- 1BrainImaging and NeuroStimulation (BINS) Laboratory, Department of Neurology, University Medical Center Hamburg-Eppendorf, Hamburg, Germany
- 2Department of Neurophysiology and Pathophysiology, University Medical Center Hamburg-Eppendorf, Hamburg, Germany
- 3Department of Neurology, Favoloro University, Medical School, Buenos Aires, Argentina
A large amount of studies of the last decades revealed an association between human behavior and oscillatory activity in the human brain. Alike, abnormalities of oscillatory activity were related with pathological behavior in many neuropsychiatric disorders, such as in Parkinson’s disease (PD) or in schizophrenia (SCZ). As a therapeutic tool, non-invasive brain stimulation (NIBS) has demonstrated the potential to improve behavioral performance in patients suffering from neuropsychiatric disorders. Since evidence accumulates that NIBS might be able to modulate oscillatory activity and related behavior in a scientific setting, this review focuses on discussing potential interventional strategies to target abnormalities in oscillatory activity in neuropsychiatric disorders. In particular, we will review oscillatory changes described in patients after stroke, with PD or suffering from SCZ. Potential ways of targeting interventionally the underlying pathological oscillations to improve related pathological behavior will be further discussed.
Importance of Oscillations for Information Processing in the Healthy Brain
Since Berger first described oscillatory activity of the brain in the 1920s, oscillations have been investigated extensively and research revealed clear relevance of human brain oscillations for information processing and behavior (Singer and Gray, 1995; Engel et al., 2001; Brown, 2003; Buzsáki and Draguhn, 2004; Pfurtscheller et al., 2005; Schoffelen et al., 2005; Fries, 2009; Jensen and Mazaheri, 2010).
Generally, an oscillation is a periodic fluctuation caused by changes in excitability of a group of neurons, measured on the scalp as differences in voltage with techniques like electroencephalography (EEG) or magnetencephalography (MEG). Oscillations are suggested to be able to temporally coordinate and control neuronal firing and are proposed to be one basic principle of information processing in the human brain (Engel et al., 2001; Varela et al., 2001; Buzsáki and Draguhn, 2004). Based on phenomenological observations, a division in frequency bands has been established. Usually, oscillation frequencies are categorized as delta-band (1.5–4 Hz), theta-band (4–7 Hz), alpha-band (8–12 Hz), beta-band (12–30 Hz) and gamma-band (30–80 Hz). Since this review will focus on specific aspects of pathological oscillatory activity, the next paragraph will briefly introduce selected relevant findings for each frequency band in the healthy brain.
Slow delta-band resting-state oscillations are prominent in sleep and are, like theta-band oscillations, associated with memory consolidation (Marshall et al., 2006; Rasch and Born, 2013). Alpha-band oscillations are prominent oscillations in the resting brain and alpha-band activity is associated with a wide range of brain functions like visual perception (Busch et al., 2009), working memory and short-term-memory retention (Palva and Palva, 2007). Alpha-band responses to eyes closing and opening respectively have been described since the first EEG-recordings and have been interpreted as desynchronization of affected neurons by information processing. This task-related suppression of alpha-band activity has been extensively investigated for other areas like motor cortex, and is called event-related desynchronization (Pfurtscheller, 1992; Klimesch et al., 2007). Besides event-related desynchronisation, there is event-related synchronization, which has been proposed to reflect cortical idling (Pfurtscheller et al., 1996). Furthermore, alpha-band activity was induced during inhibitory control processes (Hummel et al., 2002; Haegens et al., 2010; Jensen and Mazaheri, 2010; Sauseng et al., 2013). Beta-band oscillations are, like alpha-band oscillations, involved in perceptual processes (Engel and Fries, 2010). Another functional relevance of beta-band oscillations is sensorimotor control, their activity has been described during motor tasks and maintenance of contractions (Pfurtscheller and Lopes da Silva, 1999; Kilner et al., 2000; Engel and Fries, 2010). Since beta oscillations are described to be associated with a steady state of the motor system, it has been hypothesized that they signal the “status quo” (Gilbertson et al., 2005; Engel and Fries, 2010). Having been extensively investigated, task-related or evoked gamma-band oscillations respectively are thought to be involved into a broad range of behavioral components like visual perception and attention, and are associated with several memory functions (Singer and Gray, 1995; Tallon-Baudry et al., 1998; Herrmann et al., 2004; Jensen et al., 2007).
Moreover, besides local phenomena, mechanisms of oscillatory communication have been identified. For example synchronization of phases is suggested to be one basic principle for long range communication and is a component of connectivity measurements like coherence (Varela et al., 2001; Hummel and Gerloff, 2005; Schoffelen et al., 2005). Furthermore high frequency activity has been described as being depended on low frequency phase (Osipova et al., 2008; Jensen and Mazaheri, 2010; Buzsáki and Wang, 2012).
Underlining a potential causal relationship, both oscillatory activity and behavior were modulated by non-invasive brain stimulation (NIBS) in several studies. Before focussing on abnormal oscillations and pathological behavior, the influence of NIBS on oscillations of the healthy brain will be discussed in the next section.
Modulation of Oscillations by Rhythmic NIBS in the Healthy Brain
Several recent studies combining NIBS and EEG revealed that NIBS is able to modulate oscillations in the healthy brain (Plewnia et al., 2008; Pogosyan et al., 2009; Zaehle et al., 2010; Thut et al., 2011; Vernet et al., 2013). Generally, one basic technique of NIBS is Transcranial Magnetic Stimulation (TMS). By depolarization of neurons, a TMS single pulse applied over the hand knob area typically leads to a motor evoked potential in the contralateral hand (Hummel and Cohen, 2005; Hallett, 2007). Combining TMS with EEG, an increase of the intrinsic frequency of the TMS-affected area and their thalamic loops respectively has been observed (Rosanova et al., 2009). Another basic method of NIBS is Transcranial Electric Stimulation (TES). In contrast to TMS, TES is suggested to tune oscillators by modulating the membrane potential of neurons and their spontaneous firing rates (Nitsche and Paulus, 2000).
Regarding rhythmic applications of TMS, there is e.g., the theta burst stimulation (TBS) protocol, in which a triple pulse with an interpulse frequency of 50 Hz is applied in a theta rhythm. Applied continuously, TBS has an inhibitory effect, applied in an intermittent mode TBS has an excitatory effect (Huang et al., 2005; Hallett, 2007). Furthermore, repetitive TMS (rTMS) applied in a frequency below or at 1 Hz has an inhibitory effect, application of frequencies at faster rates (>5 Hz) leads to excitation (Pascual-Leone et al., 1994; Hummel and Cohen, 2005). Regarding patterned applications of TES, there is transcranial alternating current stimulation (tACS), a sinusoidal stimulation with spatially alternating anodal and cathodal components. Furthermore, there are transcranial random noise stimulation (tRNS) and direct current stimulation with a sinusoidal component, called oscillatory tDCS (Siebner and Ziemann, 2010).
Depending on their effect on oscillations, a division of rhythmic applications of NIBS into two concepts has been proposed (Siebner and Ziemann, 2010). On the one hand rhythmic NIBS can be applied in an oscillatory mode in the frequency of the targeted oscillation or its oscillator, respectively (Pogosyan et al., 2009; Zaehle et al., 2010; Thut et al., 2011). In the following, this mode will be called “direct”, because there is a direct interference of stimulation frequency and the frequency of the oscillator. On the other hand, one can apply rhythmic NIBS in a non oscillatory mode, not reflecting the targeted oscillations or the frequency of the targeted oscillator. In this case NIBS is suggested to modulate underlying oscillatory mechanisms (Nitsche and Paulus, 2000; Lapenta et al., 2013; Vernet et al., 2013). This non oscillatory mode will be called “indirect” in the following.
In a study on “direct” modulation of ongoing oscillations, five pulses of TMS with an adjusted individual alpha frequency were able to entrain a parietal alpha oscillator specifically in its natural frequency (Thut et al., 2011). tACS entrained individual parietal-central alpha oscillations if applied in the endogenous frequency at the occipital pole (Zaehle et al., 2010). Furthermore, 10 Hz repetitive bifocal TMS over left primary motor cortex and over visual cortex led to an increase of alpha-band and lower beta coherence between the stimulated sites (Plewnia et al., 2008), supporting the exciting concept to not only influence local oscillatory activity but also long-range oscillatory interactions by NIBS.
Combining NIBS with EEG, “direct” applications of NIBS also modulated both oscillatory activity and behavioral components, thereby providing evidence for a causal role of oscillations and its modulation. In a visuomotor task tACS applied at 20 Hz reduced peak velocity on the one hand and increased coherence between scalp-recorded activity and EMG activity at 20 Hz on the other hand (Pogosyan et al., 2009). Recently it has been shown by concurrent EEG-tACS that 10 Hz stimulation can entrain parieto-occipital alpha activity and modulate target detection performance in an oddball task in a phase-dependent manner (Helfrich et al., 2014). In an auditory experiment perception thresholds were dependent on the phase of the entrained oscillation using 10 Hz oscillatory tDCS (Neuling et al., 2012). Furthermore slow wave oscillatory tDCS in a frequency of 0.75 Hz applied during non-rapid-eye-movement sleep induced an increase of slow wave oscillations and enhanced the retention of declarative memories (Marshall et al., 2006). However, this study was critically evaluated by the same group showing that the total amount of current and not the oscillatory component of the oscillatory tDCS might have been the main effective variable (Groppa et al., 2010).
As an “indirect” rhythmic application of NIBS, continuous theta burst stimulation (cTBS) increased theta-band power and decreased beta-band power in an eyes-closed resting experiment (Vernet et al., 2013). As an underlying mechanism the authors suggest that cTBS modulates synchronization of relevant oscillators. In a non resting setup cTBS increased event-related lower beta power applied over primary motor cortex for at least 30 min (Noh et al., 2012) and 40 trains of ten excitatory TMS pulses at 20 Hz increased alpha and beta-band event-related synchronization at the stimulation site (Veniero et al., 2011).
Like “direct” applications, also “indirect” applications were able to modulate both oscillations and behavior. Inhibitory 1 Hz rTMS on right prefrontal cortex reduced both response times for congruent cued targets and ipsilateral alpha amplitude (Sauseng et al., 2011). In another study 5 Hz tDCS (theta tDCS) decreased both slow wave activity, frontal slow EEG spindle power and consolidation of declarative memory (Marshall et al., 2011).
