- 1Glostrup Research Institute, Department of Neurology, Danish Headache Center, Copenhagen University Hospital – Rigshospitalet, Copenhagen, Denmark
- 2Department of Odontology, Panum Institute, Faculty of Health, University of Copenhagen, Copenhagen, Denmark
Migraine is one of the leading causes of disability worldwide, affecting work and social life. It has been estimated that sales of migraine medicines will reach 12.9 billion USD in 2027. To reduce social impact, migraine treatments must improve, and the ATP-sensitive potassium (KATP) channel is a promising target because of the growing evidence of its implications in the pathogenesis of migraine. Strong human data show that opening of the KATP channel using levcromakalim is the most potent headache and migraine trigger ever tested as it induces headache in almost all healthy subjects and migraine attacks in 100% of migraine sufferers. This review will address the basics of the KATP channel together with clinical and preclinical data on migraine implications. We argue that KATP channel blocking, especially the Kir6.1/SUR2B subtype, may be a target for migraine drug development, however translational issues remain. There are no human data on the closure of the KATP channel, although blocking the channel is effective in animal models of migraine. We believe there is a good likelihood that an antagonist of the Kir6.1/SUR2B subtype of the KATP channel will be effective in the treatment of migraine. The side effects of such a blocker may be an issue for clinical use, but the risk is likely only moderate. Future clinical trials of a selective Kir6.1/SUR2B blocker will answer these questions.
1. Introduction
Migraine is by far the most prevalent neurological disease with a lifetime prevalence of 15–20% (Stovner et al., 2018). It is sexually dimorphic with a sex ratio of 2.5 women for each man and greater severity in women. Thus, approximately 80% of the total burden of migraine is on women (Steiner et al., 2020). According to WHO migraine is number two out of all diseases causing disability and the disease has huge socioeconomic costs to society in addition to the personal costs and suffering afflicted (Abbafati et al., 2020). It is obvious that a disease of these dimensions for which no cure exists requires effective drug therapy at each acute attack and as prophylaxis in those most affected. This was illustrated by the huge success of the triptans with a peak sale at several billion USD. They are still the dominant treatment for acute migraine attacks but now mostly off patent and inexpensive. The picture was further cemented by the advent of human monoclonal antibodies against CGRP or its receptor which in a very short time has obtained impressive sales. It has been estimated that total sales of migraine medicines will reach 15.6 billion USD in 2029 (IHealthcareAnalyst I, 2023).
Growing evidence suggests that ATP-sensitive potassium (KATP) channels are implicated in the pathogenesis of migraine as opening of the channel using levcromakalim is the most potent headache and migraine trigger ever tested. It has been suggested that the KATP channel may be the unifying mechanism for signaling pathways of established migraine triggers including calcitonin gene-related peptide (CGRP), pituitary adenylate cyclase-activating peptide-38 (PACAP38) and nitroglycerine (GTN) leading to migraine attacks (Figure 1). We have recently published a comprehensive review on the KATP channels (Clement et al., 2022), whereas this shorter review has a more general perspective on the basics of the KATP channel together with clinical and preclinical data on migraine implications. We argue that KATP channel blocking may be a novel promising target for migraine drug development.
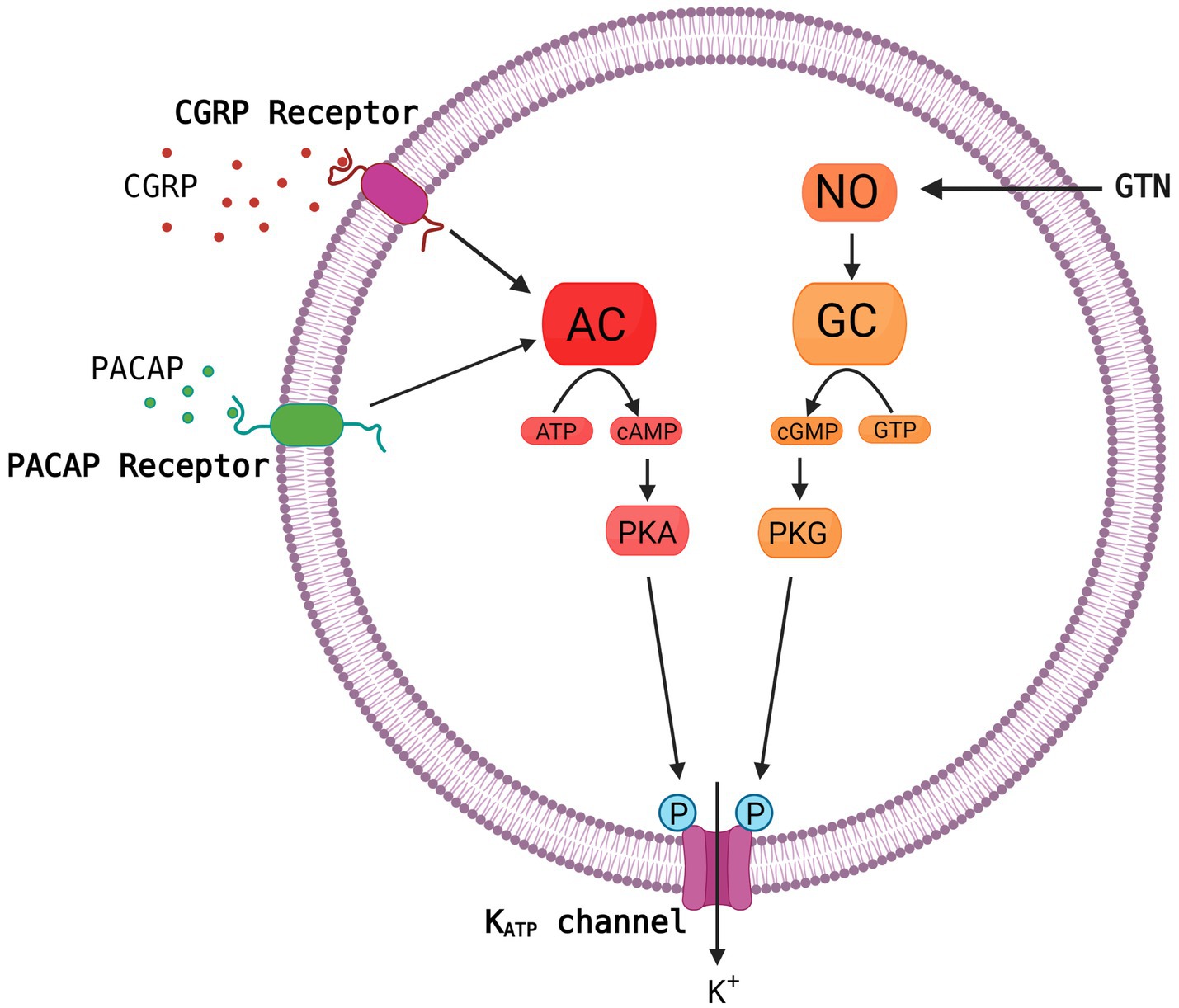
Figure 1. Common molecular pathways resulting in activation of the KATP channel. The migraine triggers CGRP, PACAP and nitroglycerine (GTN) are hypothesized to activate the KATP channel via cyclic adenosine monophosphate (cAMP) or cyclic guanosine monophosphate (cGMP) and downstream pathways. This is a simplified and hypothetical model describing the potential signaling pathways of migraine triggers and is based mainly on human experimental provocation studies (Ashina et al., 2013). More complex interactive processes and yet unknown mechanisms between signaling molecules are probably involved. AC, adenylate cyclase; NO, nitric oxide; PKA, protein kinase A; GC, guanylate cyclase; GTP, guanosine triphosphate; PKG, protein kinase G. Created with BioRender.com.
