- 1Instituto de Investigación Científica, Universidad Juárez del Estado de Durango, Durango, México
- 2Hospital General Santiago Ramón y Cajal-ISSSTE, Durango, México
- 3Unidad de Trastornos del Movimiento y Sueño, Hospital General Dr. Manuel Gea González, Ciudad de México, México
Parkinson’s disease (PD) is a complex neurodegenerative condition characterized by alpha-synuclein aggregation and dysfunctional protein degradation pathways. This study investigates the differential gene expression of pivotal components (UBE2K, PSMC4, SKP1, and HSPA8) within these pathways in a Mexican-Mestizo PD population compared to healthy controls. We enrolled 87 PD patients and 87 controls, assessing their gene expression levels via RT-qPCR. Our results reveal a significant downregulation of PSMC4, SKP1, and HSPA8 in the PD group (p = 0.033, p = 0.003, and p = 0.002, respectively). Logistic regression analyses establish a strong association between PD and reduced expression of PSMC4, SKP1, and HSPA8 (OR = 0.640, 95% CI = 0.415–0.987; OR = 0.000, 95% CI = 0.000–0.075; OR = 0.550, 95% CI = 0.368–0.823, respectively). Conversely, UBE2K exhibited no significant association or expression difference between the groups. Furthermore, we develop a gene expression model based on HSPA8, PSMC4, and SKP1, demonstrating robust discrimination between healthy controls and PD patients. Notably, the model’s diagnostic efficacy is particularly pronounced in early-stage PD. In conclusion, our study provides compelling evidence linking decreased gene expression of PSMC4, SKP1, and HSPA8 to PD in the Mexican-Mestizo population. Additionally, our gene expression model exhibits promise as a diagnostic tool, particularly for early-stage PD diagnosis.
1 Introduction
Parkinson’s disease (PD) stands as a multifaceted neurodegenerative disorder characterized by the progressive degeneration of dopaminergic neurons. Nevertheless, the intricate mechanisms underlying this condition remain shrouded in uncertainty. Emerging evidence has spotlighted dysregulation in the clearance and degradation of alpha-synuclein as a pivotal player in the pathogenesis of neuronal demise in PD (Mehra et al., 2019; Jankovic and Tan, 2020). At the crux of this degradation nexus lies the concerted interplay among chaperones, the ubiquitin-proteasome system (UPS), and autophagy-lysosomal pathways.
The UPS pathway orchestrates the disposal of damaged proteins through a meticulous tagging process involving three key enzymes: activating enzyme E1, conjugating enzyme E2, and ligase enzyme E3 (Chen and Dou, 2010). Subsequent to this tagging, the protein-ubiquitin conjugates are recognized and degraded by the 26S proteasome, a large proteolytic assembly resident within the cytosol and nucleus of eukaryotic cells. This proteasome is composed of a 20S core particle and a 19S regulatory particle (Jarome and Devulapalli, 2018).
Intriguingly, prior inquiries into the expression of genes associated with these degradation machineries in blood and brain tissues of individuals afflicted by PD, including PSMC4 (an integral component of the 19S proteasomal particle), SKP1 (an essential constituent of the SCF complex endowed with E3 ubiquitin ligase activity), UBE2K (an ubiquitin-conjugating enzyme E2K), and HSPA8 (a 70kDa heat shock protein exerting chaperone functions), have yielded divergent results (Grunblatt et al., 2004; Mandel et al., 2005, 2012b; Molochnikov et al., 2012; Su et al., 2018). This divergence may stem from genetic disparities among diverse populations, imparting an element of complexity to our understanding of PD etiology.
Thus, the principal objective of our study is to scrutinize the expression profiles of these genes in peripheral blood samples sourced from the Mexican-mestizo population. By doing so, we aim to ascertain whether these gene expression patterns possess the potential to serve as a risk profile for PD.
2 Materials and methods
2.1 Study participants and ethical criteria
We collected samples from a cohort of 87 PD patients and 87 healthy, age-matched controls devoid of familial or personal histories of neurodegenerative diseases. PD diagnoses were confirmed by neurologists employing the United Kingdom Parkinson’s disease Society Brain Bank (UKPDSBB) diagnostic criteria. Participants were recruited from three prominent public hospitals located in central and northwest Mexico: Hospital General Dr. Manuel Gea Gonzalez in Mexico City, Hospital General 450, and Hospital General Santiago Ramón y Cajal in Durango City. This study secured the ethical approval of each participating hospital’s ethics committee.
All procedures adhered strictly to the ethical principles outlined in the 1964 Helsinki Declaration and its subsequent amendments. Informed written consent was obtained from all participants, with the additional requirement of a family member’s consent for each participant. Pertinent patient data, encompassing age, gender, presence of depression, cognitive status, age of disease onset, and Unified Parkinson’s disease Rating Scale (UPDRS) scores, were meticulously documented.
