- Department of Physiology, Monash University, Clayton, VIC, Australia
The brain's life-long capacity for experience-dependent plasticity allows adaptation to new environments or to changes in the environment, and to changes in internal brain states such as occurs in brain damage. Since the initial discovery by Hebb (1947) that environmental enrichment (EE) was able to confer improvements in cognitive behavior, EE has been investigated as a powerful form of experience-dependent plasticity. Animal studies have shown that exposure to EE results in a number of molecular and morphological alterations, which are thought to underpin changes in neuronal function and ultimately, behavior. These consequences of EE make it ideally suited for investigation into its use as a potential therapy after neurological disorders, such as traumatic brain injury (TBI). In this review, we aim to first briefly discuss the effects of EE on behavior and neuronal function, followed by a review of the underlying molecular and structural changes that account for EE-dependent plasticity in the normal (uninjured) adult brain. We then extend this review to specifically address the role of EE in the treatment of experimental TBI, where we will discuss the demonstrated sensorimotor and cognitive benefits associated with exposure to EE, and their possible mechanisms. Finally, we will explore the use of EE-based rehabilitation in the treatment of human TBI patients, highlighting the remaining questions regarding the effects of EE.
Experience-dependent plasticity encompasses a vast number of paradigms that range from deprivation to alterations and enrichments in the environment, and has been investigated in great detail across development through to adulthood (see reviews by Hubel and Wiesel, 1970; Hubel, 1978; Kaas, 1991; Klintsova and Greenough, 1999; Sur and Leamey, 2001; De Villers-Sidani and Merzenich, 2011; Bengoetxea et al., 2012). For the purposes of the present review, we chose to focus on plasticity conferred by global changes to the environment, termed environmental enrichment (EE). We will focus only on the changes evoked by this manipulation when applied in adulthood as our final aim is to demonstrate that it represents an exciting potential therapy in adult traumatic brain injury (TBI). As we shall review, EE alters neuronal function through a range of morphological and molecular interactions, which lead to alterations in sensorimotor and cognitive behavior. These changes make EE an ideal candidate in the treatment of TBI. To lead to this thesis, we first commence with a review of EE's capacity to evoke plasticity in the uninjured brain, to provide the context in which we will cast the role of EE in TBI. We focus here on EE-induced changes in sensory cortices due to the demonstrated effects of TBI on altering neuronal function in sensory cortices (Hall and Lifshitz, 2010; Ding et al., 2011; Alwis et al., 2012; Johnstone et al., 2013); it is also our view that changes in neuronal activity in sensory cortices after injury must underlie a significant portion of the persistent cognitive deficits found in TBI (Caeyenberghs et al., 2009; Davis and Dean, 2010; Lew et al., 2010; Folmer et al., 2011). The review of EE effects in the normal brain is also necessary to understand the mechanisms whereby EE produces changes in neuronal function in the normal brain, before we can begin to hypothesize about how EE exerts its beneficial effects after brain injury. Finally, we discuss the current literature regarding the use of EE as a potential therapy post-TBI, in animal studies with induced TBI, and in studies of human rehabilitation after injury.
What is EE?
EE refers to an experimental paradigm in which laboratory animals are housed in an environment allowing cognitive, motor and sensory stimulation at levels much greater than those which occur under standard laboratory housing conditions (Hebb, 1947, 1949; Van Praag et al., 2000). Early studies in animals have shown that the enhanced stimulation from EE produces many remarkable benefits at anatomical, molecular and behavioral levels (Hebb, 1947; Bennett et al., 1969; Diamond et al., 1972, 1976; Greenough and Volkmar, 1973; Torasdotter et al., 1998), with numerous studies following on from this work to further characterize the effects of EE (see reviews by Van Praag et al., 2000; Nithianantharajah and Hannan, 2006). In EE, the housing environment is modified by providing a larger enclosure, natural bedding and a variety of novel objects, in the expectation that this will promote greater physical activity in exploration and interaction with a novel and complex environment (Benaroya-Milshtein et al., 2004; Zebunke et al., 2013). Social enrichment in the EE environment, involving housing animals with multiple cagemates to encourage complex social interactions (Rosenzweig et al., 1978; Mesa-Gresa et al., 2013), is also believed to contribute to an enhanced sensorimotor and cognitive experience. Enhanced physical activity and enhanced social interaction each provide benefits to the brain; physical activity on its own improves cognitive performance in parallel with a range of neural changes including enhanced neurogenesis and increased levels of neurotrophic growth factors and increased neurotransmitter subunit expression (Van Praag et al., 1999; Farmer et al., 2004; Erickson et al., 2011), while social enrichment on its own has been shown to result in an increase in brain weight (Rosenzweig et al., 1978). When the two are combined in an appropriately enriched environment, a much more extensive set of cerebral changes occurs (Rosenzweig et al., 1978; Johansson and Ohlsson, 1996; Sozda et al., 2010).
The combination of social, physical and cognitive stimulation is most often used in studies of EE and we term this “generic” EE, wherein the whole environment is non-selectively enriched. However, in some instances, in what we term “specific” EE, enrichment has been targeted to affect a specific system, e.g., auditory-specific enrichment (Engineer et al., 2004; Percaccio et al., 2005, 2007; Jakkamsetti et al., 2012) consisting of components of generic EE in combination with systems designed to produce a variety of salient sounds; or tactile-specific enrichment (Bourgeon et al., 2004; Xerri et al., 2005) where rats were raised in an environment consisting of objects with various textures. Differences in generic and specific EE will be highlighted further, in the context of EE effects on neuronal function in the cortex.
Beneficial Effects of EE on Behavior
EE exposure results in a range of sensorimotor and cognitive benefits in laboratory animals, which we only briefly summarize as these have been well reviewed elsewhere (Van Praag et al., 2000; Nithianantharajah and Hannan, 2006; Simpson and Kelly, 2011). In normal animals, EE significantly improves spatial and non-spatial learning and memory, novel object discrimination, increases the speed of spatial learning and enhances spatial searching strategies (Van Praag et al., 2000; Schrijver et al., 2002; Nithianantharajah and Hannan, 2006; Kulesskaya et al., 2011; Vedovelli et al., 2011; Leger et al., 2012). EE appears to decrease anxiety, as evidenced in a variety of tests (Fernandez-Teruel et al., 2002; Larsson et al., 2002; Galani et al., 2007; Harati et al., 2013). EE also improves task-learning, and recent and remote memory retrieval (Harati et al., 2013), likely due to a greater ability to consolidate and retain information because of social enrichment (Gardner et al., 1975). However, effects are not all positive and studies have shown both increases and decreases in aggressive social behavior after EE (Abou-Ismail, 2011; Workman et al., 2011; McQuaid et al., 2012; Mesa-Gresa et al., 2013), possibly due to factors such as differences in EE housing conditions, strain differences, and experimental design.
In a similar vein, the consensus (Nithianantharajah and Hannan, 2006; Kazlauckas et al., 2011; Landers et al., 2011) is that EE encourages activity and exploratory behavior though there are some inconsistencies: some studies show increased activity in novel environments (Benaroya-Milshtein et al., 2004), and others show faster habituation and less activity (Zimmermann et al., 2001; Schrijver et al., 2002; Elliott and Grunberg, 2005), when compared with animals housed in standard or impoverished environments (Varty et al., 2000; Zimmermann et al., 2001). Recently, Zebunke et al. (2013) showed a decrease in general activity during an open field test, with an increase in duration of exploration of novel objects by pigs exposed to cognitive enrichment. Similarly, Mesa-Gresa et al. (2013) also found that EE rats exhibited longer durations of novel object exploration, while Schrijver et al. (2002) found an increase in activity in a light/dark box in EE rats. Bruel-Jungerman et al. (2005) have also reported that EE animals were capable of retaining memory during a novel object recognition test for up to 48 h after initial exposure, despite a lower object exploration time during the learning phase of the test.
Among the most robust of findings is that EE and sensory training/learning improves stimulus discrimination (Gibson, 1953; Kendrick et al., 1992; Recanzone et al., 1993). Mandairon et al. (2006a,b) have shown that olfactory enrichment results in an improved ability to discriminate between odor pairs, likely due to changes in neuronal response properties (Buonviso and Chaput, 2000; Fletcher and Wilson, 2003). Similarly, EE enhances spatial discrimination of sound source, with faster reaction times and improved discrimination accuracy (Cai et al., 2009). Bourgeon et al. (2004) reported that while EE housing did not affect an animal's tactile ability to discriminate between textured surfaces, enriched animals did learn to perform the discrimination task faster. The changes in behavior reported above must occur as a consequence of the effects of EE on neuronal function, which in turn, occur as a result of the various molecular and morphological changes mediated by EE.
Neuronal Functional Changes Associated with Exposure to EE
The EE-induced changes in behavior can be linked to specific changes in neuronal functionality. This has been studied in best detail for behavior associated with hippocampal function (Van Praag et al., 2000; Eckert and Abraham, 2013) and we briefly describe these as a prelude to describing the changes seen in adult sensory cortices, the particular brain regions of interest here in the context of our over-arching thesis (Alwis et al., 2012) that many persistent cognitive and motor deficits in TBI have sensory deficits as an underlying cause. The studies discussed below have used electrophysiological techniques such as in vivo and in vitro intra and extracellular recordings to specifically investigate EE-induced changes in neuronal function.
EE-induced improvements in hippocampal-dependent memory function have been linked to experience-dependent changes in hippocampal synaptic strength (Kempermann et al., 1997; Schrijver et al., 2002; Vedovelli et al., 2011), with reports of increases in excitatory post-synaptic potential (EPSP) amplitudes and evoked population spikes in rats exposed to generic EE, both in in vivo studies (Sharp et al., 1985; Irvine and Abraham, 2005; Irvine et al., 2006) and in in vitro studies of slices from the dentate gyrus (Green and Greenough, 1986; Foster et al., 1996) or the CA3-CA1 pathway (Foster and Dumas, 2001; Malik and Chattarji, 2012). The enhanced synaptic efficacy in dentate gyrus appears likely to act through AMPA and NMDA receptor mediated mechanisms (Foster et al., 1996). Interestingly, these increases did not outlast the termination of EE housing (Green and Greenough, 1986), even though EE-induced changes in behavior and morphology persist after discontinuation of EE (Camel et al., 1986; Cheng et al., 2012), suggesting that information stored in the dentate gyrus may be related to more transient behavioral effects of EE. However, there is also contradiction in studies of EE-induced long-term hippocampal plasticity. Eckert and Abraham (2010) reported that long-term exposure to EE did not result in enhanced synaptic transmission in the hippocampus, both in vivo and in vitro, suggesting that the variability in these studies may have be due to different EE paradigms, or to homeostatic mechanisms to re-establish normal synaptic transmission (Turrigiano, 1999, 2008). Foster and colleagues (Foster et al., 1996; Foster and Dumas, 2001) demonstrated that EE housing inhibits LTP induction in the perforant pathway, suggesting that both experience-dependent synaptic plasticity and LTP expression share similar yet-unknown underlying mechanisms. Conversely, increased LTP expression has been reported after EE exposure (Duffy et al., 2001; Artola et al., 2006; Eckert and Abraham, 2010; Malik and Chattarji, 2012). One resolution for these effects, other than differences in the EE conditions, is that LTP induction after EE may be differentially regulated in different regions of the hippocampus.
It is worth noting here that short-term plasticity in the hippocampus has not been shown to be affected by EE (Foster et al., 1996; Foster and Dumas, 2001; Eckert and Abraham, 2010; Malik and Chattarji, 2012).
In contrast to the hippocampus, little is known about EE-induced changes in neuronal functionality in cortex. What changes there are in cortical neuronal function have mainly been examined at the level of the sensory cortices and we discuss these studies in detail below. EE-induced changes in neuronal function in the normal (uninjured) sensory cortices are particularly salient to our thesis and may provide us with insights into the role of EE on neuronal function after brain injury, of which nothing is known as yet.
EE and Sensory Cortices
The effects of EE have been studied most extensively in auditory cortex, in some detail in somatosensory cortex, and only to a limited degree in visual cortex.
In auditory cortex, the effect of EE has been studied at levels ranging from brain slices through to extracellular recordings from neurons in anaesthetized animals. In the investigation of the effects of EE on the auditory cortex, studies have used enriched environments that include specific auditory enrichment in the form of playback of various sounds within the housing environment (Engineer et al., 2004; Percaccio et al., 2005, 2007; Nichols et al., 2007). Many studies report effects that mirror those seen in the hippocampus, of increased synaptic efficiency. Thus, auditory cortex slices show that specific EE induces an increase in excitatory post-synaptic current (EPSC) amplitudes, coupled with a decrease in current rise-times in supragranular cortical layers and no changes in infragranular layer V (Nichols et al., 2007). In vivo recordings from the anaesthetized rat, primarily from Layers 4/5 of adult primary auditory cortex after specific auditory EE, have demonstrated an increase in cortical responsiveness (both spontaneous and stimulus-evoked), decreased response latencies, and an increase in frequency selectivity (Engineer et al., 2004; Percaccio et al., 2005; Cai et al., 2009). Percaccio et al. (2005, 2007) also found that EE increased paired pulse depression (PPD) in the rat auditory cortex, indicating an increased probability of synaptic transmitter release and thus, enhanced synaptic transmission. Other studies in auditory cortex found EE could cause reorganization of the cortical tonotopic map (Norena et al., 2006; Pienkowski and Eggermont, 2009; Zhou et al., 2011; Kim and Bao, 2013), and alterations in stimulus frequency selectivity over either a range of frequencies (Zhou et al., 2011) or for frequencies specific to those used as a part of the enrichment condition (Norena et al., 2006; Pienkowski and Eggermont, 2009).
The effects of auditory enrichment are not restricted to primary auditory cortex, and Jakkamsetti et al. (2012) have reported that responses in posterior auditory field (PAF) are also increased when compared with animals housed in standard environments. These increased firing rates were accompanied by decreases in response latency and duration, and a reduction in receptive field size, as seen in primary auditory cortex (Engineer et al., 2004; Zhou et al., 2011; Jakkamsetti et al., 2012).
Unlike the above-noted reports, some studies do not report increased neuronal responsiveness and sharper frequency tuning after exposure to auditory enrichment (Condon and Weinberger, 1991; Bao et al., 2003). Instead, these studies found that a repeated auditory stimulus decreased responsiveness to frequencies used in the stimulus (Condon and Weinberger, 1991), and exposure to noise burst trains produced broadly tuned receptive fields (Bao et al., 2003). Percaccio et al. (2007) have suggested that a critical variable in eliciting EE effects in auditory cortex is the nature of the enrichment, i.e., how engaging or complex the stimuli are. This would explain the increased neuronal responsiveness reported by Percaccio et al. (2007) in rats receiving even passive exposure to specific auditory EE, which included situation-dependent stimuli from the environment and from cagemates, as opposed to simple, less behaviorally relevant stimuli.
Similar to studies in auditory cortex, generic EE (i.e., non-specific enrichment) results in reorganized cortical topographic maps, decreased receptive field sizes, increased response selectivity and increased sensory evoked potentials in the somatosensory cortex (Xerri et al., 1996; Coq and Xerri, 1998; Polley et al., 2004; Devonshire et al., 2010). One interesting effect demonstrated here is that EE effects on receptive field sizes and responses to stimulation of the main topographic input to the neurons may be laminar selective (an effect that does not appear to have been explored in auditory cortex). Thus, in the rodent barrel cortex that receives tactile input from the mystacial whiskers, EE caused a decrease in receptive field size and in neuronal responses evoked by stimulation of the “Principal Whisker” (the topographically matched whisker providing the main input to a group of neurons in the barrel cortex) in supragranular cortical Layers 2/3, but there were no changes in response strength or receptive field size in input Layer 4 (Polley et al., 2004). It is worth noting that Guic et al. (2008) found that EE caused an increase in cortical representational area in Layer 4. However, these effects were seen after stimulation of only a few selective whiskers, while other whiskers were trimmed whereas Polley et al. (2004) used a non-deprived paradigm where all whiskers remained untrimmed. These different effects are likely a reflection of variations in experimental design of the EE conditions, consistent with the conclusions drawn from studies in auditory cortex that the nature of EE conditions influences neuronal outcomes.
These studies, where the emphasis was on measuring receptive field sizes and responses only to simple input from the main topographically-matched region of the body, indicated laminar specificity of effects. However, when neuronal encoding of sophisticated sensory input is the metric, the effects of generic EE occur globally across all cortical layers. Thus, in our own studies, 8–10 weeks of EE exposure increased neuronal firing rates globally across all layers 2–5 of the rat barrel cortex, and did so in response to both simple stimuli and a variety of complex, naturalistic stimuli (Alwis and Rajan, 2013). It is interesting to note that these effects occur even to the complex stimuli as our previous work on TBI (Alwis et al., 2012) had suggested that the complex stimuli may engage a diversity of intra-cortical processing mechanisms not seen with the simple stimuli. These effects occurred without any change in response latency, suggesting that the effects were specific to cortex and not due to changes at lower levels of the somatic pathways to cortex.
Although not often studied on its own in somatosensory cortex, recently EE has been combined with another manipulation that induces experience-dependent plasticity in barrel cortex, viz. whisker trimming and/or stimulation (Armstrong-James et al., 1992; Diamond et al., 1993, 1994; Rema et al., 2006; Guic et al., 2008; Megevand et al., 2009). Here the picture is rather murky, with one study suggesting that EE operates through different mechanisms than other plasticity mechanisms in barrel cortex, but another suggesting that it operates through the same mechanisms. The first seems to apply in the case of whisker trimming: when whisker pairing (all whiskers on one side of the face trimmed except for a pair of adjacent whiskers) is coupled with short (15 h) generic EE exposure, there is an accentuation of the effects induced by whisker trimming alone: a faster shift of receptive field bias toward the untrimmed whiskers, stronger evoked responses to the intact paired whisker than to deprived whiskers, and increased spontaneous activity in supra-granular and granular layers (Rema et al., 2006). In contrast EE may operate through the same mechanisms as some other plasticity cases. Thus, a short duration of whisker stimulation at a frequency often used during exploratory whisking behavior increases stimulus evoked potentials in both supra-granular and granular barrel cortex layers (Megevand et al., 2009)—but, addition of EE to the whisker stimulation paradigm does not further potentiate responses (Megevand et al., 2009).
Finally, only a limited number of studies have examined the effects of EE in the normal visual cortex. In area 17 of the adult visual cortex, similar to effects seen in the auditory cortex, generic EE results in sharper bandwidths in orientation tuned cells, increased neuronal responses to light stimuli, increased visual acuity, as well as increased stimulus contrast and temporal selectivity (Beaulieu and Cynader, 1990a,b; Mainardi et al., 2010). In addition to these effects in normal adult visual cortex, studies of EE-induced plasticity in the adult visual cortex have also examined effects in the context of monocular deprivation (MD) and amblyopia. MD during developmentally critical periods induces a shift in ocular dominance (OD) so that more neurons respond to stimulation of the open eye (Frenkel and Bear, 2004; Mrsic-Flogel et al., 2007). Such plasticity is normally not seen when MD is started in adulthood, but EE housing for 3 weeks re-activates cortical plasticity in supragranular layers of adult visual cortex such that OD changes are possible again and visual evoked potentials (VEPs) elicited by stimulation of the deprived eye are greatly depressed (Baroncelli et al., 2010b). In amblyopia, individual components of EE such as motor and visual stimulation, as well as the combination of these components, also recover visual acuity and restore OD plasticity and binocularity in supragranular layers of adult visual cortex (Sale et al., 2007; Baroncelli et al., 2012; Tognini et al., 2012).
