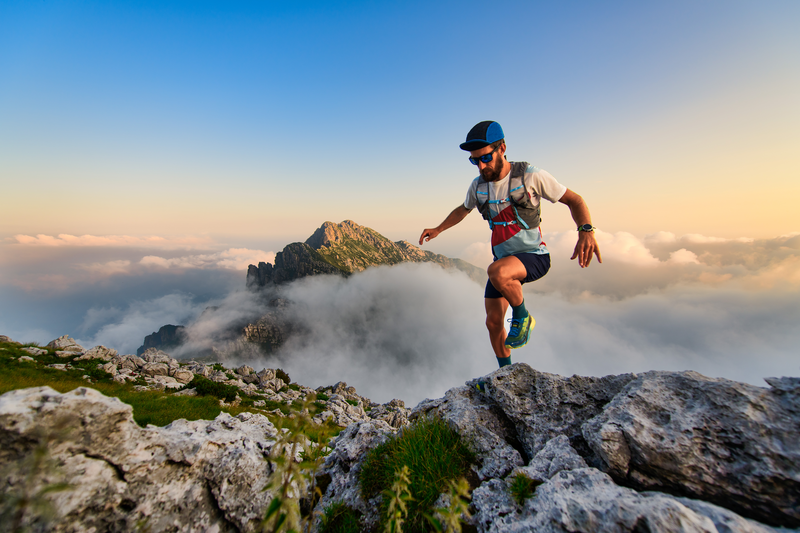
94% of researchers rate our articles as excellent or good
Learn more about the work of our research integrity team to safeguard the quality of each article we publish.
Find out more
MINI REVIEW article
Front. Synaptic Neurosci. , 02 March 2023
Volume 15 - 2023 | https://doi.org/10.3389/fnsyn.2023.1146665
This article is part of the Research Topic New Insights Into Synaptic Plasticity in Fear Conditioning View all 5 articles
Following fear conditioning, behavior can be reduced by giving many CS-alone presentations in a process known as extinction or by presenting a few CS-alone presentations and interfering with subsequent memory reconsolidation. While the two share procedural similarities, both the behavioral outcomes and the neurobiological underpinnings are distinct. Here we review the neural and behavioral mechanisms that produce these separate behavioral reductions, as well as some factors that determine whether or not a retrieval-dependent reconsolidation process or an extinction process will be in effect.
Almost a century has passed since Pavlov’s (1927) initial demonstration of extinction, a phenomenon in which conditional responding declines when a conditional stimulus (CS) is no longer presented with a biologically relevant unconditional stimulus (UCS). While significant strides have been made in understanding the behavioral and neurobiological mechanisms of Pavlovian extinction learning, it remains an active area of research due to findings that demonstrate extinguished responding will readily return under a variety of circumstances. These “relapse” effects (including spontaneous recovery, renewal, and reinstatement) suggest behavioral changes produced by extinction are impermanent and CS-UCS learning remains intact throughout extinction. While a variety of strategies have been employed to reduce return of extinguished responding (Brooks and Bouton, 1993, 1994; Vansteenwegen et al., 2006; Culver et al., 2011), behavioral relapse is the rule rather than the exception (Bouton et al., 2021). This presents a significant hurdle to extinction-based exposure therapies aiming to reduce maladaptive responding stemming from classically conditioned experiences (Bouton et al., 2001; Hermans et al., 2006).
More recently, researchers have aimed to circumvent issues associated with extinction by directly targeting the original CS-UCS memory, taking advantage of a brief period of memory lability following retrieval known as reconsolidation. During this post-retrieval period, physical (Misanin et al., 1968) or pharmacological (Nader et al., 2000) assaults can fundamentally change the memory. Reconsolidation is thought to alter or ablate the original memory trace itself, particularly within regions like the amygdala, hippocampus, and prefrontal cortex. As such, memory that has been reduced through reconsolidation is not susceptible to relapse.
However, there are procedural similarities between retrieval-induced reconsolidation and extinction: both involve some amount of exposure to unreinforced conditional stimuli. A key difference between the two is that reconsolidation typically involves limited or brief exposure to a CS whereas extinction involves more extensive exposure to the CS. Further, much of the mechanistic work has suggested that extinction-related and reconsolidation-related fear inhibition depend differentially on amygdala interactions with the hippocampus and prefrontal cortex.
Understanding the conditions that produce extinction or reconsolidation-like effects will aid the development of therapeutic strategies that aim to take advantage of reconsolidation to limit behavioral relapse associated with extinction learning. Here, we review the literature underlying the boundaries between what variables produce retrieval-induced reconsolidation and which variables result in the creation of a new memory via extinction. We will discuss both the behavioral and neural mechanisms (see Table 1), with a primary focus on basolateral amygdala (BLA), dorsal hippocampal (DH), and infralimbic prefrontal cortical (IL) contributions.
Table 1. Brief overview summarizing similarities and differences between extinction and reconsolidation.
Fear memories are formed through CS-UCS pairings, resulting in an association between the two supported by an amygdala-centric neural circuit (Helmstetter et al., 2008). Following fear conditioning, memory strength can be indicated by the amount of fear responding. While several brain regions are critical for fear processes, medial prefrontal cortical, DH, and BLA subregions are especially critical for memory formation and retention (Kwapis et al., 2011; Gilmartin et al., 2012; Likhtik et al., 2014; Zelikowsky et al., 2014). The interaction between these regions governs the amount of fear and subsequent memory strength, precision, and the ability to later modify memory.
