- 1Department of Neuroscience Intensive Care Unit, The Second Affiliated Hospital, Zhejiang University School of Medicine, Hangzhou, Zhejiang, China
- 2Department of Neurosurgery, The Second Affiliated Hospital, Zhejiang University School of Medicine, Hangzhou, Zhejiang, China
Stroke is a devastating condition that can lead to significant morbidity and mortality. The aftermath of a stroke, particularly hemorrhagic transformation (HT) and brain edema, can significantly impact the prognosis of patients. Early detection and effective management of these complications are crucial for improving outcomes in stroke patients. This review highlights the emerging diagnostic markers and therapeutic targets including claudin, occludin, zonula occluden, s100β, albumin, MMP-9, MMP-2, MMP-12, IL-1β, TNF-α, IL-6, IFN-γ, TGF-β, IL-10, IL-4, IL-13, MCP-1/CCL2, CXCL2, CXCL8, CXCL12, CCL5, CX3CL1, ICAM-1, VCAM-1, P-selectin, E-selectin, PECAM-1/CD31, JAMs, HMGB1, vWF, VEGF, ROS, NAC, and AQP4. The clinical significance and implications of these biomarkers were also discussed.
1 Introduction
In strokes, the blood supply to part of the brain is interrupted or reduced, resulting in a loss of oxygen and nutrients to the brain (Li B. et al., 2022). Brain cells begin to die within minutes. Strokes can lead to permanent disability or death and are a medical emergency. A stroke is classified as ischemic, hemorrhagic, transient ischemic attack (TIA), or cryptogenic based on its cause (Campbell et al., 2019). Despite the availability of reperfusion therapies such as thrombolytic therapy and mechanical thrombectomy, hemorrhagic transformation (HT) and brain edema remain significant challenges in stroke management (Uniken Venema et al., 2022).
Hemorrhagic transformation represents the occurrence of bleeding within an area of the brain that has suffered an ischemic insult (Hong et al., 2021). This bleeding can happen spontaneously or be precipitated by treatments, especially those that aim to restore blood flow, like thrombolytic agents (Jickling et al., 2014). Brain edema refers to the accumulation of fluid in the brain tissue, leading to increased intracranial pressure. Following an ischemic stroke, edema can develop around the infarcted area, causing a mass effect, which can further compromise brain function and even lead to herniation, a life-threatening condition (Ji et al., 2021). Both complications are associated with poor prognosis and limited treatment options. While imaging methods like CT and MRI are commonly used to diagnose HT and brain edema, the early stages of these complications may not always be detectable (von Kummer et al., 2015). Besides, there’s a lack of universally accepted, standardized treatment protocols for managing HT and brain edema, especially in the setting of large vessel occlusions and after endovascular procedures. Current treatments for brain edema, such as osmotic therapy and decompressive craniectomy, are somewhat non-specific and come with their own set of risks (Beez et al., 2019). More targeted therapies, perhaps at the molecular or cellular level, are needed. Therefore, identifying novel diagnostic markers and therapeutic targets is essential for early detection and improved management of post-stroke HT and brain edema. This review aims to explore recent advances in the pathophysiology of post-stroke HT and brain edema, as well as the identification and potential therapeutic targets of novel diagnostic markers (Figure 1).
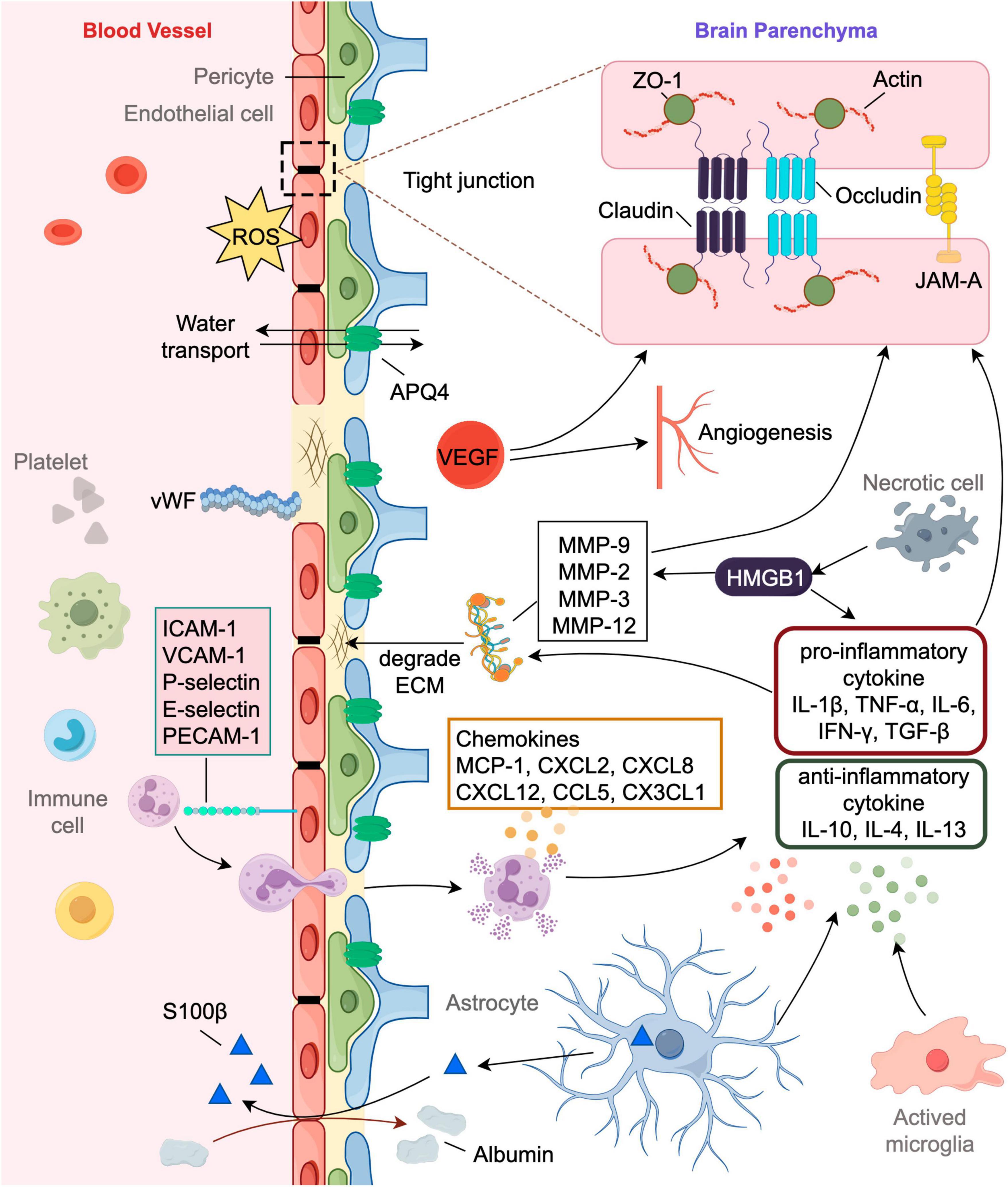
Figure 1. Involvement of markers in the pathophysiology of post-stroke HT and brain edema. The blood-brain barrier (BBB) consists of endothelial cells surrounded by pericytes, astrocytic end feet processes and the basement membrane, comprising extracellular matrix (ECM). Disruption of the BBB have significant implications for hemorrhagic transformation (HT) and brain edema. Tight junctions (TJs) are a key feature of the BBB, sealing the spaces between the endothelial cells and restricting the paracellular movement of solutes. TJs are complex structures comprised of various proteins like claudin, occludin, junction adhesion molecules (JAMs) and zonula occludens (ZO). Neural cells suffer from energy failure after stroke leading to inflammatory cascade and oxidative stress exacerbating BBB disruption. Pro-inflammatory cytokines can increase the expression and promote the activity of matrix metalloproteinases (MMP) which will degrade the ECM’s basal lamina. Markers like von Willebrand factor (vWF) and Vascular Endothelial Growth Factor (VEGF) might be indicative of endothelial disruption or activation, potentially predicting the risk of HT and edema. AQP4 is most abundantly located in the astrocyte foot processes that has insignificant effect on water homeostasis in the brain. It plays an important role in both the formation and resolution of post-stroke edema.
2 Blood-brain barrier (BBB) structural markers
Blood-brain barrier is a highly specialized structure in the central nervous system (CNS) that regulates the passage of substances between the blood and the brain. It plays a crucial role in maintaining a stable internal environment for neuronal function (Sweeney et al., 2019). Disruption of the BBB can have significant implications for brain health, leading to neuronal damage and various neurological conditions such as cerebral edema and brain hemorrhage (Sweeney et al., 2018). Tight junctions (TJs) are complex structures that form the primary barrier within the BBB, (Abdullahi et al., 2018). They seal adjacent endothelial cells together, ensuring a strict regulation of substance movement between the blood and the brain’s extracellular fluid. Tight junction proteins (TJPs) are crucial components of these junctions, and their integrity is essential for maintaining the BBB’s selective permeability (Berndt et al., 2019). Changes in the levels of TJPs like claudin, occluding, junction adhesion molecules (JAMs), and zonula occludens (ZO) after a stroke lead to heightened permeability of the BBB allowing for the extravasation of blood components, which might hint toward impending HT and vasogenic edema (Huang et al., 2012; Zhao et al., 2018).
Claudins are integral membrane proteins that play a pivotal role in the formation and maintenance of TJs. Among the claudin family, claudin-5 is particularly significant in the BBB (Lv et al., 2018). It forms the tight junction strands’ backbone and determines the selective permeability of the barrier (Li C. et al., 2022). While not as selectively determinant as claudins, occludin plays a role in the regulation and maintenance of TJ integrity. It is involved in the sealing of the paracellular pathway (Kim et al., 2020). ZO-1 is a cytoplasmic scaffolding protein that ensures structural stability by linking transmembrane proteins like claudins and occludin to the actin cytoskeleton, providing structural support (Gao and Shivers, 2004). This interaction stabilizes and strengthens the tight junctions, ensuring the selectivity and integrity of the BBB. JAM-A is an adhesion molecule that is part of the immunoglobulin superfamily found at tight junctions between endothelial cells (Williams et al., 1999; Babinska et al., 2020). It interacts with other TJPs like ZO-1 and plays a role in leukocyte transmigration, which can be relevant in neuroinflammatory conditions (Severson and Parkos, 2009; Sladojevic et al., 2014). Alterations in JAM-A expression or function can impact BBB integrity and may be associated with post-stroke complications like HT and brain edema (Haarmann et al., 2010; Xie et al., 2023). Following an ischemic stroke, there’s often inflammation and oxidative stress which can negatively impact the expression, structure, and function of TJPs (Yang et al., 2019). Disrupted TJs of BBB indicate an increased risk of blood products leaking into the brain tissue leading to HT, especially after reperfusion therapies or spontaneous reperfusion. With the disassembly or dysfunction of TJs, there’s an osmotic imbalance created by the abnormal influx of water and solutes from the bloodstream into the brain tissue. This results in cytotoxic and vasogenic edema, increasing intracranial pressure and exacerbating brain injury (Corrigan et al., 2016).
3 Matrix metalloproteinases (MMPs)
Matrix metalloproteinases are zinc-dependent proteolytic enzymes that degrade the extracellular matrix (ECM). A variety of physiological processes rely on them, including tissue remodeling, embryogenesis, and wound healing (de Almeida et al., 2022). In the context of ischemic stroke, certain MMPs are implicated in the disruption of BBB by degrading TJPs and basal lamina components directly, which will promote HT and brain edema (Rempe et al., 2016). Besides, several MMPs are reported to cleave and activate various inflammatory mediators, exacerbating post-stroke inflammation, and further contributing to brain edema.
