells in interstitial cystitis. J. Urol. 163, 1009–1015. doi: 10.1097/00005392-200003000-00088
Perry, B. D., and Pollard, R. (1998). Homeostasis, stress, trauma, and adaptation. A neurodevelopmental view of childhood trauma. Child Adolesc. Psychiatr. Clin. N Am. 7, 33–51, viii.
Peters, K. M., Carrico, D. J., and Diokno, A. C. (2008). Characterization of a clinical cohort of 87 women with interstitial cystitis/painful bladder syndrome. Urology 71, 634–640. doi: 10.1016/j.urology.2007.11.013
Pierce, A. N., and Christianson, J. A. (2015). Stress and chronic pelvic pain. Prog. Mol. Biol. Transl. Sci. 131, 509–535. doi: 10.1016/bs.pmbts.2014.11.009
Pierce, A. N., Di Silvestro, E. R., Eller, O. C., Wang, R., Ryals, J. M., and Christianson, J. A. (2016). Urinary bladder hypersensitivity and dysfunction in female mice following early life and adult stress. Brain Res. 1639, 58–73. doi: 10.1016/j.brainres.2016.02.039
Pierce, A. N., Ryals, J. M., Wang, R., and Christianson, J. A. (2014). Vaginal hypersensitivity and hypothalamic-pituitary-adrenal axis dysfunction as a result of neonatal maternal separation in female mice. Neuroscience 263, 216–230. doi: 10.1016/j.neuroscience.2014.01.022
Pierce, A. N., Zhang, Z., Fuentes, I. M., Wang, R., Ryals, J. M., and Christianson, J. A. (2015). Neonatal vaginal irritation results in long-term visceral and somatic hypersensitivity and increased hypothalamic-pituitary-adrenal axis output in female mice. Pain 156, 2021–2031. doi: 10.1097/j.pain.0000000000000264
Plante, A. F., and Kamm, M. A. (2008). Life events in patients with vulvodynia. BJOG 115, 509–514. doi: 10.1111/j.1471-0528.2007.01662.x
Plotsky, P. M., Thrivikraman, K. V., Nemeroff, C. B., Caldji, C., Sharma, S., and Meaney, M. J. (2005). Long-term consequences of neonatal rearing on central corticotropin-releasing factor systems in adult male rat offspring. Neuropsychopharmacology 30, 2192–2204. doi: 10.1038/sj.npp.1300769
Pontari, M. A., and Ruggieri, M. R. (2004). Mechanisms in prostatitis/chronic pelvic pain syndrome. J. Urol. 172, 839–845. doi: 10.1097/01.ju.0000136002.76898.04
Potts, J. M., and Payne, C. K. (2012). Urologic chronic pelvic pain. Pain 153, 755–758. doi: 10.1016/j.pain.2011.10.005
Radley, J. J., and Sawchenko, P. E. (2011). A common substrate for prefrontal and hippocampal inhibition of the neuroendocrine stress response. J. Neurosci. 31, 9683–9695. doi: 10.1523/JNEUROSCI.6040-10.2011
Randich, A., Uzzell, T., DeBerry, J. J., and Ness, T. J. (2006). Neonatal urinary bladder inflammation produces adult bladder hypersensitivity. J. Pain 7, 469–479. doi: 10.1016/j.jpain.2006.01.450
Rao, U., Hammen, C., Ortiz, L. R., Chen, L. A., and Poland, R. E. (2008). Effects of early and recent adverse experiences on adrenal response to psychosocial stress in depressed adolescents. Biol. Psychiatry 64, 521–526. doi: 10.1016/j.biopsych.2008.05.012
Ren, S., Sakai, K., and Schwartz, L. B. (1998). Regulation of human mast cell β-tryptase: conversion of inactive monomer to active tetramer at acid pH. J. Immunol. 160, 4561–4569.
