- 1Croatian Institute for Brain Research, School of Medicine, University of Zagreb, Zagreb, Croatia
- 2Department of Chemistry and Biochemistry, School of Medicine, University of Zagreb, Zagreb, Croatia
- 3Department of Medical Chemistry, Biochemistry and Clinical Chemistry, Faculty of Medicine, Josip Juraj Strossmayer University of Osijek, Osijek, Croatia
- 4Department of Neuroimaging, BRAIN Centre, Institute of Psychiatry Psychology and Neuroscience, King’s College London, London, United Kingdom
- 5Department of Medical Biology and Genetics, Faculty of Medicine, Josip Juraj Strossmayer University of Osijek, Osijek, Croatia
- 6Department of Pharmacology, Faculty of Medicine, Josip Juraj Strossmayer University of Osijek, Osijek, Croatia
- 7Clinical Institute of Laboratory Diagnostics, Osijek University Hospital, Osijek, Croatia
- 8Department of Biology, Josip Juraj Strossmayer University of Osijek, Osijek, Croatia
This paper explores the physiological consequences of decreased expression of GD3 synthase (GD3S), a biosynthetic enzyme that catalyzes the synthesis of b-series gangliosides. GD3S is a key factor in tumorigenesis, with overexpression enhancing tumor growth, proliferation, and metastasis in various cancers. Hence, inhibiting GD3S activity has potential therapeutic effects due to its role in malignancy-associated pathways across different cancer types. GD3S has also been investigated as a promising therapeutic target in treatment of various neurodegenerative disorders. Drugs targeting GD3 and GD3S have been extensively explored and underwent clinical trials, however decreased GD3S expression in mouse models, human subjects, and in vitro studies has demonstrated serious adverse effects. We highlight these negative consequences and show original mass spectrometry imaging (MSI) data indicating that inactivated GD3S can generally negatively affect energy metabolism, regulatory pathways, and mitigation of oxidative stress. The disturbance in several physiological systems induced by GD3S inhibition underscores the vital role of this enzyme in maintaining cellular homeostasis and should be taken into account when GD3S is considered as a therapeutic target.
1 Introduction
Gangliosides, complex glycosphingolipids, challenging to investigate with common molecular biology methods, are highly prevalent in the mammalian nervous system. Specifically, the human brain contains 10–30 times more gangliosides than any other organ (Svennerholm, 1994). Amongst those, GM1, GD1a, GD1b and GT1b represent 97% of all brain gangliosides (Yu et al., 2011). Gangliosides are synthesized by a series of specific glycosyl-and sialyltransferases in a metabolic pathway diverged into four branches (Yu et al., 2011). Hence, we can distinguish between asialo, a-, b-, and c-series of gangliosides according to the number of sialic acids (0, 1, 2, and 3) linked to the inner galactose residue (Schnaar, 2016, 2019). In this paper we are focusing on one of those sialyltransferases: GD3 synthase (GD3S) (alpha-N-acetylneuraminate alpha-2,8-sialyltransferase, EC 2.4. 99.8). GD3S regulates the biosynthesis of GD3 and GD2 from GM3, which are in turn starting points for the synthesis of more complex gangliosides of b-and c-series (Kasprowicz et al., 2022). It is encoded by the (ST8SIA1, St8sia1) gene (Kasprowicz et al., 2022) which is not solely expressed in the brain, but the RNA expression of ST8SIA1 as well as expression of the protein product, GD3S, has been detected in a variety of other tissues, most prominently endocrine tissues, bone marrow, and lymphoid tissues, as illustrated in Supplementary Figure S1. Even though some tissues show a relatively modest RNA expression of the ST8SIA1 gene, the protein expression is quite strong, e.g., liver and gallbladder, or the skin (Supplementary Figure S1). In this paper we aim to provide insight into the physiological consequences of decreased GD3S expression, accompanied by our own supportive observations and proposed future perspectives.
2 The good
Is achieving a favorable outcome realistic when considering the inhibition of any biosynthetic enzyme involved in ganglioside synthesis? Targeting this enzyme for inhibition has been a compelling research avenue, as elevated GD3S expression is associated with various pathological conditions, from cancer to neurodegenerative diseases.
GD3S has been recognized as one of the key factors in tumorigenesis (Tong et al., 2021). Overexpression of GD3S enhances tumor growth, proliferation, and metastasis in various cancers (Cazet et al., 2010; Schengrund, 2023) including thyroid, lung, colorectal, liver, pancreatic, renal, prostate, breast cancer, and most prominently, glioma and melanoma (Cazet et al., 2010; Yeh et al., 2016; Ma et al., 2017; Lee et al., 2018; Kasprowicz et al., 2022; Seo et al., 2024), illustrated in Supplementary Figure S2. Hence, inhibiting GD3S catalytic activity has raised interest as a potential therapeutic target considering its role in malignancy-associated pathways across different cancer types. Indeed, numerous studies have shown that decrease or abolishment of GD3S expression results in reduced malignancy, inhibition of proliferation, and decreased metastatic potential (Kasprowicz et al., 2022). Specifically, GD3S is highly expressed in GD2-positive breast cancer stem cells, and decreasing its expression results in inhibition of proliferation and self-renewal. This effect has also been demonstrated in vivo (Houghton et al., 1985). Promoting proliferation is induced by activating c-Met and the downstream targets MEK/ERK and the PI3K/AKT pathway (Ruan et al., 1999). GD3S was also found to promote metastasis of breast cancer by regulating epithelial-mesenchymal transition (Ruan et al., 1999). Furthermore, the absence of GD3S results in the downregulation of Akt, ERKs, and SFK phosphorylation in GD3S knock-out (GD3SKO) mouse glioma cells (Ohkawa et al., 2021).
Even though GD3S is responsible for the synthesis of b-and c-series gangliosides, ganglioside GD3 (Ohkawa et al., 2021) accounts for over 50% ganglioside content in glioblastoma and is most abundant in hyper-vascularized areas. It is mainly found in cell surface clusters and the cytoplasm of tumor cells (Hedberg et al., 2001). GD3-positive cells were detected in the peritumoral tissue up to 3.5 cm from the tumor edge, suggesting their implication in glioblastoma progression and invasion (Lama et al., 2016). In contrast, ganglioside GD3 is almost absent in the healthy adult human brain, making it a potential therapeutic target (Mennel et al., 2000; Iwasawa et al., 2018). Studies on GD3SKO mice found the animals develop tumors slower and the overall size of tumors was smaller than in wild-type (WT) mice, strengthening the notion that GD3S enhances the malignant properties of gliomas (Zhang et al., 2021). The introduction of GD3S cDNA into a neuroblastoma cell line (SH-SY5Y), which substantially expressed GM2 and GD1a but not GD3 and GD2, increased GD3 and GD2 expression, dispersed cell development and slowed down growth (Ruan et al., 1999).
