- 1Key Laboratory of Vascular Biology and Translational Medicine, Hunan University of Chinese Medicine, Changsha, China
- 2People’s Hospital of Ningxiang City, Hunan University of Chinese Medicine, Changsha, China
- 3National Key Laboratory Cultivation Base of Chinese Medicinal Powder & Innovative Medicinal Jointly Established by Province and Ministry, Hunan University of Chinese Medicine, Changsha, China
Objective: This study aims to visualize the trends and hotspots in the research of “ferroptosis in PD” and “pyroptosis in PD” through bibliometric analysis from the past to 2024.
Methods: Literature was retrieved from the Web of Science Core Collection (WoSCC) from the past to February 16, 2024, and bibliometric analysis was conducted using Vosviewer and Citespace.
Results: 283 and 542 papers were collected in the field of “ferroptosis in PD” and “pyroptosis in PD.” The number of publications in both fields has increased yearly, especially in “ferroptosis in PD,” which will become the focus of PD research. China, the United States and England had extensive exchanges and collaborations in both fields, and more than 60% of the top 10 institutions were from China. In the fields of “ferroptosis in PD” and “pyroptosis in PD,” the University of Melbourne and Nanjing Medical University stood out in terms of publication numbers, citation frequency, and centrality, and the most influential journals were Cell and Nature, respectively. The keyword time zone map showed that molecular mechanisms and neurons were the research hotspots of “ferroptosis in PD” in 2023, while memory and receptor 2 were the research hotspots of “pyroptosis in PD” in 2023, which may predict the future research direction.
Conclusion: This study provides insights into the development, collaborations, research themes, hotspots, and tendencies of “ferroptosis in PD” and “pyroptosis in PD.” Overall situation of these fields is available for researchers to further explore the underlying mechanisms and potential treatments.
1 Introduction
Parkinson’s disease (PD) is a common degenerative disease of the central nervous system (CNS) (Huang et al., 2024), which has special pathological changes including the degeneration and loss of dopaminergic (DA) neurons in the substantia nigra (SN), decreased DA content in the striatum, and the formation of Lewy bodies dominated by the aggregation of α-synuclein (α-syn) (Naoi et al., 2024). The symptoms include motor symptoms, and non-motor symptoms (NMS) (Israni et al., 2024), which may precede the onset of motor symptoms by several years and increase the risk of overall disability. Currently, some motor symptoms can only be controlled by anticholinergic drugs (e.g., trihexyphenidyl) and dopaminergic drugs (e.g., levodopa), etc., but cannot prevent the progression or reduce the risk of disability (Siripurapu et al., 2023). Therefore, further mechanism study to find therapeutic breakthroughs is the core issues that need to be urgently addressed.
Cell death is a fundamental biological phenomenon that holds significant importance in the growth, development, and maintenance of tissue homeostasis in organisms. Recently, controlled cell death pathways with unique pathophysiological characteristics have been discovered, including apoptosis, necroptosis, autophagy, pyroptosis and ferroptosis, as well as uncontrolled forms of cell death such as necrosis (Qiu et al., 2023). Among them, ferroptosis and pyroptosis have been widely shown to affect the disease progression of PD by modulating pathological processes such as inflammatory response (Huang and Li, 2024) and oxidative stress (Yao et al., 2024). Moreover, targeting key molecules of multiple cell death modes can effectively alleviate PD symptoms.
Ferroptosis is programmed cell death depending on iron accumulation, and is associated with increased levels of iron and reactive oxygen species (ROS), decreased glutathione (GSH) levels, and decreased glutathione peroxidase 4 (GPX4) in cells. Among them, lipid peroxides (LPO) accumulation is a key component of ferroptosis, which is regulated by multiple oxidative and antioxidant systems (Chen X. et al., 2021). Accumulation of iron in the SN has been broadly correlated with the development of PD. Increased iron content in the SN augments free radical production through the Fenton reaction, leading to neuronal degeneration. In addition, iron accumulation has been shown to promote α-syn aggregation, which occurs synergistically, inducing the formation of Lewy body and degenerative necrosis of DA neurons (Ding et al., 2023). Numerous studies have shown that PD patients have increased levels of free iron and LPO, and decreased levels of GSH. Furthermore, genetic mutations involved in iron metabolism may cause increased intracellular iron input or decreased output, constituting a predisposing factor for the development of PD (Wang et al., 2023).
Pyroptosis is an inflammatory cell death mediated by the gasdermin (GSDM) family, including canonical mechanisms mediated by caspase-1 and non-canonical mechanisms mediated by caspase-4/5/11 (Wu et al., 2024). The activation of caspase-1 or caspase-4/5/11, induced by immune activation, cleaves GSDM and leads to pyroptosis (Zhang et al., 2023). In addition, activated caspase-1 is associated with the formation and release of IL-1β and IL-18. It has been demonstrated that pyroptosis is associated with the pathomechanism of PD. α-Syn, a PD pathology-associated protein, promotes the progression of PD by activating microglia and subsequently inducing neuroinflammation after release by damaged neurons. Pyroptosis can increase inflammatory effects to damage DA neurons by promoting IL-1β secretion and activation of inflammasomes. Activated NLRP3 is detected at sites of DA cell loss in the SN of mouse PD model and cerebrospinal fluid of patients with PD. Moreover, the levels of IL-1β and IL-18 in cerebrospinal fluid and serum samples are significantly higher in PD patients than in control (Zheng et al., 2023).
Bibliometrics is a discipline that uses mathematical and statistical measurement methods to study the quantitative relationships and developmental patterns of literature and related information, which can explore the dynamic characteristics and research hotspots of a particular field (Öztürk et al., 2024). Bibliometric analysis typically utilizes various software and algorithms to present the trend of annual publications, countries/regions, institutions, journals, authors, and co-citations within a domain. Given that bibliometric analyses can offer researchers a more comprehensive understanding of the research in their field, there is a lack of systematic analysis of literature related to ferroptosis and pyroptosis in PD. Therefore, this study applied bibliometric methods to systematically summarize the current research status and hotspots in the fields of ferroptosis in PD and pyroptosis in PD from the past to February 16, 2024. Furthermore, it discussed the possible future research trends, thereby providing the literature data support for the research on the mechanism of ferroptosis and pyroptosis in PD and the development of drugs targeting key molecules of ferroptosis and pyroptosis.
2 Materials and methods
2.1 Data source
All data were obtained from the Science Citation Index Expanded (SCI-EXPANDED) in the Web of Science Core Collection (WoSCC) database. The following search strategy was used: #1 TS=Parkinson*, #2 TS = ferropto*, and #3 TS = pyropto* OR TS = inflammasome were defined, and then searches were conducted combining #1 AND #2, and #1 AND #3, respectively. The deadline for search was February 16, 2024. The methodology for data retrieval and collection is shown in Figure 1. The included documents were exported in plain text format with complete records and references, and saved as “download-txt.”
2.2 Statistical methods
Bibliometric and visualization analyses were performed using Vosviewer 1.6.19 and Citespace 6.2.R5. Focusing on the graphical depiction of bibliometrics, Vosviewer was utilized for visualizing the collaborations of countries/regions, institutions, authors and journals, co-citations of authors, journals, and references as well as the co-occurrence of keywords. Citespace can focus on extracting prospective data in the literature, so it was used for burst detection in references, dual-overlay analysis of journals, clustering analysis and time zone analysis of keywords.
3 Results
3.1 Annual publication volume and trend analysis
The number of publications in each period reflects the trend of research in the field. As shown in Figure 2, the research on “pyroptosis in PD” started earlier, with the first publication in 2010, which described the initiators and signaling pathways of inflammasome activation and its precise role in the pathogenesis of CNS inflammation (Chakraborty et al., 2010). The first study on “ferroptosis in PD” was published in 2013, which discussed in depth that the HIF prolyl hydroxylase could be an emerging target for iron chelators to inhibit ferroptosis in primary neurons and repair neuronal cell degeneration (Speer et al., 2013). Relevant studies in both fields have been reported gradually since then. Publication outputs in both areas were extremely low from the publication of the first relevant study until 2015, and the research remained stagnant, indicating that “ferroptosis in PD” and “pyroptosis in PD” were still in their infancy and had not attracted widespread attention. From 2016 to 2019, the volume of literature grew slowly in both areas, but the number of studies on “pyroptosis in PD” was higher than that on “ferroptosis in PD.” From 2020 to 2021, the research on “pyroptosis in PD” grew rapidly, while the research on “ferroptosis in PD” grew more slowly, indicating that “pyroptosis in PD” was the hotspot of the research in this stage, and the research focused on the development of a variety of PD therapeutics targeting molecules involved in the NLRP3 inflammatory pathway and the pyroptosis pathway, and much progress had been made in this phase. From 2022 to 2023, the research on “ferroptosis in PD” increased rapidly, while the research on “pyroptosis in PD” slowed down, indicating that more researchers were focusing on “ferroptosis in PD,” making it the current research hotspot, and the research mainly focused on the novel ferroptosis-related genes and molecular patterns in PD, which provided new directions for finding new therapeutic targets and early diagnostic specific biomarkers. The data collection up to February 2024 were insufficient, and including the 2024 data in the figure could negatively impact the trend analysis. Therefore, it will not be displayed in the graph.
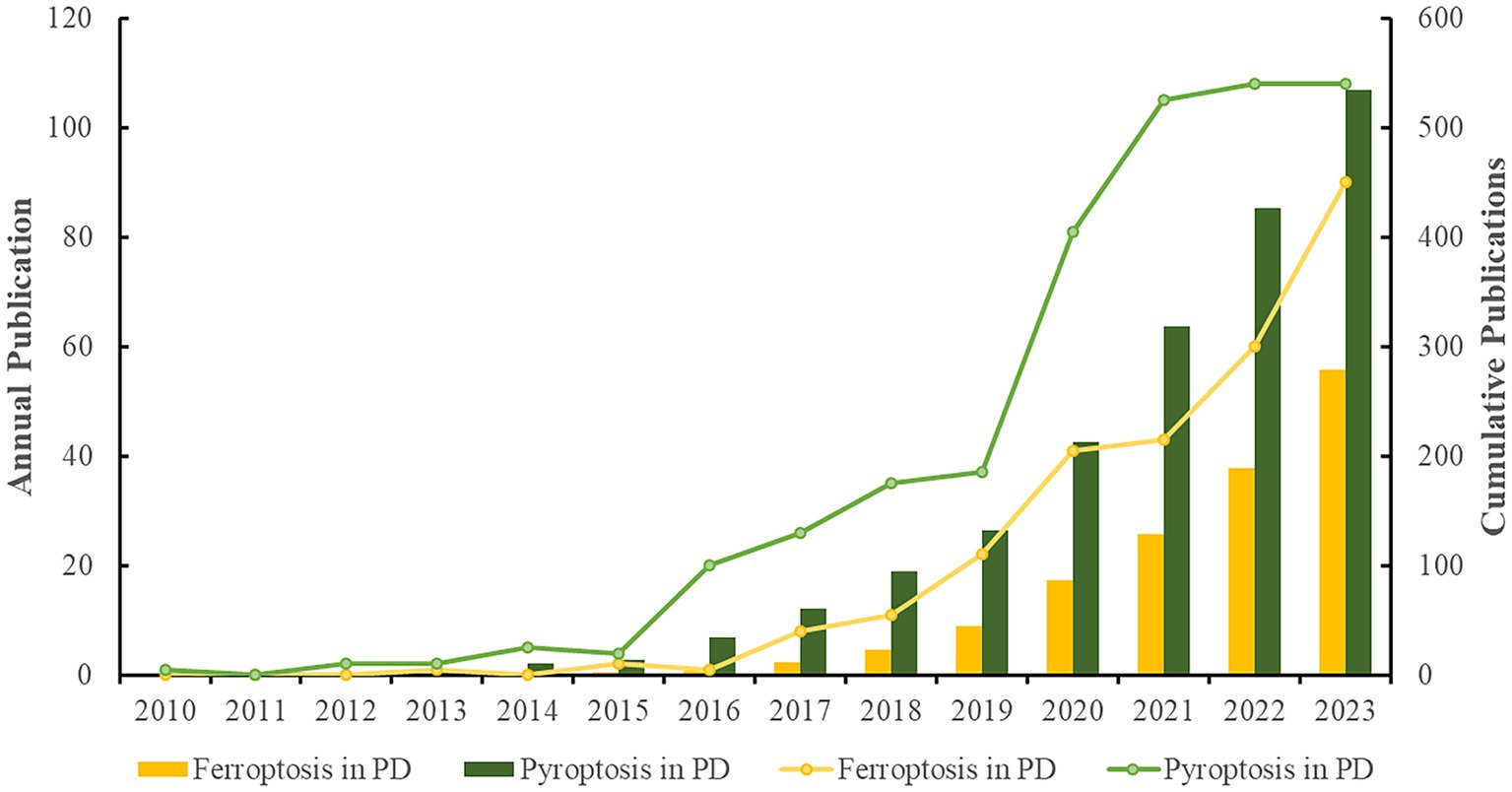
Figure 2. Analysis of annual research output (The line graphs depict the annual publication trends across two distinct domains, while the bar charts illustrate the cumulative volume of publications within these fields).