Taken together, these studies of EE effects in sensory cortices show that generic EE is potent at producing many changes in neuronal responses, such as stronger responses and greater stimulus selectivity; that the receptive field effects may be laminar-selective and depend on the type of enrichment but that effects on more sophisticated neuronal processing, particularly of naturalistic stimuli that mimic everyday events, occur across all cortical laminae; and finally, that EE may operate independent of some other mechanisms of cortical plasticity.
Mechanisms Underlying EE-Induced Changes in Neuronal Function
There are numerous well-documented structural and biochemical consequences of EE which may underlie the effects of EE on neuronal function. We broadly review these changes with EE (summarized in Figure 1). It must be noted that in most cases, we do not know how these structural and molecular changes contribute to EE-induced changes in neuronal function: to date, there has been very limited attempt only to directly manipulate these fine-scale changes to determine to what extent they cause EE-related changes in neuronal functionality.
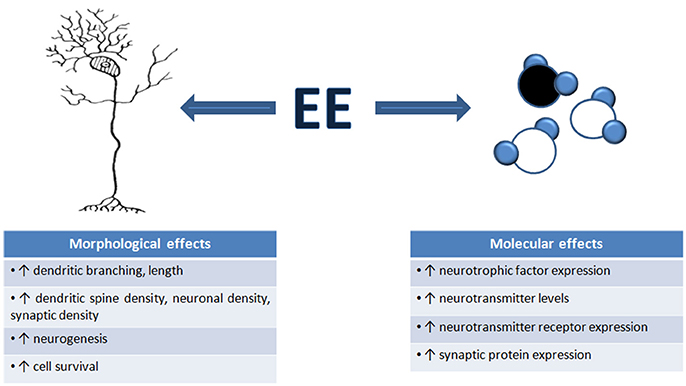
Figure 1. Environmental enrichment induces morphological and molecular changes in the brain. An overview of the number of structural and molecular mechanisms that contribute to the changes in neuronal function, and ultimately, changes in behavior, seen after EE exposure. These mechanisms are thought to underlie EE-induced neural plasticity.
Morphological Changes
It was very early recognized that exposure to complex, enriched environments causes gross morphological changes in an overall increase in brain weight, particularly in cortical and hippocampal weight and thickness (Bennett et al., 1969; Walsh et al., 1969; Diamond et al., 1972, 1976). The factors contributing to these gross morphological changes include increased neuronal density and size, increased dendritic branching and length, increased dendritic spine density, and increased turnover in pyramidal and stellate cells (Holloway, 1966; Diamond et al., 1967; Volkmar and Greenough, 1972; Globus et al., 1973; Greenough and Volkmar, 1973; Greenough et al., 1973; Uylings et al., 1978; Connor et al., 1982; Turner and Greenough, 1985; Kempermann et al., 1997; Leggio et al., 2005; Jung and Herms, 2012). Unsurprisingly, the changes in dendritic morphology are accompanied by synaptic alterations, with EE resulting in increased numbers of synapses and synaptic contacts (Jones et al., 1997; Briones et al., 2004; Landers et al., 2011), which could enhance cortical synaptic transmission and hence, alter cortical excitation/inhibition balances.
One particularly notable EE-induced change is neurogenesis, which may contribute to enhanced cognitive performance (Kempermann et al., 1997, 1998; Nilsson et al., 1999; Bruel-Jungerman et al., 2005). Cell proliferation, improved neuronal survival and functional integration of new neurons have all been demonstrated to occur in the adult dentate gyrus after EE (Kempermann et al., 1997, 1998; Van Praag et al., 2000; Lu et al., 2003; Bruel-Jungerman et al., 2005) and pharmacological inhibition of cell proliferation during EE prevented hippocampal neurogenesis and any improvement in a hippocampal-based memory task (Bruel-Jungerman et al., 2005). Decreased neurogenesis has been linked to cognitive decline (Drapeau et al., 2003, 2007), and restoration of neuronal proliferation and survival leads to an improvement in cognitive behavior (Kempermann, 2002).
The enhanced hippocampal neurogenesis, improved neuronal cell survival, increased synaptic density and dendritic branching (Kempermann et al., 1997, 1998; Bruel-Jungerman et al., 2005), and the growth factor up-regulation that is discussed below, have all been suggested to be responsible for EE-induced improvements in spatial and non-spatial learning and memory and enhanced spatial searching strategies (Van Praag et al., 2000; Schrijver et al., 2002; Nithianantharajah and Hannan, 2006; Kulesskaya et al., 2011; Vedovelli et al., 2011; Leger et al., 2012). Increased habituation to novel objects has also been attributed to a decrease in activation of hippocampal neurons during novel object exposure in enriched animals, in contrast to the increased activation seen in animals housed in standard conditions when exposed to objects in novel environments (Zhu et al., 1997; Leger et al., 2012). These results support the idea that EE-induced morphological changes contribute to alterations in behavior, through changes in overall neuronal function.
It must be noted here that similar neurogenesis has not been demonstrated to occur in cortex after EE, but this may be for a want of study not for an absence of the effect. In the absence of this effect, it is difficult to speculate to what extent neurogenesis contributes to EE-induced changes in cortical neuronal functionality or cortex-based processes. We will argue below that, in any case, neurogenesis is not required to occur in cortex to produce the EE-induced changes in responses and in functionality and that those changes can be produced by alterations in the balance between excitation/inhibition interplay that shapes neuronal responses and function.
Molecular Changes
The EE-induced structural and functional changes described above occur through molecular cascades that involve increases in neurotrophic factor and neurotransmitter levels (Van Praag et al., 2000; Mohammed et al., 2002; Will et al., 2004; Nithianantharajah and Hannan, 2006), and increased expression of regulatory proteins that enhance the number and stability of synapses, increase cell proliferation, and promote neurotransmitter release (Rampon et al., 2000; Frick and Fernandez, 2003; Nithianantharajah et al., 2004).
Neurotrophic factors of particular importance to EE include brain derived neurotrophic factor (BDNF) and nerve growth factor (NGF), with levels of both increasing following exposure to exercise and EE (Pham et al., 1999; Birch et al., 2013) in brain regions including cortex, hippocampus and cerebellum (Torasdotter et al., 1998; Angelucci et al., 2009). In the adult brain, BDNF and NGF promote experience-dependent plasticity by enhancing synaptic plasticity, signaling, learning and memory (Kang and Schuman, 1995; Torasdotter et al., 1998; Pham et al., 1999; Bekinschtein et al., 2011). Increases in neurotrophins appear to underlie improved motor and cognitive function after EE (Falkenberg et al., 1992; Henriksson et al., 1992; Linnarsson et al., 1997; Bekinschtein et al., 2011; Gelfo et al., 2011; Bechara and Kelly, 2013; Birch et al., 2013) since suppression of BDNF levels causes deficits in neurogenesis, learning behavior and memory (Linnarsson et al., 1997; Minichiello et al., 1999; Rossi et al., 2006; Heldt et al., 2007; Furini et al., 2010).
Similarly, neurotransmitter levels are also affected by exposure to EE, suggesting a role in the mediation of brain plasticity. Serotonin is important in promoting neuroplasticity (Maya Vetencourt et al., 2008; Baroncelli et al., 2010a), and EE induces an increase in serotonin receptor expression and in serotonin levels (Rasmuson et al., 1998; Koh et al., 2007; Baroncelli et al., 2010a). Levels of other neurotransmitters associated with synaptic plasticity, such as acetylcholine and noradrenaline, also increase following EE (Por et al., 1982; Galani et al., 2007; Brenes et al., 2009). Increased cortical responsiveness may then be attributed to increases in synaptic transmission efficacy and synaptic strength (Mainardi et al., 2010) brought about by EE-induced molecular changes.
In conjunction with these changes in neurotransmitter levels, excitatory activity also shifts following EE housing, with increases in hippocampal extracellular glutamate levels coupled with an enhanced expression of AMPA and NMDA receptors (Tang et al., 2001; Naka et al., 2005; Segovia et al., 2006). Changes in hippocampal field potentials have been attributed to factors that include increased AMPA receptor-mediated transmitter binding, increased expression of AMPA and NMDA receptor subunits, increased dendritic spine density and upregulation of growth factors (Sharp et al., 1985; Green and Greenough, 1986; Foster and Dumas, 2001; Eckert and Abraham, 2010). There is also evidence of synaptic plasticity, in the form of increased dentate gyrus LTP, in the hippocampus after physical activity, which is an important component of the EE experience (Van Praag et al., 1999). The role of these changes in excitation will be discussed in greater detail below where we argue that a principal mechanism through which EE alters brain function and behavior is by promoting a shift toward excitation in neuronal responses.
Role of Changes in Cortical Excitation/Inhibition Balance in EE-Induced Changes in Neuronal Functionality
As shown above, EE-induced brain plasticity is likely to occur through the combination of structural and biochemical changes that can impact on neuronal functionality. We believe that there is substantive evidence that one particularly important end-effect through which EE alters neuronal function is alterations in the balance between excitation and inhibition in cortex (Engineer et al., 2004; Percaccio et al., 2005, 2007). This E/I balance is a critical factor in regulating cortical neuronal functionality and critical periods of cortical plasticity which occur throughout development are governed by this E/I balance (Hensch and Fagiolini, 2005; Levelt and Hubener, 2012) whereby immaturity of cortical inhibition promotes plasticity while maturation of inhibitory circuits results in the decrease in plasticity associated with cortical maturation (Huang et al., 1999; Fagiolini and Hensch, 2000). Shifts in this E/I balance may also play a major role in the experience-dependent cortical plasticity, which includes plasticity induced by EE or deprivation, that occurs outside developmental critical periods (Hensch and Fagiolini, 2005; Sale et al., 2007; Benali et al., 2008; Maya Vetencourt et al., 2008; Megevand et al., 2009; Baroncelli et al., 2010b, 2011; Luz and Shamir, 2012; Maya-Vetencourt et al., 2012). Changes in neuronal function in the adult brain suggest that EE exposure causes a reactivation of forms of neuronal plasticity generally seen only in the developing, immature brain (Chang and Merzenich, 2003; Chang et al., 2005) in which inhibitory mechanisms are immature.
Experience-dependent changes in response strength and sensitivity in adult sensory cortices have been attributed to decreased levels of cortical inhibition (Buonomano and Merzenich, 1998; Baroncelli et al., 2011), which shift cortical E/I ratios to favor excitation. Studies have demonstrated the importance of GABA synthesis in promoting plasticity (Hensch et al., 1998; Harauzov et al., 2010) after MD, and disruption of GABA-ergic inhibition through pharmacological treatments or EE reinstates plasticity to restore OD plasticity in adult visual cortex (Sale et al., 2007; Maya Vetencourt et al., 2008; Harauzov et al., 2010; Zhou et al., 2011; Maya-Vetencourt et al., 2012). Similarly, Zhou et al. (2011) found that EE-induced plasticity in auditory cortex was accompanied by a decrease in GABA receptor subunit expression. Sale et al. (2007) have also shown that EE exposure results in a decrease in basal extracellular GABA levels in the visual cortex, and that EE-induced plasticity can be countered by pharmacologically increasing inhibitory activity. EE-induced decreases in cortical inhibition have been demonstrated in studies of auditory and visual cortex, through decreases in GABA receptor subunit expression, inhibitory synapse density and basal levels of GABA (Beaulieu and Colonnier, 1987; Zhou et al., 2011; Jakkamsetti et al., 2012), while GAD67 expression has also been shown to decrease following exposure to EE (Scali et al., 2012; Tognini et al., 2012).
However, decreased inhibition may just be one mechanism underlying EE-induced plasticity via shifts in the excitation/inhibition balance, with studies also suggesting an increase in cortical excitation with EE. Nichols et al. (2007) have shown that exposure to EE induces an AMPA-receptor mediated increase in EPSC amplitudes in supragranular layers of the auditory cortex, with no changes in GABA-ergic transmission, while the increase in PPD after EE demonstrated by Percaccio et al. (2005) suggests an increase in the transmitter release probability of excitatory synapses.
Taken together, the results presented in this section suggest that EE exerts its effects through molecular changes, which in turn support changes in morphology and neuronal function. These results also suggest that in conditions such as brain injury, which result in abnormal neuronal activity, EE-induced plasticity may have the potential as a therapy to steer neuronal activity toward a more functionally relevant state. This is particularly the case when it is considered that excitation/inhibition shifts may also occur in brain injury (Ding et al., 2008; Alwis et al., 2012; Johnstone et al., 2013).
EE and the Damaged Brain
Considering that the brain plasticity evoked by EE results in various behavioral benefits, it is hardly surprising that EE (and specifically the form we have termed “generic” EE) has been proposed as a putative therapy for neurological conditions ranging from Alzheimer's disease through to ischemia/stroke (Faherty et al., 2005; Jadavji et al., 2006; Buchhold et al., 2007; Nithianantharajah et al., 2008; Wang et al., 2008; Hu et al., 2010; Valero et al., 2011; Du et al., 2012). Indeed, EE ameliorates the behavioral and pathological deficits associated with many of these conditions: for example, in models of Alzheimer's disease, EE enhances neuronal proliferation, survival and maturation, leading to improved cognition (Hu et al., 2010; Valero et al., 2011), while in studies of stroke/ischemia, EE improves sensorimotor function, such as impaired gait and limb placement (Buchhold et al., 2007; Wang et al., 2008).
Our focus in this review is on the potential role of EE as a therapy for TBI, based on its known effects on brain changes induced by TBI, especially in the sensory cortices. For this consideration, it is necessary to first define some of the basic features of TBI and its effects on brain and behavior. There are two major forms of TBI—focal and diffuse. Focal brain injury is caused by direct, localized damage to the brain, while diffuse injury is most commonly caused by indirect forces, such as during rapid acceleration/deceleration of the head (Andriessen et al., 2010; Alwis et al., 2013). TBI affects approximately 2 million individuals every year in the US alone (Faul et al., 2010) and has been shown to result in a number of persistent sensory deficits, which are thought to underlie TBI-associated cognitive disabilities (Caeyenberghs et al., 2009; Davis and Dean, 2010; Lew et al., 2010; Folmer et al., 2011). People with mild to moderate diffuse TBI usually recover motor skills fully, but have other prolonged deficits, including cognitive deficits and memory loss, likely from axonal injury (Strich, 1956; Adams et al., 1999; Graham et al., 2000; Little et al., 2010).
In TBI there are often substantial and prolonged functional deficits in cognition, memory and movement (Gagnon et al., 1998; Draper and Ponsford, 2008; Park et al., 2008; Faul et al., 2010; Risdall and Menon, 2011) and these are invariably viewed as resulting from damage to brain areas specific to those functions. What has been consistently overlooked is that most TBI sufferers show deficits in how they process sensory information. What we see, hear, touch is used to understand the world and guide complex behaviors like thinking, movement, or memory; sensory processing deficits easily affect these behaviors. It has been recognized that at least some impairments may involve disruption of the integration of sensory input (Brosseau-Lachaine et al., 2008; Patel et al., 2011). In humans, speeded motor tasks and response time tasks are also affected in mild/moderate TBI (Bawden et al., 1985; Haaland et al., 1994), and animal studies have shown persistent abnormal sensory behavior (McNamara et al., 2010), again suggesting disturbances in sensorimotor processing, and there are many long-lasting sensory and cognitive impairments even after motor function has recovered (Narayan et al., 2002; Draper and Ponsford, 2009; Faul et al., 2010; Risdall and Menon, 2011).
Consistent with this hypothesis of a sensory cortices basis for persistent cognitive, memory and motor deficits in TBI, experimental TBI causes significant time-dependent changes in neuronal excitability in sensory cortices (Hall and Lifshitz, 2010; Ding et al., 2011; Alwis et al., 2012). In the immediate post-TBI period, changes in neuronal activity occur across all cortical layers, and consist in a depth-dependent (from the cortical surface) suppression of responses to all types of simple and complex naturalistic stimuli (Johnstone et al., 2013; Yan et al., 2013). However, by the long-term (8–10 weeks post-TBI), effects are found only in the upper cortical layers, layers 2 and upper layer 3, and decrease with cortical depth such that there are no long-term changes in input layer 4 (Alwis et al., 2012); further the changes in the upper layers are no longer a suppression of responses but, rather, a hyper-excitation (Alwis et al., 2012). These persistent effects are consistent with an imbalance in cortical excitation/inhibition (Ding et al., 2011; Alwis et al., 2012).
Given the known effects of EE on the E/I balance in cortex, we believe that there is potential for EE to remediate TBI-induced changes in neuronal function by restoring the cortical excitation/inhibition balance, to improve sensorimotor and cognitive behavior. However, there is no current literature probing the effects of EE specifically on neuronal function post-TBI, highlighting the need for studies examining the mechanisms underlying the EE-induced functional improvements that have been reported in the literature. Hence, in the following section of the review, we will first consolidate and discuss studies that have examined the effects of EE after experimental brain injury, which have focussed on mainly behavioral effects. We will then discuss possible mechanisms through which EE acts to improve functional outcomes post-injury, based on the known mechanisms underlying the effects of EE (as discussed above). Finally, we will review the implementation and efficacy of EE as a therapeutic option to remediate brain injury in a clinical setting.
The Beneficial Effects of EE Post-TBI
Only a few studies have investigated the effects of EE on functional recovery post-experimental TBI and shown that exposure to EE ameliorates motor and cognitive deficits and TBI-induced histopathologies (Hamm et al., 1996; Passineau et al., 2001; Hicks et al., 2002; Sozda et al., 2010; De Witt et al., 2011; Matter et al., 2011; Monaco et al., 2013; Bondi et al., 2014), with some very limited work on the effect of EE on TBI-induced sensory morbidities. These studies have predominantly studied the use of generic EE in the treatment of TBI, except for some work that has included the use of multi-modal sensory stimulation with generic EE (discussed further below).
EE improves TBI-induced cellular histopathologies: EE-treated animals show decreases in lesion volume, increased neuronal survival, and reduced neuronal degeneration in cortex (Passineau et al., 2001; Lippert-Gruner et al., 2007; Monaco et al., 2013). Muthuraju et al. (2012) recently reported an EE-mediated decrease in cell death, in conjunction with an increase in neurogenesis in the striatum, post-TBI. Additionally, in correlation with the positive effects on motor and cognitive function, decreased apoptosis and lesion volume have also been shown using a combination of multi-modal sensory stimulation and EE (Maegele et al., 2005a; Lippert-Gruner et al., 2007). These effects suggest that EE may be able to ameliorate or attenuate damage caused by secondary injury processes, which are often complex and dynamic in nature.