GD3S has also been investigated as a potential novel therapeutic target in the context of various neurodegenerative disorders (Akkhawattanangkul et al., 2017). Huntington’s disease, Parkinson’s disease (PD), and Alzheimer’s disease (AD) are all characterized by altered ganglioside metabolism (Sipione et al., 2020). GD3S has been recognized as a novel AD treatment objective for cognitive decline, amyloid plaque development, and neurodegeneration (Bernardo et al., 2009). In a study on primary neurons and astrocytes lacking GD3S, Aβ-induced cell death and aggregation were prevented (Bernardo et al., 2009). Furthermore, APP/PSEN1/GD3S−/− triple mutant mice experience improvements in behavioral deficits compared to APP/PSEN1 mice (Bernardo et al., 2009), hence elimination of GD3S improved memory and reduced amyloid-beta plaque loads (Bernardo et al., 2009). In the MPTP-induced (1-methyl-4-phenyl-1,2,3,6-tetrahydropyridine) PD model, suppression of GD3S exhibits neuroprotective qualities and may be a promising target for future research (Akkhawattanangkul et al., 2017). In another study, mice were intrastriatally injected with lentiviral-vector-mediated shRNA targeting GD3S (shGD3S) or scrambled-sequence control (scrRNA) and MPTP was administered. In shGD3S-treated mice, MPTP-induced lesions were smaller. MPTP caused bradykinesia and fine motor skill impairments in scrRNA-treated mice, while these deficiencies were absent in shGD3S-treated mice. These findings show GD3S inhibition prevents MPTP-induced nigrostriatal damage, bradykinesia, and fine motor skill impairments (Dhanushkodi et al., 2019). Furthermore, studies show that GD3 accumulation in plasma membrane lipid microdomains before mitochondrial translocation is crucial for Aβ-induced apoptosis (Kim et al., 2010). GD3S is rapidly activated in different cell types after apoptotic stimuli (Malisan et al., 2002), and GD3S overexpression was shown to cause vascular endothelial ECV304 cell death (Ha et al., 2004). Another research has provided evidence that drug-induced GD3S inhibition reduced CD95-induced apoptosis (De Maria et al., 1997). Additionally, GD3 causes mitochondrial membrane potential (ΔΨm) dissipation and swelling in isolated mitochondria, leading to cytochrome c, apoptosis-inducing factor, and caspase 9 release, prevented by enforced Bcl-2 activation (Rippo et al., 2000). In intact cells, suppression of GD3S expression significantly reduced ceramide-induced ΔΨm dissipation, demonstrating endogenous GD3 promotes mitochondrial alterations (Rippo et al., 2000).
The development of therapies targeted against GD3 and GD3S began several decades ago, utilizing various strategies, e.g., direct inhibition with monoclonal antibodies or stimulating the immune response with vaccines. Vaccine strategies have been developed mainly for potential treatment of malignant melanoma or other solid tumors (Yao et al., 1999). However, the clinical activity of some of them remained limited, leading to the cessation of vaccine development (Hein et al., 2024). Monoclonal antibodies, including R24, have been evaluated in clinical trials for melanoma patients. R24, a murine IgG3 anti-GD3 antibody, was tested in 61 patients with metastatic melanoma. Despite mild side effects, R24 confirmed the infiltration of immune cells in the tumor microenvironment following GD3 inhibition, opening new therapeutic developments (Houghton et al., 1985). Studies have shown GD3S inhibitors, such as Triptolide (TPL) from Tripterygium wilfordii, have anti-rheumatic, anti-inflammatory, immunomodulatory, and anti-tumor properties. TPL is a traditional Chinese medicinal herb that inhibits GD3S expression through NF-κB activation (Tong et al., 2021).
Furthermore, GD3SKO mice exhibit less age-related bone loss in skeletal tissue compared to WT mice (Yo et al., 2019). In GD3S KO mice, GD3 is not synthesized instead, other ganglioside species such as GD1a are present in higher amounts, and that increase could be linked to supporting bone maintenance and formation (Sasaki et al., 2022).
3 The bad
After establishing the positive effects of targeted GD3S inhibition, this section is to provide an overview of known negative consequences of decreased GD3S expression in vitro, available mouse models and human subject studies across different organ systems including the nervous system, retina, kidneys, and the liver.
GD3SKO mice, genetically engineered mice with nonfunctional St8sia1 gene are an invaluable tool in the research of physiological and biochemical properties of GD3S and altered ganglioside composition on various cell functions. They are of normal growth and overall nervous tissue morphology, however, they exhibit thermal hyperalgesia and mechanical allodynia, decreased response to formalin-induced prolonged noxious stimulation (Handa et al., 2005), morphological abnormalities in the sciatic nerve, and neuronal disturbances during peripheral nerve regeneration (Ribeiro-Resende et al., 2014). In addition, they exhibit decreased hippocampal neuronal loss following global cerebral ischemia (Wang et al., 2021), impaired olfactory and memory functions due to reduced neurogenesis in the subventricular zone and the dentate gyrus (Fuchigami et al., 2024), impairment in hippocampus-dependent memory function (Tang et al., 2021), depression-like behavior (Wang et al., 2014), a reduction in rod and retinal ganglion cell populations and electrophysiological alterations in retinal ganglion cells, photoreceptors, bipolar and amacrine cells, and reduced contrast sensitivity and visual acuity (Abreu et al., 2021), as well as mild impairment of spontaneous regeneration of neuromuscular junctions in older animals (Rupp et al., 2013). Analysis of neuronal stem cells (NSCs) obtained from GD3SKO mice has shown decreased self-renewal ability compared with those from the WT animals, accompanied by reduced expression and increased degradation rate of EGF receptor (Wang and Yu, 2013).
Ganglioside metabolism has been thoroughly examined in the neuronal retina (Panzetta and Allende, 2000), with substantial GD3S activity shown in the early and late development of rat and chicken retinas (Daniotti et al., 1991, 1992, 1994; Watanabe et al., 1996; Maxzúd and Maccioni, 1997; Panzetta and Allende, 2000). St8sia1 has been identified as a potential candidate in the acquisition of experience-dependent plasticity in murine visual cortex (Rietman et al., 2012), and polymorphisms in the human ST8SIA1 have been identified in patients with treatment-resistant ophthalmoplegia (Nel et al., 2017). The visual system of GD3SKO mice exhibits a reduction in retinal ganglion cell (RGC) density, optic nerve fiber number, RGC and photoreceptor electrical activity, visual acuity, and contrast sensitivity, indicating b-series gangliosides are essential for visual system structure and function (Abreu et al., 2021). Furthermore, gangliosides synthesized by GD3S, are present in the inner ear and contribute to the maintenance of the structure and function of auditory cells (Yoshikawa et al., 2009, 2015; Inokuchi et al., 2017; Kurabi et al., 2017).