2. How are migraine patients served by current drugs?
The so-called triptan wave rolled 20 years ago, and it was widely believed that the triptans had solved the migraine problem (Loder, 2010). Huge sales efforts by the pharmaceutical industry and enthusiastic doctors helped to promote this picture. Later, it turned out that the picture was not that rosy. The efficacy of triptans was originally based on rather soft success criteria, and when much stricter and more relevant criteria were applied the triptan response was much less satisfactory (Tfelt-Hansen and Olesen, 2012). A recent study showed that only 1/7 of all Danes with migraine currently uses a triptan (Davidsson et al., 2021). Whether this is due to lack of long-term efficacy or side effects is unclear, but it is not due to cost since medical service including prescriptions is free in Denmark. Thus, the treatment of migraine attacks is far from ideal. Better drugs or drugs working by a different mechanism are needed. The latter was fulfilled by the advent of small molecule CGRP receptor antagonists (Lipton and Dodick, 2004). They are less effective than the triptans but work via a different mechanism (Tfelt-Hansen and Loder, 2019). In prophylaxis the human antibodies against CGRP or its receptor and small molecule CGRP receptor antagonists are likewise not more effective than existing drugs but have fewer side effects than former prophylactic drugs (Charles and Pozo-Rosich, 2019; Tfelt-Hansen and Loder, 2019). Importantly, they work by a very specific mechanism and therefore have few side effects. They are also effective in non-responders to existing drugs (Ferrari et al., 2019). In conclusion, CGRP based therapies are valuable new drugs. However, these drugs are effective only in 50–60% of patients (Dodick et al., 2014a,b; Bigal et al., 2015; Sun et al., 2016) which illustrates the importance of developing more therapies with novel mechanisms of action.
3. Migraine heterogeneity suggests a need for precision medicine
Novel drugs such as the CGRP antagonists have been developed against unspecified migraine. In the international classification of headache disorder both the first, second and third edition (ICHD-3) subdivides migraine. The primary division is into migraine without aura and migraine with aura, but no study in recent years has focused specifically on migraine with aura which is less prevalent than migraine without aura and rarely has a high attack frequency indicating prophylactic treatment (Hauge et al., 2009). More understandably, no studies have focused on the rare sub-forms: migraine with brainstem aura, retinal migraine and familial hemiplegic migraine (Russell and Ducros, 2011). Finally, chronic migraine is the most severe form of migraine, but it represents a conglomerate of migraine without aura, migraine with aura and tension-type headache. It seems unlikely therefore, that a drug would ever be specific for chronic migraine. It has been suggested to subdivide migraine without aura and migraine with typical aura according to autonomic symptoms (Christensen et al., 2022), osmophobia (Chalmer et al., 2019), menstrual relation (Vetvik and MacGregor, 2021), genetics (Kogelman et al., 2019) and ability to sensitize during attacks (Bernstein and Burstein, 2012). But so far, there has been little correlation between these features and drug response. The huge prevalence of migraine and the known clinical and genetic heterogeneity suggest, however, that migraine in future will be best served by a multitude of drugs with a multitude of different mechanisms of action. Antagonists of the KATP channel, if effective, would be a needed addition to our current therapeutic armamentarium.
4. KATP channel building blocks and channel sub-types
The KATP channels belong to one of seven families of inwardly rectifying potassium channels (Kir), namely the Kir6 (Hibino et al., 2010). Kir is the building block of the ion pore which consists of an aggregation of four units (Figure 2). The Kir6 subfamily is divided into Kir6.1 and Kir6.2, and around the ion pore is the modifying unit sulfonylurea receptor called SUR, which exists in three sub-forms, SUR1, SUR2A and SUR2B (Babenko et al., 1998). Thus, many configurations of the KATP channel can exist depending on the combination of inner and outer building blocks. Drugs can either target the SUR unit or the pore itself. The distribution of the different types of KATP channels in different tissues is shown in Table 1.