Taken together evidence is increasing that oscillations, being one basic principle of information processing in the human brain, can be modulated by NIBS. In many cases, this suggests a possible mechanism for how NIBS may exert its effects on cognitive processes or behavior.
In the following paragraph, alteration of oscillatory activity will be discussed as a pathophysiological mechanism in neuropsychiatric disorders. As examples, we will consider oscillatory changes in the alpha-band after stroke, beta-band changes in patients with Parkinson’s disease (PD) and altered gamma-band activity in patients suffering from schizophrenia (SCZ). Next, we will discuss potential applications of rhythmic NIBS like rTMS and tACS in order to modulate pathological oscillations and potentially improve the clinical outcome in patients.
Role of Oscillatory Activity in the Pathophysiology of Neuropsychiatric Disorders
Stroke is one of the leading causes for acquired long-term disability in industrialized countries (Kolominsky-Rabas et al., 2001) and, therefore, studies on neurophysiological changes accompanying and following stroke have received considerable interest.
Several studies have observed changes in alpha-band activity recorded over the affected hemisphere (AH) after stroke in the resting brain (Tecchio et al., 2005, 2006; Dubovik et al., 2012; Westlake et al., 2012; Laaksonen et al., 2013). Thirty-two patients with stroke of the middle cerebral artery within the first 10 days (Tecchio et al., 2005) and 56 stroke patients in a chronic stage (Tecchio et al., 2006) showed a reduction of the individual alpha frequency in the AH. In 16 patients with affected upper limp function, amplitude of alpha-band oscillations increased in the AH compared to the unaffected hemisphere (UH) and to control subjects 1 month and 3 months after stroke (Laaksonen et al., 2013). In the latter study, alpha oscillations had a burst-like pattern and were found both in rolandic and in parietal regions. However, these changes in alpha-band activity did not correlate with the clinical outcome in patients (Tecchio et al., 2005, 2006; Laaksonen et al., 2013).
In contrast, two studies addressing interregional resting-state functional connectivity (FC, based on imaginary coherence, IC) were able to correlate changes of alpha-band activity with performance (Dubovik et al., 2012) and motor recovery (Westlake et al., 2012) after stroke. Twenty patients showed a decrease of alpha-band FC of central electrodes over the lesions to all other electrodes 3 months after stroke of middle or/and anterior cerebral artery (Dubovik et al., 2012). Moreover, motor functions correlated with IC values of the precentral gyrus and all other investigated brain regions. Since FC changes were not restricted to the boundaries of the lesions but restricted to a specific frequency, the authors emphasize that oscillatory changes are probably not due to tissue loss but to changes in affected tissue. Furthermore the study showed that changes in FC are probably due to changes in alpha phase synchrony rather than to changes in alpha amplitude (Stam et al., 2007; Dubovik et al., 2012). Another study found changes in alpha-band activity in the acute phase of stroke (Westlake et al., 2012). Greater initial functional alpha-band connectivity of ipsilesional primary sensory cortex and prefrontal cortex in the acute phase correlated with better clinical improvement 8–12 weeks after stroke in fourteen patients with motor impairment of the upper limb.
Next to changes in alpha-band activity, also changes of slow wave oscillations were found after brain injuries like head trauma and stroke (Lewine et al., 1999; Butz et al., 2004). In line with these results a shift from fast to slow rhythms could be observed after stroke (Dubovik et al., 2012) and slow delta wave oscillations of the UH have been shown to be correlated with clinical outcome after stroke (Tecchio et al., 2007). Patients with persisting abnormal slow wave oscillations (abnormal low frequency magnetic activity) had a significantly worse clinical outcome compared to patients without persisting slow wave oscillatory components (Laaksonen et al., 2013).
Besides clinical observations, slow wave activity has also been investigated in an animal stroke model, in which slow wave oscillations were associated with axonal sprouting after thermal-ischemic lesioning (Carmichael and Chesselet, 2002). In this model, a treatment with tetrodotoxin reduced both slow oscillations and axonal sprouting. In another study neuronal bursts were able to reduce inhibitory factors that surround axons (Ming et al., 2001). In a recent publication it could be shown that new patterns of axonal sprouting mediated recovery after stroke in an animal model (Overman et al., 2012). Taken together, these data provided first evidence that there might be an association of slow neuronal firing, axonal sprouting and behavioral recovery.
PD has a high prevalence in aging populations (von Campenhausen et al., 2005). Evidence is increasing that clinical symptoms of PD are related to abnormalities in beta-band activity (Levy et al., 2002; Kühn et al., 2008, 2009; Crowell et al., 2012; Heinrichs-Graham et al., 2014; Herz et al., 2014). Prominent beta-band activity has been recorded in the basal ganglia in animal models of PD and in PD patients with deep brain stimulation (DBS; Levy et al., 2000, 2002; Kühn et al., 2008; Bergmann et al., 2012; Heinrichs-Graham et al., 2014; Herz et al., 2014). Both dopamine treatment and DBS have been shown to reduce beta-band activity (Brown et al., 2001; Levy et al., 2002; Kühn et al., 2008). The modulation of beta-band activity by dopaminergic treatment (Kühn et al., 2009) and by DBS (Ray et al., 2008) was associated with a reduction of bradykinesia and rigidity. A recent study in PD also revealed that treatment with levodopa reinforced beta-band coupling between primary motor cortex and lateral premotor cortex (Herz et al., 2014). Another study demonstrated a lack of physiological event-related beta desynchronization which could be modulated by levodopa in PD (Heinrichs-Graham et al., 2014). Taken together, in PD abnormal beta oscillations are a consistent finding with a strong relation to abnormal behavior. Hence, beta-band oscillations could be a promising target for interventional strategies based on rhythmic NIBS.
SCZ has a life-time prevalence of 0.4% and belongs to the thirty most disabling disorders worldwide (Murray and Lopez, 1997; McGrath et al., 2008). Besides psychotic symptoms patients with SCZ show abnormalities in cognitive performance, e.g., in working memory, cognitive control and sensory gating. These cognitive deficits are, on the one hand, associated with clinical and functional outcome (Green et al., 2000; Gold, 2004; McGrath et al., 2008). On the other hand they are related with abnormalities in gamma-band oscillations (Johannesen et al., 2005; Uhlhaas et al., 2008; Barr et al., 2010; Sun et al., 2011). Compared to healthy controls, patients with SCZ showed excessive gamma-band oscillations in conditions with high memory load (Barr et al., 2010). Altered gamma-band activity was also found in the resting brain of SCZ patients (Andreou et al., 2014). Furthermore, evoked gamma power and phase synchronization were reduced after auditory stimulation in patients with SCZ (Light et al., 2006). In line with these results, after a 40 Hz binaural stimulation a reduced phase locking between primary auditory cortices was observed in patients with SCZ, which correlated with auditory hallucination scores (Mulert et al., 2011).
Moreover, combination of TMS and EEG has been used in SCZ research by using a TMS double pulse paradigm (Farzan et al., 2009) called long intracortical inhibition (LICI). LICI is an established, probably GABAB mediated measurement for cortical inhibition in motor cortex using motor-evoked potentials as a readout (McDonnell et al., 2006). Both GABAA and GABAB suggested to be involved in the generation of gamma-band oscillations (Wang and Buzsáki, 1996; Brown et al., 2007). Recently, EEG parameters were established as another readout for double pulse TMS paradigms in non motor areas like DLPFC (Daskalakis et al., 2008). A study in healthy subjects demonstrated that applying a TMS LICI protocol over DLPFC led to a decrease of gamma oscillations whereas gamma oscillations over motor cortex did not change after LICI application (Farzan et al., 2009). In contrast, patients with SCZ showed an impaired LICI-mediated inhibition of gamma oscillations in DLPFC compared to healthy participants and patients with bipolar disorder (Farzan et al., 2010a).
In summary, gamma-band oscillations have been related with cognitive performance in the healthy brain (Tallon-Baudry et al., 1998; Herrmann et al., 2004). In SCZ abnormal gamma-band activity was associated with behavioral impairment (Johannesen et al., 2005; Uhlhaas et al., 2008; Barr et al., 2010; Sun et al., 2011; Andreou et al., 2014). In line with these results, gamma-band activity could be one potential target of NIBS in SCZ.
Targeting Pathological Oscillations by Non-Invasive Brain Stimulation
This section will address the question of how rhythmic NIBS could be used to modulate pathological oscillations and thereby modulate behavior and clinical symptoms in stroke, PD and SCZ.
Several studies have demonstrated that NIBS can improve functional outcome of stroke patients (Hummel and Cohen, 2005; Hummel et al., 2005, 2006; Kim et al., 2006; Cazzoli et al., 2012; for review Schulz et al., 2013). To our knowledge, however, there is to date limited data on the effect of NIBS on oscillations in stroke. In six patients with severe hemiparesis, tDCS modulated alpha-band oscillations of sensorimotor areas during imaginary movements of the affected hand (Kasashima et al., 2012). Since detection of stable brain rhythms can be difficult in damaged brain areas after stroke, the aim of the latter study was to enhance oscillatory activity in the lesioned hemisphere by tDCS in order to be able to achieve a stable and well distinguishable brain signal for use in a brain-computer-interface (BCI). Event related alpha-band desynchronisation, a correlate of local activation, was significantly enhanced after tDCS stimulation compared to sham stimulation, making the signal more suitable for a BCI.
Although several studies have applied NIBS in stroke patients, only one case report has used rhythmic NIBS to modulate oscillations. In a patient with aphasia 10 Hz rTMS modulated both oscillatory activity and clinical outcome (Dammekens et al., 2014). In the treated patient the stroke lesion affected the left inferior frontal gyrus (IFG), which is part of the language network (Crosson et al., 2007). Generally, according to the concept of an interhemispheric rivalry in stroke (Murase et al., 2004; Duque et al., 2005), inhibitory rTMS protocols of 1 Hz stimulation frequency are applied to the healthy hemisphere in order to prevent interhemispheric maladaptive processes in contralesional networks. Another approach is to excite the damaged hemisphere with rTMS protocols with a frequency above 5 Hz (Lefaucheur, 2006). In the latter study, excitatory rTMS was applied over the damaged left IFG in daily sessions over 3 weeks leading to an increase of FC between left and right IFG in the theta- and high beta-band. Behaviorally, the patient improved on repetition tasks for naming and comprehension. In addition to being a case report, this study does certainly not establish a causal relation between modulation of oscillations and changes in behavior. Nevertheless, this study provides first hints that rhythmic NIBS might be effective in modulating both oscillatory activity and clinical outcome.