Other organs are also affected by the lack of GD3S. A study on GD3SKO mice has shown changes in the expression of renal connexins and pannexin1 (Meter et al., 2022). Ganglioside GM3, accumulated in GD3SKO mice, has been documented in podocytes (Savas et al., 2020) and was related to glomerular hypertrophy occurring in diabetic human and rat kidneys (Novak et al., 2013). Furthermore, advanced glycation end products were shown to inhibit bovine retinal pericyte and rat renal mesangial cell proliferation associated with GD3S activity inhibition suggesting its role in the development of diabetic retinopathy and diabetic nephropathy (Masson et al., 2005). Human pancreatic islets of Langerhans contain five distinct endocrine β-4 cell types, which are distinguished by differential expression of ST8SIA1 and CD9, and their distribution is altered in type 2 diabetes (Dorrell et al., 2016). Gene expression analysis suggests ST8SIA1− β cells secrete more insulin (Dorrell et al., 2016). Correlation analysis has revealed ST8SIA1 as one of the genes with integrative changes in RNA expression and DNA methylation in the offspring born to women with pregestational type 1 diabetes (T1DM) (Knorr et al., 2022). Furthermore, in GD3SKO mice liver, a prominent difference in expression of cholesterogenic genes Srebp1a, Insig2a, and Ldl was found, indicating a relationship between gangliosides and regulation of cholesterol metabolism (Mlinac et al., 2012). With everything stated, keeping in mind the high RNA and protein expression of GD3S in multiple organs and tissues (Supplementary Figure S1), the consequences of GD3S inhibition are under-investigated and need to be explored further.
4 The unknown
The complete spectrum of proteins and lipids interacting with GD3S and the functional consequences of these interactions are not well-documented. Identifying them could provide insight into broader cellular processes influenced by GD3S. Additionally, how GD3S activity integrates with other metabolic and signaling pathways under different physiological conditions is still unknown.
To explore the relationship between gangliosides and metabolic pathways, we analyzed nucleus caudatus and putamen (together known as the corpus striatum (CPu), an important part of the basal ganglia) of 4.5 months old GD3SKO and WT mice using mass spectrometry imaging (MSI). The obtained spectra were used for further data processing to observe differences in the lipidome between GD3KO and WT mice. Compared to WTs, CPu region in GD3SKO mice shows significantly reduced (negative t-scores greater than 2 in absolute value) expression of 8 compounds functionally involved in energy metabolism (Table 1). Putatively identified compounds indicate additional not yet identified effects of GD3S and suggest inhibition of GD3S may affect pathways linked to energy production (Table 1).
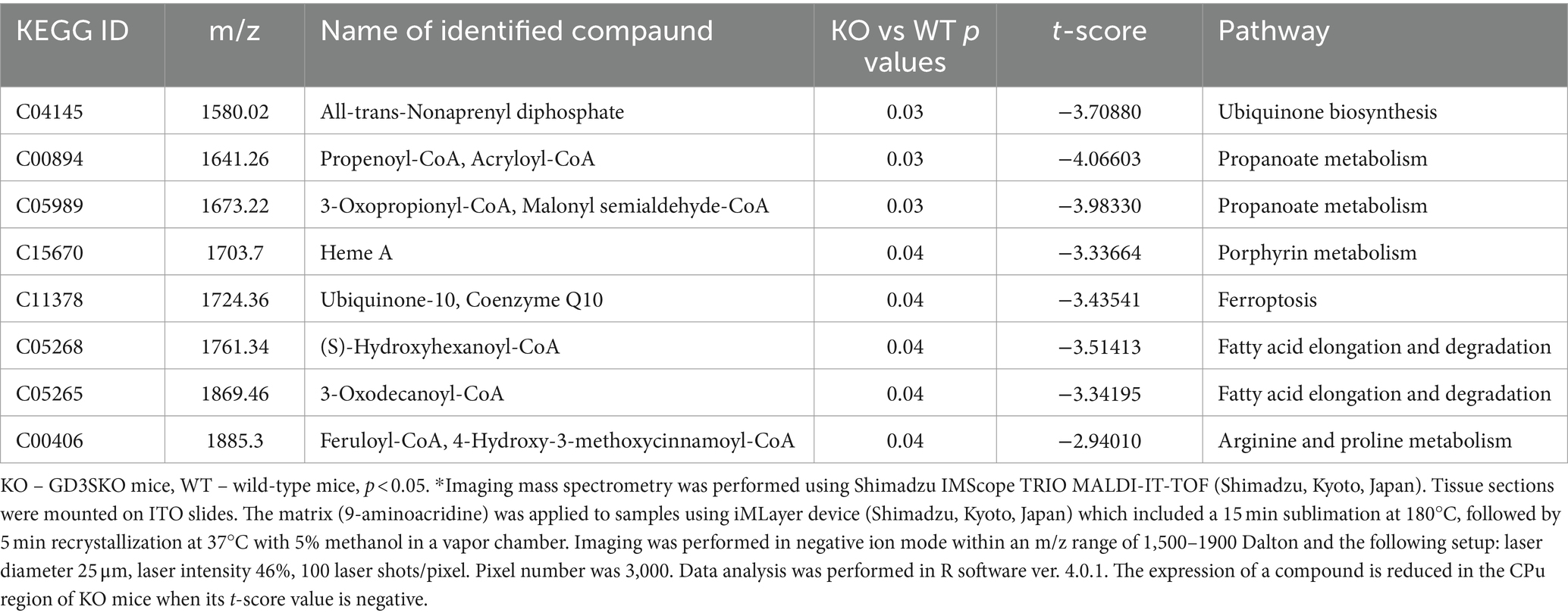
Table 1. Selected putatively identified significant m/z signals and t-scores detected by mass spectrometry imaging (MSI)* in the nucleus caudatus and putamen (CPu) region that are reduced in GD3SKO mice compared to WT mice.
Putatively identified compounds include propenoyl-CoA and 3-oxopropionyl-CoA which are part of the propanoate metabolism encompassing the metabolism of propionic acid. Propionyl-CoA is a key intermediate in the breakdown of odd-numbered fatty acids as well as some amino acids. Aberrations in this pathway are associated with various diseases, such as primary propionic acidemia and malonyl-CoA decarboxylase deficiency (Pena et al., 2012). In addition, metabolites from fatty acid metabolism can impact other pathways, including lipid and glycosphingolipid synthesis and are decreased in GD3SKO mice (Table 1). Peroxisome proliferator-activated receptors (PPARs), especially PPARα, regulate fatty acid metabolism and influence the expression of lipid metabolism genes (Hu et al., 2023), affecting ganglioside biosynthesis.