3.2 Country/region analysis
A total of 43 and 50 countries were involved in the study of “ferroptosis in PD” and “pyroptosis in PD,” respectively. Table 1 summarizes the top 10 productive countries/regions in these two fields. China and the USA were the top two countries in both the number of publications and citations in these two fields, ahead of other countries, indicating their significant contributions. It was interesting to note that Japan, ranked 9th in publication volume, had the highest average citation in the field of “ferroptosis in PD,” with Italy having the highest average number of citations in the field of “pyroptosis in PD,” which may be linked to the higher quality of their articles.
The geographic collaboration network between countries is presented in Figures 3A,B. The size of the nodes indicated the publication outputs, the color of the nodes indicated the cooperation clusters, while the line thickness between two nodes indicated the strength of the cooperation, i.e., the stronger the Total Link Strength (TLS). The top three countries in terms of TLS in these two domains were the USA, China, and England, indicating their active cooperation and exchanges in the field, which promoted the development of this field.
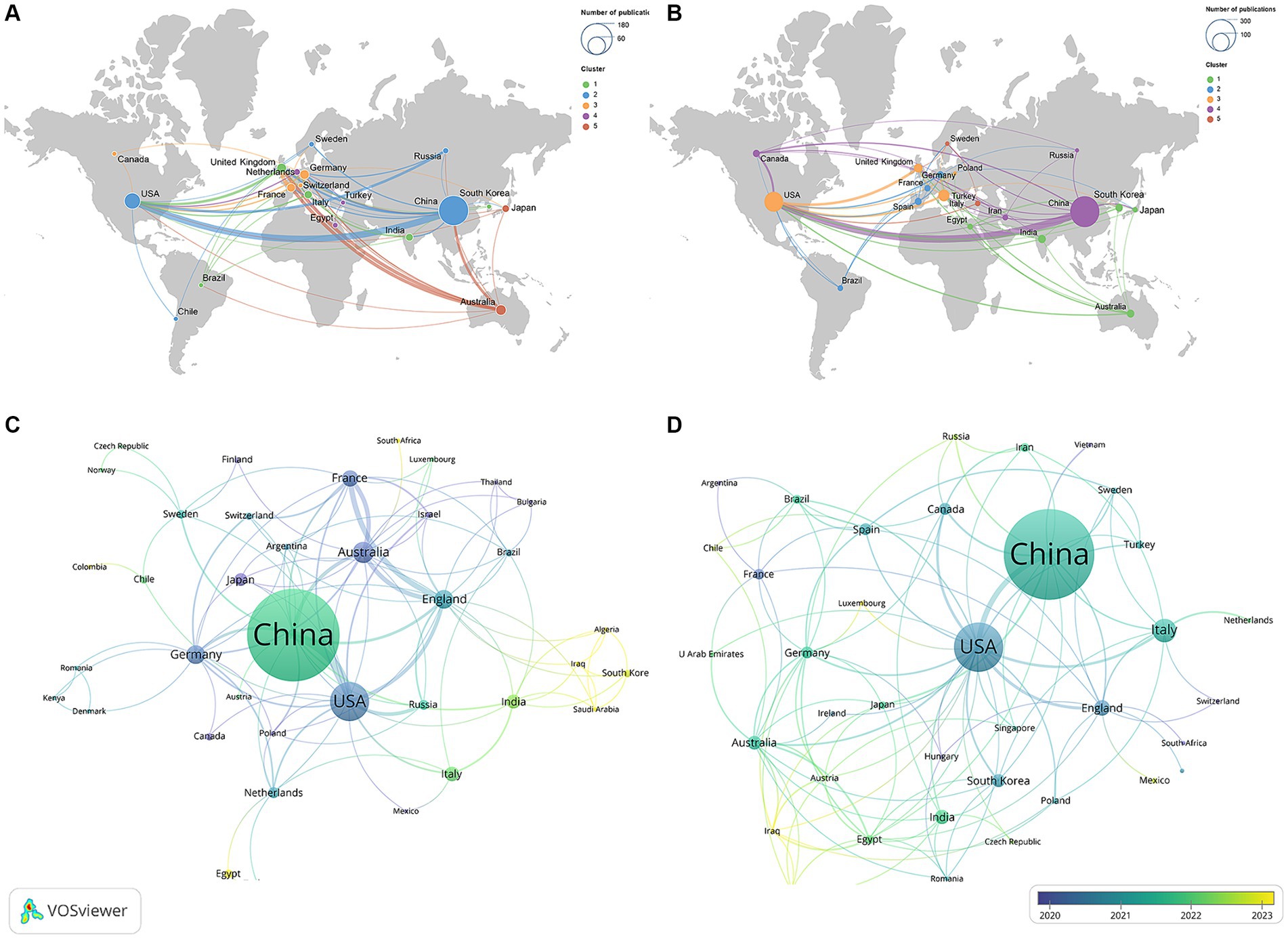
Figure 3. Geographical collaboration map related to (A) “ferroptosis in PD”, (B) “pyroptosis in PD” and time visualization of countries related to (C) “ferroptosis in PD,” (D) “pyroptosis in PD”.
Figures 3C,D show the temporal view of the countries, and the gradient color from purple to yellow represented the freshness of publications. Countries such as Japan, Canada, Poland in the field of “ferroptosis in PD” and Luxembourg, Iraq in the field of “pyroptosis in PD” were marked in a dark purple, indicating that these countries had participated in the research in this field earlier, providing a historical backdrop for current trends. In contrast, countries displayed in a dark yellow, such as Iraq, Algeria, and Saudi Arabia in the field of “ferroptosis in PD” and Switzerland, Vietnam, and Argentina in the field of “pyroptosis in PD,” indicating that these countries recently contributed some new publications, which had contributed to the progress of this research field at this stage.
3.3 Institutional analysis
A total of 474 and 727 institutions participated in research on “ferroptosis in PD” and “pyroptosis in PD” respectively, with the majority being comprehensive universities and medical universities. The 10 institutions with the highest productivity in the two fields are listed in Table 2. Among the top 10 institutions, 6 belonged to China, and the University of Melbourne in Australia ranked first in terms of the number of publications, citation frequency, and TLS in the field of “ferroptosis in PD.” Similarly, in the field of “pyroptosis in PD,” 8 of the top 10 institutions for publications were from China, and Nanjing Medical University of China ranked first in terms of publications, citations, and TLS, hinting at their broad influence in this field.
Figure 4 shows the mapping of the collaboration network among institutions, respectively. Univ Melbourne (TLS = 58), and Nanjing Med Univ (TLS = 38) were the institutions with the strongest TLS for “ferroptosis in PD” and “pyroptosis in PD,” respectively. They were key hubs for promoting international collaboration among related research institutions, with the highest value of centrality. However, there were obvious regional limitations to institutional cooperation. Therefore, for greater breakthroughs in two fields, it is highly advised that regional and disciplinary barriers should be broken down and cooperation and exchanges should be conducted between all research institutions.
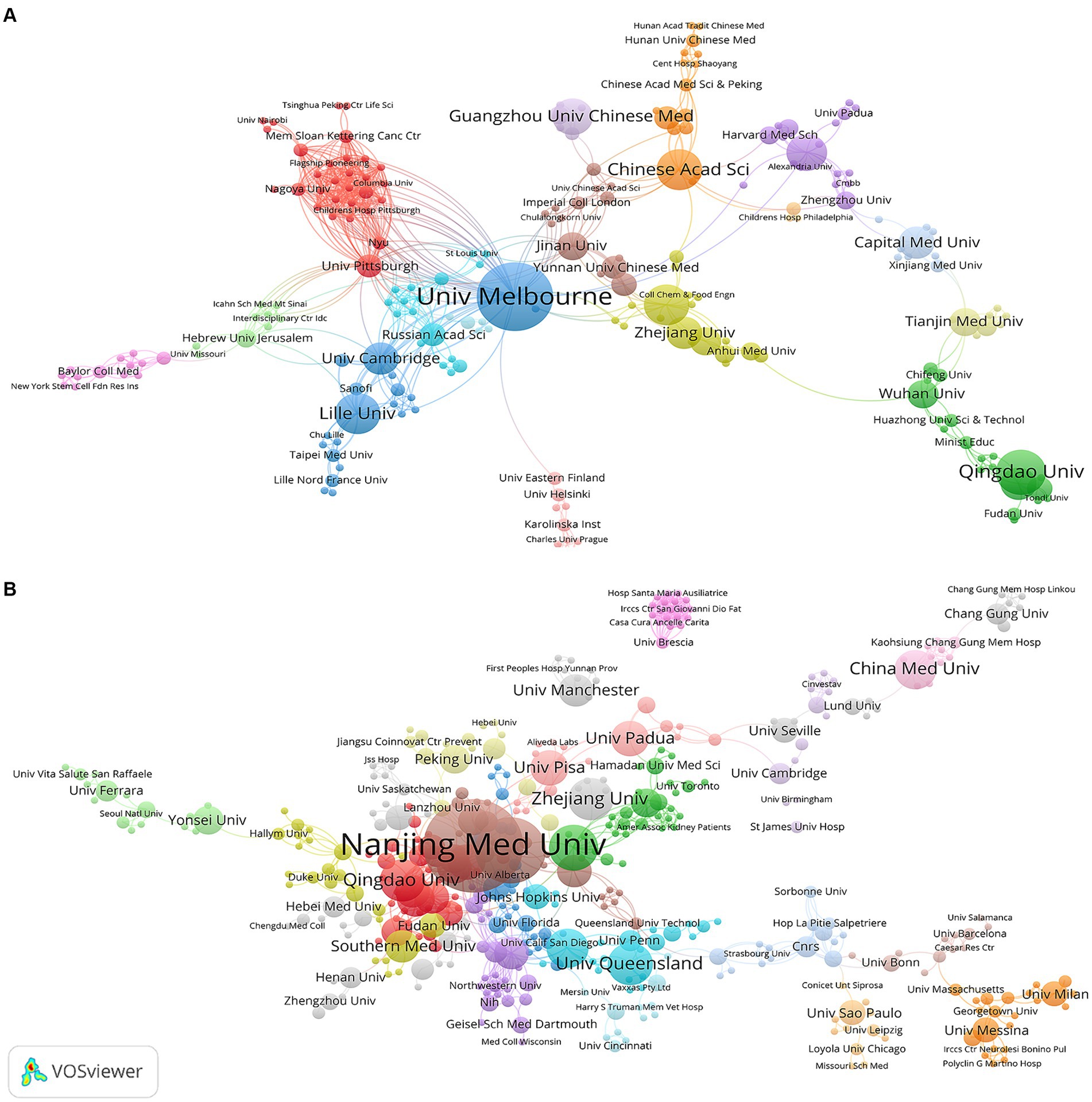
Figure 4. Visualization map of institutions related to (A) “ferroptosis in PD”, (B) “pyroptosis in PD”.
3.4 Authors and co-cited authors analysis
A total of 1,640 and 3,024 authors wrote articles on “ferroptosis in PD” and “pyroptosis in PD,” respectively. The top 10 most published and co-cited authors in the two fields are listed in Table 3. David Devos ranked first in the field of “ferroptosis in PD with 8 publications, followed by Meiling Zhu (n = 7) and Dongfeng Chen (n = 6). In the field of “pyroptosis in PD,” Gang Hu (n = 16), Ming Lu (n = 16), Anumantha G Kanthasamy (n = 9) ranked in the top 3, with more than 50% of the top 10 authors in both fields being from China, demonstrating a similar trend with the distribution of leading institutions. The above statistics underscored China’s prominent role in disseminating related research in the fields.
