- 1Department of Applied Biological Chemistry, Graduate School of Agricultural and Life Sciences, The University of Tokyo, Tokyo, Japan
- 2Department of Food and Nutrition, Kyoto Women's University, Kyoto, Japan
- 3Toyo Institute of Food Technology, Hyogo, Japan
- 4Department of Liberal Arts, The Open University of Japan, Chiba, Japan
Although salt is an essential substance vital to life, excessive salt intake could cause various health issues. Therefore, new technologies and strategies should be developed to reduce salt intake without compromising taste. However, the underlying physiological mechanisms of salt taste reception is complex and not completely understood. Sodium chloride is a typical salty substance. It is widely believed that only sodium is important for the generation of salty taste. On the other hand, from a psychophysical perspective, the importance of chloride in salty taste has been indicated. Thus, understanding the mechanisms of both sodium- and chloride-tastes generation is necessary to completely comprehended the fundamentals of salt taste reception. However, the mechanism for detecting chloride taste has remained unclear for many years. Recently, we have identified transmembrane channel-like 4 (TMC4) as the first molecule that mediates the reception of chloride taste. TMC4 functions as a voltage-dependent chloride channel and plays an important role in the reception of the chloride taste by detecting chloride ions. In this mini-review, we first introduce the known reception mechanism of salty taste, and then discuss the roles of TMC4 in the salt taste reception. The finding of TMC4 may serve as a basis for developing new technologies and formulating strategies to reduce salt intake without compromising taste.
Introduction
Taste is a sensory reception that is widely shared among many species. It receives stimulus from the external environment and transmits signals to the living organism through taste receptors. This suggests that taste is an initial trigger of physiological communication between the brain and internal organs. Taste is classified into five basic tastes (sweet, salty, sour, bitter, and umami) (Chandrashekar et al., 2006; Yarmolinsky et al., 2009). Taste receptors that detect sweet, sour, bitter, and umami tastes have been identified, and the details of their transmission are being clarified (Chandrashekar et al., 2006; Yarmolinsky et al., 2009). However, many uncertainties remain regarding the salt taste reception.
The typical substance representing salty taste is sodium chloride (NaCl). Excessive intake of sodium, found in NaCl, has been linked to various health risks, including hypertension and kidney dysfunction (Robinson et al., 2019). Therefore, the World Health Organization (WHO) recommends a daily sodium intake of 2.0 g/day (equivalent to 5.0 g/day of NaCl) (World Health Organization, 2012). However, despite understanding the need to control sodium intake, many people find it difficult to meet the WHO guidelines because they cannot suppress their craving for salty foods. In 2019, the mean global sodium intake of adults was estimated at 4.3 g/day (equivalent to 10.8 g/day of NaCl), which is more than double the WHO guidelines (World Health Organization, 2023). Therefore, new technologies and strategies should be developed to reduce sodium intake without compromising taste. However, the underlying physiological mechanism of salt taste reception is complex and not completely understood. If the molecular mechanisms underlying salt taste reception is elucidated, it could contribute to the development of salt taste enhancers that amplify salty taste even with small amounts of sodium, as well as the formulation of appropriate salt reduction policies.
Mammalian peripheral taste reception
Taste reception occurs when taste substances are detected by the taste buds on the tongue. Taste buds, which consist of 50–150 taste cells, are mainly located in the fungiform, foliate, and circumvallate papillae of the tongue epithelium (Delay et al., 1986; Kinnamon et al., 1993; Yarmolinsky et al., 2009; Muchlinski et al., 2011). The fungiform papillae at the anterior part of the tongue are mainly innervated by the chorda tympani nerve, whereas the circumvallate and foliate papillae at the posterior part of the tongue are mainly innervated by the glossopharyngeal nerve, both of which transmit taste stimulus to the brain (Smith and St John, 1999; Ohla et al., 2019). Taste cells are classified into four types, I-IV, based on their morphological features and the molecular-markers they express (Kinnamon and Finger, 2019). Type II cells are involved in the detection of sweet, umami, and bitter tastes, whereas type III cells are involved in the detection of sour taste (Zhang et al., 2003; Huang et al., 2006; Matsumoto et al., 2011). Type I cells are thought to support the structure and metabolism of taste buds and assist in the function of taste cells, similar to the glial cells in neurons (Dvoryanchikov et al., 2009; Cherkashin et al., 2016; Guarascio et al., 2021; Rodriguez et al., 2021). Type I cells constitute approximately 50% of taste cells; however, their direct involvement in taste reception is not well understood (Ma et al., 2007; Yang et al., 2020). Type IV cells, also known as basal cells, are considered precursor cells that differentiate into types I-III cells (Miura et al., 2014). The specific type of taste cells responsible for salt taste reception remains a matter of debate.
Amiloride-sensitive and -insensitive salty taste
Salty taste is divided into amiloride-sensitive and -insensitive salty tastes based on the difference in sensitivity to the diuretic amiloride (Brand et al., 1985; Kretz et al., 1999) (Figure 1).