Based on the emerging understanding about the relationship between NIBS and the modulation or induction of oscillatory activity in the last decad, hypotheses on how to enhance brain functioning and ameliorate impaired behavior after focal brain lesions can be defined. These hypotheses would be amenable to evaluation in controlled clinical trials in stroke patients. Combining the evidence that (1) changes in alpha-band activity are correlated with recovery and performance after stroke (Dubovik et al., 2012; Westlake et al., 2012) and that (2) NIBS is able modulate alpha-band activity (Plewnia et al., 2008; Thut et al., 2011) one could consider applying rhythmic alpha-NIBS after stroke. As illustrated schematically in Figure 1A, bifocal TMS at alpha frequency could entrain alpha-band activity between lesioned areas and relevant neighboring regions. In this context, bifocal application should potentially be performed with a certain phase lag between the targeted areas. An application of less focal alpha tACS in a montage covering both targeted areas would be another option. In both cases rhythmic NIBS would potentially lead to a synchronization in the alpha-band of lesioned sites and relevant connected areas like ipsilateral and contralateral premotor cortices respectively (Johansen-Berg et al., 2002; Ward et al., 2003; Gerloff et al., 2006; Rehme et al., 2011; Dubovik et al., 2012; Westlake et al., 2012).
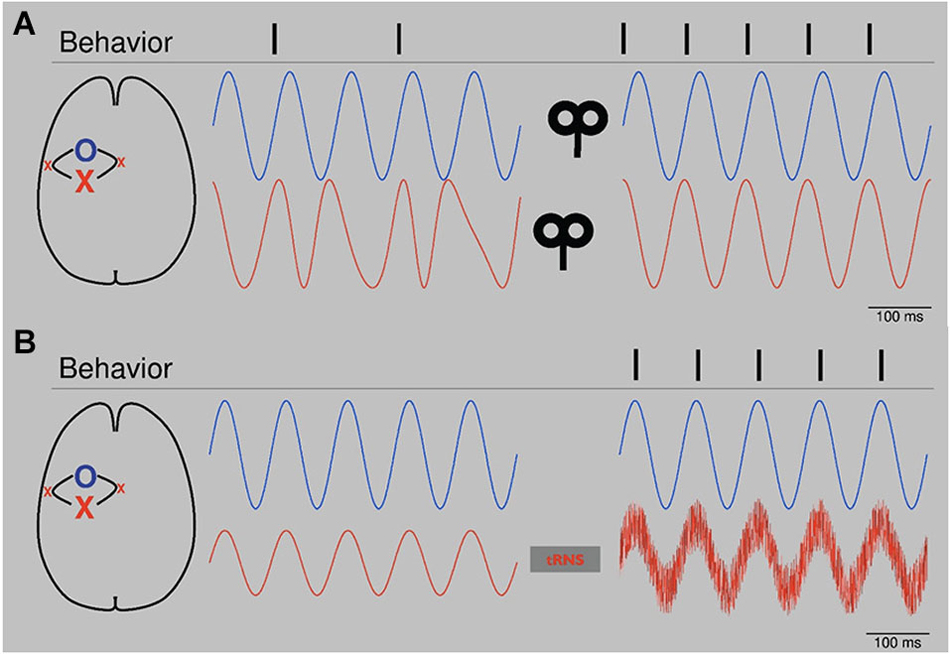
Figure 1. (A) Schematic: Bifocal repetitive TMS (rTMS) restores alpha-band activity. After stroke, alpha oscillators (red line, left) of the affected area (red X, primary motor cortex) show unsteady phase properties. Since physiological behavior depends on a constant phase relation of alpha oscillations between the affected area and connected networks (blue O, blue line, left, premotor areas), the patient is impaired (behavior, left, e.g., muscle contractions). After application of bifocal, rTMS at alpha frequency over both areas with a lag reflecting physiological offset of oscillators, oscillatory activity is synchronized (blue and red line, right) in terms of a constant phase lag. Behavior improves (right). Please note that conditions are highly simplified. First, next to phase, also amplitude is important for signal processing. Second, in the presented physiological condition (right) phases are completely locked, which is artificial. Naturally, phases would fluctuate. (B) Schematic: tRNS augments alpha oscillators. After stroke alpha activity (red line, left) of the affected area (red X) is reduced. Threshold for establishing information processing with a related network (blue line, left) is not reached and dependent normal behavior is reduced. tRNS applied over the affected oscillator augments alpha activity by adding noise (red line, right). Information processing is re-established and behavior improves. Please note that conditions are very much simplified. In both cases, phase is totally locked without any phase lag. Biological signals would fluctuate.
Another option to entrain alpha-band activity could be to add noise, as shown in Figure 1B. tRNS is a technique which has been used to modulate cortical excitability and BOLD activity (Terney et al., 2008; Saiote et al., 2013). It has been suggested that, by adding noise, tRNS is able to augment cortical oscillations of different frequencies (Antal and Paulus, 2013). In stroke patients, tRNS could elevate reduced alpha-band activity in affected parts of the brain back to suprathreshold levels (Moss et al., 2004) and thereby restore important connective functions. Analogue to the potential effects on the aging brain, rhythmic or patterned NIBS like tRNS, tACS and rTMS could be used to entrain recruitment of other brain areas to support both affected networks and functional outcome (Gutchess, 2014).
Regarding the results of reduced alpha peak frequency and increased alpha amplitude in the AH after stroke (Tecchio et al., 2005) one could argue that behavioral changes might be related to alpha induced inhibition (Jensen and Mazaheri, 2010). In contrast to changes in FC (Dubovik et al., 2012; Westlake et al., 2012), these changes were not correlated with behavioral changes, however, and gaining clinical benefit by modulating these features of local oscillatory activity remains an untested possibility.
In addition to the modulation of electrophysiological dynamics, NIBS might also influence molecular environment of neurons. By modulating slow wave activity occurring after stroke (Tecchio et al., 2005; Dubovik et al., 2012; Laaksonen et al., 2013) related neural repair might be modulated. Since there is first evidence in an animal model that there might be an association of slow neuronal firing, axonal recovery and recovery of function (Ming et al., 2001; Carmichael and Chesselet, 2002; Overman et al., 2012), one should consider a slow frequency stimulation as performed before in different contexts (Marshall et al., 2011) to gain axonal sprouting and improve clinical outcome after brain injury like stroke.
As discussed in the preceding section, pathological beta oscillations are a consistent finding in basal ganglia correlated with behavioral changes in PD patients (Levy et al., 2002; Kühn et al., 2008, 2009; Crowell et al., 2012; Heinrichs-Graham et al., 2014; Herz et al., 2014). It is known that these abnormal beta-band oscillations can be modulated by established DBS protocols. In addition, a number of studies investigated the effects of NIBS on motor function in patients with PD. Applying 5 Hz rTMS over primary motor cortex led to a short term increase of motor function in six PD patients (Siebner et al., 2000). A 5 Hz rTMS protocol consisting of 2000 pulses per day over ten days led to significant reduction of motor impairment (Khedr et al., 2003). Moreover, rTMS over inferior frontal cortex modulated event-related potentials in the subthalamic nucleus (STN) in patients with implanted DBS electrodes (Rektor et al., 2010). Though different stimulation locations were used for both techniques, resting-state functional-connectivity MRI analysis revealed, that targets of stimulation might be nodes in the same network (Fox et al., 2014).
In our view, the clinical effectiveness of these two stimulation techniques and their probably network-associated ability to modulate cortex-basal ganglia dynamics point to the great potential of NIBS applications in PD. As discussed above, pathological beta oscillations could be a promising target for rhythmic NIBS. A recent study has demonstrated the possibility to enhance the “akinetic” effect of beta oscillations. In a task where subjects got a cue to stop a planned grip-movement, tACS stimulation with 20 Hz significantly reduced grip force in that condition (Joundi et al., 2012). One the one hand these results underline the potential impact of enhanced beta oscillations in “akinetic” disorders like PD. On the other hand, the results point out the ability of cortical NIBS to affect loops involving the basal ganglia.
To our knowledge, however, it has not been possible to reduce pathological beta oscillations in PD by NIBS. Potentially, reducing beta activity could be achieved by phase cancellation. Phase cancellation by application of tACS has already been used for tremor reduction in PD. Targeting tremor-associated oscillations in such an approach in patients with tremor-dominant PD led to an almost 50% suppression of resting tremor (Brittain et al., 2013). As a first step in the study, the individual tremor frequency of each patient was measured. In a second step, tremor-frequency adjusted tACS was applied over the primary motor cortex at different phase angles. Certain phase angles led to tremor reduction whereas stimulation at different angles led to an increase of tremor. This study underlines the great potential of individualized application of rhythmic NIBS and suggests the potential efficacy of phase cancellation. Based on monkey data, phase cancellation has also been suggested as a physiological mechanism for controlling tremor in spinal cord networks (Williams et al., 2010). As illustrated schematically in Figure 2A, phase cancellation might be applied to pathological beta oscillations in PD potentially targeting bradykinesia and rigidity (Ray et al., 2008; Kühn et al., 2009).