The effects of EE on motor and cognitive function after TBI have only been investigated in TBI models that cause a mixture of focal and diffuse TBI (Hamm et al., 1996; Passineau et al., 2001; Hicks et al., 2002; Hoffman et al., 2008; Sozda et al., 2010; De Witt et al., 2011; Matter et al., 2011; Monaco et al., 2013), and to date, no studies have examined the effect of EE after a purely diffuse model of TBI. The studies of EE effects in mixed model TBI have demonstrated positive effects of EE on neuromotor and cognitive function, especially for spatial navigation and memory. Hamm et al. (1996) found that at 11–15 days after mixed-model TBI, animals exposed to generic EE displayed elevated spatial memory function in the Morris Water Maze (MWM) test, when compared with TBI animals housed in standard conditions. EE housing also improved cognitive functioning to levels comparable to those of sham controls (Hamm et al., 1996). These findings are similar to reports demonstrating EE-induced recovery of spatial navigation and spatial memory after hippocampal/cortical lesions (Will et al., 1977; Einon et al., 1980; Whishaw et al., 1984). EE-induced improvements in spatial navigation, increases in spatial acquisition task rate, as well as improved spatial memory, have been demonstrated in other experimental models of mixed-model TBI (Passineau et al., 2001; Hicks et al., 2002; Wagner et al., 2002; De Witt et al., 2011; Matter et al., 2011; Monaco et al., 2013). EE-mediated recovery of locomotor activity and motor function in beam-walking and rotatod tasks has also been documented post-TBI (Wagner et al., 2002; De Witt et al., 2011; Matter et al., 2011; Monaco et al., 2013), while EE exposure also improved recovery time of forelimb function after CCI (controlled cortical impact) injury (Smith et al., 2007).
While EE on its own has all of these benefits for motor and cognitive function, there is also evidence that the use of additional multi-modal sensorimotor stimulation together with EE can improve cognitive and motor function (Maegele et al., 2005b; Lippert-Gruener et al., 2007; Lippert-Gruner et al., 2007, 2011) at both acute (7 and 15d; Maegele et al., 2005a,b; Lippert-Gruner et al., 2007), and chronic (30d; Lippert-Gruener et al., 2007) time-points post-injury. Such enhanced stimulation is thought to better mimic rehabilitation paradigms used in clinical settings in the treatment of brain injury. Maegele et al. (2005a) have demonstrated that enhanced sensory stimulation in combination with EE improves behavioral outcomes more than the use of EE on its own (Maegele et al., 2005a), suggesting that any neuroplasticity conferred by increased stimulation may be therapeutically relevant.
Only a very few studies have examined the efficacy of EE in ameliorating sensory deficits after TBI, with only one study (Johnson et al., 2013) reporting that EE exposure completely recovers TBI-induced sensory neglect, a condition where there is a reduction in responsiveness to sensorimotor stimuli (Kim et al., 1999). Conversely, in a unilateral cortical lesion model of brain injury, Rose et al. (1987) found that EE did not facilitate recovery from sensory neglect post-lesion. These contradictory results may be explained by differences in the nature of injury, or even the timing of EE exposure: in the Johnson et al. (2013) study, 15 days of EE exposure occurred immediately prior to TBI, whilst Rose et al. (1987) examined the effects of 6 weeks of EE exposure commencing 10–12 days post-lesion. We will demonstrate below that the timing of the application of EE is absolutely critical for ameliorating TBI-induced behavior deficits.
In most of these studies, the type of EE applied has been what we have termed “generic” EE. Whether fortuitous or planned, this form of EE, which must engage a range of sensory, motor, cognitive and social behaviors, appears to greatly improve recovery post-TBI. Thus, Hoffman et al. (2008) indicate that EE-induced functional recovery may depend on task-specific experience: post-TBI, animals show enhanced recovery of motor function (e.g., beam traversing and balancing) and spatial learning and memory (decreased latency to locate a platform in the MWM), when exposed to both EE and task-specific training for the motor and cognitive tests. They also suggest that the enhanced motor, social and cognitive stimulation provided by housing in EE conditions could, in themselves, contribute to the improved motor and cognitive they observed. It is possible that exposure to general EE vs. specific EE dictates the level of functionality that is conferred; although it is true that often studies using specific EE focus on tasks related to the aspect of EE that they enhance. Considering the ambiguity that remains concerning this issue, further investigations are required to determine whether exposure to task-specific experience or specific EE can improve overall functionality in a range of tasks.
It has been suggested that improved sensorimotor function after brain injury may actually be attributed to behavioral compensation rather than functional recovery; improvements on multi-sensory tasks, such as a MWM test, could be due to the use of cues from alternate (presumably undamaged) modalities (Finger, 1978; Rose et al., 1987, 1993; Kolb et al., 1996). This view receives support from studies that show that EE has much more limited or negligible benefit in tasks involving a single sensory modality (Rose et al., 1987, 1988). However, there is also no reason why the two effects could not o-exist and in keeping with this possibility, studies have shown a degree of EE-induced functional recovery after cortical injury, accompanied by an observed difference in the movements of the animals during task performance such as skilled reaching post-injury (Whishaw et al., 1991; Rowntree and Kolb, 1997; Kolb, 1999), suggesting that perhaps both recovery and compensation may account for improved functional outcomes after injury, possibly through the recruitment of uninjured cortical circuits (Kolb, 1999). We therefore turn now to a consideration of the potential mechanisms whereby EE could improve outcomes after TBI.
Mechanisms Promoting Improved Behavioral and Pathological Recovery
To understand how EE could promote recovery after TBI, it is necessary to understand some of the mechanisms underlying TBI. This is not a simple endeavor since neurodegeneration caused by TBI occurs as a result of a number of complex and dynamic inter-related mechanisms (Smith et al., 1991; Hicks et al., 1993; Pierce et al., 1998; Hall and Lifshitz, 2010; McNamara et al., 2010). Only a few studies have examined how EE impacts on these complex molecular and anatomical factors affected in TBI or any other form of brain injury. Thus, ideas of how EE might produce improvements in sensorimotor and cognitive behavior after TBI or any brain injury are often based on extrapolations of the known actions of EE in the normal brain.
In uninjured animals, EE enhances neurogenesis, improves neuronal survival, and decreases apoptotic cell death (Kempermann et al., 1997; Van Praag et al., 2000; Lu et al., 2003; Bruel-Jungerman et al., 2005), all of which have been linked to improved behavioral recovery after TBI (Passineau et al., 2001; Gaulke et al., 2005; Sozda et al., 2010; Monaco et al., 2013). Additionally, Miller et al. (2013) have also recently demonstrated a decrease in hippocampal volume loss in TBI patients, with increased engagement with cognitively, physically and socially demanding activities, supporting the role of EE in directly preventing neuronal death.
Post-injury EE housing has been shown to decrease injury-induced lesion volume, increase synaptic density, increase post-synaptic density (PSD) thickness, increase dendritic spine density and dendritic branching in supragranular and infragranular layers of injured cortex (Biernaskie and Corbett, 2001; Ip et al., 2002; Johansson and Belichenko, 2002; Xu et al., 2009a,b). Such structural changes may lead to EE-enabled compensation and recovery of function after injury (Rose et al., 1987; Kolb and Gibb, 1991; Whishaw et al., 1991; Passineau et al., 2001; Will et al., 2004). Under uninjured conditions, EE-induced structural changes are thought to occur due to upregulation of trophic factors such as VEGF and BDNF which promote cell survival and plasticity, and an increase in activation of transcription factors of proteins mediating plasticity (Young et al., 1999; Rampon et al., 2000; Keyvani et al., 2004; Will et al., 2004; Gaulke et al., 2005; Hoffman et al., 2008; Sozda et al., 2010; Monaco et al., 2013; Ortuzar et al., 2013). Interestingly, studies have reported an increase in BDNF expression after TBI (Hicks et al., 1997; Chen et al., 2005), with no further increase following exposure to EE (Chen et al., 2005) suggesting that EE-induced benefits for recovery after TBI may not depend on increasing the levels of trophic factors.
The effects of TBI may also be exerted through inflammatory processes: as we have noted previously (Alwis et al., 2013), activation of inflammatory cascades as part of the normal cellular response to injury can cause further injury to the already damaged brain (Menge et al., 2001; Morganti-Kossmann et al., 2002). The inflammatory response in TBI involves production of pro-inflammatory cytokines like interleukin-1 (IL-1), IL-6 and tumor necrosis factor (TNF-a), and anti-inflammatory cytokines such as IL-10 and IL-12, all of which are seen in the cerebrospinal fluid of TBI patients within a few hours of the primary injury. Inflammatory cytokines IL-1a, IL-1b, and IL-18 are also increased after TBI (Menge et al., 2001; Morganti-Kossmann et al., 2002). Interestingly, EE is able to decrease levels of pro-inflammatory molecules such as tumor necrosis factor alpha (TNF-α) and interleukin 1b (IL-1b; Briones et al., 2013) in the cortex and hippocampus post-injury. Given the up-regulation of these factors by TBI, the EE effect may attenuate secondary-injury related damage.
EE also induces an increase in neurotransmitter levels such as noradrenaline and dopamine, NMDA receptor expression, and brain metabolic activity, all of which are altered in TBI and have been implicated in impairment of motor and cognitive function; thus, exposure to EE could possibly regulate TBI-induced changes in these factors (Brenner et al., 1983; Boyeson and Feeney, 1990; Liljequist et al., 1993; Dietrich et al., 1994; Hamm et al., 1996).
We noted above that some work indicates that EE in animal models of epilepsy attenuates onset of seizures, a functional consequence of aberrant neuronal excitability after TBI (Pitkanen and McIntosh, 2006; Hunt et al., 2013; Shultz et al., 2013). While the exact mechanisms underlying this protective effect are unknown, it is thought to occur through an EE-induced enhancement of trophic support, changes in receptor expression and enhanced neurogenesis (as previously described; Liu et al., 1993; Cheng et al., 1995; Young et al., 1999; Reibel et al., 2000; Korbey et al., 2008).
This review shows that overall, there are insufficient data available to decide if EE effects on all of the TBI-induced molecular events noted above are all (or any of them) involved in the beneficial effects of EE in TBI. Given the limited amount of knowledge of the effects of EE specifically in TBI, it is worth also considering how EE acts therapeutically in other neurological disorders. The general trend of how EE has benefits in other brain disorders is summarized in Table 1, where it can be seen that EE acts to increase neurogenesis, dendritic branching and spine density, and increase the expression of growth factors (Johansson and Ohlsson, 1996; Young et al., 1999; Jadavji et al., 2006; Pereira et al., 2007; Gelfo et al., 2011; Valero et al., 2011). Similarly, recent work by Koopmans et al. (2012) has also demonstrated increased spinal cord progenitor cell differentiation and increased serotonergic innervation after experimental SCI, while others have shown increased dendritic spine density in the motor cortex (Kim et al., 2008) and increased BDNF levels (Berrocal et al., 2007). These studies, as well as those focussing on TBI, show that EE appears to be a promising post-injury treatment to improve sensorimotor and cognitive function in brain injury, most likely due to similar underlying structural and molecular mechanisms. Due to the wide and inter-connected nature of the effects of EE, it is likely that a combination of both molecular and morphological changes needs to occur in order to see improvements in neuronal function and behavior.
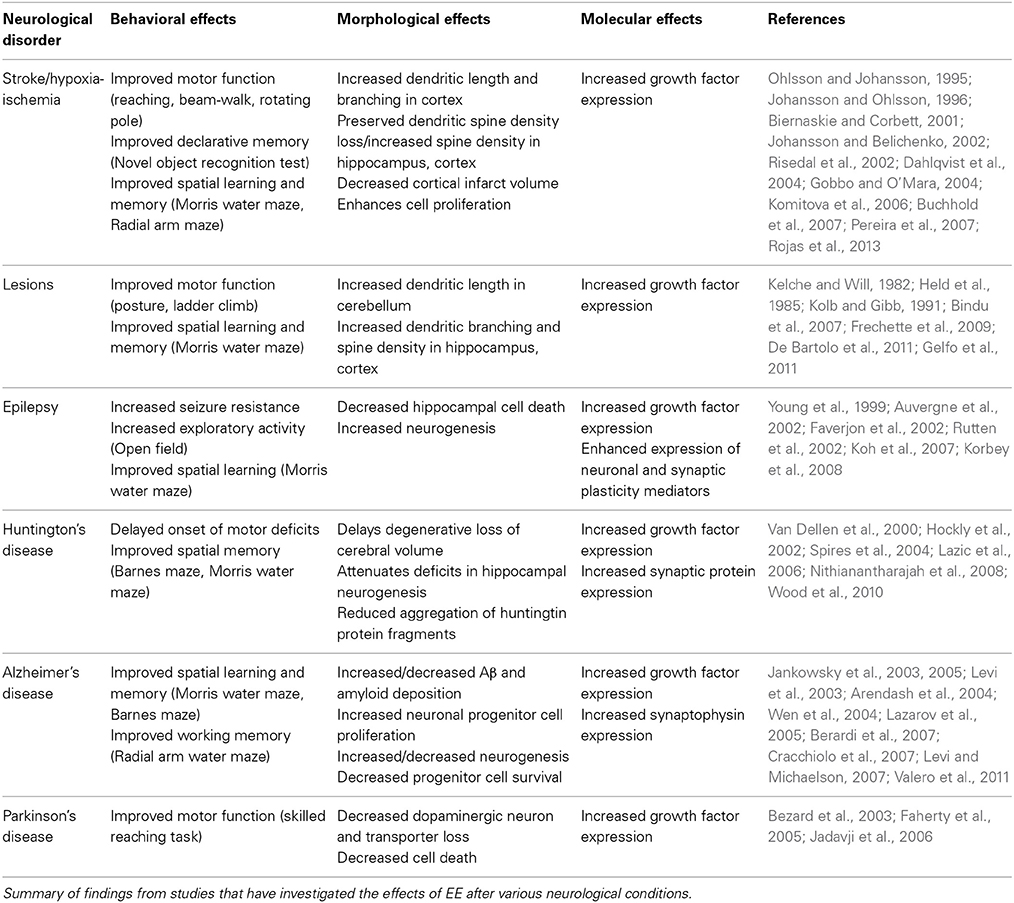
Table 1. Behavioral, morphological, and molecular effects of EE in various neurological disease conditions.
Timing of the Use of EE as a Therapy After TBI
Some clues as to the potential mechanism by which EE could rescue brain function in TBI comes from the finding that the timing and duration of EE are important factors governing motor and cognitive recovery post-TBI (Figure 2; Hoffman et al., 2008; De Witt et al., 2011; Matter et al., 2011; Cheng et al., 2012). Thus, Hoffman et al. (2008) reported that recovery of motor and cognitive function, such as beam-walking and spatial learning and memory, depended on an optimal time and length of EE exposure relative to time after injury. After mixed focal-diffuse TBI, even a short period of EE exposure (6 h) was sufficient to improve motor and cognitive behavior to a level comparable to the enhanced performance seen after much longer (3 weeks), continuous exposure to EE (Hoffman et al., 2008; De Witt et al., 2011; Matter et al., 2011). The benefits of EE were not dose-dependent, however, as task performance in animals exposed to shorter periods of EE (2 and 4 h) did not differ significantly from those of injured animals housed in standard conditions, suggesting a minimal threshold of EE exposure is needed for beneficial effects (De Witt et al., 2011). EE-induced plasticity has persisting effects such that even limited exposure (3 weeks) to EE post-TBI can result in long-term protection from memory deficits, as assessed by the MWM task, for up to 6 months after animals are withdrawn from EE conditions (Cheng et al., 2012), making it an ideal candidate for therapy post-TBI.
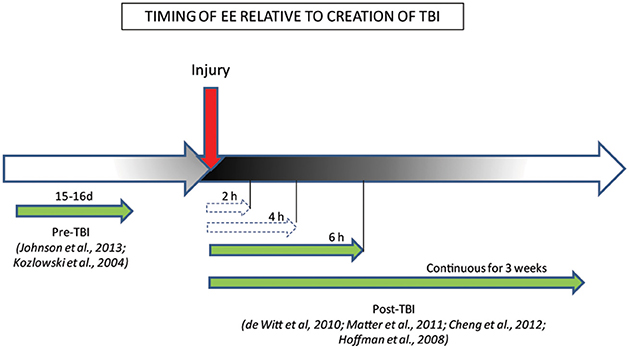
Figure 2. Behavioral benefits conferred by the timing/duration of EE relative to creation of TBI. The efficacy of EE treatment when administered pre/post-injury is represented by green full-line arrows which indicate EE timing conditions that ameliorated behaviors, while white dashed arrows indicate EE timing conditions that failed to ameliorate behaviors.
Recent studies have also demonstrated that even pre-injury exposure to EE is neuroprotective (Kozlowski et al., 2004; Johnson et al., 2013). Kozlowski et al. (2004) showed that brief (15d) EE exposure in immature rats at p21, prior to TBI in adulthood (approximately 3 months old), results in a paradoxical increase in cortical lesion volume, but coupled with faster recovery of forelimb function, assessed by foot fault and asymmetry tests. Similarly, exposure to 15 days of EE immediately pre-TBI attenuates spatial and long-term memory deficits in the MWM task, in a manner similar to that seen after post-injury EE exposure (Johnson et al., 2013). However, the relevance of such a model in a clinical setting is limited. It is however, likely that, in accord with the Hebbian theory of plasticity, pre-injury exposure to EE acts mainly to strengthen existing connections in the uninjured brain, which may carry forward post-injury. This is likely to occur through the upregulation of trophic factors such as BDNF, which are shown to increase after exposure to motor enrichment before and after injury (Kleim et al., 2003). In contrast, post-injury EE exposure acts mainly to develop and strengthen new/previously silent/remaining connections to compensate for the damage in existing pathways (Taub et al., 2002), while elevated growth factor expression acts to limit the spread of damage (Kleim et al., 2003). This would suggest that although pre- and post-injury EE-exposure is beneficial for protection and/or recovery from injury, the underlying mechanisms may be different.
The fact that even pre-injury exposure to EE can ameliorate the effects of TBI may be taken to indicate that the benefits of EE are independent of the TBI-induced molecular and structural changes that cause deficits to brain and behavior. However, this is, as yet, an unsafe assumption for the reasons that brain processes are so highly inter-linked and often use common pathways. For example, as noted above, TBI increases inflammatory cascades while EE reduces many of the same cascades; thus down-regulation of these cascades by pre-exposure to EE may reduce subsequent TBI-induced activation of the inflammatory mechanisms and thereby reduce secondary injury processes, producing neuroprotection.
Beyond the Bench
The current body of literature on the effects of EE in neurological disease indicates that EE represents significant therapeutic potential, on its own and in combination with pharmacological treatments (Kline et al., 2007, 2010, 2012), by inducing neuroprotective mechanisms involving molecular, structural, and functional processes to improve histopathologies and behavioral outcomes. Considering the many positive effects of EE demonstrated after experimental brain injury, it would be logical to try to aid recovery by applying EE in a clinical setting. Certainly, intellectually, physically and socially active lifestyles (that are akin to EE) have been linked to improved cognitive function and lower incidences of cognitive impairment, particularly in older, uninjured adults (Seeman et al., 2001; Scarmeas and Stern, 2003; Wilson et al., 2003; Newson and Kemps, 2005; Fujiwara et al., 2009; Voss et al., 2011). Similarly, cognitive enrichment early in life has also been linked to improved cognitive abilities in later life (Milgram et al., 2006), while Kramer et al. (2004) have suggested that enhanced cognitive enrichment results in improved crystallized intelligence.