Ren, T. H., Wu, J., Yew, D., Ziea, E., Lao, L., Leung, W. K., et al. (2007). Effects of neonatal maternal separation on neurochemical and sensory response to colonic distension in a rat model of irritable bowel syndrome. Am. J. Physiol. Gastrointest. Liver Physiol. 292, G849–G856. doi: 10.1152/ajpgi.00400.2006
Reul, J. M., and de Kloet, E. R. (1985). Two receptor systems for corticosterone in rat brain: microdistribution and differential occupation. Endocrinology 117, 2505–2511. doi: 10.1210/endo-117-6-2505
Reyes, T. M., Lewis, K., Perrin, M. H., Kunitake, K. S., Vaughan, J., Arias, C. A., et al. (2001). Urocortin II: a member of the corticotropin-releasing factor (CRF) neuropeptide family that is selectively bound by type 2 CRF receptors. Proc. Natl. Acad. Sci. U S A 98, 2843–2848. doi: 10.1073/pnas.051626398
Reyes, B. A., Valentino, R. J., and Van Bockstaele, E. J. (2008). Stress-induced intracellular trafficking of corticotropin-releasing factor receptors in rat locus coeruleus neurons. Endocrinology 149, 122–130. doi: 10.1210/en.2007-0705
Riegel, B., Bruenahl, C. A., Ahyai, S., Bingel, U., Fisch, M., and Löwe, B. (2014). Assessing psychological factors, social aspects and psychiatric co-morbidity associated with Chronic Prostatitis/Chronic Pelvic Pain Syndrome (CP/CPPS) in men—a systematic review. J. Psychosom. Res. 77, 333–350. doi: 10.1016/j.jpsychores.2014.09.012
Rincón-Cortés, M., and Sullivan, R. M. (2014). Early life trauma and attachment: immediate and enduring effects on neurobehavioral and stress axis development. Front. Endocrinol. 5:33. doi: 10.3389/fendo.2014.00033
Robbins, M. T., and Ness, T. J. (2008). Footshock-induced urinary bladder hypersensitivity: role of spinal corticotropin-releasing factor receptors. J. Pain 9, 991–998. doi: 10.1016/j.jpain.2008.05.006
Roman, K., Done, J. D., Schaeffer, A. J., Murphy, S. F., and Thumbikat, P. (2014). Tryptase-PAR2 axis in experimental autoimmune prostatitis, a model for chronic pelvic pain syndrome. Pain 155, 1328–1338. doi: 10.1016/j.pain.2014.04.009
Romeo, R. D., Mueller, A., Sisti, H. M., Ogawa, S., McEwen, B. S., and Brake, W. G. (2003). Anxiety and fear behaviors in adult male and female C57BL/6 mice are modulated by maternal separation. Horm. Behav. 43, 561–567. doi: 10.1016/s0018-506x(03)00063-1
Rouzade-Dominguez, M. L., Miselis, R., and Valentino, R. J. (2003a). Central representation of bladder and colon revealed by dual transsynaptic tracing in the rat: substrates for pelvic visceral coordination. Eur. J. Neurosci. 18, 3311–3324. doi: 10.1111/j.1460-9568.2003.03071.x
Rouzade-Dominguez, M. L., Pernar, L., Beck, S., and Valentino, R. J. (2003b). Convergent responses of Barrington’s nucleus neurons to pelvic visceral stimuli in the rat: a juxtacellular labelling study. Eur. J. Neurosci. 18, 3325–3334. doi: 10.1111/j.1460-9568.2003.03072.x
Sakanaka, M., Shibasaki, T., and Lederis, K. (1987). Corticotropin releasing factor-like immunoreactivity in the rat brain as revealed by a modified cobalt-glucose oxidase-diaminobenzidine method. J. Comp. Neurol. 260, 256–298. doi: 10.1002/cne.902600209
Sasaki, M. (2005). Role of Barrington’s nucleus in micturition. J. Comp. Neurol. 493, 21–26. doi: 10.1002/cne.20719