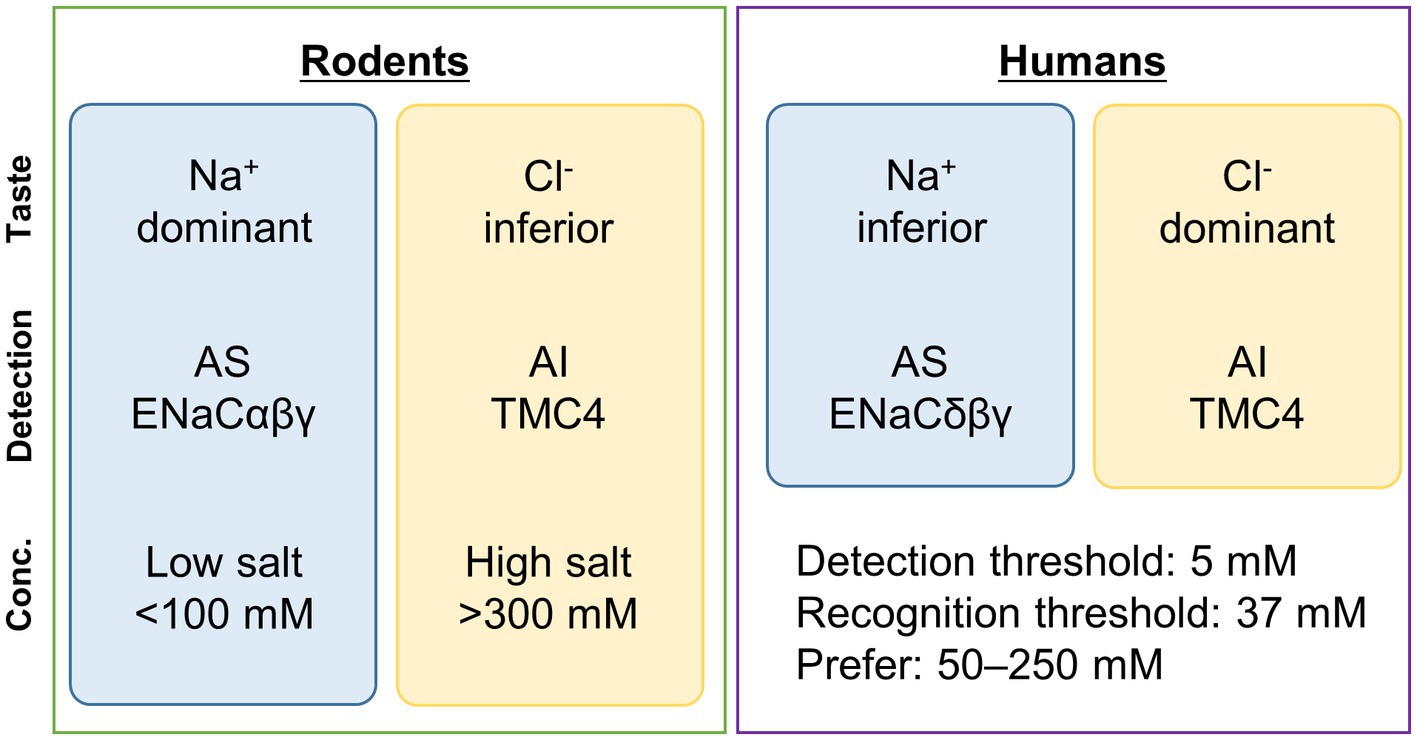
Figure 1. Summary of sensing of a typical salty taste substance, sodium chloride (NaCl). Some differences exist in the reception of salty taste between rodents and humans. In humans, the taste of Cl− is more dominant than that of Na+. In rodents, ENaCαβγ is involved in AS, while in humans, ENaCδβγ is involved. In contrast, TMC4 is involved in AI in both cases. In rodents, ENaCαβγ is mainly involved in the reception of low concentrations of salty taste (<100 mM) (Oka et al., 2013), while TMC4 is believed to be involved in the reception of high concentrations (>300 mM) (Kasahara et al., 2021a). Generally, in humans, the detection and recognition thresholds for NaCl are 5 mM and 37 mM, respectively (Webb et al., 2015), and NaCl concentrations of 50–250 mM are preferred (Chamoun et al., 2019). The concentration ranges in which ENaCδβγ and TMC4 are sensorially involved need to be examined in the future. Conc., concentration; AS, amiloride-sensitive; AI, amiloride-insensitive; TMC4, Transmembrane channel-like 4; ENaC, epithelial sodium channel.
Because amiloride is a specific inhibitor of the epithelial sodium channel (ENaC), the receptor for the amiloride-sensitive salty taste response is thought to be ENaC. ENaC is a non-voltage-gated sodium channel (Stockand et al., 2008). Each ENaC subunit has two transmembrane domains, and ENaC functions as a heterotrimer composed of either ENaCαβγ or ENaCδβγ (Stockand et al., 2008; Noreng et al., 2018). ENaCαβγ predominates in mouse taste cells, whereas ENaCδβγ is more common in human taste cells (Kretz et al., 1999; Stähler et al., 2008) (Figure 1). In ENaCα-deficient mice, both the chorda tympani nerve response and licking response for low sodium concentrations are significantly reduced (Chandrashekar et al., 2010). It has also been reported that these responses are transmitted to the central nervous system through calcium homeostasis modulator (CALHM)1/CALHM3 channels expressed in type II cells (Nomura et al., 2020). However, it has been suggested that in fungiform papillae deeply involved in amiloride-sensitive salty taste reception, each subunit is expressed exclusively and may not function as the heterotrimeric ENaCαβγ (Lossow et al., 2020). It is widely acknowledged that amiloride significantly suppresses salty taste reception in mice through the ENaCαβγ, nevertheless determining the exact stoichiometry of ENaC subunits in taste cells remains a challenge for future research.
Regarding the effect of amiloride on salt taste reception in humans, it has been reported that human salt taste reception is not significantly influenced by amiloride in sensory tests (Desor and Finn, 1989; Halpern and Darlington, 1998). Additionally, cell experiments have shown that the 50% inhibitory concentration (IC50 ≓ 2.7 μM) of ENaCδβγ, which is predominant in humans, is approximately 25 times higher than that of ENaCαβγ (IC50 ≓ 0.11 μM) (Waldmann et al., 1995; Ji et al., 2012). These suggest that ENaCδβγ is less sensitive to amiloride and amiloride-sensitive salty taste plays a minor role in salt taste reception in humans. Therefore, in order to elucidate the mechanism of salt taste reception in humans, it is important to understand the pathways that are insensitive to amiloride.
