- 1Department of Human Anatomy and Histology, I.M. Sechenov First Moscow State Medical University (Sechenov University), Moscow, Russia
- 2Laboratory of Genomic Research, Research Institute for Genetic and Molecular Epidemiology, Kursk State Medical University, Kursk, Russia
- 3STEMM Laboratory, University of Exeter, Exeter, United Kingdom
- 4Department of Physics, University of Oulu, Oulu, Finland
- 5College of Engineering and Physical Sciences, Aston University, Birmingham, United Kingdom
Our review seeks to elucidate the current state-of-the-art in studies of 70-kilodalton-weighed heat shock proteins (Hsp70) in neurodegenerative diseases (NDs). The family has already been shown to play a crucial role in pathological aggregation for a wide spectrum of brain pathologies. However, a slender boundary between a big body of fundamental data and its implementation has only recently been crossed. Currently, we are witnessing an anticipated advancement in the domain with dozens of studies published every month. In this review, we briefly summarize scattered results regarding the role of Hsp70 in the most common NDs including Alzheimer’s disease (AD), Parkinson’s disease (PD), and amyotrophic lateral sclerosis (ALS). We also bridge translational studies and clinical trials to portray the output for medical practice. Available options to regulate Hsp70 activity in NDs are outlined, too.
1. Introduction
Although neurodegenerative diseases (NDs) are rather widespread while their course is severe and prone to progression with increasing cognitive dysfunction and fatal outcomes, we still possess no effective tools to achieve a critical improvement in incidence and mortality. The problem is not only medical and not uniquely longevity is affected. Since senescence is actually a principal risk factor (Turturici et al., 2011), NDs impair person’s ability, shrinking working life. As the clinical and socioeconomic impacts remain relevant, studies of pathogenetic mechanisms in neurodegeneration are needed to develop novel approaches for its detection and treatment. The most important pathogenic links of NDs involve oxidative stress, mitochondrial dysfunction, neuroinflammation, excitotoxicity, and defects of autophagy. Finally, the hallmark is accumulation of protein aggregate deposits, reflecting critical imbalance in neuronal homeostasis.
In neurodegenerative brain, the cells tend to synthesize misfolded proteins and lose an ability to properly utilize them (Campanella et al., 2018). Because neurons form sophistic anatomically and functionally interconnected networks, each cell may serve a crucial link in numerous different circuits. Thus, for neurons it is dramatically important to stay alive as long as possible, and this is one of the reasons for the longest neuronal lifetime (Kole et al., 2013). The last feature, together with highest complexity and diversity of brain’s proteome (Mauger and Scheiffele, 2017; Negi and Guda, 2017; Korovesi et al., 2020; Sinitcyn et al., 2023) determines a need for very thorough quality control of cerebral polypeptides.
Maintaining healthy proteome requires control over newly synthesized proteins as well as clearance/cleavage or refolding of unstable mature ones. All these functions are naturally provided by highly conservative molecular machinery called chaperones. Among the others, the family of heat shock proteins with a molecular weight of 70 kDa (Hsp70) is considered the most important in terms of neurological disorders. Hsp70 represent one of the chief groups among the protectors, being phylogenetically very old and preserved (Koren et al., 2009). These facts invite us to study the potential pharmacological benefit of Hsp70 in NDs.
Numerous studies have revealed the contribution of Hsp70 in degenerative and age-associated anomalies of the brain. However, some of the positive roles of Hsp70 in brain pathology remain difficult to estimate in terms of its significance for translational medicine. Herein, we summarize the state-of-the-art and previous advances disclosing the clinical potential of the strategies based on Hsp70 management. Especially important focus in this review is given to clinical trials, which bring Hsp70 closer to the nearest practical application.
2. Essentials of Hsp70
2.1. Hsp70 family
The family of Hsp70 includes more than 10 members (Table 1). Generally, they enable adequate folding for both newly synthesized or mature proteins as well as refolding for denaturated/aggregated proteins (Hartl and Hayer-Hartl, 2002). In addition, some constitutively expressed or induced members of the family possess relatively specific functions, such as regulation of apoptosis, mitochondrial function (mtHsp70), and metabolic pathways (Lackie et al., 2017). However, Hsp70 are specified to ensure folding via ATP-dependent machinery, preventing denatured proteins from aggregating (Jores et al., 2018).
Heat shock proteins are found in almost all cellular compartments, including the nucleus and cytoplasm (Hsc70), as well as in mitochondria (Wentink et al., 2020). Moreover, Hsc70 may be found in a lysosome-specific isoform (Ciechanover and Kwon, 2017). General protective properties of Hsp70 determine both pro- and eukaryotic biology, and even plants express their own Hsp70 analogs (Chaudhary et al., 2019). However, some features are shared by principal chaperones of the group for mammals, including humans, mice, and rats (Figure 1). Hsc70, which is the most common one among the Hsp70 in healthy and undamaged state, has the largest number of unique interacting proteins among all the Hsp70 family members (Rai et al., 2021).
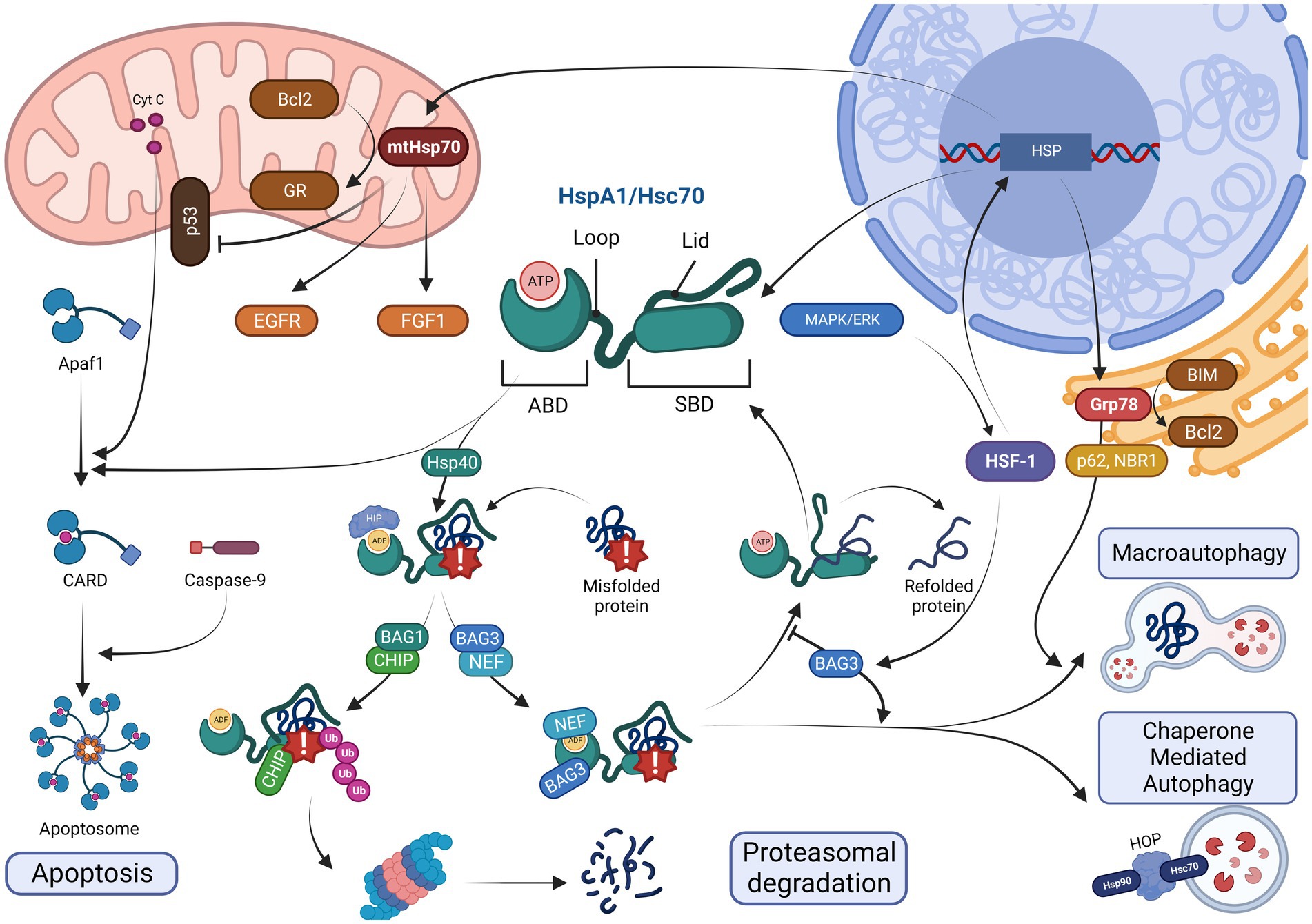
Figure 1. Toggles between crucial options: a model of intracellular pathways for common Hsp70 members (HspA1, Hsc70, Grp78, and mtHsp70) at their impact sites. At the center, one can see HspA1 and Hsc70, which are the two most prevalent players in the cytosol, with their active structures comprising four structures in large: an ABD (at the N-terminus) to engine chaperone activity, a loop (fine and folded linker) to contact many allosteric regulators, an SBD (beta-barrel) to capture misfolded proteins, and a lid (motile cap-like chain) to either come closer to the SBD or to align depending on ATP-ADP dualism. When HSF-1-upregulated transcription is completed, the cytosolic isoforms enter Fork # 1, where Hsp40 navigate a grip of a hazardous protein (down) via dephosphorylation and ADP inactivation by HIP, whereas the lid closes over the problematic chain; otherwise, apoptotic recruitment may occur (left). In apoptosis, cytochrome c-induced APAF1-driven recruitment of caspase-9 into the CARD is blocked by Hsp70. In contrast, the Hsp40-switched track comes into its Fork # 2 with a BAG1-associated choice of an allosteric CHIP regulation towards ubiquitin-mediated proteasomal degradation (below) or a BAG3-associated concurrent NEF-inhibition of the ABD up to Fork # 3 (right) with a continuous HSF-1 presence leading to either BAG3-overexpression and autophagic events or holding of the hazardous substance inside the beta-barrel until the BAG3 content decreases with NEF released and protein returned into the cytosol to refold in better conditions (up). Autophagic Fork # 4 occurs either on the ER surface for Grp78 modification of Hsp70 (on the right) driven into macroautophagy by p62 and NBR-1 or in the cytosol with Hsp90-recruiting HOP assistance, causing chaperone-mediated autophagy, which is more typical for Hsc70 immersion into lysosomes. Grp78 also acts against apoptosis, preventing BIM from Bcl2 elimination. In the left upper corner, mtHsp70 is found; it is anti-apoptotic, too, via an induction of Bcl-2 to transfer GR into the organelles. A positive impact on EGFR and FGF-1 activity as well as a negative impact on p53 chains is clear.
2.2. Cellular regulation of Hsp70
Hsp70 expression is controlled by heat shock factor 1 (HSF-1), which receives downstream signals from a number of stimuli, such as pathway of extracellular signal-regulated kinases and mitogen-activated protein-kinases (ERK/MAPK), highly influenced by increased air pressure and temperature (Matsathit et al., 2016). HSF-1 is also a thermosensor whose leucin zipper domains change in temperature bounces. Moreover, Hsp70-induced feedback permits to monomerize trimers of HSF-1 and separate it from DNA molecules (Kmiecik and Mayer, 2022).
The activity of Hsp70 is driven by small Hsp with a molecular weight of 40 kDa (Hsp40/DnaJ family), especially DnaJB11 and DnaJC5 (Braun, 2023), which are relevant for some types of tauopathies (Kampinga and Craig, 2010; Zhang et al., 2023, M). Although the activity of Hsp70 is firstly dependent on its expression, some enzymes also may introduce modifications, regulating Hsp70 in kinase dependent manner. For instance, it has been reported that cyclin-dependent kinase 1 (Cdk1) is able to temporarily downregulate chaperonic functions of Hsp70 by phosphorylation of serine in a region between ATP- and substrate-binding domains (Kao et al., 2020). Interestingly, in addition to de novo synthesis of Hsp70, neurons tend to uptake it from neighboring astrocytes (Tytell et al., 1986; Hightower and Guidon, 1989; Guzhova et al., 1998, 2001; Kalmar and Greensmith, 2017).