Schwetz, I., McRoberts, J. A., Coutinho, S. V., Bradesi, S., Gale, G., Fanselow, M., et al. (2005). Corticotropin-releasing factor receptor 1 mediates acute and delayed stress-induced visceral hyperalgesia in maternally separated Long-Evans rats. Am. J. Physiol. Gastrointest. Liver Physiol. 289, G704–G712. doi: 10.1152/ajpgi.00498.2004
Sengupta, J. N. (2009). Visceral pain: the neurophysiological mechanism. Handb. Exp. Pharmacol. 194, 31–74. doi: 10.1007/978-3-540-79090-7_2
Shoskes, D. A., Nickel, J. C., Dolinga, R., and Prots, D. (2009). Clinical phenotyping of patients with chronic prostatitis/chronic pelvic pain syndrome and correlation with symptom severity. Urology 73, 538–542; discussion 542–533. doi: 10.1016/j.urology.2008.09.074
Simons, S. H., van Dijk, M., Anand, K. S., Roofthooft, D., van Lingen, R. A., and Tibboel, D. (2003). Do we still hurt newborn babies? A prospective study of procedural pain and analgesia in neonates. Arch. Pediatr. Adolesc. Med. 157, 1058–1064. doi: 10.1001/archpedi.157.11.1058
Smith, G. W., Aubry, J. M., Dellu, F., Contarino, A., Bilezikjian, L. M., Gold, L. H., et al. (1998). Corticotropin releasing factor receptor 1-deficient mice display decreased anxiety, impaired stress response, and aberrant neuroendocrine development. Neuron 20, 1093–1102. doi: 10.1016/s0896-6273(00)80491-2
Smith, C., Nordstrom, E., Sengupta, J. N., and Miranda, A. (2007). Neonatal gastric suctioning results in chronic visceral and somatic hyperalgesia: role of corticotropin releasing factor. Neurogastroenterol. Motil. 19, 692–699. doi: 10.1111/j.1365-2982.2007.00949.x
Spanos, C., Pang, X., Ligris, K., Letourneau, R., Alferes, L., Alexacos, N., et al. (1997). Stress-induced bladder mast cell activation: implications for interstitial cystitis. J. Urol. 157, 669–672. doi: 10.1097/00005392-199702000-00085
Stasi, C., Rosselli, M., Bellini, M., Laffi, G., and Milani, S. (2012). Altered neuro-endocrine-immune pathways in the irritable bowel syndrome: the top-down and the bottom-up model. J. Gastroenterol. 47, 1177–1185. doi: 10.1007/s00535-012-0627-7
Strauss, A. C., and Dimitrakov, J. D. (2010). New treatments for chronic prostatitis/chronic pelvic pain syndrome. Nat. Rev. Urol. 7, 127–135. doi: 10.1038/nrurol.2010.4
Suskind, A. M., Berry, S. H., Suttorp, M. J., Elliott, M. N., Hays, R. D., Ewing, B. A., et al. (2013). Health-related quality of life in patients with interstitial cystitis/bladder pain syndrome and frequently associated comorbidities. Qual. Life Res. 22, 1537–1541. doi: 10.1007/s11136-012-0285-5
Sweetser, S., Camilleri, M., Linker Nord, S. J., Burton, D. D., Castenada, L., Croop, R., et al. (2009). Do corticotropin releasing factor-1 receptors influence colonic transit and bowel function in women with irritable bowel syndrome? Am. J. Physiol. Gastrointest. Liver Physiol. 296, G1299–G1306. doi: 10.1152/ajpgi.00011.2009
Taché, Y., Martinez, V., Million, M., and Wang, L. (2001). Stress and the gastrointestinal tract III. Stress-related alterations of gut motor function: role of brain corticotropin-releasing factor receptors. Am. J. Physiol. Gastrointest. Liver Physiol. 280, G173–G177. doi: 10.1152/ajpgi.2001.280.2.g173
Taché, Y., Million, M., Nelson, A. G., Lamy, C., and Wang, L. (2005). Role of corticotropin-releasing factor pathways in stress-related alterations of colonic motor function and viscerosensibility in female rodents. Gend. Med. 2, 146–154. doi: 10.1016/s1550-8579(05)80043-9