It has been suggested that type II and III cells are involved in the amiloride-insensitive salt response (Oka et al., 2013). Double mutant mice, generated by crossing transient receptor potential cation channel subfamily M member 5 (TRPM5) knock out (KO) mice and polycystic kidney disease 2-like 1 (PKD2L1)-TeNT mice, showed significant reduction in amiloride-insensitive sodium response compared to wild type (WT) and single mutant mice (Oka et al., 2013). TRPM5 is involved in the transmission of taste signals in type II cells, and PKD2L1 is a molecular marker of type III cells (Zhang et al., 2003; Ishimaru et al., 2006; LopezJimenez et al., 2006). The PKD2L1-TeNT indicated that the neural connections of PKD2L1-expressing taste cells were blocked by diphtheria toxin (Huang et al., 2006). However, the specific receptors involved in these responses remains unknown.
Besides, it has been reported that the taste variant of transient receptor potential cation channel subfamily V member 1 (TRPV1t), a splicing variant of TRPV1 is involved in amiloride-insensitive sodium responses (Lyall et al., 2004). TRPV1 is a non-selective cation channel and mediated pain, hot temperature, and pungent taste (Tominaga et al., 1998; Tominaga, 2005). However, the results of tissue staining and gene expression analysis have shown that TRPV1 is expressed in sensory nerve terminals distributed around the taste buds, but not in taste cells (Ishida et al., 2002; Kido et al., 2003; Sukumaran et al., 2017). Additionally, some reports on salty taste reception using TRPV1 KO mice do not support the involvement of TRPV1 in salty taste reception (Treesukosol et al., 2007; Smith et al., 2012). Hence, the specific receptors responsible for amiloride-insensitive salty taste remains unidentified.
Taste of chloride ion
The majority of studies on salt taste reception have focused on cations, particularly sodium ions. The inhibitory effect of amiloride on salt taste reception in rodents is highly potent, and the target molecule, ENaC, is well known. Therefore, research on amiloride-sensitive salty taste remains a mainstream approach in the study of salt taste reception. On the other hand, research on chloride taste regarding salt taste reception is limited. However, studies using human sensory evaluation have shown that Na-acetate and Na-ascorbate Na-citrate have a weaker salt taste compared to NaCl at the same concentration, implying that chloride ions are involved in salt taste reception (Van der Klaauw and Smith, 1995). Previously, Ye et al. referred to the phenomenon where sodium salts other than NaCl have a weaker salty taste as the “anion paradox” or “anion effect” (Ye et al., 1991). They hypothesized that this occurs because large anion block the depolarization of taste cells triggered by influx of sodium ion (Ye et al., 1991). Until recently, another widely accepted hypothesis was that chloride ion channels were not involved in salt taste reception in taste cells, and chloride ions were thought to flow through tight junctions between taste cells (Elliott and Simon, 1990; Ye et al., 1993).
However, it has been reported recently that taste cells that respond strongly to salty stimulus are classified into two types: anion-insensitive (respond equally to the same concentration of NaCl) and anion-sensitive (respond differently to NaCl and Na-gluconate) (Lewandowski et al., 2016). This suggests that salt taste receptor cells respond differently to various anions. In addition, another study showed that amiloride-insensitive salt taste responses depend on chloride ions rather than sodium ions, and that the receptor may exist in taste cells (Roebber et al., 2019). These reports have updated old hypotheses regarding the anion effect, strongly suggesting that this effect may be mediated by chloride channels involved in salt taste reception in taste cells.
Expression of transmembrane channel-like 4 (TMC4) in the taste bud
Based on this background, we focused on the amiloride-insensitive pathway and attempted to identify new molecules involved in human salt taste reception. First, we screened candidate molecules. The amiloride-insensitive salt pathway is prominently observed in the glossopharyngeal nerve, which connects the taste bud tissue of the circumvallate papillae and foliate papillae at the posterior of the tongue (Formaker and Hill, 1991; Chandrashekar et al., 2010; Oka et al., 2013). Therefore, we conducted a comparative analysis of gene expression in the epithelium of circumvallate papillae with taste buds and the epithelium at the edges of circumvallate papillae without taste buds (Kasahara et al., 2021a), and obtained 1,120 candidate molecules, including transmembrane channel-like (TMC) family. TMC proteins are membrane proteins that are conserved from C. elegans to humans. In mammals, eight TMC family members have been identified (Hahn et al., 2009). In mice, TMC1 and TMC2 are known that are mechanosensitive cation channels involved in hearing (Kawashima et al., 2011). In addition, it has been reported that TMC1 functions as a sodium-sensitive membrane protein in C. elegans (Chatzigeorgiou et al., 2013). Of the eight TMC members, only three molecules Tmc4, Tmc5, and Tmc7 were included in the candidate molecules. The expression level of Tmc4 was the strongest among them.
Subsequently, the localization of Tmc mRNAs expression in taste buds was observed by in situ hybridization (Kasahara et al., 2021a). The Tmc4 signals were specific to the taste bud tissues of circumvallate and foliate papillae, and strong signals were observed. On the other hand, the signal of Tmc4 in taste buds of fungiform papillae was very weak.
Molecular properties of TMC4
The function of TMC4 was analyzed by expressing mouse TMC4 (mTMC4) in cultured cells using a whole-cell patch clamp method (Kasahara et al., 2021a). When step pulses were applied to a cell expressing mTMC4, large outward currents were observed at positive potentials, which increased in a voltage-dependent manner. Furthermore, the effects of intracellular and extracellular ion compositions and the administration of various inhibitors on the current, were examined. The mTMC4-mediated current was not affected by intracellular and extracellular cations or amiloride, but was significantly reduced by the addition of several anion channel inhibitors. In addition, the mTMC4-mediated outward current did not completely disappear when NaCl was replaced with Na-gluconate in the bath solution, suggesting that mTMC4 functions as a voltage-dependent chloride channel with large pore.