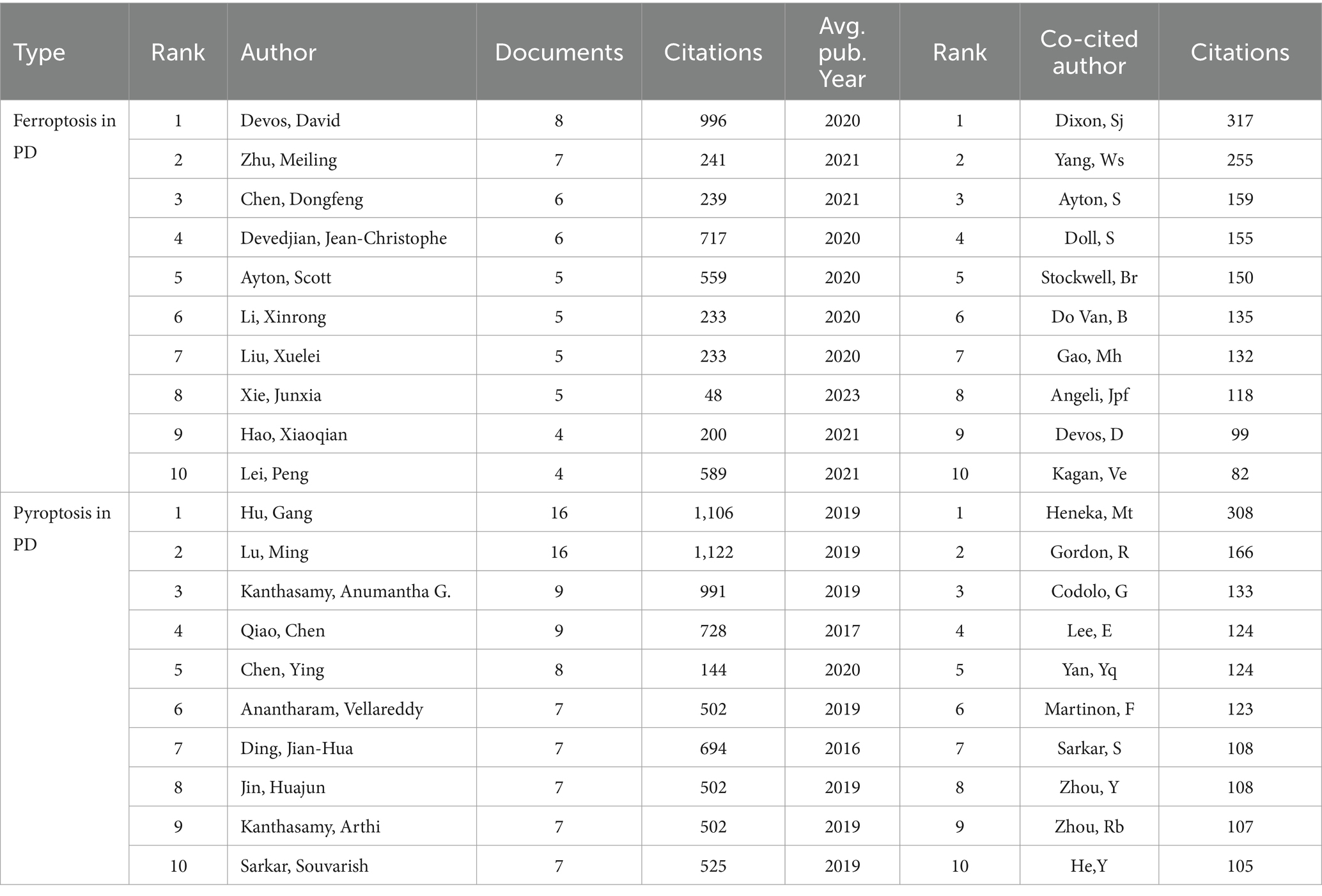
Table 3. Top 10 authors and co-cited authors related to ferroptosis, pyroptosis in Parkinson’s disease.
Figures 5A,B describe the collaborative networks of the authors of “ferroptosis in PD” and “pyroptosis in PD,” respectively. The different colors represented collaboration clusters of authors, and the lines between nodes indicated the strength of their cooperation. Among these clusters, main partnerships and outstanding researchers could be discovered. In the field of “ferroptosis in PD,” the three most significant cooperation clusters were identified, namely red, green, and blue clusters. The green clusters included Meiling Zhu, Dongfeng Chen, Xuelei Liu and Xinrong Li. In the field of “pyroptosis in PD,” three essential collaborative clusters had been formed, led by Gang Hu, Anumantha G Kanthasamy and Ying Chen, respectively, who were also among the top in publication volume, indicating that the main research authors had formed a collaborative network. However, most of the collaborations between authors were within national boundaries, suggesting that international exchange and collaboration among authors from various countries should be strengthened to produce higher-quality academic outcomes in the field.
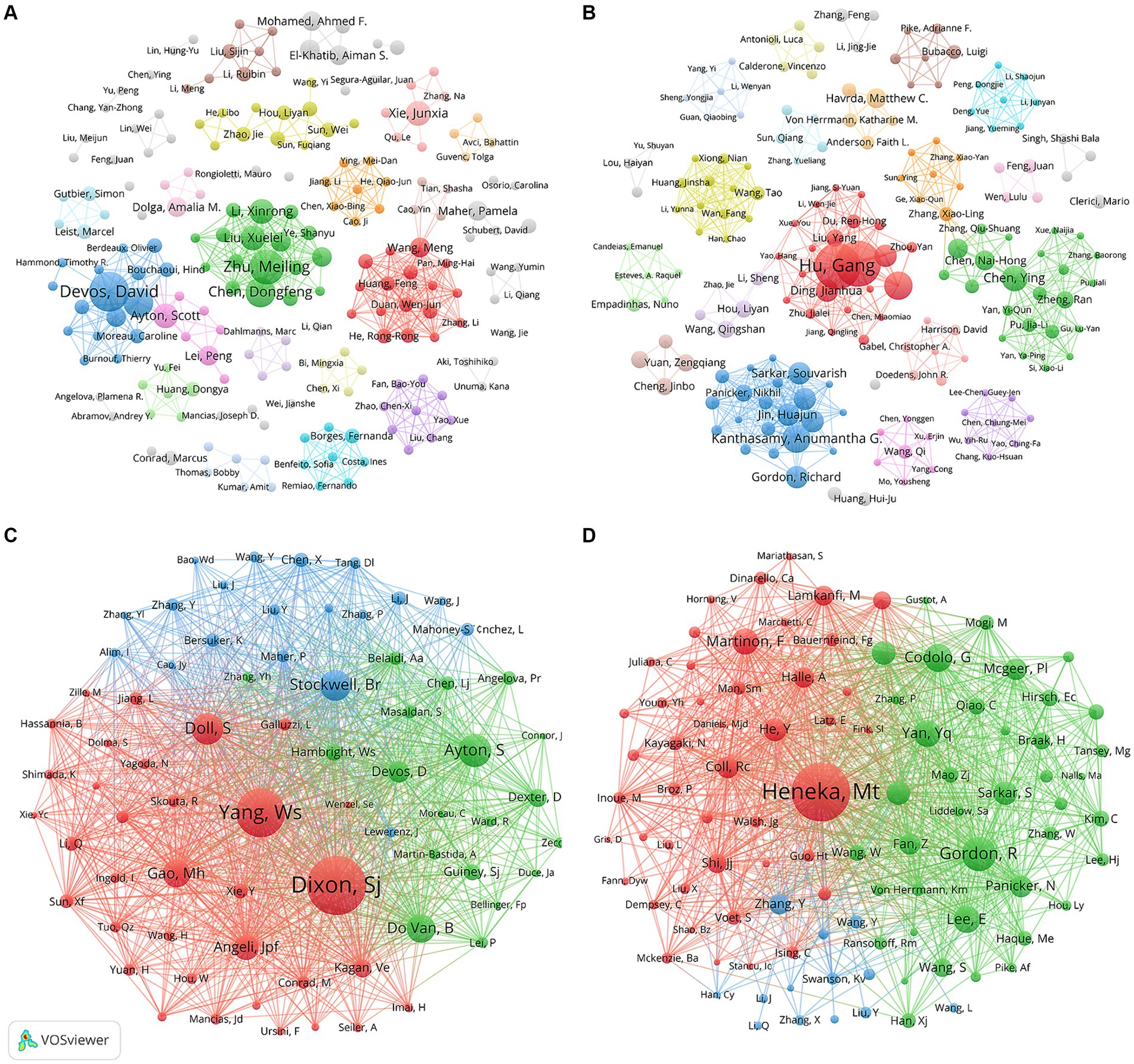
Figure 5. Visualization map of co-authorship related to (A) “ferroptosis in PD”, (B) “pyroptosis in PD” and co-cited authorship related to (C) “ferroptosis in PD”, (D) “pyroptosis in PD”.
A total of 16,486 and 27,946 co-cited authors contributed to the publication of “ferroptosis in PD” and “pyroptosis in PD,” respectively. Scott J Dixon had been co-cited 317 times, ranked the first in the field of “ferroptosis in PD,” followed by Wan Seok Yang (255 citations) and Scott Ayton (159 citations) (Figure 5C). Simultaneously, two authors, Scott Ayton and David Devos were among the top 10 in both publication volume and co-citation frequency, highlighting their significant role and influence as core leaders in this domain. In the area of “pyroptosis in PD,” the top three authors in terms of co-citations were Michael Heneka (308 citations), followed by Richard Gordon (166 citations) and Gaia Codolo (133 citations) (Figure 5D).
3.5 Journals and co-cited journals analysis
A total of 158 and 241 academic journals published articles in the fields of “ferroptosis in PD” and “pyroptosis in PD.” The top 10 most published and co-cited journals in the two fields are listed in Supplementary Table S1. Free radical biology and medicine (n = 14, IF = 7.4) and International journal of molecular sciences (n = 23, IF = 5.6) ranked first in publications on “ferroptosis in PD” and “pyroptosis in PD” (Figures 6A,B). Meanwhile, co-citation analysis revealed that the journals with the highest co-citations were Cell (n = 829) and Nature (n = 1,651) in the fields of “ferroptosis in PD” and “pyroptosis in PD,” respectively. (Figures 6C,D), indicating the high academic influence of articles published by the journals. Among the top 10 journals in terms of number of publications and co-citation frequency, most of the journals were Q1 journals, with Nature having the highest IF of 64.8. The relevance between high IFs and citation frequency emphasizes the significance of these journals in advancing the development of this topic.
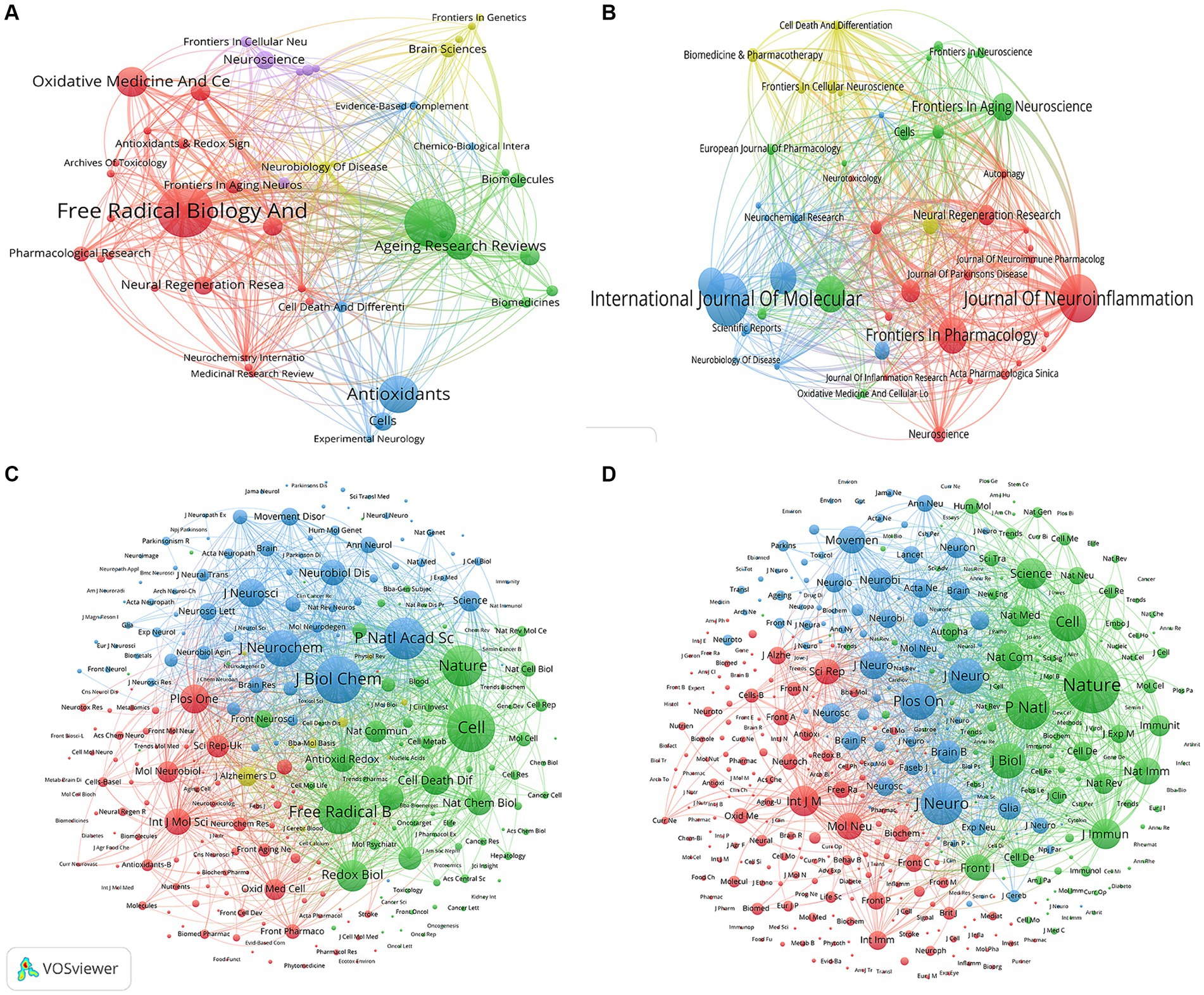
Figure 6. Visualization map of journals related to (A) “ferroptosis in PD”, (B) “pyroptosis in PD” and co-cited journals related to (C) “ferroptosis in PD”, (D) “pyroptosis in PD”.