Moreover, decreased expression of ubiquinone 10 (coenzyme Q10), a component of the mitochondrial electron transport chain, is related to a range of neurologic, renal, cardiac, and other clinical manifestations (Hargreaves et al., 2020; Mantle et al., 2023). Decreased Q10 synthetic pathway is supported by decreased expression of all-trans-nonaprenyl diphosphate which is important for biosynthesis of ubiquinone and other terpenoid-quinones. Ubiquinone acts as an antioxidant, protecting cells from oxidative damage and reducing stress, vital for maintaining enzyme activities and cellular signaling (Gutierrez-Mariscal et al., 2020). Similarly, heme, which is also identified in our study (Table 1) helps maintain cellular redox balance through the electron transport chain, influencing enzyme expression and function. Heme A is a crucial component of molecular systems required for oxygen transport and cellular respiration. Based on reduced expression of ubiquinone and heme A, GD3S could be related to increased oxidative stress in the cells. In addition, enhanced sulfur metabolism affects the redox state and overall metabolic profile, potentially modulating GD3S-related pathways and influencing ganglioside synthesis involved in tumor growth and metastasis.
When analyzing the consequences of GD3S downregulation or inactivation, other research not directly investigating the mouse or cellular GD3SKO models needs to be considered. It is established that decreased GD3 levels might compromise lipid raft integrity and function, altering cellular communication and signal transduction (Sonnino and Prinetti, 2010). Because the integrity and functionality of cellular membranes are also essential for the correct operation of ion channels and transporters, a decrease in GD3S activity can seriously disturb ion homeostasis. This may result in extensive cellular malfunction, affecting functions like potassium and sodium homeostasis and calcium signaling (Bretscher and Munro, 1993; Ledeen et al., 1998; Simons and Gerl, 2010). For example, in a recent paper exploring ganglioside interactome (Zhang et al., 2024), specific ganglioside-protein interactions were identified, previously anticipated by literature (Ilic et al., 2021; Puljko et al., 2022). They include particular subunits of Na+/K+ ATPase and plasma membrane calcium ATPase (Zhang et al., 2024). Since specific gangliosides directly bind these proteins, changed ganglioside composition caused by GD3S inhibition and the consequent lack or accumulation of specific ganglioside species could lead to their disturbed activity and function. In addition, the reshaped ganglioside milieu leads to altered membrane fluidity and consequently dysfunctional ion channels, impacting the cell’s ability to maintain proper ion gradients (Puljko et al., 2021) which adds an additional energy-demanding challenge. Furthermore, decreased activity of GD3S has the potential to interfere with calcium signaling pathways and disturb intracellular calcium homeostasis (Ledeen et al., 1998), which has severe implications across multiple signaling pathways since Ca2+ is a second messenger. Also, gangliosides play a role in modulating cell–cell/cell-matrix interactions, which are critical for maintaining tissue structure and function, and lower activity of GD3S can impair cell adhesion and migration, potentially affecting tissue integrity and repair mechanisms (Hakomori Si, 2002).
5 Conclusions and perspectives
The known, both good and bad, as well as hypothesized consequences of decreased GD3S expression, are schematically shown in Figure 1. Altered GD3S activity and consequent changed b-series gangliosides synthesis are important contributors to various pathologies, including tumors and neurodegenerative diseases. GD3S overexpression is linked to enhanced tumor proliferation and metastasis in multiple cancers while inhibition of GD3S is a potential therapeutic strategy due to its role in malignancy-associated pathways, evidenced by studies showing reduced malignancy and metastasis upon GD3S suppression (Figure 1). On the other hand, decreased activity of GD3 synthase has significant implications for disturbed cellular homeostasis, including impairing immune responses, cellular adhesion, signal transmission, and membrane integrity. Our own MSI data (Table 1) shows decreased expression of the putatively identified compounds as the consequence of GD3S inactivation in GD3SKO mice which can generally negatively affect energy metabolism, regulatory pathways, and mitigation of oxidative stress.
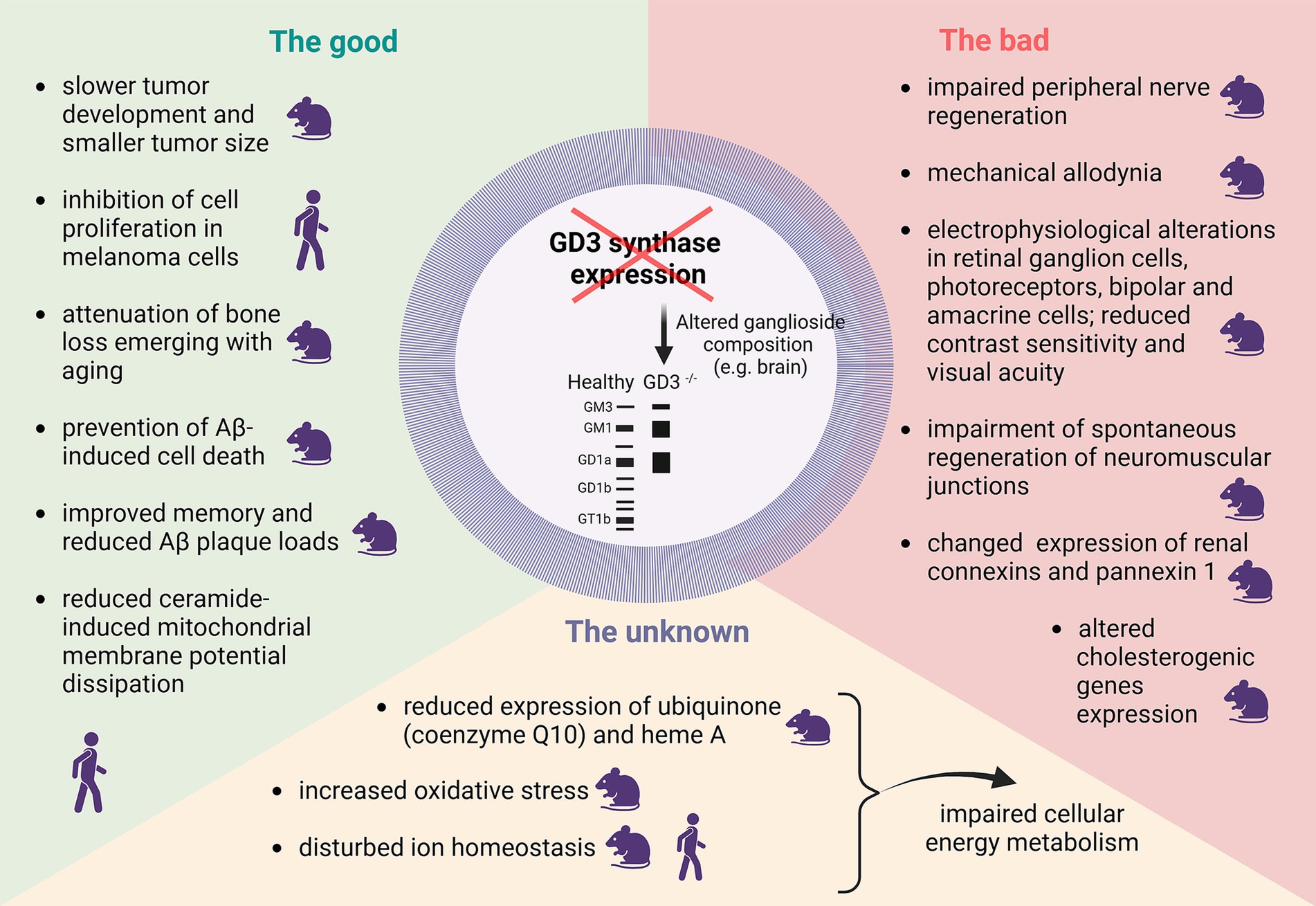
Figure 1. Schematic representation of the good, bad and the unknown consequences of decreased GD3 synthase expression. The human or mouse icon highlight whether the research was performed in mouse or human cell lines and/or tissue/ animal models. The figure was created with Biorender.com.