3. Hsp70 in NDs
3.1. Hsp70 and aging
Hsp70 play an important role in the nervous system in health and in disease. It is especially remarkable for aging brain. The aging itself is a crucial risk factor as any senescent cell exhibits a lower chaperone protein translation while its markers for labeling of misfolded proteins are not expressed well (Llewellyn et al., 2023). For instance, Hsp70 production falls by 50% in aged rat liver cells in stress (Heydari et al., 1994). Basal Hsp70 content in cells stays higher in long-living animals (de Toda et al., 2016). Furthermore, co-chaperones of Hsp70 in mice are expressed less in development of age-dependent neurodegeneration (Lackie et al., 2020). Higher levels of oxidant and lower ones of antioxidant actors may aggravate the state of chaperone action in aged individuals (Martínez de Toda and De la Fuente, 2015).
Despite these facts, not all the heat shock proteins decrease at the same rate as Hsp70 do in aging. Specifically, ATP-recruiting Hsp (foldases) experience a strong hypoexpression, while ATP-independent (holdases) continue to be actively synthesized (de Graff et al., 2020). As a result, proteostasis and preventing of aggregation suffers less than the protection of nascent proteins. Sirtuins, which are well-known for their controlling role in aging regulation, are probably responsible for proper Hsp70 expression after the stimulation by HSF-1. Common in young age, sirtuin 1 has been evidenced to potentiate binding of HSF-1 to DNA, thereby modifying levels of Hsp70 synthesis (Karvinen et al., 2016).
3.2. Hsp70 in Alzheimer’s disease
Alzheimer’s disease (AD), known as the most widespread ND, mainly affects memory and other cognitive functions related to the synaptic loss and deposition of neurofibrillary tangles (NFT) and peptide plaques (Lane et al., 2018). The plaques are principally formed by amyloid beta (Aβ; Abeta) deriving from Aβ precursor (APP) after enzyme-mediated cleavage, especially under presenilin-1 (PS1; PSEN1) action (Russo et al., 2000; Rajesh and Kanneganti, 2022). Despite being debatable, this Aβ hypothesis of AD is still considered to be generally accepted (Breijyeh and Karaman, 2020). Recent studies also claim soluble Aβ oligomers (AβOs) to have more harmful impact than their insoluble counterparts. For instance, excitotoxicity and abnormal long-term potentiation are already seen at very early stages of AD before detectable plaques (Huang and Liu, 2020). In fact, multifaceted pathways of AD imply numerous links described in detail in excellent reviews (Perrin et al., 2009).
Elevated levels of Hsp70 are found in AD, whereas the chaperones obviously attenuate the disease (Romi et al., 2011). For example, protein aggregation and neuronal death together with AD-like symptoms, caused by the use of paraquat, a popular herbicide in many countries, are accompanied by a reduced Hsp70 expression (Moyano et al., 2021). On the other hand, an upregulation of Hsp70 has correlated with a decline in Aβ content (Sun et al., 2017).
Excessive endogenous Hsp70 increases the efficiency of Aβ-degrading enzymes (Hoshino et al., 2011). Rivera and coll. Have observed Hsp70 affecting the Aβ assembling process in vitro preventing oligomer formation. Moreover, the presence of Hsp70 reduced the Aβ peptide-induced toxicity of cultured neurons (Rivera et al., 2018). In drosophila genetic models, hyper-expressing secreted Hsp70 (secHsp70) together with mutant Aβ42, reveal that the extracellular Hsp70 only provided a protective action (De Mena et al., 2017). Some studies have found a similar pattern of Hsp70 impacts for both localizations. Thus, the potential for cognitive protection remained the same for both extra- and intracellular Hsp70 for drosophilae with Aβ accumulation regardless of their age or exposure (Martín-Peña et al., 2018).
Cytoskeletal instability as a result of the production of impaired tau protein is another important component of AD pathology (Choi et al., 2020). It is generally accepted that an increased activity of tau kinases, especially of glycogen-synthase kinase 3 (GSK-3), extracellular signal-regulated kinase (ERK), and p38, leads to the hyperphosphorylation of tau (Hartz et al., 2023). As a result, tau molecules aggregate into double threads forming a net of NFT, that further intensifies the Aβ accumulation (Uematsu et al., 2018). It is shown that valosin-containing protein (p97; VCP) can eliminate the NFT with the help of Hsp70 (Saha et al., 2023). Overexpressed Hsp70 enables degradation or dephosphorylation of pathological tau improving stability of microtubules (Lackie et al., 2017).
Despite all the positive effects, at the late stages of cellular pathology Hsp70 loses its protective functions and forms epichaperomes representing inert long-living scaffolds (Bolaender et al., 2021). This phenomenon enables protein connectivity-based dysfunction and might aggravate the neurodegeneration (Inda et al., 2020). Additionally, Aβ and tau suppressive activity of Hsp70 has been shown to be abolished in the presence of ε4 isoform of apolipoprotein E (ApoE), one of the most recognizable factors contributing to AD (Osorio et al., 2007; Serrano-Pozo et al., 2021). Finally, although the subchronic intranasal administration of human Hsp70 has improved the course of disease in familial AD murine models, innate immunity and antigen presentation have been upregulated at the same time (Evgen’ev et al., 2019).
3.3. Hsp70 in Parkinson’s disease
Parkinson’s disease (PD) is a common neurodegeneration, clinically displayed in bradykinesia, postural instability, rigidity, and tremor (Ascherio and Schwarzschild, 2016). The disorder is caused by a progressive death of dopaminergic neurons in the substantia nigra (Beal, 2010). The hallmark of the disease required to confirm the diagnosis is intracellular inclusions called Lewy bodies (LB) (Spillantini et al., 1997; Sipilä et al., 2023). LB are composed of ubiquitinated abnormal protein called α-synuclein (α-Syn) (George, 2002). In addition to α-Syn, some other causative players have been shown to contribute to the disease: Parkin, encoded by PARK2 gene; phosphatase and tensin homolog-induced kinase 1, encoded by PINK1 or PARK6; protein deglycase J-1 (DJ-1), encoded by PARK7; and leucine-rich repeat kinase 2 (LRRK2), encoded by PARK8 (Poewe et al., 2017).
In PD, Hsp70 overexpression is common in damaged cells, generally in surviving neurons (Dickson, 2018; Jellinger, 2019). Consistently, Drosophila models have disclosed Hsp70 to delay death of dopaminergic cells (Auluck and Bonini, 2002). On one hand, the benefit of Hsp70 might be explained by its ability to enhance autophagy (Moors et al., 2017; McKinnon et al., 2020). Nonetheless, mitophagy and elimination of Parkin also require HspA1 presence, and of HspA1A mainly, as HspA1L potential is weaker (Hasson et al., 2013). However, Hsp70 machinery in PD is not acting alone, but strongly depends on Hsp40, Hsp90, and Hsp70-Hsp90-organizing protein (HOP) (Ebrahimi-Fakhari et al., 2012; Wu et al., 2019; Hu et al., 2021). Moreover, small molecular chaperones, e. g. clusterin (ApoJ), are probably more prominent to manage α-Syn aggregation in PD (Lenzi et al., 2020).
3.4. Hsp70 in Huntington’s disease
Huntington’s disease (HD) is a complex motor (choreal signs are rather common), cognitive, and mental neurodegenerative disorder, which is inherited via an autosomal dominant pattern (Joshi et al., 2021). HD is the most common representative of a wide range of polyglutamine-associated diseases (polyQ), including also spinocerebellar ataxias and dentatorubral-pallidoluysian atrophy (Carroll et al., 2018). All of them imply a translation of polyQ-chains forming Htt protein after posttranslational modification (Bates et al., 2015). Htt aggregates in essentially all neuronal and astrocytic compartments (Joshi et al., 2021; Lange et al., 2023).
Hsp70 may promote the collapse of polyQ chains (Choudhury et al., 2016; Davis et al., 2020; Gupta et al., 2020). In insects, Hsc70 is responsible for the effect (Rai and Tapadia, 2022). However, there is a lack of data supporting active Hsp70 engagement in the treatment of these disorders in mammals (Pratt et al., 2015; Reis et al., 2017). Maheshwari and coll. Have tried steroid hormones to manage Hsp70 machinery in HD, but no specific action is shown (Maheshwari et al., 2014). Some studies propose an anti-apoptotic role of Hsp70 in HD (Sabirzhanov et al., 2012). Besides, an indirect explanation of Hsp70 relevance in HD is given by the fact that progenitor nerve cells express more Hsp40 (co-chaperone of Hsp70) than mature neurons, whereas polyQ-associated disorders usually manifest in adult persons and not in childhood (Thiruvalluvan et al., 2020). Perhaps, the Hsp40 is the key factor to trigger polyQ removal.
3.5. Hsp70 in amyotrophic lateral sclerosis and frontotemporal dementia
Amyotrophic lateral sclerosis (ALS) is a progressive motor neuronal disorder (Amico and Antel, 1981). Despite a long history of studies, the interactions between its key actors are still not completely clear (Al-Chalabi et al., 2017). Superoxide dismutase 1 (SOD-1) is often considered to be crucial in pathology (Cleveland, 1999). However, products of fused-in-sarcoma (FUS) gene are important contributors to protein aggregation, and such faulty proteins as transactive response DNA binding protein 43 kDa (TDP-43), C9orf72, and ubiquilin-2 are also involved (Thomas et al., 2013). Moreover, there is a second row of proteins, which are able to aggravate the course of the disorder. So, NF-kappa-B activator-binding kinase 1 of TNF receptor-associated factor’s family member (TBK1), optineurin, and p62 participate in impaired autophagy, while vesicle-associated membrane protein-associated protein B/C (VAP-B) is typical for proteasomal failure. Although, VCP takes part in both machineries (Kalmar and Greensmith, 2017).
ALS and frontotemporal dementia (FTD) may be regarded together because of similar genomics, proteomics, metabolomics, and transcriptomics (Menéndez-González, 2023). Mandrioli et al. (2019) also mention that both diseases are accompanied by neuroinflammation with inflammasomes. FTD is a group of similar threatening and rapidly progressing cognitive disorders with a rather high mortality (Wang et al., 2022). The pathology comprises a storage of pathological tau, Fus, and TDP-43 with predominant harm to the neurons in the frontal and temporal cortex (Josephs et al., 2011). Furthermore, repeated sequences of G4C2 in C9orf72 genes are notorious for the synthesis of dipeptide repeat proteins (DPR) or poly-Gly-Ala (poly-GA), tending to aggregate (Lee et al., 2023). Interestingly, DPR complexes are able to be transported to neighboring cells, thereby hindering their clearance (Khosravi et al., 2020).
Hsp70 is found to assist the elimination of the abnormal proteins, especially in the DPR-related pathology (Deng et al., 2011). Generally, in ALS and FTD Hsp70 act via either autophagy to prevent aggregation of RNA-binding proteins (Mandrioli et al., 2019) or proteasomal machinery to fix DPR-induced damage. In proteasomes, ubiquilin-2 provides a rapid Hsp70 binding to poly-GA (Renaud et al., 2019). The intermediate region of ubiquilin-2 molecule includes a flexible proline-X-X-proline (PXXP) motif, which is perhaps accessible for concurrent catalysis or inhibition (Zhang et al., 2021). Meanwhile, these RNA-binding proteins comprise TDP-43 and Fus, and may be cleaved by such kinds of autophagy as chaperone-mediated autophagy and aggrephagy (Thomas et al., 2013). Aggrephagy implies a consumption of labeled stress granules from aberrant RNA (Ripin and Parker, 2022). Interestingly, TDP-43 and ubiquilin-2 seem to be tightly interacting despite their different roles in proteasomal machinery and autophagy (Seelaar et al., 2007; Wang et al., 2008).