The dual-map overlay analysis of journals (Figure 7) was made by Citespace, showing the distribution of different journal themes, depicting the various research areas covered by all journals, with colored paths representing citation relationships, and clusterings of the citing journals on the left and cited journals on the right. A primary orange citation path was identified, demonstrating that articles in the Molecular, Biology and Genetics journals for the studies “ferroptosis in PD” and “pyroptosis in PD” were mainly cited by articles in the Molecular, Biology and Immunology journals. This implies that research in these two areas requires interdisciplinary collaboration.
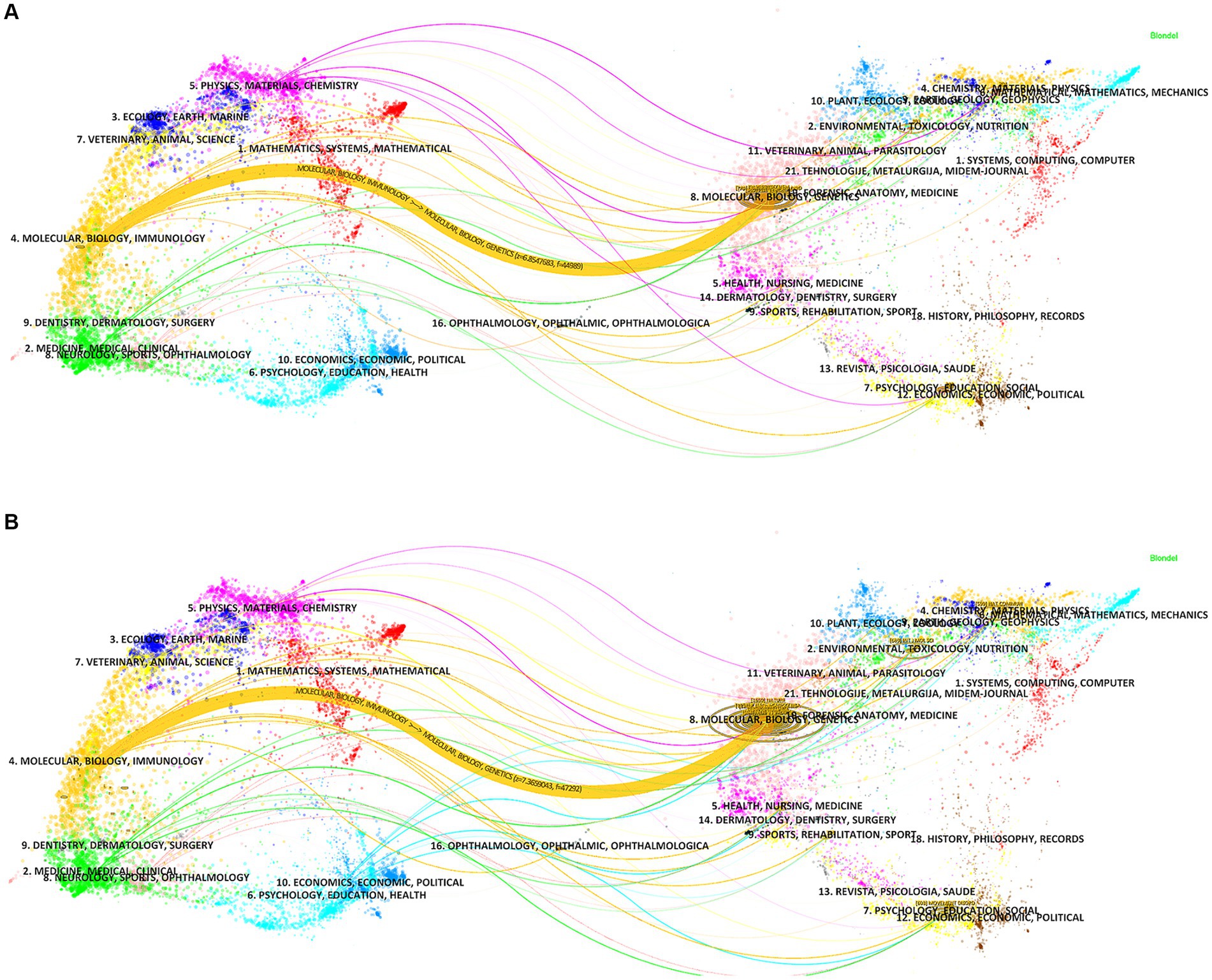
Figure 7. The dual-map overlay of journals related to (A) “ferroptosis in PD”, (B) “pyroptosis in PD”.
3.6 Co-cited references analysis
Co-cited references are two or more references that are simultaneously cited by another paper, forming a co-citation relationship. Supplementary Table S2 displays the details of the top 10 most frequently co-cited references in the two fields. As shown in Figure 8A, Scott J Dixon’s article “Ferroptosis: an iron-dependent form of nonapoptotic cell death” (Dixon et al., 2012) published in Cell was the most co-cited reference in the field of “ferroptosis in PD,” which named and established ferroptosis as a novel modality of regulated cell death, a seminal discovery that had a major impact on subsequent research in this field. Following this is Bruce Do Van’s article “Ferroptosis, a newly characterized form of cell death in Parkinson’s disease that is regulated by PKC” (Do Van et al., 2016) published in Neurobiology of Disease, highlighting the role of ferroptosis in PD, revealing its activation through a PKCα-mediated, RAS-independent mechanism and iron chelators, Fer-1, and PKC inhibitors prevent neuronal loss in PD patients, providing new insights into PD treatment. As shown in Figure 8B, Richard Gordon’s article “Inflammasome inhibition prevents α-synuclein pathology and dopaminergic neurodegeneration in mice” (Gordon et al., 2018) was the most frequently cited article in the field of “pyroptosis in PD,” revealing that NLRP3 in microglia may persistently cause neuroinflammation to drive progressive DA neurons loss, and emphasizing NLRP3 as a potential therapeutic target in PD, opening a new window for PD research. Predominantly, these key articles were published in reputable journals such as Cell and Nature, with Cell particularly including three articles of the top 10 in the area of “ferroptosis in PD.” These publications covered a range of themes related to ferroptosis in PD, including regulation, molecular mechanisms, targeted inhibition and PD therapy.
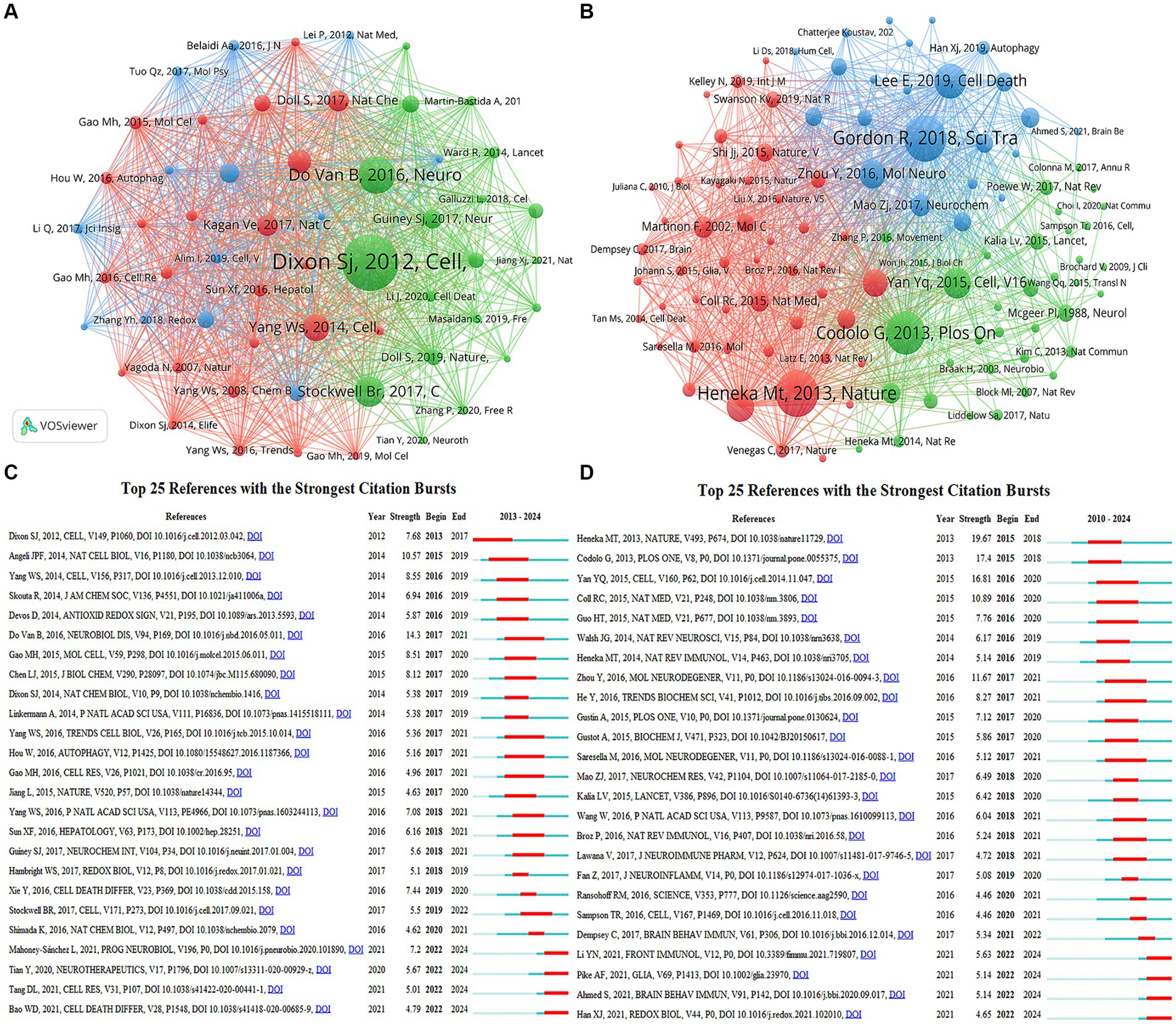
Figure 8. Visualization of co-citation references related to (A) “ferroptosis in PD”, (B) “pyroptosis in PD”. The top 25 references with the strongest citation bursts in researches related to (C) “ferroptosis in PD”, (D) “pyroptosis in PD”.
Emerging references are those whose citation frequency suddenly increases significantly within a short period, indicating research hotspots and frontier trends at that time. Figures 8C,D show the top 25 references with the strongest citation bursts in the two fields, respectively. In “ferroptosis in PD,” Bruce Do Van published “Ferroptosis, a newly characterized form of cell death in Parkinson’s disease that is regulated by PKC” (Do Van et al., 2016) had the highest burst strength of 14.3, with an burst duration of 2017–2021. Four references continued to exhibit citation bursts until 2024, describing the morphological features, regulatory mechanisms, and signaling pathways of ferroptosis, its inducers and inhibitors, and its role in diseases. In “pyroptosis in PD,” “NLRP3 is activated in Alzheimer’s disease and contributes to pathology in APP/PS1 mice” (Heneka et al., 2013) had the highest burst strength of 19.67, with a burst period of 2015–2018. Four references continued to exhibit citation bursts until 2024, describing the specific roles and interactions between GSDM family members and the family of inflammatory caspases in the process of pyroptosis, further elucidating the relationship between pyroptosis and PD, and providing new strategies for research into pyroptosis-mediated PD.
3.7 Keyword analysis
Keywords represent a high-level summary and refinement of article content, through keyword co-occurrence, it is possible to understand the hotspot and development trend of the current research field. Table 4 shows the basic information of the top 15 most frequent keywords in the two fields. It can be seen from Figure 9A that the top 5 keywords with a high occurrence frequency of “ferroptosis in PD” were “ferroptosis” “Parkinson’s disease” “oxidative stress” “cell death” and “iron.” From the results, indicating research focused on diseases like “Parkinson’s disease” “stroke” “Alzheimer’s disease” and “cancer”; pathological mechanisms including “oxidative stress” “lipid peroxidation” “glutamate” “ferroptosis” “autophagy” “apoptosis” “pyroptosis” and “reactive oxygen species”; drug research represented by “vitamin e” “acetylcysteine” and “quercetin”; and signaling pathways focusing on “Nrf2/GPX4” and “cystathionine-glutamate transporter” (Xct). As shown in Figure 9B, the keywords appearing at a high frequency of “pyroptosis in PD” were “Parkinson’s disease” “pyroptosis” “nlrp3 inflammasome” “microglia” and “Alzheimer’s-disease.” From the results, we can see that research focused on diseases like “Parkinson’s disease” “Alzheimer’s disease” and “traumatic brain injury”; pathological mechanisms including “cell death” “pyroptosis” “ferroptosis” “autophagy” “apoptosis” “pyroptosis” “microglia” “nlrp3 inflammasome” and “α-synuclein”; and signaling pathways focusing on “NF-kappa-B” “NLRP3” “interleukin-1” and “caspase-1.”