Of the MMPs, MMP-9 (gelatinase B) has been most prominently tied to BBB disruption following ischemic stroke degrading type IV and V collagens, which are significant components of the ECM’s basal lamina as well as tight junction proteins including occludin, claudins, and ZO-1 (Yang et al., 2020). This degradation weakens the structural integrity of BBB, making it more susceptible to disruption. Hence, upregulated levels of MMP-9 in the serum or CSF after stroke have been correlated with worse clinical outcomes and increased risk of hemorrhagic transformation and vasogenic edema (Rosell et al., 2008; Barr et al., 2010; Mechtouff et al., 2020). MMP-9 can also activate microglia and attract leukocytes to the site of injury, further promoting inflammation (Qiu et al., 2023). This inflammatory cascade can exacerbate brain edema. Additionally, MMP-9 contributes to cytotoxic edema by indirectly impairing cellular ion and water balance mechanisms. MMP-2 (gelatinase A) can degrade type IV collagens. Apart from its role in ECM degradation, MMP-2 can induce endothelial cell apoptosis (Zhang S. et al., 2018). Loss of endothelial cells further weakens the BBB structure and function. It’s constitutively expressed in the brain and can be upregulated after cerebral ischemia. MMP-3 (stromelysin-1) can activate other MMPs, including MMP-9, amplifying the proteolytic effects. It also has substrate specificity for various extracellular matrix components, especially proteoglycans and fibronectin, potentially contributing to BBB breakdown (Chung et al., 2013). Its role in BBB disruption has been studied in conditions like neuroinflammation and traumatic brain injury. The pro-inflammatory cytokines, especially TNF-α and IL-1β, can upregulate MMP expression via activation of transcription factors like NF-κB and AP-1. This creates a feedback loop wherein inflammation promotes MMP activity, and MMPs further augment inflammation (Huang et al., 2022).
Given their significant role in BBB disruption, MMPs, especially MMP-9, have become therapeutic targets for interventions aiming to reduce the complications of ischemic stroke. Some of the MMP inhibitors have been studied in the context of stroke. Batimastat (BB-94) is a broad-spectrum MMP inhibitor that has shown neuroprotective effects in animal models of stroke when administered either before or immediately after the onset of ischemia (Zhou et al., 2021). Despite this, poor bioavailability and potential side effects restrict its clinical application. Naturally occurring endogenous inhibitors of MMPs including TIMP-1 and TIMP-2 have been of particular interest in stroke research (Zieliñska-Turek et al., 2022). Overexpression of TIMPs or using recombinant TIMPs has shown neuroprotection in animal models of ischemic stroke. Though primarily an antibiotic, minocycline has anti-inflammatory properties and can inhibit MMPs, especially MMP-2 and MMP-9 (Switzer et al., 2011). Several preclinical studies have shown that minocycline can reduce infarct area and improve neurological outcomes in animal ischemic stroke models. Some clinical trials have also been undertaken, suggesting potential benefits, though more extensive studies are needed (Ortiz et al., 2020). Preclinical studies have shown that SB-3CT, a more selective inhibitor targeting MMP-2 and MMP-9, can reduce infarct size and preserve BBB integrity in animal models of ischemic stroke (Cai et al., 2017). However, it’s crucial to approach MMP inhibition with caution, as these enzymes also play roles in tissue repair and remodeling during the recovery phase.
4 Inflammatory markers
Stroke triggers an inflammatory cascade consisting of the activation of immune cells and the secretion of inflammatory mediators, such as cytokines, chemokines, and adhesion molecules (Chen S.-J. et al., 2022). These molecules promote leukocyte infiltration and increase vascular permeability, ultimately resulting in BBB disruption, vasogenic edema, hemorrhagic transformation, and worse neurological outcomes (Candelario-Jalil et al., 2022). Neuroinflammation can also lead to a positive feedback loop, where inflammation worsens BBB disruption and BBB disruption exacerbates inflammation.
4.1 Cytokines
Cytokines are small secreted proteins released by cells that play pivotal roles in mediating inflammatory reactions by affecting cell interaction and communications. After a stroke, there’s a rapid increase in the production of pro-inflammatory cytokines which affect endothelial cell function and integrity of BBB (Qiu et al., 2021). Increased BBB permeability allows the extravasation of blood cells, plasma proteins, and fluid into the brain parenchyma, leading to HT and vasogenic edema. Some cytokines may influence cellular ion balance and osmotic regulation, indirectly contributing to cytotoxic edema. Certain cytokines can activate astrocytes, which can exacerbate brain edema due to their role in water regulation within the CNS (Song et al., 2021).
A pro-inflammatory cytokine, IL-1β is among the earliest to be released after ischemic injury. Once released, active IL-1β binds to the IL-1 receptor (IL-1R) on various cell types, including endothelial cells, neurons, and glial cells. It triggers a cascade of inflammatory events by the NF-κB pathway, resulting in the further upregulation of other pro-inflammatory mediators and reactive oxygen species (ROS). IL-1β has been shown to alter the expression and function of TJPs, promoting MMP activity, and inducing endothelial cell activation, contributing to the development of HT and brain edema.
Tumor necrosis factor-alpha (TNF-α) can activate neighboring astrocytes, endothelial cells, and other microglia, propagating the inflammatory response. TNF-α increases the level of adhesion molecules, such as ICAM-1 and VCAM-1, on brain endothelial cells (Tang et al., 2011). This facilitates the adhesion and migration of peripheral immune cells, like neutrophils and monocytes, into the brain parenchyma. The binding of TNF-α to TNFR1 activates several downstream pathways, including NF-κB Pathway, MAPK Pathway, and Caspase Pathway. Elevated levels of TNF-α have been associated with brain edema formation and might increase the risk of HT by affecting tight junction proteins and promoting matrix metalloproteinase (MMP) activity through its signaling cascades (Chen et al., 2019).
Interleukin-6 (IL-6) levels rise following ischemic stroke from affected neurons, astrocytes, and microglia (Zhu et al., 2022). This initial release can further be augmented by other pro-inflammatory cytokines such as IL-1β and Interferon-gamma (IFN-γ) (Philipp et al., 2018). IL-6 binding to its receptor, IL-6R, further associates with the gp130 signaling protein and then activates various intracellular signaling cascades, including the JAK/STAT, MAPK, and PI3K/Akt pathways (Heinrich et al., 2003). IL-6 can contribute to BBB disruption by promoting endothelial cells into activation as well as increasing the expression of MMPs through its intracellular signaling pathways (Aliena-Valero et al., 2021). IL-6 can also modulate TJPs and contribute to the breakdown of the BBB. IL-6 has been suggested as a potential biomarker for a higher risk of developing HT and brain edema after ischemic stroke (Leasure et al., 2021). Regarding hemorrhagic stroke, higher IL-6 levels at admission are related to worse prognosis regarding function, hemorrhage volume, and edema (Leasure et al., 2021).
Interferon-gamma is primarily produced by T cells and natural killer cells and has both pro-inflammatory and immune-modulating effects (Zhang G. et al., 2018). In the context of stroke, IFN-γ can influence the production of adhesion molecules and MMPs breaking down BBB integrity. IL-6 and IFN-γ trigger the JAK-STAT pathway, leading to the expression of genes that contribute to BBB breakdown and inflammation (Zhu et al., 2021).
Transforming Growth Factor-beta (TGF-β) has been involved in both protective and detrimental effects in the context of cerebral ischemia. In response to the ischemic insult, latent TGF-β is activated. Activated TGF-β binds to its receptors, specifically, TGF-β type I and type II receptors (TβRI and TβRII), and activates the downstream Smad proteins, specifically Smad2 and Smad3. These proteins then form complexes with Smad4, translocate to the nucleus, and regulate target gene transcription (Zhang et al., 2019). TGF-β has been shown to enhance the integrity of the BBB by promoting the expression of tight junction protein occludin (Hu et al., 2023). This action can be protective against BBB breakdown, a critical factor in the development of HT and brain edema. However, chronic TGF-β activation can lead to vascular remodeling and fibrosis, potentially affecting vascular fragility (Mickael and Graham, 2019). Some studies suggest that TGF-β may increase the risk of HT, possibly by altering vascular integrity or promoting angiogenesis (Zhang et al., 2021).
Unlike the pro-inflammatory cytokines listed above, Interleukin-10 (IL-10) is primarily anti-inflammatory. IL-10 might help dampen the inflammatory response after ischemic stroke, thereby potentially reducing the risk of HT and brain edema (Piepke et al., 2021). Interleukin-4 (IL-4) and Interleukin-13 (IL-13) are typically associated with anti-inflammatory responses (Xu et al., 2020). They might play roles in shifting the immune response after stroke toward a more reparative, less inflammatory phenotype, potentially reducing the risk of HT and edema.
4.2 Chemokines
Chemokines are a subset of cytokines specifically involved in directing the migration of cells, particularly immune cells. Following ischemic stroke, chemokines are released in the brain, leading to immune cell recruitment and activation which can contribute to BBB breakdown. The disruption of the BBB potentially results in HT and vasogenic edema. Some chemokines might directly affect the permeability of brain endothelial cells or influence osmotic regulation, contributing to edema formation (Marchetti et al., 2022).
Monocyte Chemoattractant Protein-1 (MCP-1/CCL2) is one of the most studied chemokines in the pathophysiology of ischemic stroke (Zhao et al., 2020). MCP-1 recruits macrophages and monocytes to the injury site (Stowe et al., 2012). MCP-1 can activate astrocytes, the major cell type involved in maintaining the brain’s extracellular environment (Zhou M. et al., 2022). Activated astrocytes can lead to cytotoxic edema (Liu J. et al., 2023). Elevated levels have been associated with exacerbated brain injury and edema (Schuette-Nuetgen et al., 2012). CXCL2 (MIP-2α) can promote the disruption of the BBB by inducing endothelial cell activation and neutrophil recruitment, potentially leading to the extravasation of blood components and water, culminating in HT and vasogenic brain edema (Chen et al., 2021). While CXCL2 primarily acts on immune cells, there is the potential for direct effects on brain cells like neurons, astrocytes, and microglia (De Paola et al., 2007; La Cognata et al., 2023). Activated astrocytes and microglia can produce inflammatory mediators that can contribute to cytotoxic edema and neuroinflammation. CXCL8 (IL-8) also attracts neutrophils and has been linked to inflammation, BBB disruption, and subsequent brain edema (Lv et al., 2019). CXCL12 (SDF-1) has implications in ischemic brain injury and related complications such as HT and brain edema, Post-ischemia, CXCL12 is upregulated in the brain, acting as a chemoattractant for various immune cells, including monocytes, natural killer cells, and T cells, especially via its primary receptor, CXCR4 (Wang et al., 2012; Wang D. et al., 2023). These cells, once in the ischemic tissue, can release proteolytic enzymes, such as MMPs, which degrade the extracellular matrix and weaken the BBB. In addition, CXCL12 overexpression in ischemic tissues has been found to enhance bone marrow-derived cells (BMCs) recruitment from peripheral blood and then to induce angiogenesis (Zemani et al., 2008; Shiba et al., 2009; Nguyen et al., 2023). This process increases the risk of HT and brain edema. Continuous administration of the CXCR4 antagonist AMD3100 for 2 weeks can inhibit CXCL12-induced angiogenesis in a mouse model of stroke (Selvaraj et al., 2017).
Unique among chemokines, fractalkine (CX3CL1) exists in both membrane-bound and soluble forms. It’s involved in recruiting monocytes and microglia. In the context of ischemic stroke, fractalkine signaling has been associated with both neuroprotective and neurotoxic effects, and its role in HT and brain edema is still being elucidated (Cipriani et al., 2011).
4.3 Adhesion molecules
Adhesion molecules play a pivotal role in mediating the interaction between leukocytes and the endothelial cells of BBB during inflammatory processes (Supanc et al., 2011). In response to inflammatory signals, such as TNF-α and IL-1β, some adhesion molecules are upregulated on endothelial cells. The overexpression or increased activity of these molecules can lead to enhanced leukocyte infiltration into the brain parenchyma, potentially exacerbating inflammatory damage. In the context of ischemic stroke, this can contribute to complications like HT and brain edema. Here are some of the key adhesion molecules associated with these complications.
Intercellular Adhesion Molecule-1 (ICAM-1) on the endothelial cells facilitates the binding and transmigration of leukocytes, especially neutrophils, and monocytes, into the brain tissue (Huang et al., 2022; Witsch et al., 2023). ICAM-1 can bind integrins on leukocytes, facilitating their adhesion to the endothelium (Singh et al., 2023). The process of leukocyte transmigration, facilitated by ICAM-1, releases various mediators, including MMPs, and compromises the TJs between endothelial cells, leading to increased permeability of the BBB (Kunze et al., 2015). Overexpression of ICAM-1 has been linked to increased BBB permeability, inflammation, and consequent brain edema and HT (Simundic et al., 2004; Ma et al., 2011; Zhang et al., 2020). A recent study from China showed that an elevated level of serum ICAM-1 was related to the increased risk of HT after acute ischemic stroke (Wu et al., 2018).