The possible effects of the disturbances on several physiological systems underscore the vital role of GD3S in cellular homeostasis. Further studies should try to elucidate the intricate regulatory pathways governing the expression and activity of GD3S, analyze its diverse functional roles across different tissues, and explore its potential as a therapeutic target in pathological conditions such as cancer, neurodegenerative diseases, and immune disorders. Understanding the molecular underpinnings of GD3S may facilitate the creation of novel strategies for the detection, management, and prevention of a variety of disorders, creating new avenues for targeted therapies and exploiting the role of GD3 synthase in cellular processes for clinical benefit.
Data availability statement
The original contributions presented in the study are included in the article/Supplementary material, further inquiries can be directed to the corresponding authors.
Ethics statement
The animal study was approved by regional ethics committees for scientific experiments and the Croatian Ministry of Agriculture (2158-61-07-14-118). The study was conducted in accordance with the local legislation and institutional requirements.
Author contributions
BP: Conceptualization, Writing – original draft, Writing – review & editing. JG: Investigation, Writing – original draft, Writing – review & editing. VP: Writing – original draft, Writing – review & editing. KI: Writing – original draft, Writing – review & editing. BV: Supervision, Writing – original draft, Writing – review & editing. SK-B: Conceptualization, Funding acquisition, Writing – original draft, Writing – review & editing, Supervision. MH: Writing – original draft, Writing – review & editing, Conceptualization, Funding acquisition. ŽD: Writing – original draft, Writing – review & editing, Formal analysis, Investigation. SB: Conceptualization, Writing – original draft, Writing – review & editing. KM-J: Conceptualization, Funding acquisition, Supervision, Writing – original draft, Writing – review & editing.
Funding
The author(s) declare financial support was received for the research, authorship, and/or publication of this article. This work was supported by research projects funded by the Croatian Science Foundation (grants raft tuning, IP-2014-09-2324 to MH and NeuroReact, IP-2016-06-8636 to SK-B), European Union through the European Regional Development Fund, Operational Programme “Competitiveness and Cohesion 2014–2020”, grant agreement no. KK.01.1.1.02.0015, “Research and diagnostics of malignant, infectious and rare metabolic diseases based on MALDI TOF technology” and University of Zagreb research support grant NEURO-MOD-PUMP, 10106-24-1546 (to KM-J).
Acknowledgments
Figure 1 was created with Biorender.com.
Conflict of interest
The authors declare that the research was conducted in the absence of any commercial or financial relationships that could be construed as a potential conflict of interest.
Publisher’s note
All claims expressed in this article are solely those of the authors and do not necessarily represent those of their affiliated organizations, or those of the publisher, the editors and the reviewers. Any product that may be evaluated in this article, or claim that may be made by its manufacturer, is not guaranteed or endorsed by the publisher.
Supplementary material
The Supplementary material for this article can be found online at: https://www.frontiersin.org/articles/10.3389/fnmol.2024.1465013/full#supplementary-material
References
Abreu, C. A., Teixeira-Pinheiro, L. C., Lani-Louzada, R., da Silva-Junior, A. J., Vasques, J. F., Gubert, F., et al. (2021). GD3 synthase deletion alters retinal structure and impairs visual function in mice. J. Neurochem. 158, 694–709. doi: 10.1111/JNC.15443
Akkhawattanangkul, Y., Maiti, P., Xue, Y., Aryal, D., Wetsel, W. C., Hamilton, D., et al. (2017). Targeted deletion of GD3 synthase protects against MPTP-induced neurodegeneration. Genes Brain Behav. 16, 522–536. doi: 10.1111/GBB.12377
Bernardo, A., Harrison, F. E., McCord, M., Zhao, J., Bruchey, A., Davies, S. S., et al. (2009). Elimination of GD3 synthase improves memory and reduces amyloid-β plaque load in transgenic mice. Neurobiol. Aging 30, 1777–1791. doi: 10.1016/j.neurobiolaging.2007.12.022
Bretscher, M. S., and Munro, S. (1993). Cholesterol and the Golgi apparatus. Science 261, 1280–1281. doi: 10.1126/science.8362242
Cazet, A., Lefebvre, J., Adriaenssens, E., Julien, S., Bobowski, M., Grigoriadis, A., et al. (2010). GD₃ synthase expression enhances proliferation and tumor growth of MDA-MB-231 breast cancer cells through c-met activation. Mol. Cancer Res. 8, 1526–1535. doi: 10.1158/1541-7786.MCR-10-0302
Daniotti, J. L., Lancia, C. A., Rösner, H., and Maccioni, H. J. F. (1991). GD3 prevalence in adult rat retina correlates with the maintenance of a high GD3−/GM2-synthase activity ratio throughout development. J. Neurochem. 57, 2054–2058. doi: 10.1111/J.1471-4159.1991.TB06421.X
Daniotti, J. L., Landa, C. A., and Maccioni, H. J. F. (1994). Regulation of ganglioside composition and synthesis is different in developing chick retinal pigment epithelium and neural retina. J. Neurochem. 62, 1131–1136. doi: 10.1046/J.1471-4159.1994.62031131.X
Daniotti, J. L., Landa, C. A., Rosner, H., and Maccioni, H. J. F. (1992). Adult rat retina interneurons synthesize GD3: GD3 expression by these cells is regulated by cell-cell interactions. J. Neurochem. 59, 107–117. doi: 10.1111/J.1471-4159.1992.TB08881.X
