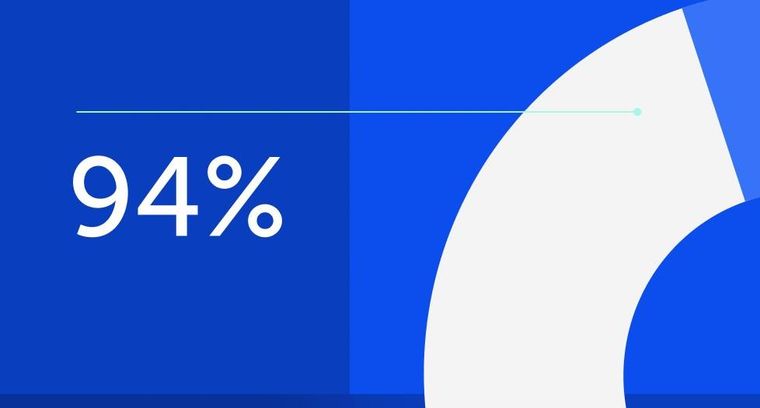
94% of researchers rate our articles as excellent or good
Learn more about the work of our research integrity team to safeguard the quality of each article we publish.
Find out more
EDITORIAL article
Front. Mol. Neurosci., 26 March 2025
Sec. Molecular Signalling and Pathways
Volume 18 - 2025 | https://doi.org/10.3389/fnmol.2025.1586940
This article is part of the Research TopicMechanisms of Cholinergic Transmission in Motivation and CognitionView all 7 articles
Editorial on the Research Topic
Mechanisms of cholinergic transmission in motivation and cognition
Acetylcholine was one of the first fast neurotransmitters to be discovered and measured in synaptic recordings (Loewi and Navratil, 1926; Dale, 1914, 1934; For a historical overview see Tansey, 2006). Since then, researchers have found a central role for acetylcholine in numerous functions including attention, memory, motivation, and mood (Tobin, 2024; Cox and Witten, 2019; Picciotto et al., 2012). Disruption of cholinergic transmission has been found in various neuropathology, from Alzheimer's Disease to Addiction disorders (Zhang et al., 2024; Tobin, 2024; Williams and Adinoff, 2008).
For this Research Topic, we have selected 6 original research and review articles exploring the molecular mechanisms of cholinergic transmission in motivation, reward, and reinforcement learning. Including, novel studies on the role of distinct receptor populations and circuitry relevant for attention, reward and motivation (Fritz et al.; Braunscheidel et al.; Kim et al.; Berezovskaia et al.), as well as two reviews of the most relevant studies on cholinergic circuits and striatal cholinergic interneurons (Ratna and Francis; Runyon et al.).
All main cholinergic brain systems have been linked to reward related computations (Ruan et al., 2022). Cholinergic input to the motivation-associated mesolimbic dopamine system arises from distinct neural populations, including projections from two brainstem nuclei, the laterodorsal tegmental nucleus and the pedunculopontine nucleus (PPN), as well as a small population of interneurons in the striatum, comprising 1%−2% of all striatal neurons (Dautan et al., 2014; Oakman et al., 1995). Acetylcholine exerts its actions through two receptor families, which originate from distinct genes and are functionally classified by their pharmacological ligand selectivity: Nicotinic and muscarinic acetylcholine receptors.
Nicotinic acetylcholine receptors are fast-acting ligand-gated ion channels that facilitate cation influx upon acetylcholine binding, while Muscarinic receptors are slow-acting G-protein coupled receptors, mediating modulatory effects on target neurons (Tobin, 2024; Mihailescu and Drucker-Colin, 2000). In the mammalian central nervous system (CNS), restrictive or selective expression of these receptors in different brain regions dictate their function (Ahmed et al., 2019). The most widely expressed nicotinic receptor subtypes are the α7 homomeric and α4β2 heteromeric nAChRs (Hendrickson et al., 2013); while the muscarinic acetylcholine receptors consist of five subtypes (M1-M5), which all are widely expressed throughout the CNS (Tobin, 2024).
For decades, the muscarinic receptor family has been the focus of drug development for treating neuropathology, despite the important role of nicotinic receptors in cognitive and reward functions (Tobin, 2024). In this Research Topic, Braunscheidel et al. explored a novel positive allosteric modulator of α4β2 nicotinic receptors, SR9883, for general reward and nicotine reinforced behaviors. They found significant effects on nicotine reward suggesting that SR9883 may hold promise as a novel treatment of tobacco use disorder (Braunscheidel et al.).