Tasker, J. G., and Herman, J. P. (2011). Mechanisms of rapid glucocorticoid feedback inhibition of the hypothalamic-pituitary-adrenal axis. Stress 14, 398–406. doi: 10.3109/10253890.2011.586446
Teicher, M. H., Andersen, S. L., Polcari, A., Anderson, C. M., and Navalta, C. P. (2002). Developmental neurobiology of childhood stress and trauma. Psychiatr. Clin. North Am. 25, 397–426, vii–viii. doi: 10.1016/s0193-953x(01)00003-x
Teicher, M. H., Andersen, S. L., Polcari, A., Anderson, C. M., Navalta, C. P., and Kim, D. M. (2003). The neurobiological consequences of early stress and childhood maltreatment. Neurosci. Biobehav. Rev. 27, 33–44. doi: 10.1016/s0149-7634(03)00007-1
Theoharides, T. C., Donelan, J. M., Papadopoulou, N., Cao, J., Kempuraj, D., and Conti, P. (2004). Mast cells as targets of corticotropin-releasing factor and related peptides. Trends Pharmacol. Sci. 25, 563–568. doi: 10.1016/j.tips.2004.09.007
Theoharides, T. C., Flaris, N., Cronin, C. T., Ucci, A., and Meares, E. (1990). Mast cell activation in sterile bladder and prostate inflammation. Int. Arch. Allergy Appl. Immunol. 92, 281–286. doi: 10.1159/000235190
Tomaszewski, J. E., Landis, J. R., Russack, V., Williams, T. M., Wang, L. P., Hardy, C., et al. (2001). Biopsy features are associated with primary symptoms in interstitial cystitis: results from the interstitial cystitis database study. Urology 57, 67–81. doi: 10.1016/s0090-4295(01)01166-9
Tore, F., Reynier-Rebuffel, A. M., Tuncel, N., Callebert, J., and Aubineau, P. (2001). Effects of sepsis on mast cells in rat dura mater: influence of L-NAME and VIP. Br. J. Pharmacol. 134, 1367–1374. doi: 10.1038/sj.bjp.0704412
Tripp, D. A., Nickel, J. C., Wang, Y., Litwin, M. S., McNaughton-Collins, M., Landis, J. R., et al. (2006). Catastrophizing and pain-contingent rest predict patient adjustment in men with chronic prostatitis/chronic pelvic pain syndrome. J. Pain 7, 697–708. doi: 10.1016/j.jpain.2006.03.006
Tyrka, A. R., Wier, L., Price, L. H., Ross, N., Anderson, G. M., Wilkinson, C. W., et al. (2008). Childhood parental loss and adult hypothalamic-pituitary-adrenal function. Biol. Psychiatry 63, 1147–1154. doi: 10.1016/j.biopsych.2008.01.011
Ulrich-Lai, Y. M., and Herman, J. P. (2009). Neural regulation of endocrine and autonomic stress responses. Nat. Rev. Neurosci. 10, 397–409. doi: 10.1038/nrn2647
U.S. Department of Health and Human Services, Administration for Children and Families, and Administration on Children, Youth and Families, Children’s Bureau. (2012). Child Maltreatment, 2011. Available online at: http://www.acf.hhs.gov/programs/cb/research-data-technology/statistics-research/child-maltreatment
Valentino, R. J., Miselis, R. R., and Pavcovich, L. A. (1999). Pontine regulation of pelvic viscera: pharmacological target for pelvic visceral dysfunctions. Trends Pharmacol. Sci. 20, 253–260. doi: 10.1016/s0165-6147(99)01332-2
Valentino, R. J., Pavcovich, L. A., and Hirata, H. (1995). Evidence for corticotropin-releasing hormone projections from Barrington’s nucleus to the periaqueductal gray and dorsal motor nucleus of the vagus in the rat. J. Comp. Neurol. 363, 402–422. doi: 10.1002/cne.903630306
Valentino, R. J., Wood, S. K., Wein, A. J., and Zderic, S. A. (2011). The bladder-brain connection: putative role of corticotropin-releasing factor. Nat. Rev. Urol. 8, 19–28. doi: 10.1038/nrurol.2010.203