4. Hsp70 as a target to treat neurodegeneration
Current drug options in NDs probably lack Hsp70 usage to improve neuronal protein quality control (Table 2). Some medications, such as BGP-15 (O-[3-piperidino-2-hydroxy-1-propyl]-nicotinic acid amidoxime dihydrochloride) and celastrol, have not shown a real positive Hsp70-mediated impact on neurodegeneration (Kalmar and Greensmith, 2017). New candidates should pass by a number of studies, and in vitro ATP-ase tests are preferred initially (Repalli and Meruelo, 2015). Surprisingly, in some cases Hsp70-related drugs have even aggravated NDs. Johnson and coll. Emphasize a positive correlation between spinocerebellar ataxia and elevated Hsc70 content in a Drosophila model (Johnson et al., 2020), though it may be explained either as a harmful phenomenon or cell resistance to the pathology.
4.1. Hsp70 enhancers in NDs
Heat shock factors are first possible tools to rule Hsp70 in NDs (Verma et al., 2014). So, HSF-1 takes part in numerous pathways, thereby being a key point of regulation (Kim et al., 2017). In particular, HSF-1 is trimerized to bind a sequence called the heat shock element in Hsp70 gene promotors (Kondo et al., 2013). However, an excessive production of HSF-1 in cerebellar neurons has decreased Htt-related toxicity in rats not via a direct Hsp70 upregulation, but via such coactor as BAG-3 (Verma et al., 2014). In Hsp70-knockout (ko) mice, increased HSF-1 levels have also improved the number of dopaminergic neurons in treatment by U-133, a compound derived from sea urchins (Ekimova et al., 2018). Independently of direct or indirect Hsp70 engagement, geranylgeranyl acetone (GGA; teprenone) mitigates neuronal damage via HSF-1 activation through the ERK/p38 MAPK pathway (Sun et al., 2017) (Table 3).
Multivector drugs, that affect different Hsp groups, attract a lot of interest. These are mainly geldanamycin and its derivatives, especially 17-AAG, 17-DMAG, IPI-504, and radicicol, which work as Hsp90-inhibitors and HSF-1-mediated Hsp70 inducers at the same time (Alam et al., 2017). Although, a predominance of Hsp70 or Hsp90 is still debatable (Rutledge et al., 2022). Several mechanisms of neuronal action can also be combined in a single medication. Thus, riluzole, which is known as anti-ALS basic treatment, is officially described by its producers as an antagonist of glutamate-associated excitotoxicity, but its effects are also provided by HSF-1 upregulation (Miller et al., 2012; Petri et al., 2023). Yang and coll. Report that riluzole may also enable Htt cleavage (Yang et al., 2008). Actually, there is no consensus on dynamics of riluzole action in literature.
Furthermore, some well-known medications may take part in the Hsp70-associated management of NDs. For instance, colchicine is actively tested together with riluzole due to its upregulation of HspB8 expression and blocking of TDP-43 accumulation (Mandrioli et al., 2019). Konturek et al. (2005) also studied pioglitazone and discovered its ability to improve Hsp70 content in the pancreas. However, a concomitant intake of pioglitazone and riluzole exhibits no clinical benefit in trials for ALS (Dupuis et al., 2012). In addition, fenofibrate causes a synchronous decrease in Hsp90 levels and elevation of Hsp70 levels, and cognitive dysfunction regresses in a rat model of dementia (Rizk et al., 2022). However, valproic acid enhances Hsp70 levels with no impact on Hsp90-machinery. Finally, carbenoxolone is efficient in proteasomal cleavage of aggregates via HSF-1 upregulation in PD rat models in the presence of rotenone (Thakur and Nehru, 2014).
Some Hsp70 enhancers with no clear mechanism found are suggested. For example, sleep deprivation-induced learning/memory impairment has been shown to recover after intracerebroventricular luteolin administration, while an increase in Hsp70 levels have accompanied the treatment (Rahimpour et al., 2023). Then, FLZ or phenlarmide alleviates motor dysfunction in animal PD models with an overexpression of Hsp70 (Kong et al., 2011; Bao et al., 2017). Additionally, some mechanisms are being developed with no pharmaceutical compounds proposed yet. Thus, cysteine string protein α (CSPα) phosphorylation by protein kinase C-γ promotes Hsp70 activity (Shirafuji et al., 2018).
Surprisingly, relative rare NDs tend to be more easily managed by Hsp70-recruiting drug options. So, arimoclomol (BRX-220), which is a low-molecular-weight hydroxylamine derivative Hsp70 enhancer, is tested in animal models of Fabry, Sandhoff, and Niemann-Pick type C (NPC) diseases (Kirkegaard et al., 2016). Mice of Gla−/− (Fabry), Hexb−/− (Sandhoff), and Npc1−/− (NPC) lines have shown a clinical improvement after arimoclomol intake. Hsp70 inducers/coinducers are generally convincing for ALS treatment (Kalmar et al., 2014; Kalmar and Greensmith, 2017), and it is especially true for arimoclomol (Phukan, 2010). Interestingly, that arimoclomol alone is effective but not efficient. It requires a preliminary heat shock induction to exhibit its potential (Kalmar et al., 2008).
Hsp70 inducers, mentioned above, are however very different by their biochemical features. This diversity has to be respected in practical implementation. For example, the compounds of geldanamycin group, in addition to their initial Hsp-related activity in cells, are able to prevent a transfer of receptors to steroid hormones into the nucleus, thereby providing a longer immune association (Czar et al., 1997). Geranyl geranylacetone acts via NF-κB-COX-2 axis to rule gene promoters, so cyclooxygenase-related adverse effects may be provoked (Nishida et al., 2007).
4.2. Recombinant Hsp70 and Hsp70-related genetic therapy
Exogenous recombinant Hsp70 (rHsp70ex) is a direct form of the chaperones that can be easily delivered into the body. It seems to be beneficial in NPC and AD models, particularly in familial AD (Kakimura et al., 2002; Mengel et al., 2021). For instance, rHsp70ex enhances memory and learning in AD models in vivo (Zatsepina et al., 2021). Then, cerebral and hippocampal cortex accumulates labeled rHsp70ex after intranasal administration (Yurinskaya et al., 2015). Further, mice with familial AD display declined Aβ levels and partial cognitive recovery after rHsp70ex administration (Bobkova et al., 2014; Evgen’ev et al., 2017). In addition, murine models of AD reflect a downregulation of neuroinflammatory markers after rHsp70ex in transcriptomic analysis of hippocampal neurons (Heppner et al., 2015; Yurinskaya et al., 2016). Moreover, neuroblasts proliferate and differentiate after intranasal rHSP70ex administration, perhaps due to the activation of cAMP 26 responsive element binding protein (CREB) cascade (Kwon et al., 2019). The treatment by rHsp70ex results in resistance to oxidative stress via mature endosomes and lysosomes with decreased apoptotic activity (Subrizi et al., 2015).
In a gene therapy study, rHsp70 has reached impaired dopaminergic neurons via an adenoviral vector with further decrease of neuronal loss (Dong et al., 2005). A similar effect is seen in Drosophila models for Hsp70 boosts both via gene therapy and induction by tanespimycin, a geldanamycin derivative (Zhang et al., 2016). Severity of PD signs has mitigated in sirtuin-1-transgenic mice due to the activation of HspA4 (Yang et al., 2022). However, HspA4 is not traditionally considered to belong to Hsp70 (Kaneko et al., 1997), although many controversial data appear recently (Shang et al., 2021; Abd El-Fadeal et al., 2023). A separate field of study includes epigenetic modifications of the chaperome, but it still remains weakly studied (Taldone et al., 2014).
4.3. Allosteric modulators of Hsp70
Allosteric modification represents an alternative way to control Hsp70 function. Surprisingly, practically all modulators can behave as Hsp inhibitors, although their overall impact depends on many factors. Avoiding concurrence, allosteric binding stays selective, supporting a comprehensive understanding of Hsp70-involving cascades (Ekimova and Plaksina, 2016; Gleixner et al., 2016; Ferraro et al., 2019). First, rhodacyanine-derived compounds are found to modify Hsp70 cooperation with its coactors in an allosteric site, inhibiting a reversive transformation of the chaperone’s molecule from ADP- into ATP-binding state and thereby improving protein holding (Li et al., 2016). The group includes MKT-077 and YM-01, which have been investigated for anti-AD activity as they may provoke Hsp70-mediated decline in pathological tau content in vitro (Abisambra et al., 2013; Martin et al., 2016). Furthermore, YM-08 is the next generation with a milder but also a less toxic action (Miyata et al., 2013), whereas its halogen-recruiting modification (JG-23) is even more chemically stable (Chang et al., 2021; Shao et al., 2021).
Allosteric Hsp70 regulation has been also proposed for some other pharmacological groups. For instance, phenothiazines such as methylene blue and azure C decrease total tau and phospho-tau levels due to the anti-ATP-ase activity, while the benefit of this effect is debatable (Martin et al., 2016; Lo Cascio and Kayed, 2018). Also, the neurotrophic compound J147 prevents synaptic loss in a transgenic murine AD model (Chen et al., 2011). However, J147 directly affects ATP-synthase, thereby triggering a bounce in intracellular calcium levels with a launch of 5′-adenosine monophosphate-activated protein kinase and mammalian target of rapamycin (AMPK/mTOR) pathway by kinase β of calcium/calmodulin-dependent protein kinase (CAMKK2) affecting mitochondrial metabolism (Goldberg et al., 2018). There is no proven Hsp70-involving action for J147.
Despite the new horizons of Hsp70 usage, current clinical trials focus mainly on Hsp90-modifiers or nonselective Hsp-controllers because of a better understanding of Hsp90 machinery (Thirstrup et al., 2016). Even more, pridopidine has been studied for HD treatment with a profound search on the impact of S1R chaperones and few data about Hsp engagement (Shenkman et al., 2021). However, chemical and biological modifications of Hsp70-involving pathways is a mighty impact spot in future management of NDs (Fontaine et al., 2016).
Perhaps, combining chemically different medications would provide additional options. So, Hsp70 overexpression strengthens in concomitant administration of Hsp90 inhibitors together with histone deacetylase inhibitors (Kuta et al., 2020). Some drugs are slightly effective with no strict pathway clear yet. That is, for example, the phenomenon of myricetin, bortezomib, and MG-132, counterparts that affect proteasome-mediated Hsp70 action (Joshi et al., 2019). Generally, these proteasome modulators (or JG substances) are studied for tumor cell management, as Grp78 and mtHsp70 machinery suffers in their presence (Cagala et al., 2020; Ferguson et al., 2022).
4.4. Nontrivial approaches for Hsp70 management in NDs
Curiously, physical methods might also contribute to Hsp70 machinery. For example, near-infrared irradiation tends to improve Hsp70 activity in the splenic and hepatic regions of mice (Escudero-Duch et al., 2023). The idea has also been developed for sauna heating inducing Hsp70 activation (Hunt et al., 2020). Hsp70 levels are generally elevated in increased physical activity (Kim et al., 2022). Phytotherapy also may represent a feasible approach. So, an extract of African walnut, or Tetracarpidium conophorum, is recently shown to improve the PD-like signs in rats, probably via Hsp70 modulation (Tokunbo et al., 2023). Neferine, derived from seeds of lotus plants, seems to increase neuronal tolerance to ischemia via Hsp70-induction machinery (Sengking et al., 2022).
