- 1 Department of Physiology, Anatomy and Genetics, University of Oxford, Oxford, UK
- 2 Department of Physiology, Anatomy and Cellular Biology, University Pablo de Olavide, Seville, Spain
- 3 Department of Physiology, Development and Neuroscience, University of Cambridge, Cambridge, UK
It has recently been discovered that some forms of timing-dependent long-term depression (t-LTD) require presynaptic N-methyl-d-aspartate (NMDA) receptors. In this review, we discuss the evidence for the presence of presynaptic NMDA receptors at cortical synapses and their possible role in the induction of t-LTD. Two basic models emerge for the induction of t-LTD at cortical synapses. In one model, coincident activation of presynaptic NMDA receptors and CB1 receptors mediates t-LTD. In a second model, CB1 receptors are not necessary, and the activation of presynaptic NMDA receptors alone appears to be sufficient for the induction of t-LTD.
Synaptic Plasticity and NMDA Receptors
One of the most interesting properties of the brain is its ability to change in response to experience. This property has been termed plasticity and is involved in the reorganization of cortical maps during development, and in learning and memory processes in the adult animal (for review, see Malenka and Bear, 2004 ). Plasticity is assumed to be mediated primarily by synaptic changes. Synaptic plasticity can be short-term (lasting from milliseconds to several minutes) or long-term (lasting from hours to months; see Citri and Malenka, 2008 for review). The most extensively studied forms of synaptic plasticity are long-term potentiation (LTP) and long-term depression (LTD). Spike timing-dependent plasticity (STDP) is a Hebbian form of long-term plasticity (Caporale and Dan, 2008 ) and is a strong candidate for a synaptic plasticity mechanism involved in cortical development (Song and Abbott, 2001 ; Feldman and Brecht, 2005 ; Dan and Poo, 2006 ; Caporale and Dan, 2008 ). In STDP, the temporal order and relative timing of pre- and postsynaptic action potentials (spikes), with millisecond precision, determine the direction and magnitude of synaptic change. Thus, timing-dependent (t-) LTP occurs when a presynaptic spike is followed by a postsynaptic spike, whereas t-LTD is induced when this order is reversed (Markram et al., 1997 ; Bi and Poo, 1998 ; Debanne et al., 1998 ; for a detailed review of STDP, see Caporale and Dan, 2008 ). Both t-LTP and t-LTD depend on a specific type of ionotropic glutamate receptor, the N-methyl-d-aspartate (NMDA) receptor, for their induction (Bi and Poo, 1998 ; Debanne et al., 1998 ; Feldman, 2000 ; Sjöström et al., 2003 ).
The ionotropic family of glutamate receptors comprises α–amino-3-hydroxy-5-methyl-4-isoxazolepropionic acid (AMPA), kainate and NMDA receptors, which are widely distributed in the central nervous system (Dingledine et al., 1999 ). NMDA receptors are ligand-gated ion channels permeable to Ca2+, Na+ and K+ ions. These receptors are hetero-tetramers composed of two essential GluN1 and two modulatory GluN2 subunits (using the subunit nomenclature recommended by the IUPHAR; Collingridge et al., 2009 ), which confer different functional, kinetic, pharmacological and signaling properties to the NMDA receptor (for review, see Cull-Candy et al., 2001 ). NMDA receptors participate in normal synaptic transmission, synaptic development and synaptic plasticity, and are involved in the pathogenesis of some neurological states and diseases including stroke, epilepsy, schizophrenia and neuropathic pain (Cull-Candy et al., 2001 ). These receptors have been localized in the postsynaptic membrane where they are activated by the co-agonists glutamate and glycine (or D-serine) and contribute to excitatory postsynaptic responses together with AMPA and kainate receptors. t-LTP depends on postsynaptic NMDA receptors acting as classical coincidence detectors where presynaptic spikes trigger the release of glutamate necessary to activate these receptors, and back-propagating action potentials produce postsynaptic depolarization which relieves the NMDA receptors of their voltage-dependent Mg2+ block leading to influx of Ca2+ ions. Surprisingly, in some cortical areas, postsynaptic loading of the NMDA receptor channel blocker MK-801 blocked t-LTP but not t-LTD, suggesting that NMDA receptors involved in t-LTD are not postsynaptic. (Bender et al., 2006 ; Nevian and Sakmann, 2006 ; Corlew et al., 2007 ; see Corlew et al., 2008 for review). This finding raises the possibility that NMDA receptors involved in t-LTD might have a presynaptic location.