Vascular Cell Adhesion Molecule-1 (VCAM-1) primarily participates in the adhesion and transmigration of monocytes (Sun et al., 2020; Bai et al., 2023). VCAM-1 interacts with its integrin counter-receptors (mainly VLA-4) found on leukocytes, promoting their adhesion to the vascular wall and transmigrating into the ischemic zone subsequently (Eidson et al., 2021; Gao et al., 2021; Bai et al., 2023). Leukocyte transmigration will disrupt BBB as previously mentioned. Its upregulation post-stroke potentially exacerbates HT and edema (Li B. et al., 2023).
P-selectin and E-selectin located on the surface of activated endothelial cells and platelets, can initiate the tethering and rolling of leukocytes on the endothelium which is a critical step preceding firm adhesion and transmigration (Geissmann et al., 2003; Liu S. et al., 2023). P-selectin is stored in the Weibel-Palade bodies of endothelial cells and the α-granules of platelets (Ling et al., 2023). In the event of a stroke, P-selectin is rapidly translocated to the cell surface, where it plays a key role in the initial tethering and rolling of leukocytes along the endothelium by interacting with its ligands (like PSGL-1) on leukocytes (Yu et al., 2023). E-selectin is distinctly inducible and expressed on endothelial cells in response to inflammatory cytokines, particularly TNF-α and IL-1 (Takeda et al., 2002). Following the initial tethering and rolling mediated by P-selectin and E-selectin, leukocytes become more activated, leading to firm adhesion [via other molecules like ICAM-1 and VCAM-1 and subsequent transmigration into the tissue (Richard et al., 2015; Hatchell et al., 2023)]. Higher levels of selectins have been associated with exacerbated post-stroke inflammation, BBB disruption, and potential complications like HT and brain edema (Jin et al., 2011; Hase et al., 2012; Salas-Perdomo et al., 2018; Li et al., 2020). Recently a prospective study demonstrated that higher serum E-selectin levels were statistically significantly associated with an increased risk of malignant brain edema in ischemic stroke patients receiving endovascular thrombectomy treatment (Hu et al., 2022; Zhou et al., 2023).
Platelet-Endothelial Cell Adhesion Molecule-1 (PECAM-1/CD31) is an immunoglobulin-like cell adhesion molecule expressed on the surface of endothelial cells, platelets, neutrophils, and some subsets of lymphocytes (Arias et al., 2022; Fu et al., 2023). It facilitates the final steps of leukocyte migration through the endothelial cell junctions post-stroke (Arias et al., 2022). PECAM-1 plays a role in transmitting survival signals in endothelial cells, preventing apoptosis (Gao et al., 2003). Any imbalance in PECAM-1 signaling might affect the stability of blood vessels, making them more susceptible to hemorrhagic events (Mahooti et al., 2000; Maeda et al., 2009). Besides, being expressed on platelets, PECAM-1 is involved in platelet aggregation and adhesion (Soriano Jerez et al., 2021). Dysregulation in platelet function can contribute to either thrombotic events or impaired clot stability, both of which might impact the risk of HT. Masashi Maeda et al. found that combined treatment of rt-PA with tacrolimus reduces HT, which might be due to its effects on PECAM-1/CD31 in thrombotic ischemia stroke rat model (Maeda et al., 2009). Its role in ischemic stroke and its complications is multifaceted and requires further investigation.
4.4 High mobility group box 1 (HMGB1)
High mobility group box 1, a nuclear protein, stabilizes nucleosome formation and promotes transcription under physiological conditions (Lotze and Tracey, 2005; Park et al., 2006). However, upon ischemic injury, HMGB1 can be released extracellularly by necrotic cells and function as a damage-associated molecular pattern (DAMP) molecule (Qiu et al., 2008; Mi et al., 2019; Chen R. et al., 2022). As a DAMP, HMGB1 binds to multiple receptors, such as the advanced glycation end-products (RAGE) receptors and toll-like receptors (TLRs), particularly TLR2 and TLR4 (Yang et al., 2010, 2011; Kim et al., 2011). The binding of HMGB1 to these receptors initiates intracellular signaling pathways that amplify inflammation (Banks et al., 2023). For example, nuclear factor-kappa B (NF-κB) and the subsequent transcription of various pro-inflammatory genes were activated afterward. This results in the production and secretion of pro-inflammatory cytokines including TNF-α, IL-1β, and IL-6 (Xie et al., 2019; Xu et al., 2023). HMGB1 can also promote the expression and activity of MMPs, especially MMP-9, thus altering the function of TJPs and consequently increasing BBB permeability (Richard et al., 2017). Therefore, HMGB1, via its inflammatory effects, plays a role in the formation of HT and vasogenic edema.
Anti-inflammatory agents might reduce the risk of HT and brain edema. Statins or 3-hydroxy-3-methylglutaryl coenzyme A (HMG-CoA) reductase inhibitors, such as Atorvastatin and Simvastatin, have anti-inflammatory and provide antioxidant properties beyond their lipid-lowering effects. Some researchers have suggested that early statin therapy might be beneficial in acute ischemic stroke (Thomas et al., 2023). Glycyrrhizin was suggested as an adjuvant therapy for avoiding HT by inhibiting the HMGB1/TLR2 signaling pathway (Chen et al., 2020). Corticosteroids are powerful anti-inflammatory agents and have been considered for use in various neurological conditions, especially in brain tumors, primarily to counteract brain edema and inflammation (Kast et al., 2021). However, the application of corticosteroids in stroke remains a topic of debate due to potential risks and the varied outcomes observed in studies. Anakinra, an IL-1 receptor antagonist (IL-1Ra), is primarily used for rheumatoid arthritis. Animal studies have suggested its potential neuroprotective effects when administered post-stroke (Sjöström et al., 2021; Venugopal et al., 2021). Natalizumab and Fingolimod are immunomodulators, primarily used for multiple sclerosis. They’ve shown promise in preclinical models of stroke, primarily by reducing the immune cell infiltration into the brain (Elkins et al., 2017; Elkind et al., 2020; Leigh et al., 2021; Ma et al., 2021; Malone et al., 2023).
5 Endothelial activation/dysfunction markers
Markers like von Willebrand factor (vWF) and Vascular Endothelial Growth Factor (VEGF) might be indicative of endothelial disruption or activation, potentially predicting the risk of HT and edema.
Von Willebrand factor is a multimeric glycoprotein that is produced in endothelial cells and megakaryocytes and stored in Weibel-Palade bodies of endothelial cells and α-granules of platelets (Zhu et al., 2016). Under conditions of vascular injury, such as that seen during an ischemic stroke, vWF binds to exposed subendothelial collagen facilitating platelet adhesion and aggregation (Menih et al., 2017). Given this, elevated vWF levels might be seen as protective against hemorrhage. However, the dynamic between vWF, thrombogenesis, reperfusion injury, and inflammation can create conditions conducive to BBB disruption and, subsequently, HT (Zhu et al., 2016). Patients with higher vWF levels post-stroke are at a higher risk of HT, especially following thrombolytic therapy (Zhang et al., 2023). The increased risk might be related to the concomitant BBB disruption and microvascular occlusions. The association of vWF with BBB integrity has implications for brain edema. Increased BBB permeability can lead to vasogenic edema due to the influx of serum proteins and fluid into the brain parenchyma (Zhu et al., 2016). Additionally, the thrombogenic role of vWF might contribute to cytotoxic edema by exacerbating ischemic injury.
Vascular Endothelial Growth Factor (VEGF) is a potent angiogenic factor (Liman and Endres, 2012). In the context of ischemic stroke, VEGF has dual and somewhat contradictory effects, with implications for both protective and detrimental outcomes, especially concerning HT and brain edema. HT can be promoted by VEGF due to its effects on vascular permeability and the creation of fragile, immature vessels and more permeable in the affected region (Zhang et al., 2000; Lai et al., 2022). In addition, VEGF increases vascular permeability by affecting the endothelial cell tight and adherens junctions, notably through its influence on occludin, claudin-5, and ZO-1 (Weis and Cheresh, 2005). As mentioned, the enhanced vascular permeability resulting from VEGF activity leads to the influx of serum proteins and fluids into the brain parenchyma, contributing to vasogenic edema (Weis and Cheresh, 2005; Lai et al., 2022). This kind of edema increases intracranial pressure, which can exacerbate neurological deficits and potentially lead to life-threatening conditions like herniation. Conversely, VEGF promotes post-stroke angiogenesis by stimulating the proliferation and migration of endothelial cells, which contribute to tissue repair and regeneration by enhancing blood flow to affected areas (Zhang and Chopp, 2002; Kaya et al., 2005). Beyond its vascular effects, VEGF has direct neuroprotective actions. It supports neuronal survival, stimulates neurogenesis, and enhances the function of neural progenitor cells (Jin et al., 2000; Sun et al., 2003). The overall balance between VEGF’s neuroprotective vs. its vascular actions can influence post-stroke outcomes, especially in the acute phase.
Delayed enhancement of VEGF signaling might be beneficial during the recovery phase, where angiogenesis and neuroprotection can support tissue repair and functional recovery. However, in the acute phase, especially in patients undergoing reperfusion strategies, VEGF inhibition might be considered to reduce the risk of HT and severe edema. Overall, understanding the temporal dynamics of VEGF expression and signaling post-stroke, as well as individual patient factors, will be crucial for developing effective therapeutic strategies centered on VEGF.
6 Oxidative stress
Oxidative stress is a condition characterized by an imbalance between reactive oxygen species (ROS) production and antioxidant defense systems (You et al., 2023). During reperfusion following an ischemic stroke, the reintroduction of blood flow causes a paradoxical surge in ROS production, exacerbating the injury (Wu et al., 2021; Hong et al., 2023). Oxidative stress damages the BBB by directly affecting the endothelial cells, pericytes, and basal lamina as well as activating MMPs, and inflammatory pathways indirectly (Shaheryar et al., 2023, 2023; Wang S. et al., 2023). A compromised BBB increases the risk of hemorrhagic transformation and vasogenic edema. Oxidative stress can reduce the availability of nitric oxide, a vasodilator, and impair endothelial function, making vessels more susceptible to injury and hemorrhage (Chen R. et al., 2023). Besides excessive ROS can damage cellular components, including lipids, proteins, and DNA (Ratliffe et al., 2023). This can disrupt cellular ion pumps leading to cellular swelling (Lipski et al., 2006). Oxidative stress can impact astrocyte function and the regulation of AQP4, influencing edema dynamics which will be described below in detail.
Antioxidants can help in mitigating the detrimental effects of oxidative stress. Edaravone, a free radical scavenger, has emerged as a therapeutic agent for ischemic stroke, particularly in some Asian countries (Ishihara and Suzuki, 2016; Wang et al., 2017). Its ability to neutralize ROS enables it to preserve the integrity of the BBB, potentially reducing vasogenic edema and the risk of hemorrhagic transformation. N-acetylcysteine (NAC) is a versatile compound with antioxidant properties that has been investigated for its potential neuroprotective effects in ischemic stroke (Zhou Z. et al., 2022; Chamorro et al., 2023). It serves as a precursor to glutathione, one of the primary intracellular antioxidants, and plays a critical role in scavenging free radicals, reducing oxidative stress, and modulating inflammatory processes (Alkandari et al., 2023). These features make NAC an intriguing candidate for addressing complications like post-stroke hemorrhagic transformation and brain edema. Vitamin E, as a lipid-soluble antioxidant, and Vitamin C, as a water-soluble antioxidant, have been extensively researched in the context of various health conditions, including its potential neuroprotective effects in stroke (Tang et al., 2022; Palakurti et al., 2023). Alpha-lipoic acid helps regenerate other antioxidants, like vitamins E and C and is involved in energy metabolism. Coenzyme Q10 (CoQ10), also known as ubiquinone, is a naturally occurring antioxidant found in almost every cell of the body. It participates in mitochondrial electron transport and also acts as a potent free radical scavenger (Crane, 2001). Due to its antioxidant and energy-promoting effects, CoQ10 holds promise as a potential therapeutic agent after stroke (Olga Nikolaevna et al., 2020). Melatonin is a hormone predominantly secreted by the pineal gland. Apart from its well-recognized role in sleep regulation, melatonin has garnered attention for its potent antioxidant, anti-inflammatory, and neuroprotective properties (Yawoot et al., 2023). These attributes have made it an intriguing candidate for various neuroprotective strategies, including those aimed at minimizing the aftermath of ischemic stroke (Li D. et al., 2023). While antioxidants offer a promising avenue for neuroprotection after stroke, further research, especially large-scale human trials, is needed to determine their efficacy, optimal timing, and dosing.