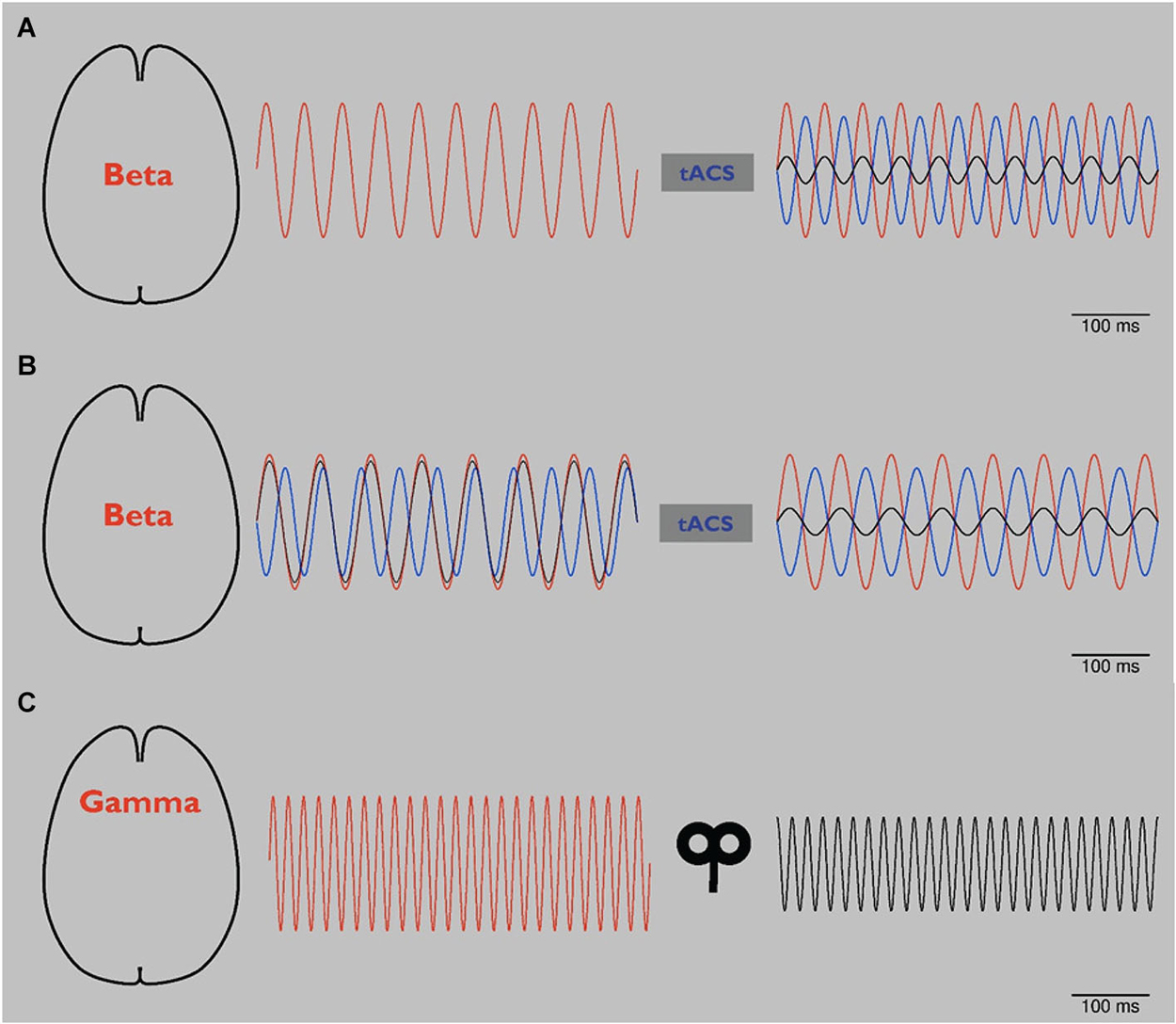
Figure 2. (A) Schematic: tACS in Parkinson’s disease (PD). Abnormal beta oscillations (red line, left and right) are related to rigidity and bradykinesia. Application of tACS with a phase lag of 180° (blue line, right) leads to a reduction of these pathological oscillations (black line, right) by phase cancellation (right). As a result, rigidity and bradykinesia are reduced. Please note that to date phase cancellation has been thought to be able to reduce tremor in PD by affecting tremor related cortical frequencies. To our knowledge, beta frequency has not yet been targeted by NIBS using the phase cancellation concept. (B) Schematic: tACS in PD using online readout techniques. Since it has been reported that during tACS application tremor frequency changed in patients with PD, more online readout techniques should be developed to adjust NIBS applications. After application of 20 Hz tACS (see panel A), pathological beta rhythm changes its frequency to 15 Hz (red line, left). tACS of 20 Hz with 180° phase lag (blue line, left) looses its effect (black line, left). After online adjustment, tACS shifts to 15 Hz (blue line, right). Pathological beta (red line, right) is again reduced (black line, right). (C) Schematic: rTMS in schizophrenia (SCZ). By modulating oscillators of abnormal gamma-band activity (red line, left), amplitude is reduced (black line, right) and related behavioral impairments are reduced.
Since tremor shifted to a different frequency in some of the patients in the study by Brittain et al. (2013), tACS application should be adjusted to ongoing tremor activity to provide an effective modulation, as shown schematically in Figure 2B. To this end, online read-out techniques that allow stimulation in dependence on ongoing oscillatory activity (Berényi et al., 2012; Bergmann et al., 2012) will be highly advantageous in order to adjust NIBS to changing pathologic frequency and provide effective phase cancellation and reduction of symptoms. In a clinical setting such an “online”, closed-loop approach has already been tested successfully: in PD DBS has been triggered based on recorded local field potentials (Little et al., 2013). These interventional strategies are interesting and potentially promising for amelioration of symptoms not well responsive to DBS.
In SCZ, combining single or double pulse TMS with EEG revealed abnormalities of cortical inhibition of gamma oscillations (Farzan et al., 2009, 2010b). Both studies point towards a scientific benefit of combining these methods and suggest that measurements on TMS-evoked oscillatory activity could be useful as a “biomarker” for neuropsychiatric disorders. Applying a similar approach, TMS evoked EEG activity has been proposed to add information to diagnostic procedures for chronic disorders of consciousness (Ragazzoni et al., 2013).
Abnormal gamma-band oscillations of patients with SCZ have been modulated by rTMS (Barr et al., 2011). In an N-back working memory task, subjects were asked to compare stimuli with those from previous trials. 20 Hz TMS applied bilaterally to DLPFC in reduced gamma activity in the SCZ patients (Barr et al., 2011). This approach, which is schematically presented in Figure 2C, could be considered as an “indirect” application, in which underlying gamma oscillators were modulated by an excitatory 20 Hz rTMS protocol not directly targeted at gamma frequencies. Since rTMS of a single session in the latter study did not change working memory behavior, the same group performed a pilot study with 20 Hz rTMS over DLPFC bilateraly for twenty sessions in a randomized double-blinded sham-controlled manner. This application led to a significant improvement of accuracy in working memory underlining that rTMS might potentially improve cognitive impairments in SCZ patients (Barr et al., 2013). Moreover, these results point towards the need for repetitive sessions of NIBS to gain long term effects. However, changes in gamma oscillations were not addressed in this follow-up study (Barr et al., 2013).
Taken together, application of rTMS seem to be promising for the treatment of negative symptoms of SCZ, although so far only demonstrated in proof-of-principle studies. Gamma oscillations show a high diversity in SCZ (Sun et al., 2011) and, hence, a direct link between the modulation of gamma oscillations and an improved cognitive performance in SCZ remains speculative. Nevertheless, further applications for the treatment of negative symptoms based on safe and low cost NIBS techniques addressing specifically gamma oscillations should be further developed and evaluated in detail in future studies.
Summary and Further Perspectives of NIBS
Evidence is increasing that NIBS may provide a novel and promising strategy to modulate both oscillatory activity and related behavior in the healthy brain. To date only a limited number of studies have employed NIBS to modulate oscillatory abnormalities in neuropsychiatric disorders as a treatment strategy. As discussed above, this concept is supported by studies suggesting, for example, that tACS can reduce tremor in PD (Brittain et al., 2013) or that rhythmic TMS can improve both working memory (Barr et al., 2013) and impaired gamma activity in patients with SCZ (Barr et al., 2011). In stroke, NIBS might modulate both oscillatory activity and clinical performance (Dammekens et al., 2014). Taken together, the application of rhythmic NIBS in order to modulate underlying, disease related oscillations is a very promising approach.
Next steps will be the development and evaluation of safe and low-cost applications of patterned NIBS in order to target pathological oscillatory activity to achieve improvement of clinical symptoms of neuropsychiatric patients impacting on their daily life. Demonstrating the expected effects of NIBS in patients might have an incremental impact on treatment of neuropsychiatric disorders and subsequently the health system, as novel devices has been developed for home-based, self-application of NIBS. As soon as such approaches will be proven save in the home-based environment, they may offer a cost-effective strategy to significantly enhance treatment intensity.
Still one can argue critically that oscillatory changes after NIBS application are not a cause but a by-product merely associated with behavioral changes. However, combining NIBS with electrophysiological recordings bears great potential to establish the specificity and reliability of oscillations as biomarkers.
Conflict of Interest Statement
The authors declare that the research was conducted in the absence of any commercial or financial relationships that could be construed as a potential conflict of interest.
Acknowledgments
This work has been supported by the German Research Foundation (DFG, SFB936/A2/A3/C4) and by the European Union (ERC-2010-AdG-269716 “MULTISENSE”).
References
Andreou, C., Nolte, G., Leicht, G., Polomac, N., Hanganu-Opatz, I. L., Lambert, M., et al. (2014). Increased resting-state gamma-band connectivity in first-episode schizophrenia. Schizophr. Bull. doi: 10.1093/schbul/sbu121. [Epub ahead of print].
PubMed Abstract | Full Text | CrossRef Full Text | Google Scholar
Antal, A., and Paulus, W. (2013). Transcranial alternating current stimulation (tACS). Front. Hum. Neurosci. 7:317. doi: 10.3389/fnhum.2013.00317
PubMed Abstract | Full Text | CrossRef Full Text | Google Scholar
Barr, M. S., Farzan, F., Arenovich, T., Chen, R., Fitzgerald, P. B., and Daskalakis, Z. J. (2011). The effect of repetitive transcranial magnetic stimulation on gamma oscillatory activity in schizophrenia. PLoS One 6:e22627. doi: 10.1371/journal.pone.0022627
PubMed Abstract | Full Text | CrossRef Full Text | Google Scholar
Barr, M. S., Farzan, F., Rajji, T. K., Voineskos, A. N., Blumberger, D. M., Arenovich, T., et al. (2013). Can repetitive magnetic stimulation improve cognition in schizophrenia? Pilot data from a randomized controlled trial. Biol. Psychiatry 73, 510–517. doi: 10.1016/j.biopsych.2012.08.020
PubMed Abstract | Full Text | CrossRef Full Text | Google Scholar
Barr, M. S., Farzan, F., Tran, L. C., Chen, R., Fitzgerald, P. B., and Daskalakis, Z. J. (2010). Evidence for excessive frontal evoked gamma oscillatory activity in schizophrenia during working memory. Schizophr. Res. 121, 146–152. doi: 10.1016/j.schres.2010.05.023
PubMed Abstract | Full Text | CrossRef Full Text | Google Scholar
Berényi, A., Belluscio, M., Mao, D., and Buzsáki, G. (2012). Closed-loop control of epilepsy by transcranial electrical stimulation. Science 337, 735–737. doi: 10.1126/science.1223154
PubMed Abstract | Full Text | CrossRef Full Text | Google Scholar
Bergmann, T. O., Mölle, M., Schmidt, M. A., Lindner, C., Marshall, L., Born, J., et al. (2012). EEG-guided transcranial magnetic stimulation reveals rapid shifts in motor cortical excitability during the human sleep slow oscillation. J. Neurosci. 32, 243–253. doi: 10.1523/JNEUROSCI.4792-11.2012
PubMed Abstract | Full Text | CrossRef Full Text | Google Scholar
Brittain, J. S., Probert-Smith, P., Aziz, T. Z., and Brown, P. (2013). Tremor suppression by rhythmic transcranial current stimulation. Curr. Biol. 23, 436–440. doi: 10.1016/j.cub.2013.01.068
PubMed Abstract | Full Text | CrossRef Full Text | Google Scholar
Brown, P. (2003). Oscillatory nature of human basal ganglia activity: relationship to the pathophysiology of Parkinson’s disease. Mov. Disord. 18, 357–363. doi: 10.1002/mds.10358
PubMed Abstract | Full Text | CrossRef Full Text | Google Scholar
Brown, J. T., Davies, C. H., and Randall, A. D. (2007). Synaptic activation of GABA(B) receptors regulates neuronal network activity and entrainment. Eur. J. Neurosci. 25, 2982–2990. doi: 10.1111/j.1460-9568.2007.05544.x
PubMed Abstract | Full Text | CrossRef Full Text | Google Scholar
Brown, P., Oliviero, A., Mazzone, P., Insola, A., Tonali, P., and Di Lazzaro, V. (2001). Dopamine dependency of oscillations between subthalamic nucleus and pallidum in Parkinson’s disease. J. Neurosci. 21, 1033–1038.
