- 1School of Basic Medical Sciences, Xianning Medical College, Hubei University of Science and Technology, Xianning, Hubei, China
- 2Department of Physiology, Hubei College of Chinese Medicine, Jingzhou, Hubei, China
Introduction: Cancer patients treated with paclitaxel often develop chemotherapy-induced peripheral neuropathy, which has not been effectively treated with drugs. The anti-diabetic drug metformin is effective in the treatment of neuropathic pain. The aim of this study was to elucidate effect of metformin on paclitaxel-induced neuropathic pain and spinal synaptic transmission.
Methods: Electrophysiological experiments on rat spinal slices were performed in vitro and mechanical allodynia quantified in vitro.
Results: The present data demonstrated that intraperitoneal injection of paclitaxel produced mechanical allodynia and potentiated spinal synaptic transmission. Intrathecal injection of metformin significantly reversed the established mechanical allodynia induced by paclitaxel in rats. Either spinal or systemic administration of metformin significantly inhibited the increased frequency of spontaneous excitatory postsynaptic currents (sEPSCs) in spinal dorsal horn neurons from paclitaxel-treated rats. We found that 1 h incubation of metformin also reduced the frequency rather than the amplitude of sEPSCs in the spinal slices from paclitaxel-treated rats.
Discussion: These results suggested that metformin was able to depress the potentiated spinal synaptic transmission, which may contribute to alleviating the paclitaxel-induced neuropathic pain.
1. Introduction
Paclitaxel is a frontline clinical drug used to treat many solid tumors (Hagiwara and Sunada, 2004). A common dose-limiting adverse side effect of paclitaxel is chemotherapy-induced peripheral neuropathy (CIPN), which can result in severe acute and chronic pain during paclitaxel treatment and after withdrawal (Han and Smith, 2013; Sisignano et al., 2014). So far, there is still a lack of effective drugs to treat the paclitaxel-induced neuropathic pain in clinic (Burgess et al., 2021). Spinal central sensitization is an important mechanism underly neuropathic pain, which is characterized by enhanced synaptic transmission in the spinal dorsal horn neurons (Woolf, 2011). There is evidence that excitatory synaptic transmission in spinal dorsal horn neurons is enhanced in CIPN, which involves increased excitability of primary sensory afferent neurons (Li et al., 2015). Electrophysiological recordings show that the frequency of excitatory postsynaptic currents (EPSCs) of dorsal horn neurons is increased in paclitaxel-treated rats (Xie et al., 2016).
Metformin is a drug widely used to treat type II diabetes. Metformin has been found to reverse the established mechanical hyperalgesia in a variety of neuropathic pain, such as pain caused by spinal cord nerve ligation and spared nerve injury (Melemedjian et al., 2011, 2013). In addition, metformin can also prevent neuropathic pain caused by chemotherapy (Mao-Ying et al., 2014; Inyang et al., 2019). It is still unclear whether metformin alleviates paclitaxel-induced neuropathic pain by affecting synaptic transmission in the spinal dorsal horn neurons. The present study indicated that spinal dorsal horn neurons displayed enhanced spontaneous excitatory postsynaptic currents (sEPSCs) in paclitaxel-treated rats, which was significantly inhibited by intrathecal and systemic administration of metformin in rats and incubation of metformin in spinal slices. Intrathecal administration of metformin also reversed the paclitaxel-induced mechanical allodynia.
2. Materials and methods
2.1. Animals
Male Sprague–Dawley rats (3–4 weeks of age) were housed in individual cages. The rats had free access to food and water. Room temperature was controlled at 23 ± 2°C during a 12 h light/dark cycles. All animal experimental protocols were approved by the Animal Research Ethics Committee of Hubei University of Science and Technology (2016-03-005). To evaluate the effect of intrathecal treatment with metformin on sEPSCs in spinal dorsal horn neurons from paclitaxel-treated rats, animals were randomly divided into three groups (n = 18, 6 rats in each group). In control group (CON), rats were injected corresponding vehicle intraperitoneally and intrathecally, respectively. In paclitaxel-treated group (PAC), rats received intraperitoneal injection (i.p.) of paclitaxel (2 mg/kg per injection at day 1, 3, 5, and 7) and intrathecal injections (i.t.) of 50 μl vehicle. In paclitaxel-metformin (i.t.)-treated group (MET), metformin (100 ug in 50 ul, at day 8, 9, and 10) was intrathecally treated in paclitaxel-treated rats. To further investigate the effect of systemic treatment with metformin on sEPSCs in spinal dorsal horn neurons from paclitaxel-treated rats, rats were randomly divided into three groups (n = 18, 6 rats in each group). In control group (CON), rats were injected corresponding vehicle intraperitoneally. In paclitaxel-treated group (PAC), rats received intraperitoneal injection (i.p.) of paclitaxel (2 mg/kg per injection at day 1, 3, 5, and 7) and 50 μl vehicle (i.p. at day 8, 9, and 10). In paclitaxel-metformin (i.p.)-treated group (MET), metformin (200 mg/kg, at day 8, 9, and 10) was intraperitoneally treated in paclitaxel-treated rats. At day 11 or 12, spinal cord slices were prepared to record sEPSCs in all rats. To study the acute effect of metformin on sEPSCs, spinal slices (at day 11 or 12) from paclitaxel-treated rats were incubated by oxygenated ACSF containing metformin (2 mM) or vehicle for 1 h, and then sEPSCs were recorded.
2.2. Intrathecal Surgeries and drug application
Intrathecal catheters were implanted on rats 15 days before paclitaxel treatment. Under deep anesthesia with a mixture of ketamine (75 mg/kg) and medetomidine (0.5 mg/kg), rats were inserted a polyethylene-10 catheter into their subarachnoid space through L5–L6 intervertebral space, and the tip of the catheter was located at the L5 spinal segmental level. To establish a mechanical allodynia model, rats received intraperitoneal injection (i.p.) of paclitaxel (Sigma-Aldrich, 2 mg/kg) at day 1, 3, 5, and 7, with a cumulative dose of 8 mg/kg. Paclitaxel was dissolved in a 1/1 Cremophor EL (Sigma-Aldrich)/ethanol solution then further prepared with 0.9% saline for injection. Rats in the control group received the vehicle (Cremophor EL/ethanol, 1:1) on the same four alternate days. Metformin (Sigma-Aldrich) was treated at day 8, 9, and 10. Metformin was dissolved in artificial cerebrospinal fluid and delivered through the intrathecal catheter. Metformin was also dissolved in 0.9% saline and intraperitoneally injected. After paclitaxel and metformin were applied, rats were sacrificed to prepare spinal cord slices for electrophysiological recordings at day 11 or 12.