De Maria, R., Lenti, L., Malisan, F., D’Agostino, F., Tomassini, B., Zeuner, A., et al. (1997). Requirement for GD3 ganglioside in CD95-and ceramide-induced apoptosis. Science 277, 1652–1655. doi: 10.1126/SCIENCE.277.5332.1652
Dhanushkodi, A., Xue, Y., Roguski, E. E., Ding, Y., Matta, S. G., Heck, D., et al. (2019). Lentiviral-mediated knock-down of GD3 synthase protects against MPTP-induced motor deficits and neurodegeneration. Neurosci. Lett. 692, 53–63. doi: 10.1016/J.NEULET.2018.10.038
Dorrell, C., Schug, J., Canaday, P. S., Russ, H. A., Tarlow, B. D., Grompe, M. T., et al. (2016). Human islets contain four distinct subtypes of β cells. Nat. Commun. 7:11756. doi: 10.1038/ncomms11756
Fuchigami, T., Itokazu, Y., and Yu, R. K. (2024). Ganglioside GD3 regulates neural stem cell quiescence and controls postnatal neurogenesis. Glia 72, 167–183. doi: 10.1002/GLIA.24468
Gutierrez-Mariscal, F. M., Arenas-de Larriva, A. P., Limia-Perez, L., Romero-Cabrera, J. L., Yubero-Serrano, E. M., and López-Miranda, J. (2020). Coenzyme Q10 supplementation for the reduction of oxidative stress: clinical implications in the treatment of chronic diseases. Int. J. Mol. Sci. 21:7870. doi: 10.3390/ijms21217870
Ha, K. T., Lee, Y. C., and Kim, C. H. (2004). Overexpression of GD3 synthase induces apoptosis of vascular endothelial ECV304 cells through downregulation of Bcl-2. FEBS Lett. 568, 183–187. doi: 10.1016/j.febslet.2004.05.020
Hakomori Si, S.-I. (2002). The glycosynapse. Proc. Natl. Acad. Sci. USA 99, 225–232. doi: 10.1073/pnas.012540899
Handa, Y., Ozaki, N., Honda, T., Furukawa, K., Tomita, Y., Inoue, M., et al. (2005). GD3 synthase gene knockout mice exhibit thermal hyperalgesia and mechanical allodynia but decreased response to formalin-induced prolonged noxious stimulation. Pain 117, 271–279. doi: 10.1016/J.PAIN.2005.06.016
Hargreaves, I., Heaton, R. A., and Mantle, D. (2020). Disorders of human coenzyme Q10 metabolism: an overview. Int. J. Mol. Sci. 21:6695. doi: 10.3390/ijms21186695
Hedberg, K. M., Mahesparan, R., Read, T. A., Tysnes, B. B., Thorsen, F., Visted, T., et al. (2001). The glioma-associated gangliosides 3′-isoLM1, GD3 and GM2 show selective area expression in human glioblastoma xenografts in nude rat brains. Neuropathol. Appl. Neurobiol. 27, 451–464. doi: 10.1046/J.1365-2990.2001.00353.X
Hein, V., Baeza-Kallee, N., Bertucci, A., Colin, C., Tchoghandjian, A., Figarella-Branger, D., et al. (2024). GD3 ganglioside is a promising therapeutic target for glioma patients. Neurooncol. Adv. 6:vdae038. doi: 10.1093/noajnl/vdae038
Houghton, A. N., Mintzer, D., Cordon-Cardo, C., Welt, S., Fliegel, B., Vadhan, S., et al. (1985). Mouse monoclonal IgG3 antibody detecting GD3 ganglioside: a phase I trial in patients with malignant melanoma. Proc. Natl. Acad. Sci. USA 82, 1242–1246. doi: 10.1073/PNAS.82.4.1242
Hu, P., Li, K., Peng, X., Kan, Y., Li, H., Zhu, Y., et al. (2023). Nuclear receptor PPARα as a therapeutic target in diseases associated with lipid metabolism disorders. Nutrients 15:4772. doi: 10.3390/nu15224772
Ilic, K., Lin, X., Malci, A., Stojanović, M., Puljko, B., Rožman, M., et al. (2021). Plasma membrane calcium ATPase-Neuroplastin complexes are selectively stabilized in GM1-containing lipid rafts. Int. J. Mol. Sci. 22:13590. doi: 10.3390/ijms222413590
Inokuchi, J.-I., Go, S., Yoshikawa, M., and Strauss, K. (2017). Gangliosides and hearing. Biochim. Biophys. Acta Gen. Subj. 1861, 2485–2493. doi: 10.1016/j.bbagen.2017.05.025
Iwasawa, T., Zhang, P., Ohkawa, Y., Momota, H., Wakabayashi, T., Ohmi, Y., et al. (2018). Enhancement of malignant properties of human glioma cells by ganglioside GD3/GD2. Int. J. Oncol. 52, 1255–1266. doi: 10.3892/IJO.2018.4266
Kasprowicz, A., Sophie, G. D., Lagadec, C., and Delannoy, P. (2022, 2022). Role of GD3 synthase ST8Sia I in cancers. Cancers 14:1299. doi: 10.3390/CANCERS14051299
Kim, J. K., Kim, S. H., Cho, H. Y., Shin, H. S., Sung, H. R., Jung, J. R., et al. (2010). GD3 accumulation in cell surface lipid rafts prior to mitochondrial targeting contributes to amyloid-β-induced apoptosis. J. Korean Med. Sci. 25:1492. doi: 10.3346/JKMS.2010.25.10.1492
Knorr, S., Skakkebæk, A., Just, J., Johannsen, E. B., Trolle, C., Vang, S., et al. (2022). Epigenetic and transcriptomic alterations in offspring born to women with type 1 diabetes (the EPICOM study). BMC Med. 20, 1–16. doi: 10.1186/S12916-022-02514-X/TABLES/3
Kurabi, A., Keithley, E. M., Housley, G. D., Ryan, A. F., and Wong, A. C. Y. (2017). Cellular mechanisms of noise-induced hearing loss. Hear. Res. 349, 129–137. doi: 10.1016/J.HEARES.2016.11.013
Lama, G., Mangiola, A., Proietti, G., Colabianchi, A., Angelucci, C., D’Alessio, A., et al. (2016). Progenitor/stem cell markers in brain adjacent to glioblastoma: GD3 ganglioside and NG2 proteoglycan expression. J. Neuropathol. Exp. Neurol. 75, 134–147. doi: 10.1093/JNEN/NLV012
Ledeen, R. W., Wu, G., Lu, Z. H., Kozireski-Chuback, D., and Fang, Y. (1998). The role of GM1 and other gangliosides in neuronal differentiation. Overview and new finding. Ann. N. Y. Acad. Sci. 845, 161–175. doi: 10.1111/j.1749-6632.1998.tb09669.x
Lee, M., Kim, K. S., Fukushi, A., Kim, D. H., Kim, C. H., and Lee, Y. C. (2018). Transcriptional activation of human GD3 synthase (hST8Sia I) gene in curcumin-induced autophagy in A549 human lung carcinoma cells. Int. J. Mol. Sci. 19:1943. doi: 10.3390/ijms19071943
