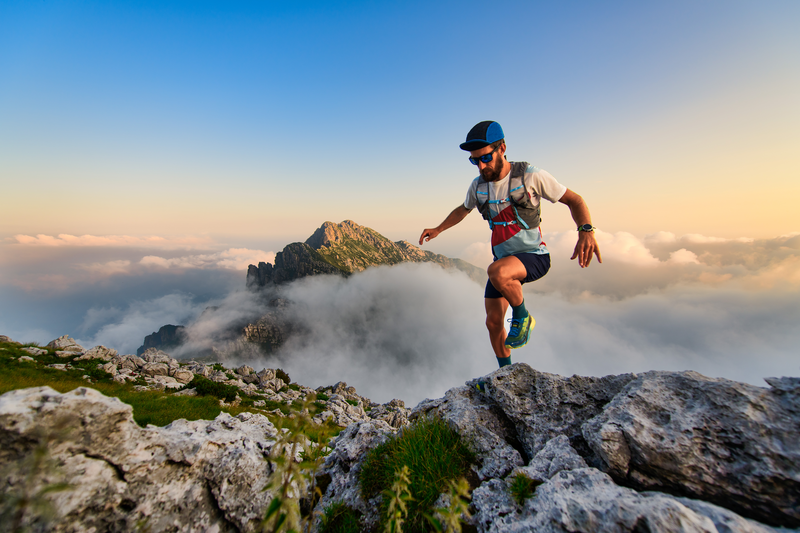
95% of researchers rate our articles as excellent or good
Learn more about the work of our research integrity team to safeguard the quality of each article we publish.
Find out more
EDITORIAL article
Front. Mol. Neurosci. , 01 August 2023
Sec. Methods and Model Organisms
Volume 16 - 2023 | https://doi.org/10.3389/fnmol.2023.1258458
This article is part of the Research Topic Gene Therapy for the Central and Peripheral Nervous System Volume II View all 11 articles
Editorial on the Research Topic
Gene therapy for the central and peripheral nervous system, volume II
After the great success of the first volume, we are proud to present Volume II of this specialized Research Topic on Gene therapy for the central and peripheral nervous system. Volume I discussed gene therapies for the treatment of diverse neurodegenerative disorders including spinocerebellar ataxia, spinal muscular atrophy (SMA), amyotrophic lateral sclerosis (ALS), as well as disorders of the central nervous system (CNS) and peripheral nervous system (PNS), including, stroke, peripheral nerve repair and neuropathic pain (Tosolini and Smith, 2018). Volume II has expanded upon these ideas and concepts, and has also included submissions focused on the basic pathomechanism(s) of various CNS/PNS disorders, with the intention to include conversations of target identification and validation for the development of future gene therapies.
The aim of most gene therapy pipelines is to transition successful and meaningful outcomes from the pre-clinical experimental setting, regardless of the model system, into viable treatment options for patients (Zhao et al., 2022). As a scientific community, we should be immensely encouraged with the recent triumphs of the clinically available gene therapies, such as Luxturna for RPE65-associated retinal dystrophy (Smalley, 2017), as well as Zolgenmsa (Mendell et al., 2017) and the antisense oligonucleotide (ASO) therapy Nusinersen (Mercuri et al., 2018) to treat SMN1-linked SMA. However, these monumental accomplishments have come from treating monogenic disorders, and harnessing those successes to treat more complicated afflictions of the CNS/PNS (e.g., ALS, spinal cord injury, etc.) appear as the next major challenge for the field (Tosolini and Sleigh, 2017).
Nevertheless, there is great excitement in expanding the list of clinically available gene therapies, with the continuous development and optimisation of technologies that result in better outcomes (Challis et al., 2022). Indeed, novel viral serotypes and capsids are being improved (Chan et al., 2017; Andari et al., 2022; Goertsen et al., 2022), and in combination with tissue/cell specific promoters (Wang et al., 2008; Jonquieres et al., 2013; Nieuwenhuis et al., 2021), enable a more exquisite targeting of select populations of subsets of neurons/cells, which reduce the potential of detrimental off-target effects. At the same time, novel ASOs and ASO-like molecules are also being developed and combat disease by altering gene expression through interactions with specific regions of the targeted gene with the ultimate goal of supressing pathological protein load (Fletcher et al., 2016).