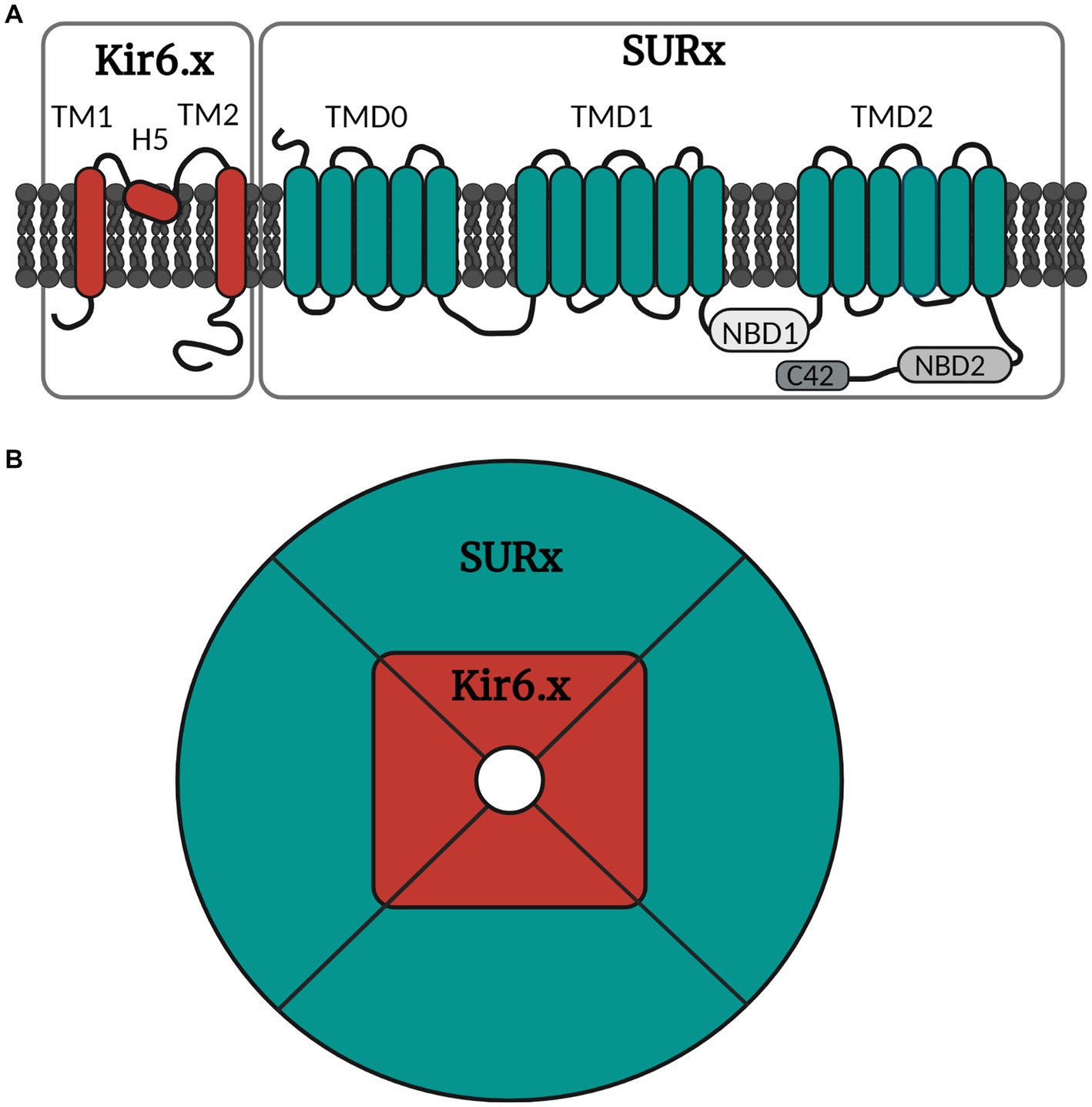
Figure 2. Simple illustration of the molecular and octameric KATP channel structure. (A) The KATP channel is arranged into subunits of Kir6.x and SURx. Kir6.x is composed of two transmembrane regions (TM1 and TM2) linked by the pore forming region H5. SURx is divided into three domains, where TMD0 consists of five transmembrane regions, while TMD1 and TMD2 consist of six transmembrane regions. SURx also holds the nucleotide binding domains (NBD1 and NBD2) and lastly the C42 C-terminal. (B) The channel is organized in four inner Kir6.x subunits and four outer SURx units. The Kir6.x subunits form the ion pore, while the SURx subunits form the outer structure of the channel, and these are necessary for channel activity. Created with BioRender.com.
5. KATP channel agonists in other diseases cause headache
Several KATP channel agonists have been developed for clinical practice including levcromakalim, nicorandil, tifenazoxide, pinacidil, minoxidil, diazoxide and with indications for asthma, ischemic heart disease, hypertension, hair loss and hypoglycaemia. Except for nicorandil, none of these drugs is widely used in clinical practice but headache is reported as a common side effect for all these KATP agonists probably due to their vasodilating effects of cranial arteries (Table 2; Jahangir and Terzic, 2005). Notably, the most frequent side effect reported for levcromakalim, nicorandil and pinacidil treatment was headache (Kidney et al., 1993) - mostly as mild to moderate in intensity that generally occurred early in treatment. Headache was also often responsible for patients discontinuing their treatment (Simpson and Wellington, 2004). Altogether, these findings sparked curiosity in investigating the role of KATP channels in basic science and human experimental models of migraine.