2.2 Blood collection, RNA extraction, cDNA synthesis, and RT-qPCR
Venous whole blood was collected from the subjects in Tempus Blood RNA tubes and reserved at −80 C until RNA extraction. We processed samples according to the manufacturer protocol to extract total RNA using MagMAX for Stabilized Blood Tubes RNA Isolation kit (Life Technologies, Norway). We then synthesized cDNA using a High-Capacity cDNA Reverse Transcription kit (Applied Biosystems, Carlsbad, CA, USA) according to the manufacturer’s instructions. Concentration and purity of RNA and cDNA were determined using NanoDrop 2000 spectrophotometer (Thermo Fisher Scientific Inc., Germering, Germany) and cDNA was stored at −20 C until further evaluation. Gene expression levels of UBE2K, HSPA8, SKP1, and PSMC4 were measured through relative quantification (RQ) of 125 ng/mL of cDNA by Real-time quantitative PCR (RT-qPCR) using a QuantStudio 3 System (Applied Biosystems, Carlsbad, CA, USA) with TaqMan assays (UBE2K assay ID Hs00193507_m1, HSPA8 assay ID Hs03044880_gH, SKP1 assay ID Hs00429069_m1 and PSMC4 assay ID Hs00197826_m1, Applied Biosystems). The expression was normalized to the RPLP0 gene (RPLP0 assay ID Hs00420895_gH, Applied Biosystems). The reaction conditions were: initial hold of 10 min at 95 C; 40 cycles of denaturation for 15 s at 95 C and annealing for 1 min at 60 C.
2.3 Statistical methods
Statistical analyses were executed using SPSS Statistics 20.0 software. Quantitative data are presented as mean ± standard deviation (SD). For normally distributed parameters, differences were assessed using a two-tailed t-test, while non-parametric tests were applied to analyze mRNA and protein levels. Logistic regression was employed to investigate the association between mRNA expression and PD, with significant P-values retaining relevance in constructing predictive classifier models. Receiver operating characteristic curve (ROC) data were derived from predictive probabilities generated via multivariate logistic regression, encompassing all PD patients and a subset of early-stage PD patients (those with less than 5 and 3 years of disease evolution). Correlations were evaluated via Spearman correlation with the two-tailed test of significance. P-value < 0.05 was considered statistically significant.
3 Results
3.1 Demographic and clinical characteristics
Table 1 presents a comprehensive overview of the demographic and clinical attributes of both the PD cases and control subjects. Comparative analysis of these variables between the two groups unveiled significant differences on the presence of depression (p = 0.040).
3.2 Gene expression profiles
Figure 1 provides a graphical representation of the observed gene expression levels in both the PD and control groups. Utilizing Relative Quantification (RQ) analysis, we identified a significant reduction in the expression levels of HSPA8, SKP1, and PSMC4 among PD cases (p = 0.002, p = 0.003, and p = 0.033, respectively). To further elucidate their relevance to PD, logistic regression analysis was conducted, adjusting for sex and age (Table 2). The results unequivocally substantiated the associations of HSPA8, SKP1, and PSMC4 with PD. However, UBE2K exhibited no statistically significant association with the disease.
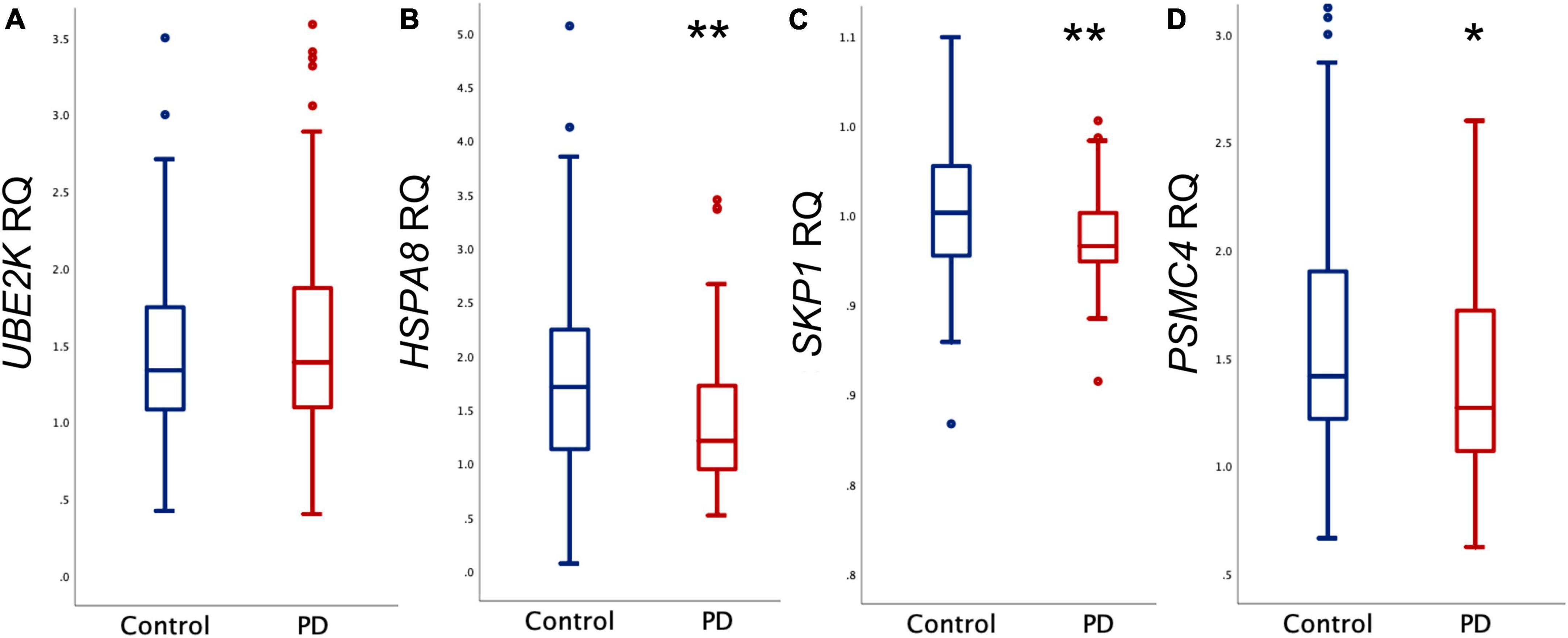
Figure 1. Relative quantification (RQ) of mRNA. Comparison between control and PD groups of mRNA levels of (A) UBE2K, (B) HSPA8, (C) SKP1, and (D) PSMC4. Outliers are denoted by dots. **p < 0.005, *p < 0.05.