Salt taste reception in Tmc4-deficient mice
Next, we observed salt responses in Tmc4-deficient mice using a taste nerve recording and a licking behavior test (Kasahara et al., 2021a; Narukawa et al., 2024). In the glossopharyngeal nerve, Tmc4-deficient mice exhibited a significant reduction in responses to high concentrations of NaCl and KCl compared with wild type (WT) mice. In contrast, in the chorda tympani nerve, no significant difference was observed in responses to NaCl and KCl at any concentration (Kasahara et al., 2021a). In the licking test, Tmc4-deficient mice exhibited a significant decrease in sensitivities to multiple chloride salts such as NaCl, KCl, and NH4Cl (Narukawa et al., 2024). Furthermore, there was no significant difference in the responses to sweet, umami, bitter, and sour taste stimulus between WT and Tmc4-deficient mice. These suggests that TMC4 plays as a key molecule in salt taste reception.
Insight into the role of chloride ion in salt taste reception
Generally, chloride ion influx regulates membrane potential, promoting repolarization (Huang et al., 2012). The molecular simulation using a model taste cell showed that TMC4 presence shortens action potential duration in response to salt, increasing firing frequency under continuous stimulation (Kasahara et al., 2021a). We hypothesize that TMC4 accelerates the salt taste signaling cycle by allowing chloride ions to enter taste cells, generating new salt taste signals.
Evolutionary viewpoint of TMC genes
Among the TMC family, TMC1 and TMC2 proteins are conserved across many vertebrates, including the green sea turtle, budgerigar, zebrafish, and pufferfish (Keresztes et al., 2003; Jia et al., 2020). In contrast, there are few reports on TMC4 expression in non-mammals. Although pufferfish is the only non-mammalian organism where TMC4 expression has been confirmed, the presence of TMC4, which is involved in salt taste reception, in both terrestrial organisms such as humans and mice, where salt is a limited resource and marine organisms like pufferfish, where salt is abundant, may provide insights into the mechanisms of terrestrial adaptation from an evolutionary medicine perspective (Keresztes et al., 2003).
Application of TMC4 for reducing salt intake
Our findings strongly suggest that TMC4 plays a role in amiloride-insensitive salty taste and that chloride ion is the primary component of amiloride-insensitive salty taste (Figure 1).
Some sensory tests have reported that human salt taste reception is influenced by temperature, pH, and non-steroidal anti-inflammatory drugs (Pangborn et al., 1970; McBurney et al., 1973; Keast and Breslin, 2003; Schiffman et al., 2000). We confirmed that human TMC4 (hTMC4) responds to these stimuli in a manner similar to human sensory evaluation (Kasahara et al., 2021b,c). Furthermore, utilizing the structure of L-arginine hydrochloride, known as a salt taste enhancer, we discovered a novel salt taste enhancer, 3-guanidinylpropanol hydrochloride (3GPrOH), and demonstrated that 3GPrOH significantly enhances hTMC4-mediated currents, thereby enhancing salty taste (Lee, 1992; Sakurai et al., 2015; Kasahara et al., 2022). In addition, some researchers report that salt taste enhancing peptides enhance the salty taste via TMC4, based on the structure of TMC4 created in silico method using artificial intelligence programs (Shen et al., 2022; Bu et al., 2024; Zhang et al., 2024; Wang et al., 2024). These results suggest that TMC4 is involved in salt taste reception in humans and can be applicable to the development of salt taste enhancers.
As indicated in the introduction, in recent years, it has been reported that excessive sodium intake is linked to the various health risk. In 2012, the WHO strongly recommended a daily NaCl intake target of 5 g for adults (World Health Organization, 2012). According to this, many countries have been exploring various approaches to reduce sodium intake. As a result, efforts to reduce salt intake have included the establishment of new nutritional guidelines, consumer education on salt, labeling the sodium content on food packaging, requests for salt reduction from food companies, and the introduction of salt taxes (Santos et al., 2021; World Health Organization, 2023). However, even with these mandatory policies, there is still a considerable gap in achieving WHO’s goals (Alonso et al., 2021; Looi, 2023; World Health Organization, 2023).
Some epidemiological studies suggest a strong positive correlation between salt intake and blood pressure (Robinson et al., 2019). Hypertension is a contributing factor to heart disease and chronic kidney disease (CKD). It has been reported that CKD patients suffer from oral diseases and have dulled taste sensitivity, leading them to prefer high concentrations of salt (Okuno-Ozeki et al., 2024). In addition to the taste papillae, TMC4 is highly expressed in the duodenum, colon, and small intestine, but is barely expressed in the brain (Fagerberg et al., 2014). Analysis of TMC4 expression and mutations in the oral cavity of subjects with an addictive preference for salt and CKD patients may provide valuable insights for developing treatments for these conditions.
Exploring substances that effectively reduce salt intake using TMC4, and elucidating the salt taste reception mechanism with TMC4, will aid in achieving this challenging goal. TMC4 is the first molecule reported to be involved in chloride taste reception. Research involving TMC4 is expected to contribute to improving our quality of life.
Author contributions
YK: Writing – original draft, Writing – review & editing. MN: Writing – original draft, Writing – review & editing. YS: Writing – review & editing. KA: Writing – review & editing. TA: Writing – original draft, Writing – review & editing.
Funding
The author(s) declare that financial support was received for the research, authorship, and/or publication of this article. This work was supported in part by a Grant-in-Aid for Scientific Research (B) 23K22216 to TA, by Challenging Research Exploratory 23K17575 to MN and TA from the Japan Society for the Promotion of Science, and by the Salt Science Research Foundation No. 24D4 to MN.