Indeed, the use of EE as a rehabilitative treatment for humans post-TBI has been suggested to be effective in positively influencing long-term outcomes. However, the concept of EE for humans is more complex to define than what constitutes as EE for animals, as factors such as engagement and motivation play a role in classifying the level of enrichment an individual receives. In a clinical setting, EE can be broadly classified as a paradigm that specifically enhances and promotes engagement with cognitive, social and physical stimulation. An important caveat to the discussion about the role of EE in the treatment of TBI is that post-TBI rehabilitation programs are widely considered to be comparable to enriched environments, in that these programs often comprise of multiple components that are considered hallmarks of EE, which include physical and cognitive therapy, multi-modal stimulation, novelty, duration, functional relevance, and social integration. It has to be noted, however, that specific skill rehabilitation paradigms often do not result in improved general performance in the post-discharge environment, and instead act to improve task-specific performance (Sohlberg et al., 2000; Park and Ingles, 2001). It has instead been suggested that a more generalized treatment would be beneficial in improving overall function (Toglia, 1991; Toglia et al., 2010). Rehabilitation paradigms treating brain injury are often implemented in the acute stages post-injury, in an in-patient hospital setting. Rehabilitation based on EE principles in TBI patients results in better general functional outcomes, such as improved cognitive and motor skills (Willer et al., 1999; Powell et al., 2002; Cifu et al., 2003; Boman et al., 2004; Hayden et al., 2013), and better community integration (Zhu et al., 2001; Cicerone et al., 2004). A number of studies have also shown that increasing the duration and intensity of exposure to rehabilitative therapy results in improved recovery times (Blackerby, 1990; Spivack et al., 1992; Shiel et al., 2001; Zhu et al., 2001, 2007; Slade et al., 2002; Cifu et al., 2003; Cicerone et al., 2004).
It has also been suggested that a lack or an absence of EE is linked to cognitive decline post-injury (Till et al., 2008; Frasca et al., 2013), demonstrating the importance of continued exposure to EE in the post-discharge stages after brain injury. In that sense, a number of factors could contribute to the provision of an appropriate level of enrichment once a patient has left an intensive rehabilitative environment. These factors include ease of access to activities and resources that are cognitively, physically and socially stimulating, as well as support that encourages participation and integration with these environments (Frasca et al., 2013). Frasca et al. (2013) have also suggested that although patients eventually return to an environment that could be considered enriched post-TBI, interactions with these environments may be restricted due to limitations in cognitive and/or physical deficits. This is especially relevant during the transition from the in-patient rehabilitation environment, to post-discharge home environments, where complexity of, and engagement with environments may reduce. A reduction in enrichment in the post-acute period could be detrimental to recovery as studies have shown that functions gained during stimulation of neural pathways (such as during rehabilitation) can be lost through under-use (Rubinov et al., 2009; Warraich and Kleim, 2010; Frasca et al., 2013). Post-discharge, the major forms of therapy mapped onto EE principles include community-based and home-based rehabilitation, with the aim that these programs would aid in improving behaviors and skills required for everyday functioning, improving community integration, and preventing cognitive decline (Fryer and Haffey, 1987; Frasca et al., 2013). Studies have shown that continued enrichment in the form of cognitive rehabilitation in the post-discharge setting (i.e., domestic or vocational environments) increases neuropsychological function, learning and memory (Willer et al., 1999; Boman et al., 2004).
Given the complexity and ethics of manipulations of the environment in humans recovering from TBI, in addition to the difficulties in accurately comparing the effectiveness of various rehabilitation paradigms, questions of the correlation between these effects and EE-induced functional changes remain. Injury heterogeneity also raises challenges in defining exactly what level of enrichment is optimal and beneficial. However, the findings presented in this section strongly suggest that EE or EE-based therapy tailored to the patient's needs could significantly improve outcomes when applied in both the in-patient, acute and post-discharge, chronic settings.
Conclusion
The studies described here well support the use of EE as a therapeutic paradigm in the treatment of TBI. However, while the results of these studies all show promise in improving TBI-induced histopathologies and sensorimotor and cognitive deficits, there is still much work to be done to clarify our understanding of how EE exerts its effects in disease conditions. Paramount to the understanding of how EE improves behavioral outcomes after injury is the investigation of how EE changes neuronal function post-TBI, of which we know nothing. Only once these effects are unveiled will we be able to implement EE as a treatment option post-injury at its maximum potential.
It is also worth adding the caution that while EE holds promise in its application as a therapeutic tool after brain injury in humans, the complex nature of utilizing EE in a clinical setting makes it difficult to standardize treatment and compare outcomes. It is also true that EE as an experimental paradigm in animal studies has yet to be standardized, with housing conditions, environmental stimuli, number of animals per cage, age of animals at onset of enrichment, as well as the duration of enrichment, varying markedly between studies. The extent of contribution of these factors is particularly relevant when considering the demonstrated neuroprotective effects of EE in neurological disease states, where little is known about how improved functional outcomes relate to changes in neuronal function. Using experimental models that are easily controlled and manipulated, however, would provide us with valuable insight into the therapeutic potential of EE, both in laboratory and clinical settings.
Conflict of Interest Statement
The authors declare that the research was conducted in the absence of any commercial or financial relationships that could be construed as a potential conflict of interest.
References
Abou-Ismail, U. A. (2011). The effects of cage enrichment on agonistic behaviour and dominance in male laboratory rats (Rattus norvegicus). Res. Vet. Sci. 90, 346–351. doi: 10.1016/j.rvsc.2010.06.010
Adams, J. H., Jennett, B., Mclellan, D. R., Murray, L. S., and Graham, D. I. (1999). The neuropathology of the vegetative state after head injury. J. Clin. Pathol. 52, 804–806. doi: 10.1136/jcp.52.11.804
Alwis, D., Yan, E. B., Morganti-Kossmann, M. C., and Rajan, R. (2012). Sensory cortex underpinnings of traumatic brain injury deficits. PLoS ONE 7:e52169. doi: 10.1371/journal.pone.0052169
Alwis, D. S., Johnstone, V., Yan, E., and Rajan, R. (2013). Diffuse traumatic brain injury and the sensory brain. Clin. Exp. Pharmacol. Physiol. 40, 473–483. doi: 10.1111/1440-1681.12100
Alwis, D. S., and Rajan, R. (2013). Environmental enrichment causes a global potentiation of neuronal responses across stimulus complexity and lamina of sensory cortex. Front. Cell. Neurosci. 7:124. doi: 10.3389/fncel.2013.00124
Andriessen, T. M., Jacobs, B., and Vos, P. E. (2010). Clinical characteristics and pathophysiological mechanisms of focal and diffuse traumatic brain injury. J. Cell. Mol. Med. 14, 2381–2392. doi: 10.1111/j.1582-4934.2010.01164.x
Angelucci, F., De Bartolo, P., Gelfo, F., Foti, F., Cutuli, D., Bossu, P., et al. (2009). Increased concentrations of nerve growth factor and brain-derived neurotrophic factor in the rat cerebellum after exposure to environmental enrichment. Cerebellum 8, 499–506. doi: 10.1007/s12311-009-0129-1
Arendash, G. W., Garcia, M. F., Costa, D. A., Cracchiolo, J. R., Wefes, I. M., and Potter, H. (2004). Environmental enrichment improves cognition in aged Alzheimer's transgenic mice despite stable beta-amyloid deposition. Neuroreport 15, 1751–1754. doi: 10.1097/01.wnr.0000137183.68847.4e
Armstrong-James, M., Fox, K., and Das-Gupta, A. (1992). Flow of excitation within rat barrel cortex on striking a single vibrissa. J. Neurophysiol. 68, 1345–1358.
Artola, A., Von Frijtag, J. C., Fermont, P. C., Gispen, W. H., Schrama, L. H., Kamal, A., et al. (2006). Long-lasting modulation of the induction of LTD and LTP in rat hippocampal CA1 by behavioural stress and environmental enrichment. Eur. J. Neurosci. 23, 261–272. doi: 10.1111/j.1460-9568.2005.04552.x
Auvergne, R., Lere, C., El Bahh, B., Arthaud, S., Lespinet, V., Rougier, A., et al. (2002). Delayed kindling epileptogenesis and increased neurogenesis in adult rats housed in an enriched environment. Brain Res. 954, 277–285. doi: 10.1016/S0006-8993(02)03355-3
Bao, S., Chang, E. F., Davis, J. D., Gobeske, K. T., and Merzenich, M. M. (2003). Progressive degradation and subsequent refinement of acoustic representations in the adult auditory cortex. J. Neurosci. 23, 10765–10775.
Baroncelli, L., Bonaccorsi, J., Milanese, M., Bonifacino, T., Giribaldi, F., Manno, I., et al. (2012). Enriched experience and recovery from amblyopia in adult rats: impact of motor, social and sensory components. Neuropharmacology 62, 2388–2397. doi: 10.1016/j.neuropharm.2012.02.010
Baroncelli, L., Braschi, C., Spolidoro, M., Begenisic, T., Maffei, L., and Sale, A. (2011). Brain plasticity and disease: a matter of inhibition. Neural Plast. 2011, 286073. doi: 10.1155/2011/286073
Baroncelli, L., Braschi, C., Spolidoro, M., Begenisic, T., Sale, A., and Maffei, L. (2010a). Nurturing brain plasticity: impact of environmental enrichment. Cell Death Differ. 17, 1092–1103. doi: 10.1038/cdd.2009.193
Baroncelli, L., Sale, A., Viegi, A., Maya Vetencourt, J. F., De Pasquale, R., Baldini, S., et al. (2010b). Experience-dependent reactivation of ocular dominance plasticity in the adult visual cortex. Exp. Neurol. 226, 100–109. doi: 10.1016/j.expneurol.2010.08.009
Bawden, H. N., Knights, R. M., and Winogron, H. W. (1985). Speeded performance following head injury in children. J. Clin. Exp. Neuropsychol. 7, 39–54. doi: 10.1080/01688638508401241
Beaulieu, C., and Colonnier, M. (1987). Effect of the richness of the environment on the cat visual cortex. J. Comp. Neurol. 266, 478–494. doi: 10.1002/cne.902660404
Beaulieu, C., and Cynader, M. (1990a). Effect of the richness of the environment on neurons in cat visual cortex. I. Receptive field properties. Brain Res. Dev. Brain Res. 53, 71–81.
Beaulieu, C., and Cynader, M. (1990b). Effect of the richness of the environment on neurons in cat visual cortex. II. Spatial and temporal frequency characteristics. Brain Res. Dev. Brain Res. 53, 82–88.
Bechara, R. G., and Kelly, A. M. (2013). Exercise improves object recognition memory and induces BDNF expression and cell proliferation in cognitively enriched rats. Behav. Brain Res. 245, 96–100. doi: 10.1016/j.bbr.2013.02.018
Bekinschtein, P., Oomen, C. A., Saksida, L. M., and Bussey, T. J. (2011). Effects of environmental enrichment and voluntary exercise on neurogenesis, learning and memory, and pattern separation: BDNF as a critical variable? Semin. Cell Dev. Biol. 22, 536–542. doi: 10.1016/j.semcdb.2011.07.002
Benali, A., Weiler, E., Benali, Y., Dinse, H. R., and Eysel, U. T. (2008). Excitation and inhibition jointly regulate cortical reorganization in adult rats. J. Neurosci. 28, 12284–12293. doi: 10.1523/JNEUROSCI.1952-08.2008
Benaroya-Milshtein, N., Hollander, N., Apter, A., Kukulansky, T., Raz, N., Wilf, A., et al. (2004). Environmental enrichment in mice decreases anxiety, attenuates stress responses and enhances natural killer cell activity. Eur. J. Neurosci. 20, 1341–1347. doi: 10.1111/j.1460-9568.2004.03587.x
Bengoetxea, H., Ortuzar, N., Bulnes, S., Rico-Barrio, I., Lafuente, J. V., and Argandona, E. G. (2012). Enriched and deprived sensory experience induces structural changes and rewires connectivity during the postnatal development of the brain. Neural Plast. 2012:305693. doi: 10.1155/2012/305693
Bennett, E. L., Rosenzweig, M. R., and Diamond, M. C. (1969). Rat brain: effects of environmental enrichment on wet and dry weights. Science 163, 825–826. doi: 10.1126/science.163.3869.825
Berardi, N., Braschi, C., Capsoni, S., Cattaneo, A., and Maffei, L. (2007). Environmental enrichment delays the onset of memory deficits and reduces neuropathological hallmarks in a mouse model of Alzheimer-like neurodegeneration. J. Alzheimers Dis. 11, 359–370.
Berrocal, Y., Pearse, D. D., Singh, A., Andrade, C. M., Mcbroom, J. S., Puentes, R., et al. (2007). Social and environmental enrichment improves sensory and motor recovery after severe contusive spinal cord injury in the rat. J. Neurotrauma 24, 1761–1772. doi: 10.1089/neu.2007.0327
Bezard, E., Dovero, S., Belin, D., Duconger, S., Jackson-Lewis, V., Przedborski, S., et al. (2003). Enriched environment confers resistance to 1-methyl-4-phenyl-1,2,3,6-tetrahydropyridine and cocaine: involvement of dopamine transporter and trophic factors. J. Neurosci. 23, 10999–11007.
Biernaskie, J., and Corbett, D. (2001). Enriched rehabilitative training promotes improved forelimb motor function and enhanced dendritic growth after focal ischemic injury. J. Neurosci. 21, 5272–5280.
Bindu, B., Alladi, P. A., Mansooralikhan, B. M., Srikumar, B. N., Raju, T. R., and Kutty, B. M. (2007). Short-term exposure to an enriched environment enhances dendritic branching but not brain-derived neurotrophic factor expression in the hippocampus of rats with ventral subicular lesions. Neuroscience 144, 412–423. doi: 10.1016/j.neuroscience.2006.09.057
Birch, A. M., Mcgarry, N. B., and Kelly, A. M. (2013). Short-term environmental enrichment, in the absence of exercise, improves memory, and increases NGF concentration, early neuronal survival, and synaptogenesis in the dentate gyrus in a time-dependent manner. Hippocampus 23, 437–450. doi: 10.1002/hipo.22103
Blackerby, W. F. (1990). Intensity of rehabilitation and length of stay. Brain Inj. 4, 167–173. doi: 10.3109/02699059009026162
Boman, I. L., Lindstedt, M., Hemmingsson, H., and Bartfai, A. (2004). Cognitive training in home environment. Brain Inj. 18, 985–995. doi: 10.1080/02699050410001672396
Bondi, C. O., Klitsch, K. C., Leary, J. B., and Kline, A. E. (2014). Environmental enrichment as a viable neurorehabilitation strategy for experimental traumatic brain injury. J. Neurotrauma 31, 873–888. doi: 10.1089/neu.2014.3328
Bourgeon, S., Xerri, C., and Coq, J. O. (2004). Abilities in tactile discrimination of textures in adult rats exposed to enriched or impoverished environments. Behav. Brain Res. 153, 217–231. doi: 10.1016/j.bbr.2003.12.002
Boyeson, M. G., and Feeney, D. M. (1990). Intraventricular norepinephrine facilitates motor recovery following sensorimotor cortex injury. Pharmacol. Biochem. Behav. 35, 497–501. doi: 10.1016/0091-3057(90)90279-Q
Brenes, J. C., Padilla, M., and Fornaguera, J. (2009). A detailed analysis of open-field habituation and behavioral and neurochemical antidepressant-like effects in postweaning enriched rats. Behav. Brain Res. 197, 125–137. doi: 10.1016/j.bbr.2008.08.014
Brenner, E., Mirmiran, M., Uylings, H. B., and Van Der Gugten, J. (1983). Impaired growth of the cerebral cortex of rats treated neonatally with 6-hydroxydopamine under different environmental conditions. Neurosci. Lett. 42, 13–17. doi: 10.1016/0304-3940(83)90414-7
Briones, T. L., Klintsova, A. Y., and Greenough, W. T. (2004). Stability of synaptic plasticity in the adult rat visual cortex induced by complex environment exposure. Brain Res. 1018, 130–135. doi: 10.1016/j.brainres.2004.06.001
Briones, T. L., Woods, J., and Rogozinska, M. (2013). Decreased neuroinflammation and increased brain energy homeostasis following environmental enrichment after mild traumatic brain injury is associated with improvement in cognitive function. Acta Neuropathol. Commun. 1, 57. doi: 10.1186/2051-5960-1-57
Brosseau-Lachaine, O., Gagnon, I., Forget, R., and Faubert, J. (2008). Mild traumatic brain injury induces prolonged visual processing deficits in children. Brain Inj. 22, 657–668. doi: 10.1080/02699050802203353
Bruel-Jungerman, E., Laroche, S., and Rampon, C. (2005). New neurons in the dentate gyrus are involved in the expression of enhanced long-term memory following environmental enrichment. Eur. J. Neurosci. 21, 513–521. doi: 10.1111/j.1460-9568.2005.03875.x
Buchhold, B., Mogoanta, L., Suofu, Y., Hamm, A., Walker, L., Kessler, C., et al. (2007). Environmental enrichment improves functional and neuropathological indices following stroke in young and aged rats. Restor. Neurol. Neurosci. 25, 467–484.