5. Discussion
Molecular chaperones, mainly Hsp70 and Hsp90, play one of central biochemical roles providing structural volatility of proteins (Gupta et al., 2020). Hsp70 content should change rapidly to answer challenges of constantly transforming environment, and that is especially true for neurons as there are almost no proliferative or reconstructive machinery. In NDs, neuronal chaperome is usually impaired, so Hsp70 has a great potential to manage the pathology (Kim et al., 2021).
The challenge is that Hsp70 content in NDs shows no strict linear relation with the development of disease. For example, a decrease in plasma Hsp levels is observed at initial stages of AD and FTD, but changed into a recovery in moderate and severe cases (Chanteloup et al., 2019). It might be explained by gradual Hsp70 accumulation in the brain to repair neurons. However, the clinical implementation of this theoretical basis is more complex, because the state-of-the-art in Hsp70 studies for NDs still demonstrates a lot of white spots.
Nevertheless, Hsp70 have generally been found to be useful in neuronal functional and/or structural damage (Beretta and Shala, 2022). For instance, geranylgeranyl acetone induces Hsp70 expression in mice via HSF-1 with proven cognitive improvement (Sun et al., 2017). However, we still have no certain concept about principal differences between extra- and intracellular effects of Hsp70 in NDs. Theoretically, an intrinsic Hsp70 in NDs is responsible for aggregate cleavage, whereas an extrinsic Hsp70 joins immune interactions.
We consider Hsp70 to be a large field of studies in cellular and molecular biology for the upcoming years. For instance, we lack comprehensive research of Hsp70 potential in transgenic animals. Additionally, it seems that a well-known practice of physiological experiments with moderate exposure to high temperatures in humans and rats would obtain a second wind for testing the issue of Hsp70-modifying medications and transgenic Hsp70 for NDs. In a recent work, we have already tried an approach to synchronize physiological and morphological findings by laser speckle contrast imaging (Piavchenko et al., 2021; Konovalov et al., 2023).
Author contributions
GP and AV: conceptualization. AV, VK, and AM: writing (original draft preparation). IM, AB, OB, GP, and AV: writing (review and editing). EK and AV: rendering, style design, and content presentation. All authors have read and agreed to the published version of the manuscript.
Funding
This study was supported by the Russian Science Foundation (project no. 23–25-00448, https://www.rscf.ru/project/23-25-00448/). Our review launches a series of works according to the grant in study of neuroimmunological impacts of extra- and intracellular Hsp70 in murine models of neurodegeneration.
Conflict of interest
The authors declare that the research was conducted in the absence of any commercial or financial relationships that could be construed as a potential conflict of interest.
Publisher’s note
All claims expressed in this article are solely those of the authors and do not necessarily represent those of their affiliated organizations, or those of the publisher, the editors and the reviewers. Any product that may be evaluated in this article, or claim that may be made by its manufacturer, is not guaranteed or endorsed by the publisher.
References
Abd El-Fadeal, N. M., Ellawindy, A., Jeraiby, M. A., Qusti, S. Y., Alshammari, E. M., Alzahrani, A. K., et al. (2023). HSP70 expression signature in renal cell carcinoma: a clinical and bioinformatic analysis approach. Genes (Basel) 14:355. doi: 10.3390/genes14020355
Abisambra, J., Jinwal, U. K., Miyata, Y., Rogers, J., Blair, L., Li, X., et al. (2013). Allosteric heat shock protein 70 inhibitors rapidly rescue synaptic plasticity deficits by reducing aberrant tau. Biol. Psychiatry 74, 367–374. doi: 10.1016/j.biopsych.2013.02.027
Alam, Q., Alam, M. Z., Sait, K. H. W., Anfinan, N., Noorwali, A. W., Kamal, M. A., et al. (2017). Translational shift of HSP90 as a novel therapeutic target from Cancer to neurodegenerative disorders: an emerging trend in the cure of Alzheimer’s and Parkinson’s diseases. Curr. Drug Metab. 18, 868–876. doi: 10.2174/1389200218666170728115606
Al-Chalabi, A., van den Berg, L. H., and Veldink, J. (2017). Gene discovery in amyotrophic lateral sclerosis: implications for clinical management. Nat. Rev. Neurol. 13, 96–104. doi: 10.1038/nrneurol.2016.182
Amico, L. L., and Antel, J. P. (1981). Amyotrophic lateral sclerosis: current concepts. Postgrad. Med. 70, 50–61. doi: 10.1080/00325481.1981.11715822
Ascherio, A., and Schwarzschild, M. A. (2016). The epidemiology of Parkinson’s disease: risk factors and prevention. Lancet Neurol. 15, 1257–1272. doi: 10.1016/S1474-4422(16)30230-7
Auluck, P. K., and Bonini, N. M. (2002). Pharmacological prevention of Parkinson disease in Drosophila. Nat. Med. 8, 1185–1186. doi: 10.1038/nm1102-1185
Bao, X. Q., Wang, X. L., and Zhang, D. (2017). FLZ attenuates α-Synuclein-induced neurotoxicity by activating heat shock protein 70. Mol. Neurobiol. 54, 349–361. doi: 10.1007/s12035-015-9572-9
Bates, G. P., Dorsey, R., Gusella, J. F., Hayden, M. R., Kay, C., Leavitt, B. R., et al. (2015). Huntington disease. Nat Rev Dis Primers. 1:15005. doi: 10.1038/nrdp.2015.5
Beretta, G., and Shala, A. L. (2022). Impact of heat shock proteins in neurodegeneration: possible Therapeutical targets. Ann. Neurosci. 29, 71–82. doi: 10.1177/09727531211070528
Bi, M., Kang, W., and Sun, Y. (2023). Expression of HSPA14 in patients with acute HIV-1 infection and its effect on HIV-1 replication. Front. Immunol. 14:1123600. doi: 10.3389/fimmu.2023.1123600
Bobkova, N. V., Garbuz, D. G., Nesterova, I., Medvinskaya, N., Samokhin, A., Alexandrova, I., et al. (2014). Therapeutic effect of exogenous Hsp70 in mouse models of Alzheimer’s disease. J. Alzheimers Dis. 38, 425–435. doi: 10.3233/JAD-130779
Bolaender, A., Zatorska, D., He, H., Joshi, S., Sharma, S., Digwal, C. S., et al. (2021). Chemical tools for epichaperome-mediated interactome dysfunctions of the central nervous system. Nat. Commun. 12:4669. doi: 10.1038/s41467-021-24821-2
Braun, J. E. A. (2023). Extracellular chaperone networks and the export of J-domain proteins. J. Biol. Chem. 299:102840. doi: 10.1016/j.jbc.2022.102840
Breijyeh, Z., and Karaman, R. (2020). Comprehensive review on Alzheimer’s disease: causes and treatment. Molecules 25:5789. doi: 10.3390/molecules25245789
Cagala, M., Pavlikova, L., Seres, M., Kadlecikova, K., Breier, A., and Sulova, Z. (2020). Development of resistance to endoplasmic reticulum stress-inducing agents in mouse leukemic L1210 cells. Molecules 25:2517. doi: 10.3390/molecules25112517
Campanella, C., Pace, A., Caruso Bavisotto, C., Marzullo, P., Marino Gammazza, A., Buscemi, S., et al. (2018). Heat shock proteins in Alzheimer’s disease: role and targeting. Int. J. Mol. Sci. 19:2603. doi: 10.3390/ijms19092603
Carroll, L. S., Massey, T. H., Wardle, M., and Peall, K. J. (2018). Dentatorubral-pallidoluysian atrophy: an update. Tremor Other Hyperkinet Mov (N Y). 8:577. doi: 10.7916/D81N9HST
Chang, C. S., Kumar, V., Lee, D. Y., Chen, Y., Wu, Y. C., Gao, J. Y., et al. (2021). Development of novel Rhodacyanine-based heat shock protein 70 inhibitors. Curr. Med. Chem. 28, 5431–5446. doi: 10.2174/0929867328666210203204254
Chanteloup, G., Cordonnier, M., Moreno-Ramos, T., Pytel, V., Matías-Guiu, J., Gobbo, J., et al. (2019). Exosomal HSP70 for monitoring of frontotemporal dementia and Alzheimer’s disease: clinical and FDG-PET correlation. J. Alzheimers Dis. 71, 1263–1269. doi: 10.3233/JAD-190545
Chaudhary, R., Baranwal, V. K., Kumar, R., Sircar, D., and Chauhan, H. (2019). Genome-wide identification and expression analysis of Hsp70, Hsp90, and Hsp100 heat shock protein genes in barley under stress conditions and reproductive development. Funct. Integr. Genomics 19, 1007–1022. doi: 10.1007/s10142-019-00695-y
Chen, Q., Prior, M., Dargusch, R., Roberts, A., Riek, R., Eichmann, C., et al. (2011). A novel neurotrophic drug for cognitive enhancement and Alzheimer’s disease. PLoS One 6:e27865. doi: 10.1371/journal.pone.0027865
Choi, H., Kim, H. J., Yang, J., Chae, S., Lee, W., Chung, S., et al. (2020). Acetylation changes tau interactome to degrade tau in Alzheimer’s disease animal and organoid models. Aging Cell 19:e13081. doi: 10.1111/acel.13081
Choudhury, K. R., Das, S., and Bhattacharyya, N. P. (2016). Differential proteomic and genomic profiling of mouse striatal cell model of Huntington’s disease and control; probable implications to the disease biology. J. Proteome 132, 155–166. doi: 10.1016/j.jprot.2015.11.007
Ciechanover, A., and Kwon, Y. T. (2017). Protein quality control by molecular chaperones in neurodegeneration. Front. Neurosci. 11:185. doi: 10.3389/fnins.2017.00185
Clark, M. S., Peck, L. S., and Thyrring, J. (2021). Resilience in Greenland intertidal Mytilus: the hidden stress defense. Sci. Total Environ. 767:144366. doi: 10.1016/j.scitotenv.2020.144366
Cleveland, D. W. (1999). From Charcot to SOD1: mechanisms of selective motor neuron death in ALS. Neuron 24, 515–520. doi: 10.1016/s0896-6273(00)81108-3
Czar, M. J., Galigniana, M. D., Silverstein, A. M., and Pratt, W. B. (1997). Geldanamycin, a heat shock protein 90-binding benzoquinone ansamycin, inhibits steroid-dependent translocation of the glucocorticoid receptor from the cytoplasm to the nucleus. Biochemistry 36, 7776–7785. doi: 10.1021/bi970648x
Davis, A. K., Pratt, W. B., Lieberman, A. P., and Osawa, Y. (2020). Targeting Hsp70 facilitated protein quality control for treatment of polyglutamine diseases. Cell. Mol. Life Sci. 77, 977–996. doi: 10.1007/s00018-019-03302-2
de Graff, A. M., Mosedale, D. E., Sharp, T., Dill, K. A., and Grainger, D. J. (2020). Proteostasis is adaptive: balancing chaperone holdases against foldases. PLoS Comput. Biol. 16:e1008460. doi: 10.1371/journal.pcbi.1008460
De Mena, L., Chhangani, D., Fernandez-Funez, P., and Rincon-Limas, D. E. (2017). secHsp70 as a tool to approach amyloid-β42 and other extracellular amyloids. Fly (Austin). 11, 179–184. doi: 10.1080/19336934.2017.1291104
de Toda, I. M., Vida, C., Ortega, E., and De La Fuente, M. (2016). Hsp70 basal levels, a tissue marker of the rate of aging and longevity in mice. Exp. Gerontol. 84, 21–28. doi: 10.1016/j.exger.2016.08.013
Deng, H. X., Chen, W., Hong, S. T., Boycott, K. M., Gorrie, G. H., Siddique, N., et al. (2011). Mutations in UBQLN2 cause dominant X-linked juvenile and adult-onset ALS and ALS/dementia. Nature 477, 211–215. doi: 10.1038/nature10353
Dickson, D. W. (2018). Neuropathology of Parkinson disease. Parkinsonism Relat. Disord. 46, S30–S33. doi: 10.1016/j.parkreldis.2017.07.033
Dong, Z., Wolfer, D. P., Lipp, H. P., and Büeler, H. (2005). Hsp70 gene transfer by adeno-associated virus inhibits MPTP-induced nigrostriatal degeneration in the mouse model of Parkinson disease. Mol. Ther. 11, 80–88. doi: 10.1016/j.ymthe.2004.09.007
Dubaniewicz, A. (2018). Teoria danger jako wspólny mechanizm indukcji sarkoidozy przez czynniki infekcyjne i nieinfekcyjne – rola czynników środowiskowych i autoimmunizacji [danger theory as a common mechanism of sarcoidosis induction by infectious and non- infectious factors - a role of environmental factors and autoimmunity]. Pol Merkur Lekarski 44, 97–100.