Conflict of interest
The authors declare that the research was conducted in the absence of any commercial or financial relationships that could be construed as a potential conflict of interest.
Publisher’s note
All claims expressed in this article are solely those of the authors and do not necessarily represent those of their affiliated organizations, or those of the publisher, the editors and the reviewers. Any product that may be evaluated in this article, or claim that may be made by its manufacturer, is not guaranteed or endorsed by the publisher.
References
Alonso, S., Tan, M., Wang, C., Kent, S., Cobiac, L., MacGregor, G. A., et al. (2021). Impact of the 2003 to 2018 population salt intake reduction program in England. Hypertension 77, 1086–1094. doi: 10.1161/HYPERTENSIONAHA.120.16649
Brand, J. G., Teeter, J. H., and Silver, W. L. (1985). Inhibition by amiloride of chorda tympani responses evoked by monovalent salts. Brain Res. 334, 207–214. doi: 10.1016/0006-8993(85)90212-4
Bu, Y., Sun, C., Guo, J., Zhu, W., Li, J., Li, X., et al. (2024). Identification novel salt-enhancing peptides from largemouth bass and exploration their action mechanism with transmembrane channel-like 4 (TMC4) by molecular simulation. Food Chem. 435:137614. doi: 10.1016/j.foodchem.2023.137614
Chamoun, E., Liu, A. A. S., Duizer, L. M., Darlington, G., Duncan, A. M., Haines, J., et al. (2019). Taste sensitivity and taste preference measures are correlated in healthy young adults. Chem. Senses 44, 129–134. doi: 10.1093/chemse/bjy082
Chandrashekar, J., Hoon, M. A., Ryba, N. J., and Zuker, C. S. (2006). The receptors and cells for mammalian taste. Nature 444, 288–294. doi: 10.1038/nature05401
Chandrashekar, J., Kuhn, C., Oka, Y., Yarmolinsky, D. A., Hummler, E., Ryba, N. J. P., et al. (2010). The cells and peripheral representation of sodium taste in mice. Nature 464, 297–301. doi: 10.1038/nature08783
Chatzigeorgiou, M., Bang, S., Hwang, S. W., and Schafer, W. R. (2013). Tmc-1 encodes a sodium-sensitive channel required for salt chemosensation in C. elegans. Nature 494, 95–99. doi: 10.1038/nature11845
Cherkashin, A. P., Kolesnikova, A. S., Tarasov, M. V., Romanov, R. A., Rogachevskaja, O. A., Bystrova, M. F., et al. (2016). Expression of calcium-activated chloride channels Ano1 and Ano2 in mouse taste cells. Pflugers Arch. 468, 305–319. doi: 10.1007/s00424-015-1751-z
Delay, R. J., Roper, S. D., and Kinnamon, J. C. (1986). Ultrastructure of mouse vallate taste buds: II. Cell types and cell lineage. J. Comp. Neurol. 253, 242–252. doi: 10.1002/cne.902530210
Desor, J. A., and Finn, J. (1989). Effects of amiloride on salt taste in humans. Chem. Senses 14, 793–803. doi: 10.1093/chemse/14.6.793
Dvoryanchikov, G., Sinclair, M. S., Perea-Martinez, I., Wang, T., and Chaudhari, N. (2009). Inward rectifier channel, ROMK, is localized to the apical tips of glial-like cells in mouse taste buds. J. Comp. Neurol. 517, 1–14. doi: 10.1002/cne.22152
Elliott, E. J., and Simon, S. A. (1990). The anion in salt taste: a possible role for paracellular pathways. Brain Res. 535, 9–17. doi: 10.1016/0006-8993(90)91817-z
Fagerberg, L., Hallström, B. M., Oksvold, P., Kampf, C., Djureinovic, D., Odeberg, J., et al. (2014). Analysis of the human tissue-specific expression by genome-wide integration of transcriptomics and antibody-based proteomics. Mol. Cell. Proteomics 13, 397–406. doi: 10.1074/mcp.M113.035600
Formaker, B. K., and Hill, D. L. (1991). Lack of amiloride sensitivity in SHR and WKY glossopharyngeal taste responses to NaCl. Physiol. Behav. 50, 765–769. doi: 10.1016/0031-9384(91)90015-g
Guarascio, D. M., Gonzalez-Velandia, K. Y., Hernandez-Clavijo, A., Menini, A., and Pifferi, S. (2021). Functional expression of TMEM16A in taste bud cells. J. Physiol. 599, 3697–3714. doi: 10.1113/JP281645
Hahn, Y., Kim, D. S., Pastan, I. H., and Lee, B. (2009). Anoctamin and transmembrane channel-like proteins are evolutionarily related. Int. J. Mol. Med. 24, 51–55. doi: 10.3892/ijmm_00000205
Halpern, B. P., and Darlington, R. B. (1998). Effects of amiloride on gustatory quality descriptions and temporal patterns produced by NaCl. Chem. Senses 23, 501–511. doi: 10.1093/chemse/23.5.501