Buonomano, D. V., and Merzenich, M. M. (1998). Cortical plasticity: from synapses to maps. Annu. Rev. Neurosci. 21, 149–186. doi: 10.1146/annurev.neuro.21.1.149
Buonviso, N., and Chaput, M. (2000). Olfactory experience decreases responsiveness of the olfactory bulb in the adult rat. Neuroscience 95, 325–332. doi: 10.1016/S0306-4522(99)00450-9
Caeyenberghs, K., Wenderoth, N., Smits-Engelsman, B. C., Sunaert, S., and Swinnen, S. P. (2009). Neural correlates of motor dysfunction in children with traumatic brain injury: exploration of compensatory recruitment patterns. Brain 132, 684–694. doi: 10.1093/brain/awn344
Cai, R., Guo, F., Zhang, J., Xu, J., Cui, Y., and Sun, X. (2009). Environmental enrichment improves behavioral performance and auditory spatial representation of primary auditory cortical neurons in rat. Neurobiol. Learn. Mem. 91, 366–376. doi: 10.1016/j.nlm.2009.01.005
Camel, J. E., Withers, G. S., and Greenough, W. T. (1986). Persistence of visual cortex dendritic alterations induced by postweaning exposure to a “superenriched” environment in rats. Behav. Neurosci. 100, 810–813. doi: 10.1037/0735-7044.100.6.810
Chang, E. F., Bao, S., Imaizumi, K., Schreiner, C. E., and Merzenich, M. M. (2005). Development of spectral and temporal response selectivity in the auditory cortex. Proc. Natl. Acad. Sci. U.S.A. 102, 16460–16465. doi: 10.1073/pnas.0508239102
Chang, E. F., and Merzenich, M. M. (2003). Environmental noise retards auditory cortical development. Science 300, 498–502. doi: 10.1126/science.1082163
Chen, X., Li, Y., Kline, A. E., Dixon, C. E., Zafonte, R. D., and Wagner, A. K. (2005). Gender and environmental effects on regional brain-derived neurotrophic factor expression after experimental traumatic brain injury. Neuroscience 135, 11–17. doi: 10.1016/j.neuroscience.2005.05.041
Cheng, B., Furukawa, K., O'Keefe, J. A., Goodman, Y., Kihiko, M., Fabian, T., et al. (1995). Basic fibroblast growth factor selectively increases AMPA-receptor subunit GluR1 protein level and differentially modulates Ca2+ responses to AMPA and NMDA in hippocampal neurons. J. Neurochem. 65, 2525–2536. doi: 10.1046/j.1471-4159.1995.65062525.x
Cheng, J. P., Shaw, K. E., Monaco, C. M., Hoffman, A. N., Sozda, C. N., Olsen, A. S., et al. (2012). A relatively brief exposure to environmental enrichment after experimental traumatic brain injury confers long-term cognitive benefits. J. Neurotrauma 29, 2684–2688. doi: 10.1089/neu.2012.2560
Cicerone, K. D., Mott, T., Azulay, J., and Friel, J. C. (2004). Community integration and satisfaction with functioning after intensive cognitive rehabilitation for traumatic brain injury. Arch. Phys. Med. Rehabil. 85, 943–950. doi: 10.1016/j.apmr.2003.07.019
Cifu, D. X., Kreutzer, J. S., Kolakowsky-Hayner, S. A., Marwitz, J. H., and Englander, J. (2003). The relationship between therapy intensity and rehabilitative outcomes after traumatic brain injury: a multicenter analysis. Arch. Phys. Med. Rehabil. 84, 1441–1448. doi: 10.1016/S0003-9993(03)00272-7
Condon, C. D., and Weinberger, N. M. (1991). Habituation produces frequency-specific plasticity of receptive fields in the auditory cortex. Behav. Neurosci. 105, 416–430. doi: 10.1037/0735-7044.105.3.416
Connor, J. R., Wang, E. C., and Diamond, M. C. (1982). Increased length of terminal dendritic segments in old adult rats' somatosensory cortex: an environmentally induced response. Exp. Neurol. 78, 466–470. doi: 10.1016/0014-4886(82)90064-4
Coq, J. O., and Xerri, C. (1998). Environmental enrichment alters organizational features of the forepaw representation in the primary somatosensory cortex of adult rats. Exp. Brain Res. 121, 191–204. doi: 10.1007/s002210050452
Cracchiolo, J. R., Mori, T., Nazian, S. J., Tan, J., Potter, H., and Arendash, G. W. (2007). Enhanced cognitive activity–over and above social or physical activity–is required to protect Alzheimer's mice against cognitive impairment, reduce Abeta deposition, and increase synaptic immunoreactivity. Neurobiol. Learn. Mem. 88, 277–294. doi: 10.1016/j.nlm.2007.07.007
Dahlqvist, P., Ronnback, A., Bergstrom, S. A., Soderstrom, I., and Olsson, T. (2004). Environmental enrichment reverses learning impairment in the Morris water maze after focal cerebral ischemia in rats. Eur. J. Neurosci. 19, 2288–2298. doi: 10.1111/j.0953-816X.2004.03248.x
Davis, A. S., and Dean, R. S. (2010). Assessing sensory-motor deficits in pediatric traumatic brain injury. Appl. Neuropsychol. 17, 104–109. doi: 10.1080/09084281003708951
De Bartolo, P., Gelfo, F., Burello, L., De Giorgio, A., Petrosini, L., and Granato, A. (2011). Plastic changes in striatal fast-spiking interneurons following hemicerebellectomy and environmental enrichment. Cerebellum 10, 624–632. doi: 10.1007/s12311-011-0275-0
De Villers-Sidani, E., and Merzenich, M. M. (2011). Lifelong plasticity in the rat auditory cortex: basic mechanisms and role of sensory experience. Prog. Brain Res. 191, 119–131. doi: 10.1016/B978-0-444-53752-2.00009-6
Devonshire, I. M., Dommett, E. J., Grandy, T. H., Halliday, A. C., and Greenfield, S. A. (2010). Environmental enrichment differentially modifies specific components of sensory-evoked activity in rat barrel cortex as revealed by simultaneous electrophysiological recordings and optical imaging in vivo. Neuroscience 170, 662–669. doi: 10.1016/j.neuroscience.2010.07.029
De Witt, B. W., Ehrenberg, K. M., Mcaloon, R. L., Panos, A. H., Shaw, K. E., Raghavan, P. V., et al. (2011). Abbreviated environmental enrichment enhances neurobehavioral recovery comparably to continuous exposure after traumatic brain injury. Neurorehabil. Neural Repair 25, 343–350. doi: 10.1177/1545968310390520
Diamond, M. C., Ingham, C. A., Johnson, R. E., Bennett, E. L., and Rosenzweig, M. R. (1976). Effects of environment on morphology of rat cerebral cortex and hippocampus. J. Neurobiol. 7, 75–85. doi: 10.1002/neu.480070108
Diamond, M. C., Lindner, B., and Raymond, A. (1967). Extensive cortical depth measurements and neuron size increases in the cortex of environmentally enriched rats. J. Comp. Neurol. 131, 357–364. doi: 10.1002/cne.901310305
Diamond, M. C., Rosenzweig, M. R., Bennett, E. L., Lindner, B., and Lyon, L. (1972). Effects of environmental enrichment and impoverishment on rat cerebral cortex. J. Neurobiol. 3, 47–64. doi: 10.1002/neu.480030105
Diamond, M. E., Armstrong-James, M., and Ebner, F. F. (1993). Experience-dependent plasticity in adult rat barrel cortex. Proc. Natl. Acad. Sci. U.S.A. 90, 2082–2086. doi: 10.1073/pnas.90.5.2082
Diamond, M. E., Huang, W., and Ebner, F. F. (1994). Laminar comparison of somatosensory cortical plasticity. Science 265, 1885–1888. doi: 10.1126/science.8091215
Dietrich, W. D., Alonso, O., Busto, R., and Ginsberg, M. D. (1994). Widespread metabolic depression and reduced somatosensory circuit activation following traumatic brain injury in rats. J. Neurotrauma 11, 629–640. doi: 10.1089/neu.1994.11.629
Ding, M. C., Lo, E. H., and Stanley, G. B. (2008). Sustained focal cortical compression reduces electrically-induced seizure threshold. Neuroscience 154, 551–555. doi: 10.1016/j.neuroscience.2008.03.088
Ding, M. C., Wang, Q., Lo, E. H., and Stanley, G. B. (2011). Cortical excitation and inhibition following focal traumatic brain injury. J. Neurosci. 31, 14085–14094. doi: 10.1523/JNEUROSCI.3572-11.2011
Drapeau, E., Mayo, W., Aurousseau, C., Le Moal, M., Piazza, P. V., and Abrous, D. N. (2003). Spatial memory performances of aged rats in the water maze predict levels of hippocampal neurogenesis. Proc. Natl. Acad. Sci. U.S.A. 100, 14385–14390. doi: 10.1073/pnas.2334169100
Drapeau, E., Montaron, M. F., Aguerre, S., and Abrous, D. N. (2007). Learning-induced survival of new neurons depends on the cognitive status of aged rats. J. Neurosci. 27, 6037–6044. doi: 10.1523/JNEUROSCI.1031-07.2007
Draper, K., and Ponsford, J. (2008). Cognitive functioning ten years following traumatic brain injury and rehabilitation. Neuropsychology 22, 618–625. doi: 10.1037/0894-4105.22.5.618
Draper, K., and Ponsford, J. (2009). Long-term outcome following traumatic brain injury: a comparison of subjective reports by those injured and their relatives. Neuropsychol. Rehabil. 19, 645–661. doi: 10.1080/17405620802613935
Du, X., Leang, L., Mustafa, T., Renoir, T., Pang, T. Y., and Hannan, A. J. (2012). Environmental enrichment rescues female-specific hyperactivity of the hypothalamic-pituitary-adrenal axis in a model of Huntington's disease. Transl. Psychiatry 2, e133. doi: 10.1038/tp.2012.58
Duffy, S. N., Craddock, K. J., Abel, T., and Nguyen, P. V. (2001). Environmental enrichment modifies the PKA-dependence of hippocampal LTP and improves hippocampus-dependent memory. Learn. Mem. 8, 26–34. doi: 10.1101/lm.36301
Eckert, M. J., and Abraham, W. C. (2010). Physiological effects of enriched environment exposure and LTP induction in the hippocampus in vivo do not transfer faithfully to in vitro slices. Learn. Mem. 17, 480–484. doi: 10.1101/lm.1822610
Eckert, M. J., and Abraham, W. C. (2013). Effects of environmental enrichment exposure on synaptic transmission and plasticity in the hippocampus. Curr. Top. Behav. Neurosci. 15, 165–187. doi: 10.1007/7854_2012_215
Einon, D. F., Morgan, M. J., and Will, B. E. (1980). Effects of post-operative environment on recovery from dorsal hippocampal lesions in young rats: tests of spatial memory and motor transfer. Q. J. Exp. Psychol. 32, 137–148. doi: 10.1080/00335558008248239
Elliott, B. M., and Grunberg, N. E. (2005). Effects of social and physical enrichment on open field activity differ in male and female Sprague-Dawley rats. Behav. Brain Res. 165, 187–196. doi: 10.1016/j.bbr.2005.06.025
Engineer, N. D., Percaccio, C. R., Pandya, P. K., Moucha, R., Rathbun, D. L., and Kilgard, M. P. (2004). Environmental enrichment improves response strength, threshold, selectivity, and latency of auditory cortex neurons. J. Neurophysiol. 92, 73–82. doi: 10.1152/jn.00059.2004
Erickson, K. I., Voss, M. W., Prakash, R. S., Basak, C., Szabo, A., Chaddock, L., et al. (2011). Exercise training increases size of hippocampus and improves memory. Proc. Natl. Acad. Sci. U.S.A. 108, 3017–3022. doi: 10.1073/pnas.1015950108
Fagiolini, M., and Hensch, T. K. (2000). Inhibitory threshold for critical-period activation in primary visual cortex. Nature 404, 183–186. doi: 10.1038/35004582
Faherty, C. J., Raviie Shepherd, K., Herasimtschuk, A., and Smeyne, R. J. (2005). Environmental enrichment in adulthood eliminates neuronal death in experimental Parkinsonism. Brain Res. Mol. Brain Res. 134, 170–179. doi: 10.1016/j.molbrainres.2004.08.008
Falkenberg, T., Mohammed, A. K., Henriksson, B., Persson, H., Winblad, B., and Lindefors, N. (1992). Increased expression of brain-derived neurotrophic factor mRNA in rat hippocampus is associated with improved spatial memory and enriched environment. Neurosci. Lett. 138, 153–156. doi: 10.1016/0304-3940(92)90494-R
Farmer, J., Zhao, X., Van Praag, H., Wodtke, K., Gage, F. H., and Christie, B. R. (2004). Effects of voluntary exercise on synaptic plasticity and gene expression in the dentate gyrus of adult male Sprague-Dawley rats in vivo. Neuroscience 124, 71–79. doi: 10.1016/j.neuroscience.2003.09.029
Faul, M., Xu, L., Wald, M. M., and Coronado, V. G. (2010). Traumatic Brain Injury in the United States: Emergency Department Visits, Hospitalizations, and Deaths 2002-2006. Atlanta, GA: Centers for Disease Control and Prevention; National Center for Injury Prevention and Control.
Faverjon, S., Silveira, D. C., Fu, D. D., Cha, B. H., Akman, C., Hu, Y., et al. (2002). Beneficial effects of enriched environment following status epilepticus in immature rats. Neurology 59, 1356–1364. doi: 10.1212/01.WNL.0000033588.59005.55
Fernandez-Teruel, A., Gimenez-Llort, L., Escorihuela, R. M., Gil, L., Aguilar, R., Steimer, T., et al. (2002). Early-life handling stimulation and environmental enrichment: are some of their effects mediated by similar neural mechanisms? Pharmacol. Biochem. Behav. 73, 233–245. doi: 10.1016/S0091-3057(02)00787-6
Fletcher, M. L., and Wilson, D. A. (2003). Olfactory bulb mitral-tufted cell plasticity: odorant-specific tuning reflects previous odorant exposure. J. Neurosci. 23, 6946–6955.
Folmer, R. L., Billings, C. J., Diedesch-Rouse, A. C., Gallun, F. J., and Lew, H. L. (2011). Electrophysiological assessments of cognition and sensory processing in TBI: applications for diagnosis, prognosis and rehabilitation. Int. J. Psychophysiol. 82, 4–15. doi: 10.1016/j.ijpsycho.2011.03.005
Foster, T. C., and Dumas, T. C. (2001). Mechanism for increased hippocampal synaptic strength following differential experience. J. Neurophysiol. 85, 1377–1383.
Foster, T. C., Gagne, J., and Massicotte, G. (1996). Mechanism of altered synaptic strength due to experience: relation to long-term potentiation. Brain Res. 736, 243–250.
Frasca, D., Tomaszczyk, J., McFadyen, B. J., and Green, R. E. (2013). Traumatic brain injury and post-acute decline: what role does environmental enrichment play? A scoping review. Front. Hum. Neurosci. 7:31. doi: 10.3389/fnhum.2013.00031
Frechette, M., Rennie, K., and Pappas, B. A. (2009). Developmental forebrain cholinergic lesion and environmental enrichment: behaviour, CA1 cytoarchitecture and neurogenesis. Brain Res. 1252, 172–182. doi: 10.1016/j.brainres.2008.11.082
Frenkel, M. Y., and Bear, M. F. (2004). How monocular deprivation shifts ocular dominance in visual cortex of young mice. Neuron 44, 917–923. doi: 10.1016/j.neuron.2004.12.003
Frick, K. M., and Fernandez, S. M. (2003). Enrichment enhances spatial memory and increases synaptophysin levels in aged female mice. Neurobiol. Aging 24, 615–626. doi: 10.1016/S0197-4580(02)00138-0
Fryer, L. J., and Haffey, W. J. (1987). Cognitive rehabilitation and community readaptation: outcomes from two program models. J. Head Trauma Rehabil. 2, 51–63. doi: 10.1097/00001199-198709000-00007
Fujiwara, Y., Chaves, P. H., Yoshida, H., Amano, H., Fukaya, T., Watanabe, N., et al. (2009). Intellectual activity and likelihood of subsequently improving or maintaining instrumental activities of daily living functioning in community-dwelling older Japanese: a longitudinal study. Int. J. Geriatr. Psychiatry 24, 547–555. doi: 10.1002/gps.2148
Furini, C. R., Rossato, J. I., Bitencourt, L. L., Medina, J. H., Izquierdo, I., and Cammarota, M. (2010). Beta-adrenergic receptors link NO/sGC/PKG signaling to BDNF expression during the consolidation of object recognition long-term memory. Hippocampus 20, 672–683. doi: 10.1002/hipo.20656
Gagnon, I., Forget, R., Sullivan, S. J., and Friedman, D. (1998). Motor performance following a mild traumatic brain injury in children: an exploratory study. Brain Inj. 12, 843–853. doi: 10.1080/026990598122070
Galani, R., Berthel, M. C., Lazarus, C., Majchrzak, M., Barbelivien, A., Kelche, C., et al. (2007). The behavioral effects of enriched housing are not altered by serotonin depletion but enrichment alters hippocampal neurochemistry. Neurobiol. Learn. Mem. 88, 1–10. doi: 10.1016/j.nlm.2007.03.009
Gardner, E. B., Boitano, J. J., Mancino, N. S., and D'amico, D. P. (1975). Environmental enrichment and deprivation: effects on learning, memory and exploration. Physiol. Behav. 14, 321–327. doi: 10.1016/0031-9384(75)90040-2
Gaulke, L. J., Horner, P. J., Fink, A. J., Mcnamara, C. L., and Hicks, R. R. (2005). Environmental enrichment increases progenitor cell survival in the dentate gyrus following lateral fluid percussion injury. Brain Res. Mol. Brain Res. 141, 138–150. doi: 10.1016/j.molbrainres.2005.08.011
Gelfo, F., Cutuli, D., Foti, F., Laricchiuta, D., De Bartolo, P., Caltagirone, C., et al. (2011). Enriched environment improves motor function and increases neurotrophins in hemicerebellar lesioned rats. Neurorehabil. Neural Repair 25, 243–252. doi: 10.1177/1545968310380926
Gibson, E. J. (1953). Improvement in perceptual judgments as a function of controlled practice or training. Psychol. Bull. 50, 401–431. doi: 10.1037/h0055517
Globus, A., Rosenzweig, M. R., Bennett, E. L., and Diamond, M. C. (1973). Effects of differential experience on dendritic spine counts in rat cerebral cortex. J. Comp. Physiol. Psychol. 82, 175–181. doi: 10.1037/h0033910
Gobbo, O. L., and O'Mara, S. M. (2004). Impact of enriched-environment housing on brain-derived neurotrophic factor and on cognitive performance after a transient global ischemia. Behav. Brain Res. 152, 231–241. doi: 10.1016/j.bbr.2003.10.017
Graham, D. I., Mcintosh, T. K., Maxwell, W. L., and Nicoll, J. A. (2000). Recent advances in neurotrauma. J. Neuropathol. Exp. Neurol. 59, 641–651.
Green, E. J., and Greenough, W. T. (1986). Altered synaptic transmission in dentate gyrus of rats reared in complex environments: evidence from hippocampal slices maintained in vitro. J. Neurophysiol. 55, 739–750.