3.3 Influence of depression on gene expression
We observed a notable disparity in the frequency of depression between the PD and control groups (Table 1), consequently, we conducted a gene expression association analysis considering while adjusting for sex, age, and depression. The findings revealed that, even after factoring in depression, the previously established associations for HSPA8 and SKP1 remained statistically significant (Table 3).
3.4 Correlation analysis of gene expression
An exploratory analysis of gene expression within the control group unveiled intriguing interrelationships among the four genes under scrutiny. Specifically, we observed a positive correlation between the expression levels of HSPA8, UBE2K, and PSMC4. Conversely, SKP1 exhibited a negative correlation with these three genes (Figure 2A). Similar results were observed when testing the PD group, except for the correlation between SKP1 and PSMC4, which was not statistically significant (p = 0.165, Figure 2B). Furthermore, a correlation analysis within the PD group revealed no significant correlation between PD severity (measured with UPDRS) and the expression levels of SKP1A (p = 0.455), PSMC4 (p = 0.655), UBE2K (p = 0.305) and HSPA8 (p = 0.786).
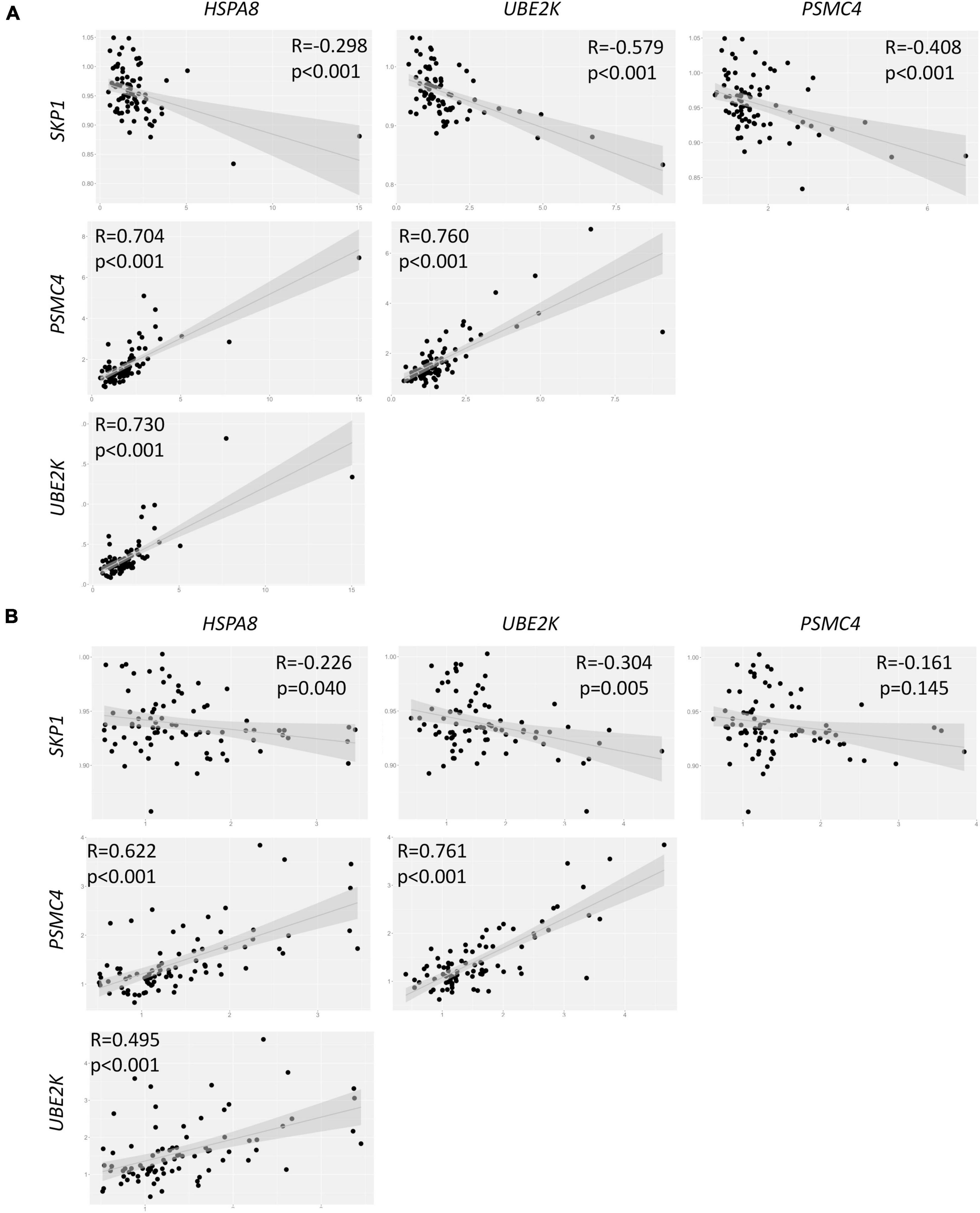
Figure 2. Correlation analysis of gene expression in both control (A) and PD (B) groups. R = Spearman correlations coefficient.