Huang, A. L., Chen, X., Hoon, M. A., Chandrashekar, J., Guo, W., Tränkner, D., et al. (2006). The cells and logic for mammalian sour taste detection. Nature 442, 934–938. doi: 10.1038/nature05084
Huang, W. C., Xiao, S., Huang, F., Harfe, B. D., Jan, Y. N., and Jan, L. Y. (2012). Calcium-activated chloride channels (CaCCs) regulate action potential and synaptic response in hippocampal neurons. Neuron 74, 179–192. doi: 10.1016/j.neuron.2012.01.033
Ishida, Y., Ugawa, S., Ueda, T., Murakami, S., and Shimada, S. (2002). Vanilloid receptor subtype-1 (VR1) is specifically localized to taste papillae. Mol. Brain Res. 107, 17–22. doi: 10.1016/S0169-328X(02)00441-2
Ishimaru, Y., Inada, H., Kubota, M., Zhuang, H., Tominaga, M., and Matsunami, H. (2006). Transient receptor potential family members PKD1L3 and PKD2L1 form a candidate sour taste receptor. Proc. Natl. Acad. Sci. USA 103, 12569–12574. doi: 10.1073/pnas.0602702103
Ji, H.-L., Zhao, R.-Z., Chen, Z.-X., Shetty, S., Idell, S., and Matalon, S. (2012). δ ENaC: A novel divergent amiloride-inhibitable sodium channel. Am. J. Physiol. Lung Cell. Mol. Physiol. 303, L1013–L1026. doi: 10.1152/ajplung.00206.2012
Jia, Y., Zhao, Y., Kusakizako, T., Wang, Y., Pan, C., Zhang, Y., et al. (2020). TMC1 and TMC2 proteins are pore-forming subunits of mechanosensitive ion channels. Neuron 105, 310–321.e3. doi: 10.1016/j.neuron.2019.10.017
Kasahara, Y., Narukawa, M., Ishimaru, Y., Kanda, S., Umatani, C., Takayama, Y., et al. (2021a). TMC4 is a novel chloride channel involved in high-concentration salt taste sensation. J. Physiol. Sci. 71:23. doi: 10.1186/s12576-021-00807-z
Kasahara, Y., Narukawa, M., Kanda, S., Tominaga, M., Abe, K., Misaka, T., et al. (2021b). Transmembrane channel-like 4 is involved in pH and temperature-dependent modulation of salty taste. Biosci. Biotechnol. Biochem. 85, 2295–2299. doi: 10.1093/bbb/zbab152
Kasahara, Y., Narukawa, M., Nakagita, T., Abe, K., Misaka, T., and Asakura, T. (2021c). Ibuprofen inhibits oral NaCl response through transmembrane channel-like 4. Biochem. Biophys. Res. Commun. 573, 76–79. doi: 10.1016/j.bbrc.2021.07.082
Kasahara, Y., Yamashita, H., Narukawa, M., Abe, K., and Asakura, T. (2022). 3-Guanidinyl propanol enhances salt taste via TMC4-mediated current. ACS Food Sci. Technol. 2, 1213–1216. doi: 10.1021/acsfoodscitech.2c00149
Kawashima, Y., Géléoc, G. S. G., Kurima, K., Labay, V., Lelli, A., Asai, Y., et al. (2011). Mechanotransduction in mouse inner ear hair cells requires transmembrane channel-like genes. J. Clin. Invest. 121, 4796–4809. doi: 10.1172/JCI60405
Keast, R. S. J., and Breslin, P. A. S. (2003). An overview of binary taste–taste interactions. Food Qual. Prefer. 14, 111–124. doi: 10.1016/S0950-3293(02)00110-6
Keresztes, G., Mutai, H., and Heller, S. (2003). TMC and EVER genes belong to a larger novel family, the TMC gene family encoding transmembrane proteins. BMC Genomics 4:24. doi: 10.1186/1471-2164-4-24
Kido, M. A., Muroya, H., Yamaza, T., Terada, Y., and Tanaka, T. (2003). Vanilloid receptor expression in the rat tongue and palate. J. Dent. Res. 82, 393–397. doi: 10.1177/154405910308200513
Kinnamon, S. C., and Finger, T. E. (2019). Recent advances in taste transduction and signaling. F1000Res. 8:2117. doi: 10.12688/f1000research.21099.1
Kinnamon, J. C., Henzler, D. M., and Royer, S. M. (1993). HVEM ultrastructural analysis of mouse fungiform taste buds, cell types, and associated synapses. Microsc. Res. Tech. 26, 142–156. doi: 10.1002/jemt.1070260207
Kretz, O., Barbry, P., Bock, R., and Lindemann, B. (1999). Differential expression of RNA and protein of the three pore-forming subunits of the amiloride-sensitive epithelial sodium channel in taste buds of the rat. F1000Res. 47, 51–64. doi: 10.1177/002215549904700106
Lewandowski, B. C., Sukumaran, S. K., Margolskee, R. F., and Bachmanov, A. A. (2016). Amiloride-insensitive salt taste is mediated by two populations of type III taste cells with distinct transduction mechanisms. J. Neurosci. Off. J. Soc. Neurosci. 36, 1942–1953. doi: 10.1523/JNEUROSCI.2947-15.2016
Looi, M.-K. (2023). Salt intake: 97% of the world is failing to cut consumption, warns WHO. BMJ 380:p570. doi: 10.1136/bmj.p570