Evidence for Presynaptic NMDA Receptors
The existence of presynaptic NMDA receptors was first proposed following the finding that NMDA receptor agonists facilitated noradrenaline release in synaptosome preparations from the hippocampus (Pittaluga and Raiteri, 1990 , 1992 ; Wang et al., 1992 ) and cerebral cortex (Fink et al., 1990 ; Wang et al., 1992 ), and dopamine release in the striatum (Johnson and Jeng, 1991 ; Krebs et al., 1991 ; Wang, 1991 ). Recently, more evidence has appeared for presynaptic NMDA receptors involved in dopamine release in synaptosomes and synaptoneurosomes in the striatum (Whittaker et al., 2008 ). Evidence for presynaptic NMDA receptors was also found at neuromuscular synapses from Xenopus in culture where NMDA enhances transmitter release (Fu et al., 1995 ). The existence of presynaptic NMDA receptors has also been supported by anatomical evidence. Anatomical support for presynaptic NMDA receptors has come from immuno-electron microscopy experiments which have identified NMDA receptor immunolabeling in presynaptic elements of the neocortex (Aoki et al., 1994 ; DeBiasi et al., 1996 ; Charton et al., 1999 ; Corlew et al., 2007 ), the hippocampus (Siegel et al., 1994 ; Charton et al., 1999 ; Jourdain et al., 2007 ), the spinal cord (Liu et al., 1994 ), the amygdala (Farb et al., 1995 ; Pickel et al., 2006 ) and the cerebellum (Petralia et al., 1994 ; Bidoret et al., 2009 ). Functionally, presynaptic NMDA receptors have been proposed to exist on both excitatory and inhibitory boutons, where they could modulate transmitter release. At cortical glutamatergic synapses they have generally been suggested to serve as facilitatory autoreceptors, reversibly enhancing glutamate release. A transient decrease of miniature excitatory postsynaptic current (mEPSC) frequency was seen following the application of the NMDA receptor antagonist D-AP5 when postsynaptic NMDA receptors were previously blocked by intracellular loading of MK-801 or by hyperpolarization. This was first demonstrated in the entorhinal cortex (Berretta and Jones, 1996 ; Woodhall et al., 2001 ) and subsequently in the visual cortex (Sjöström et al., 2003 ; Corlew et al., 2007 ; Li and Han, 2007 ; Li et al., 2008 ), somatosensory cortex (Bender et al., 2006 ; Brasier and Feldman, 2008 ) and hippocampus (Mameli et al., 2005 ; Jourdain et al., 2007 ; see Corlew et al., 2008 for review). Apart from the cerebral cortex, there is also evidence for physiologically active presynaptic NMDA receptors in the cerebellum (Glitsch and Marty, 1999 ; Casado et al., 2000 ; Duguid and Smart, 2004 ; Fiszman et al., 2005 ), amygdala (Humeau et al., 2003 ) and spinal cord (Liu et al., 1997 ; Bardoni et al., 2004 ). For broader reviews on the evidence for presynaptic glutamate receptors, see MacDermott et al. (1999) , Engelman and MacDermott (2004) and Pinheiro and Mulle (2008) .
Presynaptic NMDA receptors have been implicated in plasticity at both excitatory and inhibitory synapses, including heterosynaptic associative LTP at thalamic and cortical afferent synapses in the amygdala (Humeau et al., 2003 ), depolarization-induced potentiation (Duguid and Smart, 2004 ) and LTD (Casado et al., 2002 ) in the cerebellum, LTD at GABAergic synapses in the tadpole optic tectum (Lien et al., 2006 ) and t-LTD in different cortical areas as discussed by Duguid and Sjöström (2006) and Corlew et al. (2008) .
While these putative functional NMDA receptors are generally assumed to be at axonal locations, the existence of presynaptic, axonal NMDA receptors has been challenged by the discovery that somatodendritic NMDA receptor activation can affect axonal Ca2+ levels through voltage-dependent calcium channel activation, at least in cerebellar stellate cells (Christie and Jahr, 2008 ). A further challenge has come from the apparent lack of direct effect of NMDA application on axonal Ca2+ levels and axon excitability in cortical layer (L) 5 pyramidal neurons (Christie and Jahr, 2009 ).
To summarize, experiments in synaptosomes are suggestive of NMDA receptors being present in presynaptic boutons; immuno-electron microscopy experiments are also consistent with axonal NMDA receptors since immunolabeling has been found in axons in different regions. In slices, the existence of axonal presynaptic NMDA receptors has been proposed based on the observation that the addition of an NMDA receptor antagonist affects spontaneous, miniature and evoked neurotransmitter release, even after intracellular blockade of postsynaptic NMDA receptors (see Corlew et al., 2008 for review). The recent experiments by Christie and Jahr (2008 , 2009) question the interpretation of these results, suggesting that the observed effects could be mediated by NMDA receptors located in the somatodendritic compartment of the presynaptic neuron.
To unequivocally demonstrate the existence of functional presynaptic axonal NMDA receptors a combination of different approaches will be required (see Corlew et al., 2008 for review):
(1) Immunogold electron microscopy (Farb et al., 1995 );
(2) Direct monitoring of presynaptic function by calcium imaging whilst adding agonists or antagonists at NMDA receptors (Shin and Linden, 2005 );
(3) Direct electrophysiological recording from presynaptic boutons (Fiszman et al., 2005 );
(4) Direct loading of NMDA receptor antagonists into the presynaptic neuron (Rodríguez-Moreno and Paulsen, 2008 ); and
(5) Compartment-specific interference with NMDA receptor function using molecular or genetic tools (Lynch, 2004 ; Safo and Regehr, 2005 ).
Role of Presynaptic NMDA Receptors in Spike Timing-Dependent LTD
The first evidence for a role of presynaptic NMDA receptors in STDP came from experiments at L5-L5 synapses of visual cortex where an NMDA receptor-dependent presynaptic form of t-LTD was described (Sjöström et al., 2003 ). This t-LTD requires activation of postsynaptic group I mGluRs and postsynaptic Ca2+ elevation. Results indicate that this form of t-LTD is expressed as a reduction in the probability of neurotransmitter release, thus implicating a retrograde signal from the postsynaptic to the presynaptic compartment (Sjöström et al., 2003 ). This retrograde messenger has been suggested to be endocannabinoids, which mediate many forms of short-term (Wilson et al., 2001 ; Brown et al., 2004 ) and long-term plasticity (Chevaleyre et al., 2006 ). Thus, it has been proposed that the coincidence detector for t-LTD at this synapse is presynaptic and involves both presynaptic NMDA receptors and cannabinoid receptor type 1 (CB1 receptors) (Sjöström et al., 2003 ) (Figure 1 ).