In the research article, “Nicotinic α7 receptors on cholinergic neurons in the striatum mediate cocaine reinforcement, but not food reward,” Fritz et al. uncovered a new role for nicotinic α7 receptors on striatal cholinergic interneurons selective in regulating cocaine seeking and reward, but not natural food reward. These findings suggest that acetylcholine signaling through nicotinic α7 receptors regulate drug selective behaviors, linking nicotinic receptor signaling not only to nicotine reward but also to psychostimulant addiction (Fritz et al.).
Striatal cholinergic interneurons (CINs) are rare but exert widespread influence on striatal output by regulating dopamine and glutamate signaling (Cox and Witten, 2019; Picciotto et al., 2012). These interneurons exhibit tonic firing but can generate phasic responses to salient stimuli, contributing to processes such as learning, plasticity, and motor control (Zhang and Cragg, 2017). In the review article “Extrinsic and intrinsic control of striatal cholinergic interneuron activity,” Ratna and Francis explore the role of CINs, providing an overview of significant findings on their activity. They focus on intrinsic factors and neuromodulators that govern phasic CIN responses involved in learning and plasticity (Ratna and Francis).
Acetylcholine exerts neuromodulatory actions through muscarinic GPCRs on CINs, including the M4-receptors (Zhang et al., 2002; Caulfield, 1993). Berezovskaia et al. found that selective ablation of muscarinic M4 receptors from cholinergic neurons influences locomotor responses to cocaine and scopolamine in a sex-specific manner. The study points out important discrepancies between experimental findings, potentially arising from distinct mouse-lines and gender differences, relevant for both study design considerations and interpretation within the field (Berezovskaia et al.).
In the paper “Distinct cholinergic circuits underlie discrete effects of reward on attention,” Runyon et al. review the role of cholinergic circuits in reward and cognition, further examining the contexts in which attention and reward computations interact, to propose two discrete neural circuits responsible for stimulus-reward associations (Runyon et al.).
Kim et al. explored the role of PPN cholinergic neurons for updating action-outcome expectations in a reward reversal learning paradigm using a Designer Receptors Exclusively Activated by Designer Drugs (DREADDs) strategy in rats (Roth, 2016). Collectively, their findings suggest that cholinergic PPN neurons are essential for flexible update of behavioral strategies for maximizing reward (Kim et al.).
The articles of our Research Topic provide insight in the most recent findings on cholinergic receptor and circuit dynamics in motivation and cognition. We hope that this Research Topic will contribute to the understanding and furthering of scientific progress in the field; and that it will be as interesting to read as it was for us to compose it.
TF: Writing – original draft, Writing – review & editing, Conceptualization, Project administration. AK: Writing – original draft, Writing – review & editing, Conceptualization, Project administration.
The author(s) declare that financial support was received for the research and/or publication of this article. Lundbeck Foundation DANDRITE group leader grant R361-2020-2654 (AK) and Independent Research Fund Denmark Inge Lehmann research project grant 1133-00039B (AK), NIH/NIMH R00MH123673 (TF) and R21MH135207 (TF), and the Brain and Behavior Research Foundation Early Investigator NARSAD award (TF).
The authors declare that the research was conducted in the absence of any commercial or financial relationships that could be construed as a potential conflict of interest.
All claims expressed in this article are solely those of the authors and do not necessarily represent those of their affiliated organizations, or those of the publisher, the editors and the reviewers. Any product that may be evaluated in this article, or claim that may be made by its manufacturer, is not guaranteed or endorsed by the publisher.
Ahmed, N. Y., Knowles, R., and Dehorter, N. (2019). New insights into cholinergic neuron diversity. Front. Mol. Neurosci. 12:204. doi: 10.3389/fnmol.2019.00204
Caulfield, M. P. (1993). Muscarinic receptors–characterization, coupling and function. Pharmcol. Ther. 58, 319–379. doi: 10.1016/0163-7258(93)90027-B
Cox, J., and Witten, I. B. (2019). Striatal circuits for reward learning and decision-making. Nat. Rev. Neurosci. 20, 482–494. doi: 10.1038/s41583-019-0189-2
Dale, H. H. (1914). The action of certain esters and ethers of choline, and their relation to muscarine. J. Pharmacol. Exper. Therapeut. 6, 147–190. doi: 10.1016/S0022-3565(25)08268-0
Dale, H. H. (1934). Nomenclature of fibres in the autonomic nervous system and their effects. J. Physiol. 80, 10–11.