To complement the advances in gene therapy bioengineering, developments in assistive technology, such as focused ultrasound methods (Blesa et al., 2023), are being implemented to improve blood-brain-barrier permeability and augment access of gene therapies to targeted CNS/PNS regions. Furthermore, novel delivery methods, such as subpial injections, have also been implemented to enhance therapeutic efficacy of gene therapies, and are being applied to small and large mammals, such as mice and non-human primates (Bravo-Hernandez et al., 2020). Alternatively, as viral-vectors can be internalized in axon terminals and undergo retrograde axonal transport in motor and sensory neurons, administering gene therapies to skeletal muscle is a minimally invasive approach to transduce the CNS (Tosolini and Sleigh, 2020). On the other hand, combining muscle-specific viral-serotypes with promoters will restrict transduction to skeletal muscle, but can therapeutically influence the innervating neuronal populations (Sleigh et al., 2023).
We launched the second installment in 2021 and we are proud to showcase the ten publications in this Research Topic that are comprised of six original research articles (Table 1) and four review articles (Table 2).
We would like to thank all the authors who have contributed to the continued important discussion on gene therapy to treat the CNS/PNS.
All authors listed have made a substantial, direct, and intellectual contribution to the work and approved it for publication.
This work has been supported by a Junior Non-Clinical Fellowship from the Motor Neuron Disease Association (Tosolini/Oct20/973-799) (AT), a FightMND Grant awarded to Giovanni Nardo (Istituto di Ricerche Farmacologiche Mario Negri IRCCS) (DDG-73) (AT), and a NIH NINDS grant (R01NS117749-01) (GS).
The authors declare that the research was conducted in the absence of any commercial or financial relationships that could be construed as a potential conflict of interest.
All claims expressed in this article are solely those of the authors and do not necessarily represent those of their affiliated organizations, or those of the publisher, the editors and the reviewers. Any product that may be evaluated in this article, or claim that may be made by its manufacturer, is not guaranteed or endorsed by the publisher.
Andari, J. E., Renaud-Gabardos, E., Tulalamba, W., Weinmann, J., Mangin, L., Pham, Q. H., et al. (2022). Semirational bioengineering of AAV vectors with increased potency and specificity for systemic gene therapy of muscle disorders. Sci. Adv. 8, eabn4704. doi: 10.1126/sciadv.abn4704
Blesa, J., Pineda-Pardo, J. A., Inoue, K., Gasca-Salas, C., Balzano, T., Del Rey, N. L. G., et al. (2023). BBB opening with focused ultrasound in nonhuman primates and Parkinson's disease patients: targeted AAV vector delivery and PET imaging. Sci. Adv. 9, eadf4888. doi: 10.1126/sciadv.adf4888
Bravo-Hernandez, M., Tadokoro, T., Navarro, M. R., Platoshyn, O., Kobayashi, Y., Marsala, S., et al. (2020). Spinal subpial delivery of AAV9 enables widespread gene silencing and blocks motoneuron degeneration in ALS. Nat. Med. 5, 1–39. doi: 10.1038/s41591-019-0674-1
Challis, R. C., Ravindra Kumar, S., Chen, X., Goertsen, D., Coughlin, G. M., Hori, A. M., et al. (2022). Adeno-associated virus toolkit to target diverse brain cells. Annu. Rev. Neurosci. 45, 447–469. doi: 10.1146/annurev-neuro-111020-100834
Chan, K. Y., Jang, M. J., Yoo, B. B., Greenbaum, A., Ravi, N., Wu, W. L., et al. (2017). Engineered AAVs for efficient noninvasive gene delivery to the central and peripheral nervous systems. Nat. Neurosci. 20, 1172–1179. doi: 10.1038/nn.4593
Fletcher, S., Bellgard, M. I., Price, L., Akkari, A. P., and Wilton, S. D. (2016). Translational development of splice-modifying antisense oligomers. Exp. Opin. Biol. Ther. 17, 15–30. doi: 10.1080/14712598.2017.1250880
Goertsen, D., Flytzanis, N. C., Goeden, N., Chuapoco, M. R., Cummins, A., Chen, Y., et al. (2022). AAV capsid variants with brain-wide transgene expression and decreased liver targeting after intravenous delivery in mouse and marmoset. Nat. Neurosci. 25, 106–115. doi: 10.1038/s41593-021-00969-4