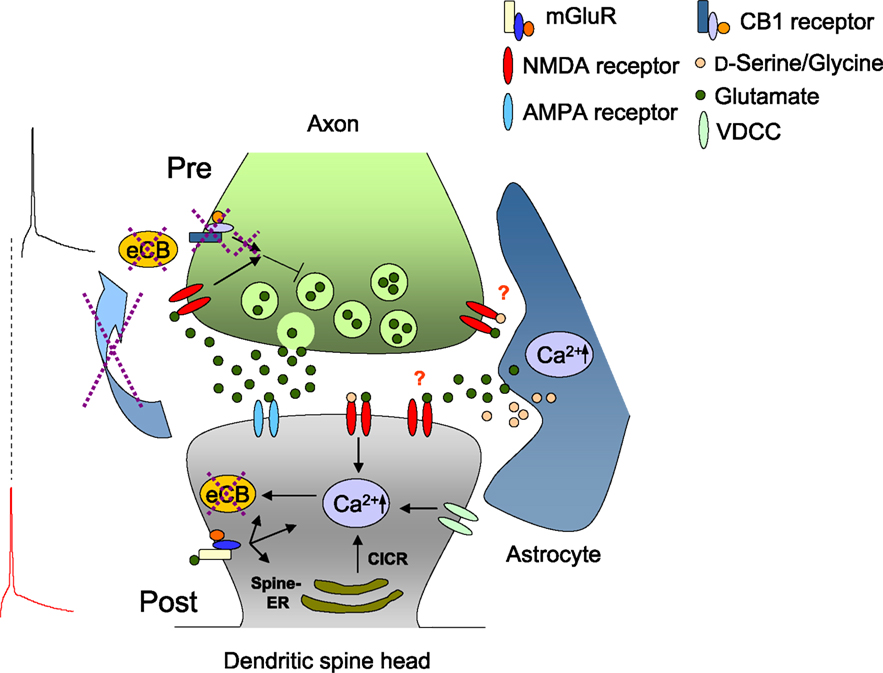
Figure 1. Two models of presynaptic NMDA receptor-dependent t-LTD. Model 1: Presynaptic NMDA receptors and CB1 receptors drive t-LTD. In this model, during post-before-pre pairing, presynaptically released glutamate activates mGluRs and postsynaptic action potentials enhance Ca2+ influx. This would lead to endocannabinoid (eCB) synthesis. eCB diffuses retrogradely and binds to presynaptic CB1 receptors. Co-activation of presynaptic CB1 receptors and presynaptic NMDA receptors causes synaptic depression. In this model, presynaptic NMDA receptors are activated by glutamate release from the presynaptic terminal. Astrocytic release of gliotransmitters (glutamate as agonist and/or D-serine or glycine as co-agonist) may contribute to activating neuronal NMDA receptors. Model 2: In a second model, eCB-dependent retrograde signaling is not necessary for induction of t-LTD (dashed crosses), and activation of presynaptic NMDA receptors alone appears to be sufficient to drive presynaptic t-LTD. The source of transmitter activating presynaptic NMDA receptors is unknown.
Direct evidence for presynaptic NMDA receptor involvement in t-LTD has recently been obtained at L4-L2/3 synapses of somatosensory cortex using dual whole-cell recordings of synaptically-connected L4 and L2/3 neurons by loading the NMDA receptor channel blocker MK-801 intracellularly via a patch pipette into pre- or postsynaptic neurons. Induction of t-LTD was unaffected by postsynaptic loading of MK-801 but completely blocked by presynaptic MK-801, indicating that t-LTD requires presynaptic, but not postsynaptic NMDA receptors (Rodríguez-Moreno and Paulsen, 2008 ).
The subunit composition of these presynaptic receptors has been analyzed (Banerjee et al., 2009 ). For this purpose, two GluN2C/D subunit-preferring NMDA receptor antagonists, PPDA and UBP141 were used. These compounds selectively blocked t-LTD at L4-L2/3 synapses with no effect on t-LTP at the same synapses, which was instead blocked by the GluN2A subunit-preferring antagonist NVP-AAM077. They also had no blocking effect on t-LTD at L2/3-L2/3 synapses, which was, however, blocked by a GluN2B subunit-selective antagonist (Ro 25-6981; Banerjee et al., 2009 ). Compounds currently available are not highly selective for GluN2C/D-containing NMDA receptors and, moreover, are competitive antagonists. Thus, the inhibition produced depends on the effective glutamate concentration (Neyton and Paoletti, 2006 ), precluding strong conclusions to be drawn. Nevertheless, the double dissociation found, and the fact that these GluN2C/D subunit-preferring antagonists did not block t-LTD at a different synapse, suggest that L4-L2/3 presynaptic NMDA receptors contain GluN2C/D subunits (Banerjee et al., 2009 ). When available, experiments with GluN2C/D non-competitive antagonists should confirm whether results are due to different subunit composition of the receptors at pre- and postsynaptic sites or to different kinetics of glutamate transients at different locations. The current lack of selective compounds that can distinguish between GluN2C and GluN2D subunits precludes an investigation into whether it is GluN2C or GluN2D that is the important subunit for the induction of t-LTD. The possible involvement of presynaptic GluN2C/D subunits in t-LTD at layer 4-to-layer 2/3 synapses is particularly interesting because the deactivation time constant of GluN2C/D subunit-containing receptors is very slow (Momiyama et al., 1996 ; Brothwell et al., 2008 ; Wyllie, 2008 ). This might be relevant for the particularly broad time window for induction of t-LTD at this synapse (Feldman, 2000 ).