Dautan, D., Huerta-Ocampo, I., Witten, I. B., Deisseroth, K., Bolam, J. P., Gerdjikov, T., et al. (2014). A major external source of cholinergic innervation of the striatum and nucleus accumbens originates in the brainstem. J. Neurosci. 34, 4509–4518. doi: 10.1523/JNEUROSCI.5071-13.2014
Hendrickson, L. M., Guildford, M. J., and Tapper, A. R. (2013). Neuronal nicotinic acetylcholine receptors: common molecular substrates of nicotine and alcohol dependence. Front. Psychiatry 4:29. doi: 10.3389/fpsyt.2013.00029
Loewi, O., and Navratil, E. (1926). Über humorale Übertragbarkeit der Herznervenwirkung. X Mitteilung: Über das Schicksal des Vagusstoffs. Pfluger's Arch. 214, 678–688. doi: 10.1007/BF01741946
Mihailescu, S., and Drucker-Colin, R. (2000). Nicotine, brain nicotinic receptors, and neuropsychiatric disorders. Arch. Med. Res. 31, 131–144. doi: 10.1016/S0188-4409(99)00087-9
Oakman, S. A., Faris, P. L., Kerr, P. E., Cozzari, C., and Hartman, B. K. (1995). Distribution of pontomesencephalic cholinergic neurons projecting to substantia nigra differs significantly from those projecting to ventral tegmental area. J. Neurosci. 15, 5859–5869. doi: 10.1523/JNEUROSCI.15-09-05859.1995
Picciotto, M. R., Higley, M. J., and Mineur, Y. S. (2012). Acetylcholine as a neuromodulator: cholinergic signaling shapes nervous system function and behavior. Neuron 76, 116–129. doi: 10.1016/j.neuron.2012.08.036
Roth, B. L. (2016). DREADDs for neuroscientists. Neuron 89, 683–694. doi: 10.1016/j.neuron.2016.01.040
Ruan, Y., Li, K. Y., Zhen, R., Yan, Y. Q., Wang, Z. X., Chen, Y., et al. (2022). Cholinergic neurons in the pedunculopontine nucleus guide reversal learning by signaling the changing reward contingency. Cell Rep. 38:110437. doi: 10.1016/j.celrep.2022.110437
Tansey, E. M. (2006). Henry Dale and the discovery of acetylcholine. C. R. Biol. 329, 419–425. doi: 10.1016/j.crvi.2006.03.012
Tobin, A. B. (2024). A golden age of muscarinic acetylcholine receptor modulation in neurological diseases. Nat. Rev. Drug Discov. 23, 743–758. doi: 10.1038/s41573-024-01007-1
Williams, M. J., and Adinoff, B. (2008). The role of acetylcholine in cocaine addiction. Neuropsychopharmacology. 33, 1779–1797. doi: 10.1038/sj.npp.1301585
Zhang, J., Zhang, Y., Wang, J., Xia, Y., Zhang, J., and Chen, L. (2024). Recent advances in Alzheimer's disease: mechanisms, clinical trials and new drug development strategies. Signal Transduct. Target Ther. 9:211. doi: 10.1038/s41392-024-01911-3
Zhang, W., Basile, A. S., Gomeza, J., Volpicelli, L. A., Levey, A. I., and Wess, J. (2002). Characterization of central inhibitory muscarinic autoreceptors by the use of muscarinic acetylcholine receptor knock-out mice. J. Neurosci. 22, 1709–1717. doi: 10.1523/JNEUROSCI.22-05-01709.2002
Keywords: acetylcholine, motivation, reward, cognition, addiction, nicotinic receptor (nAChR), muscarinic receptor
Citation: Francis TC and Klawonn AM (2025) Editorial: Mechanisms of cholinergic transmission in motivation and cognition. Front. Mol. Neurosci. 18:1586940. doi: 10.3389/fnmol.2025.1586940
Received: 03 March 2025; Accepted: 12 March 2025;
Published: 26 March 2025.
Edited and reviewed by: Jean-Marc Taymans, Institut National de la Santé et de la Recherche Médicale (INSERM), France
Copyright © 2025 Francis and Klawonn. This is an open-access article distributed under the terms of the Creative Commons Attribution License (CC BY). The use, distribution or reproduction in other forums is permitted, provided the original author(s) and the copyright owner(s) are credited and that the original publication in this journal is cited, in accordance with accepted academic practice. No use, distribution or reproduction is permitted which does not comply with these terms.
*Correspondence: Anna M. Klawonn, a2xhd29ubkBkYW5kcml0ZS5hdS5kaw==
Disclaimer: All claims expressed in this article are solely those of the authors and do not necessarily represent those of their affiliated organizations, or those of the publisher, the editors and the reviewers. Any product that may be evaluated in this article or claim that may be made by its manufacturer is not guaranteed or endorsed by the publisher.
Research integrity at Frontiers
Learn more about the work of our research integrity team to safeguard the quality of each article we publish.