7 Aquaporin-4 (AQP4)
Aquaporin-4 is the predominant water channel in the CNS that has an insignificant effect on water homeostasis in the brain (Saadoun and Papadopoulos, 2010). Specifically, AQP4 is most abundantly located in the astrocyte foot processes that ensheath blood vessels, which is a strategic location for water transport in and out of the brain parenchyma (Han et al., 2015). The polarized distribution of AQP4 underscores its role in edema dynamics (Abbott et al., 2006). In the early phases after an ischemic stroke, the shortage of oxygen and nutrients, leading to cellular energy failure, causes failure of ion pumps, resulting in an accumulation of intracellular sodium, which subsequently draws water into cells (Chen Y.-Y. et al., 2023). AQP4 facilitates this water movement into neurons and astrocytes during this phase, leading to cell swelling, a hallmark of cytotoxic edema (Manley et al., 2000). In the later phases post-stroke, the upregulation of AQP4 expression aids in the leaking of plasma proteins and water into the interstitial space of the brain by disrupting the BBB integrity, exacerbating vasogenic edema (Datta et al., 2022). While AQP4 contributes to edema formation, it also affects edema resolution. Once the initial injury settles and repair mechanisms are activated, the excess water needs to be cleared from the brain. AQP4 assists in channeling this excess water back into the vasculature or toward the cerebrospinal fluid (CSF), helping in reducing edema (Hirt et al., 2009; Zador et al., 2009). After a stroke, ionic homeostasis is disturbed, creating osmotic gradients. AQP4 helps in equilibrating these gradients by allowing water to move in response to the osmotic differences.
Given the dual role of AQP4 in both the formation and resolution of post-stroke edema, therapeutic strategies targeting AQP4 need to be carefully timed and executed. During the early phase post-stroke, inhibiting AQP4 might help reduce cytotoxic edema (Sucha et al., 2022; Gono et al., 2023). TGN-020 is a selective AQP4 inhibitor. In animal models of ischemic stroke, administration of TGN-020 has been shown to reduce brain water content, suggesting its potential efficacy in reducing cerebral edema (Sun et al., 2022). Another approach to inhibit AQP4 is through RNA interference, targeting AQP4 mRNA and preventing its translation (Zhang et al., 2022). While RNAi technology offers specificity, its delivery and stability in vivo can be challenging. During recovery, promoting the AQP4 function might expedite the clearance of excess water, aiding in the resolution of vasogenic edema. To date, there aren’t many specific agents known to directly activate AQP4.
8 Soluble molecular markers of BBB leakage
When the BBB becomes compromised, several soluble molecules can be detected at altered levels or locations.
S100β is a small calcium-binding protein predominantly found in astrocytes within the CNS (Kleindienst et al., 2007). On account of its location and the specificity of its distribution, S100β has garnered interest as a potential biomarker for BBB integrity and astrocyte-related brain injury. Under normal physiological conditions, the levels of S100β in the bloodstream are relatively low due to the restrictive nature of the BBB. When the BBB is compromised, such as during trauma, inflammation, stroke, or after certain therapeutic interventions, there’s a heightened permeability that allows S100β to leak from the brain into the bloodstream (Taylor et al., 2022). Therefore, elevated serum levels of S100β might be indicative of BBB disruption and the potential for edema. A recent study found that pre-administration with Leonuri Herba Total Alkali (LHA) could significantly reduce neurological deficit scores, infarction volume, the serum levels of S100β (Li et al., 2021).
Albumin is normally restricted from entering the brain by the BBB. By measuring the ratio of albumin in the cerebrospinal fluid (CSF) to that in the blood (often referred to as the CSF/serum albumin quotient or Qalb), clinicians can gauge the degree of BBB disruption. An in vivo experiment showed that albumin aggravates cerebral edema in rats with ischemic stroke by increasing BBB permeability (Li C. et al., 2022).
Recognizing and understanding these above markers allows for better diagnostic, prognostic, and therapeutic strategies.
9 Unresolved questions and future directions
Essentially, deeper investigations into the mechanism of these markers, elucidating how they interact with cellular components and signaling pathways, must be conducted. Developing selective inhibitors of specific markers is crucial to avoid off-target effects and potential toxicity. Besides, longitudinal studies exploring the temporal dynamics of these markers will illustrate their role across different stages after stroke, determining the optimal timing of interventions. Most importantly, conducting clinical trials to assess the utility and reliability of these markers in predicting and monitoring hemorrhagic transformation and brain edema in stroke patients is pivotal for bridging the translational gap. Research exploring individual genetic, epigenetic, and environmental factors affecting marker expression and response will facilitate the development of personalized medicine approaches for stroke patients. Moreover, investigating the synergistic effects of multi-target interventions can provide a comprehensive approach to mitigating the complex pathological processes involved in post-stroke complications. Of course, there are other yet unidentified markers of hemorrhagic transformation and brain edema post-stroke that need further study. Addressing these unresolved questions and exploring the proposed future directions will significantly advance the field, providing innovative and effective strategies for managing post-stroke hemorrhagic transformation and brain edema.
10 Conclusion
Post-stroke HT and brain edema are serious complications following ischemic stroke, resulting in increased morbidity and mortality rates. This review highlights the recent advancements in diagnostic markers and therapeutic targets for these complications. By better understanding the underlying mechanisms and identifying novel diagnostic tools and therapeutic strategies, we can improve patient outcomes and reduce the burden of post-stroke HT and brain edema. Rigorous clinical trials and translational studies are paramount to bring these innovations from bench to bedside.
Author contributions
YiY: Writing – original draft. FL: Writing – original draft. ZG: Writing – review and editing. JW: Writing – review and editing. LX: Writing – review and editing. YuY: Writing – review and editing. JC: Writing – review and editing. RR: Writing – review and editing.
Funding
The author(s) declare financial support was received for the research, authorship, and/or publication of this article. This work was supported by National Natural Science Foundation of China (No. 82272584) and Zhejiang Provincial Natural Science Foundation of China (No. LQ23H090009).
Acknowledgments
We thank platform Figdraw for drawing the figure.
Conflict of interest
The authors declare that the research was conducted in the absence of any commercial or financial relationships that could be construed as a potential conflict of interest.
Publisher’s note
All claims expressed in this article are solely those of the authors and do not necessarily represent those of their affiliated organizations, or those of the publisher, the editors and the reviewers. Any product that may be evaluated in this article, or claim that may be made by its manufacturer, is not guaranteed or endorsed by the publisher.
References
Abbott, N. J., Rönnbäck, L., and Hansson, E. (2006). Astrocyte-endothelial interactions at the blood-brain barrier. Nat. Rev. Neurosci. 7, 41–53. doi: 10.1038/nrn1824
Abdullahi, W., Tripathi, D., and Ronaldson, P. T. (2018). Blood-brain barrier dysfunction in ischemic stroke: targeting tight junctions and transporters for vascular protection. Am. J. Physiol. Cell Physiol. 315, C343–C356. doi: 10.1152/ajpcell.00095.2018
Aliena-Valero, A., Rius-Pérez, S., Baixauli-Martín, J., Torregrosa, G., Chamorro, Á, Pérez, S., et al. (2021). Uric acid neuroprotection associated to IL-6/STAT3 signaling pathway activation in rat ischemic stroke. Mol. Neurobiol. 58, 408–423. doi: 10.1007/s12035-020-02115-w
Alkandari, A. F., Madhyastha, S., and Rao, M. S. (2023). N-acetylcysteine amide against Aβ-induced Alzheimer’s-like pathology in rats. Int. J. Mol. Sci. 24:12733. doi: 10.3390/ijms241612733
Arias, E., Nadkarni, N., Fang, R., Haynes, M., Batra, A., Muller, W., et al. (2022). Inhibition of PECAM-1 significantly delays leukocyte extravasation into the subcortex post-stroke. FASEB J. 36. doi: 10.1096/fasebj.2022.36.S1.R5646
Babinska, A., Clement, C. C., Li, Y., Wzorek, J., Przygodzki, T., Talar, M., et al. (2020). In vivo data: treatment with the F11R/JAM-A peptide 4D decreases mortality and reduces the generation of atherosclerotic plaques in ApoE-deficient mice. Data Brief 30:105516. doi: 10.1016/j.dib.2020.105516
Bai, M., Sun, R., Cao, B., Feng, J., and Wang, J. (2023). Monocyte-related cytokines/chemokines in cerebral ischemic stroke. CNS Neurosci. Ther. 29, 3693–3712. doi: 10.1111/cns.14368
Banks, W. A., Hansen, K. M., Erickson, M. A., and Crews, F. T. (2023). High-mobility group box 1 (HMGB1) crosses the BBB bidirectionally. Brain Behav. Immun. 111, 386–394. doi: 10.1016/j.bbi.2023.04.018
Barr, T. L., Latour, L. L., Lee, K.-Y., Schaewe, T. J., Luby, M., Chang, G. S., et al. (2010). Blood-brain barrier disruption in humans is independently associated with increased matrix metalloproteinase-9. Stroke 41, e123–e128. doi: 10.1161/STROKEAHA.109.570515
Beez, T., Munoz-Bendix, C., Steiger, H.-J., and Beseoglu, K. (2019). Decompressive craniectomy for acute ischemic stroke. Crit. Care (London, England) 23:209. doi: 10.1186/s13054-019-2490-x
Berndt, P., Winkler, L., Cording, J., Breitkreuz-Korff, O., Rex, A., Dithmer, S., et al. (2019). Tight junction proteins at the blood-brain barrier: far more than claudin-5. Cell. Mol. Life Sci. 76, 1987–2002. doi: 10.1007/s00018-019-03030-7
Cai, H., Ma, Y., Jiang, L., Mu, Z., Jiang, Z., Chen, X., et al. (2017). Hypoxia response element-regulated MMP-9 promotes neurological recovery via glial scar degradation and angiogenesis in delayed stroke. Mol. Ther. 25, 1448–1459. doi: 10.1016/j.ymthe.2017.03.020
Campbell, B. C. V., De Silva, D. A., Macleod, M. R., Coutts, S. B., Schwamm, L. H., Davis, S. M., et al. (2019). Ischaemic stroke. Nat. Rev. Dis. Primers 5:70. doi: 10.1038/s41572-019-0118-8
Candelario-Jalil, E., Dijkhuizen, R. M., and Magnus, T. (2022). Neuroinflammation, stroke, blood-brain barrier dysfunction, and imaging modalities. Stroke 53, 1473–1486. doi: 10.1161/STROKEAHA.122.036946
Chamorro, B., Izquierdo-Bermejo, S., Martín-de-Saavedra, M. D., López-Muñoz, F., Chioua, M., Marco-Contelles, J., et al. (2023). Neuroprotective and antioxidant properties of CholesteroNitrone ChN2 and QuinolylNitrone QN23 in an experimental model of cerebral ischemia: involvement of necrotic and apoptotic cell death. Antioxidants (Basel, Switzerland) 12:1364. doi: 10.3390/antiox12071364
Chen, A.-Q., Fang, Z., Chen, X.-L., Yang, S., Zhou, Y.-F., Mao, L., et al. (2019). Microglia-derived TNF-α mediates endothelial necroptosis aggravating blood brain-barrier disruption after ischemic stroke. Cell Death Dis. 10:487. doi: 10.1038/s41419-019-1716-9
Chen, H., Guan, B., Wang, B., Pu, H., Bai, X., Chen, X., et al. (2020). Glycyrrhizin prevents hemorrhagic transformation and improves neurological outcome in ischemic stroke with delayed thrombolysis through targeting peroxynitrite-mediated HMGB1 signaling. Transl. Stroke Res. 11, 967–982. doi: 10.1007/s12975-019-00772-1
Chen, J., Jin, J., Zhang, X., Yu, H., Zhu, X., Yu, L., et al. (2021). Microglial lnc-U90926 facilitates neutrophil infiltration in ischemic stroke via MDH2/CXCL2 axis. Mol. Ther. 29, 2873–2885. doi: 10.1016/j.ymthe.2021.04.025
Chen, R., Kang, R., and Tang, D. (2022). The mechanism of HMGB1 secretion and release. Exp. Mol. Med. 54, 91–102. doi: 10.1038/s12276-022-00736-w
Chen, R., Qian, L., Fu, J., Qin, J., Chen, X., and Xu, X. (2023). Downregulation of Preso protects against ischemic/reperfusion-mediated neuronal injury through regulating PSD95-nNOS/YAP pathways. Neurochem. Int. 169:105586. doi: 10.1016/j.neuint.2023.105586
Chen, S.-J., Yuan, X.-Q., Xue, Q., Lu, H.-F., and Chen, G. (2022). Current research progress of isoflurane in cerebral ischemia/reperfusion injury: a narrative review. Med. Gas Res. 12, 73–76. doi: 10.4103/2045-9912.330689
Chen, Y.-Y., Gong, Z.-C., Zhang, M.-M., and Huang, Z.-H. (2023). Brain-targeting emodin mitigates ischemic stroke via inhibiting AQP4-mediated swelling and neuroinflammation. Transl. Stroke Res. doi: 10.1007/s12975-023-01170-4 [Epub ahead of print].