2.3. Spinal slice preparations
The rats were anesthetized with 1.5 mg/kg urethane (10%, i.p.). A laminectomy was performed, and the lumbar segment was quickly removed and immersed in oxygenated (95% O2 and 5% CO2) cold ACSF. The ACSF contained 95.0 mM NaCl, 1.8 mM KCl, 0.5 mM CaCl2, 7.0 mM MgSO4, 1.2 mM KH2PO4, 26.0 mM NaHCO3, 15.0 mM Glucose and 50.0 mM Sucrose. Its pH was adjusted to 7.3–7.4 with NaOH and osmolarity to 310–320 mOsm/L with sucrose. The pial-arachnoid membrane was removed from the section. The L5 spinal segment was fixed on the cutting bracket with cyanoacrylate glue, then, 350 μm thick spinal cord slices were cut with a vibratome (Leica VT1200S Microsystem). Slices were incubated in ACSF oxygenated at 35°C for at least 1 h and then transferred to the recording chamber.
2.4. Electrophysiological recordings
The slice was placed into a recording chamber and continuously perfused with recording solution at a speed of 3–4 ml/min. The recording solution contained 127.0 mM NaCl, 1.8 mM KCl, 2.4 mM CaCl2, 1.3 mM MgSO4, 1.2 mM KH2PO4, 15.0 mM glucose, and 26.0 mM NaHCO3, oxygenated with 95% O2, and 5% CO2, at a pH of 7.3–7.4, and an osmolarity of 300–310 mOsm/L. Recordings of sEPSCs were performed using whole-cell voltage-clamp. All recordings were conducted in lamina II neurons at the L5 spinal level. Lamina II outer zone was identified by its distinctive translucent appearance and neurons were visualized using a water-immersion objective on an upright infrared Olympus microscope (BX51WI, Japan) with differential interference contrast/infrared illumination. sEPSCs were recorded using an EPC-10 amplifier and PULSE program (HEKA Electronics, Lambrecht, Germany). The recording pipette had a resistance of 8–10 MΩ when filled with the internal solution containing: 140.0 mM K-gluconate, 3.0 mM KCl, 4.0 mM NaCl, 0.2 mM EGTA, 10 mM HEPE and 2.0 mM Mg-ATP. Only a seal resistance of ≥ 2 GΩ and an access resistance of 20–35 MΩ were used for electrophysiological recording. The series resistance was optimally compensated by ≥70% and constantly monitored throughout the experiments. In the presence of 10 μM bicuculline and 5 μM strychnine, sEPSCs were recorded from neurons clamped at −70 mV. After a 15 min stabilization period, synaptic events were recorded for 4 min in every slice. A spinal cord slice was obtained from each animal and only a neuron was recorded in a slice.
2.5. Behavioral tests
Male rats were placed in a Plexiglas chamber and adapted to the environment for at least 30 min before behavior experiments. Mechanical allodynia was measured by paw withdrawal threshold (PWT). After rats were coded and pretreated with paclitaxel, different groups of rats then received intrathecal injection of vehicle or different dose (10, 30, and 100 ug in 50 ul, i.t. at day 8, 9, and 10) of metformin, separately. After 30 min metformin injection, the other experimenter tested PWT of the hind plantar using a series of von Frey filaments (Stoelting, Wood Dale, IL, USA). Experimenters who performed behavioral tests were blinded to all treatments.
2.6. Data analysis
Spontaneous events were performed and analyzed using Mini Analysis Program version 6.0.3 (Synaptosoft, Decatur, GA, USA). Analysis was performed in Igor pro6.10A software. Data were expressed as mean ± SEM and statistically compared using unpaired t-test or analysis of variance (ANOVA), followed by Bonferroni’s post-hoc test.
3. Result
3.1. Paclitaxel-induced mechanical allodynia is prevented by intrathecal injection of metformin
To evaluate the effect of metformin on paclitaxel-induced mechanical allodynia, rats were intrathecally injected with different dose of metformin versus vehicle, and then their mechanical thresholds were compared. We observed that rats displayed mechanical allodynia after administration with paclitaxel (4 × 2 mg/kg, cumulative dose 8 mg/kg). Figure 1 shows that the mechanical withdrawal thresholds of rats were decreased 1 day after paclitaxel injection. The decrease was further intensified, which was significantly different from baseline value at day 0. Since day 7, withdrawal threshold of paclitaxel-treated rats remained stable at low levels throughout the experiment. Figure 1 shows intrathecal administration of metformin (10, 30, and 100 ug in 50 ul, i.t. at day 8, 9, and 10) dose-dependently prevented the paclitaxel-induced mechanical allodynia (P < 0.01 and 0.001, Bonferroni’s post-hoc test, compared with vehicle, n = 10 rats). Mechanical allodynia only partially developed in the paclitaxel and 100 ug metformin-treated rats, which was significantly less than that of the paclitaxel–vehicle-treated rats at days 8–15. These results suggested that intrathecal injection of metformin dose-dependently prevents the established mechanical allodynia induced by paclitaxel in rats.