Fear memory is supported by changes in synaptic strength and plasticity in the BLA, characterized by AMPA receptor synaptic presence and NMDA-dependent long-term potentiation (Clugnet and LeDoux, 1990; Maren and Fanselow, 1995; Rogan and LeDoux, 1995; Humeau et al., 2007). Synaptic potentiation is supported by several intracellular processes that contribute to changes in gene expression and the synthesis of new proteins, including MAPK and PKA cellular signaling, epigenetic modifications, and subsequent changes in gene expression. These together support persistent changes needed for long-term memory storage. Fear memory formation can be disrupted by BLA inactivation with inhibitory GABAergic manipulations (Helmstetter and Bellgowan, 1994; Gilmartin et al., 2012), interference with cellular signaling cascades (Schafe and LeDoux, 2000; Schafe et al., 2000; Parsons et al., 2006), blockade of epigenetic modifiers (Monsey et al., 2011; Maddox et al., 2013a,b), or disruptions in glutamatergic receptor trafficking (Joels and Lamprecht, 2010; Migues et al., 2010).
Following memory formation, behavior can be reduced through extinction, where a CS or context that was previously predictive of a UCS is presented alone. While retrieval-based reconsolidation is observed after relatively few CS-alone presentations, extinction is observed following many CS-alone presentations. Extinction results in the formation of a new, context-dependent CS-No UCS memory (Bouton and Bolles, 1979; Trask et al., 2017).
Extinction is dependent on BLA inhibitory GABAergic neuronal activity (Akirav et al., 2006; Sangha et al., 2009; Heldt et al., 2012). Synaptic plasticity within the BLA is governed by NMDA receptor activity, which triggers cellular and synaptic changes needed to support long-term memory. Because extinction learning represents a new form of learning, it requires many of the same modifications as initial memory formation (Walker et al., 2002; Zimmerman and Maren, 2010). While these effects are often thought to occur on glutamatergic neurons, GABAergic populations also contain NMDA receptors and GABAergic neuronal activity is mediated by NMDA receptors (Royer and Pare, 2002; Szinyei et al., 2003; Polepalli et al., 2010; Spampanato et al., 2011). Thus, NMDA receptor effects may result from GABAergic regulation.
The increase in GABAergic tone within the BLA decreases the activity of excitatory neurons needed for fear expression. Extinction learning is dependent on IL projections to the BLA; direct IL inputs to the BLA engage GABAergic neurons that suppress principal excitatory neurons (Selleck et al., 2018; Bukalo et al., 2021). Extinction increases the excitability of BLA-projecting IL neurons to increase the capacity of the IL to suppress BLA activity (Bloodgood et al., 2018; Ferrara et al., 2020). Inhibiting IL activity during extinction learning impairs its acquisition (Sierra-Mercado et al., 2011; Do-Monte et al., 2015) and epigenetic manipulations within the IL modify the strength and persistence of extinction memories (Lattal et al., 2007; Marek et al., 2011; Bahari-Javan et al., 2012; Stafford et al., 2012; Kwapis and Wood, 2014), suggesting plasticity in this region is critical for extinction. Similarly, manipulations that directly suppress BLA excitation enhance extinction. This includes both manipulations that activate GABAergic inputs from the mPFC, and those that inhibit excitatory inputs to the BLA from the auditory thalamus and cortex (Cho et al., 2013; Ferrara et al., 2020). In this manner, extinction memories are thought to recruit BLA inhibition to suppress the original, excitatory fear memory. However, several results have demonstrated that IL activity is not necessary for the expression of extinguished behavior (Do-Monte et al., 2015), which instead depends on the ventral hippocampus (Sierra-Mercado et al., 2011), likely through projections inhibiting the prelimbic cortex (Vasquez et al., 2019).
Extinguished fear renews when tested outside of the extinction context or after a sufficient amount of time (Bouton et al., 2006). The DH is important for encoding contextual attributes of a memory, and DH lesions prevent context-dependent return of fear after extinction (Corcoran and Maren, 2004; Ji and Maren, 2005; Helmstetter et al., 2008; Gafford et al., 2011). However, DH-dependency is based on the context in which renewal occurs, as DH regulates renewal in novel contexts but not the training context (Corcoran and Maren, 2004). Impaired extinction is believed to be a result of increased BLA-DH synchronization when GABAergic processes are disrupted (Sangha et al., 2009). Importantly, changes at inputs supporting initial fear memory storage remain unchanged after fear extinction, suggesting that the return of fear following extinction is a result of a persisting neural trace (Kim and Cho, 2017).
Together, this suggests extinction memory is characterized by increases in GABAergic tone, dependent on IL-BLA pathway, and persisting/relapsed fear is supported by DH-BLA interaction. Activity among these regions drives fear expression following extinction.
Following retrieval, memory becomes temporarily sensitive to disruption and must be stored again in a process called reconsolidation. In an initial demonstration, following CS retrieval, rats were given electroconvulsive shock and substantially decreased their CS-elicited fear relative to controls that did not receive electroconvulsive shock (Misanin et al., 1968). Other types of amnesic events following retrieval, like hypothermia, have similar deleterious effects on memory (Mactutus et al., 1979). Interest in this topic renewed following work that demonstrated protein synthesis blockade with BLA anisomycin infusions immediately after cued fear retrieval impaired later CS memory (Nader et al., 2000) and conditions that create this effect began to be explored.