Chung, Y. C., Kim, Y.-S., Bok, E., Yune, T. Y., Maeng, S., and Jin, B. K. (2013). MMP-3 contributes to nigrostriatal dopaminergic neuronal loss, BBB damage, and neuroinflammation in an MPTP mouse model of Parkinson’s disease. Mediators Inflamm. 2013:370526. doi: 10.1155/2013/370526
Cipriani, R., Villa, P., Chece, G., Lauro, C., Paladini, A., Micotti, E., et al. (2011). CX3CL1 is neuroprotective in permanent focal cerebral ischemia in rodents. J. Neurosci. 31, 16327–16335. doi: 10.1523/JNEUROSCI.3611-11.2011
Corrigan, F., Mander, K. A., Leonard, A. V., and Vink, R. (2016). Neurogenic inflammation after traumatic brain injury and its potentiation of classical inflammation. J. Neuroinflammation 13:264. doi: 10.1186/s12974-016-0738-9
Crane, F. L. (2001). Biochemical functions of coenzyme Q10. J. Am. Coll. Nutr. 20, 591–598. doi: 10.1080/07315724.2001.10719063
Datta, A., Sarmah, D., Kaur, H., Chaudhary, A., Mounica, K. L., Kalia, K., et al. (2022). Post-stroke impairment of the blood-brain barrier and perifocal vasogenic edema is alleviated by endovascular mesenchymal stem cell administration: modulation of the PKCδ/MMP9/AQP4-mediated pathway. Mol. Neurobiol. 59, 2758–2775. doi: 10.1007/s12035-022-02761-2
de Almeida, L. G. N., Thode, H., Eslambolchi, Y., Chopra, S., Young, D., Gill, S., et al. (2022). Matrix metalloproteinases: from molecular mechanisms to physiology, pathophysiology, and pharmacology. Pharmacol. Rev. 74, 712–768. doi: 10.1124/pharmrev.121.000349
De Paola, M., Buanne, P., Biordi, L., Bertini, R., Ghezzi, P., and Mennini, T. (2007). Chemokine MIP-2/CXCL2, acting on CXCR2, induces motor neuron death in primary cultures. Neuroimmunomodulation 14, 310–316. doi: 10.1159/000123834
Eidson, L. N., Gao, Q., Qu, H., Kikuchi, D. S., Campos, A. C. P., Faidley, E. A., et al. (2021). Poldip2 controls leukocyte infiltration into the ischemic brain by regulating focal adhesion kinase-mediated VCAM-1 induction. Sci. Rep. 11:5533. doi: 10.1038/s41598-021-84987-z
Elkind, M. S. V., Veltkamp, R., Montaner, J., Johnston, S. C., Singhal, A. B., Becker, K., et al. (2020). Natalizumab in acute ischemic stroke (ACTION II): a randomized, placebo-controlled trial. Neurology 95, e1091–e1104. doi: 10.1212/WNL.0000000000010038
Elkins, J., Veltkamp, R., Montaner, J., Johnston, S. C., Singhal, A. B., Becker, K., et al. (2017). Safety and efficacy of natalizumab in patients with acute ischaemic stroke (ACTION): a randomised, placebo-controlled, double-blind phase 2 trial. Lancet Neurol. 16, 217–226. doi: 10.1016/S1474-4422(16)30357-X
Fu, T., Sullivan, D. P., Gonzalez, A. M., Haynes, M. E., Dalal, P. J., Rutledge, N. S., et al. (2023). Mechanotransduction via endothelial adhesion molecule CD31 initiates transmigration and reveals a role for VEGFR2 in diapedesis. Immunity 56, 2311–2324.e6. doi: 10.1016/j.immuni.2023.08.001
Gao, C., Jia, W., Xu, W., Wu, Q., and Wu, J. (2021). Downregulation of CD151 restricts VCAM-1 mediated leukocyte infiltration to reduce neurobiological injuries after experimental stroke. J. Neuroinflammation 18:118. doi: 10.1186/s12974-021-02171-6
Gao, C., Sun, W., Christofidou-Solomidou, M., Sawada, M., Newman, D. K., Bergom, C., et al. (2003). PECAM-1 functions as a specific and potent inhibitor of mitochondrial-dependent apoptosis. Blood 102, 169–179. doi: 10.1182/blood-2003-01-0003
Gao, P., and Shivers, R. R. (2004). Correlation of the presence of blood-brain barrier tight junctions and expression of zonula occludens protein ZO-1 in vitro: a freeze-fracture and immunofluorescence study. J. Submicrosc. Cytol. Pathol. 36, 7–15.
Geissmann, F., Jung, S., and Littman, D. R. (2003). Blood monocytes consist of two principal subsets with distinct migratory properties. Immunity 19, 71–82. doi: 10.1016/s1074-7613(03)00174-2
Gono, R., Sugimoto, K., Yang, C., Murata, Y., Nomura, R., Shirazaki, M., et al. (2023). Molecular mechanism of cerebral edema improvement via IL-1RA released from the stroke-unaffected hindlimb by treadmill exercise after cerebral infarction in rats. J. Cereb. Blood Flow Metab. 43, 812–827. doi: 10.1177/0271678X231151569
Haarmann, A., Deiss, A., Prochaska, J., Foerch, C., Weksler, B., Romero, I., et al. (2010). Evaluation of soluble junctional adhesion molecule-A as a biomarker of human brain endothelial barrier breakdown. PLoS One 5:e13568. doi: 10.1371/journal.pone.0013568
Han, D., Sun, M., He, P.-P., Wen, L.-L., Zhang, H., and Feng, J. (2015). Ischemic postconditioning alleviates brain edema after focal cerebral ischemia reperfusion in rats through down-regulation of aquaporin-4. J. Mol. Neurosci. 56, 722–729. doi: 10.1007/s12031-015-0504-y
Hase, Y., Okamoto, Y., Fujita, Y., Kitamura, A., Nakabayashi, H., Ito, H., et al. (2012). Cilostazol, a phosphodiesterase inhibitor, prevents no-reflow and hemorrhage in mice with focal cerebral ischemia. Exp. Neurol. 233, 523–533. doi: 10.1016/j.expneurol.2011.11.038
Hatchell, D., Alshareef, M., Vasas, T., Guglietta, S., Borucki, D., Guo, C., et al. (2023). A role for P-selectin and complement in the pathological sequelae of germinal matrix hemorrhage. J. Neuroinflammation 20:143. doi: 10.1186/s12974-023-02828-4
Heinrich, P. C., Behrmann, I., Haan, S., Hermanns, H. M., Müller-Newen, G., and Schaper, F. (2003). Principles of interleukin (IL)-6-type cytokine signalling and its regulation. Biochem. J. 374, 1–20. doi: 10.1042/BJ20030407
Hirt, L., Ternon, B., Price, M., Mastour, N., Brunet, J.-F., and Badaut, J. (2009). Protective role of early aquaporin 4 induction against postischemic edema formation. J. Cereb. Blood Flow Metab. 29, 423–433. doi: 10.1038/jcbfm.2008.133
Hong, J. M., Kim, D. S., and Kim, M. (2021). Hemorrhagic transformation after ischemic stroke: mechanisms and management. Front. Neurol. 12:703258. doi: 10.3389/fneur.2021.703258
Hong, W.-M., Xie, Y.-W., Zhao, M.-Y., Yu, T.-H., Wang, L.-N., Xu, W.-Y., et al. (2023). Vasoprotective effects of hyperoside against cerebral ischemia/reperfusion injury in rats: activation of large-conductance Ca-activated K channels. Neural Plast. 2023:5545205. doi: 10.1155/2023/5545205
Hu, R., Zhang, D., Hu, Z., Chen, Y., and Li, L. (2022). Serum inflammatory cell adhesion molecules predict malignant cerebral edema and clinical outcome early after mechanical thrombectomy in stroke. Clin. Neurol. Neurosurg. 223:107507. doi: 10.1016/j.clineuro.2022.107507
Hu, X., Dong, J., Geng, P., Sun, Y., Du, W., Zhao, X., et al. (2023). Nicotine treatment ameliorates blood-brain barrier damage after acute ischemic stroke by regulating endothelial scaffolding protein Pdlim5. Transl. Stroke Res. doi: 10.1007/s12975-023-01158-0 [Epub ahead of print].
Huang, L., Chen, Y., Liu, R., Li, B., Fei, X., Li, X., et al. (2022). P-glycoprotein aggravates blood brain barrier dysfunction in experimental ischemic stroke by inhibiting endothelial autophagy. Aging Dis. 13, 1546–1561. doi: 10.14336/AD.2022.0225
Huang, P., Zhou, C.-M., Qin-Hu, Liu, Y.-Y., Hu, B.-H., Chang, X., et al. (2012). Cerebralcare Granule® attenuates blood-brain barrier disruption after middle cerebral artery occlusion in rats. Exp. Neurol. 237, 453–463. doi: 10.1016/j.expneurol.2012.07.017
Ishihara, H., and Suzuki, M. (2016). [Japanese Guidelines for the Management of Stroke 2015: overview of the chapter on Subarachnoid Hemorrhage]. Nihon Rinsho 74, 677–680.