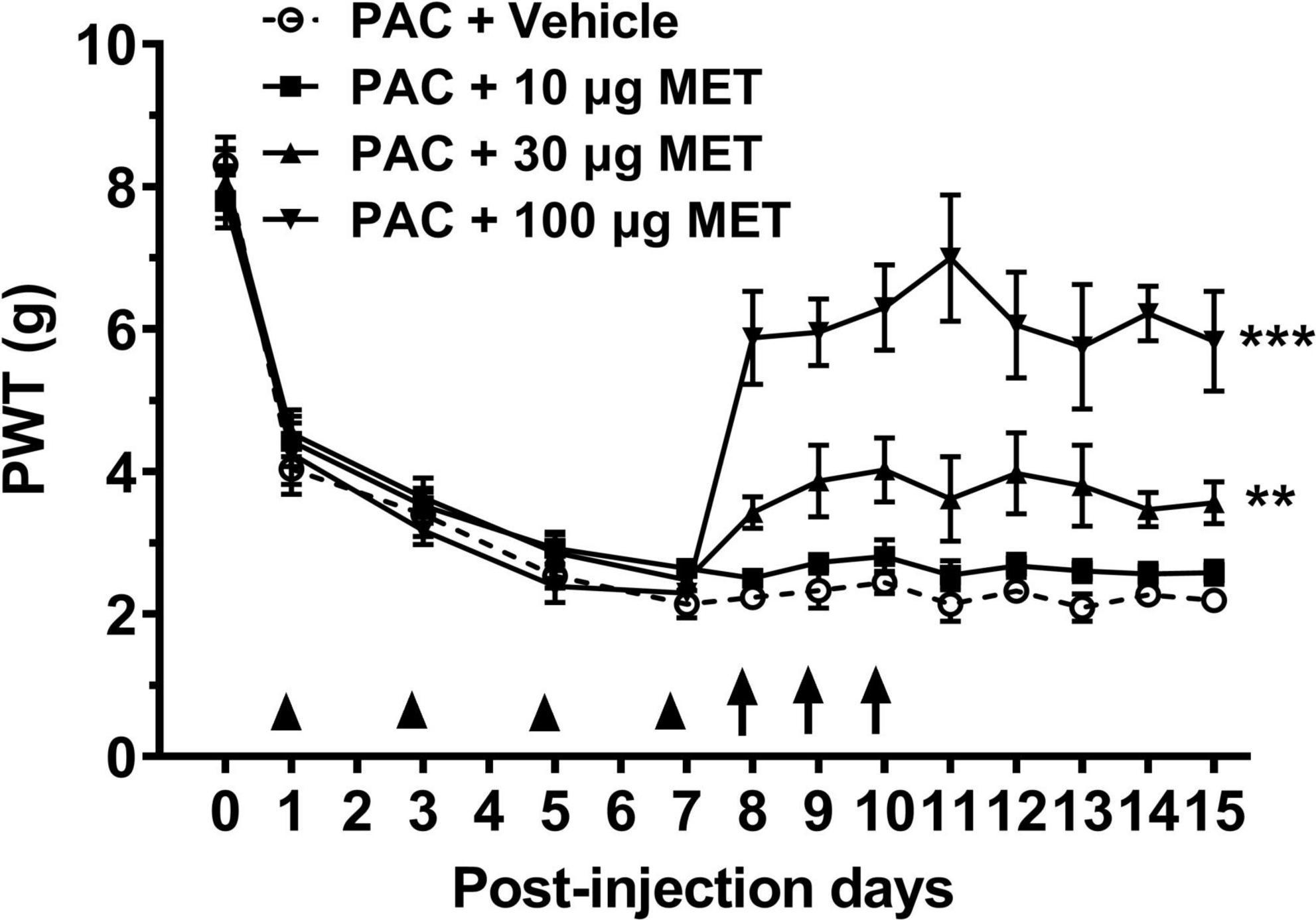
Figure 1. Intrathecal injection of metformin reverses paclitaxel-induced mechanical allodynia in rats. Treatment with paclitaxel (8 mg/kg at day 1, 3, 5, and 7) significantly reduced the paw withdraw threshold (PWT, in grams) in rats. Intrathecal injection of metformin (at day 8, 9, and 10) prevented paclitaxel-induced mechanical allodynia in a dose-dependent manner. N = 10 rats in each group, **P < 0.01, ***P < 0.001, two-way ANOVA followed by Bonferroni post-hoc test, compared with PAC + Vehicle group.
3.2. Intrathecal treatment with metformin inhibits the increased frequency of sEPSCs in spinal dorsal horn neurons from paclitaxel-treated rats
At day 11 or 12, sEPSCs in spinal dorsal horn neurons were recorded. Figure 2A shows the sEPSCs traces from three groups of rats. Cumulative distributions shows that the curve of sEPSC interevent interval was significantly shifted leftward in paclitaxel-treated (PAC) rats (Figure 2B), but no change in the curve of sEPSC amplitude (Figure 2D), compared with those from control (CON) rats. Figures 2C, E shows that sEPSC frequency was significantly increased (1.86 ± 0.26 Hz vs. 0.6 ± 0.07 Hz, P < 0.01, n = 6 neurons from 6 rats) without change in amplitude with paclitaxel, compared with those from control treatment. Metformin reversed this effect (Figures 2B, C). Intrathecal treatment with metformin significantly inhibited the increased frequency of sEPSCs with paclitaxel, as observed in paclitaxel-metformin treated (MET) rats (0.87 ± 0.07 Hz vs. 1.86 ± 0.26 Hz, P < 0.05, n = 6 neurons from 6 rats; Figures 2B, C), but not affected the sEPSC amplitude (Figures 2D, E). The results indicated that intrathecal administration of metformin inhibited the increased frequency of sEPSCs in spinal dorsal horn neurons from paclitaxel-treated rats.