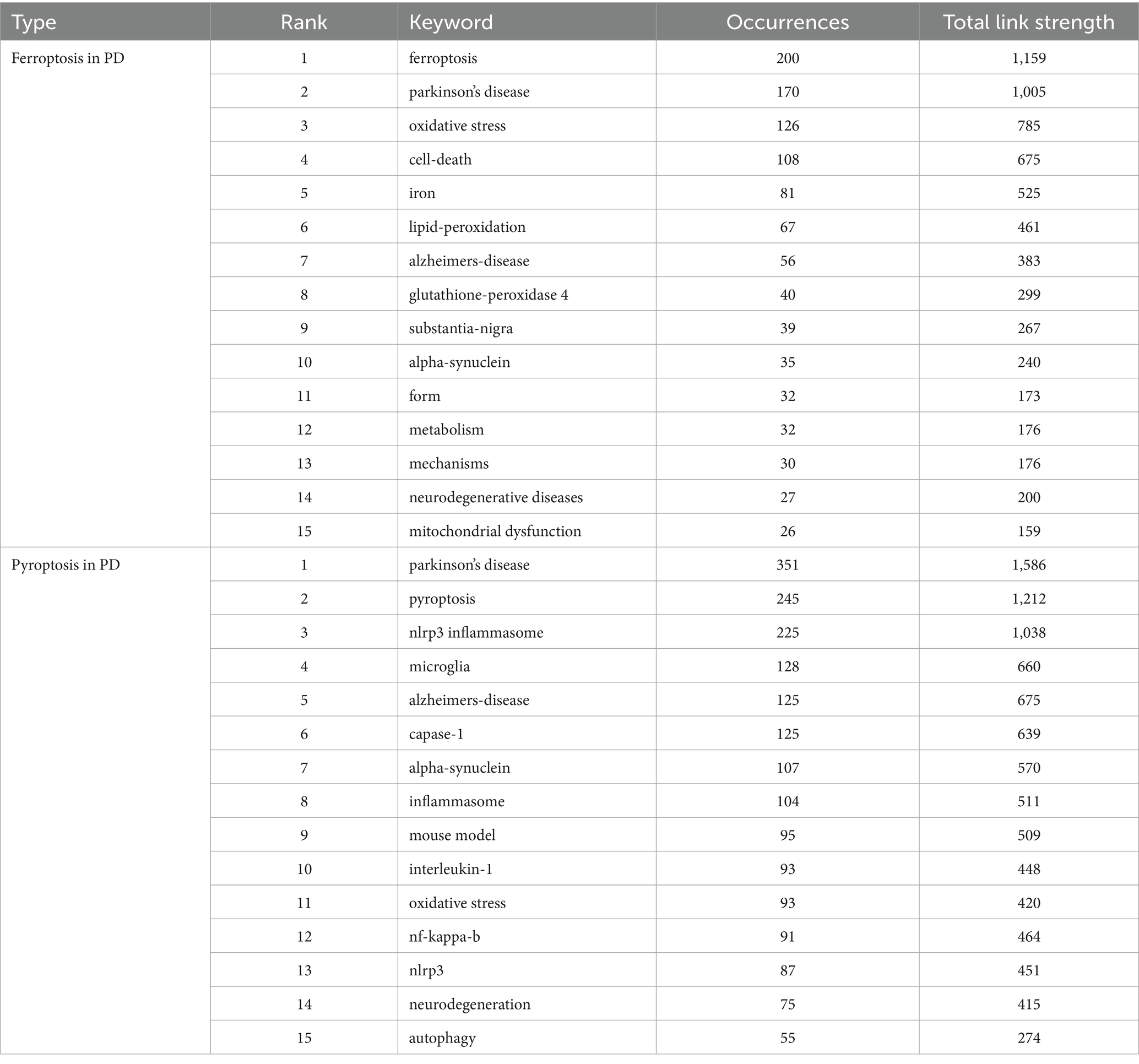
Table 4. Top 15 occurrences keywords associated with ferroptosis, pyroptosis in Parkinson’s disease.
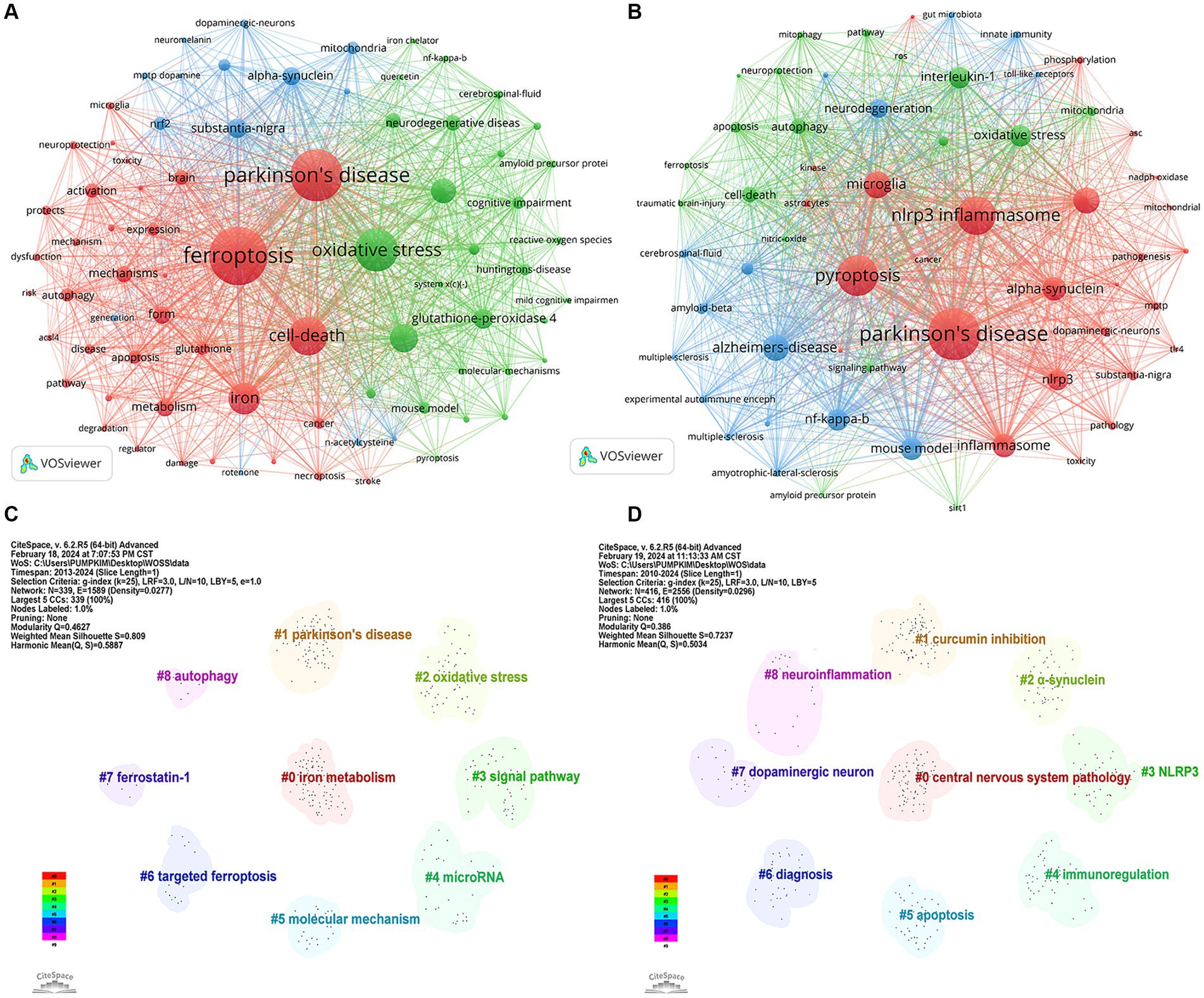
Figure 9. Visualization of keywords related to (A) “ferroptosis in PD”, (B) “pyroptosis in PD” and keyword clusters related to (C) “ferroptosis in PD”, (D) “pyroptosis in PD”.
Based on the keyword co-occurrence analysis, the keywords with similar features were clustered, reflecting the basic knowledge structure of the related research fields. In CiteSpace, the modularity (Q) value and silhouette (S) value, are calculated based on the network structure and the clarity of the clustering. Generally, Q > 0.3 indicates a significant clustering structure; when S > 0.5, the clustering is deemed reasonable, and when S > 0.7, the clustering is considered credible. Figure 9C identified nine relevant clusters of “ferroptosis in PD”, ranging from #0 (iron metabolism) to #8 (autophagy), as delineated by CiteSpace, with a Q value of 0.4627 and an S value of 0.809, reinforcing remarkable network homogeneity and clustering structure. Among them, #0 iron metabolism, #5 molecular mechanism, #3 signal pathway, #2 oxidative stress, and #4 microRNA mainly explored the regulatory mechanism of ferroptosis in PD. #1 Parkinson’s disease was the exploration of related diseases. #7 ferrostatin-1 and #6 targeted ferroptosis explored therapeutic strategies. #8 autophagy explored other forms of cell death
As shown in Figure 9D, the keywords of “pyroptosis in PD” were clustered into 9 clusters, ranging from #0 (CNS diseases) to #8 (neuroinflammation), with a Q value of 0.386 and an S value of 0.7237, indicating distinct research themes and convincing clustering. Among them, #2 α-synuclein, #3 NLRP3, #7 dopaminergic neuron, and #8 neuroinflammation mainly explored the regulatory mechanism of pyroptosis in PD. #0 central nervous system pathology focused on related disorders. #1 curcumin inhibition, #6 diagnosis explored the diagnostic modality and treatment in traditional Chinese medicine. #5 apoptosis explored other forms of cell death.
The keyword time zone map is used to identify research themes from various periods to analyze the evolutionary lineage and predict the direction of development. Figure 10A shows that in the field of “ferroptosis in PD,” the earliest appearing keywords were “oxidative stress,” “mouse model,” and “nitric oxide,” which were the basis of the research in this field and provide historical background for the current research trends. In 2023, research mainly focused on “molecular mechanisms,” “neurons,” “neuroinflammation” and “generation,” which may be the current hotspots in the field. The research already touched upon in 2024 included “ferritin,” “Ca2+ channels,” and “forsythia fructus.” As shown in Figure 10B, in the area of “pyroptosis in PD,” the earliest appearing keywords included “Alzheimer’s disease,” “NF kappa B,” and “amyotrophic lateral sclerosis.” The research in 2023 focused on “receptor 2,” “mesenchymal stem cell,” and “delivery,” marking them as hotspots in the field. The research mentioned for 2024 included “cholesterol,” “attenuates neuroinflammation,” and “cellular senescence.” The research involved in 2024 cannot conclusively prove to be a trend or hotspot, with insufficient data resulting from the early cutoff of literature retrieval.
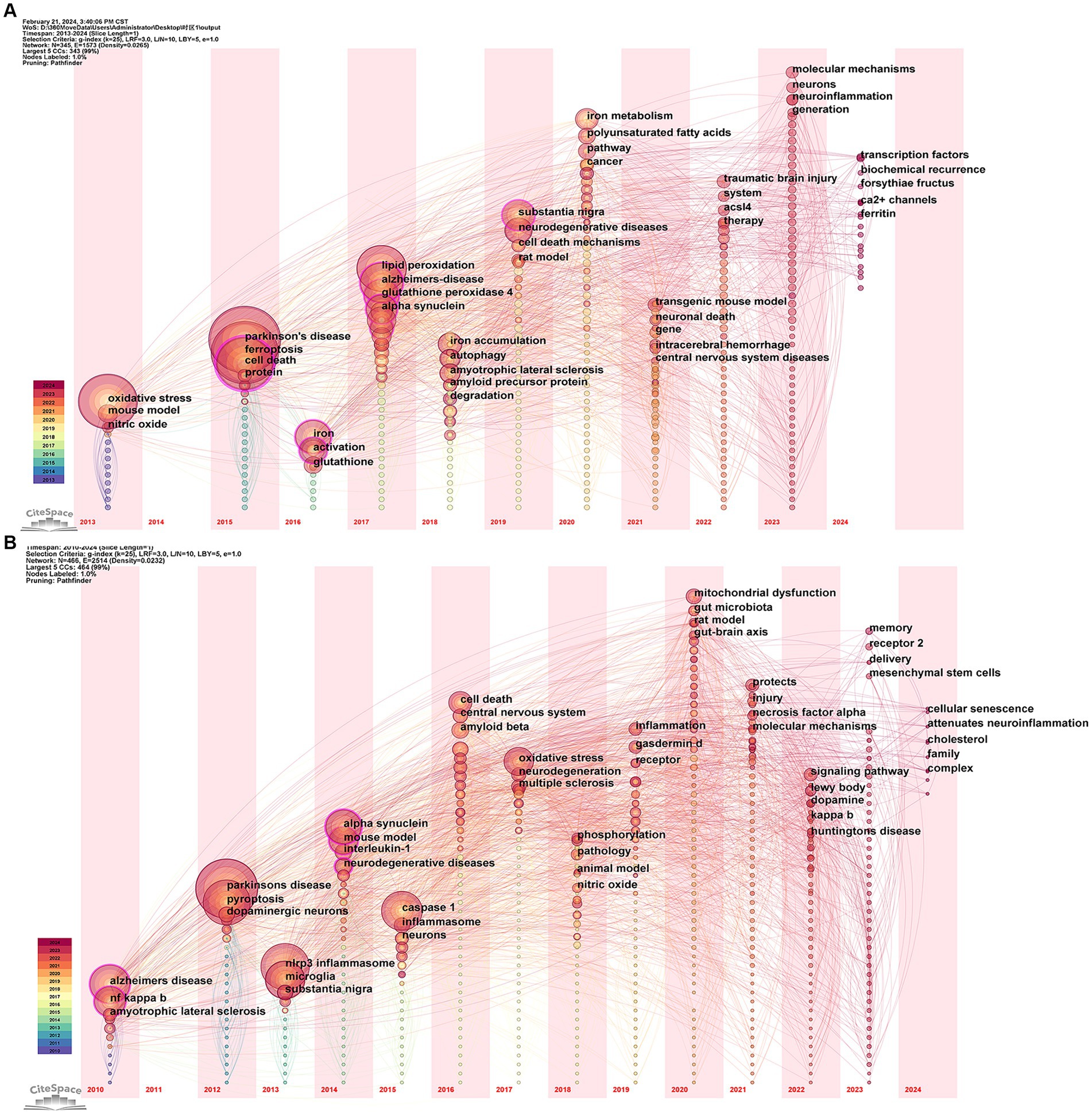
Figure 10. Visualization of the keyword time zone related to (A) “ferroptosis in PD”, (B) “pyroptosis in PD”.