Several lines of evidence indicate that this form of t-LTD is presynaptic: (i) t-LTD is blocked when presynaptic NMDA receptors are blocked by internal MK-801 in recordings from pairs of synaptically-connected L4 and L2/3 cells (Rodríguez-Moreno and Paulsen, 2008 ), (ii) an increase in paired-pulse ratio is observed after a t-LTD protocol (Bender et al., 2006 ), and (iii) coefficient of variation (CV) analysis is consistent with presynaptic expression (Rodríguez-Moreno and Paulsen, 2008 ).
Although previous studies have implicated endocannabinoid signaling through CB1 receptors in this form of t-LTD at rat L4-L2/3 synapses (Bender et al., 2006 ), it was recently reported that t-LTD does not need CB1 receptor activation at the mouse L4-L2/3 synapse (Hardingham et al., 2008 ; Banerjee et al., 2009 ), suggesting a possible species and/or age difference. In contrast, CB1 receptors are necessary for induction of t-LTD at horizontal synapses (L2/3-L2/3), supporting the idea that different excitatory synapses onto the same postsynaptic neurons can have different requirements for the induction of synaptic plasticity (Banerjee et al., 2009 ).
It is clear from these results that endocannabinoids are not obligatory for all forms of timing-dependent synaptic depression. The results also suggest that at least two distinct forms of presynaptic NMDA receptor-dependent LTD can be dissociated, one dependent on endocannabinoid signaling and the GluN2B subunit (Sjöström et al., 2003 ; Banerjee et al., 2009 ) (Figure 1 ), and another, independent of endocannabinoids but dependent on presynaptic NMDA receptors containing GluN2C/D subunits (Rodríguez-Moreno and Paulsen, 2008 ; Banerjee et al., 2009 ) (Figure 1 ). Notably, both of these forms of t-LTD have in common a dependence on presynaptic NMDA receptors, suggesting that NMDA receptors mediate t-LTD while CB1 receptors may have a permissive role.
Mechanism of Presynaptic t-LTD
The results described above suggest that, at L4-L2/3 synapses, t-LTD is mediated by presynaptic NMDA receptors that contain GluN2C/D subunits. Since there is no evidence that other presynaptic receptors are implicated, we suggest that presynaptic NMDA receptors are effectively mediating this form of t-LTD. In this model, postsynaptic spikes allow the activation of presynaptic NMDA receptors when followed by a presynaptic spike. It is, however, unknown whether the depolarization requirement often observed for unblocking NMDA receptors is necessary for activation of presynaptic NMDA receptors, since GluN2C/D and GluN3A-containing NMDA receptors show less voltage sensitivity than other NMDA receptor types (Cull-Candy et al., 2001 ; Clarke and Johnson, 2006 ). The presence of NMDA receptors with low conductance and reduced susceptibility to Mg2+ block in the presynaptic layer 4 spiny stellate cells was reported earlier using transgenic mice (Binshtok et al., 2006 ). Another interesting aspect to consider is the relationship with frequency in the induction of this form of t-LTD. t-LTD has been observed in neocortical slices using different stimulation frequencies from 0.1 to 20 Hz, indicating that this form of plasticity can be elicited at low frequencies of stimulation (Feldman, 2000 ; Sjöström et al., 2001 , 2003 ; Bender et al., 2006 ; Nevian and Sakmann, 2006 ; Rodríguez-Moreno and Paulsen, 2008 ; Banerjee et al., 2009 ). However, at 40 Hz and above, only t-LTP was observed, irrespective of the timing between pre- and postsynaptic action potentials (Sjöström et al., 2001 ). Timing-dependent LTD at L4-L2/3 synapses in synaptically-connected cells during paired recordings can be induced by pairing single presynaptic and postsynaptic action potentials at 0.2 Hz (Rodríguez-Moreno and Paulsen, 2008 ), constraining the possible mechanisms involved in this form of t-LTD.
The requirement of presynaptic NMDA receptors for t-LTD raises several interesting questions:
(i) What is the source of the transmitter that activates presynaptic NMDA receptors?
In principle, there are several possible different sources of transmitter mediating the activation of presynaptic NMDA receptors. Glutamate could be released by the presynaptic neuron and NMDA receptors activated as autoreceptors. Glutamate could also be released by the postsynaptic neuron, as retrograde release of glutamate has been suggested (Harkany et al., 2004 ). Glial cells have also been shown to release glutamate and modulate synaptic transmission and plasticity (see Perea et al., 2009 for review). Co-agonists at NMDA receptors, such as d-serine, are also released by astrocytes and have recently been shown to be involved in plasticity (Henneberger et al., 2010 ). Glutamate (and/or co-agonists at NMDA receptors) of glial origin could reach presynaptic NMDA receptors and activate them (Jourdain et al., 2007 ). Another possible source of transmitter could be the spillover from neighboring synapses. Spillover of transmitter appears less likely, however, since t-LTD can be induced at very low frequency in pairs of synaptically-connected cells, leaving the postsynaptic neuron and glial cells as the most likely sources. The exact source of transmitter that activates presynaptic NMDA receptors remains to be determined.