Ma, W., Zhao, X., Liang, L., Wang, G., Li, Y., Miao, X., et al. (2017). miR-146a and miR-146b promote proliferation, migration and invasion of follicular thyroid carcinoma via inhibition of ST8SIA4. Oncotarget 8, 28028–28041. doi: 10.18632/ONCOTARGET.15885
Malisan, F., Franchi, L., Tomassini, B., Ventura, N., Condò, I., Rippo, M. R., et al. (2002). Acetylation suppresses the proapoptotic activity of GD3 ganglioside. J. Exp. Med. 196, 1535–1541. doi: 10.1084/JEM.20020960
Mantle, D., Millichap, L., Castro-Marrero, J., and Hargreaves, I. P. (2023). Primary coenzyme Q10 deficiency: an update. Antioxidants (Basel) 12:1652. doi: 10.3390/antiox12081652
Masson, E., Troncy, L., Ruggiero, D., Wiernsperger, N., Lagarde, M., and El Bawab, S. (2005). A-series gangliosides mediate the effects of advanced glycation end products on Pericyte and mesangial cell ProliferationA common mediator for retinal and renal Microangiopathy? Diabetes 54, 220–227. doi: 10.2337/DIABETES.54.1.220
Maxzúd, M. K., and Maccioni, H. J. F. (1997). Compartmental organization of the synthesis of GM3, GD3, and GM2 in golgi membranes from neural retina cells. Neurochem. Res. 22, 455–461. doi: 10.1023/A:1027311811334
Mennel, H. D., Bosslet, K., Geisseli, H., and Bauer, B. L. (2000). Immunohistochemically visualized localisation of gangliosides Glac2 (GD3) and Gtri2 (GD2) in cells of human intracranial tumors. Exp. Toxicol. Pathol. 52, 277–285. doi: 10.1016/S0940-2993(00)80046-9
Meter, D., Racetin, A., Vukojević, K., Balog, M., Ivić, V., Zjalić, M., et al. (2022). A lack of GD3 synthase leads to impaired renal expression of connexins and Pannexin1 in St8sia1 knockout mice. Int. J. Mol. Sci. 23:6237. doi: 10.3390/ijms23116237
Mlinac, K., Fon Tacer, K., Heffer, M., Rozman, D., and Kalanj Bognar, S. (2012). Cholesterogenic genes expression in brain and liver of ganglioside-deficient mice. Mol. Cell. Biochem. 369, 127–133. doi: 10.1007/S11010-012-1375-Y
Nel, M., Dashti, J. S., Gamieldien, J., and Heckmann, J. M. (2017). Exome sequencing identifies targets in the treatment-resistant ophthalmoplegic subphenotype of myasthenia gravis. Neuromuscul. Disord. 27, 816–825. doi: 10.1016/J.NMD.2017.06.009
Novak, A., Režić Mužinić, N., Čikeš Čulić, V., Božić, J., Tičinović Kurir, T., Ferhatović, L., et al. (2013). Renal distribution of ganglioside GM3 in rat models of types 1 and 2 diabetes. J. Physiol. Biochem. 69, 727–735. doi: 10.1007/s13105-013-0249-4
Ohkawa, Y., Zhang, P., Momota, H., Kato, A., Hashimoto, N., Ohmi, Y., et al. (2021). Lack of GD3 synthase (St8sia1) attenuates malignant properties of gliomas in genetically engineered mouse model. Cancer Sci. 112, 3756–3768. doi: 10.1111/CAS.15032
Panzetta, P., and Allende, M. L. (2000). Ganglioside expression during differentiation of chick retinal cells in vitro. Neurochem. Res. 25, 163–169. doi: 10.1023/A:1007560004244
Pena, L., Franks, J., Chapman, K. A., Gropman, A., Ah Mew, N., Chakrapani, A., et al. (2012). Natural history of propionic acidemia. Mol. Genet. Metab. 105, 5–9. doi: 10.1016/j.ymgme.2011.09.022
Puljko, B., Stojanović, M., Ilic, K., Kalanj-Bognar, S., and Mlinac-Jerkovic, K. (2022). Start me up: how can surrounding gangliosides affect sodium-potassium ATPase activity and steer towards pathological ion imbalance in neurons? Biomedicines 10:1518. doi: 10.3390/biomedicines10071518
Puljko, B., Stojanović, M., Ilic, K., Maček Hrvat, N., Zovko, A., Damjanović, V., et al. (2021). Redistribution of gangliosides accompanies thermally induced Na+, K+-ATPase activity alternation and submembrane localisation in mouse brain. Biochim Biophys Acta Biomembr 1863:183475. doi: 10.1016/J.BBAMEM.2020.183475
Ribeiro-Resende, V. T., Araújo Gomes, T., de Lima, S., Nascimento-Lima, M., Bargas-Rega, M., Santiago, M. F., et al. (2014). Mice lacking GD3 synthase display morphological abnormalities in the sciatic nerve and neuronal disturbances during peripheral nerve regeneration. PLoS One 9:e108919. doi: 10.1371/journal.pone.0108919
Rietman, M. L., Sommeijer, J.-P., Neuro-Bsik Mouse Phenomics ConsortiumLevelt, C. N., and Heimel, J. A. (2012). Candidate genes in ocular dominance plasticity. Front. Neurosci. 6:11. doi: 10.3389/fnins.2012.00011
Rippo, M. R., Malisan, F., Rayagnan, L., Tomassini, B., Condo, I., Costantini, P., et al. (2000). GD3 ganglioside directly targets mitochondria in a bcl-2-controlled fashion. FASEB J. 14, 2047–2054. doi: 10.1096/FJ.99-1028COM
Ruan, S., Raj, B. K. M., and Lloyd, K. O. (1999). Relationship of glycosyltransferases and mRNA levels to ganglioside expression in neuroblastoma and melanoma cells. J. Neurochem. 72, 514–521. doi: 10.1046/J.1471-4159.1999.0720514.X
Rupp, A., Cunningham, M. E., Yao, D., Furukawa, K., and Willison, H. J. (2013). The effects of age and ganglioside composition on the rate of motor nerve terminal regeneration following antibody-mediated injury in mice. Synapse 67, 382–389. doi: 10.1002/SYN.21648
Sasaki, E., Hamamura, K., Mishima, Y., Furukawa, K., Nagao, M., Kato, H., et al. (2022). Attenuation of bone formation through a decrease in osteoblasts in mutant mice lacking the GM2/GD2 synthase gene. Int. J. Mol. Sci. 23:9044. doi: 10.3390/ijms23169044
Savas, B., Astarita, G., Aureli, M., Sahali, D., and Ollero, M. (2020). Gangliosides in Podocyte biology and disease. Int. J. Molec. Sci. 21:9645. doi: 10.3390/IJMS21249645
Schengrund, C.-L. (2023). The Ying and yang of ganglioside function in cancer. Cancers (Basel) 15:5362. doi: 10.3390/cancers15225362
Schnaar, R. L. (2016). Gangliosides of the vertebrate nervous system. J. Mol. Biol. 428, 3325–3336. doi: 10.1016/J.JMB.2016.05.020