Busch, N. A., Dubois, J., and VanRullen, R. (2009). The phase of ongoing EEG oscillations predicts visual perception. J. Neurosci. 29, 7869–7876. doi: 10.1523/JNEUROSCI.0113-09.2009
PubMed Abstract | Full Text | CrossRef Full Text | Google Scholar
Butz, M., Gross, J., Timmermann, L., Moll, M., Freund, H.-J., Witte, O. W., et al. (2004). Perilesional pathological oscillatory activity in the magnetoencephalogram of patients with cortical brain lesions. Neurosci. Lett. 355, 93–96. doi: 10.1016/j.neulet.2003.10.065
PubMed Abstract | Full Text | CrossRef Full Text | Google Scholar
Buzsáki, G., and Draguhn, A. (2004). Neuronal oscillations in cortical networks. Science 304, 1926–1929. doi: 10.1126/science.1099745
PubMed Abstract | Full Text | CrossRef Full Text | Google Scholar
Buzsáki, G., and Wang, X.-J. (2012). Mechanisms of gamma oscillations. Annu. Rev. Neurosci. 35, 203–225. doi: 10.1146/annurev-neuro-062111-150444
PubMed Abstract | Full Text | CrossRef Full Text | Google Scholar
Carmichael, S. T., and Chesselet, M.-F. (2002). Synchronous neuronal activity is a signal for axonal sprouting after cortical lesions in the adult. J. Neurosci. 22, 6062–6070.
Cazzoli, D., Müri, R. M., Schumacher, R., von Arx, S., Chaves, S., Gutbrod, K., et al. (2012). Theta burst stimulation reduces disability during the activities of daily living in spatial neglect. Brain 135(Pt. 11), 3426–3439. doi: 10.1093/brain/aws182
PubMed Abstract | Full Text | CrossRef Full Text | Google Scholar
Crosson, B., McGregor, K., Gopinath, K. S., Conway, T. W., Benjamin, M., Chang, Y., et al. (2007). Functional MRI of language in aphasia: a review of the literature and the methodological challenges. Neuropsychol. Rev. 17, 157–177. doi: 10.1007/s11065-007-9024-z
PubMed Abstract | Full Text | CrossRef Full Text | Google Scholar
Crowell, A. L., Ryapolova-Webb, E. S., Ostrem, J. L., Galifianakis, N. B., Shimamoto, S., Lim, D. A., et al. (2012). Oscillations in sensorimotor cortex in movement disorders: an electrocorticography study. Brain 135(Pt. 2), 615–630. doi: 10.1093/brain/awr332
PubMed Abstract | Full Text | CrossRef Full Text | Google Scholar
Dammekens, E., Vanneste, S., Ost, J., and De Ridder, D. (2014). Neural correlates of high frequency repetitive transcranial magnetic stimulation improvement in post-stroke non-fluent aphasia: a case study. Neurocase 20, 1–9. doi: 10.1080/13554794.2012.713493
PubMed Abstract | Full Text | CrossRef Full Text | Google Scholar
Daskalakis, Z. J., Farzan, F., Barr, M. S., Maller, J. J., Chen, R., and Fitzgerald, P. B. (2008). Long-interval cortical inhibition from the dorsolateral prefrontal cortex: a TMS-EEG study. Neuropsychopharmacology 33, 2860–2869. doi: 10.1038/npp.2008.22
PubMed Abstract | Full Text | CrossRef Full Text | Google Scholar
Dubovik, S., Pignat, J.-M., Ptak, R., Aboulafia, T., Allet, L., Gillabert, N., et al. (2012). The behavioral significance of coherent resting-state oscillations after stroke. Neuroimage 61, 249–257. doi: 10.1016/j.neuroimage.2012.03.024
PubMed Abstract | Full Text | CrossRef Full Text | Google Scholar
Duque, J., Hummel, F., Celnik, P., Murase, N., Mazzocchio, R., and Cohen, L. G. (2005). Transcallosal inhibition in chronic subcortical stroke. Neuroimage 28, 940–946. doi: 10.1016/j.neuroimage.2005.06.033
PubMed Abstract | Full Text | CrossRef Full Text | Google Scholar
Engel, A. K., and Fries, P. (2010). Beta-band oscillations–signalling the status quo? Curr. Opin. Neurobiol. 20, 156–165. doi: 10.1016/j.conb.2010.02.015
PubMed Abstract | Full Text | CrossRef Full Text | Google Scholar
Engel, A. K., Fries, P., and Singer, W. (2001). Dynamic predictions: oscillations and synchrony in top-down processing. Nat. Rev. Neurosci. 2, 704–716. doi: 10.1038/35094565
PubMed Abstract | Full Text | CrossRef Full Text | Google Scholar
Farzan, F., Barr, M. S., Levinson, A. J., Chen, R., Wong, W., Fitzgerald, P. B., et al. (2010a). Evidence for gamma inhibition deficits in the dorsolateral prefrontal cortex of patients with schizophrenia. Brain 133(Pt. 5), 1505–1514. doi: 10.1093/brain/awq046
PubMed Abstract | Full Text | CrossRef Full Text | Google Scholar
Farzan, F., Barr, M. S., Levinson, A. J., Chen, R., Wong, W., Fitzgerald, P. B., et al. (2010b). Reliability of long-interval cortical inhibition in healthy human subjects: a TMS-EEG study. J. Neurophysiol. 104, 1339–1346. doi: 10.1152/jn.00279.2010
PubMed Abstract | Full Text | CrossRef Full Text | Google Scholar
Farzan, F., Barr, M. S., Wong, W., Chen, R., Fitzgerald, P. B., and Daskalakis, Z. J. (2009). Suppression of gamma-oscillations in the dorsolateral prefrontal cortex following long interval cortical inhibition: a TMS-EEG study. Neuropsychopharmacology 34, 1543–1551. doi: 10.1038/npp.2008.211
PubMed Abstract | Full Text | CrossRef Full Text | Google Scholar
Fox, M. D., Buckner, R. L., Liu, H., Chakravarty, M. M., Lozano, A. M., and Pascual-Leone, A. (2014). Resting-state networks link invasive and noninvasive brain stimulation across diverse psychiatric and neurological diseases. Proc. Natl. Acad. Sci. U S A 111, E4367–E4375. doi: 10.1073/pnas.1405003111
PubMed Abstract | Full Text | CrossRef Full Text | Google Scholar
Fries, P. (2009). Neuronal gamma-band synchronization as a fundamental process in cortical computation. Annu. Rev. Neurosci. 32, 209–224. doi: 10.1146/annurev.neuro.051508.135603
PubMed Abstract | Full Text | CrossRef Full Text | Google Scholar
Gerloff, C., Bushara, K., Sailer, A., Wassermann, E. M., Chen, R., Matsuoka, T., et al. (2006). Multimodal imaging of brain reorganization in motor areas of the contralesional hemisphere of well recovered patients after capsular stroke. Brain 129(Pt. 3), 791–808. doi: 10.1093/brain/awh713
PubMed Abstract | Full Text | CrossRef Full Text | Google Scholar
Gilbertson, T., Lalo, E., Doyle, L., Di Lazzaro, V., Cioni, B., and Brown, P. (2005). Existing motor state is favored at the expense of new movement during 13–35 Hz oscillatory synchrony in the human corticospinal system. J. Neurosci. 25, 7771–7779. doi: 10.1523/jneurosci.1762-05.2005
PubMed Abstract | Full Text | CrossRef Full Text | Google Scholar
Gold, J. M. (2004). Cognitive deficits as treatment targets in schizophrenia. Schizophr. Res. 72, 21–28. doi: 10.1016/j.schres.2004.09.008
PubMed Abstract | Full Text | CrossRef Full Text | Google Scholar
Green, M. F., Kern, R. S., Braff, D. L., and Mintz, J. (2000). Neurocognitive deficits and functional outcome in schizophrenia: are we measuring the “right stuff”? Schizophr. Bull. 26, 119–136. doi: 10.1093/oxfordjournals.schbul.a033430
PubMed Abstract | Full Text | CrossRef Full Text | Google Scholar
Groppa, S., Bergmann, T. O., Siems, C., Mölle, M., Marshall, L., and Siebner, H. R. (2010). Slow-oscillatory transcranial direct current stimulation can induce bidirectional shifts in motor cortical excitability in awake humans. Neuroscience 166, 1219–1225. doi: 10.1016/j.neuroscience.2010.01.019
PubMed Abstract | Full Text | CrossRef Full Text | Google Scholar
Gutchess, A. (2014). Plasticity of the aging brain: new directions in cognitive neuroscience. Science 346, 579–582. doi: 10.1126/science.1254604
PubMed Abstract | Full Text | CrossRef Full Text | Google Scholar
Haegens, S., Osipova, D., Oostenveld, R., and Jensen, O. (2010). Somatosensory working memory performance in humans depends on both engagement and disengagement of regions in a distributed network. Hum. Brain Mapp. 31, 26–35. doi: 10.1002/hbm.20842
PubMed Abstract | Full Text | CrossRef Full Text | Google Scholar
Hallett, M. (2007). Transcranial magnetic stimulation: a primer. Neuron 55, 187–199. doi: 10.1016/j.neuron.2007.06.026
PubMed Abstract | Full Text | CrossRef Full Text | Google Scholar
Heinrichs-Graham, E., Wilson, T. W., Santamaria, P. M., Heithoff, S. K., Torres-Russotto, D., Hutter-Saunders, J. A. L., et al. (2014). Neuromagnetic evidence of abnormal movement-related beta desynchronization in Parkinson’s disease. Cereb. Cortex 24, 2669–2678. doi: 10.1093/cercor/bht121
PubMed Abstract | Full Text | CrossRef Full Text | Google Scholar