(ii) Is the activation of these presynaptic NMDA receptors tonic or phasic in nature?
Rodríguez-Moreno and Paulsen (2008) showed that the application of the NMDA receptor antagonist d-AP5 did not alter the EPSP slope at the L4-L2/3 synapse, suggesting that these receptors are not tonically active. Brasier and Feldman (2008) found that addition of d-AP5 caused a reduction of AMPA currents at the L4-L2/3 synapse, suggesting that, in principle, these receptors could be tonically activated, though these results were obtained in the presence of glutamate transporter blockers.
(iii) What is the role of the postsynaptic action potential in the pairing protocol?
Induction of t-LTD at this synapse requires pairing of postsynaptic action potentials with presynaptic activity. The exact role of the postsynaptic action potentials has not yet been determined. Previous experiments have shown that this form of LTD requires a rise in postsynaptic Ca2+ as it is blocked by the presence of BAPTA in the postsynaptic cell (Bender et al., 2006 ; Nevian and Sakmann, 2006 ). The postsynaptic action potential could mediate Ca2+ entry through voltage-dependent calcium channels and/or induce release of Ca2+ from internal stores. This implies that a Ca2+–dependent signal from the postsynaptic neuron is most probably necessary, but the nature of this signal remains to be determined.
(iv) Why do t-LTP protocols not also produce LTD?
If a slow Ca2+–dependent postsynaptic process is involved in the induction of t-LTD, one might expect that the postsynaptic condition for induction of t-LTD would also be satisfied during a pre-before-post paradigm. Why do t-LTP protocols not also induce t-LTD? Postsynaptic action potentials might trigger the release of a retrograde messenger that acts on the presynaptic element during the presynaptic action potential. Because the time window for post-before-pre pairing is relatively narrow, it would suggest that this retrograde signal has to act in a similarly short time window (∼10 ms) to operate as a presynaptic coincidence signal. This time scale would appear to make postsynaptic enzyme-dependent mechanisms less likely candidates to provide the presynaptic coincidence signal during induction of t-LTD. There is clearly more work to be done before we understand the detailed mechanisms of induction of presynaptic NMDA receptor-dependent t-LTD.
Conclusion
In conclusion, spike timing-dependent LTD requires presynaptic NMDA receptors at some cortical synapses. Two basic models are emerging to explain the mechanism of these presynaptic forms of t-LTD. In one model, presynaptic NMDA receptors and CB1 receptors mediate t-LTD (Sjöström et al., 2003 ). A second model, suggests that presynaptic NMDA receptors mediate a form of t-LTD that is independent of CB1 receptor activation (Rodríguez-Moreno and Paulsen, 2008 ; Banerjee et al., 2009 ). Notably, both forms of t-LTD have in common a dependence of presynaptic NMDA receptors, suggesting that NMDA receptors, might be essential to mediate t-LTD, while CB1 receptors have a permissive role when involved.
Conflict of Interest Statement
The authors declare that the research was conducted in the absence of any commercial or financial relationships that could be construed as a potential conflict of interest.
Acknowledgments
Antonio Rodríguez-Moreno was supported by a Marie Curie Intra-European Fellowship, by a Royal Society International Short Visit Grant and by a Network Grant from the McDonnell Network for Cognitive Neuroscience, University of Oxford. Abhishek Banerjee was supported by a Felix scholarship from the University of Oxford.
References
Aoki, C., Venkatesam, C., Go, C. G., Mong, J. A., and Dawson, T. M. (1994). Cellular and subcellular localization of NMDA-R1subunit immunorreactivity in the visual cortex of adult and neonatal rats. J. Neurosci. 14, 5202–5222.
Banerjee, A., Meredith, R. M., Rodríguez-Moreno, A., Mierau, S. B., Auberson, Y. P., and Paulsen, O. (2009). Double dissociation of spike timing-dependent potentiation and depression by subunit-preferring NMDA receptor antagonists in mouse barrel cortex. Cereb. Cortex 19, 2959–2969.
Bardoni, R., Torsney, C., Tong, C.-T., Prandini, M., and MacDermott, A. B. (2004). Presynaptic NMDA receptors modulate glutamate release from primary sensory neurons in rat spinal cord dorsal horn. J. Neurosci. 24, 2774–2781.
Bender, V. A., Bender, K. J., Brasier, D. J., and Feldman, D. E. (2006). Two coincidence detectors for spike timing-dependent plasticity in somatosensory cortex. J. Neurosci. 16, 4166–4177.
Berretta, N., and Jones, R. S. (1996). Tonic facilitation of glutamate release by presynaptic N-methyl-D-aspartate autoreceptors in the entorhinal cortex. Neuroscience 75, 339–344.
Bi, G.-Q., and Poo, M.-M. (1998). Synaptic modifications in cultured hippocampal neurons: dependence of spike timing, synaptic strength, and postsynaptic cell type. J. Neurosci. 18, 10464–10472.
Bidoret, C., Ayon, A., Barbour, B., and Casado, M. (2009). Presynaptic NR2A-containing NMDA receptors implement a high-pass filter synaptic plasticity rule. Proc. Natl. Acad. Sci. U.S.A. 106, 14126–14131.