4 Discussion
4.1 General information
The study used bibliometric tools to analyze the literature in the fields of “ferroptosis in PD” and “pyroptosis in PD.” These fields are found to be on the rise, especially “ferroptosis in PD,” which has become a hot research topic in recent years and may become a future research trend.
In the analysis of countries/regions and institutions, China and the United States ranked the top two in the number of publications in the fields of “ferroptosis in PD” and “pyroptosis in PD,” which were far ahead of other countries/regions, highlighting the leading position of these two countries in the above research fields. Moreover, more than 60% of the top 10 institutions in both fields were from China, demonstrating that the above fields had attracted widespread attention in China, and the University of Melbourne and Nanjing Medical University were the leaders in terms of the number of documents, citation frequency and centrality of “ferroptosis in PD” and “pyroptosis in PD,” respectively. However, a dispersed layout among some countries and institutions were evident, with international collaborations being notably less common compared to domestic ones. Thus, constructing a robust network of cooperation represents a key challenge for further advancements in the two fields.
In the analysis of authors and co-cited authors, in the field of “ferroptosis in PD,” David Devos was the most prolific author, with a notable collaboration among high-publishing authors from Guangdong University of Traditional Chinese Medicine such as Meiling Zhu, Dongfeng Chen, Xuelei Liu, and Xinrong Li. For example, they discovered in 2021 that miR-335 decreases FTH1 level leading to ferroptosis in PD model, providing new ideas and potential for the study of pharmacological targets of PD (Li X. R. et al., 2021). Scott J Dixon had the most co-cited frequency, whose 2012 article in Cell “Ferroptosis: an iron-dependent form of nonapoptotic cell death” distinguished ferroptosis from traditional apoptosis and necrosis and autophagy, identifying ferrostatin-1 as a potent ferroptosis inhibitor (Dixon et al., 2012). In the field of “pyroptosis in PD,” Gang Hu was the most published author, with close collaborations with other high-publication authors such as Ming Lu, Chen Qiao, and Jianhua Ding, demonstrating that Nlrp3 is a target gene of microRNA-7 (miR-7) (Zhou et al., 2016). Michael Heneka, with the highest co-citation frequency, had made important contributions to the mechanism of pyroptosis and the clinical application of PD therapy. The co-citation frequency of these people reflected their significant influence and recognition in the scientific community.
In the analysis of journals and co-cited journals, Free radical biology and medicine had the highest number of publications in the field of “ferroptosis in PD,” while Cell had the highest co-citation frequency. In the field of “pyroptosis in PD,” International journal of molecular sciences led in publication count with Nature having the highest number of co-citations. Most of these journals were in the Q1 JCR segment, especially the top journals such as Nature (IF = 64.8) and Cell (IF = 64.5), indicating the participation of numerous high-quality journals in this field, thus suggesting a promising research prospect. Overall, the findings will help researchers to quickly and accurately find appropriate journals for the latest advances in the above-mentioned fields.
In the analysis of co-cited references, “Ferroptosis: an iron-dependent form of nonapoptotic cell death” and “Inflammasome inhibition prevents α-synuclein pathology and dopaminergic neurodegeneration in mice” were the most cited articles in the analysis of “ferroptosis in PD” and “pyroptosis in PD,” respectively. Overall, the top 10 most co-cited references in both fields provided a comprehensive overview of ferroptosis and pyroptosis, including their mechanisms, cellular pathways, and disease relevance, forming a strong evidence base that advances the understanding and potentially shifts disease treatment strategies by targeting ferroptosis and pyroptosis.
4.2 Research hotspots and frontiers
Keywords are highly condensed and summarized for the themes and core research content of a paper, which can reflect the distribution and development of different research hotspots in a specific field. Through the visualization and analysis of keywords, keyword clustering and time-zone maps, the research hotspots and development frontiers of “ferroptosis in PD” and “pyroptosis in PD” have been identified in this study, which are summarized as follows:
4.2.1 Ferroptosis in PD
Ferroptosis is a novel mode of programmed cell death that is iron-dependent and closely related to lipid peroxidation, involving multiple molecular and metabolic pathways. Iron accumulation is a critical factor in ferroptosis. Increased iron intake, decreased efflux, or decreased storage can lead to iron overload. Excess Fe2+ generates large amounts of ROS via the Fenton reaction, exacerbating oxidative damage and providing oxidizing-capable free radicals that hydroxylate intracellular polyunsaturated fatty acids (PUFAs), generating large amounts of lipid hydroperoxides (LOOHs). These damage DNA, lipid membranes, and other biomolecules, which in turn leads to cell death (Jiang et al., 2021). The genesis of lipid peroxides can occur both through iron ion catalysis and enzymatic reactions, the latter involving key enzymes such as acyl-CoA synthetase long-chain family members (ACSLs), lysophosphatidylcholine acyltransferase 3 (LPCAT3), and arachidonate 15-lipoxygenase (ALOX15) (Bouchaoui et al., 2023). These toxic lipid peroxides can be reduced to lipid alcohols (L-OH) by GSH and GPX4, and the system Xc− is a key regulator of GSH synthesis, and solute carrier family 3 member 2 (SLC3A2) and solute carrier family 7 member 11 (SLC7A11) are major components of this transporter. System Xc− and GPX4 are therefore important targets for the regulation of ferroptosis, and when their metabolism is imbalanced, lipid peroxidation is unchecked, leading to ferroptosis (Dar et al., 2023).
In recent years, a series of studies have confirmed that ferroptosis is associated with PD. Significant increases in iron ion levels are often observed in the substantia nigra region of the brain in patients with PD. Furthermore, regulatory proteins involved in iron metabolism, including iron-regulated protein (IRP)/iron response element (IRE) (Lee and Hyun, 2023), transferrin(Tf)/transferrin receptor(TfR), and ceruloplasmin (CP) (Liu L. et al., 2023) are abnormally expressed or dysfunctional in PD patients. In a mouse model of PD, accumulation of L-OOH, a marker of ferroptosis, and a decrease in GPX4 are found to increase the toxicity of dopaminergic neurons, leading to motor dysfunction in mice (Sun et al., 2023). Increased concentrations of malondialdehyde (MDA), a lipid peroxidation byproduct, and 4-hydroxy-2,3-trans-nonenal (4-HNE), the end-product of ferroptosis, were found in an MPTP-induced rat model of PD, with 4-HNE mediating the inflammatory response through the promotion of Lewy bodies formation in PD (Liu et al., 2021). Neuroinflammatory responses are also closely associated with ferroptosis in PD, as evidenced by the excessive activation of microglia in the brains of PD patients (Guo et al., 2021). Iron accumulation causes alterations in microglial transcription, thereby inducing the release of various cytokines and pro-inflammatory substances, such as IL-1β, TNF-α, and IL-6. This process promotes the uptake of iron by neuronal cells, further exacerbating ferroptosis. Recent discoveries have revealed that the regulatory mechanisms of ferroptosis in PD are also closely related to the p53/SLC7A11/GPX4 and Nrf2-related pathways. p53 activation can downregulate Xc− by inhibiting SLC7A11, these actions further reduces the expression of GSH and GPX4, leading to DN loss and motor deficits in the PD mouse model.(Li S. S. et al., 2021). Nrf2 can enhance the resistance of DA to ferroptosis by regulating iron-homeostasis-related proteins (e.g., IRP), heme metabolism, mitochondrial function and by up-regulating the transcription of GPX protein family (Shen et al., 2024). The evidence mentioned above suggests that ferroptosis is one of the key pathological mechanisms of neuronal injury and dysfunction after PD, making it an important target for intervention.
Currently, therapeutic studies targeting ferroptosis in PD have focused on the use of drugs such as iron chelators, antioxidants, and autophagy activators. For instance, iron chelators like deferoxamine have been shown to reduce iron deposition in SN of PD patients, thereby mitigating the progression of motor disorders (Negida et al., 2024). Antioxidants targeting ferroptosis, including N-Acetylcysteine (NAC), Vitamin E, Coenzyme Q10, ACSL4 inhibitors, LOX inhibitors, GPX4 activators, and Nrf2 activators, have also shown potential in slowing PD progression in the study. For example, the probiotic L. lactis MG1363-pMG36e-GLP-1 can inhibit ferroptosis in brain tissues of PD mice by activating the Keap1-Nrf2-GPX4 signaling pathway and decreasing the level of oxidative stress, thus improving PD symptoms (Yue et al., 2022). Rapamycin targets ferroptosis in PD by activating the autophagy pathway, reducing the accumulation of iron and attenuating lipid peroxidation, offering various neuroprotective effects on PD (Liu T. et al., 2023). In recent years, the treatment of PD with traditional Chinese medicine (TCM) has attracted more and more attention, both herbal components and acupuncture have shown good potential for anti-PD. Forsythia suspense can enhance antioxidant capacity by activating the Nrf2/GPX4 axis and inhibiting the TLR4 signaling pathway, to modulate ferroptosis-mediated inflammatory response, protect dopamine neurons, and alleviate PD (Zhang et al., 2024). In the 6-OHDA-induced PD rat model, acupuncture treatment at acupoints such as Baihui (GU20) and Taichong (LR3) can promote the release of DA and inhibit the production and aggregation of α-syn in the SN, reducing the loss of dopaminergic neurons and improving the motor symptoms in PD rats (Wang et al., 2024). Moreover, acupuncture therapy can also reduce oxidative stress by activating the Nrf2/ARE pathway (Huang and Hsieh, 2021). Moxibustion at Baihui acupoint can increase the expression of GPX4 in the SN of PD model rats, reduce the level of ROS, improve ferroptosis injury, protect dopaminergic neurons, and thus improve their motor disorders (Huang et al., 2021). The above studies suggest that inhibition of ferroptosis is a potentially important intervention for PD, but more direct clinical evidence remains to be explored.
4.2.2 Pyroptosis in PD
In the progression of PD, pyroptosis is closely associated with the release of inflammatory mediators and the excessive activation of neuroglial cells, which together contribute to the neuroinflammatory state characteristic of the disease. Typically, neuronal pyroptosis is triggered by a confluence of intracellular and extracellular factors, including accumulation of neurotoxins, excessive production of ROS by mitochondria, and disruption of mitochondrial autophagy. These factors collectively activate microglia within the CNS, triggering neuroinflammation and leading to a decrease in dopaminergic neurons and aggregation of α-syn proteins (Pike et al., 2021). Once α-syn is released extracellularly, it further activates microglia and NLRP3 inflammasome through the Toll-like receptor/NF-κB pathway, triggering pyroptosis (Li Y. N. et al., 2021). Caspase-1, recruited during pyroptosis, promotes further aggregation of α-syn to generate Lewy bodies with toxic α-syn protofibrils as the main component, and the formation of Lewy bodies further leads to neuronal death and dopamine loss. In addition, caspase-1 catalyzes the maturation and release of IL-1β and IL-18 and pore formation of GSDMD, further damaging dopamine neurons and promoting the progression of PD (Gao et al., 2020). Significantly elevated levels of IL-1β and IL-18, indicative of pyroptosis, have been found in patients with PD (Zhang et al., 2020). Clinically, biomarkers associated with pyroptosis, such as miR-675-5p, miR-1247-5p, and lncH19, can be used for the early diagnosis of PD and therapeutic and prognostic assessment (Liang et al., 2024). In addition to the NLRP3/caspase-1/GSDMD pathway described above, the onset of pyroptosis in PD is also regulated by the TLR4/TAK1/IRF7 and p38-TFEB pathways. TLR4 is an innate immune receptor that is significantly activated in PD. TLR4 can modulate downstream TAK1 expression to upregulate IRF7, which can mediate DA pyroptosis and promote PD by binding to the GSDMD (Quan et al., 2024). In a PD mouse model, P38 inhibits molecular chaperone-mediated autophagy (CMA) by downregulating TFEB, which further inhibits the degradation of NLRP3 inflammasome (Chen J. L. et al., 2021). These two pathways have the opportunity to be potential therapeutic targets for NLRP3 inflammasome -driven PD.