Helfrich, R. F., Schneider, T. R., Rach, S., Trautmann-Lengsfeld, S. A., Engel, A. K., and Herrmann, C. S. (2014). Entrainment of brain oscillations by transcranial alternating current stimulation. Curr. Biol. 24, 333–339. doi: 10.1016/j.cub.2013.12.041
PubMed Abstract | Full Text | CrossRef Full Text | Google Scholar
Herrmann, C. S., Munk, M. H. J., and Engel, A. K. (2004). Cognitive functions of gamma-band activity: memory match and utilization. Trends Cogn. Sci. 8, 347–355. doi: 10.1016/j.tics.2004.06.006
PubMed Abstract | Full Text | CrossRef Full Text | Google Scholar
Herz, D. M., Florin, E., Christensen, M. S., Reck, C., Barbe, M. T., Tscheuschler, M. K., et al. (2014). Dopamine replacement modulates oscillatory coupling between premotor and motor cortical areas in Parkinson’s disease. Cereb. Cortex 24, 2873–2883. doi: 10.1093/cercor/bht140
PubMed Abstract | Full Text | CrossRef Full Text | Google Scholar
Huang, Y.-Z., Edwards, M. J., Rounis, E., Bhatia, K. P., and Rothwell, J. C. (2005). Theta burst stimulation of the human motor cortex. Neuron 45, 201–206. doi: 10.1016/j.neuron.2004.12.033
PubMed Abstract | Full Text | CrossRef Full Text | Google Scholar
Hummel, F., Andres, F., Altenmüeller, E., Dichgans, J., and Gerloff, C. (2002). Inhibitory control of acquired motor programmes in the human brain. Brain 125(Pt. 2), 404–420. doi: 10.1093/brain/awf030
PubMed Abstract | Full Text | CrossRef Full Text | Google Scholar
Hummel, F., Celnik, P., Giraux, P., Floel, A., Wu, W.-H., Gerloff, C., et al. (2005). Effects of non-invasive cortical stimulation on skilled motor function in chronic stroke. Brain 128(Pt. 3), 490–499. doi: 10.1093/brain/awh369
PubMed Abstract | Full Text | CrossRef Full Text | Google Scholar
Hummel, F. C., and Cohen, L. G. (2005). Drivers of brain plasticity. Curr. Opin. Neurol. 18, 667–674. doi: 10.1097/01.wco.0000189876.37475.42
PubMed Abstract | Full Text | CrossRef Full Text | Google Scholar
Hummel, F., and Gerloff, C. (2005). Larger interregional synchrony is associated with greater behavioral success in a complex sensory integration task in humans. Cereb. Cortex 15, 670–678. doi: 10.1093/cercor/bhh170
PubMed Abstract | Full Text | CrossRef Full Text | Google Scholar
Hummel, F. C., Voller, B., Celnik, P., Floel, A., Giraux, P., Gerloff, C., et al. (2006). Effects of brain polarization on reaction times and pinch force in chronic stroke. BMC. Neurosci. 7:73. doi: 10.1186/1471-2202-7-73
PubMed Abstract | Full Text | CrossRef Full Text | Google Scholar
Jensen, O., Kaiser, J., and Lachaux, J.-P. (2007). Human gamma-frequency oscillations associated with attention and memory. Trends Neurosci. 30, 317–324. doi: 10.1016/j.tins.2007.05.001
PubMed Abstract | Full Text | CrossRef Full Text | Google Scholar
Jensen, O., and Mazaheri, A. (2010). Shaping functional architecture by oscillatory alpha activity: gating by inhibition. Front. Hum. Neurosci. 4:186. doi: 10.3410/f.6253958.6325058
PubMed Abstract | Full Text | CrossRef Full Text | Google Scholar
Johannesen, J. K., Kieffaber, P. D., O’Donnell, B. F., Shekhar, A., Evans, J. D., and Hetrick, W. P. (2005). Contributions of subtype and spectral frequency analyses to the study of P50 ERP amplitude and suppression in schizophrenia. Schizophr. Res. 78, 269–284. doi: 10.1016/j.schres.2005.05.022
PubMed Abstract | Full Text | CrossRef Full Text | Google Scholar
Johansen-Berg, H., Rushworth, M. F. S., Bogdanovic, M. D., Kischka, U., Wimalaratna, S., and Matthews, P. M. (2002). The role of ipsilateral premotor cortex in hand movement after stroke. Proc. Natl. Acad. Sci. U S A 99, 14518–14523. doi: 10.1073/pnas.222536799
PubMed Abstract | Full Text | CrossRef Full Text | Google Scholar
Joundi, R. A., Jenkinson, N., Brittain, J.-S., Aziz, T. Z., and Brown, P. (2012). Driving oscillatory activity in the human cortex enhances motor performance. Curr. Biol. 22, 403–407. doi: 10.1016/j.cub.2012.01.024
PubMed Abstract | Full Text | CrossRef Full Text | Google Scholar
Kasashima, Y., Fujiwara, T., Matsushika, Y., Tsuji, T., Hase, K., Ushiyama, J., et al. (2012). Modulation of event-related desynchronization during motor imagery with transcranial direct current stimulation (tDCS) in patients with chronic hemiparetic stroke. Exp. Brain Res. 221, 263–268. doi: 10.1007/s00221-012-3166-9
PubMed Abstract | Full Text | CrossRef Full Text | Google Scholar
Khedr, E. M., Farweez, H. M., and Islam, H. (2003). Therapeutic effect of repetitive transcranial magnetic stimulation on motor function in Parkinson’s disease patients. Eur. J. Neurol. 10, 567–572. doi: 10.1046/j.1468-1331.2003.00649.x
PubMed Abstract | Full Text | CrossRef Full Text | Google Scholar
Kilner, J. M., Baker, S. N., Salenius, S., Hari, R., and Lemon, R. N. (2000). Human cortical muscle coherence is directly related to specific motor parameters. J. Neurosci. 20, 8838–8845.
Kim, Y.-H., You, S. H., Ko, M.-H., Park, J.-W., Lee, K. H., Jang, S. H., et al. (2006). Repetitive transcranial magnetic stimulation-induced corticomotor excitability and associated motor skill acquisition in chronic stroke. Stroke 37, 1471–1476. doi: 10.1161/01.str.0000221233.55497.51
PubMed Abstract | Full Text | CrossRef Full Text | Google Scholar
Klimesch, W., Sauseng, P., and Hanslmayr, S. (2007). EEG alpha oscillations: the inhibition—timing hypothesis. Brain Res. Rev. 53, 63–88. doi: 10.1016/j.brainresrev.2006.06.003
PubMed Abstract | Full Text | CrossRef Full Text | Google Scholar
Kolominsky-Rabas, P. L., Weber, M., Gefeller, O., Neundoerfer, B., and Heuschmann, P. U. (2001). Epidemiology of ischemic stroke subtypes according to TOAST criteria: incidence, recurrence and long-term survival in ischemic stroke subtypes: a population-based study. Stroke 32, 2735–2740. doi: 10.1161/hs1201.100209
PubMed Abstract | Full Text | CrossRef Full Text | Google Scholar
Kühn, A. A., Kempf, F., Brücke, C., Gaynor Doyle, L., Martinez-Torres, I., Pogosyan, A., et al. (2008). High-frequency stimulation of the subthalamic nucleus suppresses oscillatory beta activity in patients with Parkinson’s disease in parallel with improvement in motor performance. J. Neurosci. 28, 6165–6173. doi: 10.1523/JNEUROSCI.0282-08.2008
PubMed Abstract | Full Text | CrossRef Full Text | Google Scholar
Kühn, A. A., Tsui, A., Aziz, T., Ray, N., Brücke, C., Kupsch, A., et al. (2009). Pathological synchronisation in the subthalamic nucleus of patients with Parkinson’s disease relates to both bradykinesia and rigidity. Exp. Neurol. 215, 380–387. doi: 10.1016/j.expneurol.2008.11.008
PubMed Abstract | Full Text | CrossRef Full Text | Google Scholar
Laaksonen, K., Helle, L., Parkkonen, L., Kirveskari, E., Mäkelä, J. P., Mustanoja, S., et al. (2013). Alterations in spontaneous brain oscillations during stroke recovery. PLoS One 8:e61146. doi: 10.1371/journal.pone.0061146
PubMed Abstract | Full Text | CrossRef Full Text | Google Scholar
Lapenta, O. M., Minati, L., Fregni, F., and Boggio, P. S. (2013). Je pense donc je fais: transcranial direct current stimulation modulates brain oscillations associated with motor imagery and movement observation. Front. Hum. Neurosci. 7:256. doi: 10.3389/fnhum.2013.00256
PubMed Abstract | Full Text | CrossRef Full Text | Google Scholar
Lefaucheur, J.-P. (2006). Stroke recovery can be enhanced by using repetitive transcranial magnetic stimulation (rTMS). Neurophysiol. Clin. 36, 105–115. doi: 10.1016/j.neucli.2006.08.011
PubMed Abstract | Full Text | CrossRef Full Text | Google Scholar
Levy, R., Ashby, P., Hutchison, W. D., Lang, A. E., Lozano, A. M., and Dostrovsky, J. O. (2002). Dependence of subthalamic nucleus oscillations on movement and dopamine in Parkinson’s disease. Brain 125(Pt. 6), 1196–1209. doi: 10.1093/brain/awf128
PubMed Abstract | Full Text | CrossRef Full Text | Google Scholar
Levy, R., Hutchison, W. D., Lozano, A. M., and Dostrovsky, J. O. (2000). High-frequency synchronization of neuronal activity in the subthalamic nucleus of parkinsonian patients with limb tremor. J. Neurosci. 20, 7766–7775.