Dupuis, L., Dengler, R., Heneka, M. T., Meyer, T., Zierz, S., Kassubek, J., et al. (2012). A randomized, double blind, placebo-controlled trial of pioglitazone in combination with riluzole in amyotrophic lateral sclerosis. PLoS One 7:e37885. doi: 10.1371/journal.pone.0037885
Ebrahimi-Fakhari, D., Wahlster, L., and McLean, P. J. (2012). Protein degradation pathways in Parkinson’s disease: curse or blessing. Acta Neuropathol. 124, 153–172. doi: 10.1007/s00401-012-1004-6
Ekimova, I. V., and Plaksina, D. V. (2016). Effect of quercetin on neurodegenerative and compensatory processes in nigrostriatal system in a model of preclinical PARKINSON’s disease stage in rats. Ross. Fiziol. Zh. Im. I M Sechenova 102, 647–658.
Ekimova, I. V., Plaksina, D. V., Pastukhov, Y. F., Lapshina, K. V., Lazarev, V. F., Mikhaylova, E. R., et al. (2018). New HSF1 inducer as a therapeutic agent in a rodent model of Parkinson’s disease. Exp. Neurol. 306, 199–208. doi: 10.1016/j.expneurol.2018.04.012
Escudero-Duch, C., Muñoz-Moreno, L., Martin-Saavedra, F., Sanchez-Casanova, S., Lerma-Juarez, M. A., and Vilaboa, N. (2023). Remote control of transgene expression using noninvasive near-infrared irradiation. J. Photochem. Photobiol. B 242:112697. doi: 10.1016/j.jphotobiol.2023.112697
Espinoza, M. F., Nguyen, K. K., Sycks, M. M., Lyu, Z., Quanrud, G. M., Montoya, M. R., et al. (2022). Heat shock protein Hspa13 regulates endoplasmic reticulum and cytosolic proteostasis through modulation of protein translocation. J. Biol. Chem. 298:102597. doi: 10.1016/j.jbc.2022.102597
Evgen’ev, M., Bobkova, N., Krasnov, G., Garbuz, D., Funikov, S., Kudryavtseva, A., et al. (2019). The effect of human HSP70 administration on a mouse model of Alzheimer’s disease strongly depends on transgenicity and age. J. Alzheimers Dis. 67, 1391–1404. doi: 10.3233/JAD-180987
Evgen’ev, M. B., Krasnov, G. S., Nesterova, I. V., Garbuz, D. G., Karpov, V. L., Morozov, A. V., et al. (2017). Molecular mechanisms underlying neuroprotective effect of intranasal administration of human Hsp70 in mouse model of Alzheimer’s disease. J. Alzheimers Dis. 59, 1415–1426. doi: 10.3233/JAD-170398
Feng, H., Guo, Z., Chen, X., Liu, K., Li, H., Jia, W., et al. (2022). Excessive HSP70/TLR2 activation leads to remodeling of the tumor immune microenvironment to resist chemotherapy sensitivity of mFOLFOX in colorectal cancer. Clin. Immunol. 245:109157. doi: 10.1016/j.clim.2022.109157
Ferguson, I. D., Lin, Y. T., Lam, C., Shao, H., Tharp, K. M., Hale, M., et al. (2022). Allosteric HSP70 inhibitors perturb mitochondrial proteostasis and overcome proteasome inhibitor resistance in multiple myeloma. Cell Chem Biol. 29, 1288–1302.e7. doi: 10.1016/j.chembiol.2022.06.010
Ferraro, M., D’Annessa, I., Moroni, E., Morra, G., Paladino, A., Rinaldi, S., et al. (2019). Allosteric modulators of HSP90 and HSP70: dynamics meets function through structure-based drug design. J. Med. Chem. 62, 60–87. doi: 10.1021/acs.jmedchem.8b00825
Fontaine, S. N., Martin, M. D., and Dickey, C. A. (2016). Neurodegeneration and the heat shock protein 70 machinery: implications for therapeutic development. Curr. Top. Med. Chem. 16, 2741–2752. doi: 10.2174/1568026616666160413140741
Gabriele, T., Tavaria, M., Kola, I., and Anderson, R. L. (1996). Analysis of heat shock protein 70 in human chromosome 21 containing hybrids. Int. J. Biochem. Cell Biol. 28, 905–910. doi: 10.1016/1357-2725(96)00027-1
Gauthier, S., Feldman, H. H., Schneider, L. S., Wilcock, G. K., Frisoni, G. B., Hardlund, J. H., et al. (2016). Efficacy and safety of tau-aggregation inhibitor therapy in patients with mild or moderate Alzheimer’s disease: a randomized, controlled, double-blind, parallel-arm, phase 3 trial. Lancet 388, 2873–2884. doi: 10.1016/S0140-6736(16)31275-2
George, J. M. (2002). The synucleins. Genome Biol. 3:REVIEWS3002. doi: 10.1186/gb-2001-3-1-reviews3002
Gleixner, A. M., Posimo, J. M., Pant, D. B., Henderson, M. P., and Leak, R. K. (2016). Astrocytes surviving severe stress can still protect neighboring neurons from Proteotoxic injury. Mol. Neurobiol. 53, 4939–4960. doi: 10.1007/s12035-015-9427-4
Goldberg, J., Currais, A., Prior, M., Fischer, W., Chiruta, C., Ratliff, E., et al. (2018). The mitochondrial ATP synthase is a shared drug target for aging and dementia. Aging Cell 17:e12715. doi: 10.1111/acel.12715
Gupta, A., Bansal, A., and Hashimoto-Torii, K. (2020). HSP70 and HSP90 in neurodegenerative diseases. Neurosci. Lett. 716:134678. doi: 10.1016/j.neulet.2019.134678
Guzhova, I. V., Arnholdt, A. C., Darieva, Z. A., Kinev, A. V., Lasunskaia, E. B., Nilsson, K., et al. (1998). Effects of exogenous stress protein 70 on the functional properties of human promonocytes through binding to cell surface and internalization. Cell Stress Chaperones 3, 67–77. doi: 10.1379/1466-1268(1998)003<0067:eoespo>2.3.co;2
Guzhova, I., Kislyakova, K., Moskaliova, O., Fridlanskaya, I., Tytell, M., Cheetham, M., et al. (2001). In vitro studies show that Hsp70 can be released by glia and that exogenous Hsp70 can enhance neuronal stress tolerance. Brain Res. 914, 66–73. doi: 10.1016/s0006-8993(01)02774-3
Hartl, F. U., and Hayer-Hartl, M. (2002). Molecular chaperones in the cytosol: from nascent chain to folded protein. Science 295, 1852–1858. doi: 10.1126/science.1068408
Hartz, R. A., Ahuja, V. T., Sivaprakasam, P., Xiao, H., Krause, C. M., Clarke, W. J., et al. (2023). Design, structure-activity relationships, and in vivo evaluation of potent and brain-penetrant Imidazo[1,2-b] pyridazines as glycogen synthase kinase-3β (GSK-3β) inhibitors. J. Med. Chem. 66, 4231–4252. doi: 10.1021/acs.jmedchem.3c00133
Hasson, S. A., Kane, L. A., Yamano, K., Huang, C. H., Sliter, D. A., Buehler, E., et al. (2013). High-content genome-wide RNAi screens identify regulators of parkin upstream of mitophagy. Nature 504, 291–295. doi: 10.1038/nature12748
Havalová, H., Ondrovičová, G., Keresztesová, B., Bauer, J. A., Pevala, V., Kutejová, E., et al. (2021). Mitochondrial hsp70 chaperone system-the influence of post-translational modifications and involvement in human diseases. Int. J. Mol. Sci. 22:8077. doi: 10.3390/ijms22158077
Heppner, F. L., Ransohoff, R. M., and Becher, B. (2015). Immune attack: the role of inflammation in Alzheimer disease. Nat. Rev. Neurosci. 16, 358–372. doi: 10.1038/nrn3880
Heydari, A. R., Takahashi, R., Gutsmann, A., You, S., and Richardson, A. (1994). Hsp70 and aging. Experientia 50, 1092–1098. doi: 10.1007/BF01923466
Hightower, L. E., and Guidon, P. T. Jr. (1989). Selective release from cultured mammalian cells of heat-shock (stress) proteins that resemble glia-axon transfer proteins. J. Cell. Physiol. 138, 257–266. doi: 10.1002/jcp.1041380206
Hoshino, T., Murao, N., Namba, T., Takehara, M., Adachi, H., Katsuno, M., et al. (2011). Suppression of Alzheimer’s disease-related phenotypes by expression of heat shock protein 70 in mice. J. Neurosci. 31, 5225–5234. doi: 10.1523/JNEUROSCI.5478-10.2011
Hu, B., Li, M., Yu, X., Xun, X., Lu, W., Li, X., et al. (2019). Diverse expression regulation of Hsp70 genes in scallops after exposure to toxic Alexandrium dinoflagellates. Chemosphere 234, 62–69. doi: 10.1016/j.chemosphere.2019.06.034
Hu, S., Tan, J., Qin, L., Lu, L., Yan, W., Zhang, H., et al. (2021). Molecular chaperones and Parkinson’s disease. Neurobiol. Dis. 160:105527. doi: 10.1016/j.nbd.2021.105527
Huang, Y. R., and Liu, R. T. (2020). The toxicity and polymorphism of β-amyloid oligomers. Int. J. Mol. Sci. 21:4477. doi: 10.3390/ijms21124477
Hunt, A. P., Minett, G. M., Gibson, O. R., Kerr, G. K., and Stewart, I. B. (2020). Could heat therapy be an effective treatment for Alzheimer’s and Parkinson’s diseases? A Narrative Review. Front Physiol. 10:1556. doi: 10.3389/fphys.2019.01556
Inda, M. C., Joshi, S., Wang, T., Bolaender, A., Gandu, S., Koren Iii, J., et al. (2020). The epichaperome is a mediator of toxic hippocampal stress and leads to protein connectivity-based dysfunction. Nat. Commun. 11:319. doi: 10.1038/s41467-019-14082-5
Jellinger, K. A. (2019). Neuropathology and pathogenesis of extrapyramidal movement disorders: a critical update-I. Hypokinetic-rigid movement disorders. J Neural Transm (Vienna). 126, 933–995. doi: 10.1007/s00702-019-02028-6
Johnson, S. L., Ranxhi, B., Libohova, K., Tsou, W. L., and Todi, S. V. (2020). Ubiquitin-interacting motifs of ataxin-3 regulate its polyglutamine toxicity through Hsc70-4-dependent aggregation. elife 9:e60742. doi: 10.7554/eLife.60742
Jores, T., Lawatscheck, J., Beke, V., Franz-Wachtel, M., Yunoki, K., Fitzgerald, J. C., et al. (2018). Cytosolic Hsp70 and Hsp40 chaperones enable the biogenesis of mitochondrial β-barrel proteins. J. Cell Biol. 217, 3091–3108. doi: 10.1083/jcb.201712029
Josephs, K. A., Hodges, J. R., Snowden, J. S., Mackenzie, I. R., Neumann, M., Mann, D. M., et al. (2011). Neuropathological background of phenotypical variability in frontotemporal dementia. Acta Neuropathol. 122, 137–153. doi: 10.1007/s00401-011-0839-6
Joshi, T., Kumar, V., Kaznacheyeva, E. V., and Jana, N. R. (2021). Withaferin a induces heat shock response and ameliorates disease progression in a mouse model of Huntington’s disease. Mol. Neurobiol. 58, 3992–4006. doi: 10.1007/s12035-021-02397-8
Joshi, V., Mishra, R., Upadhyay, A., Amanullah, A., Poluri, K. M., Singh, S., et al. (2019). Polyphenolic flavonoid (Myricetin) upregulated proteasomal degradation mechanisms: eliminates neurodegenerative proteins aggregation. J. Cell. Physiol. 234, 20900–20914. doi: 10.1002/jcp.28695