3.5 Predictive utility of gene expression
To assess the potential utility of gene expression as a prognostic biomarker for PD, we constructed Receiver Operating Characteristic (ROC) curves based on predictive values derived from logistic regression. We formulated three distinct models, each contingent on the duration of PD evolution (Figure 3). Evaluation of these curves unveiled increasing values for the Area under the Curve (AUC), signifying that a shorter duration of PD evolution positively impacts the predictive value of the model. At a cut-off point of 0.25 it was possible to distinguish between PD individuals and healthy controls with sensitivity and specificity values of 77% and 72.3%, respectively (Figure 3C).
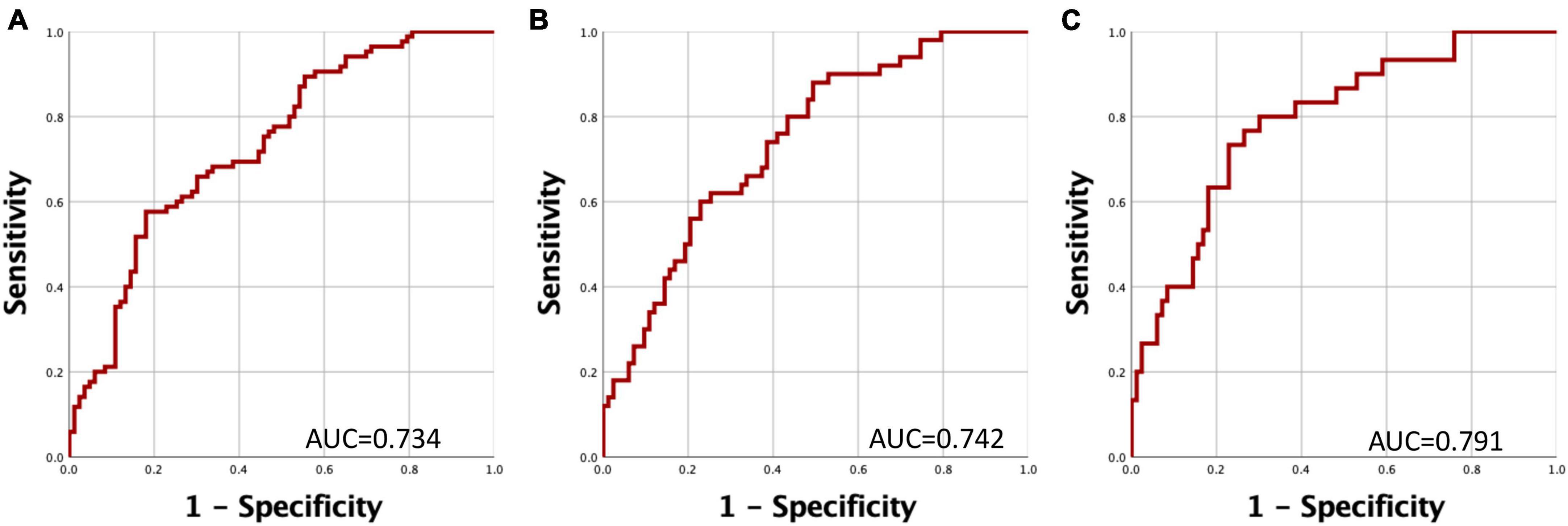
Figure 3. Receiver Operating Characteristic (ROC) Curves for Discriminating Between PD Patients and Controls. The curve represents the relationship between specificity and sensitivity based on the predictive probability derived from gene expression levels of HSPA8, SKP1, and PSMC4. (A) Comparison of PD patients versus healthy controls. (B) Comparison of PD patients with a disease duration of less than 5 years versus healthy controls. (C) Comparison of PD patients with a disease duration of less than 3 years versus healthy controls.