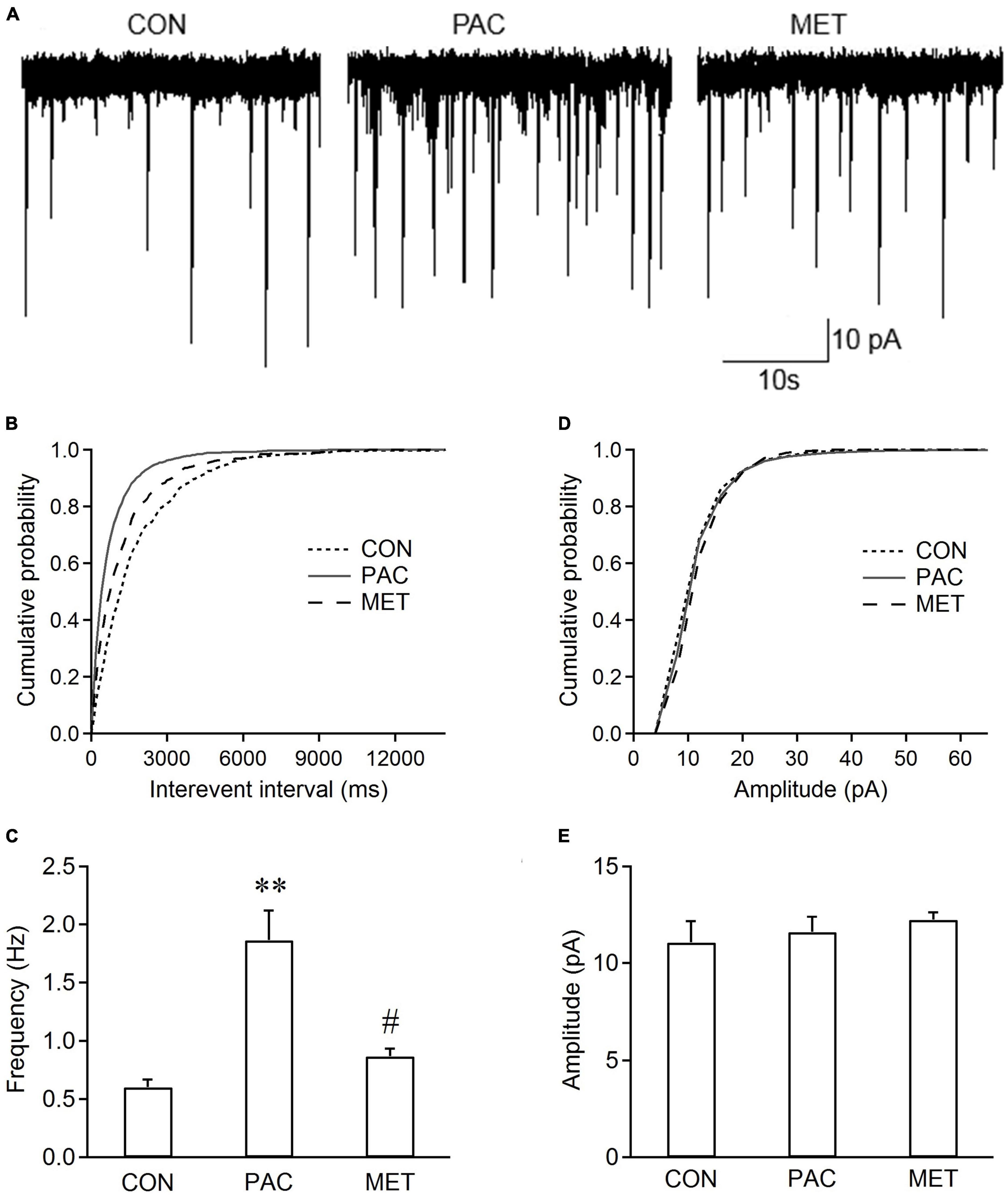
Figure 2. Intrathecal injection of metformin decreases the frequency rather than the amplitude of sEPSCs in spinal cord slices from paclitaxel-treated rats. (A) Typical traces of sEPSC recordings in spinal cord slices from control (CON), paclitaxel-treated (PAC), and paclitaxel-metformin (i.t.)-treated (MET) rats. Metformin (Met, 100 ug in 50 ul) was intrathecally injected daily in paclitaxel-treated rats at day 8, 9, and 10. Cumulative distributions (B,D) and the bar graphs (C,E) show the frequency rather than the amplitude of sEPSCs increased in spinal dorsal horn neurons from paclitaxel-treated rats, The increased sEPSC frequency recorded in slices from paclitaxel-treated rats was significantly decreased by intrathecal injection of metformin. However, metformin had no effect on sEPSC amplitude. Data are expressed as means ± S.E.M. N = 6 neurons from 6 rats, one-way ANOVA followed by Bonferroni post-hoc test, **P < 0.01, compared with control rats, #P < 0.05, compared with paclitaxel-treated rats. VH = –70 mV.
3.3. Incubation of metformin reduces sEPSC frequency in spinal dorsal horn neurons from paclitaxel-treated rats
Figure 3 shows that incubation of slices with metformin for 1 h had also effects on sEPSCs in spinal dorsal horn neurons from paclitaxel-treated rats. Cumulative distributions shows that metformin incubation significantly shifted the curve of sEPSC interevent interval rightward and had no effects on the curve of sEPSC amplitude, indicating that metformin made the intervals between sEPSC events longer, but not significantly changed sEPSC amplitude, compared with vehicle incubation (Figures 3B, D). Figures 3B, D show that metformin incubation also significantly reduced the sEPSC frequency (0.99 ± 0.19 Hz vs. 1.75 ± 0.38 Hz, P < 0.05, n = 6 neurons from 6 rats), rather than sEPSC amplitude, in the slices from paclitaxel-treated rats. These results indicated that metformin had an acute effect on sEPSCs in spinal dorsal horn neurons from paclitaxel-treated rats.