Activation of the NLRP3 inflammasome-mediated pyroptosis is involved in dopamine neuron death, emerging as a therapeutic target for PD. Numerous NLRP3 inhibitors have been found to intervene in PD by inhibiting the activity of related enzymes and the expression of inflammatory factors. Exendin-4 and linagliptin can be used as novel anti-inflammatory agents in PD by reducing ROS production and attenuating neuroinflammation through inhibition of NLRP3-cellular pyroptosis pathway (Yu et al., 2023). MCC950 attenuates the inflammatory response and reduces murine nigrostriatal inflammatory response through inhibition of NLRP3/IL-1β pathway and reduces hyperphosphorylated α-syn aggregates in the mouse substantia nigra striata thereby protecting dopaminergic neurons and ameliorating PD (Cheng et al., 2020). Transplantation of mesenchymal stem cells and their exosomes has shown great potential in the treatment of PD. Transplantation of adipose mesenchymal stem cell-derived exosomes in the substantia nigra resulted in a significant reduction in the levels of NLRP3 and pro-inflammatory cytokines, and inhibited the pyroptosis of dopamine neuron cells, as well as improved motor function (Li Q. et al., 2021). It has been found that quercetin (QC) prevents neuronal damage by inhibiting the NLRP3 pathway (Lin et al., 2022), but factors such as its low solubility and poor stability limit its effectiveness. Zhang et al. (Zhao et al., 2023) developed a carrier-free nanomedicine (NanoQC), which improves the brain delivery of QC, predominantly accumulating in the SN, reducing the loss of dopamine neurons, thus realizing an efficient and precise therapeutic effect. Consequently, nanotechnology combined with targeted drugs or physical modalities are new and highly promising therapeutic strategies for PD.
4.2.3 The association between ferroptosis and pyroptosis
The above studies highlight the critical roles of ferroptosis and pyroptosis in the development of PD, with both being interconnected at multiple levels. In terms of the inflammasome pathway, excessive iron accumulation can trigger NLRP3 inflammasome activation through the CGAS-STING1 pathway, leading to oxidative stress and ferroptosis in cells (Gupta et al., 2023). In addition, the ferroptosis inhibitor ferrostatin-1 can reduce the expression of NLRP3, IL-1β, and caspase-1, while the ferroptosis inducer erastin can drive the activation of inflammasomes in cells (Li M. H. et al., 2021). Therefore, changes in inflammasome activity also accompany alterations in ferroptosis. As mentioned earlier, the activation of the NLRP3 inflammasome is a crucial triggering event in the neuronal cell pyroptosis pathway. Therefore, it is speculated that pyroptosis and ferroptosis have a synergistic effect in the inflammatory response mediated by the inflammasome pathway. In the MAPK signaling pathway, in a neonatal rat model of hypoxic–ischemic injury, the TLR4-p38MAPK pathway is activated, promoting the production of inflammatory cytokines IL-1β and IL-18, while decreasing the expression of SLC7A11 and GPX4, leading to neuroinflammation and ferroptosis (Zhu et al., 2021). Moreover, iron accumulation and excessive lipid peroxidation have been shown to affect the activity of the MAPK signaling pathway. In mouse models treated with pNaktide, the MAPK signaling pathway is inhibited, resulting in reduced cleavage of GSDMD mediated by caspase-1 and decreased expression levels of pyroptosis-related genes, exerting neuroprotective effects (Zong et al., 2023). This indicates that the MAPK pathway not only plays a role in the inflammatory activation of pyroptosis but also has an important relationship with ferroptosis. In terms of the autophagy-related pathway, autophagy can degrade ferritin, thereby increasing iron levels, ultimately leading to the accumulation of ROS and triggering ferroptosis (Yang et al., 2024). Components of the NLRP3 inflammasome (including NLRP3 and ASC) are co-localized with autophagosomes, which can be phagocytosed and degraded by autophagosomes. Therefore, inhibiting the autophagy pathway can enhance NLRP3 inflammasome activation, thereby mediating pyroptosis (Zhao et al., 2021). Autophagy has opposing effects on pyroptosis and ferroptosis, and exploring the balance point is crucial for disease treatment. The shared pathways of ferroptosis and pyroptosis in PD pathogenesis are currently not well understood. In the future, we can focus on studying the common molecular pathways of pyroptosis and ferroptosis, further clarifying the relationship between neuronal damage in PD patients and pyroptosis and ferroptosis. This will provide new directions for the discovery of specific biomarkers and novel targeted therapeutic drugs for PD patients.
5 Limitations
This is the first comprehensive bibliometric analysis of “ferroptosis in PD” and “pyroptosis in PD,” but there are some limitations. First, the search was conducted on February 16, 2024, but the database is continuously updated. Second, the completeness of keyword inclusion in the literature search process may have affected the results due to partial keyword analysis. Third, due to the limitations of the Citespace software, only relevant literature in the WOSCC database was analyzed in this study, and some articles were not included in the WOSCC database and were excluded, resulting in source bias. Finally, the variable quality of the literature selected for this study may reduce the credibility of the overall analysis.
6 Conclusion
The fields of “ferroptosis in PD” and “pyroptosis in PD” involve an increasing number of scholars, organizations, and countries, resulting in a significant number of high-quality publications. Particularly, “ferroptosis in PD” has witnessed a rapid increase in research outputs in recent years and will become a research hotspot in the field of PD in the coming period. Furthermore, elucidating the relationship between ferroptosis and pyroptosis may offer new directions for the treatment of PD.
Data availability statement
The original contributions presented in the study are included in the article/Supplementary material, further inquiries can be directed to the corresponding authors.
Author contributions
ZW: Investigation, Methodology, Writing – original draft, Writing – review & editing. KZ: Investigation, Methodology, Writing – original draft, Writing – review & editing. BT: Funding acquisition, Writing – review & editing. SX: Writing – review & editing, Investigation.
Funding
The author(s) declare that financial support was received for the research, authorship, and/or publication of this article. This work was supported by the Hunan Provincial Health and Family Planning Commission Project [grant number B202303079716], Hunan Provincial Postgraduate Innovation Foundation [grant numbers CX20220822 and QL2023021], and Scientific Research Foundation of the Hunan University of Chinese Medicine [grant numbers S202110541013 and 22JB Z030]. The funding sources had no involvement in the study process.
Conflict of interest
The authors declare that the research was conducted in the absence of any commercial or financial relationships that could be construed as a potential conflict of interest.
Publisher's note
All claims expressed in this article are solely those of the authors and do not necessarily represent those of their affiliated organizations, or those of the publisher, the editors and the reviewers. Any product that may be evaluated in this article, or claim that may be made by its manufacturer, is not guaranteed or endorsed by the publisher.
Supplementary material
The Supplementary material for this article can be found online at: https://www.frontiersin.org/articles/10.3389/fnmol.2024.1400668/full#supplementary-material
References
Bouchaoui, H., Mahoney-Sanchez, L., Garçon, G., Berdeaux, O., Alleman, L. Y., Devos, D., et al. (2023). ACSL4 and the lipoxygenases 15/15B are pivotal for ferroptosis induced by iron and PUFA dyshomeostasis in dopaminergic neurons. Free Radic. Biol. Med. 195, 145–157. doi: 10.1016/j.freeradbiomed.2022.12.086
Chakraborty, S., Kaushik, D. K., Gupta, M., and Basu, A. (2010). Inflammasome signaling at the heart of central nervous system pathology. J. Neurosci. Res. 88, 1615–1631. doi: 10.1002/jnr.22343
Chen, X., Kang, R., Kroemer, G., and Tang, D. L. (2021). Broadening horizons: the role of ferroptosis in cancer. Nat. Rev. Clin. Oncol. 18, 280–296. doi: 10.1038/s41571-020-00462-0
Chen, J. L., Mao, K. M., Yu, H. L., Wen, Y., She, H., Zhang, H., et al. (2021). p38-TFEB pathways promote microglia activation through inhibiting CMA-mediated NLRP3 degradation in Parkinson's disease. J. Neuroinflammation 18:295. doi: 10.1186/s12974-021-02349-y
Cheng, J., Liao, Y., Dong, Y., Hu, H., Yang, N., Kong, X., et al. (2020). Microglial autophagy defect causes parkinson disease-like symptoms by accelerating inflammasome activation in mice. Autophagy 16, 2193–2205. doi: 10.1080/15548627.2020.1719723
Dar, N. J., John, U., Bano, N., Khan, S., and Bhat, S. A. (2023). Oxytosis/Ferroptosis in neurodegeneration: the underlying role of master regulator glutathione peroxidase 4 (GPX4). Mol. Neurobiol. 61, 1507–1526. doi: 10.1007/s12035-023-03646-8
Ding, X. S., Gao, L., Han, Z., Eleuteri, S., Shi, W., Shen, Y., et al. (2023). Ferroptosis in Parkinson's disease: molecular mechanisms and therapeutic potential. Ageing Res. Rev. 91:102077. doi: 10.1016/j.arr.2023.102077
Dixon, S. J., Lemberg, K. M., Lamprecht, M. R., Skouta, R., Zaitsev, E. M., Gleason, C. E., et al. (2012). Ferroptosis: An Iron-dependent form of nonapoptotic cell death. Cell 149, 1060–1072. doi: 10.1016/j.cell.2012.03.042
Do Van, B., Gouel, F., Jonneaux, A., Timmerman, K., Gele, P., Petrault, M., et al. (2016). Ferroptosis, a newly characterized form of cell death in Parkinson's disease that is regulated by PKC. Neurobiol. Dis. 94, 169–178. doi: 10.1016/j.nbd.2016.05.011
Gao, Y., Tu, D. Z., Yang, R., Chu, C. H., Hong, J. S., and Gao, H. M. (2020). Through reducing ROS production, IL-10 suppresses Caspase-1-dependent IL-1β maturation, thereby preventing chronic Neuroinflammation and neurodegeneration. Int. J. Mol. Sci. 21:465. doi: 10.3390/ijms21020465
Gordon, R., Albornoz, E. A., Christie, D. C., Langley, M. R., Kumar, V., Mantovani, S., et al. (2018). Inflammasome inhibition prevents α-synuclein pathology and dopaminergic neurodegeneration in mice. Sci. Transl. Med. 10:aah4066. doi: 10.1126/scitranslmed.aah4066
Guo, J.-J., Yue, F., Song, D.-Y., Bousset, L., Liang, X., Tang, J., et al. (2021). Intranasal administration of α-synuclein preformed fibrils triggers microglial iron deposition in the substantia nigra of Macaca fascicularis. Cell Death Dis. 12:81. doi: 10.1038/s41419-020-03369-x
Gupta, U., Ghosh, S., Wallace, C. T., Shang, P., Xin, Y., Nair, A. P., et al. (2023). Increased LCN2 (lipocalin 2) in the RPE decreases autophagy and activates inflammasome-ferroptosis processes in a mouse model of dry AMD. Autophagy 19, 92–111. doi: 10.1080/15548627.2022.2062887
Heneka, M. T., Kummer, M. P., Stutz, A., Delekate, A., Schwartz, S., Vieira-Saecker, A., et al. (2013). NLRP3 is activated in Alzheimer's disease and contributes to pathology in APP/PS1 mice. Nature 493:674-+. doi: 10.1038/nature11729