Binshtok, A. M., Fleidervish, I. A., Sprengel, R., and Gutnick, M. J. (2006). NMDA receptors in layer 4 spiny stellate cells of the mouse barrel cortex contain the NR2C subunit. J. Neurosci. 26, 708–715.
Brasier, D. J., and Feldman, D. E. (2008). Synapse-specific expression of functional presynaptic NMDA receptors in rat somatosensory cortex. J. Neurosci. 28, 2199–2211.
Brothwell, S. L., Barber, J. L., Monaghan, D. T., Jane, D. E., Gibb, A. J., and Jones, S. (2008). NR2B- and NR2D-containing synaptic NMDA receptors in developing rat substantia nigra pars compacta dopaminergic neurones. J. Physiol. 586, 739–750.
Brown, S. P., Safo, P. K., and Regehr, W. G. (2004). Endocannabinoids inhibit transmission at granule cell to Purkinje cell synapses by modulating three types of presynaptic calcium channels. J. Neurosci. 24, 5623–5631.
Caporale, N., and Dan, Y. (2008). Spike timing-dependent plasticity: A Hebbian learning rule. Annu. Rev. Neurosci. 31, 25–46.
Casado, M., and Dieudonne, S, Ascher, P. (2000). Presynaptic N-methyl-D-aspartate receptors at the parallel fiber-Purkinje cell synapse. Proc. Natl. Acad. Sci. U.S.A. 97, 11593–11597.
Casado, M., Isope, P., and Asher, P. (2002). Involvement of presynaptic N-methyl-D-aspartate receptors in cerebellar long-term depression. Neuron 33, 123–130.
Charton, J. P., Herkert, M., Becker, C. M., and Schroder, H. (1999). Cellular and subcellular localization of the 2B-subunit of the NMDA receptor in the adult rat telencephalon. Brain Res. 816, 609–617.
Chevaleyre, V., Takahashi, K. A., and Castillo, P. E. (2006). Endocannabinoid-mediated synaptic plasticity in the CNS. Annu. Rev. Neurosci. 29, 37–76.
Christie, J. M., and Jahr, C. E. (2008). Dendritic NMDA receptors activate axonal calcium channels. Neuron 60, 298–307.
Christie, J. M., and Jahr, C. E. (2009). Selective expression of ligand-gated ion channels in L5 pyramidal cell axons. J. Neurosci. 29, 11441–11450.
Citri, A., and Malenka, R. C. (2008). Synaptic plasticity: multiple forms, functions and mechanisms. Neuropsychopharmacology 33, 18–41.
Clarke, R. J., and Johnson, J. W. (2006). NMDA receptor NR2 subunit dependence of the slow component of magnesium unblock. J. Neurosci. 26, 5825–5834.
Collingridge, G. L., Olsen, R. W., Peters, J., and Spedding, M. (2009). A nomenclature for ligand-gated ion channels. Neuropharmacology 56, 2–5.
Corlew, R., Brasier, D. J., Feldman, D. E., and Philpot, B. D. (2008). Presynaptic NMDA receptors: newly appreciated roles in cortical synaptic function and plasticity. Neuroscientist 14, 609–625.
Corlew, R., Wang, Y., Ghermazien, H., Erisir, A., and Philpot, B. D. (2007). Developmental switch in the contribution of presynaptic and postsynaptic NMDA receptors to long-term depression. J. Neurosci. 27, 9835–9845.
Cull-Candy, S., Brickely, S., and Farrant, M. (2001). NMDA receptor subunits: diversity, development and disease. Curr. Opin. Neurobiol. 11, 327–335.
Dan, Y., and Poo, M. M. (2006). Spike timing-dependent plasticity: from synapse to perception. Physiol. Rev. 86, 1033–1048.
Debanne, D., Gahwiler, B. H., and Thompson, S. M. (1998). Long-term synaptic plasticity between pairs of individual CA3 pyramidal cells in rat hippocampal slice cultures. J. Physiol. 507, 237–247.
DeBiasi, S., Minnelli, A., Melone, M., and Conti, F. (1996). Presynaptic NMDA receptors in the neocortex are both auto- and heteroreceptors. Neuroreport 7, 2773–2776.
Dingledine, R., Borges, K., Bowie, D., and Traynelis, S. F. (1999). The glutamate receptor ion channels. Pharmacol. Rev. 51, 7–61.
Duguid, I. C., and Sjöström, P. J. (2006). Novel presynaptic mechanisms for coincidence detection in synaptic plasticity. Curr. Opin. Neurobiol. 16, 312–322.
Duguid, I. C., and Smart, T. G. (2004). Retrograde activation of presynaptic NMDA receptors enhances GABA release at cerebellar interneuron-Purkinje cell synapses. Nat. Neurosci. 7, 525–533.
Engelman, H. S., and MacDermott, A. B. (2004). Presynaptic ionotropic receptors and control of transmitter release. Nat. Rev. Neurosci. 5, 135–145.
Farb, C. R., Aoki, C., and Ledoux, J. E. (1995). Differential localization of NMDA and AMPA receptor subunits in the lateral and basal nuclei of the amygdala: a light and electron microscopic study. J. Comp. Neurol. 362, 86–108.
Feldman, D. E. (2000). Timing-based LTP and LTD at vertical inputs to layer II/III pyramidal cells in rat barrel cortex. Neuron 27, 45–56.
Feldman, D. E., and Brecht, M. (2005). Map plasticity in somatosensory cortex. Science 310, 810–815.