6. Cephalic localization and function of KATP channels (in vitro)
6.1. Cranio-vascular
In intracranial arteries from rat, pig and human Kir6.1 and SUR2B mRNA and protein are predominantly expressed. Only low levels of Kir6.2 and SUR1 and no SUR2A are detected (Ploug et al., 2006). The subdivided location of KATP channels in cephalic arterial smooth muscle cells and endothelial cells have not yet been examined. However, KATP channels in endothelial and smooth muscle cells of peripheral arteries are of the Kir6.1/SUR2B subtype (Aziz et al., 2017; Li et al., 2020). Activation of KATP channels leads to hyperpolarization of smooth muscle cells (Brayden, 2002) that prevents the opening of depolarization activated Ca2+ channels. This causes a decrease in Ca2+ entry to the cell leading to vasodilatation (Quast, 1996). In endothelial cells activation of KATP channels elevate intracellular calcium by inducing hyperpolarization and augmenting the driving force for potential dependent Ca2+ influx. In this way, the KATP channel agonist may promote Ca2+-dependent formation of endothelium-derived relaxing factors such as nitric oxide (Lückhoff and Busse, 1990).
Pharmacological ex vivo and in vivo studies show vasodilatory effects of KATP channel openers in rat and pig intracranial arteries. These responses are inhibited by high doses of the non-selective KATP channel blocker glibenclamide and lower doses of the Kir6.1 blocker PNU-37883A supporting involvement of Kir6.1/SUR2B KATP channels in cranio-vascular responses (Gozalov et al., 2005; Ploug et al., 2006, 2008). Removal of rat basilar artery endothelium slightly but significantly, inhibited KATP channel opener induced vasodilation, suggesting a partial involvement of endothelial KATP channels (Jansen-Olesen et al., 2005). In vivo studies have shown CGRP to activate KATP channels and thereby participate in CGRP mediated dilation of rat meningeal arteries (Gozalov et al., 2008). Interestingly, glibenclamide attenuated this CGRP-induced vasodilation in vivo but was without effect ex vivo (Gozalov et al., 2008).
6.2. Neuronal
In the nervous system, KATP channels are highly expressed in neurons, where they cause hyperpolarization resulting in reduced excitability, potassium efflux (Babenko et al., 1998; Seino and Miki, 2003; Hibino et al., 2010) and consequently reduced neurotransmitter release (Soundarapandian et al., 2007; Yildirim et al., 2021; Clement et al., 2022). Furthermore, KATP channels of the nervous system are suggested to mediate neuroprotection in different scenarios like hypoxia and oxidative stress (Zoga et al., 2010; Country and Jonz, 2021; Clement et al., 2022). Especially the mitochondrial KATP channels have been highlighted in neuroprotection during excitatory toxicity stimulation (Soundarapandian et al., 2007), as activation of mitochondrial KATP channels cause depolarization and reduces neuronal death probably by decreasing influx of Ca2+ which lowers mitochondrial Ca2+ levels (Soundarapandian et al., 2007). This process hinders loss of oxidative phosphorylation and ATP depletion (Country and Jonz, 2021; Clement et al., 2022), but overall the mechanisms underlying the mitochondrial KATP channel-related neuroprotective effects remain unclear.
KATP channels have been found on nerve terminals of rat hippocampal slices, likely on both noradrenergic and glutamatergic nerve endings (Freiman et al., 2001). Likewise, KATP channels are found on nerve terminals prepared from rat motor cortex (Lee et al., 1995). KATP channels (Kir6.2/SUR1 and Kir6.2/SUR2) were also identified on neurons, glial satellite cells and Schwann cells of dorsal root ganglion isolated from rat (Zoga et al., 2010). In this study, KATP channel expression was reduced after painful axotomy, suggesting an involvement in pain perception and a potential target for therapeutic intervention.
Lastly, both Kir6.1 and Kir6.2 expression (using RT-PCR studies) are found in pure glial cultures of rat midbrain (Toulorge et al., 2010). Interestingly, expression of KATP channels was not found on the migraine relevant trigeminal ganglia isolated from mouse, using both RT-PCR and Western blotting, but high expression on dura mater and brain arteries (Christensen et al., 2022). This pattern is supported by RNAseq data from human and mouse trigeminal ganglion (Yang et al., 2022).
7. Human KATP agonist and antagonist studies
Both levcromakalim (KATP channel agonist) and glibenclamide (KATP channel blocker) have been tested in human experimental studies of headache.
Intravenous infusion of levcromakalim induced migraine attacks in 100% of migraine patients without aura (n = 16) making it the most potent migraine-triggering compound to date ever tested (Al-Karagholi et al., 2019). The provoked headache developed very quickly with a median time to onset of 20 min after infusion, and for that reason, no prodrome symptoms were registered. Interestingly, when levcromakalim was given to migraine patients with visual aura (n = 17), 59% developed migraine attacks with visual aura (Al-Karagholi et al., 2021). These findings suggest that levcromakalim is a migraine aura-inducing compound and that the KATP channel may play a role in both migraine aura and migraine headache. It has been argued that levcromakalim most likely induces visual aura and migraine headache through distinct mechanisms, because it very potently triggers migraine without aura, and no aura symptoms, in migraine patients who have never experienced aura. Also, levcromakalim was also able to induce migraine without aura in some patients who previously have only experienced visual aura symptoms not accompanied by headache or migraine (Al-Karagholi et al., 2021). A possible underlying mechanism may be that levcromakalim increases extracellular potassium concentrations in neurons, glial cells, and brain vasculature, which depolarizes neighboring cells thereby triggering a wave of cortical spreading depression (CSD) underlying the visual aura. Nevertheless, it is unclear whether levcromakalim can cross the blood–brain barrier (BBB) but based on its small molecular weight (286.33 Da) and probable lipophilic properties, a central effect cannot be ruled out.