LopezJimenez, N. D., Cavenagh, M. M., Sainz, E., Cruz-Ithier, M. A., Battey, J. F., and Sullivan, S. L. (2006). Two members of the TRPP family of ion channels, Pkd1l3 and Pkd2l1, are co-expressed in a subset of taste receptor cells. J. Neurochem. 98, 68–77. doi: 10.1111/j.1471-4159.2006.03842.x
Lossow, K., Hermans-Borgmeyer, I., Meyerhof, W., and Behrens, M. (2020). Segregated expression of ENaC subunits in taste cells. Chem. Senses 45, 235–248. doi: 10.1093/chemse/bjaa004
Lyall, V., Heck, G. L., Vinnikova, A. K., Ghosh, S., Phan, T.-H. T., Alam, R. I., et al. (2004). The mammalian amiloride-insensitive non-specific salt taste receptor is a vanilloid receptor-1 variant. J. Physiol. 558, 147–159. doi: 10.1113/jphysiol.2004.065656
Ma, H., Yang, R., Thomas, S. M., and Kinnamon, J. C. (2007). Qualitative and quantitative differences between taste buds of the rat and mouse. BMC Neurosci. 8:5. doi: 10.1186/1471-2202-8-5
Matsumoto, I., Ohmoto, M., Narukawa, M., Yoshihara, Y., and Abe, K. (2011). Skn-1a/Pou2f3 specifies taste receptor cell lineage. Nat. Neurosci. 14, 685–687. doi: 10.1038/nn.2820
McBurney, D. H., Collings, V. B., and Glanz, L. M. (1973). Temperature dependence of human taste responses. Physiol. Behav. 11, 89–94. doi: 10.1016/0031-9384(73)90127-3
Miura, H., Scott, J. K., Harada, S., and Barlow, L. A. (2014). Sonic hedgehog-expressing basal cells are general post-mitotic precursors of functional taste receptor cells. Dev. Dyn. 243, 1286–1297. doi: 10.1002/dvdy.24121
Muchlinski, M. N., Docherty, B. A., Alport, L. J., Burrows, A. M., Smith, T. D., and Paesani, S. M. (2011). Behavioral and ecological consequences of sex-based differences in gustatory anatomy in Cebus apella. Anat. Rec. 294, 2179–2192. doi: 10.1002/ar.21507
Narukawa, M., Masago, A., Murata, M., Saito, Y., Kasahara, Y., Abe, K., et al. (2024). Mouse TMC4 is involved in the detection of chloride taste of salts. Biosci. Biotechnol. Biochem. 88, 203–205. doi: 10.1093/bbb/zbad155
Nomura, K., Nakanishi, M., Ishidate, F., Iwata, K., and Taruno, A. (2020). All-electrical Ca2+-independent signal transduction mediates attractive sodium taste in taste buds. Neuron 106, 816–829.e6. doi: 10.1016/j.neuron.2020.03.006
Noreng, S., Bharadwaj, A., Posert, R., Yoshioka, C., and Baconguis, I. (2018). Structure of the human epithelial sodium channel by cryo-electron microscopy. eLife 7:e39340. doi: 10.7554/eLife.39340
Ohla, K., Yoshida, R., Roper, S. D., Di Lorenzo, P. M., Victor, J. D., Boughter, J. D., et al. (2019). Recognizing taste: coding patterns along the neural axis in mammals. Chem. Senses 44, 237–247. doi: 10.1093/chemse/bjz013
Oka, Y., Butnaru, M., von Buchholtz, L., Ryba, N. J. P., and Zuker, C. S. (2013). High salt recruits aversive taste pathways. Nature 494, 472–475. doi: 10.1038/nature11905
Okuno-Ozeki, N., Kohama, Y., Taguchi, H., Kawate, Y., Umehara, M., Minamida, A., et al. (2024). Aversion to a high salt taste is disturbed in patients with CKD. Kidney Int. Rep. 9, 1254–1264. doi: 10.1016/j.ekir.2024.02.1393
Pangborn, R. M., Chrisp, R. B., and Bertolero, L. L. (1970). Gustatory, salivary, and oral thermal responses to solutions of sodium chloride at four temperatures. Percept. Psychophys. 8, 69–75. doi: 10.3758/BF03210177
Robinson, A. T., Edwards, D. G., and Farquhar, W. B. (2019). The influence of dietary salt beyond blood pressure. Curr. Hypertens. Rep. 21:42. doi: 10.1007/s11906-019-0948-5
Rodriguez, Y. A., Roebber, J. K., Dvoryanchikov, G., Makhoul, V., Roper, S. D., and Chaudhari, N. (2021). “Tripartite synapses” in taste buds: a role for type I glial-like taste cells. J. Neurosci. 41, 9860–9871. doi: 10.1523/JNEUROSCI.1444-21.2021
Roebber, J. K., Roper, S. D., and Chaudhari, N. (2019). The role of the anion in salt (NaCl) detection by mouse taste buds. J. Neurosci. Off. J. Soc. Neurosci. 39, 6224–6232. doi: 10.1523/JNEUROSCI.2367-18.2019
Sakurai, T., Kasahara, Y., Tanaka, M., Abe, K., Asakura, T., and Yamashita, H. (2015). Salty taste-enhancing agent and manufacturing method therefor, and salty taste-enhancing method (United States patent US20150250217A1).