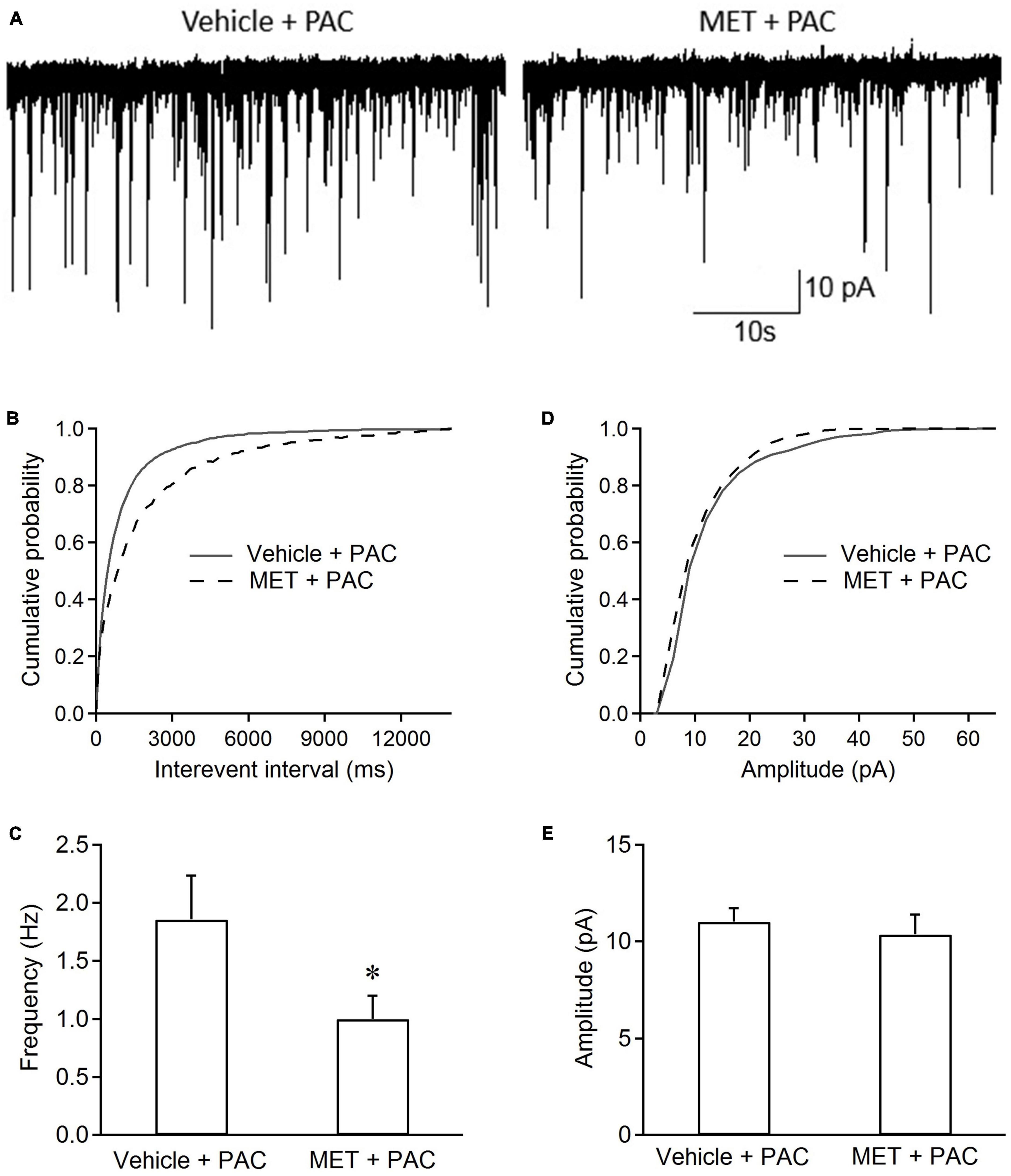
Figure 3. Incubation of metformin inhibits the frequency of sEPSCs in spinal dorsal horn neurons from paclitaxel-injected rats. (A) Typical recording traces of sEPSCs in spinal cord slices incubated with ACSF containing vehicle or metformin. Cumulative distributions (B,D) and the bar graphs (C,E) show incubation ACSF containing metformin (2 mM) decreased the frequency rather than the amplitude of EPSCs in spinal dorsal horn neurons from paclitaxel-treated rats. Data are expressed as means ± S.E.M. N = 6 neurons from 6 rats, *P < 0.05, unpaired t-test, compared with vehicle + PAC. VH = –70 mV. All slices (at day 10 or 11) from paclitaxel-treated rats were pre-incubated in ACSF containing metformin or vehicle for 1 h at 35°C.
3.4. Systemic treatment with metformin reduces the increased frequency of sEPSCs in spinal dorsal horn neurons from paclitaxel-treated rats
Figure 4A shows the sEPSCs traces in spinal dorsal horn neurons from three groups of rats. Cumulative distributions shows that the curve of sEPSC interevent interval was significantly shifted rightward in paclitaxel-metformin (i.p.)-treated (MET) rats, compared with those from paclitaxel-treated (PAC) rats (Figure 4B). However, no change in the curve of sEPSC amplitude was observer between the two groups of rats (Figure 4D). Figures 4C, E show that intraperitoneal injection of metformin also significantly decreased the increased frequency of sEPSCs (1.18 ± 0.37 Hz vs. 1.89 ± 0.42 Hz, P < 0.01, n = 6 neurons from 6 rats), but did not affect the sEPSC amplitude in spinal dorsal horn neurons from paclitaxel-treated rats. The results indicated that systemic treatment with metformin also suppressed the increased frequency of sEPSCs in spinal dorsal horn neurons from paclitaxel-treated rats.
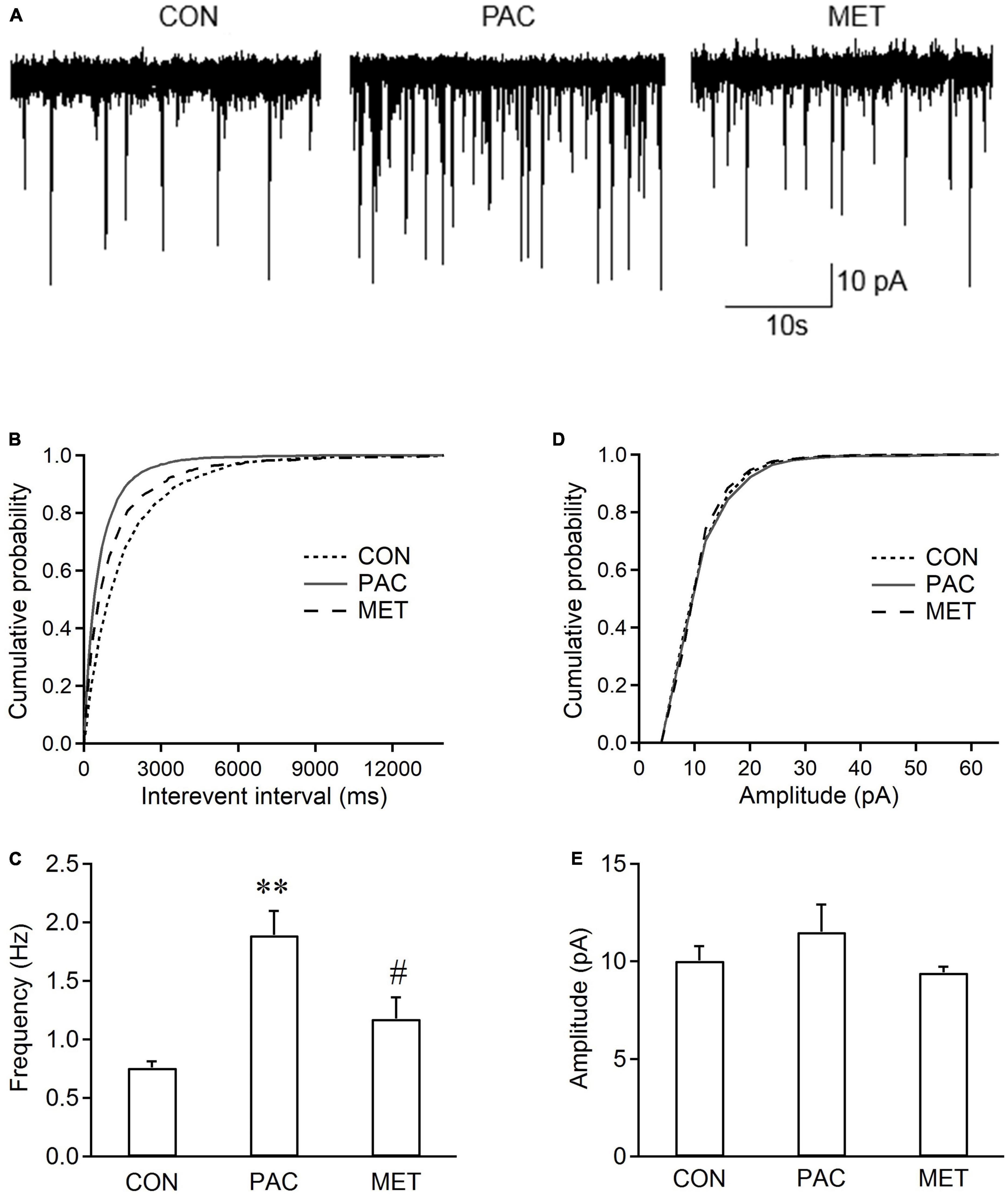
Figure 4. Systemic metformin inhibits the frequency of sEPSCs in spinal dorsal horn neurons from paclitaxel-injected rats. (A) Typical recording traces of sEPSCs in spinal dorsal horn neurons from control (CON), paclitaxel-treated (PAC, i.p.), and paclitaxel (i.p.)-metformin (i.p.)-treated (MET) rats. Metformin (Met, 200 mg/kg) was intraperitoneally injected daily in paclitaxel-treated rats at day 8, 9, and 10. Cumulative distributions (B,D) and the bar graphs (C,E) show systemic administration of metformin reduced the frequency rather than the amplitude of sEPSCs in spinal dorsal horn neurons from paclitaxel-treated rats. Data are expressed as means ± S.E.M. N = 6 neurons from 6 rats, one-way ANOVA followed by Bonferroni post-hoc test, **P < 0.01, compared with control rats, #P < 0.05, compared with paclitaxel-treated rats. VH = –70 mV.
4. Discussion
This study showed that mechanical allodynia was induced by intraperitoneal injection of paclitaxel and reached its peak within the 7th day to the 15th day after paclitaxel administration. We demonstrated that consecutive intrathecal injection of metformin at day 8, 9, and 10 dose-dependently alleviated the established mechanical allodynia in paclitaxel treated rats. Consistently, spinal and systemic application of metformin could suppress the potentiated spinal synaptic transmission in paclitaxel-treated rats.