Schnaar, R. L. (2019). The biology of gangliosides. Adv. Carbohydr. Chem. Biochem. 76, 113–148. doi: 10.1016/BS.ACCB.2018.09.002
Seo, S. Y., Ju, W. S., Kim, K., Kim, J., Yu, J. O., Ryu, J.-S., et al. (2024). Quercetin induces mitochondrial apoptosis and downregulates ganglioside GD3 expression in melanoma cells. Int. J. Mol. Sci. 25:5146. doi: 10.3390/IJMS25105146
Simons, K., and Gerl, M. J. (2010). Revitalizing membrane rafts: new tools and insights. Nat. Rev. Mol. Cell Biol. 11, 688–699. doi: 10.1038/nrm2977
Sipione, S., Monyror, J., Galleguillos, D., Steinberg, N., and Kadam, V. (2020). Gangliosides in the brain: physiology, pathophysiology and therapeutic applications. Front. Neurosci. 14:572965. doi: 10.3389/FNINS.2020.572965
Sonnino, S., and Prinetti, A. (2010). Gangliosides as regulators of cell membrane organization and functions. Adv. Exp. Med. Biol. 688, 165–184. doi: 10.1007/978-1-4419-6741-1_12
Svennerholm, L. (1994). Designation and schematic structure of gangliosides and allied glycosphingolipids. Prog. Brain Res. 101, xi–xiv. doi: 10.1016/S0079-6123(08)61935-4
Tang, F. L., Wang, J., Itokazu, Y., and Yu, R. K. (2021). Ganglioside GD3 regulates dendritic growth in newborn neurons in adult mouse hippocampus via modulation of mitochondrial dynamics. J. Neurochem. 156, 819–833. doi: 10.1111/JNC.15137
Tong, L., Zhao, Q., Datan, E., Lin, G. Q., Minn, I., Pomper, M. G., et al. (2021). Triptolide: reflections on two decades of research and prospects for the future. Nat. Prod. Rep. 38, 843–860. doi: 10.1039/D0NP00054J
Wang, J., Cheng, A., Wakade, C., and Yu, R. K. (2014). Ganglioside GD3 is required for neurogenesis and long-term maintenance of neural stem cells in the postnatal mouse brain. J. Neurosci. 34, 13790–13800. doi: 10.1523/JNEUROSCI.2275-14.2014
Wang, J., and Yu, R. K. (2013). Interaction of ganglioside GD3 with an EGF receptor sustains the self-renewal ability of mouse neural stem cells in vitro. Proc. Natl. Acad. Sci. USA 110, 19137–19142. doi: 10.1073/PNAS.1307224110
Wang, J., Zhang, Q., Lu, Y., Dong, Y., Dhandapani, K. M., Brann, D. W., et al. (2021). Ganglioside GD3 is up-regulated in microglia and regulates phagocytosis following global cerebral ischemia. J. Neurochem. 158, 737–752. doi: 10.1111/JNC.15455
Watanabe, Y., Nara, K., Takahashi, H., Nagai, Y., and Sanai, Y. (1996). The molecular cloning and expression of α2,8-Sialyltransferase (GD3 synthase) in a rat brain. J. Biochem. 120, 1020–1027. doi: 10.1093/OXFORDJOURNALS.JBCHEM.A021494
Yao, T. J., Meyers, M., Livingston, P. O., Houghton, A. N., and Chapman, P. B. (1999). Immunization of melanoma patients with BEC2-keyhole limpet hemocyanin plus BCG intradermally followed by intravenous booster immunizations with BEC2 to induce anti-GD3 ganglioside antibodies. Clin. Cancer Res. 5, 77–81.
Yeh, S. C., Wang, P. Y., Lou, Y. W., Khoo, K. H., Hsiao, M., Hsu, T. L., et al. (2016). Glycolipid GD3 and GD3 synthase are key drivers for glioblastoma stem cells and tumorigenicity. Proc. Natl. Acad. Sci. USA 113, 5592–5597. doi: 10.1073/pnas.1604721113
Yo, S., Hamamura, K., Mishima, Y., Hamajima, K., Mori, H., Furukawa, K., et al. (2019). Deficiency of GD3 synthase in mice resulting in the attenuation of bone loss with aging. Int. J. Mol. Sci. 20:20. doi: 10.3390/ijms20112825
Yoshikawa, M., Go, S., Suzuki, S.-I., Suzuki, A., Katori, Y., Morlet, T., et al. (2015). Ganglioside GM3 is essential for the structural integrity and function of cochlear hair cells. Hum. Mol. Genet. 24, 2796–2807. doi: 10.1093/HMG/DDV041
Yoshikawa, M., Go, S., Takasaki, K., Kakazu, Y., Ohashi, M., Nagafuku, M., et al. (2009). Mice lacking ganglioside GM3 synthase exhibit complete hearing loss due to selective degeneration of the organ of Corti. Proc. Natl. Acad. Sci. USA 106, 9483–9488. doi: 10.1073/PNAS.0903279106
Yu, R. K., Tsai, Y. T., Ariga, T., and Yanagisawa, M. (2011). Structures, biosynthesis, and functions of gangliosides—an overview. J. Oleo Sci. 60, 537–544. doi: 10.5650/JOS.60.537
Zhang, P., Ohkawa, Y., Yamamoto, S., Momota, H., Kato, A., Kaneko, K., et al. (2021). St8sia1-deficiency in mice alters tumor environments of gliomas, leading to reduced disease severity. Nagoya J. Med. Sci. 83, 535–549. doi: 10.18999/NAGJMS.83.3.535
Keywords: ST8SIA1, gangliosides, glycosphingolipids, lipid metabolism, lipidomics
Citation: Puljko B, Grbavac J, Potočki V, Ilic K, Viljetić B, Kalanj-Bognar S, Heffer M, Debeljak Ž, Blažetić S and Mlinac-Jerkovic K (2024) The good, the bad, and the unknown nature of decreased GD3 synthase expression. Front. Mol. Neurosci. 17:1465013. doi: 10.3389/fnmol.2024.1465013
Edited by:
Riccardo Ghidoni, University of Milan, ItalyReviewed by:
Francesca Cencetti, University of Florence, ItalyCopyright © 2024 Puljko, Grbavac, Potočki, Ilic, Viljetić, Kalanj-Bognar, Heffer, Debeljak, Blažetić and Mlinac-Jerkovic. This is an open-access article distributed under the terms of the Creative Commons Attribution License (CC BY). The use, distribution or reproduction in other forums is permitted, provided the original author(s) and the copyright owner(s) are credited and that the original publication in this journal is cited, in accordance with accepted academic practice. No use, distribution or reproduction is permitted which does not comply with these terms.
*Correspondence: Senka Blažetić, c2Vua2FAYmlvbG9naWphLnVuaW9zLmhy; Kristina Mlinac-Jerkovic, a3Jpc3RpbmEubWxpbmFjLmplcmtvdmljQG1lZi5ocg==