Santos, J. A., Tekle, D., Rosewarne, E., Flexner, N., Cobb, L., Al-Jawaldeh, A., et al. (2021). A systematic review of salt reduction initiatives around the world: a midterm evaluation of progress towards the 2025 global non-communicable diseases salt reduction target. Adv. Nut. (Bethesda) 12, 1768–1780. doi: 10.1093/advances/nmab008
Schiffman, S. S., Zervakis, J., Westall, H. L., Graham, B. G., Metz, A., Bennett, J. L., et al. (2000). Effect of antimicrobial and anti-inflammatory medications on the sense of taste. Physiol. Behav. 69, 413–424. doi: 10.1016/s0031-9384(99)00262-0
Shen, D.-Y., Pan, F., Yang, Z.-C., Song, H.-L., Zou, T., Xiong, J., et al. (2022). Identification of novel saltiness-enhancing peptides from yeast extract and their mechanism of action for transmembrane channel-like 4 (TMC4) protein through experimental and integrated computational modeling. Food Chem. 388:132993. doi: 10.1016/j.foodchem.2022.132993
Smith, D. V., and St John, S. J. (1999). Neural coding of gustatory information. Curr. Opin. Neurobiol. 9, 427–435. doi: 10.1016/S0959-4388(99)80064-6
Smith, K. R., Treesukosol, Y., Paedae, A. B., Contreras, R. J., and Spector, A. C. (2012). Contribution of the TRPV1 channel to salt taste quality in mice as assessed by conditioned taste aversion generalization and chorda tympani nerve responses. Am. J. Physiol. Regul. Integr. Comp. Physiol. 303, R1195–R1205. doi: 10.1152/ajpregu.00154.2012
Stähler, F., Riedel, K., Demgensky, S., Neumann, K., Dunkel, A., Täubert, A., et al. (2008). A role of the epithelial sodium channel in human salt taste transduction? Chemosens. Percept. 1, 78–90. doi: 10.1007/s12078-008-9006-4
Stockand, J. D., Staruschenko, A., Pochynyuk, O., Booth, R. E., and Silverthorn, D. U. (2008). Insight toward epithelial Na+ channel mechanism revealed by the acid-sensing ion channel 1 structure. IUBMB Life 60, 620–628. doi: 10.1002/iub.89
Sukumaran, S. K., Lewandowski, B. C., Qin, Y., Kotha, R., Bachmanov, A. A., and Margolskee, R. F. (2017). Whole transcriptome profiling of taste bud cells. Sci. Rep. 7:7595. doi: 10.1038/s41598-017-07746-z
Tominaga, M. (2005). Molecular mechanisms of trigeminal nociception and sensation of pungency. Chem. Senses 30, i191–i192. doi: 10.1093/chemse/bjh179
Tominaga, M., Caterina, M. J., Malmberg, A. B., Rosen, T. A., Gilbert, H., Skinner, K., et al. (1998). The cloned capsaicin receptor integrates multiple pain-producing stimuli. Neuron 21, 531–543. doi: 10.1016/s0896-6273(00)80564-4
Treesukosol, Y., Lyall, V., Heck, G. L., DeSimone, J. A., and Spector, A. C. (2007). A psychophysical and electrophysiological analysis of salt taste in Trpv1 null mice. Am. J. Physiol. Regul. Integr. Comp. Physiol. 292, R1799–R1809. doi: 10.1152/ajpregu.00587.2006
Van der Klaauw, N. J., and Smith, D. V. (1995). Taste quality profiles for fifteen organic and inorganic salts. Physiol. Behav. 58, 295–306. doi: 10.1016/0031-9384(95)00056-o
Waldmann, R., Champigny, G., Bassilana, F., Voilley, N., and Lazdunski, M. (1995). Molecular cloning and functional expression of a novel amiloride-sensitive Na+ channel. J. Biol. Chem. 270, 27411–27414. doi: 10.1074/jbc.270.46.27411
Wang, H., Chen, D., Lu, W., Dang, Y., Liu, Z., Chen, G., et al. (2024). Novel salty peptides derived from bovine bone: identification, taste characteristic, and salt-enhancing mechanism. Food Chem. 447:139035. doi: 10.1016/j.foodchem.2024.139035
Webb, J., Bolhuis, D. P., Cicerale, S., Hayes, J. E., and Keast, R. (2015). The relationships between common measurements of taste function. Chemosens. Percept. 8, 11–18. doi: 10.1007/s12078-015-9183-x
World Health Organization (2012). Guideline: Sodium intake for adults and children. Geneva: World Health Organization.
World Health Organization (2023). WHO global report on sodium intake reduction. Geneva: World Health Organization.
Yang, R., Dzowo, Y. K., Wilson, C. E., Russell, R. L., Kidd, G. J., Salcedo, E., et al. (2020). Three-dimensional reconstructions of mouse circumvallate taste buds using serial blockface scanning electron microscopy: I. Cell types and the apical region of the taste bud. J. Comp. Neurol. 528, 756–771. doi: 10.1002/cne.24779
Yarmolinsky, D. A., Zuker, C. S., and Ryba, N. J. (2009). Common sense about taste: from mammals to insects. Cell 139, 234–244. doi: 10.1016/j.cell.2009.10.001
Ye, Q., Heck, G. L., and DeSimone, J. A. (1991). The anion paradox in sodium taste reception: resolution by voltage-clamp studies. Science (New York, N.Y.) 254, 724–726. doi: 10.1126/science.1948054
Ye, Q., Heck, G. L., and DeSimone, J. A. (1993). Voltage dependence of the rat chorda tympani response to Na+ salts: implications for the functional organization of taste receptor cells. J. Neurophysiol. 70, 167–178. doi: 10.1152/jn.1993.70.1.167
Zhang, J., He, W., Liang, L., Sun, B., and Zhang, Y. (2024). Study on the saltiness-enhancing mechanism of chicken-derived umami peptides by sensory evaluation and molecular docking to transmembrane channel-like protein 4 (TMC4). Food Res. Int. 182:114139. doi: 10.1016/j.foodres.2024.114139
Keywords: salty taste, chloride channel, TMC4, chloride taste, amiloride-insensitive
Citation: Kasahara Y, Narukawa M, Saito Y, Abe K and Asakura T (2024) The complexities of salt taste reception: insights into the role of TMC4 in chloride taste detection. Front. Mol. Neurosci. 17:1468438. doi: 10.3389/fnmol.2024.1468438
Edited by:
Hiroaki Masuzaki, University of the Ryukyus, JapanReviewed by:
Tsugumi Uema, University of the Ryukyus, JapanCopyright © 2024 Kasahara, Narukawa, Saito, Abe and Asakura. This is an open-access article distributed under the terms of the Creative Commons Attribution License (CC BY). The use, distribution or reproduction in other forums is permitted, provided the original author(s) and the copyright owner(s) are credited and that the original publication in this journal is cited, in accordance with accepted academic practice. No use, distribution or reproduction is permitted which does not comply with these terms.
*Correspondence: Masataka Narukawa, bmFydWthd2FAa3lvdG8td3UuYWMuanA=; Tomiko Asakura, YXNha3VyYUBnLmVjYy51LXRva3lvLmFjLmpw