It has been well documented that enhanced neuronal activation in the spinal dorsal horn is involved in a variety of pathological pain (Woolf, 2011). These include neuropathic pain induced by sciatic nerve-injury, diabetes, paclitaxel or vincristine chemotherapy (Weng et al., 2003; Li et al., 2010; Nie and Weng, 2010; Yan et al., 2015). Our results showed the frequency rather than the amplitude of sEPSCs in superficial dorsal horn neurons was increased in spinal cord slice from paclitaxel-treated rat. Previous studies have shown that the nociceptive synaptic transmission is enhanced by intraperitoneal injection of paclitaxel, which contributes to chemotherapy-induced neuropathic pain (Zhu et al., 2015; Xie et al., 2016). We observed that the increased frequency of sEPSCs did not occur after consecutive intrathecal or systemic administration of metformin in paclitaxel-treated rats, suggesting metformin prevented the paclitaxel-induced potentiation of spinal synaptic transmission. The increased frequency of sEPSCs was also significantly suppressed after the spinal cord slices from paclitaxel-treated rats were pre-incubated with metformin for 1 h, indicating the short-term treatment with metformin has an acute effect on enhanced synaptic transmission. However, pretreatment with metformin decreases sEPSC amplitudes and has no effect sEPSC frequency in oxaliplatin-incubating spinal slices (Ling et al., 2017). The possible reason for this discrepancy is the different effects of oxaliplatin on spinal synaptic transmission. Oxaliplatin only increases sEPSC amplitudes in spinal cord slice without change in sEPSC frequency (Ling et al., 2017). The present and previous studies have shown that paclitaxel had a direct effect on the increasing frequency of EPSCs in the spinal cord (Li et al., 2015). It has been found that DRG neurons, including their terminals, are the targets of paclitaxel attack (Matsumoto et al., 2006; Scuteri et al., 2006; Hara et al., 2013; Li et al., 2021). These findings suggest that paclitaxel may interact with presynaptic terminals in the dorsal horn, but not directly with postsynaptic neurons and other spinal neurons.
The current results cannot reveal the mechanisms underlying inhibition of sEPSC frequency by metformin in paclitaxel-treated rat spinal cord slices. Recently, metformin has been reported to inhibit L-type voltage-dependent calcium channel (Wang et al., 2020). In spinal lamina, the L-type calcium channel is located at presynaptic terminal and involved in synaptic transmission (Kim et al., 2001; Qian et al., 2013). The L-type calcium channel blocker can reduce the frequency of sEPSCs in spinal lamina in peripheral nerve injury model (Alles et al., 2018). It needs to be further verified whether metformin prevented the enhanced synaptic transmission in paclitaxel-treated rat spinal cord slices through inhibition of L-type calcium channel.