Fink, K., Bonisch, H., and Gothert, M. (1990). Presynaptic NMDA receptors stimulate noradrenaline release in the cerebral cortex. Eur. J. Pharmacol. 185, 115–117.
Fiszman, M. L., Barberis, A., Lu, C., Fu, Z., Erdelyi, F., Szabo, G., and Vicini, S. (2005). NMDA receptors increase the size of GABAergic terminals and enhance GABA release. J. Neurosci. 25, 2024–2031.
Fu, W., Liou, J., Lee, Y., and Liou, H. (1995). Potentiation of neurotransmitter release by activation of presynaptic glutamate receptors at developing neuromuscular synapses of Xenopus. J. Physiol. 489, 813–823.
Glitsch, M., and Marty, A. (1999). Presynaptic effects of NMDA in cerebellar Purkinje cells and interneurons. J. Neurosci. 19, 511–519.
Hardingham, N., Wright, N., Dachtler, J., and Fox, K. (2008). Sensory deprivation unmasks a PKA-dependent synaptic plasticity mechanism that operates in parallel with CaMKII. Neuron 60, 861–874.
Harkany, T., Holmgren, C., Härtig, W., Qureshi, T., Chaudhry, F. A., Storm-Mathisen, J., Dobszay, M. B., Berghuis, P., Schulte, G., Sousa, K. M., Fremeau, R. T. Jr., Edwards, R. H., Mackie, K., Ernfors, P., and Zilberter,Y. (2004). Endocannabinoid-independent retrograde signaling at inhibitory synapses in layer 2/3 of neocortex: involvement of vesicular glutamate transporter 3. J. Neurosci. 24, 4978–4988.
Henneberger, C., Papouin, T., Oliet, S. H., and Rusakov, D. A. (2010). Long-term potentiation depends on release of D-serine from astrocytes. Nature 463, 232–236.
Humeau, Y., Shaban, H., Bissiere, S., and Lüthi, A. (2003). Presynaptic induction of heterosynaptic associative plasticity in the mammalian brain. Nature 426, 841–845.
Johnson, K. M., and Jeng, Y. J. (1991). Pharmacological evidence for N-methyl-D-aspartate receptors on nigrostriatal dopaminergic terminals. Can. J. Physiol. Pharmacol. 69, 1416–1421.
Jourdain, P., Bergersen, L. H., Bhaukaurally, K., Bezzi, P., Santello, M., Domercq, M., Matute, C., Tonello, F., Gundersen, V., and Volterra, A. (2007). Glutamate exocytosis from astrocytes controls synaptic strength. Nat. Neurosci. 10, 331–339.
Krebs, M. O., Desce, J. M., Kemel, M. L., Gauchy, C., Godeheu, G., Cheramy, A., and Glowinski, J. (1991). Glutamatergic control of dopamine release in the rat striatum: evidence for presynaptic N-methyl-D-aspartate receptors on dopamine nerve terminals. J. Neurochem. 56, 81–85.
Li, Y. H., and Han, T. Z. (2007). Glycine binding sites of presynaptic NMDA receptors may tonically regulate glutamate release in the rat visual cortex. J. Neurophysiol. 97, 817–823.
Li, Y. H., Han, T. Z., and Meng, K. (2008). Tonic facilitation of glutamate release by glycine binding sites on presynaptic NR2B-containing NMDA autoreceptors in the rat visual cortex. Neurosci. Lett. 432, 212–216.
Lien, C. C., Mu, Y. Vargas-Caballero, M., and Poo, M. M. (2006). Visual stimuli-induced LTD of GABAergic synapses mediated by presynaptic NMDA receptors. Nat. Neurosci. 9, 372–380.
Liu, H., Mantyh, P. W., and Basbaum, A. I. (1997). NMDA-receptor regulation of substance P release from primary afferent nociceptors. Nature 386, 721–724.
Liu, H., Wang, H., Sheng, M., Jan, L. Y., Jan, Y. N., and Basbaum, A. I. (1994). Evidence for presynaptic N-methyl-D-aspartate autoreceptors in the spinal cord dorsal horn. Proc. Natl. Acad. Sci. U.S.A. 91, 8383–8387.
MacDermott, A. B., Role, L. W., and Siegelbaum, S. A. (1999). Presynaptic ionotropic receptors and the control of transmitter release. Annu. Rev. Neurosci. 22, 443–485.
Mameli, M., Carta, M., Partridge, L. D., and Valenzuela, C. F. (2005). Neurosteroid-induced plasticity of immature synapses via retrograde modulation of presynaptic NMDA receptors. J. Neurosci. 25, 2285–2294.
Markram, H., Lübke, J. Frotscher, M., and Sakmann, B. (1997). Regulation of synaptic efficacy by coincidence of postsynaptic APs and EPSPs. Science 275, 213–215.
Momiyama, A., Feldmeyer, D., and Cull-Candy, S. G. (1996). Identification of a native low-conductance NMDA channel with reduced sensitivity to Mg2+ in rat central neurones. J. Physiol. 494, 479–492.
Nevian, T., and Sakmann, B. (2006). Spine Ca2+ signaling in spike-timing-dependent plasticity. J. Neurosci. 43, 11001–11013.
Neyton, J., and Paoletti, P. (2006). Relating NMDA receptor function to receptor subunit composition: limitations of the pharmacological approach. J. Neurosci. 26,1331–1333.