In healthy volunteers (n = 14), levcromakalim induced headache in 86% of participants and dilated the middle meningeal arteries (MMA). The dilatation was reversed by sumatriptan injection (Al-Karagholi et al., 2019). Notably, 14% of the healthy participants reported migraine-like attacks after levcromakalim. Also, the headache-associated dilatation of MMA is noteworthy, as the MMA is the only cranial artery with dilation on the pain side during the early phase of experimentally induced migraine attacks (Khan et al., 2019). However, intradermal and intramuscular levcromakalim injections did not produce more pain than placebo (Al-Karagholi et al., 2019) indicating that levcromakalim-induced headache pain is unlikely to be the result of direct activation of peripheral nociceptors.
Glibenclamide is a non-specific KATP channel blocker widely used for the treatment of diabetes mellitus type 2 to increase insulin secretion. It belongs to the second generation of sulfonylureas (Gribble and Reimann, 2003; Mannhold, 2004). It is yet unknown whether glibenclamide can prevent migraine attacks in adults with migraine. Nevertheless, in healthy volunteers, pre-treatment with glibenclamide did not prevent levcromakalim-induced headache (Al-Karagholi et al., 2020) and did not attenuate levcromakalim-induced vascular changes, e.g., mean global CBF, intracranial artery circumferences, mean arterial blood pressure and heart rate (Al-Karagholi et al., 2021). However, glibenclamide seemingly delayed the onset of levcromakalim-induced headache with median time to headache onset 180 min (Al-Karagholi et al., 2020) versus 20 min (Al-Karagholi et al., 2019). As previously mentioned, glibenclamide is a non-selective KATP channel blocker with a higher affinity for the SUR1 subunit compared to the SUR2 subunits. The KATP channel opener levcromakalim which is one of the most effective drugs to induce headache in humans, has a high affinity to the SUR2B subtype. The high expression of SUR1 in pancreas mediate an increase in insulin secretion which limits the maximum tolerated dose of glibenclamide in humans due to severe risk of hypoglycaemia. Although the blood glucose levels were stabilised by infusion of glucose the dose of glibenclamide could still be too low to inhibit the SUR2B subtype of the KATP channel upon which levcromakalim acts. Glibenclamide did not prevent CGRP- and PACAP-induced headache and hemodynamic changes in healthy volunteers (Coskun et al., 2021; Kokoti et al., 2022). Collectively, these findings imply that glibenclamide does not inhibit the headache-inducing effects of KATP channel activation, probably because glibenclamide is non-specific and primarily inhibits the SUR1 subunit of the KATP channel. Thus, more selective KATP channel blockers selective for the Kir6.1/SUR2B subtype are needed to further examine the therapeutic potential of KATP channel inhibitors in migraine.
8. Preclinical KATP channel agonist and antagonist studies
A range of migraine triggering substances identified in studies of human experimental migraine have also been studied in rodent models where they induce a state of hypersensitivity to various sensory stimuli (Bates et al., 2010; Pradhan et al., 2014; Rea et al., 2018; Demartini et al., 2019; Christensen et al., 2021; Kuburas et al., 2021; Ernstsen et al., 2022). Repeated systemic administration of levcromakalim induced hypersensitivity to tactile stimulation with von Frey filaments (Christensen et al., 2020, 2021; Wu et al., 2022), heat (Christensen et al., 2022), and increased c-Fos expression in the spinal trigeminal nucleus (Wu et al., 2022). In contrast, local intraplantar and intracerebroventricular administration did not lower sensory threshold (Wu et al., 2011; Christensen et al., 2022).
Tactile hypersensitivity induced by migraine triggers GTN, cilostazol, levcromakalim and PACAP38 was fully or partially prevented by pre-administration of glibenclamide (Christensen et al., 2020, 2021; Ernstsen et al., 2022). In a distinct rat model of migraine presenting with spontaneous (inheritable) hypersensitivity in cephalic dermatomes (Oshinsky et al., 2012; Munro et al., 2018), both glibenclamide and gliquidone treatment normalized cephalic sensitivity thresholds, but did not increase the otherwise normal hind-paw threshold (Christensen et al., 2020). These findings initiated further investigation of KATP channel subtype specificity. Significant contribution of the vascular Kir6.1/SUR2B channel subtype to migraine pain generation was indicated as mice lacking Kir6.1 specifically in smooth muscle cells did not sensitize to the same degree as controls following repeated dosing of neither GTN nor levcromakalim (Christensen et al., 2022). Although, vasodilation seems to play a role in the levcromakalim-induced hypersensitivity, it alone may not explain it. Other unclear non-vascular mechanisms are likely to be at play as well. Because glibenclamide in high doses only partially blocked the hypersensitivity induced by PACAP38, whereas the relatively low dose of glibenclamide inhibited levcromakalim-induced hypersensitivity in rodents without affecting the vasodilation produced by levcromakalim in vivo and ex-vivo (Christensen et al., 2020, 2021; Ernstsen et al., 2022). Also, the levcromakalim-induced hypersensitivity can be abolished by CGRP-antagonizing drugs, suggesting that levcromakalim causes CGRP release via an unknown mechanism. The CGRP release may also not be secondary to vasodilation produced by KATP activation because the vasodilation is not inhibited by glibenclamide at concentrations that inhibit the hypersensitivity. In ex vivo organ preparations of dura mater and trigeminal ganglion glibenclamide inhibited capsaicin induced CGRP release (Christensen et al., 2020) which also supports the fact that KATP channel blockers may have a positive effect on migraine via non-vascular mechanisms. Opening of KATP channels does not directly cause CGRP release in the isolated tissue (Ploug et al., 2012; Christensen et al., 2021).