The present study showed that intrathecal injection of metformin reversed the established mechanical allodynia produced by paclitaxel in rats, similar to its role in paclitaxel-induced and nerve injury-induced neuropathic pain (Melemedjian et al., 2011, 2013; Inyang et al., 2019). However, intraperitoneal injection of metformin could prevent development of mechanical allodynia induced by cisplatin and paclitaxel in mice only when the injection was started before the administration of these chemotherapeutics, and has no effect when metformin was injected after the cisplatin application (Mao-Ying et al., 2014). It is unclear whether the reason for this discrepancy is related to the route of administration and animal species. Most studies believe that the analgesic effect of metformin is mainly depends on the activation of AMP-activated protein kinase (AMPK), which can alleviate the nociceptive behavior of animals in a variety of pain models, such as those caused by nerve injury, surgical incision, diabetes neuropathy and chemotherapy (Melemedjian et al., 2011; Tillu et al., 2012; Mao-Ying et al., 2014; Ma et al., 2015; Maixner et al., 2015; Wang et al., 2018; Inyang et al., 2019). Decreased AMPK activity was found in the dorsal horn of the spinal cord in animals with partial sciatic nerve ligation. Spinal AMPK knockdown by siRNA results in behavioral hypersensitivity (Maixner et al., 2015). Especially, mice lacking AMPKα1 display increased glutamatergic synaptic activity in the spinal dorsal horn and mechanical allodynia (Maixner et al., 2016). These findings provide a clue that metformin may suppress the potentiated spinal synaptic transmission and relieve paclitaxel-induced neuropathic pain by activating spinal AMPK signaling, although it need to examine whether metformin could increase the expression of AMPK in the spinal dorsal horn of rats with the neuropathic pain.
5. Conclusions
In conclusions, metformin was able to depress the potentiated spinal synaptic transmission, which may contribute to alleviating the paclitaxel-induced neuropathic pain.
Data availability statement
The original contributions presented in this study are included in the article/supplementary material, further inquiries can be directed to the corresponding author.
Ethics statement
The animal study was reviewed and approved by the Animal Research Ethics Committee of Hubei University of Science and Technology (2016-03-005).
Author contributions
W-PH designed the research. T-TL and C-YQ performed the experiments. T-TL participated in the data analysis. T-TL and W-PH wrote the manuscript. All authors contributed substantially to this research and reviewed this manuscript.
Funding
This work was supported by the National Natural Science Foundation of China (No. 81671101).
Conflict of interest
The authors declare that the research was conducted in the absence of any commercial or financial relationships that could be construed as a potential conflict of interest.
Publisher’s note
All claims expressed in this article are solely those of the authors and do not necessarily represent those of their affiliated organizations, or those of the publisher, the editors and the reviewers. Any product that may be evaluated in this article, or claim that may be made by its manufacturer, is not guaranteed or endorsed by the publisher.
References
Alles, S. R., Garcia, E., Balasubramanyan, S., Jones, K., Tyson, J. R., Joy, T., et al. (2018). Peripheral nerve injury increases contribution of L-type calcium channels to synaptic transmission in spinal lamina II: role of alpha2delta-1 subunits. Mol. Pain 14:1744806918765806. doi: 10.1177/1744806918765806
Burgess, J., Ferdousi, M., Gosal, D., Boon, C., Matsumoto, K., Marshall, A., et al. (2021). Chemotherapy-induced peripheral neuropathy: epidemiology, pathomechanisms and treatment. Oncol. Ther. 9, 385–450.
Han, Y., and Smith, M. T. (2013). Pathobiology of cancer chemotherapy-induced peripheral neuropathy (CIPN). Front. Pharmacol. 4:156. doi: 10.3389/fphar.2013.00156
Hara, T., Chiba, T., Abe, K., Makabe, A., Ikeno, S., Kawakami, K., et al. (2013). Effect of paclitaxel on transient receptor potential vanilloid 1 in rat dorsal root ganglion. Pain 154, 882–889.
Inyang, K. E., Mcdougal, T. A., Ramirez, E. D., Williams, M., Laumet, G., Kavelaars, A., et al. (2019). Alleviation of paclitaxel-induced mechanical hypersensitivity and hyperalgesic priming with AMPK activators in male and female mice. Neurobiol. Pain 6:100037. doi: 10.1016/j.ynpai.2019.100037
Kim, D. S., Yoon, C. H., Lee, S. J., Park, S. Y., Yoo, H. J., and Cho, H. J. (2001). Changes in voltage-gated calcium channel alpha(1) gene expression in rat dorsal root ganglia following peripheral nerve injury. Brain Res. Mol. Brain Res. 96, 151–156. doi: 10.1016/s0169-328x(01)00285-6
Li, J. Q., Chen, S. R., Chen, H., Cai, Y. Q., and Pan, H. L. (2010). Regulation of increased glutamatergic input to spinal dorsal horn neurons by mGluR5 in diabetic neuropathic pain. J. Neurochem. 112, 162–172. doi: 10.1111/j.1471-4159.2009.06437.x
Li, Y., Adamek, P., Zhang, H., Tatsui, C. E., Rhines, L. D., Mrozkova, P., et al. (2015). The cancer chemotherapeutic paclitaxel increases human and rodent sensory neuron responses to TRPV1 by activation of TLR4. J. Neurosci. 35, 13487–13500. doi: 10.1523/JNEUROSCI.1956-15.2015
Li, Y., Marri, T., North, R. Y., Rhodes, H. R., Uhelski, M. L., Tatsui, C. E., et al. (2021). Chemotherapy-induced peripheral neuropathy in a dish: dorsal root ganglion cells treated in vitro with paclitaxel show biochemical and physiological responses parallel to that seen in vivo. Pain 162, 84–96. doi: 10.1097/j.pain.0000000000002005
Ling, Y. Z., Li, Z. Y., Ou-Yang, H. D., Ma, C., Wu, S. L., Wei, J. Y., et al. (2017). The inhibition of spinal synaptic plasticity mediated by activation of AMP-activated protein kinase signaling alleviates the acute pain induced by oxaliplatin. Exp. Neurol. 288, 85–93. doi: 10.1016/j.expneurol.2016.11.009
Ma, J., Yu, H., Liu, J., Chen, Y., Wang, Q., and Xiang, L. (2015). Metformin attenuates hyperalgesia and allodynia in rats with painful diabetic neuropathy induced by streptozotocin. Eur. J. Pharmacol. 764, 599–606.