Greenough, W. T., and Volkmar, F. R. (1973). Pattern of dendritic branching in occipital cortex of rats reared in complex environments. Exp. Neurol. 40, 491–504. doi: 10.1016/0014-4886(73)90090-3
Greenough, W. T., Volkmar, F. R., and Juraska, J. M. (1973). Effects of rearing complexity on dendritic branching in frontolateral and temporal cortex of the rat. Exp. Neurol. 41, 371–378. doi: 10.1016/0014-4886(73)90278-1
Guic, E., Carrasco, X., Rodriguez, E., Robles, I., and Merzenich, M. M. (2008). Plasticity in primary somatosensory cortex resulting from environmentally enriched stimulation and sensory discrimination training. Biol. Res. 41, 425–437. doi: 10.4067/S0716-97602008000400008
Haaland, K. Y., Temkin, N., Randahl, G., and Dikmen, S. (1994). Recovery of simple motor skills after head injury. J. Clin. Exp. Neuropsychol. 16, 448–456. doi: 10.1080/01688639408402655
Hall, K. D., and Lifshitz, J. (2010). Diffuse traumatic brain injury initially attenuates and later expands activation of the rat somatosensory whisker circuit concomitant with neuroplastic responses. Brain Res. 1323, 161–173. doi: 10.1016/j.brainres.2010.01.067
Hamm, R. J., Temple, M. D., O'Dell, D. M., Pike, B. R., and Lyeth, B. G. (1996). Exposure to environmental complexity promotes recovery of cognitive function after traumatic brain injury. J. Neurotrauma 13, 41–47. doi: 10.1089/neu.1996.13.41
Harati, H., Barbelivien, A., Herbeaux, K., Muller, M. A., Engeln, M., Kelche, C., et al. (2013). Lifelong environmental enrichment in rats: impact on emotional behavior, spatial memory vividness, and cholinergic neurons over the lifespan. Age (Dordr). 35, 1027–1043. doi: 10.1007/s11357-012-9424-8
Harauzov, A., Spolidoro, M., Dicristo, G., De Pasquale, R., Cancedda, L., Pizzorusso, T., et al. (2010). Reducing intracortical inhibition in the adult visual cortex promotes ocular dominance plasticity. J. Neurosci. 30, 361–371. doi: 10.1523/JNEUROSCI.2233-09.2010
Hayden, M. E., Plenger, P., Bison, K., Kowalske, K., Masel, B., and Qualls, D. (2013). Treatment effect versus pretreatment recovery in persons with traumatic brain injury: a study regarding the effectiveness of postacute rehabilitation. PM R 5, 319–327; quiz 327. doi: 10.1016/j.pmrj.2012.12.005
Hebb, D. O. (1947). The effects of early experience on problem-solving at maturity. Am. Psychol. 2, 306–307.
Held, J. M., Gordon, J., and Gentile, A. M. (1985). Environmental influences on locomotor recovery following cortical lesions in rats. Behav. Neurosci. 99, 678–690. doi: 10.1037/0735-7044.99.4.678
Heldt, S. A., Stanek, L., Chhatwal, J. P., and Ressler, K. J. (2007). Hippocampus-specific deletion of BDNF in adult mice impairs spatial memory and extinction of aversive memories. Mol. Psychiatry 12, 656–670. doi: 10.1038/sj.mp.4001957
Henriksson, B. G., Soderstrom, S., Gower, A. J., Ebendal, T., Winblad, B., and Mohammed, A. H. (1992). Hippocampal nerve growth factor levels are related to spatial learning ability in aged rats. Behav. Brain Res. 48, 15–20. doi: 10.1016/S0166-4328(05)80134-2
Hensch, T. K., and Fagiolini, M. (2005). Excitatory-inhibitory balance and critical period plasticity in developing visual cortex. Prog. Brain Res. 147, 115–124. doi: 10.1016/S0079-6123(04)47009-5
Hensch, T. K., Fagiolini, M., Mataga, N., Stryker, M. P., Baekkeskov, S., and Kash, S. F. (1998). Local GABA circuit control of experience-dependent plasticity in developing visual cortex. Science 282, 1504–1508. doi: 10.1126/science.282.5393.1504
Hicks, R. R., Numan, S., Dhillon, H. S., Prasad, M. R., and Seroogy, K. B. (1997). Alterations in BDNF and NT-3 mRNAs in rat hippocampus after experimental brain trauma. Brain Res. Mol. Brain Res. 48, 401–406. doi: 10.1016/S0169-328X(97)00158-7
Hicks, R. R., Smith, D. H., Lowenstein, D. H., Saint Marie, R., and Mcintosh, T. K. (1993). Mild experimental brain injury in the rat induces cognitive deficits associated with regional neuronal loss in the hippocampus. J. Neurotrauma 10, 405–414. doi: 10.1089/neu.1993.10.405
Hicks, R. R., Zhang, L., Atkinson, A., Stevenon, M., Veneracion, M., and Seroogy, K. B. (2002). Environmental enrichment attenuates cognitive deficits, but does not alter neurotrophin gene expression in the hippocampus following lateral fluid percussion brain injury. Neuroscience 112, 631–637. doi: 10.1016/S0306-4522(02)00104-5
Hockly, E., Cordery, P. M., Woodman, B., Mahal, A., Van Dellen, A., Blakemore, C., et al. (2002). Environmental enrichment slows disease progression in R6/2 Huntington's disease mice. Ann. Neurol. 51, 235–242. doi: 10.1002/ana.10094
Hoffman, A. N., Malena, R. R., Westergom, B. P., Luthra, P., Cheng, J. P., Aslam, H. A., et al. (2008). Environmental enrichment-mediated functional improvement after experimental traumatic brain injury is contingent on task-specific neurobehavioral experience. Neurosci. Lett. 431, 226–230. doi: 10.1016/j.neulet.2007.11.042
Holloway, R. L. Jr. (1966). Dendritic branching: some preliminary results of training and complexity in rat visual cortex. Brain Res. 2, 393–396.
Hu, Y. S., Xu, P., Pigino, G., Brady, S. T., Larson, J., and Lazarov, O. (2010). Complex environment experience rescues impaired neurogenesis, enhances synaptic plasticity, and attenuates neuropathology in familial Alzheimer's disease-linked APPswe/PS1DeltaE9 mice. FASEB J. 24, 1667–1681. doi: 10.1096/fj.09-136945
Huang, Z. J., Kirkwood, A., Pizzorusso, T., Porciatti, V., Morales, B., Bear, M. F., et al. (1999). BDNF regulates the maturation of inhibition and the critical period of plasticity in mouse visual cortex. Cell 98, 739–755. doi: 10.1016/S0092-8674(00)81509-3
Hubel, D. H. (1978). Effects of deprivation on the visual cortex of cat and monkey. Harvey Lect. 72, 1–51.
Hubel, D. H., and Wiesel, T. N. (1970). The period of susceptibility to the physiological effects of unilateral eye closure in kittens. J. Physiol. 206, 419–436.
Hunt, R. F., Boychuk, J. A., and Smith, B. N. (2013). Neural circuit mechanisms of post-traumatic epilepsy. Front. Cell. Neurosci. 7:89. doi: 10.3389/fncel.2013.00089
Ip, E. Y., Giza, C. C., Griesbach, G. S., and Hovda, D. A. (2002). Effects of enriched environment and fluid percussion injury on dendritic arborization within the cerebral cortex of the developing rat. J. Neurotrauma 19, 573–585. doi: 10.1089/089771502753754055
Irvine, G. I., and Abraham, W. C. (2005). Enriched environment exposure alters the input-output dynamics of synaptic transmission in area CA1 of freely moving rats. Neurosci. Lett. 391, 32–37. doi: 10.1016/j.neulet.2005.08.031
Irvine, G. I., Logan, B., Eckert, M., and Abraham, W. C. (2006). Enriched environment exposure regulates excitability, synaptic transmission, and LTP in the dentate gyrus of freely moving rats. Hippocampus 16, 149–160. doi: 10.1002/hipo.20142
Jadavji, N. M., Kolb, B., and Metz, G. A. (2006). Enriched environment improves motor function in intact and unilateral dopamine-depleted rats. Neuroscience 140, 1127–1138. doi: 10.1016/j.neuroscience.2006.03.027
Jakkamsetti, V., Chang, K. Q., and Kilgard, M. P. (2012). Reorganization in processing of spectral and temporal input in the rat posterior auditory field induced by environmental enrichment. J. Neurophysiol. 107, 1457–1475. doi: 10.1152/jn.01057.2010
Jankowsky, J. L., Melnikova, T., Fadale, D. J., Xu, G. M., Slunt, H. H., Gonzales, V., et al. (2005). Environmental enrichment mitigates cognitive deficits in a mouse model of Alzheimer's disease. J. Neurosci. 25, 5217–5224. doi: 10.1523/JNEUROSCI.5080-04.2005
Jankowsky, J. L., Xu, G., Fromholt, D., Gonzales, V., and Borchelt, D. R. (2003). Environmental enrichment exacerbates amyloid plaque formation in a transgenic mouse model of Alzheimer disease. J. Neuropathol. Exp. Neurol. 62, 1220–1227.
Johansson, B. B., and Belichenko, P. V. (2002). Neuronal plasticity and dendritic spines: effect of environmental enrichment on intact and postischemic rat brain. J. Cereb. Blood Flow Metab. 22, 89–96. doi: 10.1097/00004647-200201000-00011
Johansson, B. B., and Ohlsson, A. L. (1996). Environment, social interaction, and physical activity as determinants of functional outcome after cerebral infarction in the rat. Exp. Neurol. 139, 322–327. doi: 10.1006/exnr.1996.0106
Johnson, E. M., Traver, K. L., Hoffman, S. W., Harrison, C. R., and Herman, J. P. (2013). Environmental enrichment protects against functional deficits caused by traumatic brain injury. Front. Behav. Neurosci. 7:44. doi: 10.3389/fnbeh.2013.00044
Johnstone, V. P., Yan, E. B., Alwis, D. S., and Rajan, R. (2013). Cortical hypoexcitation defines neuronal responses in the immediate aftermath of traumatic brain injury. PLoS ONE 8:e63454. doi: 10.1371/journal.pone.0063454
Jones, T. A., Klintsova, A. Y., Kilman, V. L., Sirevaag, A. M., and Greenough, W. T. (1997). Induction of multiple synapses by experience in the visual cortex of adult rats. Neurobiol. Learn. Mem. 68, 13–20. doi: 10.1006/nlme.1997.3774
Jung, C. K., and Herms, J. (2012). Structural dynamics of dendritic spines are influenced by an environmental enrichment: an in vivo imaging study. Cereb. Cortex 24, 377–384. doi: 10.1093/cercor/bhs31
Kaas, J. H. (1991). Plasticity of sensory and motor maps in adult mammals. Annu. Rev. Neurosci. 14, 137–167. doi: 10.1146/annurev.ne.14.030191.001033
Kang, H., and Schuman, E. M. (1995). Long-lasting neurotrophin-induced enhancement of synaptic transmission in the adult hippocampus. Science 267, 1658–1662. doi: 10.1126/science.7886457
Kazlauckas, V., Pagnussat, N., Mioranzza, S., Kalinine, E., Nunes, F., Pettenuzzo, L., et al. (2011). Enriched environment effects on behavior, memory and BDNF in low and high exploratory mice. Physiol. Behav. 102, 475–480. doi: 10.1016/j.physbeh.2010.12.025
Kelche, C., and Will, B. (1982). Effects of postoperative environments following dorsal hippocampal lesions on dendritic branching and spines in rat occipital cortex. Brain Res. 245, 107–115.
Kempermann, G. (2002). Why new neurons? Possible functions for adult hippocampal neurogenesis. J. Neurosci. 22, 635–638.
Kempermann, G., Kuhn, H. G., and Gage, F. H. (1997). More hippocampal neurons in adult mice living in an enriched environment. Nature 386, 493–495. doi: 10.1038/386493a0
Kempermann, G., Kuhn, H. G., and Gage, F. H. (1998). Experience-induced neurogenesis in the senescent dentate gyrus. J. Neurosci. 18, 3206–3212.
Kendrick, K. M., Levy, F., and Keverne, E. B. (1992). Changes in the sensory processing of olfactory signals induced by birth in sheep. Science 256, 833–836. doi: 10.1126/science.1589766
Keyvani, K., Sachser, N., Witte, O. W., and Paulus, W. (2004). Gene expression profiling in the intact and injured brain following environmental enrichment. J. Neuropathol. Exp. Neurol. 63, 598–609.
Kim, B. G., Dai, H. N., Mcatee, M., and Bregman, B. S. (2008). Modulation of dendritic spine remodeling in the motor cortex following spinal cord injury: effects of environmental enrichment and combinatorial treatment with transplants and neurotrophin-3. J. Comp. Neurol. 508, 473–486. doi: 10.1002/cne.21686
Kim, H., and Bao, S. (2013). Experience-dependent over-representation of ultrasonic vocalization frequencies in the rat primary auditory cortex. J. Neurophysiol. 110, 1087–1096. doi: 10.1152/jn.00230.2013
Kim, M., Na, D. L., Kim, G. M., Adair, J. C., Lee, K. H., and Heilman, K. M. (1999). Ipsilesional neglect: behavioural and anatomical features. J. Neurol. Neurosurg. Psychiatr. 67, 35–38. doi: 10.1136/jnnp.67.1.35
Kleim, J. A., Jones, T. A., and Schallert, T. (2003). Motor enrichment and the induction of plasticity before or after brain injury. Neurochem. Res. 28, 1757–1769. doi: 10.1023/A:1026025408742
Kline, A. E., Mcaloon, R. L., Henderson, K. A., Bansal, U. K., Ganti, B. M., Ahmed, R. H., et al. (2010). Evaluation of a combined therapeutic regimen of 8-OH-DPAT and environmental enrichment after experimental traumatic brain injury. J. Neurotrauma 27, 2021–2032. doi: 10.1089/neu.2010.1535
Kline, A. E., Olsen, A. S., Sozda, C. N., Hoffman, A. N., and Cheng, J. P. (2012). Evaluation of a combined treatment paradigm consisting of environmental enrichment and the 5-HT1A receptor agonist buspirone after experimental traumatic brain injury. J. Neurotrauma 29, 1960–1969. doi: 10.1089/neu.2012.2385
Kline, A. E., Wagner, A. K., Westergom, B. P., Malena, R. R., Zafonte, R. D., Olsen, A. S., et al. (2007). Acute treatment with the 5-HT(1A) receptor agonist 8-OH-DPAT and chronic environmental enrichment confer neurobehavioral benefit after experimental brain trauma. Behav. Brain Res. 177, 186–194. doi: 10.1016/j.bbr.2006.11.036
Klintsova, A. Y., and Greenough, W. T. (1999). Synaptic plasticity in cortical systems. Curr. Opin. Neurobiol. 9, 203–208. doi: 10.1016/S0959-4388(99)80028-2
Koh, S., Magid, R., Chung, H., Stine, C. D., and Wilson, D. N. (2007). Depressive behavior and selective down-regulation of serotonin receptor expression after early-life seizures: reversal by environmental enrichment. Epilepsy Behav. 10, 26–31. doi: 10.1016/j.yebeh.2006.11.008
Kolb, B. (1999). Synaptic plasticity and the organization of behaviour after early and late brain injury. Can. J. Exp. Psychol. 53, 62–76. doi: 10.1037/h0087300
Kolb, B., and Gibb, R. (1991). Environmental enrichment and cortical injury: behavioral and anatomical consequences of frontal cortex lesions. Cereb. Cortex 1, 189–198. doi: 10.1093/cercor/1.2.189
Kolb, B., Ladowski, R., Gibb, R., and Gorny, G. (1996). Does dendritic growth underly recovery from neonatal occipital lesions in rats. Behav. Brain Res. 77, 125–133. doi: 10.1016/0166-4328(95)00208-1
Komitova, M., Perfilieva, E., Mattsson, B., Eriksson, P. S., and Johansson, B. B. (2006). Enriched environment after focal cortical ischemia enhances the generation of astroglia and NG2 positive polydendrocytes in adult rat neocortex. Exp. Neurol. 199, 113–121. doi: 10.1016/j.expneurol.2005.12.007
Koopmans, G. C., Deumens, R., Honig, W. M., Hamers, F. P., Mey, J., Van Kleef, M., et al. (2012). Functional recovery, serotonergic sprouting, and endogenous progenitor fates in response to delayed environmental enrichment after spinal cord injury. J. Neurotrauma 29, 514–527. doi: 10.1089/neu.2011.1949
Korbey, S. M., Heinrichs, S. C., and Leussis, M. P. (2008). Seizure susceptibility and locus ceruleus activation are reduced following environmental enrichment in an animal model of epilepsy. Epilepsy Behav. 12, 30–38. doi: 10.1016/j.yebeh.2007.09.013
Kozlowski, D. A., Nahed, B. V., Hovda, D. A., and Lee, S. M. (2004). Paradoxical effects of cortical impact injury on environmentally enriched rats. J. Neurotrauma 21, 513–519. doi: 10.1089/089771504774129856
Kramer, A. F., Bherer, L., Colcombe, S. J., Dong, W., and Greenough, W. T. (2004). Environmental influences on cognitive and brain plasticity during aging. J. Gerontol. A Biol. Sci. Med. Sci. 59, M940–M957. doi: 10.1093/gerona/59.9.M940
Kulesskaya, N., Rauvala, H., and Voikar, V. (2011). Evaluation of social and physical enrichment in modulation of behavioural phenotype in C57BL/6J female mice. PLoS ONE 6:e24755. doi: 10.1371/journal.pone.0024755
Landers, M. S., Knott, G. W., Lipp, H. P., Poletaeva, I., and Welker, E. (2011). Synapse formation in adult barrel cortex following naturalistic environmental enrichment. Neuroscience 199, 143–152. doi: 10.1016/j.neuroscience.2011.10.040
Larsson, F., Winblad, B., and Mohammed, A. H. (2002). Psychological stress and environmental adaptation in enriched vs. impoverished housed rats. Pharmacol. Biochem. Behav. 73, 193–207. doi: 10.1016/S0091-3057(02)00782-7
Lazarov, O., Robinson, J., Tang, Y. P., Hairston, I. S., Korade-Mirnics, Z., Lee, V. M., et al. (2005). Environmental enrichment reduces Abeta levels and amyloid deposition in transgenic mice. Cell 120, 701–713. doi: 10.1016/j.cell.2005.01.015
Lazic, S. E., Grote, H. E., Blakemore, C., Hannan, A. J., Van Dellen, A., Phillips, W., et al. (2006). Neurogenesis in the R6/1 transgenic mouse model of Huntington's disease: effects of environmental enrichment. Eur. J. Neurosci. 23, 1829–1838. doi: 10.1111/j.1460-9568.2006.04715.x
Leger, M., Quiedeville, A., Paizanis, E., Natkunarajah, S., Freret, T., Boulouard, M., et al. (2012). Environmental enrichment enhances episodic-like memory in association with a modified neuronal activation profile in adult mice. PLoS ONE 7:e48043. doi: 10.1371/journal.pone.0048043
Leggio, M. G., Mandolesi, L., Federico, F., Spirito, F., Ricci, B., Gelfo, F., et al. (2005). Environmental enrichment promotes improved spatial abilities and enhanced dendritic growth in the rat. Behav. Brain Res. 163, 78–90. doi: 10.1016/j.bbr.2005.04.009
Levelt, C. N., and Hubener, M. (2012). Critical-period plasticity in the visual cortex. Annu. Rev. Neurosci. 35, 309–330. doi: 10.1146/annurev-neuro-061010-113813
Levi, O., Jongen-Relo, A. L., Feldon, J., Roses, A. D., and Michaelson, D. M. (2003). ApoE4 impairs hippocampal plasticity isoform-specifically and blocks the environmental stimulation of synaptogenesis and memory. Neurobiol. Dis. 13, 273–282. doi: 10.1016/S0969-9961(03)00045-7
Levi, O., and Michaelson, D. M. (2007). Environmental enrichment stimulates neurogenesis in apolipoprotein E3 and neuronal apoptosis in apolipoprotein E4 transgenic mice. J. Neurochem. 100, 202–210. doi: 10.1111/j.1471-4159.2006.04189.x
Lew, H. L., Weihing, J., Myers, P. J., Pogoda, T. K., and Goodrich, G. L. (2010). Dual sensory impairment (DSI) in traumatic brain injury (TBI)–An emerging interdisciplinary challenge. Neurorehabilitation 26, 213–222. doi: 10.3233/NRE-2010-0557
Liljequist, R., Henriksson, B. G., Latif, N., Pham, T., Winblad, B., and Mohammed, A. H. (1993). Subchronic MK-801 treatment to juvenile rats attenuates environmental effects on adult spatial learning. Behav. Brain Res. 56, 107–114. doi: 10.1016/0166-4328(93)90027-N
Linnarsson, S., Bjorklund, A., and Ernfors, P. (1997). Learning deficit in BDNF mutant mice. Eur. J. Neurosci. 9, 2581–2587. doi: 10.1111/j.1460-9568.1997.tb01687.x
Lippert-Gruener, M., Maegele, M., Garbe, J., and Angelov, D. N. (2007). Late effects of enriched environment (EE) plus multimodal early onset stimulation (MEOS) after traumatic brain injury in rats: ongoing improvement of neuromotor function despite sustained volume of the CNS lesion. Exp. Neurol. 203, 82–94. doi: 10.1016/j.expneurol.2006.07.025
Lippert-Gruner, M., Maegele, M., Pokorny, J., Angelov, D. N., Svestkova, O., Wittner, M., et al. (2007). Early rehabilitation model shows positive effects on neural degeneration and recovery from neuromotor deficits following traumatic brain injury. Physiol. Res. 56, 359–368.