9. Likelihood of effectiveness of KATP antagonists
There are many examples of therapeutic efficacy of antagonists of migraine provocation (Ashina et al., 2017). Angiography provoked attacks of migraine with aura and studies of regional cerebral blood flow demonstrated that cortical spreading depression is the likely mechanism of the aura (Olesen et al., 1981, 1990). A blocker of cortical spreading depression, tonabersat, was effective in the prophylaxis of migraine with aura (Hauge et al., 2009). GTN, via liberation of nitric oxide, was effective in provoking migraine without aura attacks and the non-selective blocker of nitric oxide synthases L-NMMA was effective in treating acute attacks of migraine without aura (Lassen et al., 1997). Finally, and most convincingly, CGRP was effective in inducing migraine attacks without aura in patients (Lassen et al., 2002) and this was crucial for developing brain non-penetrant small molecule CGRP receptor antagonists as well as non-penetrant human monoclonal antibodies against CGRP or its receptor. Antagonism to a migraine provoking substance is therefore often predictive of clinical efficacy but not always. Histamine is equally effective to nitroglycerin in inducing migraine attacks (Lassen et al., 1995), but antihistamines have been on the market for more the 50 years and have received several trials in migraine without convincing results (Lassen et al., 1996; Vollesen et al., 2019). It makes sense that migraine can be experimentally induced by mechanisms that also occur during spontaneous attacks but, likewise, it can be induced by mechanisms that are not part of the mechanisms of spontaneous migraine attacks. Therefore, it cannot be concluded that the strong migraine-provoking effect of levcromakalim in adults with migraine proofs that an antagonist of the KATP channel will effectively prevent or resolve migraine attacks. However, we believe it to be likely. But there are other caveats. KATP channels are abundant in the heart and knockout of specific KATP subfamilies in rodent models have been lethal in mice (Miki et al., 2002). To date, there are no studies of a selective Kir6.1/SUR2B knockout model, which is less abundant in the heart. If opening of the channel is important for migraine attack development, then the opening would primarily be in KATP channel subtypes localized in the cephalic vasculature or other cephalic structures and therefore a selective blocker would selectively affect these structures and not the heart.
10. Conclusion
KATP channels are acknowledged as viable therapeutic targets for migraine treatment as human data show that opening of the KATP channel induces headache in healthy subjects and migraine in migraine sufferers. Still, drug discovery remains a formidable obstacle. Currently, only data from migraine rodent models has illustrated a migraine-relevant effect of blocking the KATP channel activity. To move forward, additional research must be conducted on the specific subtypes of the KATP channel to gain a deeper comprehension of their structures, functions, and distribution for the development of more selective and effective drugs. We believe there is a likelihood that an antagonist of the Kir6.1/SUR2B sub-type will be effective in migraine. Only development and clinical testing of a future selective KATP channel blocker with attention on side effects can answer these questions.
Author contributions
All authors listed have made a substantial, direct, and intellectual contribution to the work, and approved it for publication.
Funding
SG is supported by the BRIDGE – Translational Excellence Programme (bridge.ku.dk) at the Faculty of Health and Medical Sciences, University of Copenhagen, funded by the Novo Nordisk Foundation. Grant agreement no. NNF20SA0064340 (2021 fellows). Also, this work was supported by Candys Foundation.
Conflict of interest
JO owns shares in the start-up company Cephagenix.
The remaining authors declare that the research was conducted in the absence of any commercial or financial relationships that could be construed as a potential conflict of interest.
Publisher’s note
All claims expressed in this article are solely those of the authors and do not necessarily represent those of their affiliated organizations, or those of the publisher, the editors and the reviewers. Any product that may be evaluated in this article, or claim that may be made by its manufacturer, is not guaranteed or endorsed by the publisher.
References
Abbafati, C., Abbas, K. M., Abbasi-Kangevari, M., Abd-Allah, F., Abdelalim, A., Abdollahi, M., et al. (2020). Global burden of 369 diseases and injuries in 204 countries and territories, 1990-2019: a systematic analysis for the global burden of disease study 2019. Lancet 396, 1204–1222. doi: 10.1016/S0140-6736(20)30925-9
al-Karagholi, M. A. M., Ghanizada, H., Hansen, J. M., Aghazadeh, S., Skovgaard, L. T., Olesen, J., et al. (2019). Extracranial activation of ATP-sensitive potassium channels induces vasodilation without nociceptive effects. Cephalalgia 39, 1789–1797. doi: 10.1177/0333102419888490
al-Karagholi, M. A. M., Ghanizada, H., Hansen, J. M., Skovgaard, L. T., Olesen, J., Larsson, H. B. W., et al. (2019). Levcromakalim, an adenosine triphosphate-sensitive Potassium Channel opener, dilates Extracerebral but not cerebral arteries. Headache 59, 1468–1480. doi: 10.1111/head.13634