Maixner, D. W., Yan, X., Gao, M., Yadav, R., and Weng, H. R. (2015). Adenosine monophosphate-activated protein kinase regulates Interleukin-1beta expression and glial glutamate transporter function in rodents with neuropathic pain. Anesthesiology 122, 1401–1413. doi: 10.1097/ALN.0000000000000619
Maixner, D. W., Yan, X., Hooks, S. B., and Weng, H. R. (2016). AMPKalpha1 knockout enhances nociceptive behaviors and spinal glutamatergic synaptic activities via production of reactive oxygen species in the spinal dorsal horn. Neuroscience 326, 158–169. doi: 10.1016/j.neuroscience.2016.03.061
Mao-Ying, Q. L., Kavelaars, A., Krukowski, K., Huo, X. J., Zhou, W., Price, T. J., et al. (2014). The anti-diabetic drug metformin protects against chemotherapy-induced peripheral neuropathy in a mouse model. PLoS One 9:e100701. doi: 10.1371/journal.pone.0100701
Matsumoto, M., Inoue, M., Hald, A., Xie, W., and Ueda, H. (2006). Inhibition of paclitaxel-induced A-fiber hypersensitization by gabapentin. J. Pharmacol. Exp. Ther. 318, 735–740. doi: 10.1124/jpet.106.103614
Melemedjian, O. K., Asiedu, M. N., Tillu, D. V., Sanoja, R., Yan, J., Lark, A., et al. (2011). Targeting adenosine monophosphate-activated protein kinase (AMPK) in preclinical models reveals a potential mechanism for the treatment of neuropathic pain. Mol. Pain 7:70. doi: 10.1186/1744-8069-7-70
Melemedjian, O. K., Khoutorsky, A., Sorge, R. E., Yan, J., Asiedu, M. N., Valdez, A., et al. (2013). mTORC1 inhibition induces pain via IRS-1-dependent feedback activation of ERK. Pain 154, 1080–1091. doi: 10.1016/j.pain.2013.03.021
Nie, H., and Weng, H. R. (2010). Impaired glial glutamate uptake induces extrasynaptic glutamate spillover in the spinal sensory synapses of neuropathic rats. J. Neurophysiol. 103, 2570–2580. doi: 10.1152/jn.00013.2010
Qian, A., Song, D., Li, Y., Liu, X., Tang, D., Yao, W., et al. (2013). Role of voltage gated Ca2+ channels in rat visceral hypersensitivity change induced by 2,4,6-trinitrobenzene sulfonic acid. Mol. Pain 9:15. doi: 10.1186/1744-8069-9-15
Scuteri, A., Nicolini, G., Miloso, M., Bossi, M., Cavaletti, G., Windebank, A. J., et al. (2006). Paclitaxel toxicity in post-mitotic dorsal root ganglion (DRG) cells. Anticancer Res. 26, 1065–1070.
Sisignano, M., Baron, R., Scholich, K., and Geisslinger, G. (2014). Mechanism-based treatment for chemotherapy-induced peripheral neuropathic pain. Nat. Rev. Neurol. 10, 694–707.
Tillu, D. V., Melemedjian, O. K., Asiedu, M. N., Qu, N., De Felice, M., Dussor, G., et al. (2012). Resveratrol engages AMPK to attenuate ERK and mTOR signaling in sensory neurons and inhibits incision-induced acute and chronic pain. Mol. Pain 8:5. doi: 10.1186/1744-8069-8-5
Wang, H., Wang, C., Lu, Y., Yan, Y., Leng, D., Tian, S., et al. (2020). Metformin shortens prolonged QT interval in diabetic mice by inhibiting L-Type calcium current: a possible therapeutic approach. Front. Pharmacol. 11:614. doi: 10.3389/fphar.2020.00614
Wang, S., Kobayashi, K., Kogure, Y., Yamanaka, H., Yamamoto, S., Yagi, H., et al. (2018). Negative regulation of TRPA1 by AMPK in primary sensory neurons as a potential mechanism of painful diabetic neuropathy. Diabetes 67, 98–109. doi: 10.2337/db17-0503
Weng, H. R., Cordella, J. V., and Dougherty, P. M. (2003). Changes in sensory processing in the spinal dorsal horn accompany vincristine-induced hyperalgesia and allodynia. Pain 103, 131–138. doi: 10.1016/s0304-3959(02)00445-1
Woolf, C. J. (2011). Central sensitization: implications for the diagnosis and treatment of pain. Pain 152, S2–S15.
Xie, J. D., Chen, S. R., Chen, H., Zeng, W. A., and Pan, H. L. (2016). Presynaptic N-Methyl-d-aspartate (n.d.) receptor activity is increased through protein kinase C in paclitaxel-induced neuropathic pain. J. Biol. Chem 291, 19364–19373. doi: 10.1074/jbc.M116.732347
Yan, X., Maixner, D. W., Yadav, R., Gao, M., Li, P., Bartlett, M. G., et al. (2015). Paclitaxel induces acute pain via directly activating toll like receptor 4. Mol. Pain 11:10.
Keywords: metformin, paclitaxel, sEPSCs, nociception, CIPN
Citation: Liu T-T, Qiu C-Y and Hu W-P (2023) Metformin inhibits spontaneous excitatory postsynaptic currents in spinal dorsal cord neurons from paclitaxel-treated rats. Front. Synaptic Neurosci. 15:1191383. doi: 10.3389/fnsyn.2023.1191383
Received: 22 March 2023; Accepted: 20 April 2023;
Published: 05 May 2023.
Edited by:
Marco Atzori, Santa Clara University, United StatesReviewed by:
Juan Carlos Pineda, Universidad Autónoma de Yucatán, MexicoMarcela Miranda-Morales, Autonomous University of San Luis Potosí, Mexico
Copyright © 2023 Liu, Qiu and Hu. This is an open-access article distributed under the terms of the Creative Commons Attribution License (CC BY). The use, distribution or reproduction in other forums is permitted, provided the original author(s) and the copyright owner(s) are credited and that the original publication in this journal is cited, in accordance with accepted academic practice. No use, distribution or reproduction is permitted which does not comply with these terms.
*Correspondence: Wang-Ping Hu, d2FuZ3BpbmdfaHVAMTYzLmNvbQ==