Early experiments compared how post-retrieval and extinction injections of anisomycin affected behavior. BLA protein synthesis inhibition following either retrieval or extinction resulted in impaired reconsolidation, consistent with a weaker fear memory (Duvarci et al., 2006). One likely explanation for this finding is that extinction and reconsolidation rely on non-overlapping circuitry, with extinction processes being dependent on input from the infralimbic cortex (e.g., Do-Monte et al., 2015). Interestingly, post-retrieval anisomycin prevented both spontaneous recovery and reinstatement, suggesting that retrieval-based manipulations may affect the original memory itself (Duvarci and Nader, 2004). The efficacy of anisomycin inhibition in the BLA is contingent on brief decreases in AMPA receptor-mediated synaptic transmission. Memory retrieval is characterized by persistent increases in AMPA- relative to NMDA-mediated currents, with transient changes in the contribution of calcium-impermeable and calcium-permeable AMPA receptors (Hong et al., 2013). While calcium-impermeable AMPA receptors are present in BLA synapses, these AMPA receptors internalize and are replaced with calcium-permeable AMPA receptors (Hong et al., 2013). This exchange is believed to mediate intracellular plastic changes required for memory lability, as this AMPA receptor trafficking occurs along the same timeframe that anisomycin impairs memory retention and interferes with anisomycin memory impairments when this transient internalization of calcium impermeable AMPA receptors is inhibited (Hong et al., 2013). This work identified the importance of AMPA receptor trafficking, and specifically calcium influx for protein synthesis-dependent memory lability required for memory modification.
A number of changes occur within the BLA during fear memory reconsolidation, including intracellular signaling cascades, epigenetic modifications, and changes in gene expression (Johansen et al., 2011; Kwapis and Wood, 2014). One particularly important intracellular change that occurs in the BLA is altered protein degradation, critical for memory destabilization (Jarome et al., 2011, 2013, 2016). Transient internalization of calcium-impermeable AMPA receptors allows for postsynaptic calcium influx, and this regulates protein degradation-related activity (Ferrara et al., 2019a). Some of the proteins targeted for degradation include synaptic scaffolds and factors mediating protein synthesis, suggesting the proteasome system plays an important role in the reorganization of synaptic structure following memory retrieval and in the regulation of anisomycin-related memory impairments (Jarome et al., 2011). Interestingly, these processes are regulated by CaMKII activity, which is initiated by calcium influx (Jarome et al., 2013, 2016). Inhibition of any of these factors prevents protein synthesis and subsequent effects of anisomycin. Cellular mechanisms downstream from AMPA receptor trafficking therefore play a pivotal role in preservation of fear memory.
The necessity of protein synthesis was also extended to hippocampus-dependent context fear memories, where local DH anisomycin infusions reduced fear (Debiec et al., 2002). In a delay fear conditioning task new contextual information introduced during memory retrieval is sufficient to drive reconsolidation processes and the absence of new contextual information leaves memory resistant to disruption (Jarome et al., 2015). Synaptic activity in the BLA following memory retrieval in a new context is mediated by DH activity; inhibition of DH activity reduces the necessity of contextual novelty for reconsolidation-induced changes in behavior and calcium-impermeable GluA2-containing AMPA receptor trafficking within the synapse (Ferrara et al., 2019b). Together, these results suggest that the DH and BLA are critical sites for fear memory storage and can influence reconsolidation-like processes.
Some work has demonstrated that prediction error is necessary to induce reconsolidation-like effects (Sevenster et al., 2014), in line with findings suggesting new information must be included in to induce memory lability during retrieval (see Exton-McGuinness et al., 2015, for a review). However, prediction error may not be the only factor driving memory lability accompanied by a brief retrieval. This has been clearly seen when a CS-UCS retrieval presentation (i.e., no prediction error is likely as the CS is paired with what is predicted from initial learning) still leaves a memory susceptible to disruption with a post-retrieval anisomycin injection (Duvarci and Nader, 2004). Interestingly, this is only the case in situations in which the memory is not well-learned, suggesting the ability for protein synthesis inhibition to impair a memory without the introduction of prediction error may instead be reflective of impaired consolidation that accompanies new learning rather than impaired reconsolidation indicative of retrieval of a well-learned memory.
New information integrated during memory retrieval and mediated by reconsolidation can also change the neural circuitry that supports memory, suggesting that reorganization happens more broadly than at the synapse. Memory updating with a single trial of delay conditioning following initial trace fear conditioning, which is dependent on cortical structures, resulted in responding that was no longer dependent on cortex for expression (Kwapis et al., 2017). This suggests that new information integrated during the single-trial updating session can not only change behavior but also can change the neural circuit that supports fear memory. The reorganized circuit supporting updated memory is less complex than the circuit that supported the original memory, which might be easier to target therapeutically.
Some work challenges the notion that interfering with reconsolidation in the post-retrieval period impacts the original memory. For example, anisomycin injections following context fear retrieval impaired fear expression the next day but had no impact when testing occurred 21 days later (Lattal and Abel, 2004), in line with early results reporting that reactivated memory impaired by hypothermia-induced amnesia was susceptible to spontaneous recovery but new memory was not (Mactutus et al., 1979). Further, protein synthesis inhibition following fear acquisition has a larger, more persistent effect on behavior than following memory retrieval (Stafford and Lattal, 2009), suggesting that a reactivated memory is not as susceptible to disruption as was originally assumed. These results complement early work on reconsolidation-like effects, in which a second exposure to an amnesic event would rescue memory impairments observed following the first exposure (Hinderliter et al., 1975).