Lewine, J. D., Davis, J. T., Sloan, J. H., Kodituwakku, P. W., and Orrison, W. W. (1999). Neuromagnetic assessment of pathophysiologic brain activity induced by minor head trauma. AJNR Am. J. Neuroradiol. 20, 857–866.
Light, G. A., Hsu, J. L., Hsieh, M. H., Meyer-Gomes, K., Sprock, J., Swerdlow, N. R., et al. (2006). Gamma band oscillations reveal neural network cortical coherence dysfunction in schizophrenia patients. Biol. Psychiatry 60, 1231–1240. doi: 10.1016/j.biopsych.2006.03.055
PubMed Abstract | Full Text | CrossRef Full Text | Google Scholar
Little, S., Pogosyan, A., Neal, S., Zavala, B., Zrinzo, L., Hariz, M., et al. (2013). Adaptive deep brain stimulation in advanced Parkinson’s disease. Ann. Neurol. 74, 449–457. doi: 10.1002/ana.23951
PubMed Abstract | Full Text | CrossRef Full Text | Google Scholar
Marshall, L., Helgadóttir, H., Mölle, M., and Born, J. (2006). Boosting slow oscillations during sleep potentiates memory. Nature 444, 610–613. doi: 10.1038/nature05278
PubMed Abstract | Full Text | CrossRef Full Text | Google Scholar
Marshall, L., Kirov, R., Brade, J., Mölle, M., and Born, J. (2011). Transcranial electrical currents to probe EEG brain rhythms and memory consolidation during sleep in humans. PLoS One 6:e16905. doi: 10.1371/journal.pone.0016905
PubMed Abstract | Full Text | CrossRef Full Text | Google Scholar
McDonnell, M. N., Orekhov, Y., and Ziemann, U. (2006). The role of GABA(B) receptors in intracortical inhibition in the human motor cortex. Exp. Brain Res. 173, 86–93. doi: 10.1007/s00221-006-0365-2
PubMed Abstract | Full Text | CrossRef Full Text | Google Scholar
McGrath, J., Saha, S., Chant, D., and Welham, J. (2008). Schizophrenia: a concise overview of incidence, prevalence and mortality. Epidemiol. Rev. 30, 67–76. doi: 10.1093/epirev/mxn001
PubMed Abstract | Full Text | CrossRef Full Text | Google Scholar
Ming, G., Henley, J., Tessier-Lavigne, M., Song, H., and Poo, M. (2001). Electrical activity modulates growth cone guidance by diffusible factors. Neuron 29, 441–452. doi: 10.1016/s0896-6273(01)00217-3
PubMed Abstract | Full Text | CrossRef Full Text | Google Scholar
Moss, F., Ward, L. M., and Sannita, W. G. (2004). Stochastic resonance and sensory information processing: a tutorial and review of application. Clin. Neurophysiol. 115, 267–281. doi: 10.1016/j.clinph.2003.09.014
PubMed Abstract | Full Text | CrossRef Full Text | Google Scholar
Mulert, C., Kirsch, V., Pascual-Marqui, R., McCarley, R. W., and Spencer, K. M. (2011). Long-range synchrony of γ oscillations and auditory hallucination symptoms in schizophrenia. Int. J. Psychophysiol. 79, 55–63. doi: 10.1016/j.ijpsycho.2010.08.004
PubMed Abstract | Full Text | CrossRef Full Text | Google Scholar
Murase, N., Duque, J., Mazzocchio, R., and Cohen, L. G. (2004). Influence of interhemispheric interactions on motor function in chronic stroke. Ann. Neurol. 55, 400–409. doi: 10.1002/ana.10848
PubMed Abstract | Full Text | CrossRef Full Text | Google Scholar
Murray, C. J., and Lopez, A. D. (1997). Global mortality, disability and the contribution of risk factors: global burden of disease study. Lancet 349, 1436–1442. doi: 10.1016/s0140-6736(96)07495-8
PubMed Abstract | Full Text | CrossRef Full Text | Google Scholar
Neuling, T., Rach, S., Wagner, S., Wolters, C. H., and Herrmann, C. S. (2012). Good vibrations: oscillatory phase shapes perception. Neuroimage 63, 771–778. doi: 10.1016/j.neuroimage.2012.07.024
PubMed Abstract | Full Text | CrossRef Full Text | Google Scholar
Nitsche, M. A., and Paulus, W. (2000). Excitability changes induced in the human motor cortex by weak transcranial direct current stimulation. J. Physiol. 527(Pt. 3), 633–639. doi: 10.1111/j.1469-7793.2000.t01-1-00633.x
PubMed Abstract | Full Text | CrossRef Full Text | Google Scholar
Noh, N. A., Fuggetta, G., Manganotti, P., and Fiaschi, A. (2012). Long lasting modulation of cortical oscillations after continuous theta burst transcranial magnetic stimulation. PLoS One 7:e35080. doi: 10.1371/journal.pone.0035080
PubMed Abstract | Full Text | CrossRef Full Text | Google Scholar
Osipova, D., Hermes, D., and Jensen, O. (2008). Gamma power is phase-locked to posterior alpha activity. PLoS One 3:e3990. doi: 10.1371/journal.pone.0003990
PubMed Abstract | Full Text | CrossRef Full Text | Google Scholar
Overman, J. J., Clarkson, A. N., Wanner, I. B., Overman, W. T., Eckstein, I., and Maguire, J. L. (2012). A role for ephrin-A5 in axonal sprouting , recovery and activity-dependent plasticity after stroke. Proc. Natl. Acad. Sci. U S A 109, E2230–E2239. doi: 10.1073/pnas.1204386109
PubMed Abstract | Full Text | CrossRef Full Text | Google Scholar
Palva, S., and Palva, J. M. (2007). New vistas for alpha-frequency band oscillations. Trends Neurosci. 30, 150–158. doi: 10.1016/j.tins.2007.02.001
PubMed Abstract | Full Text | CrossRef Full Text | Google Scholar
Pascual-Leone, A., Valls-Solé, J., Wassermann, E. M., and Hallett, M. (1994). Responses to rapid-rate transcranial magnetic stimulation of the human motor cortex. Brain 117(Pt. 4), 847–858. doi: 10.1093/brain/117.4.847
PubMed Abstract | Full Text | CrossRef Full Text | Google Scholar
Pfurtscheller, G. (1992). Event-related synchronization (ERS): an electrophysiological correlate of cortical areas at rest. Electroencephalogr. Clin. Neurophysiol. 83, 62–69. doi: 10.1016/0013-4694(92)90133-3
PubMed Abstract | Full Text | CrossRef Full Text | Google Scholar
Pfurtscheller, G., and Lopes da Silva, F. H. (1999). Event-related EEG/MEG synchronization and desynchronization: basic principles. Clin. Neurophysiol. 110, 1842–1857. doi: 10.1016/s1388-2457(99)00141-8
PubMed Abstract | Full Text | CrossRef Full Text | Google Scholar
Pfurtscheller, G., Neuper, C., Brunner, C., and da Silva, F. L. (2005). Beta rebound after different types of motor imagery in man. Neurosci. Lett. 378, 156–159. doi: 10.1016/j.neulet.2004.12.034
PubMed Abstract | Full Text | CrossRef Full Text | Google Scholar
Pfurtscheller, G., Stancák, A. Jr., and Neuper, C. (1996). Event-related synchronization (ERS) in the alpha band-an electrophysiological correlate of cortical idling: a review. Int. J. Psychophysiol. 24, 39–46. doi: 10.1016/s0167-8760(96)00066-9
PubMed Abstract | Full Text | CrossRef Full Text | Google Scholar
Plewnia, C., Rilk, A. J., Soekadar, S. R., Arfeller, C., Huber, H. S., Sauseng, P., et al. (2008). Enhancement of long-range EEG coherence by synchronous bifocal transcranial magnetic stimulation. Eur. J. Neurosci. 27, 1577–1583. doi: 10.1111/j.1460-9568.2008.06124.x
PubMed Abstract | Full Text | CrossRef Full Text | Google Scholar
Pogosyan, A., Gaynor, L. D., Eusebio, A., and Brown, P. (2009). Boosting cortical activity at beta-band frequencies slows movement in humans. Curr. Biol. 19, 1637–1641. doi: 10.1016/j.cub.2009.07.074
PubMed Abstract | Full Text | CrossRef Full Text | Google Scholar
Ragazzoni, A., Pirulli, C., Veniero, D., Feurra, M., Cincotta, M., Giovannelli, F., et al. (2013). Vegetative versus minimally conscious states: a study using TMS-EEG, sensory and event-related potentials. PLoS One 8:e57069. doi: 10.1371/journal.pone.0057069
PubMed Abstract | Full Text | CrossRef Full Text | Google Scholar
Rasch, B., and Born, J. (2013). About sleep’s role in memory. Physiol. Rev. 93, 681–766. doi: 10.1152/physrev.00032.2012
PubMed Abstract | Full Text | CrossRef Full Text | Google Scholar
Ray, N. J., Jenkinson, N., Wang, S., Holland, P., Brittain, J. S., Joint, C., et al. (2008). Local field potential beta activity in the subthalamic nucleus of patients with Parkinson’s disease is associated with improvements in bradykinesia after dopamine and deep brain stimulation. Exp. Neurol. 213, 108–113. doi: 10.1016/j.expneurol.2008.05.008
PubMed Abstract | Full Text | CrossRef Full Text | Google Scholar
Rehme, A. K., Eickhoff, S. B., Wang, L. E., Fink, G. R., and Grefkes, C. (2011). Dynamic causal modeling of cortical activity from the acute to the chronic stage after stroke. Neuroimage 55, 1147–1158. doi: 10.1016/j.neuroimage.2011.01.014
PubMed Abstract | Full Text | CrossRef Full Text | Google Scholar
Rektor, I., Baláz, M., and Bocková, M. (2010). Cognitive event-related potentials and oscillations in the subthalamic nucleus. Neurodegener. Dis. 7, 160–162. doi: 10.1159/000289228
PubMed Abstract | Full Text | CrossRef Full Text | Google Scholar
Rosanova, M., Casali, A., Bellina, V., Resta, F., Mariotti, M., and Massimini, M. (2009). Natural frequencies of human corticothalamic circuits. J. Neurosci. 29, 7679–7685. doi: 10.1523/JNEUROSCI.0445-09.2009
PubMed Abstract | Full Text | CrossRef Full Text | Google Scholar