Lippert-Gruner, M., Magele, M., Svestkova, O., Angerova, Y., Ester-Bode, T., and Angelov, D. N. (2011). Rehabilitation intervention in animal model can improve neuromotor and cognitive functions after traumatic brain injury: pilot study. Physiol. Res. 60, 367–375.
Little, D. M., Kraus, M. F., Joseph, J., Geary, E. K., Susmaras, T., Zhou, X. J., et al. (2010). Thalamic integrity underlies executive dysfunction in traumatic brain injury. Neurology 74, 558–564. doi: 10.1212/WNL.0b013e3181cff5d5
Liu, Z., D'amore, P. A., Mikati, M., Gatt, A., and Holmes, G. L. (1993). Neuroprotective effect of chronic infusion of basic fibroblast growth factor on seizure-associated hippocampal damage. Brain Res. 626, 335–338.
Lu, L., Bao, G., Chen, H., Xia, P., Fan, X., Zhang, J., et al. (2003). Modification of hippocampal neurogenesis and neuroplasticity by social environments. Exp. Neurol. 183, 600–609. doi: 10.1016/S0014-4886(03)00248-6
Luz, Y., and Shamir, M. (2012). Balancing feed-forward excitation and inhibition via Hebbian inhibitory synaptic plasticity. PLoS Comput. Biol. 8:e1002334. doi: 10.1371/journal.pcbi.1002334
Maegele, M., Lippert-Gruener, M., Ester-Bode, T., Garbe, J., Bouillon, B., Neugebauer, E., et al. (2005a). Multimodal early onset stimulation combined with enriched environment is associated with reduced CNS lesion volume and enhanced reversal of neuromotor dysfunction after traumatic brain injury in rats. Eur. J. Neurosci. 21, 2406–2418. doi: 10.1111/j.1460-9568.2005.04070.x
Maegele, M., Lippert-Gruener, M., Ester-Bode, T., Sauerland, S., Schafer, U., Molcanyi, M., et al. (2005b). Reversal of neuromotor and cognitive dysfunction in an enriched environment combined with multimodal early onset stimulation after traumatic brain injury in rats. J. Neurotrauma 22, 772–782. doi: 10.1089/neu.2005.22.772
Mainardi, M., Landi, S., Gianfranceschi, L., Baldini, S., De Pasquale, R., Berardi, N., et al. (2010). Environmental enrichment potentiates thalamocortical transmission and plasticity in the adult rat visual cortex. J. Neurosci. Res. 88, 3048–3059. doi: 10.1002/jnr.22461
Malik, R., and Chattarji, S. (2012). Enhanced intrinsic excitability and EPSP-spike coupling accompany enriched environment-induced facilitation of LTP in hippocampal CA1 pyramidal neurons. J. Neurophysiol. 107, 1366–1378. doi: 10.1152/jn.01009.2011
Mandairon, N., Stack, C., Kiselycznyk, C., and Linster, C. (2006a). Enrichment to odors improves olfactory discrimination in adult rats. Behav. Neurosci. 120, 173–179. doi: 10.1037/0735-7044.120.1.173
Mandairon, N., Stack, C., and Linster, C. (2006b). Olfactory enrichment improves the recognition of individual components in mixtures. Physiol. Behav. 89, 379–384. doi: 10.1016/j.physbeh.2006.07.013
Matter, A. M., Folweiler, K. A., Curatolo, L. M., and Kline, A. E. (2011). Temporal effects of environmental enrichment-mediated functional improvement after experimental traumatic brain injury in rats. Neurorehabil. Neural Repair 25, 558–564. doi: 10.1177/1545968310397206
Maya-Vetencourt, J. F., Baroncelli, L., Viegi, A., Tiraboschi, E., Castren, E., Cattaneo, A., et al. (2012). IGF-1 restores visual cortex plasticity in adult life by reducing local GABA levels. Neural Plast. 2012:250421. doi: 10.1155/2012/250421
Maya Vetencourt, J. F., Sale, A., Viegi, A., Baroncelli, L., De Pasquale, R., O'Leary, O. F., et al. (2008). The antidepressant fluoxetine restores plasticity in the adult visual cortex. Science 320, 385–388. doi: 10.1126/science.1150516
McNamara, K. C. S., Lisembee, A. M., and Lifshitz, J. (2010). The whisker nuisance task identifies a late-onset, persistent sensory sensitivity in diffuse brain-injured rats. J. Neurotrauma 27, 695–706. doi: 10.1089/neu.2009.1237
McQuaid, R. J., Audet, M. C., and Anisman, H. (2012). Environmental enrichment in male CD-1 mice promotes aggressive behaviors and elevated corticosterone and brain norepinephrine activity in response to a mild stressor. Stress 15, 354–360. doi: 10.3109/10253890.2011.623249
Megevand, P., Troncoso, E., Quairiaux, C., Muller, D., Michel, C. M., and Kiss, J. Z. (2009). Long-term plasticity in mouse sensorimotor circuits after rhythmic whisker stimulation. J. Neurosci. 29, 5326–5335. doi: 10.1523/JNEUROSCI.5965-08.2009
Menge, T., Jander, S., and Stoll, G. (2001). Induction of the proinflammatory cytokine interleukin-18 by axonal injury. J. Neurosci. Res. 65, 332–339. doi: 10.1002/jnr.1158
Mesa-Gresa, P., Perez-Martinez, A., and Redolat, R. (2013). Environmental enrichment improves novel object recognition and enhances agonistic behavior in male mice. Aggress. Behav. 39, 269–279. doi: 10.1002/ab.21481
Milgram, N. W., Siwak-Tapp, C. T., Araujo, J., and Head, E. (2006). Neuroprotective effects of cognitive enrichment. Ageing Res. Rev. 5, 354–369. doi: 10.1016/j.arr.2006.04.004
Miller, L. S., Colella, B., Mikulis, D., Maller, J., and Green, R. E. (2013). Environmental enrichment may protect against hippocampal atrophy in the chronic stages of traumatic brain injury. Front. Hum. Neurosci. 7:506. doi: 10.3389/fnhum.2013.00506
Minichiello, L., Korte, M., Wolfer, D., Kuhn, R., Unsicker, K., Cestari, V., et al. (1999). Essential role for TrkB receptors in hippocampus-mediated learning. Neuron 24, 401–414. doi: 10.1016/S0896-6273(00)80853-3
Mohammed, A. H., Zhu, S. W., Darmopil, S., Hjerling-Leffler, J., Ernfors, P., Winblad, B., et al. (2002). Environmental enrichment and the brain. Prog. Brain Res. 138, 109–133. doi: 10.1016/S0079-6123(02)38074-9
Monaco, C. M., Mattiola, V. V., Folweiler, K. A., Tay, J. K., Yelleswarapu, N. K., Curatolo, L. M., et al. (2013). Environmental enrichment promotes robust functional and histological benefits in female rats after controlled cortical impact injury. Exp. Neurol. 247, 410–418. doi: 10.1016/j.expneurol.2013.01.007
Morganti-Kossmann, M. C., Rancan, M., Stahel, P. F., and Kossmann, T. (2002). Inflammatory response in acute traumatic brain injury: a double-edged sword. Curr. Opin. Crit. Care 8, 101–105. doi: 10.1097/00075198-200204000-00002
Mrsic-Flogel, T. D., Hofer, S. B., Ohki, K., Reid, R. C., Bonhoeffer, T., and Hubener, M. (2007). Homeostatic regulation of eye-specific responses in visual cortex during ocular dominance plasticity. Neuron 54, 961–972. doi: 10.1016/j.neuron.2007.05.028
Muthuraju, S., Pati, S., Rafiqul, M., Abdullah, J. M., and Jaafar, H. (2012). IntelliCage provides voluntary exercise and an enriched environment, improving locomotive activity in mice following fluid percussion injury. Basal Ganglia 2, 143–151. doi: 10.1016/j.baga.2012.06.004
Naka, F., Narita, N., Okado, N., and Narita, M. (2005). Modification of AMPA receptor properties following environmental enrichment. Brain Dev. 27, 275–278. doi: 10.1016/j.braindev.2004.07.006
Narayan, R. K., Michel, M. E., Ansell, B., Baethmann, A., Biegon, A., Bracken, M. B., et al. (2002). Clinical trials in head injury. J. Neurotrauma 19, 503–557. doi: 10.1089/089771502753754037
Newson, R. S., and Kemps, E. B. (2005). General lifestyle activities as a predictor of current cognition and cognitive change in older adults: a cross-sectional and longitudinal examination. J. Gerontol. B Psychol. Sci. Soc. Sci. 60, P113–P120. doi: 10.1093/geronb/60.3.P113
Nichols, J. A., Jakkamsetti, V. P., Salgado, H., Dinh, L., Kilgard, M. P., and Atzori, M. (2007). Environmental enrichment selectively increases glutamatergic responses in layer II/III of the auditory cortex of the rat. Neuroscience 145, 832–840. doi: 10.1016/j.neuroscience.2006.12.061
Nilsson, M., Perfilieva, E., Johansson, U., Orwar, O., and Eriksson, P. S. (1999). Enriched environment increases neurogenesis in the adult rat dentate gyrus and improves spatial memory. J. Neurobiol. 39, 569–578.
Nithianantharajah, J., Barkus, C., Murphy, M., and Hannan, A. J. (2008). Gene-environment interactions modulating cognitive function and molecular correlates of synaptic plasticity in Huntington's disease transgenic mice. Neurobiol. Dis. 29, 490–504. doi: 10.1016/j.nbd.2007.11.006
Nithianantharajah, J., and Hannan, A. J. (2006). Enriched environments, experience-dependent plasticity and disorders of the nervous system. Nat. Rev. Neurosci. 7, 697–709. doi: 10.1038/nrn1970
Nithianantharajah, J., Levis, H., and Murphy, M. (2004). Environmental enrichment results in cortical and subcortical changes in levels of synaptophysin and PSD-95 proteins. Neurobiol. Learn. Mem. 81, 200–210. doi: 10.1016/j.nlm.2004.02.002
Norena, A. J., Gourevitch, B., Aizawa, N., and Eggermont, J. J. (2006). Spectrally enhanced acoustic environment disrupts frequency representation in cat auditory cortex. Nat. Neurosci. 9, 932–939. doi: 10.1038/nn1720
Ohlsson, A. L., and Johansson, B. B. (1995). Environment influences functional outcome of cerebral infarction in rats. Stroke 26, 644–649. doi: 10.1161/01.STR.26.4.644
Ortuzar, N., Rico-Barrio, I., Bengoetxea, H., Argandona, E. G., and Lafuente, J. V. (2013). VEGF reverts the cognitive impairment induced by a focal traumatic brain injury during the development of rats raised under environmental enrichment. Behav. Brain Res. 246, 36–46. doi: 10.1016/j.bbr.2013.02.036
Park, E., Bell, J. D., and Baker, A. J. (2008). Traumatic brain injury: can the consequences be stopped? CMAJ 178, 1163–1170. doi: 10.1503/cmaj.080282
Park, N. W., and Ingles, J. L. (2001). Effectiveness of attention rehabilitation after an acquired brain injury: a meta-analysis. Neuropsychology 15, 199–210. doi: 10.1037/0894-4105.15.2.199
Passineau, M. J., Green, E. J., and Dietrich, W. D. (2001). Therapeutic effects of environmental enrichment on cognitive function and tissue integrity following severe traumatic brain injury in rats. Exp. Neurol. 168, 373–384. doi: 10.1006/exnr.2000.7623
Patel, R., Ciuffreda, K. J., Tannen, B., and Kapoor, N. (2011). Elevated coherent motion thresholds in mild traumatic brain injury. Optometry 82, 284–289. doi: 10.1016/j.optm.2010.10.012
Percaccio, C. R., Engineer, N. D., Pruette, A. L., Pandya, P. K., Moucha, R., Rathbun, D. L., et al. (2005). Environmental enrichment increases paired-pulse depression in rat auditory cortex. J. Neurophysiol. 94, 3590–3600. doi: 10.1152/jn.00433.2005
Percaccio, C. R., Pruette, A. L., Mistry, S. T., Chen, Y. H., and Kilgard, M. P. (2007). Sensory experience determines enrichment-induced plasticity in rat auditory cortex. Brain Res. 1174, 76–91. doi: 10.1016/j.brainres.2007.07.062
Pereira, L. O., Arteni, N. S., Petersen, R. C., Da Rocha, A. P., Achaval, M., and Netto, C. A. (2007). Effects of daily environmental enrichment on memory deficits and brain injury following neonatal hypoxia-ischemia in the rat. Neurobiol. Learn. Mem. 87, 101–108. doi: 10.1016/j.nlm.2006.07.003
Pham, T. M., Ickes, B., Albeck, D., Soderstrom, S., Granholm, A. C., and Mohammed, A. H. (1999). Changes in brain nerve growth factor levels and nerve growth factor receptors in rats exposed to environmental enrichment for one year. Neuroscience 94, 279–286. doi: 10.1016/S0306-4522(99)00316-4
Pienkowski, M., and Eggermont, J. J. (2009). Long-term, partially-reversible reorganization of frequency tuning in mature cat primary auditory cortex can be induced by passive exposure to moderate-level sounds. Hear. Res. 257, 24–40. doi: 10.1016/j.heares.2009.07.011
Pierce, J. E., Smith, D. H., Trojanowski, J. Q., and McIntosh, T. K. (1998). Enduring cognitive, neurobehavioral and histopathological changes persist for up to one year following severe experimental brain injury in rats. Neuroscience 87, 359–369. doi: 10.1016/S0306-4522(98)00142-0
Pitkanen, A., and McIntosh, T. K. (2006). Animal models of post-traumatic epilepsy. J. Neurotrauma 23, 241–261. doi: 10.1089/neu.2006.23.241
Polley, D. B., Kvasnak, E., and Frostig, R. D. (2004). Naturalistic experience transforms sensory maps in the adult cortex of caged animals. Nature 429, 67–71. doi: 10.1038/nature02469
Por, S. B., Bennett, E. L., and Bondy, S. C. (1982). Environmental enrichment and neurotransmitter receptors. Behav. Neural Biol. 34, 132–140. doi: 10.1016/S0163-1047(82)91514-X
Powell, J., Heslin, J., and Greenwood, R. (2002). Community based rehabilitation after severe traumatic brain injury: a randomised controlled trial. J. Neurol. Neurosurg. Psychiatry 72, 193–202. doi: 10.1136/jnnp.72.2.193
Rampon, C., Jiang, C. H., Dong, H., Tang, Y. P., Lockhart, D. J., Schultz, P. G., et al. (2000). Effects of environmental enrichment on gene expression in the brain. Proc. Natl. Acad. Sci. U.S.A. 97, 12880–12884. doi: 10.1073/pnas.97.23.12880
Rasmuson, S., Olsson, T., Henriksson, B. G., Kelly, P. A., Holmes, M. C., Seckl, J. R., et al. (1998). Environmental enrichment selectively increases 5-HT1A receptor mRNA expression and binding in the rat hippocampus. Brain Res. Mol. Brain Res. 53, 285–290.
Recanzone, G. H., Schreiner, C. E., and Merzenich, M. M. (1993). Plasticity in the frequency representation of primary auditory cortex following discrimination training in adult owl monkeys. J. Neurosci. 13, 87–103.
Reibel, S., Larmet, Y., Le, B. T., Carnahan, J., Marescaux, C., and Depaulis, A. (2000). Brain-derived neurotrophic factor delays hippocampal kindling in the rat. Neuroscience 100, 777–788. doi: 10.1016/S0306-4522(00)00351-1
Rema, V., Armstrong-James, M., Jenkinson, N., and Ebner, F. F. (2006). Short exposure to an enriched environment accelerates plasticity in the barrel cortex of adult rats. Neuroscience 140, 659–672. doi: 10.1016/j.neuroscience.2006.02.043
Risdall, J. E., and Menon, D. K. (2011). Traumatic brain injury. Philos. Trans. R. Soc. Lond. B. Biol. Sci. 366, 241–250. doi: 10.1098/rstb.2010.0230
Risedal, A., Mattsson, B., Dahlqvist, P., Nordborg, C., Olsson, T., and Johansson, B. B. (2002). Environmental influences on functional outcome after a cortical infarct in the rat. Brain Res. Bull. 58, 315–321. doi: 10.1016/S0361-9230(02)00796-7
Rojas, J. J., Deniz, B. F., Miguel, P. M., Diaz, R., Hermel Edo, E., Achaval, M., et al. (2013). Effects of daily environmental enrichment on behavior and dendritic spine density in hippocampus following neonatal hypoxia-ischemia in the rat. Exp. Neurol. 241, 25–33. doi: 10.1016/j.expneurol.2012.11.026
Rose, F. D., Al-Khamees, K., Davey, M. J., and Attree, E. A. (1993). Environmental enrichment following brain damage: an aid to recovery or compensation? Behav. Brain Res. 56, 93–100.
Rose, F. D., Davey, M. J., Love, S., and Dell, P. A. (1987). Environmental enrichment and recovery from contralateral sensory neglect in rats with large unilateral neocortical lesions. Behav. Brain Res. 24, 195–202. doi: 10.1016/0166-4328(87)90057-X
Rose, F. D., Dell, P. A., Love, S., and Davey, M. J. (1988). Environmental enrichment and recovery from a complex Go/No-Go reversal deficit in rats following large unilateral neocortical lesions. Behav. Brain Res. 31, 37–45. doi: 10.1016/0166-4328(88)90156-8
Rosenzweig, M. R., Bennett, E. L., Hebert, M., and Morimoto, H. (1978). Social grouping cannot account for cerebral effects of enriched environments. Brain Res. 153, 563–576.