Some work has combined retrieval with extinction to enhance extinction learning. For example, Monfils et al. (2009) found that a brief retrieval to open the reconsolidation window followed by an extended extinction session resulted in more effective extinction and reduced behavioral relapse. This suggests reactivation makes the original memory susceptible to disruption and under these conditions, extinction can act on the original memory instead of creating a new memory. This was supported by work showing this effect was dependent on changes in calcium-permeable AMPA receptors in the BLA, while regular extinction was not (Clem and Huganir, 2010). This reactivation-extinction method is especially attractive as a therapeutic target because it is readily applicable to and effective in humans and does not require pharmacological manipulations (Schiller et al., 2010). However, failures to replicate in both humans (Chalkia et al., 2020a) and rats (Luyten and Beckers, 2017) or to verify the Schiller et al. (2010) report (Chalkia et al., 2020b) have caused scrutiny on the generality of these effects.
Additional work has found that unpaired presentations of the UCS during extinction can reduce both fear renewal and rapid reacquisition (Lipp et al., 2021), suggesting that UCS exposures during extinction might engage different processes than CS-alone presentations and capitalize on reconsolidation-like mechanisms (see also Siegel et al., 2022).
In auditory fear conditioning, a few unreinforced CS presentations following conditioning will result in memory lability (reconsolidation), but many unreinforced CS presentations will result in new inhibitory learning (extinction). Presenting few (4) CSs will engage reconsolidation-like processes (indicated by internalization of GluA2-containing AMPA receptors) but presenting many (40) CSs will result in extinction learning (indicated by decreased CREB phosphorylation). However, inhibiting excitatory thalamic projections in the BLA during a brief retrieval can shift both the behavioral and molecular profile to that of extinction, driving decreased CREB phosphorylation and context-dependent reduction in fear susceptible to fear renewal (Ferrara et al., 2021). It has also been suggested that while prediction error is needed to drive memory reconsolidation (Sevenster et al., 2014), too much prediction error or dissimilarity between memory acquisition and retrieval might instead drive new memory formation like that observed in extinction learning (Sevenster et al., 2013). The specific behavioral protocol that is used may ultimately determine whether reconsolidation or extinction is triggered, depending on whether mPFC inputs to the BLA (and subsequent activation of BLA GABAergic circuitry) is recruited. In this circumstance, brief retrieval sessions engage excitatory BLA processes that are initiated with AMPA receptor trafficking. As the retrieval session is extended, this increasingly recruits the mPFC→BLA pathway increasing BLA GABAergic tone.
Like retrieval and extinction procedures, presenting few or many weak shocks following fear conditioning can differentially impact behavior. While 2 weak shocks following contextual fear conditioning can increase fear elicited by that context, 10 weak shocks decreases contextual fear (Ferrara et al., 2019a). Follow-up work comparing this procedure to extinction found that, unlike extinction, the effects of weak shock transcend context and rely on long-term changes at BLA synapses (Bonanno et al., 2023). However, as with standard retrieval and extinction protocols, the specific conditions that increase freezing behavior (Ferrara et al., 2019a) or weaken freezing behavior (Bonanno et al., 2023), are not yet known; it is unclear how many weak shocks are needed to tip memory from strengthening to weakening. However, fear following weak shock exposures decreases with amount of weak UCS presentations, as reduced-intensity UCS presentations did not need to occur within a single session but could occur over several days (Popik et al., 2020). While it seems to function similarly to CS-alone presentations, work needs to identify any boundary conditions that may exist for this procedure.
While systematic work directly comparing retrieval-dependent reconsolidation to extinction is still sparse, it seems that number of CS presentations is the crucial variable that drives the behavioral and neurobiological differences between reconsolidation and extinction. Other factors, however, may contribute substantially to these effects. This includes introducing new information (e.g., contextual novelty) and optimizing prediction error, which may contribute to the likelihood that reconsolidation-like processes are preferentially engaged.
ST, JLK, and NCF wrote the manuscript. All authors contributed to the article and approved the submitted version.
The authors declare that the research was conducted in the absence of any commercial or financial relationships that could be construed as a potential conflict of interest.
All claims expressed in this article are solely those of the authors and do not necessarily represent those of their affiliated organizations, or those of the publisher, the editors and the reviewers. Any product that may be evaluated in this article, or claim that may be made by its manufacturer, is not guaranteed or endorsed by the publisher.
Akirav, I., Raizel, H., and Maroun, M. (2006). Enhancement of conditioned fear extinction by infusion of the GABAA agonist muscimol into the rat prefrontal cortex and amygdala. Europ. J. Neurosci. 23, 758–764. doi: 10.1111/j.1460-9568.2006.04603.x
Bahari-Javan, S., Maddalena, A., Kerimoglu, C., Wittnam, J., Held, T., Bähr, M., et al. (2012). HDAC1 regulates fear extinction in mice. J. Neurosci. 32, 5062–5073.
Bloodgood, D. W., Sugam, J. A., Holmes, A., and Kash, T. L. (2018). Fear extinction requires infralimbic cortex projections to the basolateral amygdala. Translat. Psych. 8:60. doi: 10.1038/s41398-018-0106-x
Bonanno, G. R., Met Hoxha, E., Robinson, P. K., Ferrara, N. C., and Trask, S. (2023). Fear reduced through unconditional stimulus deflation is behaviorally distinct from extinction and differentially engages the amygdala. Biol. Psych.. 13, 216–223.
Bouton, M. E., and Bolles, R. C. (1979). Contextual control of the extinction of conditioned fear. Learn. Motivat. 10, 445–466.
Bouton, M. E., Maren, S., and McNally, G. P. (2021). Behavioral and neurobiological mechanisms of Pavlovian and instrumental extinction learning. Physiol. Rev. 101, 611–681.