4 Discussion
In this study, we delved into the gene expression profiles of UBE2K, HSPA8, SKP1, and PSMC4 within both control and PD cohorts to evaluate their potential implications in the pathogenesis of PD. Our findings unveiled marked reductions in the gene expression levels of HSPA8, SKP1, and PSMC4 in the PD group in comparison to the control group.
The PSMC4 gene encodes the proteasomal protein S6 ATPase, a constituent of the 19S regulatory subunit essential for the assembly of the 26S proteasome (Dahlmann, 2016). Previous investigations have identified the presence of the PSMC4 protein in Lewy bodies, demarcating their periphery within dopaminergic neurons of the substantia nigra (Grünblatt et al., 2018). Notably, our observations concerning PSMC4 gene expression in blood align with prior reports (Molochnikov et al., 2012). Similar reductions in mRNA levels of PSMC4 within the substantia nigra pars compacta (SNpc) of the brain have been documented in PD patients when compared to controls (Grunblatt et al., 2004; Grünblatt, 2012). The correlation between gene expression levels, protein presence in the SNpc, and localization within Lewy bodies in post-mortem SN samples from PD patients suggests the potential biological relevance of our findings derived from blood samples (Marx et al., 2007; Grünblatt et al., 2018). Recent research indicated a decreased expression of PSMC4 mRNA, particularly evident after 3 years of disease progression, and established a correlation with disease severity. Nevertheless, this correlation, while noteworthy, did not achieve the requisite strength to be incorporated into a predictive PD classifier model (Rabey et al., 2020).
The SKP1 gene encodes the SKP1 protein, involved in the formation of the SCF complex, endowed with E3 ubiquitin ligase activity. This intricate assembly plays a pivotal role in identifying target proteins for degradation via the ubiquitin-proteasome system, primarily through interactions with F-box proteins (Zheng et al., 2002). SKP1 exhibits the ability to directly interact with FBXO7, an F-box protein implicated in the regulation of alpha-synuclein (Zhao et al., 2013; Conedera et al., 2016). Perturbations in SKP1 function could potentially contribute to PD development by disrupting the proper degradation of proteins, leading to an accrual of misfolded proteins (Mandel et al., 2012a). Consistent with our findings, reduced expression levels of SKP1 in both the SNpc and blood have been documented in PD (Grunblatt et al., 2004; Mandel et al., 2009; Molochnikov et al., 2012). It is important to underscore that silencing of SKP1 has been experimentally demonstrated to promote the accumulation of cytoplasmic inclusions reminiscent of Lewy bodies while concurrently exerting a negative regulatory effect on HSPA8 gene expression (Fishman-Jacob et al., 2009; Mandel et al., 2012a). In vitro studies have further validated that SKP1 deficiency exacerbates PD pathology, culminating in the formation of Lewy body-like inclusions and ensuing neuronal demise (Mandel et al., 2012b). Notably, our identification of a negative correlation between SKP1 expression and all three genes within our control group implies that SKP1 may potentially exert its effects only in the presence of cellular damage. Consequently, normal SKP1 expression might not suffice to deter PD progression in the absence of underlying cellular damage.
HSC70 protein, encoded by the HSPA8 gene, stands as an integral component of Lewy bodies in PD (Grünblatt et al., 2018). This chaperone protein, orchestrates the selective degradation of proteins, maintaining cellular proteostasis through chaperone-mediated autophagy, a process contingent on lysosomes (Nie et al., 2021). Our findings regarding HSPA8 expression harmonize with prior assessments encompassing transcript and protein levels in both peripheral blood and brain tissues (Grunblatt et al., 2004; Tanaka, 2009; Alvarez-Erviti et al., 2010; Papagiannakis et al., 2015). Nonetheless, it is worth noting that Molochnikov et al. (2012) reported an elevation in HSPA8 expression levels in PD patients, although the biological significance of this elevation was not explained.
The differential expression profiles observed in our study suggest potential perturbations in protein degradation pathways within our study population, which could underlie the abnormal aggregation of proteins integral to Lewy bodies and consequently impact dopaminergic neuron function.
Our comprehensive ROC curve analysis illuminates the potential utility of HSPA8, PSMC4, and SKP1 gene expression levels as effective discriminators between healthy controls and individuals with PD. Similar results in a multi- center study in the German, Italian and Israeli population suggest the ability of a five-gene panel, including HSPA8, PSMC4, SKP1 and UBE2K, to diagnose early/mild PD in Italy, Germany, and Israel populations (Molochnikov et al., 2012).
As depicted in Figure 3, this discriminatory capacity is further augmented when PD has a shorter duration. These observations collectively indicate that the expression levels of these genes experience significant reductions during the early stages of PD. Importantly, these reductions may be partially ameliorated through medication-induced epigenetic modifications.