Ji, C., Yu, X., Xu, W., Lenahan, C., Tu, S., and Shao, A. (2021). The role of glymphatic system in the cerebral edema formation after ischemic stroke. Exp. Neurol. 340:113685. doi: 10.1016/j.expneurol.2021.113685
Jickling, G. C., Liu, D., Stamova, B., Ander, B. P., Zhan, X., Lu, A., et al. (2014). Hemorrhagic transformation after ischemic stroke in animals and humans. J. Cereb. Blood Flow Metab. 34, 185–199. doi: 10.1038/jcbfm.2013.203
Jin, K. L., Mao, X. O., and Greenberg, D. A. (2000). Vascular endothelial growth factor: direct neuroprotective effect in in vitro ischemia. Proc. Natl. Acad. Sci. U.S.A. 97, 10242–10247. doi: 10.1073/pnas.97.18.10242
Jin, R., Song, Z., Yu, S., Piazza, A., Nanda, A., Penninger, J. M., et al. (2011). Phosphatidylinositol-3-kinase gamma plays a central role in blood-brain barrier dysfunction in acute experimental stroke. Stroke 42, 2033–2044. doi: 10.1161/STROKEAHA.110.601369
Kast, R. E., Burns, T. C., and Halatsch, M.-E. (2021). Short review of SEC, a potential dexamethasone-sparing regimen for glioblastoma: spironolactone, ecallantide, clotrimazole. Neurochirurgie 67, 508–515. doi: 10.1016/j.neuchi.2020.12.008
Kaya, D., Gürsoy-Ozdemir, Y., Yemisci, M., Tuncer, N., Aktan, S., and Dalkara, T. (2005). VEGF protects brain against focal ischemia without increasing blood–brain permeability when administered intracerebroventricularly. J. Cereb. Blood Flow Metab. 25, 1111–1118. doi: 10.1038/sj.jcbfm.9600109
Kim, K.-A., Kim, D., Kim, J.-H., Shin, Y.-J., Kim, E.-S., Akram, M., et al. (2020). Autophagy-mediated occludin degradation contributes to blood-brain barrier disruption during ischemia in bEnd.3 brain endothelial cells and rat ischemic stroke models. Fluids Barriers CNS 17:21. doi: 10.1186/s12987-020-00182-8
Kim, S.-W., Lim, C.-M., Kim, J.-B., Shin, J.-H., Lee, S., Lee, M., et al. (2011). Extracellular HMGB1 released by NMDA treatment confers neuronal apoptosis via RAGE-p38 MAPK/ERK signaling pathway. Neurotox. Res. 20, 159–169. doi: 10.1007/s12640-010-9231-x
Kleindienst, A., Hesse, F., Bullock, M. R., and Buchfelder, M. (2007). The neurotrophic protein S100B: value as a marker of brain damage and possible therapeutic implications. Prog. Brain Res. 161, 317–325. doi: 10.1016/S0079-6123(06)61022-4
Kunze, R., Urrutia, A., Hoffmann, A., Liu, H., Helluy, X., Pham, M., et al. (2015). Dimethyl fumarate attenuates cerebral edema formation by protecting the blood-brain barrier integrity. Exp. Neurol. 266, 99–111. doi: 10.1016/j.expneurol.2015.02.022
La Cognata, V., D’Amico, A. G., Maugeri, G., Morello, G., Guarnaccia, M., Magrì, B., et al. (2023). CXCR2 is deregulated in ALS spinal cord and its activation triggers apoptosis in motor neuron-like cells overexpressing hSOD1-G93A. Cells 12:1813. doi: 10.3390/cells12141813
Lai, A. K. W., Ng, T. C., Hung, V. K. L., Tam, K. C., Cheung, C. W., Chung, S. K., et al. (2022). Exacerbated VEGF up-regulation accompanies diabetes-aggravated hemorrhage in mice after experimental cerebral ischemia and delayed reperfusion. Neural Regen. Res. 17, 1566–1575. doi: 10.4103/1673-5374.330612
Leasure, A. C., Kuohn, L. R., Vanent, K. N., Bevers, M. B., Kimberly, W. T., Steiner, T., et al. (2021). Association of serum IL-6 (Interleukin 6) with functional outcome after intracerebral hemorrhage. Stroke 52, 1733–1740. doi: 10.1161/STROKEAHA.120.032888
Leigh, R., Hitomi, E., Hutchison, R. M., and Elkins, J. (2021). Post-stroke blood-brain barrier disruption predicts poor outcome in patients enrolled in the ACTION study. J. Neuroimaging 31, 751–757. doi: 10.1111/jon.12862
Li, B., Xiong, J., Liu, H.-X., Li, D., and Chen, G. (2022). Devil or angel: two roles of carbon monoxide in stroke. Med. Gas Res. 12, 125–130. doi: 10.4103/2045-9912.337993
Li, B., Zhang, B., Li, Z., Li, S., Li, J., Wang, A., et al. (2023). Ginkgolide C attenuates cerebral ischemia/reperfusion-induced inflammatory impairments by suppressing CD40/NF-κB pathway. J. Ethnopharmacol. 312:116537. doi: 10.1016/j.jep.2023.116537
Li, C., Zhang, S., Jiang, X., Li, Z., Zhang, Y., Li, X., et al. (2022). Human albumin aggravates cerebral edema by disrupting the blood-brain barrier in a rat model of ischemic stroke. Acta Neurobiol. Exp. (Wars) 82, 284–294. doi: 10.55782/ane-2022-027
Li, D., He, T., Zhang, Y., Liu, J., Zhao, H., Wang, D., et al. (2023). Melatonin regulates microglial polarization and protects against ischemic stroke-induced brain injury in mice. Exp. Neurol. 367:114464. doi: 10.1016/j.expneurol.2023.114464
Li, Y., Wang, C., Zhang, L., Chen, B., Mo, Y., and Zhang, J. (2022). Claudin-5a is essential for the functional formation of both zebrafish blood-brain barrier and blood-cerebrospinal fluid barrier. Fluids Barriers CNS 19:40. doi: 10.1186/s12987-022-00337-9
Li, Y., Xiang, L., Wang, C., Song, Y., Miao, J., and Miao, M. (2021). Protection against acute cerebral ischemia/reperfusion injury by Leonuri Herba Total Alkali via modulation of BDNF-TrKB-PI3K/Akt signaling pathway in rats. Biomed. Pharmacother. 133:111021. doi: 10.1016/j.biopha.2020.111021
Li, Z., Xiao, G., Lyu, M., Wang, Y., He, S., Du, H., et al. (2020). Shuxuening injection facilitates neurofunctional recovery via down-regulation of G-CSF-mediated granulocyte adhesion and diapedesis pathway in a subacute stroke mouse model. Biomed. Pharmacother. 127:110213. doi: 10.1016/j.biopha.2020.110213
Liman, T. G., and Endres, M. (2012). New vessels after stroke: postischemic neovascularization and regeneration. Cerebrovasc. Dis. 33, 492–499. doi: 10.1159/000337155
Ling, T., Liu, J., Dong, L., and Liu, J. (2023). The roles of P-selectin in cancer cachexia. Med. Oncol. 40:338. doi: 10.1007/s12032-023-02207-2
Lipski, J., Park, T. I. H., Li, D., Lee, S. C. W., Trevarton, A. J., Chung, K. K. H., et al. (2006). Involvement of TRP-like channels in the acute ischemic response of hippocampal CA1 neurons in brain slices. Brain Res. 1077, 187–199. doi: 10.1016/j.brainres.2006.01.016
Liu, J., Guo, Y., Zhang, Y., Zhao, X., Fu, R., Hua, S., et al. (2023). Astrocytes in ischemic stroke: crosstalk in central nervous system and therapeutic potential. Neuropathology doi: 10.1111/neup.12928 [Epub ahead of print].
Liu, S., Zhang, Z., He, Y., Kong, L., Jin, Q., Qi, X., et al. (2023). Inhibiting leukocyte-endothelial cell interactions by Chinese medicine Tongxinluo capsule alleviates no-reflow after arterial recanalization in ischemic stroke. CNS Neurosci. Ther. 29, 3014–3030. doi: 10.1111/cns.14242
Lotze, M. T., and Tracey, K. J. (2005). High-mobility group box 1 protein (HMGB1): nuclear weapon in the immune arsenal. Nat. Rev. Immunol. 5, 331–342. doi: 10.1038/nri1594
Lv, H., Li, J., and Che, Y.-Q. (2019). CXCL8 gene silencing promotes neuroglial cells activation while inhibiting neuroinflammation through the PI3K/Akt/NF-κB-signaling pathway in mice with ischemic stroke. J. Cell. Physiol. 234, 7341–7355. doi: 10.1002/jcp.27493
Lv, J., Hu, W., Yang, Z., Li, T., Jiang, S., Ma, Z., et al. (2018). Focusing on claudin-5: a promising candidate in the regulation of BBB to treat ischemic stroke. Prog. Neurobiol. 161, 79–96. doi: 10.1016/j.pneurobio.2017.12.001
Ma, G., Pan, Z., Kong, L., and Du, G. (2021). Neuroinflammation in hemorrhagic transformation after tissue plasminogen activator thrombolysis: potential mechanisms, targets, therapeutic drugs and biomarkers. Int. Immunopharmacol. 90:107216. doi: 10.1016/j.intimp.2020.107216
Ma, Q., Manaenko, A., Khatibi, N. H., Chen, W., Zhang, J. H., and Tang, J. (2011). Vascular adhesion protein-1 inhibition provides antiinflammatory protection after an intracerebral hemorrhagic stroke in mice. J. Cereb. Blood Flow Metab. 31, 881–893. doi: 10.1038/jcbfm.2010.167
Maeda, M., Furuichi, Y., Noto, T., Matsuoka, N., Mutoh, S., and Yoneda, Y. (2009). Tacrolimus (FK506) suppresses rt-PA-induced hemorrhagic transformation in a rat thrombotic ischemia stroke model. Brain Res. 1254, 99–108. doi: 10.1016/j.brainres.2008.11.080
Mahooti, S., Graesser, D., Patil, S., Newman, P., Duncan, G., Mak, T., et al. (2000). PECAM-1 (CD31) expression modulates bleeding time in vivo. Am. J. Pathol. 157, 75–81. doi: 10.1016/S0002-9440(10)64519-1
Malone, K., Shearer, J. A., Waeber, C., and Moore, A. C. (2023). The impact of fingolimod on Treg function in brain ischaemia. Eur. J. Immunol. 53:e2350370. doi: 10.1002/eji.202350370
Manley, G. T., Fujimura, M., Ma, T., Noshita, N., Filiz, F., Bollen, A. W., et al. (2000). Aquaporin-4 deletion in mice reduces brain edema after acute water intoxication and ischemic stroke. Nat. Med. 6, 159–163. doi: 10.1038/72256
Marchetti, L., Francisco, D., Soldati, S., Haghayegh Jahromi, N., Barcos, S., Gruber, I., et al. (2022). ACKR1 favors transcellular over paracellular T-cell diapedesis across the blood-brain barrier in neuroinflammation in vitro. Eur. J. Immunol. 52, 161–177. doi: 10.1002/eji.202149238
Mechtouff, L., Bochaton, T., Paccalet, A., Crola Da Silva, C., Buisson, M., Amaz, C., et al. (2020). Matrix metalloproteinase-9 and monocyte chemoattractant protein-1 are associated with collateral status in acute ischemic stroke with large vessel occlusion. Stroke 51, 2232–2235. doi: 10.1161/STROKEAHA.120.029395
Menih, M., Križmarić, M., and Hojs Fabjan, T. (2017). Clinical role of von Willebrand factor in acute ischemic stroke. Wien. Klin. Wochenschr. 129, 491–496.