Al-Karagholi, M. A. M., Ghanizada, H., Kokoti, L., Paulsen, J. S., Hansen, J. M., and Ashina, M. (2020). Effect of KATP channel blocker glibenclamide on levcromakalim-induced headache. Cephalalgia 40, 1045–1054. doi: 10.1177/0333102420949863
al-Karagholi, M. A. M., Ghanizada, H., Nielsen, C. A. W., Ansari, A., Gram, C., Younis, S., et al. (2021). Cerebrovascular effects of glibenclamide investigated using high-resolution magnetic resonance imaging in healthy volunteers. J. Cereb. Blood Flow Metab. 41, 1328–1337. doi: 10.1177/0271678X20959294
Al-Karagholi, M. A. M., Ghanizada, H., Nielsen, C. A. W., Hougaard, A., and Ashina, M. (2021). Opening of ATP sensitive potassium channels causes migraine attacks with aura. Brain 144, 2322–2332. doi: 10.1093/brain/awab136
Al-Karagholi, M. A. M., Hansen, J. M., Guo, S., Olesen, J., and Ashina, M. (2019). Opening of ATP-sensitive potassium channels causes migraine attacks: a new target for the treatment of migraine. Brain 142, 2644–2654. doi: 10.1093/brain/awz199
Ashina, M., Hansen, J. M., á Dunga, B. O., and Olesen, J. (2017). Human models of migraine-short-term pain for long-term gain. Nat. Clin. Pract. Neurol. 13, 713–724. doi: 10.1038/nrneurol.2017.137
Ashina, M., Hansen, J. M., and Olesen, J. (2013). Pearls and pitfalls in human pharmacological models of migraine: 30 years’ experience. Cephalalgia 33, 540–553. doi: 10.1177/0333102412475234
Aziz, Q., Li, Y., Anderson, N., Ojake, L., Tsisanova, E., and Tinker, A. (2017). Molecular and functional characterization of the endothelial ATP-sensitive potassium channel. J. Biol. Chem. 292, 17587–17597. doi: 10.1074/jbc.M117.810325
Babenko, A. P., Aguilar-Bryan, L., and Bryan, J. (1998). A view of Sur/Kir6.X, KATP channels. Annu. Rev. Physiol. 60, 667–687. doi: 10.1146/annurev.physiol.60.1.667
Bao, L., Kefaloyianni, E., Lader, J., Hong, M., Morley, G., Fishman, G. I., et al. (2011). Unique properties of the ATP-sensitive K+ channel in the mouse ventricular cardiac conduction system. Circ. Arrhythm. Electrophysiol. 4, 926–935. doi: 10.1161/CIRCEP.111.964643
Bates, E. A., Nikai, T., Brennan, K. C., Fu, Y. H., Charles, A. C., Basbaum, A. I., et al. (2010). Sumatriptan alleviates nitroglycerin-induced mechanical and thermal allodynia in mice. Cephalalgia 30, 170–178. doi: 10.1111/j.1468-2982.2009.01864.x
Bernstein, C., and Burstein, R. (2012). Sensitization of the trigeminovascular pathway: perspective and implications to migraine pathophysiology. J Clin Neurol 8, 89–99. doi: 10.3988/jcn.2012.8.2.89
Bigal, M. E., Edvinsson, L., Rapoport, A. M., Lipton, R. B., Spierings, E. L. H., Diener, H.-C., et al. (2015). Safety, tolerability, and efficacy of TEV-48125 for preventive treatment of chronic migraine: a multicentre, randomised, double-blind, placebo-controlled, phase 2b study. Lancet Neurol. 14, 1091–1100. doi: 10.1016/S1474-4422(15)00245-8
Brayden, J. E. (2002). Functional roles of KATP channels in vascular smooth muscle. Clin. Exp. Pharmacol. Physiol. 29, 312–316. doi: 10.1046/j.1440-1681.2002.03650.x
Chalmer, M. A., Hansen, T. F., and Olesen, J. (2019). Nosographic analysis of osmophobia and field testing of diagnostic criteria including osmophobia. Cephalalgia 39, 38–43. doi: 10.1177/0333102418771375
Charles, A., and Pozo-Rosich, P. (2019). Targeting calcitonin gene-related peptide: a new era in migraine therapy. Lancet 394, 1765–1774. doi: 10.1016/S0140-6736(19)32504-8
Christensen, S. L., Munro, G., Petersen, S., Shabir, A., Jansen-Olesen, I., Kristensen, D. M., et al. (2020). ATP sensitive potassium (KATP) channel inhibition: a promising new drug target for migraine. Cephalalgia 40, 650–664. doi: 10.1177/0333102420925513
Christensen, S. L., Rasmussen, R. H., Cour, S. L., Ernstsen, C., Hansen, T. F., Kogelman, L. J., et al. (2022). Smooth muscle ATP-sensitive potassium channels mediate migraine-relevant hypersensitivity in mouse models. Cephalalgia 42, 93–107. doi: 10.1177/03331024211053570
Christensen, S. L., Rasmussen, R. H., Ernstsen, C., la Cour, S., David, A., Chaker, J., et al. (2021). CGRP-dependent signalling pathways involved in mouse models of GTN- cilostazol- and levcromakalim-induced migraine. Cephalalgia 41, 1413–1426. doi: 10.1177/03331024211038884
Christensen, C. G., Techlo, T. R., Kogelman, L. J. A., Wegner Thørner, L., Nissen, J., Sørensen, E., et al. (2022). Population-based prevalence of cranial autonomic symptoms in migraine and proposed diagnostic appendix criteria. Cephalalgia 42, 1160–1171. doi: 10.1177/03331024221094548
Clement, A., Guo, S., Jansen-Olesen, I., and Christensen, S. L. (2022). ATP-sensitive potassium channels in migraine: translational findings and therapeutic potential. Cells 11, 1–23. doi: 10.3390/cells11152406
Coskun, H., Elbahi, F. A., Al-Karagholi, M. A. M., Ghanizada, H., Sheykhzade, M., and Ashina, M. (2021). The effect of K ATP Channel blocker Glibenclamide on CGRP-induced headache and hemodynamic in healthy volunteers. Front. Physiol. 12:12. doi: 10.3389/fphys.2021.652136
Country, M. W., and Jonz, M. G. (2021). Mitochondrial KATP channels stabilize intracellular Ca2+ during hypoxia in retinal horizontal cells of goldfish (Carassius auratus). J. Exp. Biol. 224:jeb242634. doi: 10.1242/jeb.242634