Perea, G., Navarrete, M., and Araque, A. (2009). Tripartite synapses: astrocytes process and control synaptic information. Trends Neurosci. 32, 421–431.
Petralia, R. S., Wang, Y. X., and Wenthold, R. J. (1994). The NMDA receptor subunits NR2A and NR2B show histological and ultrastructural localization patterns similar to those of NR1. J. Neurosci. 14, 6102–6120.
Pickel, V. M., Colago, E. E., Mania, I., Molosh, A. I., and Rainnie, D. G. (2006). Dopamine D1 receptors co-distribute with N-methyl-D-aspartic acid type-1 subunits and modulate synaptically-evoked N-methyl-D-aspartic acid currents in basolateral amygdala. Neuroscience 142, 671–690.
Pinheiro, P. S., and Mulle, C. (2008). Presynaptic glutamate receptors: physiological functions and mechanisms of action. Nat. Rev. Neurosci. 9, 423–435.
Pittaluga, A., and Raiteri, M. (1990). Release-enhancing glycine-dependent presynaptic NMDA receptors exist on noradrenergic terminals of hippocampus. Eur. J. Pharmacol. 191, 231–234.
Pittaluga, A., and Raiteri, M. (1992). N-methyl-D-aspartic acid (NMDA) and non-NMDA receptors regulating hippocampal norepinephrine release. I. Location on axon terminals and pharmacological characterization. J. Pharmacol. Exp. Ther. 260, 232–237.
Rodríguez-Moreno, A., and Paulsen, O. (2008). Spike timing-dependent long-term depression requires presynaptic NMDA receptors. Nat. Neurosci. 11, 744–745.
Safo, P. K., and Regehr, W. G. (2005). Endocannabinoids control the induction of LTD. Neuron 48, 647–659.
Shin, J. H., and Linden, D. J. (2005). An NMDA receptor/nitric oxide cascade is involved in cerebellar LTD but is not localized to the parallel fiber terminal. J. Neurophysiol. 94, 4281–4289.
Siegel, S. J., Brose, N., Janssen, W. G., Gasic, G. P., Jahn, R., Heinemann, S. F., and Morrison, J. H. (1994). Regional, cellular, and ultrastructural distribution of N-methyl-D-aspartate receptor subunit 1 in monkey hippocampus. Proc. Natl. Acad. Sci. U.S.A. 91, 564–568.
Sjöström, P. J., Turrigiano, G. G., and Nelson, S. B. (2001). Rate, timing, and cooperativity jointly determine cortical synaptic plasticity. Neuron 32, 1149–1164.
Sjöström, P. J., Turrigiano, G. G., and Nelson, S. B. (2003). Neocortical LTD via coincident activation of presynaptic NMDA and cannabinoid receptors. Neuron 39, 641–654.
Song, S., and Abbott, L. F. (2001). Cortical development and remapping through spike timing-dependent plasticity. Neuron 39, 339–350.
Wang, J. K. T. (1991). Presynaptic NMDA receptors modulate dopamine release from striatal synaptosomes. J. Neurochem. 57, 819–822.
Wang, J. K. T., Andrews, H., and Thkral, V. (1992). Presynaptic NMDA receptors regulate noradrenaline release from isolated nerve terminals. J. Neurochem. 58, 204–211.
Whittaker, M. T., Gibbs, T. T., and Farb, D. H. (2008). Pregnenolone sulfate induces NMDA receptor dependent release of dopamine from synaptic terminals in the striatum. J. Neurochem. 107, 510–521.
Wilson, R. I., Kunos, G., and Nicoll, R. A. (2001). Presynaptic specificity of endocannabinoid signaling in the hippocampus. Neuron 31, 453–462.
Woodhall, G., Evans, D. I., Cunningham, M. O., and Jones, R. S. (2001). NR2B-containing NMDA autoreceptors at synapses on entorhinal cortical neurons. J. Neurophysiol. 86, 1644–1651.
Keywords: plasticity, STDP, t-LTD, NMDA, presynaptic mechanisms
Citation: Rodríguez-Moreno A, Banerjee A and Paulsen O (2010) Presynaptic NMDA receptors and spike timing-dependent long-term depression at cortical synapses. Front. Syn. Neurosci. 2:18. doi: 10.3389/fnsyn.2010.00018
Received: 12 February 2010;
Paper pending published: 05 March 2010;
Accepted: 24 May 2010;
Published online: 17 June 2010
Edited by:
Per Jesper Sjöström, University College London, UKReviewed by:
Ian Duguid, The University of Edinburgh, UKMariano Casado, Ecole Normale Supérieure, France
Copyright: © 2010 Rodríguez-Moreno, Banerjee and Paulsen. This is an open-access article subject to an exclusive license agreement between the authors and the Frontiers Research Foundation, which permits unrestricted use, distribution, and reproduction in any medium, provided the original authors and source are credited.
*Correspondence:
Antonio Rodríguez-Moreno, Department of Physiology, Anatomy and Cellular Biology, University Pablo de Olavide, Laboratory of Cellular Neuroscience and Plasticity, Ctra. de Utrera, Km. 141013, Seville, Spain. e-mail: arodmor@upo.es;
Ole Paulsen, Department of Physiology, Development and Neuroscience, University of Cambridge, The Neuronal Oscillations Group, Physiological Laboratory, Downing Street, Cambridge CB2 3EG, UK. e-mail: op210@cam.ac.uk