van den Wijngaard, R. M., Klooker, T. K., Welting, O., Stanisor, O. I., Wouters, M. M., van der Coelen, D., et al. (2009). Essential role for TRPV1 in stress-induced (mast cell-dependent) colonic hypersensitivity in maternally separated rats. Neurogastroenterol. Motil. 21, e1107–e1194. doi: 10.1111/j.1365-2982.2009.01339.x
van den Wijngaard, R. M., Stanisor, O. I., van Diest, S. A., Welting, O., Wouters, M. M., de Jonge, W. J., et al. (2012). Peripheral α-helical CRF (9–41) does not reverse stress-induced mast cell dependent visceral hypersensitivity in maternally separated rats. Neurogastroenterol. Motil. 24, 274.e111–282.e111. doi: 10.1111/j.1365-2982.2011.01840.x
van Haarst, A. D., Oitzl, M. S., and de Kloet, E. R. (1997). Facilitation of feedback inhibition through blockade of glucocorticoid receptors in the hippocampus. Neurochem. Res. 22, 1323–1328. doi: 10.1023/A:1022010904600
Van Pett, K., Viau, V., Bittencourt, J. C., Chan, R. K., Li, H. Y., Arias, C., et al. (2000). Distribution of mRNAs encoding CRF receptors in brain and pituitary of rat and mouse. J. Comp. Neurol. 428, 191–212. doi: 10.1002/1096-9861(20001211)428:2<191::aid-cne1>3.0.co;2-u
Vaughan, J., Donaldson, C., Bittencourt, J., Perrin, M. H., Lewis, K., Sutton, S., et al. (1995). Urocortin, a mammalian neuropeptide related to fish urotensin I and to corticotropin-releasing factor. Nature 378, 287–292. doi: 10.1038/378287a0
Vázquez, D. M. (1998). Stress and the developing limbic-hypothalamic-pituitary-adrenal axis. Psychoneuroendocrinology 23, 663–700. doi: 10.1016/s0306-4530(98)00029-8
Venihaki, M., Sakihara, S., Subramanian, S., Dikkes, P., Weninger, S. C., Liapakis, G., et al. (2004). Urocortin III, a brain neuropeptide of the corticotropin-releasing hormone family: modulation by stress and attenuation of some anxiety-like behaviours. J. Neuroendocrinol. 16, 411–422. doi: 10.1111/j.1365-2826.2004.01170.x
Videlock, E. J., Adeyemo, M., Licudine, A., Hirano, M., Ohning, G., Mayer, M., et al. (2009). Childhood trauma is associated with hypothalamic-pituitary-adrenal axis responsiveness in irritable bowel syndrome. Gastroenterology 137, 1954–1962. doi: 10.1053/j.gastro.2009.08.058
Vincent, S. R., and Satoh, K. (1984). Corticotropin-releasing factor (CRF) immunoreactivity in the dorsolateral pontine tegmentum: further studies on the micturition reflex system. Brain Res. 308, 387–391. doi: 10.1016/0006-8993(84)91085-0
Vykhovanets, E. V., Resnick, M. I., MacLennan, G. T., and Gupta, S. (2007). Experimental rodent models of prostatitis: limitations and potential. Prostate Cancer Prostatic Dis. 10, 15–29. doi: 10.1038/sj.pcan.4500930
Wang, J., Gu, C., and Al-Chaer, E. D. (2008). Altered behavior and digestive outcomes in adult male rats primed with minimal colon pain as neonates. Behav. Brain Funct. 4:28. doi: 10.1186/1744-9081-4-28
Warren, J. W., Clauw, D. J., Wesselmann, U., Langenberg, P. W., Howard, F. M., and Morozov, V. (2011a). Sexuality and reproductive risk factors for interstitial cystitis/painful bladder syndrome in women. Urology 77, 570–575. doi: 10.1016/j.urology.2010.10.018
Warren, J. W., van de Merwe, J. P., and Nickel, J. C. (2011b). Interstitial cystitis/bladder pain syndrome and nonbladder syndromes: facts and hypotheses. Urology 78, 727–732. doi: 10.1016/j.urology.2011.06.014