Bouton, M. E., Mineka, S., and Barlow, D. H. (2001). A modern learning theory perspective on the etiology of panic disorder. Psychol. Rev. 4:108. doi: 10.1037/0033-295x.108.1.4
Bouton, M. E., Westbrook, R. F., Corcoran, K. A., and Maren, S. (2006). Contextual and temporal modulation of extinction: behavioral and biological mechanisms. Biol. Psych. 60, 352–360.
Brooks, D. C., and Bouton, M. E. (1993). A retrieval cue for extinction attenuates spontaneous recovery. J. Exp. Psychol. 19:77.
Brooks, D. C., and Bouton, M. E. (1994). A retrieval cue for extinction attenuates response recovery (renewal) caused by a return to the conditioning context. J. Exp. Psychol. 20:366.
Bukalo, O., Nonaka, M., Weinholtz, C. A., Mendez, A., Taylor, W. W., and Holmes, A. (2021). Effects of optogenetic photoexcitation of infralimbic cortex inputs to the basolateral amygdala on conditioned fear and extinction. Behav. Brain Res. 396:112913. doi: 10.1016/j.bbr.2020.112913
Chalkia, A., Schroyens, N., Leng, L., Vanhasbroeck, N., Zenses, A. K., Van Oudenhove, L., et al. (2020a). No persistent attenuation of fear memories in humans: A registered replication of the reactivation-extinction effect. Cortex 129, 496–509. doi: 10.1016/j.cortex.2020.04.017
Chalkia, A., Van Oudenhove, L., and Beckers, T. (2020b). Preventing the return of fear in humans using reconsolidation update mechanisms: A verification report of Schiller. (2010). Cortex 129, 510–525. doi: 10.1038/nature08637
Cho, J. H., Deisseroth, K., and Bolshakov, V. Y. (2013). Synaptic encoding of fear extinction in mPFC-amygdala circuits. Neuron 80, 1491–1507. doi: 10.1016/j.neuron.2013.09.025
Clem, R. L., and Huganir, R. L. (2010). Calcium-permeable AMPA receptor dynamics mediate fear memory erasure. Science 330, 1108–1112. doi: 10.1126/science.1195298
Clugnet, M. C., and LeDoux, J. E. (1990). Synaptic plasticity in fear conditioning circuits: induction of LTP in the lateral nucleus of the amygdala by stimulation of the medial geniculate body. J. Neurosci. 10, 2818–2824.
Corcoran, K. A., and Maren, S. (2004). Factors regulating the effects of hippocampal inactivation on renewal of conditional fear after extinction. Learn. Memory 11, 598–603. doi: 10.1101/lm.78704
Culver, N. C., Stoyanova, M., and Craske, M. G. (2011). Clinical relevance of retrieval cues for attenuating context renewal of fear. J. Anxiety Disor. 25, 284–292. doi: 10.1016/j.janxdis.2010.10.002
Debiec, J., LeDoux, J. E., and Nader, K. (2002). Cellular and systems reconsolidation in the hippocampus. Neuron 36, 527–538.
Do-Monte, F. H., Manzano-Nieves, G., Quiñones-Laracuente, K., Ramos-Medina, L., and Quirk, G. J. (2015). Revisiting the role of infralimbic cortex in fear extinction with optogenetics. J. Neurosci. 35, 3607–3615. doi: 10.1523/JNEUROSCI.3137-14.2015
Duvarci, S., Mamou, C. B., and Nader, K. (2006). Extinction is not a sufficient condition to prevent fear memories from undergoing reconsolidation in the basolateral amygdala. Europ. J. Neurosci. 24, 249–260.
Duvarci, S., and Nader, K. (2004). Characterization of fear memory reconsolidation. J. Neurosci. 24, 9269–9275.
Exton-McGuinness, M. T., Lee, J. L., and Reichelt, A. C. (2015). Updating memories—the role of prediction errors in memory reconsolidation. Behav. Brain Res. 278, 375–384. doi: 10.1016/j.bbr.2014.10.011
Ferrara, N. C., Jarome, T. J., Cullen, P. K., Orsi, S. A., Kwapis, J. L., Trask, S., et al. (2019a). GluR2 endocytosis-dependent protein degradation in the amygdala mediates memory updating. Scientif. Rep. 9:5180. doi: 10.1038/s41598-019-41526-1
Ferrara, N. C., Trask, S., Pullins, S. E., and Helmstetter, F. J. (2019b). The dorsal hippocampus mediates synaptic destabilization and memory lability in the amygdala in the absence of contextual novelty. Neurobiol. Learn. Mem. 166:107089. doi: 10.1016/j.nlm.2019.107089
Ferrara, N. C., Mrackova, E., Loh, M. K., Padival, M., and Rosenkranz, J. A. (2020). Fear learning enhances prefrontal cortical suppression of auditory thalamic inputs to the amygdala in adults, but not adolescents. Int. J. Mole. Sci. 21:3008. doi: 10.3390/ijms21083008
Ferrara, N. C., Trask, S., Pullins, S. E., Helmstetter, F. J., Gafford, G. M., Parsons, R. G., et al. (2021). Regulation of learned fear expression through the MgN-amygdala pathway. Neurobiol. Learn. Mem. 182, 98–104. doi: 10.1016/j.nlm.2021.107526
Gafford, G. M., Parsons, R. G., and Helmstetter, F. (2011). Consolidation and reconsolidation of contextual fear memory requires mammalian target of rapamycin-dependent translation in the dorsal hippocampus. Neuroscience 182, 98–104. doi: 10.1016/j.neuroscience.2011.03.023
Gilmartin, M. R., Kwapis, J. L., and Helmstetter, F. J. (2012). Trace and contextual fear conditioning are impaired following unilateral microinjection of muscimol in the ventral hippocampus or amygdala, but not the medial prefrontal cortex. Neurobiol. Learn. Mem. 97, 452–464.