Kakimura, J., Kitamura, Y., Takata, K., Umeki, M., Suzuki, S., Shibagaki, K., et al. (2002). Microglial activation and amyloid-beta clearance induced by exogenous heat-shock proteins. FASEB J. 16, 601–603. doi: 10.1096/fj.01-0530fje
Kalmar, B., and Greensmith, L. (2017). Cellular chaperones as therapeutic targets in ALS to restore protein homeostasis and improve cellular function. Front. Mol. Neurosci. 10:251. doi: 10.3389/fnmol.2017.00251
Kalmar, B., Lu, C. H., and Greensmith, L. (2014). The role of heat shock proteins in amyotrophic lateral sclerosis: the therapeutic potential of Arimoclomol. Pharmacol. Ther. 141, 40–54. doi: 10.1016/j.pharmthera.2013.08.003
Kalmar, B., Novoselov, S., Gray, A., Cheetham, M. E., Margulis, B., and Greensmith, L. (2008). Late stage treatment with arimoclomol delays disease progression and prevents protein aggregation in the SOD1 mouse model of ALS. J. Neurochem. 107, 339–350. doi: 10.1111/j.1471-4159.2008.05595.x
Kampinga, H. H., and Craig, E. A. (2010). The HSP70 chaperone machinery: J proteins as drivers of functional specificity. Nat Rev Mol Cell Biol 11, 579–592. doi: 10.1038/nrm2941
Kaneko, Y., Kimura, T., Kishishita, M., Noda, Y., and Fujita, J. (1997). Cloning of apg-2 encoding a novel member of heat shock protein 110 family. Gene 189, 19–24. doi: 10.1016/s0378-1119(96)00807-4
Kao, C. H., Ryu, S. W., Kim, M. J., Wen, X., Wimalarathne, O., and Paull, T. T. (2020). Growth-regulated Hsp70 phosphorylation regulates stress responses and prion maintenance. Mol. Cell. Biol. 40, e00628–e00619. doi: 10.1128/MCB.00628-19
Karvinen, S., Silvennoinen, M., Vainio, P., Sistonen, L., Koch, L. G., Britton, S. L., et al. (2016). Effects of intrinsic aerobic capacity, aging and voluntary running on skeletal muscle sirtuins and heat shock proteins. Exp. Gerontol. 79, 46–54. doi: 10.1016/j.exger.2016.03.015
Khosravi, B., LaClair, K. D., Riemenschneider, H., Zhou, Q., Frottin, F., Mareljic, N., et al. (2020). Cell-to-cell transmission of C9orf72 poly-(Gly-ala) triggers key features of ALS/FTD. EMBO J. 39:e102811. doi: 10.15252/embj.2019102811
Kim, C. K., Park, J. S., Kim, E., Oh, M. K., Lee, Y. T., Yoon, K. J., et al. (2022). The effects of early exercise in traumatic brain-injured rats with changes in motor ability, brain tissue, and biomarkers. BMB Rep. 55, 512–517. doi: 10.5483/BMBRep.2022.55.10.097
Kim, E., Sakata, K., and Liao, F. F. (2017). Bidirectional interplay of HSF1 degradation and UPR activation promotes tau hyperphosphorylation. PLoS Genet. 13:e1006849. doi: 10.1371/journal.pgen.1006849
Kim, K. Y., Shin, K. Y., and Chang, K. A. (2021). Brain-derived Exosomal proteins as effective biomarkers for Alzheimer’s disease: a systematic review and Meta-analysis. Biomol. Ther. 11:980. doi: 10.3390/biom11070980
Kirkegaard, T., Gray, J., Priestman, D. A., Wallom, K. L., Atkins, J., Olsen, O. D., et al. (2016). Heat shock protein-based therapy as a potential candidate for treating the sphingolipidoses. Sci. Transl. Med. 8:355ra118. doi: 10.1126/scitranslmed.aad9823
Kmiecik, S. W., and Mayer, M. P. (2022). Molecular mechanisms of heat shock factor 1 regulation. Trends Biochem. Sci. 47, 218–234. doi: 10.1016/j.tibs.2021.10.004
Kole, A. J., Annis, R. P., and Deshmukh, M. (2013). Mature neurons: equipped for survival. Cell Death Dis. 4:e689. doi: 10.1038/cddis.2013.220
Kondo, N., Katsuno, M., Adachi, H., Minamiyama, M., Doi, H., Matsumoto, S., et al. (2013). Heat shock factor-1 influences pathological lesion distribution of polyglutamine-induced neurodegeneration. Nat. Commun. 4:1405. doi: 10.1038/ncomms2417
Kong, X. C., Zhang, D., Qian, C., Liu, G. T., and Bao, X. Q. (2011). FLZ, a novel HSP27 and HSP70 inducer, protects SH-SY5Y cells from apoptosis caused by MPP (+). Brain Res. 1383, 99–107. doi: 10.1016/j.brainres.2011.01.093
Konovalov, A., Gadzhiagaev, V., Grebenev, F., Stavtsev, D., Piavchenko, G., Gerasimenko, A., et al. (2023). Laser speckle contrast imaging in neurosurgery: a systematic review. World Neurosurg. 171, 35–40. doi: 10.1016/j.wneu.2022.12.048
Konturek, P. C., Dembinski, A., Warzecha, Z., Burnat, G., Ceranowicz, P., Hahn, E. G., et al. (2005). Pioglitazone, a specific ligand of peroxisome proliferator-activated receptor-gamma, protects pancreas against acute cerulein-induced pancreatitis. World J. Gastroenterol. 11, 6322–6329. doi: 10.3748/wjg.v11.i40.6322
Koren, J. 3rd, Jinwal, U. K., Lee, D. C., Jones, J. R., Shults, C. L., Johnson, A. G., et al. (2009). Chaperone signaling complexes in Alzheimer’s disease. J. Cell. Mol. Med. 13, 619–630. doi: 10.1111/j.1582-4934.2008.00557.x
Korovesi, A. G., Anagnostopoulos, A. K., Pierros, V., Stravopodis, D. J., and Tsangaris, G. T. (2020). Normal mouse brain proteome II: analysis of brain regions by high-resolution mass spectrometry. Cancer Genomics Proteomics 17, 757–767. doi: 10.21873/cgp.20230
Kuta, R., Larochelle, N., Fernandez, M., Pal, A., Minotti, S., Tibshirani, M., et al. (2020). Depending on the stress, histone deacetylase inhibitors act as heat shock protein co-inducers in motor neurons and potentiate arimoclomol, exerting neuroprotection through multiple mechanisms in ALS models. Cell Stress Chaperones 25, 173–191. doi: 10.1007/s12192-019-01064-1
Kwon, H. J., Kim, W., Jung, H. Y., Kang, M. S., Kim, J. W., Hahn, K. R., et al. (2019). Heat shock protein 70 increases cell proliferation, neuroblast differentiation, and the phosphorylation of CREB in the hippocampus. Lab Anim Res. 35:21. doi: 10.1186/s42826-019-0020-2
Lackie, R. E., Maciejewski, A., Ostapchenko, V. G., Marques-Lopes, J., Choy, W. Y., Duennwald, M. L., et al. (2017). The Hsp70/Hsp90 chaperone machinery in neurodegenerative diseases. Front. Neurosci. 11:254. doi: 10.3389/fnins.2017.00254
Lackie, R. E., Razzaq, A. R., Farhan, S. M. K., Qiu, L. R., Moshitzky, G., Beraldo, F. H., et al. (2020). Modulation of hippocampal neuronal resilience during aging by the Hsp70/Hsp90 co-chaperone STI1. J. Neurochem. 153, 727–758. doi: 10.1111/jnc.14882
Lane, C. A., Hardy, J., and Schott, J. M. (2018). Alzheimer’s disease. Eur. J. Neurol. 25, 59–70. doi: 10.1111/ene.13439
Lange, J., Gillham, O., Flower, M., Ging, H., Eaton, S., Kapadia, S., et al. (2023). PolyQ length-dependent metabolic alterations and DNA damage drive human astrocyte dysfunction in Huntington’s disease. Prog. Neurobiol. :102448. doi: 10.1016/j.pneurobio.2023.102448
Lee, S., Jun, Y. W., Linares, G. R., Butler, B., Yuva-Adyemir, Y., Moore, J., et al. (2023). Downregulation of Hsp90 and the antimicrobial peptide Mtk suppresses poly (GR)-induced neurotoxicity in C9ORF72-ALS/FTD. Neuron S0896-6273, 00133–00132. doi: 10.1016/j.neuron.2023.02.029
Lenzi, C., Ramazzina, I., Russo, I., Filippini, A., Bettuzzi, S., and Rizzi, F. (2020). The Down-regulation of Clusterin expression enhances the α-Synuclein aggregation process. Int. J. Mol. Sci. 21:7181. doi: 10.3390/ijms21197181
Li, X., Shao, H., Taylor, I. R., and Gestwicki, J. E. (2016). Targeting allosteric control mechanisms in heat shock protein 70 (Hsp70). Curr. Top. Med. Chem. 16, 2729–2740. doi: 10.2174/1568026616666160413140911
Lizama, B. N., Palubinsky, A. M., Raveendran, V. A., Moore, A. M., Federspiel, J. D., Codreanu, S. G., et al. (2018). Neuronal preconditioning requires the mitophagic activity of c-terminus of hsc70-interacting protein. J. Neurosci. 38, 6825–6840. doi: 10.1523/JNEUROSCI.0699-18.2018
Llewellyn, J., Mallikarjun, V., Appleton, E., Osipova, M., Gilbert, H. T. J., Richardson, S. M., et al. (2023). Loss of regulation of protein synthesis and turnover underpins an attenuated stress response in senescent human mesenchymal stem cells. Proc. Natl. Acad. Sci. U. S. A. 120:e2210745120. doi: 10.1073/pnas.2210745120
Lo Cascio, F., and Kayed, R. (2018). Azure C targets and modulates toxic tau oligomers. ACS Chem. Neurosci. 9, 1317–1326. doi: 10.1021/acschemneuro.7b00501
Maheshwari, M., Bhutani, S., Das, A., Mukherjee, R., Sharma, A., Kino, Y., et al. (2014). Dexamethasone induces heat shock response and slows down disease progression in mouse and fly models of Huntington’s disease. Hum. Mol. Genet. 23, 2737–2751. doi: 10.1093/hmg/ddt667
Mandrioli, J., Crippa, V., Cereda, C., Bonetto, V., Zucchi, E., Gessani, A., et al. (2019). Proteostasis and ALS: protocol for a phase II, randomized, double-blind, placebo-controlled, multicentre clinical trial for colchicine in ALS (co-ALS). BMJ Open 9:e028486. doi: 10.1136/bmjopen-2018-028486
Martin, M. D., Baker, J. D., Suntharalingam, A., Nordhues, B. A., Shelton, L. B., Zheng, D., et al. (2016). Inhibition of both Hsp70 activity and tau aggregation in vitro best predicts tau lowering activity of small molecules. ACS Chem. Biol. 11, 2041–2048. doi: 10.1021/acschembio.6b00223
Martínez de Toda, I., and De la Fuente, M. (2015). The role of Hsp70 in oxi-inflamm-aging and its use as a potential biomarker of lifespan. Biogerontology 16, 709–721. doi: 10.1007/s10522-015-9607-7
Martín-Peña, A., Rincón-Limas, D. E., and Fernandez-Fúnez, P. (2018). Engineered Hsp70 chaperones prevent Aβ42-induced memory impairments in a Drosophila model of Alzheimer’s disease. Sci. Rep. 8:9915. doi: 10.1038/s41598-018-28341-w
Matsathit, U., Mekseepralard, C., Wongsawatkul, O., Ratanachamnong, P., Upapan, P., and Phivthong-Ngam, L. (2016). Thermal steam Aerosolization protects against lipopolysaccharide-induced Sepsis in rats. J. Med. Assoc. Thail. 99, S206–S215.