Saiote, C., Polanía, R., Rosenberger, K., Paulus, W., and Antal, A. (2013). High-frequency TRNS reduces BOLD activity during visuomotor learning. PLoS One 8:e59669. doi: 10.1371/journal.pone.0059669
PubMed Abstract | Full Text | CrossRef Full Text | Google Scholar
Sauseng, P., Feldheim, J. F., Freunberger, R., and Hummel, F. C. (2011). Right prefrontal TMS disrupts interregional anticipatory EEG alpha activity during shifting of visuospatial attention. Front. Psychol. 2:241. doi: 10.3389/fpsyg.2011.00241
PubMed Abstract | Full Text | CrossRef Full Text | Google Scholar
Sauseng, P., Gerloff, C., and Hummel, F. C. (2013). Two brakes are better than one: the neural bases of inhibitory control of motor memory traces. Neuroimage 65, 52–58. doi: 10.1016/j.neuroimage.2012.09.048
PubMed Abstract | Full Text | CrossRef Full Text | Google Scholar
Schoffelen, J.-M., Oostenveld, R., and Fries, P. (2005). Neuronal coherence as a mechanism of effective corticospinal interaction. Science 308, 111–113. doi: 10.1126/science.1107027
PubMed Abstract | Full Text | CrossRef Full Text | Google Scholar
Schulz, R., Gerloff, C., and Hummel, F. C. (2013). Non-invasive brain stimulation in neurological diseases. Neuropharmacology 64, 579–587. doi: 10.1016/j.neuropharm.2012.05.016
PubMed Abstract | Full Text | CrossRef Full Text | Google Scholar
Siebner, H. R., Rossmeier, C., Mentschel, C., Peinemann, A., and Conrad, B. (2000). Short-term motor improvement after sub-threshold 5-Hz repetitive transcranial magnetic stimulation of the primary motor hand area in Parkinson’s disease. J. Neurol. Sci. 178, 91–94. doi: 10.1016/s0022-510x(00)00370-1
PubMed Abstract | Full Text | CrossRef Full Text | Google Scholar
Siebner, H. R., and Ziemann, U. (2010). Rippling the cortex with high-frequency (>100 Hz) alternating current stimulation. J. Physiol. 588(Pt. 24), 4851–4852. doi: 10.1113/jphysiol.2010.200857
PubMed Abstract | Full Text | CrossRef Full Text | Google Scholar
Singer, W., and Gray, C. M. (1995). Visual feature integration and the temporal correlation hypothesis. Annu. Rev. Neurosci. 18, 555–586. doi: 10.1146/annurev.neuro.18.1.555
PubMed Abstract | Full Text | CrossRef Full Text | Google Scholar
Stam, C. J., Nolte, G., and Daffertshofer, A. (2007). Phase lag index: assessment of functional connectivity from multi channel EEG and MEG with diminished bias from common sources. Hum. Brain Mapp. 28, 1178–1193. doi: 10.1002/hbm.20346
PubMed Abstract | Full Text | CrossRef Full Text | Google Scholar
Sun, Y., Farzan, F., Barr, M. S., Kirihara, K., Fitzgerald, P. B., Light, G. A., et al. (2011). γ Oscillations in schizophrenia: mechanisms and clinical significance. Brain Res. 1413, 98–114. doi: 10.1016/j.brainres.2011.06.065
PubMed Abstract | Full Text | CrossRef Full Text | Google Scholar
Tallon-Baudry, C., Bertrand, O., Peronnet, F., and Pernier, J. (1998). Induced gamma-band activity during the delay of a visual short-term memory task in humans. J. Neurosci. 18, 4244–4254.
Tecchio, F., Pasqualetti, P., Zappasodi, F., Tombini, M., Lupoi, D., Vernieri, F., et al. (2007). Outcome prediction in acute monohemispheric stroke via magnetoencephalography. J. Neurol. 254, 296–305. doi: 10.1007/s00415-006-0355-0
PubMed Abstract | Full Text | CrossRef Full Text | Google Scholar
Tecchio, F., Zappasodi, F., Pasqualetti, P., Tombini, M., Caulo, M., Ercolani, M., et al. (2006). Long-term effects of stroke on neuronal rest activity in rolandic cortical areas. J. Neurosci. Res. 83, 1077–1087. doi: 10.1002/jnr.20796
PubMed Abstract | Full Text | CrossRef Full Text | Google Scholar
Tecchio, F., Zappasodi, F., Pasqualetti, P., Tombini, M., Salustri, C., Oliviero, A., et al. (2005). Rhythmic brain activity at rest from rolandic areas in acute mono-hemispheric stroke: a magnetoencephalographic study. Neuroimage 28, 72–83. doi: 10.1016/j.neuroimage.2005.05.051
PubMed Abstract | Full Text | CrossRef Full Text | Google Scholar
Terney, D., Chaieb, L., Moliadze, V., Antal, A., and Paulus, W. (2008). Increasing human brain excitability by transcranial high-frequency random noise stimulation. J. Neurosci. 28, 14147–14155. doi: 10.1523/JNEUROSCI.4248-08.2008
PubMed Abstract | Full Text | CrossRef Full Text | Google Scholar
Thut, G., Veniero, D., Romei, V., Miniussi, C., Schyns, P., and Gross, J. (2011). Rhythmic TMS causes local entrainment of natural oscillatory signatures. Curr. Biol. 21, 1176–1185. doi: 10.1016/j.cub.2011.05.049
PubMed Abstract | Full Text | CrossRef Full Text | Google Scholar
Uhlhaas, P. J., Haenschel, C., Nikolić, D., and Singer, W. (2008). The role of oscillations and synchrony in cortical networks and their putative relevance for the pathophysiology of schizophrenia. Schizophr. Bull. 34, 927–943. doi: 10.1093/schbul/sbn062
PubMed Abstract | Full Text | CrossRef Full Text | Google Scholar
Varela, F., Lachaux, J. P., Rodriguez, E., and Martinerie, J. (2001). The brainweb: phase synchronization and large-scale integration. Nat. Rev. Neurosci. 2, 229–239. doi: 10.1038/35067550
PubMed Abstract | Full Text | CrossRef Full Text | Google Scholar
Veniero, D., Brignani, D., Thut, G., and Miniussi, C. (2011). Alpha-generation as basic response-signature to transcranial magnetic stimulation (TMS) targeting the human resting motor cortex: a TMS/EEG co-registration study. Psychophysiology 48, 1381–1389. doi: 10.1111/j.1469-8986.2011.01218.x
PubMed Abstract | Full Text | CrossRef Full Text | Google Scholar
Vernet, M., Bashir, S., Yoo, W.-K., Perez, J. M., Najib, U., and Pascual-Leone, A. (2013). Insights on the neural basis of motor plasticity induced by theta burst stimulation from TMS-EEG. Eur. J. Neurosci. 37, 598–606. doi: 10.1111/ejn.12069
PubMed Abstract | Full Text | CrossRef Full Text | Google Scholar
von Campenhausen, S., Bornschein, B., Wick, R., Bötzel, K., Sampaio, C., Poewe, W., et al. (2005). Prevalence and incidence of Parkinson’s disease in Europe. Eur. Neuropsychopharmacol. 15, 473–490. doi: 10.1016/j.euroneuro.2005.04.007
PubMed Abstract | Full Text | CrossRef Full Text | Google Scholar
Wang, X. J., and Buzsáki, G. (1996). Gamma oscillation by synaptic inhibition in a hippocampal interneuronal network model. J. Neurosci. 16, 6402–6413.
Ward, N. S., Brown, M. M., Thompson, A. J., and Frackowiak, R. S. J. (2003). Neural correlates of motor recovery after stroke: a longitudinal fMRI study. Brain 126(Pt. 11), 2476–2496. doi: 10.1093/brain/awg245
PubMed Abstract | Full Text | CrossRef Full Text | Google Scholar
Westlake, K. P., Hinkley, L. B., Bucci, M., Guggisberg, A. G., Byl, N., Findlay, A. M., et al. (2012). Resting state α-band functional connectivity and recovery after stroke. Exp. Neurol. 237, 160–169. doi: 10.1016/j.expneurol.2012.06.020
PubMed Abstract | Full Text | CrossRef Full Text | Google Scholar
Williams, E. R., Soteropoulos, D. S., and Baker, S. N. (2010). Spinal interneuron circuits reduce approximately 10-Hz movement discontinuities by phase cancellation. Proc. Natl. Acad. Sci. U S A 107, 11098–11103. doi: 10.1073/pnas.0913373107
PubMed Abstract | Full Text | CrossRef Full Text | Google Scholar
Zaehle, T., Rach, S., and Herrmann, C. S. (2010). Transcranial alternating current stimulation enhances individual alpha activity in human EEG. PLoS One 5:e13766. doi: 10.1371/journal.pone.0013766
PubMed Abstract | Full Text | CrossRef Full Text | Google Scholar
Keywords: non-invasive brain stimulation, oscillatory activity, stroke, Parkinson’s disease, schizophrenia
Citation: Krawinkel LA, Engel AK and Hummel FC (2015) Modulating pathological oscillations by rhythmic non-invasive brain stimulation—a therapeutic concept? Front. Syst. Neurosci. 9:33. doi: 10.3389/fnsys.2015.00033
Received: 23 November 2014; Accepted: 18 February 2015;
Published online: 17 March 2015.
Edited by:
Manuel Fernando Casanova, University of Louisville, USAReviewed by:
Yu Liu, The University of Tennessee Health Science Center, USAEstate M. Sokhadze, University of Louisville, USA
Copyright © 2015 Krawinkel, Engel and Hummel. This is an open-access article distributed under the terms of the Creative Commons Attribution License (CC BY). The use, distribution and reproduction in other forums is permitted, provided the original author(s) or licensor are credited and that the original publication in this journal is cited, in accordance with accepted academic practice. No use, distribution or reproduction is permitted which does not comply with these terms.
*Correspondence: Friedhelm C. Hummel, BrainImaging and NeuroStimulation (BINS) Laboratory, Department of Neurology, University Medical Center Hamburg-Eppendorf, Building O10, Martinistraße 52, 20246 Hamburg, Germany f.hummel@uke.de