Mi, L., Zhang, Y., Xu, Y., Zheng, X., Zhang, X., Wang, Z., et al. (2019). HMGB1/RAGE pro-inflammatory axis promotes vascular endothelial cell apoptosis in limb ischemia/reperfusion injury. Biomed. Pharmacother. 116:109005. doi: 10.1016/j.biopha.2019.109005
Mickael, C. S., and Graham, B. B. (2019). The role of Type 2 inflammation in schistosoma-induced pulmonary hypertension. Front. Immunol. 10:27. doi: 10.3389/fimmu.2019.00027
Nguyen, J. N., Mohan, E. C., Pandya, G., Ali, U., Tan, C., Kofler, J. K., et al. (2023). CD13 facilitates immune cell migration and aggravates acute injury but promotes chronic post-stroke recovery. J. Neuroinflammation 20:232. doi: 10.1186/s12974-023-02918-3
Olga Nikolaevna, O., Evgeniya Aronovna, G., Elena Igorevna, K., Margarita Alekseevna, B., Mikhail Vladimirovich, G., Valery Gennadievich, M., et al. (2020). Intravenous administration of coenzyme Q10 in acute period of cerebral ischemia decreases mortality by reducing brain necrosis and limiting its increase within 4 days in rat stroke model. Antioxidants (Basel, Switzerland) 9:1240. doi: 10.3390/antiox9121240
Ortiz, J. F., Ruxmohan, S., Saxena, A., Morillo Cox, Á, Bashir, F., Tambo, W., et al. (2020). Minocycline and magnesium as neuroprotective agents for ischemic stroke: a systematic review. Cureus 12:e12339. doi: 10.7759/cureus.12339
Palakurti, R., Biswas, N., Roy, S., Gnyawali, S. C., Sinha, M., Singh, K., et al. (2023). Inducible miR-1224 silences cerebrovascular Serpine1 and restores blood flow to the stroke-affected site of the brain. Mol. Ther. Nucleic Acids 31, 276–292. doi: 10.1016/j.omtn.2022.12.019
Park, J. S., Gamboni-Robertson, F., He, Q., Svetkauskaite, D., Kim, J.-Y., Strassheim, D., et al. (2006). High mobility group box 1 protein interacts with multiple Toll-like receptors. Am. J. Physiol. Cell Physiol. 290, C917–C924. doi: 10.1152/ajpcell.00401.2005
Philipp, D., Suhr, L., Wahlers, T., Choi, Y.-H., and Paunel-Görgülü, A. (2018). Preconditioning of bone marrow-derived mesenchymal stem cells highly strengthens their potential to promote IL-6-dependent M2b polarization. Stem Cell Res. Ther. 9:286. doi: 10.1186/s13287-018-1039-2
Piepke, M., Clausen, B. H., Ludewig, P., Vienhues, J. H., Bedke, T., Javidi, E., et al. (2021). Interleukin-10 improves stroke outcome by controlling the detrimental Interleukin-17A response. J. Neuroinflammation 18:265. doi: 10.1186/s12974-021-02316-7
Qiu, J., Nishimura, M., Wang, Y., Sims, J. R., Qiu, S., Savitz, S. I., et al. (2008). Early release of HMGB-1 from neurons after the onset of brain ischemia. J. Cereb. Blood Flow Metab. 28, 927–938. doi: 10.1038/sj.jcbfm.9600582
Qiu, L., Wang, Y., Wang, Y., Liu, F., Deng, S., Xue, W., et al. (2023). Ursolic acid ameliorated neuronal damage by restoring microglia-activated MMP/TIMP imbalance in vitro. Drug Design Dev. Ther. 17, 2481–2493. doi: 10.2147/DDDT.S411408
Qiu, Y.-M., Zhang, C.-L., Chen, A.-Q., Wang, H.-L., Zhou, Y.-F., Li, Y.-N., et al. (2021). Immune cells in the BBB disruption after acute ischemic stroke: targets for immune therapy? Front. Immunol. 12:678744. doi: 10.3389/fimmu.2021.678744
Ratliffe, J., Kataura, T., Otten, E. G., and Korolchuk, V. I. (2023). The evolution of selective autophagy as a mechanism of oxidative stress response: the evolutionarily acquired ability of selective autophagy receptors to respond to oxidative stress is beneficial for human longevity. Bioessays 45:e2300076. doi: 10.1002/bies.202300076
Rempe, R. G., Hartz, A. M. S., and Bauer, B. (2016). Matrix metalloproteinases in the brain and blood-brain barrier: versatile breakers and makers. J. Cereb. Blood Flow Metab. 36, 1481–1507. doi: 10.1177/0271678X16655551
Richard, S. A., Sackey, M., Su, Z., and Xu, H. (2017). Pivotal neuroinflammatory and therapeutic role of high mobility group box 1 in ischemic stroke. Biosci. Rep. 37:BSR20171104. doi: 10.1042/BSR20171104
Richard, S., Lagerstedt, L., Burkhard, P. R., Debouverie, M., Turck, N., and Sanchez, J.-C. (2015). E-selectin and vascular cell adhesion molecule-1 as biomarkers of 3-month outcome in cerebrovascular diseases. J. Inflamm. (Lond.) 12:61. doi: 10.1186/s12950-015-0106-z
Rosell, A., Cuadrado, E., Ortega-Aznar, A., Hernández-Guillamon, M., Lo, E. H., and Montaner, J. (2008). MMP-9-positive neutrophil infiltration is associated to blood-brain barrier breakdown and basal lamina type IV collagen degradation during hemorrhagic transformation after human ischemic stroke. Stroke 39, 1121–1126. doi: 10.1161/STROKEAHA.107.500868
Saadoun, S., and Papadopoulos, M. C. (2010). Aquaporin-4 in brain and spinal cord oedema. Neuroscience 168, 1036–1046. doi: 10.1016/j.neuroscience.2009.08.019
Salas-Perdomo, A., Miró-Mur, F., Urra, X., Justicia, C., Gallizioli, M., Zhao, Y., et al. (2018). T cells prevent hemorrhagic transformation in ischemic stroke by P-selectin binding. Arterioscler. Thromb. Vasc. Biol. 38, 1761–1771. doi: 10.1161/ATVBAHA.118.311284
Schuette-Nuetgen, K., Strecker, J.-K., Minnerup, J., Ringelstein, E. B., and Schilling, M. (2012). MCP-1/CCR-2-double-deficiency severely impairs the migration of hematogenous inflammatory cells following transient cerebral ischemia in mice. Exp. Neurol. 233, 849–858. doi: 10.1016/j.expneurol.2011.12.011
Selvaraj, U. M., Ortega, S. B., Hu, R., Gilchrist, R., Kong, X., Partin, A., et al. (2017). Preconditioning-induced CXCL12 upregulation minimizes leukocyte infiltration after stroke in ischemia-tolerant mice. J. Cereb. Blood Flow Metab. 37, 801–813. doi: 10.1177/0271678X16639327
Severson, E. A., and Parkos, C. A. (2009). Mechanisms of outside-in signaling at the tight junction by junctional adhesion molecule A. Ann. N. Y. Acad. Sci. 1165, 10–18. doi: 10.1111/j.1749-6632.2009.04034.x
Shaheryar, Z. A., Khan, M. A., Hameed, H., Zaidi, S. A. A., Anjum, I., and Rahman, M. S. U. (2023). Lauric acid provides neuroprotection against oxidative stress in mouse model of hyperglycaemic stroke. Eur. J. Pharmacol. 956:175990. doi: 10.1016/j.ejphar.2023.175990
Shiba, Y., Takahashi, M., Hata, T., Murayama, H., Morimoto, H., Ise, H., et al. (2009). Bone marrow CXCR4 induction by cultivation enhances therapeutic angiogenesis. Cardiovasc. Res. 81, 169–177. doi: 10.1093/cvr/cvn247
Simundic, A.-M., Basic, V., Topic, E., Demarin, V., Vrkic, N., Kunovic, B., et al. (2004). Soluble adhesion molecules in acute ischemic stroke. Clin. Invest. Med. 27, 86–92.
Singh, V., Kaur, R., Kumari, P., Pasricha, C., and Singh, R. (2023). ICAM-1 and VCAM-1: gatekeepers in various inflammatory and cardiovascular disorders. Clin. Chim. Acta 548:117487. doi: 10.1016/j.cca.2023.117487
Sjöström, E. O., Culot, M., Leickt, L., Åstrand, M., Nordling, E., Gosselet, F., et al. (2021). Transport study of interleukin-1 inhibitors using a human in vitro model of the blood-brain barrier. Brain Behav. Immun. Health 16:100307. doi: 10.1016/j.bbih.2021.100307
Sladojevic, N., Stamatovic, S. M., Keep, R. F., Grailer, J. J., Sarma, J. V., Ward, P. A., et al. (2014). Inhibition of junctional adhesion molecule-A/LFA interaction attenuates leukocyte trafficking and inflammation in brain ischemia/reperfusion injury. Neurobiol. Dis. 67, 57–70. doi: 10.1016/j.nbd.2014.03.010
Song, S., Huang, H., Guan, X., Fiesler, V., Bhuiyan, M. I. H., Liu, R., et al. (2021). Activation of endothelial Wnt/β-catenin signaling by protective astrocytes repairs BBB damage in ischemic stroke. Prog. Neurobiol. 199:101963. doi: 10.1016/j.pneurobio.2020.101963
Soriano Jerez, E. M., Gibbins, J. M., and Hughes, C. E. (2021). Targeting platelet inhibition receptors for novel therapies: PECAM-1 and G6b-B. Platelets 32, 761–769. doi: 10.1080/09537104.2021.1882668
Stowe, A. M., Wacker, B. K., Cravens, P. D., Perfater, J. L., Li, M. K., Hu, R., et al. (2012). CCL2 upregulation triggers hypoxic preconditioning-induced protection from stroke. J. Neuroinflammation 9:33. doi: 10.1186/1742-2094-9-33
Sucha, P., Hermanova, Z., Chmelova, M., Kirdajova, D., Camacho Garcia, S., Marchetti, V., et al. (2022). The absence of AQP4/TRPV4 complex substantially reduces acute cytotoxic edema following ischemic injury. Front. Cell. Neurosci. 16:1054919. doi: 10.3389/fncel.2022.1054919
Sun, C., Lin, L., Yin, L., Hao, X., Tian, J., Zhang, X., et al. (2022). Acutely inhibiting AQP4 with TGN-020 improves functional outcome by attenuating edema and peri-infarct astrogliosis after cerebral ischemia. Front. Immunol. 13:870029. doi: 10.3389/fimmu.2022.870029
Sun, Y., Jin, K., Xie, L., Childs, J., Mao, X. O., Logvinova, A., et al. (2003). VEGF-induced neuroprotection, neurogenesis, and angiogenesis after focal cerebral ischemia. J. Clin. Invest. 111, 1843–1851. doi: 10.1172/JCI17977
Sun, Z., Wu, K., Gu, L., Huang, L., Zhuge, Q., Yang, S., et al. (2020). IGF-1R stimulation alters microglial polarization via TLR4/NF-κB pathway after cerebral hemorrhage in mice. Brain Res. Bull. 164, 221–234. doi: 10.1016/j.brainresbull.2020.08.026
Supanc, V., Biloglav, Z., Kes, V. B., and Demarin, V. (2011). Role of cell adhesion molecules in acute ischemic stroke. Ann. Saudi Med. 31, 365–370. doi: 10.4103/0256-4947.83217
Sweeney, M. D., Sagare, A. P., and Zlokovic, B. V. (2018). Blood-brain barrier breakdown in Alzheimer disease and other neurodegenerative disorders. Nat. Rev. Neurol. 14, 133–150. doi: 10.1038/nrneurol.2017.188
Sweeney, M. D., Zhao, Z., Montagne, A., Nelson, A. R., and Zlokovic, B. V. (2019). Blood-brain barrier: from physiology to disease and back. Physiol. Rev. 99, 21–78. doi: 10.1152/physrev.00050.2017
Switzer, J. A., Hess, D. C., Ergul, A., Waller, J. L., Machado, L. S., Portik-Dobos, V., et al. (2011). Matrix metalloproteinase-9 in an exploratory trial of intravenous minocycline for acute ischemic stroke. Stroke 42, 2633–2635. doi: 10.1161/STROKEAHA.111.618215
Takeda, H., Spatz, M., Ruetzler, C., McCarron, R., Becker, K., and Hallenbeck, J. (2002). Induction of mucosal tolerance to E-selectin prevents ischemic and hemorrhagic stroke in spontaneously hypertensive genetically stroke-prone rats. Stroke 33, 2156–2164. doi: 10.1161/01.STR.0000029821.82531.8B
Tang, C., Xue, H.-L., Bai, C.-L., and Fu, R. (2011). Regulation of adhesion molecules expression in TNF-α-stimulated brain microvascular endothelial cells by tanshinone IIA: involvement of NF-κB and ROS generation. Phytother. Res. 25, 376–380. doi: 10.1002/ptr.3278
Tang, X., Liu, H., Xiao, Y., Wu, L., and Shu, P. (2022). Vitamin C intake and ischemic stroke. Front. Nutr. 9:935991. doi: 10.3389/fnut.2022.935991
Taylor, J., Parker, M., Casey, C. P., Tanabe, S., Kunkel, D., Rivera, C., et al. (2022). Postoperative delirium and changes in the blood-brain barrier, neuroinflammation, and cerebrospinal fluid lactate: a prospective cohort study. Br. J. Anaesth. 129, 219–230. doi: 10.1016/j.bja.2022.01.005
Thomas, R. G., Kim, J.-H., Kim, J.-H., Yoon, J., Choi, K.-H., and Jeong, Y.-Y. (2023). Treatment of ischemic stroke by atorvastatin-loaded PEGylated liposome. Transl. Stroke Res. doi: 10.1007/s12975-023-01125-9 [Epub ahead of print].