Heldt, S. A., Mou, L., and Ressler, K. (2012). In vivo knockdown of GAD67 in the amygdala disrupts fear extinction and the anxiolytic-like effect of diazepam in mice. Translat. Psych. 2:e181. doi: 10.1038/tp.2012.101
Helmstetter, F. J., and Bellgowan, P. S. (1994). Effects of muscimol applied to the basolateral amygdala on acquisition and expression of contextual fear conditioning in rats. Behav. Neurosci. 108:1005. doi: 10.1037//0735-7044.108.5.1005
Helmstetter, F. J., Parsons, R. G., and Gafford, G. M. (2008). Macromolecular synthesis, distributed synaptic plasticity, and fear conditioning. Neurobiol. Learn. Mem. 89, 324–337.
Hermans, D., Craske, M. G., Mineka, S., and Lovibond, P. F. (2006). Extinction in human fear conditioning. Biol. Psych. 60, 361–368.
Hinderliter, C. F., Webster, T., and Riccio, D. C. (1975). Amnesia induced by hypothermia as a function of treatment-test interval and recooling in rats. Anim. Learn. Behav. 3, 257–263.
Hong, I., Kim, J., Kim, J., Lee, S., Ko, H. G., Nader, K., et al. (2013). AMPA receptor exchange underlies transient memory destabilization on retrieval. Proc. Natl. Acad. Sci. U.S.A. 110, 8218–8223. doi: 10.1073/pnas.1305235110
Humeau, Y., Reisel, D., Johnson, A. W., Borchardt, T., Jensen, V., Gebhardt, C., et al. (2007). A pathway-specific function for different AMPA receptor subunits in amygdala long-term potentiation and fear conditioning. J. Neurosci. 27, 10947–10956. doi: 10.1523/JNEUROSCI.2603-07.2007
Jarome, T. J., Ferrara, N. C., Kwapis, J. L., and Helmstetter, F. J. (2015). Contextual information drives the reconsolidation-dependent updating of retrieved fear memories. Neuropsychopharmacology 40, 3044–3052. doi: 10.1038/npp.2015.161
Jarome, T. J., Ferrara, N. C., Kwapis, J. L., and Helmstetter, F. J. (2016). CaMKII regulates proteasome phosphorylation and activity and promotes memory destabilization following retrieval. Neurobiol. Learn. Mem. 128, 103–109. doi: 10.1016/j.nlm.2016.01.001
Jarome, T. J., Kwapis, J. L., Ruenzel, W. L., and Helmstetter, F. J. (2013). CaMKII, but not protein kinase A, regulates Rpt6 phosphorylation and proteasome activity during the formation of long-term memories. Front. Behav. Neurosci. 7:115. doi: 10.3389/fnbeh.2013.00115
Jarome, T. J., Werner, C. T., Kwapis, J. L., and Helmstetter, F. J. (2011). Activity dependent protein degradation is critical for the formation and stability of fear memory in the amygdala. PLoS One 6:e24349. doi: 10.1371/journal.pone.0024349
Ji, J., and Maren, S. (2005). Electrolytic lesions of the dorsal hippocampus disrupt renewal of conditional fear after extinction. Learn. Mem. 12, 270–276. doi: 10.1101/lm.91705
Joels, G., and Lamprecht, R. (2010). Interaction between N-ethylmaleimide-sensitive factor and GluR2 is essential for fear memory formation in lateral amygdala. J. Neurosci. 30, 15981–15986. doi: 10.1523/JNEUROSCI.1872-10.2010
Johansen, J. P., Cain, C. K., Ostroff, L. E., and LeDoux, J. E. (2011). Molecular mechanisms of fear learning and memory. Cell 147, 509–524.
Kim, W. B., and Cho, J. H. (2017). Encoding of discriminative fear memory by input-specific LTP in the amygdala. Neuron 95, 1129–1146. doi: 10.1016/j.neuron.2017.08.004
Kwapis, J. L., Jarome, T. J., Ferrara, N. C., and Helmstetter, F. J. (2017). Updating procedures can reorganize the neural circuit supporting a fear memory. Neuropsychopharmacology 42, 1688–1697. doi: 10.1038/npp.2017.23
Kwapis, J. L., Jarome, T. J., Schiff, J. C., and Helmstetter, F. J. (2011). Memory consolidation in both trace and delay fear conditioning is disrupted by intra-amygdala infusion of the protein synthesis inhibitor anisomycin. Learn. Mem. 18, 728–732. doi: 10.1101/lm.023945.111
Kwapis, J. L., and Wood, M. A. (2014). Epigenetic mechanisms in fear conditioning: implications for treating post-traumatic stress disorder. Trends Neurosci. 37, 706–720.
Lattal, K. M., and Abel, T. (2004). Behavioral impairments caused by injections of the protein synthesis inhibitor anisomycin after contextual retrieval reverse with time. Proc. Natl. Acad. Sci. U.S.A. 101, 4667–4672. doi: 10.1073/pnas.0306546101
Lattal, K. M., Barrett, R. M., and Wood, M. A. (2007). Systemic or intrahippocampal delivery of histone deacetylase inhibitors facilitates fear extinction. Behav. Neurosci. 121, 1125–1131.