Previously, it has been shown that the accuracy of clinical diagnosis for PD could range from 53–74% (Tolosa et al., 2006). More recently, accuracies ranging from 69% to over 90% have been reported in some populations with advanced symptoms (Rizzo et al., 2016). However, a recent study describes an accuracy of 26% in clinical diagnoses for PD in patients with recent symptom onset (Prajjwal et al., 2023). Our model suggests an accuracy of 79.1% for predicting PD in the early stage, as indicated by the AUC values obtained, suggesting it as a promising tool for PD prediction.
One noteworthy limitation of our study resides in the fact that all PD patients were undergoing L-dopa treatment, a factor that has been previously suggested to potentially influence gene expression patterns (Taravini et al., 2016). Reports indicated that L-dopa influenced DNA methylation, resulting in reduced expression of the SNCA gene. Nevertheless, it was suggested that its epigenetic influence on other genes could have been possible (Schmitt et al., 2015; Guhathakurta et al., 2017; Song et al., 2017).
The variances in our findings when compared to other populations underscore the necessity of exploring associations between single nucleotide polymorphisms (SNPs) and gene expression to elucidate these disparities (Soldner et al., 2016; Salas-Leal et al., 2021). Consequently, future endeavors should encompass genotyping to modulate transcript variants, shedding light on these discrepancies. Additionally, the execution of cohort studies will be indispensable in delineating the potential utility of our model as a predictive tool for PD.
Data availability statement
The raw data supporting the conclusions of this article will be made available by the authors, without undue reservation.
Ethics statement
The studies involving humans were approved by the Comité de ética del Hospital General Dr. Manuel Gea González, Comité de ética del Hospital General 450 and Comité de ética del Hospital General Santiago Ramón y Cajal. The studies were conducted in accordance with the local legislation and institutional requirements. Written informed consent for participation in this study was provided by the participants’ legal guardians/next of kin.
Author contributions
AS-L: Conceptualization, Methodology, Writing−original draft. SS-P: Data curation, Formal analysis, Methodology, Writing−review and editing. EH-C: Data curation, Formal analysis, Writing−review and editing. LV-V: Investigation, Methodology, Writing−review and editing. EA-S: Investigation, Methodology, Writing−review and editing. FC-J: Investigation, Methodology, Writing−review and editing. EM-H: Data curation, Methodology, Writing−review and editing. OL-L: Data curation, Formal analysis, Writing−review and editing. GQ-C: Data curation, Investigation, Writing−review and editing. OA-C: Data curation, Formal analysis, Writing−review and editing. AS-C: Conceptualization, Formal analysis, Funding acquisition, Supervision, Writing−review and editing. JS-P: Conceptualization, Methodology, Supervision, Visualization, Writing−review and editing.
Funding
The author(s) declare financial support was received for the research, authorship, and/or publication of the article. This study was supported by the grants from CONACyT (FOSISS 2014:233092 and CB 2015:253857 giving to AS-C).
Acknowledgments
We would like to thank all the participants in the project and their families for their willingness and cooperation throughout the development of this study.
Conflict of interest
The authors declare that the research was conducted in the absence of any commercial or financial relationships that could be construed as a potential conflict of interest.
Publisher’s note
All claims expressed in this article are solely those of the authors and do not necessarily represent those of their affiliated organizations, or those of the publisher, the editors and the reviewers. Any product that may be evaluated in this article, or claim that may be made by its manufacturer, is not guaranteed or endorsed by the publisher.
References
Alvarez-Erviti, L., Rodriguez-Oroz, M., Cooper, J., Caballero, C., Ferrer, I., Obeso, J., et al. (2010). Chaperone-mediated autophagy markers in Parkinson disease brains. Arch. Neurol. 67, 1464–1472. doi: 10.1001/archneurol.2010.198
Chen, D., and Dou, Q. (2010). The ubiquitin-proteasome system as a prospective molecular target for cancer treatment and prevention. Curr. Protein Pept. Sci. 11, 459–470. doi: 10.2174/138920310791824057