Mauger, O., and Scheiffele, P. (2017). Beyond proteome diversity: alternative splicing as a regulator of neuronal transcript dynamics. Curr. Opin. Neurobiol. 45, 162–168. doi: 10.1016/j.conb.2017.05.012
McKinnon, C., De Snoo, M. L., Gondard, E., Neudorfer, C., Chau, H., Ngana, S. G., et al. (2020). Early-onset impairment of the ubiquitin-proteasome system in dopaminergic neurons caused by α-synuclein. Acta Neuropathol. Commun. 8:17. doi: 10.1186/s40478-020-0894-0
Menéndez-González, M. (2023). Toward a new nosology of neurodegenerative diseases. Alzheimers Dement. 19, 3731–3737. doi: 10.1002/alz.13041
Mengel, E., Patterson, M. C., Da Riol, R. M., Del Toro, M., Deodato, F., Gautschi, M., et al. (2021). Efficacy and safety of arimoclomol in Niemann-pick disease type C: results from a double-blind, randomized, placebo-controlled, multinational phase 2/3 trial of a novel treatment. J. Inherit. Metab. Dis. 44, 1463–1480. doi: 10.1002/jimd.12428
Miller, R. G., Mitchell, J. D., and Moore, D. H. (2012). Riluzole for amyotrophic lateral sclerosis (ALS)/motor neuron disease (MND). Cochrane Database Syst. Rev. 2012:CD001447. doi: 10.1002/14651858.CD001447.pub3
Miyata, Y., Li, X., Lee, H. F., Jinwal, U. K., Srinivasan, S. R., Seguin, S. P., et al. (2013). Synthesis and initial evaluation of YM-08, a blood-brain barrier permeable derivative of the heat shock protein 70 (Hsp70) inhibitor MKT-077, which reduces tau levels. ACS Chem. Neurosci. 4, 930–939. doi: 10.1021/cn300210g
Mizukoshi, E., Suzuki, M., Loupatov, A., Uruno, T., Hayashi, H., Misono, T., et al. (1999). Fibroblast growth factor-1 interacts with the glucose-regulated protein GRP75/mortalin. Biochem. J. 343, 461–466.
Moors, T. E., Hoozemans, J. J., Ingrassia, A., Beccari, T., Parnetti, L., Chartier-Harlin, M. C., et al. (2017). Therapeutic potential of autophagy-enhancing agents in Parkinson’s disease. Mol. Neurodegener. 12:11. doi: 10.1186/s13024-017-0154-3
Moyano, P., Sanjuna, J., Garcia, J. M., Garcia, J., Frejo, M. T., Naval, M. V., et al. (2021). Paraquat treatment compromises the clearance of β-amyloid and tau proteins and induces primary hippocampal neuronal cell death through HSP70, P20S, and TFEB disruption. Chem. Res. Toxicol. 34, 1240–1244. doi: 10.1021/acs.chemrestox.0c00370
Negi, S. K., and Guda, C. (2017). Global gene expression profiling of healthy human brain and its application in studying neurological disorders. Sci. Rep. 7:897. doi: 10.1038/s41598-017-00952-9
Nishida, T., Yabe, Y., Fu, H. Y., Hayashi, Y., Asahi, K., Eguchi, H., et al. (2007). Geranylgeranylacetone induces cyclooxygenase-2 expression in cultured rat gastric epithelial cells through NF-kappaB. Dig. Dis. Sci. 52, 1890–1896. doi: 10.1007/s10620-006-9661-8
Osorio, C., Sullivan, P. M., He, D. N., Mace, B. E., Ervin, J. F., Strittmatter, W. J., et al. (2007). Mortalin is regulated by APOE in hippocampus of AD patients and by human APOE in TR mice. Neurobiol. Aging 28, 1853–1862. doi: 10.1016/j.neurobiolaging.2006.08.011
Perrin, R. J., Fagan, A. M., and Holtzman, D. M. (2009). Multimodal techniques for diagnosis and prognosis of Alzheimer’s disease. Nature 461, 916–922. doi: 10.1038/nature08538
Petri, S., Grehl, T., Grosskreutz, J., Hecht, M., Hermann, A., Jesse, S., et al. (2023). Guideline “motor neuron diseases” of the German Society of Neurology (deutsche Gesellschaft für Neurologie). Neurol Res Pract. 5:25. doi: 10.1186/s42466-023-00251-x
Phukan, J. (2010). Arimoclomol, a coinducer of heat shock proteins for the potential treatment of amyotrophic lateral sclerosis. IDrugs 13, 482–496.
Piavchenko, G., Kozlov, I., Dremin, V., Stavtsev, D., Seryogina, E., Kandurova, K., et al. (2021). Impairments of cerebral blood flow microcirculation in rats brought on by cardiac cessation and respiratory arrest. J. Biophotonics 14:e202100216. doi: 10.1002/jbio.202100216
Poewe, W., Seppi, K., Tanner, C. M., Halliday, G. M., Brundin, P., Volkmann, J., et al. (2017). Parkinson disease. Nat Rev Dis Primers. 3:17013. doi: 10.1038/nrdp.2017.13
Pratt, W. B., Gestwicki, J. E., Osawa, Y., and Lieberman, A. P. (2015). Targeting Hsp90/Hsp70-based protein quality control for treatment of adult-onset neurodegenerative diseases. Annu. Rev. Pharmacol. Toxicol. 55, 353–371. doi: 10.1146/annurev-pharmtox-010814-124332
Radons, J. (2016). The human HSP70 family of chaperones: where do we stand? Cell Stress Chaperones 21, 379–404. doi: 10.1007/s12192-016-0676-6
Rahimpour, P., Nasehi, M., Zarrindast, M. R., and Khalifeh, S. (2023). Dose-dependent manner of luteolin in the modulation of spatial memory with respect to the hippocampal level of HSP70 and HSP90 in sleep-deprived rats. Gene 852:147046. doi: 10.1016/j.gene.2022.147046
Rai, R., Kennedy, A. L., Isingizwe, Z. R., Javadian, P., and Benbrook, D. M. (2021). Similarities and differences of Hsp70, hsc70, Grp78 and Mortalin as Cancer biomarkers and drug targets. Cells 10:2996. doi: 10.3390/cells10112996
Rai, S., and Tapadia, M. G. (2022). Hsc70-4 aggravates PolyQ-mediated neurodegeneration by modulating NF-κB mediated immune response in Drosophila. Front. Mol. Neurosci. 15:857257. doi: 10.3389/fnmol.2022.857257
Rajesh, Y., and Kanneganti, T. D. (2022). Innate immune cell death in Neuroinflammation and Alzheimer’s disease. Cells 11:1885. doi: 10.3390/cells11121885
Reis, S. D., Pinho, B. R., and Oliveira, J. M. A. (2017). Modulation of molecular chaperones in Huntington’s disease and other Polyglutamine disorders. Mol. Neurobiol. 54, 5829–5854. doi: 10.1007/s12035-016-0120-z
Renaud, L., Picher-Martel, V., Codron, P., and Julien, J. P. (2019). Key role of UBQLN2 in pathogenesis of amyotrophic lateral sclerosis and frontotemporal dementia. Acta Neuropathol. Commun. 7:103. doi: 10.1186/s40478-019-0758-7
Repalli, J., and Meruelo, D. (2015). Screening strategies to identify HSP70 modulators to treat Alzheimer’s disease. Drug Des. Devel. Ther. 9, 321–331. doi: 10.2147/DDDT.S72165
Ripin, N., and Parker, R. (2022). Are stress granules the RNA analogs of misfolded protein aggregates? RNA 28, 67–75. doi: 10.1261/rna.079000.121
Rivera, I., Capone, R., Cauvi, D. M., Arispe, N., and De Maio, A. (2018). Modulation of Alzheimer’s amyloid β peptide oligomerization and toxicity by extracellular Hsp70. Cell Stress Chaperones 23, 269–279. doi: 10.1007/s12192-017-0839-0
Rizk, F. H., Soliman, N. A., Heabah, N. A., Abdel Ghafar, M. T., El-Attar, S. H., and Elsaadany, A. (2022). Fenofibrate improves cognitive impairment induced by high-fat high-fructose diet: a possible role of Irisin and heat shock proteins. ACS Chem. Neurosci. 13, 1782–1789. doi: 10.1021/acschemneuro.2c00186
Romi, F., Helgeland, G., and Gilhus, N. E. (2011). Heat-shock proteins in clinical neurology. Eur. Neurol. 66, 65–69. doi: 10.1159/000329373
Russo, C., Schettini, G., Saido, T. C., Hulette, C., Lippa, C., Lannfelt, L., et al. (2000). Presenilin-1 mutations in Alzheimer’s disease. Nature 405, 531–532. doi: 10.1038/35014735
Rutledge, B. S., Choy, W. Y., and Duennwald, M. L. (2022). Folding or holding? - Hsp70 and Hsp90 chaperoning of misfolded proteins in neurodegenerative disease. J. Biol. Chem. 298:101905. doi: 10.1016/j.jbc.2022.101905
Sabirzhanov, B., Stoica, B. A., Hanscom, M., Piao, C. S., and Faden, A. I. (2012). Over-expression of HSP70 attenuates caspase-dependent and caspase-independent pathways and inhibits neuronal apoptosis. J. Neurochem. 123, 542–554. doi: 10.1111/j.1471-4159.2012.07927.x
Saha, I., Yuste-Checa, P., Da Silva, P. M., Guo, Q., Körner, R., Holthusen, H., et al. (2023). The AAA+ chaperone VCP disaggregates tau fibrils and generates aggregate seeds in a cellular system. Nat. Commun. 14:560. doi: 10.1038/s41467-023-36058-2
Seelaar, H., Schelhaas, H. J., Azmani, A., Küsters, B., Rosso, S., Majoor-Krakauer, D., et al. (2007). TDP-43 pathology in familial frontotemporal dementia and motor neuron disease without Progranulin mutations. Brain 130, 1375–1385. doi: 10.1093/brain/awm024
Sengking, J., Oka, C., Yawoot, N., Tocharus, J., Chaichompoo, W., Suksamrarn, A., et al. (2022). Protective effect of Neferine in permanent cerebral ischemic rats via anti-oxidative and anti-apoptotic mechanisms. Neurotox. Res. 40, 1348–1359. doi: 10.1007/s12640-022-00568-6
Serrano-Pozo, A., Das, S., and Hyman, B. T. (2021). APOE and Alzheimer’s disease: advances in genetics, pathophysiology, and therapeutic approaches. Lancet Neurol. 20, 68–80. doi: 10.1016/S1474-4422(20)30412-9
Shang, B. B., Chen, J., Wang, Z. G., and Liu, H. (2021). Significant correlation between HSPA4 and prognosis and immune regulation in hepatocellular carcinoma. PeerJ. 9:e12315. doi: 10.7717/peerj.12315
Shao, H., Li, X., Hayashi, S., Bertron, J. L., Schwarz, D. M. C., Tang, B. C., et al. (2021). Inhibitors of heat shock protein 70 (Hsp70) with enhanced metabolic stability reduce tau levels. Bioorg. Med. Chem. Lett. 41:128025. doi: 10.1016/j.bmcl.2021.128025
Shenkman, M., Geva, M., Gershoni-Emek, N., Hayden, M. R., and Lederkremer, G. Z. (2021). Pridopidine reduces mutant huntingtin-induced endoplasmic reticulum stress by modulation of the Sigma-1 receptor. J. Neurochem. 158, 467–481. doi: 10.1111/jnc.15366