Likhtik, E., Stujenske, J. M. A., Topiwala, M., Harris, A. Z., and Gordon, J. A. (2014). Prefrontal entrainment of amygdala activity signals safety in learned fear and innate anxiety. Nat. Neurosci. 17, 106–113. doi: 10.1038/nn.3582
Lipp, O. V., Ryan, K. M., Luck, C. C., Craske, M. G., and Waters, A. M. (2021). Presentation of unpaired unconditional stimuli during extinction reduces renewal of conditional fear and slows re−acquisition. Psychophysiology 58:e13899. doi: 10.1111/psyp.13899
Luyten, L., and Beckers, T. (2017). A preregistered, direct replication attempt of the retrieval-extinction effect in cued fear conditioning in rats. Neurobiol. Learn. Mem. 144, 208–215. doi: 10.1016/j.nlm.2017.07.014
Mactutus, C. F., Riccio, D. C., and Ferek, J. M. (1979). Retrograde amnesia for old (reactivated) memory: some anomalous characteristics. Science 204, 1319–1320. doi: 10.1126/science.572083
Maddox, S. A., Watts, C. S., Doyère, V., and Schafe, G. E. (2013a). A naturally-occurring histone acetyltransferase inhibitor derived from Garcinia indica impairs newly acquired and reactivated fear memories. PLoS One 8:e54463. doi: 10.1371/journal.pone.0054463
Maddox, S. A., Watts, C. S., and Schafe, G. E. (2013b). p300/CBP histone acetyltransferase activity is required for newly acquired and reactivated fear memories in the lateral amygdala. Learn. Mem. 20, 109–119. doi: 10.1101/lm.029157.112
Marek, R., Coelho, C. M., Sullivan, R. K. P., Baker-Andresen, D., Li, X., Ratnu, V., et al. (2011). Paradoxical enhancement of fear extinction memory and synaptic plasticity by inhibition of the histone acetyltransferase p300. J. Neurosci. 31, 7486–7491. doi: 10.1523/JNEUROSCI.0133-11.2011
Maren, S., and Fanselow, M. S. (1995). Synaptic plasticity in the basolateral amygdala induced by hippocampal formation stimulation in vivo. J. Neurosci. 15, 7548–7564.
Migues, P. V., Hardt, O., Wu, D. C., Gamache, K., Sacktor, T. C., Wang, Y. T., et al. (2010). PKMζ maintains memories by regulating GluR2-dependent AMPA receptor trafficking. Nat. Neurosci. 13, 630–634. doi: 10.1038/nn.2531
Misanin, J. R., Miller, R. R., and Lewis, D. J. (1968). Retrograde amnesia produced by electroconvulsive shock after reactivation of a consolidated memory trace. Science 160, 554–555. doi: 10.1126/science.160.3827.554
Monfils, M. H., Cowansage, K. K., Klann, E., and LeDoux, J. E. (2009). Extinction-reconsolidation boundaries: key to persistent attenuation of fear memories. Science 324, 951–955. doi: 10.1126/science.1167975
Monsey, M. M., Ota, K. T., Akingbade, I. F., Hong, E. S., and Schafe, G. E. (2011). Epigenetic alterations are critical for fear memory consolidation and synaptic plasticity in the lateral amygdala. PLoS One 6:e19958. doi: 10.1371/journal.pone.0019958
Nader, K., Schafe, G. E., and Le Doux, J. E. (2000). Fear memories require protein synthesis in the amygdala for reconsolidation after retrieval. Nature 406, 722–726.
Parsons, R. G., Gafford, G. M., and Helmstetter, F. J. (2006). Translational control via the mammalian target of rapamycin pathway is critical for the formation and stability of long-term fear memory in amygdala neurons. J. Neurosci. 26, 12977–12983. doi: 10.1523/JNEUROSCI.4209-06.2006
Pavlov, I. (1927). Conditioned reflexes: An investigation of the physiological activity of the cerebral cortex. London: Oxford Univeresity Press.
Polepalli, J. S., Sullivan, R. K., Yanagawa, Y., and Sah, P. (2010). A specific class of interneuron mediates inhibitory plasticity in the lateral amygdala. J. Neurosci. 30, 14619–14629. doi: 10.1523/JNEUROSCI.3252-10.2010
Popik, B., Amorim, F. E., Amaral, O. B., and Alvares, L. D. O. (2020). Shifting from fear to safety through deconditioning-update. eLife 9:e51207. doi: 10.7554/eLife.51207
Rogan, M. T., and LeDoux, J. E. (1995). LTP is accompanied by commensurate enhancement of auditory-evoked responses in a fear conditioning circuit. Neuron 15, 127–136. doi: 10.1016/0896-6273(95)90070-5
Royer, S., and Pare, D. (2002). Bidirectional synaptic plasticity in intercalated amygdala neurons and the extinction of conditioned fear responses. Neuroscience 115, 455–462. doi: 10.1016/s0306-4522(02)00455-4
Sangha, S., Narayanan, R. T., Bergado-Acosta, J. R., Stork, O., Seidenbecher, T., and Pape, H. C. (2009). Deficiency of the 65 kDa isoform of glutamic acid decarboxylase impairs extinction of cued but not contextual fear memory. J. Neurosci. 29, 15713–15720. doi: 10.1523/JNEUROSCI.2620-09.2009
Schafe, G. E., Atkins, C. M., Swank, M. W., Bauer, E. P., Sweatt, J. D., and LeDoux, J. E. (2000). Activation of ERK/MAP kinase in the amygdala is required for memory consolidation of pavlovian fear conditioning. J. Neurosci. 20, 8177–8187.