Conedera, S., Apaydin, H., Li, Y., Yoshino, H., Ikeda, A., Matsushima, T., et al. (2016). FBXO7 mutations in Parkinson’s disease and multiple system atrophy. Neurobiol. Aging 40:192.e1–192.e5. doi: 10.1016/j.neurobiolaging.2016.01.003
Dahlmann, B. (2016). Mammalian proteasome subtypes: Their diversity in structure and function. Arch. Biochem. Biophys. 591, 132–140. doi: 10.1016/j.abb.2015.12.012
Fishman-Jacob, T., Reznichenko, L., Youdim, M., and Mandel, S. A. (2009). A sporadic Parkinson disease model via silencing of the ubiquitin-proteasome/E3 ligase component SKP1A. J. Biol. Chem. 284, 32835–32845. doi: 10.1074/jbc.M109.034223
Grünblatt, E. (2012). Parkinson’s disease: Molecular risk factors. Parkinsonism Relat. Disord. 18, S45–S48. doi: 10.1016/S1353-8020(11)70016-5
Grunblatt, E., Mandel, S., Jacob-Hirsch, J., Zeligson, S., Amariglo, N., Rechavi, G., et al. (2004). Gene expression profiling of parkinsonian substantia nigra pars compacta; alterations in ubiquitin-proteasome, heat shock protein, iron and oxidative stress regulated proteins, cell adhesion/cellular matrix and vesicle trafficking genes. J. Neural Transm. 111, 1543–1573. doi: 10.1007/s00702-004-0212-1
Grünblatt, E., Ruder, J., Monoranu, C., Riederer, P., Youdim, M., and Mandel, S. (2018). Differential alterations in metabolism and proteolysis-related proteins in human Parkinson’s Disease substantia nigra. Neurotox Res. 33, 560–568. doi: 10.1007/s12640-017-9843-5
Guhathakurta, S., Bok, E., Evangelista, B., and Kim, Y. (2017). Deregulation of α-synuclein in Parkinson’s disease: Insight from epigenetic structure and transcriptional regulation of SNCA. Prog. Neurobiol. 154, 21–36. doi: 10.1016/j.pneurobio.2017.04.004
Jankovic, J., and Tan, E. (2020). Parkinson’s disease: Etiopathogenesis and treatment. J. Neurol. Neurosurg. Psychiatry 91, 795–808. doi: 10.1136/jnnp-2019-322338
Jarome, T., and Devulapalli, R. (2018). The ubiquitin-proteasome system and memory: Moving beyond protein degradation. Neurosci 24, 639–651. doi: 10.1177/1073858418762317
Mandel, S., Fishman-Jacob, T., and Youdim, M. (2009). Modeling sporadic Parkinson’s disease by silencing the ubiquitin E3 ligase component, SKP1A. Parkinsonism Relat. Disord. 15(Suppl. 3), S148–S151. doi: 10.1016/S1353-8020(09)70803-X
Mandel, S., Fishman-Jacob, T., and Youdim, M. (2012b). Targeting Skp1, an ubiquitin E3 ligase component found decreased in sporadic Parkinsons Disease. Neurodegener. Dis. 10, 220–223. doi: 10.1159/000333223
Mandel, S., Fishman-Jacob, T., and Youdim, M. (2012a). Genetic reduction of the E3 ubiquitin ligase element, SKP1A and environmental manipulation to emulate cardinal features of Parkinson’s disease. Parkinsonism Relat. Disord. 18(Suppl. 1), S177–S179. doi: 10.1016/S1353-8020(11)70055-4
Mandel, S., Grunblatt, E., Riederer, P., Amariglio, N., Jacob-Hirsch, J., Rechavi, G., et al. (2005). Gene expression profiling of sporadic Parkinson’s disease substantia nigra pars compacta reveals impairment of ubiquitin-proteasome subunits. SKP1A, aldehyde dehydrogenase, and chaperone HSC-70. Ann. N. Y. Acad. Sci. 1053, 356–375. doi: 10.1196/annals.1344.031
Marx, F., Soehn, A., Berg, D., Melle, C., Schiesling, C., Lang, M., et al. (2007). The proteasomal subunit S6 ATPase is a novel synphilin-1 interacting protein–implications for Parkinson’s disease. FASEB J. Off. Publ. Fed. Am. Soc. Exp. Biol. 21, 1759–1767. doi: 10.1096/fj.06-6734com
Mehra, S., Sahay, S., and Maji, S. (2019). α-Synuclein misfolding and aggregation: Implications in Parkinson’s disease pathogenesis. Biochim. Biophys. Acta Proteins Proteomics 1867, 890–908. doi: 10.1016/j.bbapap.2019.03.001
Molochnikov, L., Rabey, J., Dobronevsky, E., Bonuccelli, U., Ceravolo, R., Frosini, D., et al. (2012). A molecular signature in blood identifies early Parkinson’s disease. Mol. Neurodegener. 7:26. doi: 10.1186/1750-1326-7-26
Nie, T., Tao, K., Zhu, L., Huang, L., Hu, S., Yang, R., et al. (2021). Chaperone-mediated autophagy controls the turnover of E3 ubiquitin ligase MARCHF5 and regulates mitochondrial dynamics. Autophagy 17, 2923–2938. doi: 10.1080/15548627.2020.1848128
Papagiannakis, N., Xilouri, M., Koros, C., Stamelou, M., Antonelou, R., Maniati, M., et al. (2015). Lysosomal alterations in peripheral blood mononuclear cells of Parkinson’s disease patients. Mov. Disord. 30, 1830–1834. doi: 10.1002/mds.26433