Watanabe, T., Inoue, M., Sasaki, K., Araki, M., Uehara, S., Monden, K., et al. (2011). Nerve growth factor level in the prostatic fluid of patients with chronic prostatitis/chronic pelvic pain syndrome is correlated with symptom severity and response to treatment. BJU Int. 108, 248–251. doi: 10.1111/j.1464-410X.2010.09716.x
Whitfield, M. F., and Grunau, R. E. (2000). Behavior, pain perception, and the extremely low-birth weight survivor. Clin. Perinatol. 27, 363–379. doi: 10.1016/s0095-5108(05)70026-9
Winston, J., Shenoy, M., Medley, D., Naniwadekar, A., and Pasricha, P. J. (2007). The vanilloid receptor initiates and maintains colonic hypersensitivity induced by neonatal colon irritation in rats. Gastroenterology 132, 615–627. doi: 10.1053/j.gastro.2006.11.014
Wood, S. K., Baez, M. A., Bhatnagar, S., and Valentino, R. J. (2009). Social stress-induced bladder dysfunction: potential role of corticotropin-releasing factor. Am. J. Physiol. Regul. Integr. Comp. Physiol. 296, R1671–R1678. doi: 10.1152/ajpregu.91013.2008
Yehuda, R., Yang, R. K., Buchsbaum, M. S., and Golier, J. A. (2006). Alterations in cortisol negative feedback inhibition as examined using the ACTH response to cortisol administration in PTSD. Psychoneuroendocrinology 31, 447–451. doi: 10.1016/j.psyneuen.2005.10.007
Yuan, P. Q., Wu, S. V., Elliott, J., Anton, P. A., Chatzaki, E., Million, M., et al. (2012). Expression of corticotropin releasing factor receptor type 1 (CRF1) in the human gastrointestinal tract and upregulation in the colonic mucosa in patients with ulcerative colitis. Peptides 38, 62–69. doi: 10.1016/j.peptides.2012.07.028
Yun, S. K., Laub, D. J., Weese, D. L., Lad, P. M., Leach, G. E., and Zimmern, P. E. (1992). Stimulated release of urine histamine in interstitial cystitis. J. Urol. 148, 1145–1148. doi: 10.1016/s0022-5347(17)36844-1
Zhang, X. J., Li, Z., Chung, E. K., Zhang, H. Q., Xu, H. X., Sung, J. J., et al. (2009). Activation of extracellular signal-regulated protein kinase is associated with colorectal distension-induced spinal and supraspinal neuronal response and neonatal maternal separation-induced visceral hyperalgesia in rats. J. Mol. Neurosci. 37, 274–287. doi: 10.1007/s12031-008-9134-y
Keywords: early life stress, neonatal maternal separation (NMS), visceral hypersensitivity, hypothalamic-pituitary-adrenal (HPA) axis, CRF, chronic pelvic pain
Citation: Fuentes IM and Christianson JA (2018) The Influence of Early Life Experience on Visceral Pain. Front. Syst. Neurosci. 12:2. doi: 10.3389/fnsys.2018.00002
Received: 29 September 2017; Accepted: 12 January 2018;
Published: 26 January 2018.
Edited by:
Jyoti N. Sengupta, Medical College of Wisconsin, United StatesReviewed by:
Catherine S. Hubbard, Massachusetts General Hospital, Harvard Medical School, United StatesDervla O’Malley, University College Cork, Ireland
Copyright © 2018 Fuentes and Christianson. This is an open-access article distributed under the terms of the Creative Commons Attribution License (CC BY). The use, distribution or reproduction in other forums is permitted, provided the original author(s) and the copyright owner are credited and that the original publication in this journal is cited, in accordance with accepted academic practice. No use, distribution or reproduction is permitted which does not comply with these terms.
*Correspondence: Julie A. Christianson, amNocmlzdGlhbnNvbkBrdW1jLmVkdQ==