Uniken Venema, S. M., Dankbaar, J. W., van der Lugt, A., Dippel, D. W. J., and van der Worp, H. B. (2022). Cerebral collateral circulation in the era of reperfusion therapies for acute ischemic stroke. Stroke 53, 3222–3234. doi: 10.1161/STROKEAHA.121.037869
Venugopal, J., Wang, J., Mawri, J., Guo, C., and Eitzman, D. (2021). Interleukin-1 receptor inhibition reduces stroke size in a murine model of sickle cell disease. Haematologica 106, 2469–2477. doi: 10.3324/haematol.2020.252395
von Kummer, R., Dzialowski, I., and Gerber, J. (2015). Therapeutic efficacy of brain imaging in acute ischemic stroke patients. J. Neuroradiol. 42, 47–54. doi: 10.1016/j.neurad.2014.10.004
Wang, D., Li, B., Wang, S., Hao, Y., Wang, H., Sun, W., et al. (2023). Engineered inhaled nanocatalytic therapy for ischemic cerebrovascular disease by inducing autophagy of abnormal mitochondria. NPJ Regen. Med. 8:44. doi: 10.1038/s41536-023-00315-1
Wang, S., de Fabritus, L., Kumar, P. A., Werner, Y., Ma, M., Li, D., et al. (2023). Brain endothelial CXCL12 attracts protective natural killer cells during ischemic stroke. J. Neuroinflammation 20:8. doi: 10.1186/s12974-023-02689-x
Wang, Y., Huang, J., Li, Y., and Yang, G.-Y. (2012). Roles of chemokine CXCL12 and its receptors in ischemic stroke. Curr. Drug Targets 13, 166–172. doi: 10.2174/138945012799201603
Wang, Y., Liu, M., and Pu, C. (2017). 2014 Chinese guidelines for secondary prevention of ischemic stroke and transient ischemic attack. Int. J. Stroke 12, 302–320. doi: 10.1177/1747493017694391
Weis, S. M., and Cheresh, D. A. (2005). Pathophysiological consequences of VEGF-induced vascular permeability. Nature 437, 497–504. doi: 10.1038/nature03987
Williams, L. A., Martin-Padura, I., Dejana, E., Hogg, N., and Simmons, D. L. (1999). Identification and characterisation of human Junctional Adhesion Molecule (JAM). Mol. Immunol. 36, 1175–1188. doi: 10.1016/s0161-5890(99)00122-4
Witsch, J., Roh, D., Oh, S., Iadecola, C., Diaz-Arrastia, R., Kasner, S. E., et al. (2023). Association between soluble intercellular adhesion molecule-1 and intracerebral hemorrhage outcomes in the FAST trial. Stroke 54, 1726–1734. doi: 10.1161/STROKEAHA.123.042466
Wu, B.-N., Wu, J., Hao, D.-L., Mao, L.-L., Zhang, J., and Huang, T.-T. (2018). High serum sICAM-1 is correlated with cerebral microbleeds and hemorrhagic transformation in ischemic stroke patients. Br. J. Neurosurg. 32, 631–636. doi: 10.1080/02688697.2018.1518515
Wu, X., Liang, T.-Y., Wang, Z., and Chen, G. (2021). The role of hyperbaric oxygen therapy in inflammatory bowel disease: a narrative review. Med. Gas Res. 11, 66–71. doi: 10.4103/2045-9912.311497
Xie, L., Zhang, S., Huang, L., Peng, Z., Lu, H., He, Q., et al. (2023). Single-cell RNA sequencing of peripheral blood reveals that monocytes with high cathepsin S expression aggravate cerebral ischemia-reperfusion injury. Brain Behav. Immun. 107, 330–344. doi: 10.1016/j.bbi.2022.11.001
Xie, W., Zhu, T., Dong, X., Nan, F., Meng, X., Zhou, P., et al. (2019). HMGB1-triggered inflammation inhibition of notoginseng leaf triterpenes against cerebral ischemia and reperfusion injury via MAPK and NF-κB signaling pathways. Biomolecules 9:512. doi: 10.3390/biom9100512
Xu, J., Chen, Z., Yu, F., Liu, H., Ma, C., Xie, D., et al. (2020). IL-4/STAT6 signaling facilitates innate hematoma resolution and neurological recovery after hemorrhagic stroke in mice. Proc. Natl. Acad. Sci. U.S.A. 117, 32679–32690. doi: 10.1073/pnas.2018497117
Xu, Y., Zhang, J., Gao, F., Cheng, W., Zhang, Y., Wei, C., et al. (2023). Engeletin alleviates cerebral ischemia reperfusion-induced neuroinflammation via the HMGB1/TLR4/NF-κB network. J. Cell. Mol. Med. 27, 1653–1663. doi: 10.1111/jcmm.17758
Yang, C., Hawkins, K. E., Doré, S., and Candelario-Jalil, E. (2019). Neuroinflammatory mechanisms of blood-brain barrier damage in ischemic stroke. Am. J. Physiol. Cell Physiol. 316, C135–C153. doi: 10.1152/ajpcell.00136.2018
Yang, C., Yang, Y., DeMars, K. M., Rosenberg, G. A., and Candelario-Jalil, E. (2020). Genetic deletion or pharmacological inhibition of cyclooxygenase-2 reduces blood-brain barrier damage in experimental ischemic stroke. Front. Neurol. 11:887. doi: 10.3389/fneur.2020.00887
Yang, Q., Wang, J.-Z., Li, J.-C., Zhou, Y., Zhong, Q., Lu, F.-L., et al. (2010). High-mobility group protein box-1 and its relevance to cerebral ischemia. J. Cereb. Blood Flow Metab. 30, 243–254. doi: 10.1038/jcbfm.2009.202
Yang, Q.-W., Lu, F.-L., Zhou, Y., Wang, L., Zhong, Q., Lin, S., et al. (2011). HMBG1 mediates ischemia-reperfusion injury by TRIF-adaptor independent Toll-like receptor 4 signaling. J. Cereb. Blood Flow Metab. 31, 593–605. doi: 10.1038/jcbfm.2010.129
Yawoot, N., Sengking, J., Govitrapong, P., Tocharus, C., and Tocharus, J. (2023). Melatonin modulates the aggravation of pyroptosis, necroptosis, and neuroinflammation following cerebral ischemia and reperfusion injury in obese rats. Biochim. Biophys. Acta Mol. Basis Dis. 1869:166785. doi: 10.1016/j.bbadis.2023.166785
You, Y., Liu, Y., Ma, C., Xu, J., Xie, L., Tong, S., et al. (2023). Surface-tethered ROS-responsive micelle backpacks for boosting mesenchymal stem cell vitality and modulating inflammation in ischemic stroke treatment. J. Control. Release 362, 210–224. doi: 10.1016/j.jconrel.2023.08.039
Yu, M., Xiao, G., Han, L., Peng, L., Wang, H., He, S., et al. (2023). QiShen YiQi and its components attenuate acute thromboembolic stroke and carotid thrombosis by inhibition of CD62P/PSGL-1-mediated platelet-leukocyte aggregate formation. Biomed. Pharmacother. 160:114323. doi: 10.1016/j.biopha.2023.114323
Zador, Z., Stiver, S., Wang, V., and Manley, G. T. (2009). “Role of aquaporin-4 in cerebral edema and stroke,” in Aquaporins. Handbook of experimental pharmacology, Vol. 190, ed. E. Beitz (Berlin: Springer). doi: 10.1007/978-3-540-79885-9_7
Zemani, F., Silvestre, J.-S., Fauvel-Lafeve, F., Bruel, A., Vilar, J., Bieche, I., et al. (2008). Ex vivo priming of endothelial progenitor cells with SDF-1 before transplantation could increase their proangiogenic potential. Arterioscler. Thromb. Vasc. Biol. 28, 644–650. doi: 10.1161/ATVBAHA.107.160044
Zhang, G., Chen, L., Chen, W., Li, B., Yu, Y., Lin, F., et al. (2018). Neural stem cells alleviate inflammation via neutralization of IFN- γ negative effect in ischemic stroke model. J. Biomed. Nanotechnol. 14, 1178–1188. doi: 10.1166/jbn.2018.2568
Zhang, K., Zhang, Q., Deng, J., Li, J., Li, J., Wen, L., et al. (2019). ALK5 signaling pathway mediates neurogenesis and functional recovery after cerebral ischemia/reperfusion in rats via Gadd45b. Cell Death Dis. 10:360. doi: 10.1038/s41419-019-1596-z
Zhang, L., Wei, W., Ai, X., Kilic, E., Hermann, D. M., Venkataramani, V., et al. (2021). Extracellular vesicles from hypoxia-preconditioned microglia promote angiogenesis and repress apoptosis in stroke mice via the TGF-β/Smad2/3 pathway. Cell Death Dis. 12:1068. doi: 10.1038/s41419-021-04363-7
Zhang, L., Xu, S., Wu, X., Chen, J., Guo, X., Cao, Y., et al. (2020). Combined treatment With 2-(2-Benzofu-Ranyl)-2-imidazoline and recombinant tissue plasminogen activator protects blood-brain barrier integrity in a rat model of embolic middle cerebral artery occlusion. Front. Pharmacol. 11:801. doi: 10.3389/fphar.2020.00801
Zhang, S., An, Q., Wang, T., Gao, S., and Zhou, G. (2018). Autophagy- and MMP-2/9-mediated reduction and redistribution of ZO-1 contribute to hyperglycemia-increased blood-brain barrier permeability during early reperfusion in stroke. Neuroscience 377, 126–137. doi: 10.1016/j.neuroscience.2018.02.035
Zhang, X., Gong, P., Chen, S., Wan, T., Wang, X., Wang, M., et al. (2023). Endothelial dysfunction and parenchymal hematoma in ischemic stroke patients after endovascular thrombectomy. Cerebrovasc. Dis. (Basel, Switzerland). doi: 10.1159/000530372 [Epub ahead of print].
Zhang, Y., Lei, L., Zhou, H., Lu, X., Cai, F., and Li, T. (2022). Roles of micro ribonucleic acids in astrocytes after cerebral stroke. Front. Cell. Neurosci. 16:890762. doi: 10.3389/fncel.2022.890762
Zhang, Z. G., Zhang, L., Jiang, Q., Zhang, R., Davies, K., Powers, C., et al. (2000). VEGF enhances angiogenesis and promotes blood-brain barrier leakage in the ischemic brain. J. Clin. Invest. 106, 829–838. doi: 10.1172/JCI9369
Zhang, Z., and Chopp, M. (2002). Vascular endothelial growth factor and angiopoietins in focal cerebral ischemia. Trends Cardiovasc. Med. 12, 62–66. doi: 10.1016/s1050-1738(01)00149-9
Zhao, M., Tuo, H., Wang, S., and Zhao, L. (2020). The roles of monocyte and monocyte-derived macrophages in common brain disorders. Biomed Res. Int. 2020:9396021. doi: 10.1155/2020/9396021
Zhao, Y.-J., Nai, Y., Li, S.-Y., and Zheng, Y.-H. (2018). Retigabine protects the blood-brain barrier by regulating tight junctions between cerebral vascular endothelial cells in cerebral ischemia-reperfusion rats. Eur. Rev. Med. Pharmacol. Sci. 22, 8509–8518. doi: 10.26355/eurrev_201812_16552
Zhou, D., Huang, Z., Zhu, X., Hong, T., and Zhao, Y. (2021). Combination of endothelial progenitor cells and BB-94 significantly alleviates brain damage in a mouse model of diabetic ischemic stroke. Exp. Ther. Med. 22:789. doi: 10.3892/etm.2021.10221
Zhou, F., Du, M., Yan, E., Chen, S., Wang, W., Shi, H., et al. (2023). Higher serum E-selectin levels associated with malignant brain edema after endovascular thrombectomy for ischemic stroke: a pilot study. Brain Sci. 13:1097. doi: 10.3390/brainsci13071097
Zhou, M., Li, R., Venkat, P., Qian, Y., Chopp, M., Zacharek, A., et al. (2022). Post-stroke administration of L-4F promotes neurovascular and white matter remodeling in Type-2 diabetic stroke mice. Front. Neurol. 13:863934. doi: 10.3389/fneur.2022.863934
Zhou, Z., Dun, L., Xu, H., Yu, P., Chen, C., Si, T., et al. (2022). The neuroprotective effect of YaoYi-moxibustion on ischemic stroke by attenuating NK-κB expression in rats. Ann. Transl. Med. 10:791. doi: 10.21037/atm-22-3198
Zhu, H., Hu, S., Li, Y., Sun, Y., Xiong, X., Hu, X., et al. (2022). Interleukins and ischemic stroke. Front. Immunol. 13:828447. doi: 10.3389/fimmu.2022.828447
Zhu, H., Jian, Z., Zhong, Y., Ye, Y., Zhang, Y., Hu, X., et al. (2021). Janus kinase inhibition ameliorates ischemic stroke injury and neuroinflammation through reducing NLRP3 inflammasome activation via JAK2/STAT3 pathway inhibition. Front. Immunol. 12:714943. doi: 10.3389/fimmu.2021.714943
Zhu, X., Cao, Y., Wei, L., Cai, P., Xu, H., Luo, H., et al. (2016). von Willebrand factor contributes to poor outcome in a mouse model of intracerebral haemorrhage. Sci. Rep. 6:35901. doi: 10.1038/srep35901
Keywords: diagnostic marker, therapeutic target, stroke, hemorrhagic transformation, edema, zonula occluden, S100β, albumin
Citation: Yao Y, Liu F, Gu Z, Wang J, Xu L, Yu Y, Cai J and Ren R (2023) Emerging diagnostic markers and therapeutic targets in post-stroke hemorrhagic transformation and brain edema. Front. Mol. Neurosci. 16:1286351. doi: 10.3389/fnmol.2023.1286351
Received: 31 August 2023; Accepted: 13 November 2023;
Published: 21 December 2023.
Edited by:
Qin Hu, Shanghai Jiao Tong University, ChinaReviewed by:
Xiang Li, The First Affiliated Hospital of Soochow University, ChinaChao Li, Henry Ford Hospital, United States
Cameron Lenahan, Naval Medical Center Portsmouth, United States
Copyright © 2023 Yao, Liu, Gu, Wang, Xu, Yu, Cai and Ren. This is an open-access article distributed under the terms of the Creative Commons Attribution License (CC BY). The use, distribution or reproduction in other forums is permitted, provided the original author(s) and the copyright owner(s) are credited and that the original publication in this journal is cited, in accordance with accepted academic practice. No use, distribution or reproduction is permitted which does not comply with these terms.
*Correspondence: Jing Cai, MTE3MTgzNDdAemp1LmVkdS5jbg==; Reng Ren, cmVucmVuZzEyMDNAemp1LmVkdS5jbg==
†These authors have contributed equally to this work and share first authorship