Prajjwal, P., Flores Sanga, H., Acharya, K., Tango, T., John, J., and Rodriguez, R. (2023). Parkinson’s disease updates: Addressing the pathophysiology, risk factors, genetics, diagnosis, along with the medical and surgical treatment. Ann. Med. Surg. 85, 4887–4902. doi: 10.1097/MS9.0000000000001142
Rabey, J., Yarden, J., Dotan, N., Mechlovich, D., Riederer, P., and Youdim, M. (2020). Creation of a gene expression classifier for predicting Parkinson’s disease rate of progression. J. Neural. Transm. 127, 755–762. doi: 10.1007/s00702-020-02194-y
Rizzo, G., Copetti, M., Arcuti, S., Martino, D., Fontana, A., and Logroscino, G. (2016). Accuracy of clinical diagnosis of Parkinson disease: A systematic review and meta-analysis. Neurology 86, 566–576. doi: 10.1212/WNL.0000000000002350
Salas-Leal, A., Salas-Pacheco, S., Gavilán-Ceniceros, J., Castellanos-Juárez, F., Méndez-Hernández, E., and La Llave-León, O. (2021). α-syn and SNP rs356219 as a potential biomarker in blood for Parkinson’s disease in Mexican Mestizos. Neurosci. Lett. 754:135901. doi: 10.1016/j.neulet.2021.135901
Schmitt, I., Kaut, O., Khazneh, H., deBoni, L., Ahmad, A., Berg, D., et al. (2015). L-dopa increases α-synuclein DNA methylation in Parkinson’s disease patients in vivo and in vitro. Mov. Disord. 30, 1794–1801. doi: 10.1002/mds.26319
Soldner, F., Stelzer, Y., Shivalila, C., Abraham, B., Latourelle, J., Barrasa, M., et al. (2016). Parkinson-associated risk variant in distal enhancer of α-synuclein modulates target gene expression. Nature 533, 95–99. doi: 10.1038/nature17939
Song, J., Kim, B., Nguyen, D., Samidurai, M., and Choi, S. (2017). Levodopa (L-DOPA) attenuates endoplasmic reticulum stress response and cell death signaling through DRD2 in SH-SY5Y neuronal cells under α-synuclein-induced toxicity. Neuroscience 358, 336–348. doi: 10.1016/j.neuroscience.2017.06.060
Su, J., Huang, P., Qin, M., Lu, Q., Sang, X., Cai, Y., et al. (2018). Reduction of HIP2 expression causes motor function impairment and increased vulnerability to dopaminergic degeneration in Parkinson’s disease models. Cell Death Dis. 9:1020. doi: 10.1038/s41419-018-1066-z
Tanaka, K. (2009). The proteasome: Overview of structure and functions. Proc. Jpn. Acad. Ser. B Phys. Biol. Sci. 85, 12–36. doi: 10.2183/pjab.85.12
Taravini, I., Larramendy, C., Gomez, G., Saborido, M., Spaans, F., Fresno, C., et al. (2016). Contrasting gene expression patterns induced by levodopa and pramipexole treatments in the rat model of Parkinson’s disease. Neuropharmacology 101, 576–589. doi: 10.1016/j.neuropharm.2015.04.018
Tolosa, E., Wenning, G., and Poewe, W. (2006). The diagnosis of Parkinson’s disease. Lancet Neurol. 5, 75–86. doi: 10.1016/S1474-4422(05)70285-4
Zhao, T., Severijnen, L., van der Weiden, M., Zheng, P., Oostra, B., Hukema, R., et al. (2013). FBXO7 immunoreactivity in α-synuclein-containing inclusions in Parkinson disease and multiple system atrophy. J. Neuropathol. Exp. Neurol. 72, 482–488. doi: 10.1097/NEN.0b013e318293c586
Keywords: Parkinson’s disease, UBE2K, PSMC4, SKP1, HSPA8, protein degradation systems
Citation: Salas-Leal AC, Salas-Pacheco SM, Hernández-Cosaín EI, Vélez-Vélez LM, Antuna-Salcido EI, Castellanos-Juárez FX, Méndez-Hernández EM, La Llave-León O, Quiñones-Canales G, Arias-Carrión O, Sandoval-Carrillo AA and Salas-Pacheco JM (2023) Differential expression of PSMC4, SKP1, and HSPA8 in Parkinson’s disease: insights from a Mexican mestizo population. Front. Mol. Neurosci. 16:1298560. doi: 10.3389/fnmol.2023.1298560
Received: 21 September 2023; Accepted: 13 November 2023;
Published: 05 December 2023.
Edited by:
Hai Sun, The State University of New Jersey, United StatesReviewed by:
Sung Ung Kang, Johns Hopkins University, United StatesSangjune Kim, Chungbuk National University, Republic of Korea
Copyright © 2023 Salas-Leal, Salas-Pacheco, Hernández-Cosaín, Vélez-Vélez, Antuna-Salcido, Castellanos-Juárez, Méndez-Hernández, Llave-León, Quiñones-Canales, Arias-Carrión, Sandoval-Carrillo and Salas-Pacheco. This is an open-access article distributed under the terms of the Creative Commons Attribution License (CC BY). The use, distribution or reproduction in other forums is permitted, provided the original author(s) and the copyright owner(s) are credited and that the original publication in this journal is cited, in accordance with accepted academic practice. No use, distribution or reproduction is permitted which does not comply with these terms.
*Correspondence: José M. Salas-Pacheco, anNhbGFzX3BhY2hlY29AaG90bWFpbC5jb20=; Ada A. Sandoval-Carrillo, YWRkYS1zYW5kb3ZhbEBob3RtYWlsLmNvbQ==