Schafe, G. E., and LeDoux, J. E. (2000). Memory consolidation of auditory pavlovian fear conditioning requires protein synthesis and protein kinase A in the amygdala. J. Neurosci. 20:RC96. doi: 10.1523/JNEUROSCI.20-18-j0003.2000
Schiller, D., Monfils, M. H., Raio, C. M., Johnson, D. C., LeDoux, J. E., and Phelps, E. A. (2010). Preventing the return of fear in humans using reconsolidation update mechanisms. Nature 463, 49–53.
Selleck, R. A., Zhang, W., Samberg, H. D., Padival, M., and Rosenkranz, J. A. (2018). Limited prefrontal cortical regulation over the basolateral amygdala in adolescent rats. Scientif. Rep. 8:70. doi: 10.1038/s41598-018-35649-0
Sevenster, D., Beckers, T., and Kindt, M. (2013). Prediction error governs pharmacologically induced amnesia for learned fear. Science 339, 830–833. doi: 10.1126/science.1231357
Sevenster, D., Beckers, T., and Kindt, M. (2014). Prediction error demarcates the transition from retrieval, to reconsolidation, to new learning. Learn. Mem. 21, 580–584. doi: 10.1101/lm.035493.114
Siegel, P., Cohen, B., and Warren, R. (2022). Nothing to fear but fear itself: A mechanistic test of unconscious exposure. Biol. Psych. 91, 294–302. doi: 10.1016/j.biopsych.2021.08.022
Sierra-Mercado, D., Padilla-Coreano, N., and Quirk, G. J. (2011). Dissociable roles of prelimbic and infralimbic cortices, ventral hippocampus, and basolateral amygdala in the expression and extinction of conditioned fear. Neuropsychopharmacology 36, 529–538. doi: 10.1038/npp.2010.184
Spampanato, J., Polepalli, J., and Sah, P. (2011). Interneurons in the basolateral amygdala. Neuropharmacology 60, 765–773.
Stafford, J. M., and Lattal, K. M. (2009). Direct comparisons of the size and persistence of anisomycin-induced consolidation and reconsolidation deficits. Learn. Mem. 16, 494–503. doi: 10.1101/lm.1452209
Stafford, J. M., Raybuck, J. D., Ryabinin, A. E., and Lattal, K. M. (2012). Increasing histone acetylation in the hippocampus-infralimbic network enhances fear extinction. Biol. Psych. 72, 25–33. doi: 10.1016/j.biopsych.2011.12.012
Szinyei, C., Stork, O., and Pape, H. C. (2003). Contribution of NR2B subunits to synaptic transmission in amygdaloid interneurons. J. Neurosci. 23, 2549–2556. doi: 10.1523/JNEUROSCI.23-07-02549.2003
Trask, S., Thrailkill, E. A., and Bouton, M. E. (2017). Occasion setting, inhibition, and the contextual control of extinction in Pavlovian and instrumental (operant) learning. Behav. Proc. 137, 64–72. doi: 10.1016/j.beproc.2016.10.003
Vansteenwegen, D., Vervliet, B., Hermans, D., Beckers, T., Baeyens, F., and Eelen, P. (2006). Stronger renewal in human fear conditioning when tested with an acquisition retrieval cue than with an extinction retrieval cue. Behav. Res. Therapy 44, 1717–1725. doi: 10.1016/j.brat.2005.10.014
Vasquez, J. H., Leong, K. C., Gagliardi, C. M., Harland, B., Apicella, A. J., and Muzzio, I. A. (2019). Pathway specific activation of ventral hippocampal cells projecting to the prelimbic cortex diminishes fear renewal. Neurobiol. Learn. Mem. 161, 63–71. doi: 10.1016/j.nlm.2019.03.003
Walker, D. L., Ressler, K. J., Lu, K. T., and Davis, M. (2002). Facilitation of conditioned fear extinction by systemic administration or intra-amygdala infusions of D-cycloserine as assessed with fear-potentiated startle in rats. J. Neurosci. 22, 2343–2351.
Zelikowsky, M., Hersman, S., Chawla, M. K., Barnes, C. A., and Fanselow, M. S. (2014). Neuronal ensembles in amygdala, hippocampus, and prefrontal cortex track differential components of contextual fear. J. Neurosci. 34, 8462–8466. doi: 10.1523/JNEUROSCI.3624-13.2014
Keywords: fear, extinction, reconsolidation, memory, amygdala
Citation: Ferrara NC, Kwapis JL and Trask S (2023) Memory retrieval, reconsolidation, and extinction: Exploring the boundary conditions of post-conditioning cue exposure. Front. Synaptic Neurosci. 15:1146665. doi: 10.3389/fnsyn.2023.1146665
Received: 17 January 2023; Accepted: 17 February 2023;
Published: 02 March 2023.
Edited by:
Ana Cicvaric, Albert Einstein College of Medicine, United StatesReviewed by:
Ryan Parsons, Stony Brook University, United StatesCopyright © 2023 Ferrara, Kwapis and Trask. This is an open-access article distributed under the terms of the Creative Commons Attribution License (CC BY). The use, distribution or reproduction in other forums is permitted, provided the original author(s) and the copyright owner(s) are credited and that the original publication in this journal is cited, in accordance with accepted academic practice. No use, distribution or reproduction is permitted which does not comply with these terms.
*Correspondence: Sydney Trask, c210cmFza0BwdXJkdWUuZWR1
Disclaimer: All claims expressed in this article are solely those of the authors and do not necessarily represent those of their affiliated organizations, or those of the publisher, the editors and the reviewers. Any product that may be evaluated in this article or claim that may be made by its manufacturer is not guaranteed or endorsed by the publisher.
Research integrity at Frontiers
Learn more about the work of our research integrity team to safeguard the quality of each article we publish.