Rossi, C., Angelucci, A., Costantin, L., Braschi, C., Mazzantini, M., Babbini, F., et al. (2006). Brain-derived neurotrophic factor (BDNF) is required for the enhancement of hippocampal neurogenesis following environmental enrichment. Eur. J. Neurosci. 24, 1850–1856. doi: 10.1111/j.1460-9568.2006.05059.x
Rowntree, S., and Kolb, B. (1997). Blockade of basic fibroblast growth factor retards recovery from motor cortex injury in rats. Eur. J. Neurosci. 9, 2432–2441. doi: 10.1111/j.1460-9568.1997.tb01660.x
Rubinov, M., McIntosh, A. R., Valenzuela, M. J., and Breakspear, M. (2009). Simulation of neuronal death and network recovery in a computational model of distributed cortical activity. Am. J. Geriatr. Psychiatry 17, 210–217. doi: 10.1097/JGP.0b013e318187137a
Rutten, A., Van Albada, M., Silveira, D. C., Cha, B. H., Liu, X., Hu, Y. N., et al. (2002). Memory impairment following status epilepticus in immature rats: time-course and environmental effects. Eur. J. Neurosci. 16, 501–513. doi: 10.1046/j.1460-9568.2002.02103.x
Sale, A., Maya Vetencourt, J. F., Medini, P., Cenni, M. C., Baroncelli, L., De Pasquale, R., et al. (2007). Environmental enrichment in adulthood promotes amblyopia recovery through a reduction of intracortical inhibition. Nat. Neurosci. 10, 679–681. doi: 10.1038/nn1899
Scali, M., Baroncelli, L., Cenni, M. C., Sale, A., and Maffei, L. (2012). A rich environmental experience reactivates visual cortex plasticity in aged rats. Exp. Gerontol. 47, 337–341. doi: 10.1016/j.exger.2012.01.007
Scarmeas, N., and Stern, Y. (2003). Cognitive reserve and lifestyle. J. Clin. Exp. Neuropsychol. 25, 625–633. doi: 10.1076/jcen.25.5.625.14576
Schrijver, N. C., Bahr, N. I., Weiss, I. C., and Wurbel, H. (2002). Dissociable effects of isolation rearing and environmental enrichment on exploration, spatial learning and HPA activity in adult rats. Pharmacol. Biochem. Behav. 73, 209–224. doi: 10.1016/S0091-3057(02)00790-6
Seeman, T. E., Lusignolo, T. M., Albert, M., and Berkman, L. (2001). Social relationships, social support, and patterns of cognitive aging in healthy, high-functioning older adults: MacArthur studies of successful aging. Health Psychol. 20, 243–255. doi: 10.1037/0278-6133.20.4.243
Segovia, G., Yague, A. G., Garcia-Verdugo, J. M., and Mora, F. (2006). Environmental enrichment promotes neurogenesis and changes the extracellular concentrations of glutamate and GABA in the hippocampus of aged rats. Brain Res. Bull. 70, 8–14. doi: 10.1016/j.brainresbull.2005.11.005
Sharp, P. E., McNaughton, B. L., and Barnes, C. A. (1985). Enhancement of hippocampal field potentials in rats exposed to a novel, complex environment. Brain Res. 339, 361–365.
Shiel, A., Burn, J. P., Henry, D., Clark, J., Wilson, B. A., Burnett, M. E., et al. (2001). The effects of increased rehabilitation therapy after brain injury: results of a prospective controlled trial. Clin. Rehabil. 15, 501–514. doi: 10.1191/026921501680425225
Shultz, S. R., Cardamone, L., Liu, Y. R., Hogan, R. E., Maccotta, L., Wright, D. K., et al. (2013). Can structural or functional changes following traumatic brain injury in the rat predict epileptic outcome? Epilepsia 54, 1240–1250. doi: 10.1111/epi.12223
Simpson, J., and Kelly, J. P. (2011). The impact of environmental enrichment in laboratory rats–behavioural and neurochemical aspects. Behav. Brain Res. 222, 246–264. doi: 10.1016/j.bbr.2011.04.002
Slade, A., Tennant, A., and Chamberlain, M. A. (2002). A randomised controlled trial to determine the effect of intensity of therapy upon length of stay in a neurological rehabilitation setting. J. Rehabil. Med. 34, 260–266. doi: 10.1080/165019702760390347
Smith, D. H., Okiyama, K., Thomas, M. J., Claussen, B., and McIntosh, T. K. (1991). Evaluation of memory dysfunction following experimental brain injury using the Morris water maze. J. Neurotrauma 8, 259–269. doi: 10.1089/neu.1991.8.259
Smith, J. M., Lunga, P., Story, D., Harris, N., Le Belle, J., James, M. F., et al. (2007). Inosine promotes recovery of skilled motor function in a model of focal brain injury. Brain 130, 915–925. doi: 10.1093/brain/awl393
Sohlberg, M. M., McLaughlin, K. A., Pavese, A., Heidrich, A., and Posner, M. I. (2000). Evaluation of attention process training and brain injury education in persons with acquired brain injury. J. Clin. Exp. Neuropsychol. 22, 656–676. doi: 10.1076/1380-3395(200010)22:5;1-9;FT656
Sozda, C. N., Hoffman, A. N., Olsen, A. S., Cheng, J. P., Zafonte, R. D., and Kline, A. E. (2010). Empirical comparison of typical and atypical environmental enrichment paradigms on functional and histological outcome after experimental traumatic brain injury. J. Neurotrauma 27, 1047–1057. doi: 10.1089/neu.2010.1313
Spires, T. L., Grote, H. E., Varshney, N. K., Cordery, P. M., Van Dellen, A., Blakemore, C., et al. (2004). Environmental enrichment rescues protein deficits in a mouse model of Huntington's disease, indicating a possible disease mechanism. J. Neurosci. 24, 2270–2276. doi: 10.1523/JNEUROSCI.1658-03.2004
Spivack, G., Spettell, C. M., Ellis, D. W., and Ross, S. E. (1992). Effects of intensity of treatment and length of stay on rehabilitation outcomes. Brain Inj. 6, 419–434. doi: 10.3109/02699059209008138
Strich, S. J. (1956). Diffuse degeneration of the cerebral white matter in severe dementia following head injury. J. Neurol. Neurosurg. Psychiatry 19, 163–185. doi: 10.1136/jnnp.19.3.163
Sur, M., and Leamey, C. A. (2001). Development and plasticity of cortical areas and networks. Nat. Rev. Neurosci. 2, 251–262. doi: 10.1038/35067562
Tang, Y. P., Wang, H., Feng, R., Kyin, M., and Tsien, J. Z. (2001). Differential effects of enrichment on learning and memory function in NR2B transgenic mice. Neuropharmacology 41, 779–790. doi: 10.1016/S0028-3908(01)00122-8
Taub, E., Uswatte, G., and Elbert, T. (2002). New treatments in neurorehabilitation founded on basic research. Nat. Rev. Neurosci. 3, 228–236. doi: 10.1038/nrn754
Till, C., Colella, B., Verwegen, J., and Green, R. E. (2008). Postrecovery cognitive decline in adults with traumatic brain injury. Arch. Phys. Med. Rehabil. 89, S25–S34. doi: 10.1016/j.apmr.2008.07.004
Toglia, J., Johnston, M. V., Goverover, Y., and Dain, B. (2010). A multicontext approach to promoting transfer of strategy use and self regulation after brain injury: an exploratory study. Brain Inj. 24, 664–677. doi: 10.3109/02699051003610474
Toglia, J. P. (1991). Generalization of treatment: a multicontext approach to cognitive perceptual impairment in adults with brain injury. Am. J. Occup. Ther. 45, 505–516. doi: 10.5014/ajot.45.6.505
Tognini, P., Manno, I., Bonaccorsi, J., Cenni, M. C., Sale, A., and Maffei, L. (2012). Environmental enrichment promotes plasticity and visual acuity recovery in adult monocular amblyopic rats. PLoS ONE 7:e34815. doi: 10.1371/journal.pone.0034815
Torasdotter, M., Metsis, M., Henriksson, B. G., Winblad, B., and Mohammed, A. H. (1998). Environmental enrichment results in higher levels of nerve growth factor mRNA in the rat visual cortex and hippocampus. Behav. Brain Res. 93, 83–90. doi: 10.1016/S0166-4328(97)00142-3
Turner, A. M., and Greenough, W. T. (1985). Differential rearing effects on rat visual cortex synapses. I. Synaptic and neuronal density and synapses per neuron. Brain Res. 329, 195–203.
Turrigiano, G. G. (1999). Homeostatic plasticity in neuronal networks: the more things change, the more they stay the same. Trends Neurosci. 22, 221–227. doi: 10.1016/S0166-2236(98)01341-1
Turrigiano, G. G. (2008). The self-tuning neuron: synaptic scaling of excitatory synapses. Cell 135, 422–435. doi: 10.1016/j.cell.2008.10.008
Uylings, H. B., Kuypers, K., Diamond, M. C., and Veltman, W. A. (1978). Effects of differential environments on plasticity of dendrites of cortical pyramidal neurons in adult rats. Exp. Neurol. 62, 658–677. doi: 10.1016/0014-4886(78)90276-5
Valero, J., Espana, J., Parra-Damas, A., Martin, E., Rodriguez-Alvarez, J., and Saura, C. A. (2011). Short-term environmental enrichment rescues adult neurogenesis and memory deficits in APP(Sw,Ind) transgenic mice. PLoS ONE 6:e16832. doi: 10.1371/journal.pone.0016832
Van Dellen, A., Blakemore, C., Deacon, R., York, D., and Hannan, A. J. (2000). Delaying the onset of Huntington's in mice. Nature 404, 721–722. doi: 10.1038/35008142
Van Praag, H., Christie, B. R., Sejnowski, T. J., and Gage, F. H. (1999). Running enhances neurogenesis, learning, and long-term potentiation in mice. Proc. Natl. Acad. Sci. U.S.A. 96, 13427–13431. doi: 10.1073/pnas.96.23.13427
Van Praag, H., Kempermann, G., and Gage, F. H. (2000). Neural consequences of environmental enrichment. Nat. Rev. Neurosci. 1, 191–198. doi: 10.1038/35044558
Varty, G. B., Paulus, M. P., Braff, D. L., and Geyer, M. A. (2000). Environmental enrichment and isolation rearing in the rat: effects on locomotor behavior and startle response plasticity. Biol. Psychiatry 47, 864–873. doi: 10.1016/S0006-3223(99)00269-3
Vedovelli, K., Silveira, E., Velho, E., Stertz, L., Kapczinski, F., Schroder, N., et al. (2011). Effects of increased opportunity for physical exercise and learning experiences on recognition memory and brain-derived neurotrophic factor levels in brain and serum of rats. Neuroscience 199, 284–291. doi: 10.1016/j.neuroscience.2011.08.012
Volkmar, F. R., and Greenough, W. T. (1972). Rearing complexity affects branching of dendrites in the visual cortex of the rat. Science 176, 1445–1447. doi: 10.1126/science.176.4042.1445
Voss, M. W., Nagamatsu, L. S., Liu-Ambrose, T., and Kramer, A. F. (2011). Exercise, brain, and cognition across the life span. J. Appl. Physiol. (1985) 111, 1505–1513. doi: 10.1152/japplphysiol.00210.2011
Wagner, A. K., Kline, A. E., Sokoloski, J., Zafonte, R. D., Capulong, E., and Dixon, C. E. (2002). Intervention with environmental enrichment after experimental brain trauma enhances cognitive recovery in male but not female rats. Neurosci. Lett. 334, 165–168. doi: 10.1016/S0304-3940(02)01103-5
Walsh, R. N., Budtz-Olsen, O. E., Penny, J. E., and Cummins, R. A. (1969). The effects of environmental complexity on the histology of the rat hippocampus. J. Comp. Neurol. 137, 361–366. doi: 10.1002/cne.901370309
Wang, Y., Bontempi, B., Hong, S. M., Mehta, K., Weinstein, P. R., Abrams, G. M., et al. (2008). A comprehensive analysis of gait impairment after experimental stroke and the therapeutic effect of environmental enrichment in rats. J. Cereb. Blood Flow Metab. 28, 1936–1950. doi: 10.1038/jcbfm.2008.82
Warraich, Z., and Kleim, J. A. (2010). Neural plasticity: the biological substrate for neurorehabilitation. PM R 2, S208–S219. doi: 10.1016/j.pmrj.2010.10.016
Wen, P. H., Hof, P. R., Chen, X., Gluck, K., Austin, G., Younkin, S. G., et al. (2004). The presenilin-1 familial Alzheimer disease mutant P117L impairs neurogenesis in the hippocampus of adult mice. Exp. Neurol. 188, 224–237. doi: 10.1016/j.expneurol.2004.04.002
Whishaw, I. Q., Pellis, S. M., Gorny, B. P., and Pellis, V. C. (1991). The impairments in reaching and the movements of compensation in rats with motor cortex lesions: an endpoint, videorecording, and movement notation analysis. Behav. Brain Res. 42, 77–91. doi: 10.1016/S0166-4328(05)80042-7
Whishaw, I. Q., Zaborowski, J. A., and Kolb, B. (1984). Postsurgical enrichment aids adult hemidecorticate rats on a spatial navigation task. Behav. Neural Biol. 42, 183–190. doi: 10.1016/S0163-1047(84)91046-X
Will, B., Galani, R., Kelche, C., and Rosenzweig, M. R. (2004). Recovery from brain injury in animals: relative efficacy of environmental enrichment, physical exercise or formal training (1990-2002). Prog. Neurobiol. 72, 167–182. doi: 10.1016/j.pneurobio.2004.03.001
Will, B. E., Rosenzweig, M. R., Bennett, E. L., Hebert, M., and Morimoto, H. (1977). Relatively brief environmental enrichment aids recovery of learning capacity and alters brain measures after postweaning brain lesions in rats. J. Comp. Physiol. Psychol. 91, 33–50. doi: 10.1037/h0077306
Willer, B., Button, J., and Rempel, R. (1999). Residential and home-based postacute rehabilitation of individuals with traumatic brain injury: a case control study. Arch. Phys. Med. Rehabil. 80, 399–406. doi: 10.1016/S0003-9993(99)90276-9
Wilson, R., Barnes, L., and Bennett, D. (2003). Assessment of lifetime participation in cognitively stimulating activities. J. Clin. Exp. Neuropsychol. 25, 634–642. doi: 10.1076/jcen.25.5.634.14572
Wood, N. I., Carta, V., Milde, S., Skillings, E. A., McAllister, C. J., Ang, Y. L., et al. (2010). Responses to environmental enrichment differ with sex and genotype in a transgenic mouse model of Huntington's disease. PLoS ONE 5:e9077. doi: 10.1371/journal.pone.0009077
Workman, J. L., Fonken, L. K., Gusfa, J., Kassouf, K. M., and Nelson, R. J. (2011). Post-weaning environmental enrichment alters affective responses and interacts with behavioral testing to alter nNOS immunoreactivity. Pharmacol. Biochem. Behav. 100, 25–32. doi: 10.1016/j.pbb.2011.07.008
Xerri, C., Bourgeon, S., and Coq, J. O. (2005). Perceptual context-dependent remodeling of the forepaw map in the SI cortex of rats trained on tactile discrimination. Behav. Brain Res. 162, 207–221. doi: 10.1016/j.bbr.2005.03.003
Xerri, C., Coq, J. O., Merzenich, M. M., and Jenkins, W. M. (1996). Experience-induced plasticity of cutaneous maps in the primary somatosensory cortex of adult monkeys and rats. J. Physiol. Paris 90, 277–287. doi: 10.1016/S0928-4257(97)81438-6
Xu, T., Yu, X., Perlik, A. J., Tobin, W. F., Zweig, J. A., Tennant, K., et al. (2009a). Rapid formation and selective stabilization of synapses for enduring motor memories. Nature 462, 915–919. doi: 10.1038/nature08389
Xu, X., Ye, L., and Ruan, Q. (2009b). Environmental enrichment induces synaptic structural modification after transient focal cerebral ischemia in rats. Exp. Biol. Med. (Maywood) 234, 296–305. doi: 10.3181/0804-RM-128
Yan, E. B., Johnstone, V. P., Alwis, D. S., Morganti-Kossmann, M. C., and Rajan, R. (2013). Characterising effects of impact velocity on brain and behaviour in a model of diffuse traumatic axonal injury. Neuroscience 248C, 17–29. doi: 10.1016/j.neuroscience.2013.05.045
Young, D., Lawlor, P. A., Leone, P., Dragunow, M., and During, M. J. (1999). Environmental enrichment inhibits spontaneous apoptosis, prevents seizures and is neuroprotective. Nat. Med. 5, 448–453. doi: 10.1038/7449
Zebunke, M., Puppe, B., and Langbein, J. (2013). Effects of cognitive enrichment on behavioural and physiological reactions of pigs. Physiol. Behav. 118, 70–79. doi: 10.1016/j.physbeh.2013.05.005
Zhou, X., Panizzutti, R., De Villers-Sidani, E., Madeira, C., and Merzenich, M. M. (2011). Natural restoration of critical period plasticity in the juvenile and adult primary auditory cortex. J. Neurosci. 31, 5625–5634. doi: 10.1523/JNEUROSCI.6470-10.2011
Zhu, X. L., Poon, W. S., Chan, C. C., and Chan, S. S. (2007). Does intensive rehabilitation improve the functional outcome of patients with traumatic brain injury (TBI)? A randomized controlled trial. Brain Inj. 21, 681–690. doi: 10.1080/02699050701468941
Zhu, X. L., Poon, W. S., Chan, C. H., and Chan, S. H. (2001). Does intensive rehabilitation improve the functional outcome of patients with traumatic brain injury? Interim result of a randomized controlled trial. Br. J. Neurosurg. 15, 464–473. doi: 10.1080/02688690120097688
Zhu, X. O., McCabe, B. J., Aggleton, J. P., and Brown, M. W. (1997). Differential activation of the rat hippocampus and perirhinal cortex by novel visual stimuli and a novel environment. Neurosci. Lett. 229, 141–143. doi: 10.1016/S0304-3940(97)00437-0
Keywords: EE, sensory cortices, traumatic brain injury, neuronal excitability
Citation: Alwis DS and Rajan R (2014) Environmental enrichment and the sensory brain: the role of enrichment in remediating brain injury. Front. Syst. Neurosci. 8:156. doi: 10.3389/fnsys.2014.00156
Received: 29 May 2014; Accepted: 12 August 2014;
Published online: 02 September 2014.
Edited by:
Mikhail Lebedev, Duke University, USACopyright © 2014 Alwis and Rajan. This is an open-access article distributed under the terms of the Creative Commons Attribution License (CC BY). The use, distribution or reproduction in other forums is permitted, provided the original author(s) or licensor are credited and that the original publication in this journal is cited, in accordance with accepted academic practice. No use, distribution or reproduction is permitted which does not comply with these terms.
*Correspondence: Ramesh Rajan, Department of Physiology, Monash University, Building 13F, Wellington Road, Clayton, VIC 3800, Australia e-mail: ramesh.rajan@monash.edu