Shirafuji, T., Ueyama, T., Adachi, N., Yoshino, K. I., Sotomaru, Y., Uwada, J., et al. (2018). The role of cysteine string protein α phosphorylation at serine 10 and 34 by protein kinase Cγ for presynaptic maintenance. J. Neurosci. 38, 278–290. doi: 10.1523/JNEUROSCI.1649-17.2017
Sinitcyn, P., Richards, A. L., Weatheritt, R. J., Brademan, D. R., Marx, H., Shishkova, E., et al. (2023). Global detection of human variants and isoforms by deep proteome sequencing. Nat. Biotechnol. doi: 10.1038/s41587-023-01714-x
Sipilä, J. O. T., Kytövuori, L., Rauramaa, T., Rauhamaa, H., Kaasinen, V., and Majamaa, K. (2023). A severe neurodegenerative disease with Lewy bodies and a mutation in the glucocerebrosidase gene. NPJ Parkinsons Dis. 9:53. doi: 10.1038/s41531-023-00501-4
Smulders, L., Altman, R., Briseno, C., Saatchi, A., Wallace, L., AlSebaye, M., et al. (2022). Phosphatidylinositol Monophosphates Regulate the Membrane Localization of HSPA1A, a Stress-Inducible 70-kDa Heat Shock Protein. Biomolecules. 12:856. doi: 10.3390/biom12060856
Sojka, D. R., Abramowicz, A., Adamiec-Organiściok, M., Karnas, E., Mielańczyk, Ł., Kania, D., et al. (2023). Heat shock protein A2 is a novel extracellular vesicle-associated protein. Sci. Rep. 13:4734. doi: 10.1038/s41598-023-31962-5
Spillantini, M. G., Schmidt, M. L., Lee, V. M., Trojanowski, J. Q., Jakes, R., and Goedert, M. (1997). Alpha-synuclein in Lewy bodies. Nature 388, 839–840. doi: 10.1038/42166
Subrizi, A., Toropainen, E., Ramsay, E., Airaksinen, A. J., Kaarniranta, K., and Urtti, A. (2015). Oxidative stress protection by exogenous delivery of rhHsp70 chaperone to the retinal pigment epithelium (RPE), a possible therapeutic strategy against RPE degeneration. Pharm. Res. 32, 211–221. doi: 10.1007/s11095-014-1456-6
Sun, Y., Zhang, J. R., and Chen, S. (2017). Suppression of Alzheimer’s disease-related phenotypes by the heat shock protein 70 inducer, geranylgeranylacetone, in APP/PS1 transgenic mice via the ERK/p38 MAPK signaling pathway. Exp. Ther. Med. 14, 5267–5274. doi: 10.3892/etm.2017.5253
Taldone, T., Ochiana, S. O., Patel, P. D., and Chiosis, G. (2014). Selective targeting of the stress chaperome as a therapeutic strategy. Trends Pharmacol. Sci. 35, 592–603. doi: 10.1016/j.tips.2014.09.001
Thakur, P., and Nehru, B. (2014). Long-term heat shock proteins (HSPs) induction by carbenoxolone improves hallmark features of Parkinson’s disease in a rotenone-based model. Neuropharmacology 79, 190–200. doi: 10.1016/j.neuropharm.2013.11.016
Thirstrup, K., Sotty, F., Montezinho, L. C., Badolo, L., Thougaard, A., Kristjánsson, M., et al. (2016). Linking HSP90 target occupancy to HSP70 induction and efficacy in mouse brain. Pharmacol. Res. 104, 197–205. doi: 10.1016/j.phrs.2015.12.028
Thiruvalluvan, A., de Mattos, E. P., Brunsting, J. F., Bakels, R., Serlidaki, D., Barazzuol, L., et al. (2020). DNAJB6, a key factor in neuronal sensitivity to amyloidogenesis. Mol. Cell 78, 346–358.e9. doi: 10.1016/j.molcel.2020.02.022
Thomas, M., Alegre-Abarrategui, J., and Wade-Martins, R. (2013). RNA dysfunction and aggrephagy at the Centre of an amyotrophic lateral sclerosis/frontotemporal dementia disease continuum. Brain 136, 1345–1360. doi: 10.1093/brain/awt030
Tokunbo, O. S., Arogundade, T. T., Abayomi, T. A., Lewu, S. F., Abayomi, O. A., Obembe, O. O., et al. (2023). African walnut (Tetracarpidium conophorum) extract upregulates Glucocerebrosidase activity and circumvents parkinsonian changes in the Hippocampus via the activation of heat-shock proteins. J. Chem. Neuroanat. :102271. doi: 10.1016/j.jchemneu.2023.102271
Turturici, G., Sconzo, G., and Geraci, F. (2011). HSP70 and its molecular role in nervous system diseases. Biochem. Res. Int. 2011:618127. doi: 10.1155/2011/618127
Tytell, M., Greenberg, S. G., and Lasek, R. J. (1986). Heat shock-like protein is transferred from glia to axon. Brain Res. 363, 161–164. doi: 10.1016/0006-8993(86)90671-2
Uematsu, M., Nakamura, A., Ebashi, M., Hirokawa, K., Takahashi, R., and Uchihara, T. (2018). Brainstem tau pathology in Alzheimer’s disease is characterized by increase of three repeat tau and independent of amyloid β. Acta Neuropathol. Commun. 6:1. doi: 10.1186/s40478-017-0501-1
Verma, P., Pfister, J. A., Mallick, S., and D’Mello, S. R. (2014). HSF1 protects neurons through a novel trimerization- and HSP-independent mechanism. J. Neurosci. 34, 1599–1612. doi: 10.1523/JNEUROSCI.3039-13.2014
Wadhwa, R., Yaguchi, T., Hasan, M. K., Mitsui, Y., Reddel, R. R., and Kaul, S. C. (2002). Hsp70 family member, mot-2/mthsp70/GRP75, binds to the cytoplasmic sequestration domain of the p53 protein. Exp Cell Res. 274, 246–53. doi: 10.1006/excr.2002.5468
Wang, J., Wang, B., and Zhou, T. (2022). The advance on frontotemporal dementia (FTD)’s neuropathology and molecular genetics. Mediat. Inflamm. 2022:5003902. doi: 10.1155/2022/5003902
Wang, I. F., Wu, L. S., Chang, H. Y., and Shen, C. K. (2008). TDP-43, the signature protein of FTLD-U, is a neuronal activity-responsive factor. J. Neurochem. 105, 797–806. doi: 10.1111/j.1471-4159.2007.05190.x
Wentink, A. S., Nillegoda, N. B., Feufel, J., Ubartaitė, G., Schneider, C. P., De Los, R. P., et al. (2020). Molecular dissection of amyloid disaggregation by human HSP70. Nature 587, 483–488. doi: 10.1038/s41586-020-2904-6
Wu, J. Z., Ardah, M., Haikal, C., Svanbergsson, A., Diepenbroek, M., Vaikath, N. N., et al. (2019). Dihydromyricetin and Salvianolic acid B inhibit alpha-synuclein aggregation and enhance chaperone-mediated autophagy. Transl Neurodegener. 8:18. doi: 10.1186/s40035-019-0159-7
Yang, J., Bridges, K., Chen, K. Y., and Liu, A. Y. (2008). Riluzole increases the amount of latent HSF1 for an amplified heat shock response and cytoprotection. PLoS One 3:e2864. doi: 10.1371/journal.pone.0002864
Yang, Y., Zhang, S., Guan, J., Jiang, Y., Zhang, J., Luo, L., et al. (2022). SIRT1 attenuates neuroinflammation by deacetylating HSPA4 in a mouse model of Parkinson’s disease. Biochim. Biophys. Acta Mol. basis Dis. 1868:166365. doi: 10.1016/j.bbadis.2022.166365
Yurinskaya, M. M., Mit’kevich, V. A., Evgen’ev, M. B., Makarov, A. A., and Vinokurov, M. G. (2016). Heat-shock protein HSP70 reduces the secretion of TNFα by neuroblastoma cells and human monocytes induced with beta-amyloid peptides. Mol. Biol. (Mosk) 50, 1053–1056. doi: 10.7868/S0026898416060239
Yurinskaya, M., Zatsepina, O. G., Vinokurov, M. G., Bobkova, N. V., Garbuz, D. G., Morozov, A. V., et al. (2015). The fate of exogenous human HSP70 introduced into animal cells by different means. Curr. Drug Deliv. 12, 524–532. doi: 10.2174/1567201812666150724094207
Zatsepina, O. G., Evgen’ev, M. B., and Garbuz, D. G. (2021). Role of a heat shock transcription factor and the major heat shock protein Hsp70 in memory formation and neuroprotection. Cells 10:1638. doi: 10.3390/cells10071638
Zhang, C. W., Adeline, H. B., Chai, B. H., Hong, E. T., Ng, C. H., and Lim, K. L. (2016). Pharmacological or genetic activation of Hsp70 protects against loss of Parkin function. Neurodegener Dis 16, 304–316. doi: 10.1159/000443668
Zhang, M., Chen, C., Peng, Q., Wu, X., Zhou, R., Ma, Y., et al. (2023). A novel gene therapy for methamphetamine- induced cognitive disorder with a hyper-acidified fusion variant of DnaJB1. Mol Ther Nucleic Acids. 31, 703–716. doi: 10.1016/j.omtn.2023.02.017
Zhang, Y., Liu, R., Ni, M., Gill, P., and Lee, A. S. (2010). Cell surface relocalization of the endoplasmic reticulum chaperone and unfolded protein response regulator GRP78/BiP. J. Biol. Chem. 285, 15065–15075. doi: 10.1074/jbc.M109.087445
Zhang, K., Wang, A., Zhong, K., Qi, S., Wei, C., Shu, X., et al. (2021). UBQLN2-HSP70 axis reduces poly-Gly-ala aggregates and alleviates behavioral defects in the C9ORF72 animal model. Neuron 109, 1949–1962.e6. doi: 10.1016/j.neuron.2021.04.023
Keywords: Hsp70, neuropharmacology, Alzheimer’s disease, amyotrophic lateral sclerosis, neurodegenerative diseases
Citation: Venediktov AA, Bushueva OY, Kudryavtseva VA, Kuzmin EA, Moiseeva AV, Baldycheva A, Meglinski I and Piavchenko GA (2023) Closest horizons of Hsp70 engagement to manage neurodegeneration. Front. Mol. Neurosci. 16:1230436. doi: 10.3389/fnmol.2023.1230436
Edited by:
Eva Zerovnik, Institut Jožef Stefan (IJS), SloveniaReviewed by:
Boris A. Margulis, Russian Academy of Sciences (RAS), RussiaXabier Bengoetxea, Achucarro Basque Center for Neuroscience, Spain
Copyright © 2023 Venediktov, Bushueva, Kudryavtseva, Kuzmin, Moiseeva, Baldycheva, Meglinski and Piavchenko. This is an open-access article distributed under the terms of the Creative Commons Attribution License (CC BY). The use, distribution or reproduction in other forums is permitted, provided the original author(s) and the copyright owner(s) are credited and that the original publication in this journal is cited, in accordance with accepted academic practice. No use, distribution or reproduction is permitted which does not comply with these terms.
*Correspondence: Gennadii A. Piavchenko, gennadii.piavchenko@staff.sechenov.ru; Igor Meglinski, i.meglinski@aston.ac.uk; Anna Baldycheva, a.baldycheva@exeter.ac.uk