Huang, T. I., and Hsieh, C. L. (2021). Effects of acupuncture on oxidative stress amelioration via Nrf2/ARE-related pathways in Alzheimer and Parkinson diseases. Evid. Based Complement. Alternat. Med. 2021, 1–11. doi: 10.1155/2021/6624976
Huang, J. H., and Li, W. W. (2024). Molecular crosstalk between circadian clock and NLRP3 inflammasome signaling in Parkinson's disease. Heliyon 10:e24752. doi: 10.1016/j.heliyon.2024.e24752
Huang, Z. F., Si, W. W., Li, X. R., Ye, S. Y., Liu, X. L., Ji, Y. C., et al. (2021). Moxibustion protects dopaminergic neurons in Parkinson's disease through Antiferroptosis. Evid. Based Complement. Alternat. Med. 2021:6668249. doi: 10.1155/2021/6668249
Huang, Q., Zhao, Y. Q., Wang, Y., Qiao, H. F., Xu, Z. W., Meng, J., et al. (2024). Identification and validation of senescence-related genes in Parkinson's disease. Hum. Gene Ther. 39:201258. doi: 10.1016/j.humgen.2024.201258
Israni, P. D., Yadav, V., and Sasun, A. R. (2024). Effectiveness of neurorehabilitation in improving the functional recovery and quality of life of patients with Parkinson's disease: a case report. Cureus 16:e52021. doi: 10.7759/cureus.52021
Jiang, X., Stockwell, B. R., and Conrad, M. (2021). Ferroptosis: mechanisms, biology and role in disease. Nat. Rev. Mol. Cell Biol. 22, 266–282. doi: 10.1038/s41580-020-00324-8
Lee, J., and Hyun, D.-H. (2023). The interplay between intracellular Iron homeostasis and Neuroinflammation in neurodegenerative diseases. Antioxidants 12:918. doi: 10.3390/antiox12040918
Li, M. H., Shan, G., Kang, M. C., Wu, X. L., Peng, A., Xili, W., et al. (2021). The Ferroptosis-NLRP1 Inflammasome: the vicious cycle of an adverse pregnancy. Front. Cell Dev. Biol. 9:707959. doi: 10.3389/fcell.2021.707959
Li, X. R., Si, W. W., Li, Z., Tian, Y., Liu, X. L., Ye, S. Y., et al. (2021). miR-335 promotes ferroptosis by targeting ferritin heavy chain 1 in in vivo and in vitro models of Parkinson's disease. Int. J. Mol. Med. 47:4894. doi: 10.3892/ijmm.2021.4894
Li, S. S., Wang, M., Wang, Y. L., Guo, Y. T., Tao, X. X., Wang, X. C., et al. (2021). p53-mediated ferroptosis is required for 1-methyl-4-phenylpyridinium-in-duced senescence of PC12 cells. Toxicol. In Vitro 73:105146. doi: 10.1016/j.tiv.2021.105146
Li, Q., Wang, Z. H., Xing, H., Wang, Y., and Guo, Y. (2021). Exosomes derived from miR-188-3p-modified adipose-derived mesenchymal stem cells protect Parkinson's disease. Mol. Ther. Nucleic Acids 23, 1334–1344. doi: 10.1016/j.omtn.2021.01.022
Li, Y. N., Xia, Y., Yin, S. J., Wan, F., Hu, J. J., Kou, L., et al. (2021). Targeting microglial α-Synuclein/TLRs/NF-kappaB/NLRP3 Inflammasome Axis in Parkinson's disease. Front. Immunol. 12:719807. doi: 10.3389/fimmu.2021.719807
Liang, J., Wan, Z., Qian, C., Rasheed, M., Cao, C., Sun, J., et al. (2024). The pyroptosis mediated biomarker pattern: an emerging diagnostic approach for Parkinson's disease. Cell. Mol. Biol. Lett. 29:7. doi: 10.1186/s11658-023-00516-y
Lin, Z. H., Liu, Y., Xue, N. J., Zheng, R., Yan, Y. Q., Wang, Z. X., et al. (2022). Quercetin protects against MPP+/MPTP-induced dopaminergic neuron death in Parkinson's disease by inhibiting Ferroptosis. Oxidative Med. Cell. Longev. 2022, 1–17. doi: 10.1155/2022/7769355
Liu, L., Cui, Y. E., Chang, Y. Z., and Yu, P. (2023). Ferroptosis-related factors in the substantia nigra are associated with Parkinson's disease. Sci. Rep. 13:15365. doi: 10.1038/s41598-023-42574-4
Liu, T., Wang, P., Yin, H., Wang, X., Lv, J., Yuan, J., et al. (2023). Rapamycin reverses ferroptosis by increasing autophagy in MPTP/MPP+-induced models of Parkinson's disease. Neural Regen. Res. 18, 2514–2519. doi: 10.4103/1673-5374.371381
Liu, L., Yang, S. Q., and Wang, H. (2021). α-Lipoic acid alleviates ferroptosis in the MPP+-induced PC12 cells via activating the PI3K/Akt/Nrf2 pathway. Cell Biol. Int. 45, 422–431. doi: 10.1002/cbin.11505
Naoi, M., Maruyama, W., Shamoto-Nagai, M., and Riederer, P. (2024). Toxic interactions between dopamine, α-synuclein, monoamine oxidase, and genes in mitochondria of Parkinson's disease. J. Neural Transm. 9:6. doi: 10.1007/s00702-023-02730-6
Negida, A., Hassan, N. M., Aboeldahab, H., Zain, Y. E., Negida, Y., Cadri, S., et al. (2024). Efficacy of the iron-chelating agent, deferiprone, in patients with Parkinson's disease: a systematic review and meta-analysis. CNS Neurosci. Ther. 30:e14607. doi: 10.1111/cns.14607
Öztürk, O., Kocaman, R., and Kanbach, D. K. (2024). How to design bibliometric research: an overview and a framework proposal. Rev. Manag. Sci. 24:29. doi: 10.1007/s11846-024-00738-0
Pike, A. F., Varanita, T., Herrebout, M. A. C., Plug, B. C., Kole, J., Musters, R. J. P., et al. (2021). α-Synuclein evokes NLRP3 inflammasome-mediated IL-1β secretion from primary human microglia. Glia 69, 1413–1428. doi: 10.1002/glia.23970
Qiu, Z., Zhang, H., Xia, M., Gu, J., Guo, K., Wang, H., et al. (2023). Programmed death of microglia in Alzheimer's disease: autophagy, Ferroptosis, and Pyroptosis. J. Prev. Alzheimers Dis. 10, 95–103. doi: 10.14283/jpad.2023.3
Quan, W., Liu, Y., Li, J., Chen, D. W., Xu, J., Song, J., et al. (2024). Investigating the TLR4/TAK1/IRF7 axis in NLRP3-mediated Pyroptosis in Parkinson's disease. Inflammation 47, 404–420. doi: 10.1007/s10753-023-01918-y
Shen, J., Chen, S. S., Li, X., Wu, L. L., Mao, X., Jiang, J. J., et al. (2024). Salidroside mediated the Nrf2/GPX4 pathway to attenuates Ferroptosis in Parkinson's disease. Neurochem. Res. 49, 1291–1305. doi: 10.1007/s11064-024-04116-w
Siripurapu, G., Verma, B., Biswas, D., Reghu, A., Vishnoi, A., Radhakrishnan, D. M., et al. (2023). Social cognition in Parkinson's disease: a case-control study. Mov. Disord. Clin. Pract. 10, 399–405. doi: 10.1002/mdc3.13653
Speer, R. E., Karuppagounder, S. S., Basso, M., Sleiman, S. F., Kumar, A., Brand, D., et al. (2013). Hypoxia-inducible factor prolyl hydroxylases as targets for neuroprotection by "antioxidant" metal chelators: from ferroptosis to stroke. Free Radic. Biol. Med. 62, 26–36. doi: 10.1016/j.freeradbiomed.2013.01.026
Sun, J., Lin, X.-M., Lu, D.-H., Wang, M., Li, K., Li, S.-R., et al. (2023). Midbrain dopamine oxidation links ubiquitination of glutathione peroxidase 4 to ferroptosis of dopaminergic neurons. J. Clin. Invest. 133:e165228. doi: 10.1172/jci165228
Wang, Z. F., Kou, M. L., Deng, Q. Y., Yu, H. T., Mei, J. L., Gao, J., et al. (2024). Acupuncture activates IRE1/XBP1 endoplasmic reticulum stress pathway in Parkinson's disease model rats. Behav. Brain Res. 462:114871. doi: 10.1016/j.bbr.2024.114871
Wang, Y. M., Wu, S., Li, Q., Sun, H. Y., and Wang, H. Q. (2023). Pharmacological inhibition of Ferroptosis as a therapeutic target for neurodegenerative diseases and strokes. Adv. Sci. 10:e2300325. doi: 10.1002/advs.202300325
Wu, L. Y., Shan, L. N., Xu, D. Y., Lin, D. F., and Bai, B. J. (2024). Pyroptosis in cancer treatment and prevention: the role of natural products and their bioactive compounds. Med. Oncol. 41:66. doi: 10.1007/s12032-023-02293-2
Yang, Y. T., Liu, C., Wang, M., Cheng, H., Wu, H., Luo, S. Y., et al. (2024). Arenobufagin regulates the p62-Keap1-Nrf2 pathway to induce autophagy-dependent ferroptosis in HepG2 cells. Naunyn Schmiedeberg's Arch. Pharmacol. 2:5. doi: 10.1007/s00210-023-02916-5
Yao, Y., Li, P., Jiang, S., Meng, X., Gao, H., and Yang, X. (2024). A mechanism study on the antioxidant pathway of Keap1-Nrf2-ARE inhibiting Ferroptosis in dopaminergic neurons. Curr. Mol. Med. 24:44938. doi: 10.2174/0115665240266555231120044938
Yu, H.-Y., Sun, T., Wang, Z., Li, H., Xu, D., An, J., et al. (2023). Exendin-4 and linagliptin attenuate neuroinflammation in a mouse model of Parkinson's disease. Neural Regen. Res. 18, 1818–1826. doi: 10.4103/1673-5374.360242
Yue, M., Wei, J., Chen, W., Hong, D., Chen, T., and Fang, X. (2022). Neurotrophic role of the next-generation probiotic strain L. lactis MG1363-pMG36e-GLP-1 on Parkinson's disease via inhibiting Ferroptosis. Nutrients 14:4886. doi: 10.3390/nu14224886
Zhang, L., Lang, F., Feng, J., and Wang, J. (2024). Review of the therapeutic potential of Forsythiae Fructus on the central nervous system: active ingredients and mechanisms of action. J. Ethnopharmacol. 319:117275. doi: 10.1016/j.jep.2023.117275
Zhang, X., Wang, Q. Y., Cao, G. R., Luo, M. L., Hou, H. L., and Yue, C. (2023). Pyroptosis by NLRP3/caspase-1/gasdermin-D pathway in synovial tissues of rheumatoid arthritis patients. J. Cell. Mol. Med. 27, 2448–2456. doi: 10.1111/jcmm.17834
Zhang, H., Wu, J., Shen, F.-F., Yuan, Y.-S., Li, X., Ji, P., et al. (2020). Activated Schwann cells and increased inflammatory cytokines IL-1β, IL-6, and TNF-α in patients' sural nerve are lack of tight relationship with specific sensory disturbances in Parkinson's disease. CNS Neurosci. Ther. 26, 518–526. doi: 10.1111/cns.13282
Zhao, S. Z., Li, X. T., Wang, J., and Wang, H. G. (2021). The role of the effects of autophagy on NLRP3 Inflammasome in inflammatory nervous system diseases. Front. Cell Dev. Biol. 9:657478. doi: 10.3389/fcell.2021.657478
Zhao, D. J., Tian, C., Cheng, M., Yang, F., Tian, C. X., Liu, Y. N., et al. (2023). Carrier-free quercetin nanomedicine blocks NLRP3 deubiquitination and TXNIP recruitment for Parkinson?S disease therapy. Chem. Eng. J. 464:142697. doi: 10.1016/j.cej.2023.142697
Zheng, R., Yan, Y. Q., Dai, S. B., Ruan, Y., Chen, Y., Hu, C. J., et al. (2023). ASC specks exacerbate α-synuclein pathology via amplifying NLRP3 inflammasome activities. J. Neuroinflammation 20:26. doi: 10.1186/s12974-023-02709-w
Zhou, Y., Lu, M., Du, R. H., Qiao, C., Jiang, C. Y., Zhang, K. Z., et al. (2016). MicroRNA-7 targets nod-like receptor protein 3 inflammasome to modulate neuroinflammation in the pathogenesis of Parkinson's disease. Mol. Neurodegener. 11:28. doi: 10.1186/s13024-016-0094-3
Zhu, K. Y., Zhu, X., Sun, S. H., Yang, W., Liu, S. Q., Tang, Z., et al. (2021). Inhibition of TLR4 prevents hippocampal hypoxic-ischemic injury by regulating ferroptosis in neonatal rats. Exp. Neurol. 345:113828. doi: 10.1016/j.expneurol.2021.113828
Keywords: Parkinson’s disease, ferroptosis, pyroptosis, bibliometric analysis, Citespace, VOSviewer
Citation: Wu Z, Zhong K, Tang B and Xie S (2024) Research trends of ferroptosis and pyroptosis in Parkinson’s disease: a bibliometric analysis. Front. Mol. Neurosci. 17:1400668. doi: 10.3389/fnmol.2024.1400668
Edited by:
Junke Song, Chinese Academy of Medical Sciences and Peking Union Medical College, ChinaReviewed by:
Hongquan Wang, Tianjin Medical University Cancer Institute and Hospital, ChinaEmir Malovic, University of Illinois Chicago, United States
Copyright © 2024 Wu, Zhong, Tang and Xie. This is an open-access article distributed under the terms of the Creative Commons Attribution License (CC BY). The use, distribution or reproduction in other forums is permitted, provided the original author(s) and the copyright owner(s) are credited and that the original publication in this journal is cited, in accordance with accepted academic practice. No use, distribution or reproduction is permitted which does not comply with these terms.
*Correspondence: Biao Tang, biaotang@hnucm.edu.cn; Sijian Xie, xsj@hnucm.edu.cn
†These authors have contributed equally to this work and share first authorship