Jonquieres, G., von Mersmann, N., Klugmann, C. B., Harasta, A. E., Lutz, B., Teahan, O., et al. (2013). Glial promoter selectivity following aav-delivery to the immature brain. PLoS ONE 8, e65646. doi: 10.1371/journal.pone.0065646
Mendell, J. R., Al-Zaidy, S., Shell, R., Arnold, W. D., Rodino-Klapac, L. R., Prior, T. W., et al. (2017). Single-dose gene-replacement therapy for spinal muscular atrophy. N. Engl. J. Med. 377, 1713–1722. doi: 10.1056/NEJMoa1706198
Mercuri, E., Darras, B. T., Chiriboga, C. A., Day, J. W., Campbell, C., Connolly, A. M., et al. (2018). Nusinersen versus sham control in later-onset spinal muscular atrophy. N. Engl. J. Med. 378, 625–635. doi: 10.1056/NEJMoa1710504
Nieuwenhuis, B., Haenzi, B., Hilton, S., Carnicer-Lombarte, A., Hobo, B., Verhaagen, J., et al. (2021). Optimization of adeno-associated viral vector-mediated transduction of the corticospinal tract: comparison of four promoters. Gene Ther. 28, 56–74. doi: 10.1038/s41434-020-0169-1
Sleigh, J. N., Villarroel-Campos, D., Surana, S., Wickenden, T., Tong, Y., Simkin, R. L., et al. (2023). Boosting peripheral BDNF rescues impaired in vivo axonal transport in CMT2D mice. JCI Insight 8, e157191. doi: 10.1172/jci.insight.157191
Smalley, E. (2017). First AAV gene therapy poised for landmark approval. Nat. Biotechnol. 35, 998–999. doi: 10.1038/nbt1117-998
Tosolini, A. P., and Sleigh, J. N. (2017). Motor neuron gene therapy: lessons from spinal muscular atrophy for amyotrophic lateral sclerosis. Front. Mol. Neurosci. 10, 610. doi: 10.3389/fnmol.2017.00405
Tosolini, A. P., and Sleigh, J. N. (2020). Intramuscular delivery of gene therapy for targeting the nervous system. Front. Mol. Neurosci. 13, 1047–1016. doi: 10.3389/fnmol.2020.00129
Tosolini, A. P., and Smith, G. M. (2018). Editorial: gene therapy for the central and peripheral nervous system. Front. Mol. Neurosci. 11, 54. doi: 10.3389/fnmol.2018.00054
Wang, B., Li, J., Fu, F. H., Chen, C., Zhu, X., Zhou, L., et al. (2008). Construction and analysis of compact muscle-specific promoters for AAV vectors. Gene Ther. 15, 1489–1499. doi: 10.1038/gt.2008.104
Keywords: gene therapy, AAV, miRNA, CNS, PNS, transduction, lncRNA, circRNAs
Citation: Tosolini AP and Smith GM (2023) Editorial: Gene therapy for the central and peripheral nervous system, volume II. Front. Mol. Neurosci. 16:1258458. doi: 10.3389/fnmol.2023.1258458
Received: 14 July 2023; Accepted: 14 July 2023;
Published: 01 August 2023.
Edited and reviewed by: Gregg E. Homanics, University of Pittsburgh, United States
Copyright © 2023 Tosolini and Smith. This is an open-access article distributed under the terms of the Creative Commons Attribution License (CC BY). The use, distribution or reproduction in other forums is permitted, provided the original author(s) and the copyright owner(s) are credited and that the original publication in this journal is cited, in accordance with accepted academic practice. No use, distribution or reproduction is permitted which does not comply with these terms.
*Correspondence: Andrew P. Tosolini, YS50b3NvbGluaUB1cS5lZHUuYXU=; George M. Smith, Z2VvcmdlLnNtaXRoQHRlbXBsZS5lZHU=
†Present addresses: Andrew P. Tosolini, Australian Institute for Bioengineering and Nanotechnology, The University of Queensland, St Lucia, QLD, Australia; School of Biomedical Sciences, Faculty of Medicine, The University of Queensland, St Lucia, QLD, Australia
Disclaimer: All claims expressed in this article are solely those of the authors and do not necessarily represent those of their affiliated organizations, or those of the publisher, the editors and the reviewers. Any product that may be evaluated in this article or claim that may be made by its manufacturer is not guaranteed or endorsed by the publisher.
Research integrity at Frontiers
Learn more about the work of our research integrity team to safeguard the quality of each article we publish.