Davidsson, O. B., Olofsson, I. A., Kogelman, L. J. A., Andersen, M. A., Rostgaard, K., Hjalgrim, H., et al. (2021). Twenty-five years of triptans - a nationwide population study. Cephalalgia 41, 894–904. doi: 10.1177/0333102421991809
Demartini, C., Greco, R., Zanaboni, A. M., Sances, G., de Icco, R., Borsook, D., et al. (2019). Nitroglycerin as a comparative experimental model of migraine pain: from animal to human and back. Prog. Neurobiol. 177, 15–32. doi: 10.1016/j.pneurobio.2019.02.002
Dodick, D. W., Goadsby, P. J., Silberstein, S. D., Lipton, R. B., Olesen, J., Ashina, M., et al. (2014a). Safety and efficacy of ALD403, an antibody to calcitonin gene-related peptide, for the prevention of frequent episodic migraine: a randomised, double-blind, placebo-controlled, exploratory phase 2 trial. Lancet Neurol. 13, 1100–1107. doi: 10.1016/S1474-4422(14)70209-1
Dodick, D. W., Goadsby, P. J., Spierings, E. L. H., Scherer, J. C., Sweeney, S. P., and Grayzel, D. S. (2014b). Safety and efficacy of LY2951742, a monoclonal antibody to calcitonin gene-related peptide, for the prevention of migraine: a phase 2, randomised, double-blind, placebo-controlled study. Lancet Neurol. 13, 885–892. doi: 10.1016/S1474-4422(14)70128-0
Donley, V. R., Hiskett, E. K., Kidder, A. C., and Schermerhorn, T. (2005). ATP-sensitive potassium channel (KATP channel) expression in the normal canine pancreas and in canine insulinomas. BMC Vet. Res. 1:8. doi: 10.1186/1746-6148-1-8
Eaton, M. J., Skatchkov, S. N., Brune, A., Biedermann, B., Veh, R. W., and Reichenbach, A. (2002). SURI and Kir6.1 subunits of K(ATP)-channels are co-localized in retinal glial (Müller) cells. Neuroreport 13, 57–60. doi: 10.1097/00001756-200201210-00016
Ernstsen, C., Christensen, S. L., Rasmussen, R. H., Nielsen, B. S., Jansen-Olesen, I., Olesen, J., et al. (2022). The PACAP pathway is independent of CGRP in mouse models of migraine: possible new drug target? Brain 145, 2450–2460. doi: 10.1093/brain/awac040
Ferrari, M. D., Diener, H. C., Ning, X., Galic, M., Cohen, J. M., Yang, R., et al. (2019). Fremanezumab versus placebo for migraine prevention in patients with documented failure to up to four migraine preventive medication classes (FOCUS): a randomised, double-blind, placebo-controlled, phase 3b trial. Lancet 394, 1030–1040. doi: 10.1016/S0140-6736(19)31946-4
Flagg, T. P., Kurata, H. T., Masia, R., Caputa, G., Magnuson, M. A., Lefer, D. J., et al. (2008). Differential structure of atrial and ventricular KATP: atrial KATP channels require SUR1. Circ. Res. 103, 1458–1465. doi: 10.1161/CIRCRESAHA.108.178186
Freiman, T. M., Kukolja, J., Heinemeyer, J., Eckhardt, K., Aranda, H., Rominger, A., et al. (2001). Modulation of K+-evoked [3H]-noradrenaline release from rat and human brain slices by gabapentin: involvement of KATP channels. Naunyn-Schmiedeberg’s Arch Pharmacol 363, 537–542. doi: 10.1007/s002100100408
Goldberg, M. R. (1988). Clinical pharmacology of pinacidil, a prototype for drugs that affect potassium channels. J. Cardiovasc. Pharmacol. 12, S41–S47. doi: 10.1097/00005344-198812002-00008
Gozalov, A., Jansen-Olesen, I., Klaerke, D., and Olesen, J. (2008). Role of KATP channels in cephalic vasodilatation induced by calcitonin gene-related peptide, nitric oxide, and transcranial electrical stimulation in the rat. Headache 48, 1202–1213. doi: 10.1111/j.1526-4610.2008.01205.x
Gozalov, A., Petersen, K. A., Mortensen, C., Jansen-Olesen, I., Klaerke, D., and Olesen, J. (2005). Role of KATP channels in the regulation of rat dura and pia artery diameter. Cephalalgia 25, 249–260. doi: 10.1111/j.1468-2982.2004.00848.x
Gribble, F. M., and Reimann, F. (2003). Sulphonylurea action revisited: the post-cloning era. Diabetologia 46, 875–891. doi: 10.1007/s00125-003-1143-3
Hauge, A. W., Asghar, M. S., Schytz, H. W., Christensen, K., and Olesen, J. (2009). Effects of tonabersat on migraine with aura: a randomised, double-blind, placebo-controlled crossover study. Lancet Neurol. 8, 718–723. doi: 10.1016/S1474-4422(09)70135-8
Hibino, H., Inanobe, A., Furutani, K., Murakami, S., Findlay, I., and Kurachi, Y. (2010). Inwardly rectifying potassium channels: their structure, function, and physiological roles. Physiol. Rev. 90, 291–366. doi: 10.1152/physrev.00021.2009
IHealthcareAnalyst I . (2023). Global Anti-migraine Drugs Market $15.6 Billion by 2029. Available at: https://www.ihealthcareanalyst.com/global-anti-migraine-drugs-market/ (Accessed January 30, 2023).
Inagaki, N., Gonoi, T., Iv, J. P. C., Wang, C. Z., Aguilar-Bryan, L., Bryan, J., et al. (1996). A family of sulfonylurea receptors determines the pharmacological properties of ATP-sensitive K+ channels. Neuron 16, 1011–1017. doi: 10.1016/S0896-6273(00)80124-5
Isomoto, S., Kondo, C., Yamada, M., Matsumoto, S., Higashiguchi, O., Horio, Y., et al. (1996). A novel sulfonylurea receptor forms with BIR (Kir6.2) a smooth muscle type ATP-sensitive K+ channel. J. Biol. Chem. 271, 24321–24324. doi: 10.1074/jbc.271.40.24321
Jahangir, A., and Terzic, A. (2005). K(ATP) channel therapeutics at the bedside. J. Mol. Cell. Cardiol. 39, 99–112. doi: 10.1016/j.yjmcc.2005.04.006
Jansen-Olesen, I., Mortensen, C. H., El-Bariaki, N., and Ploug, K. B. (2005). Characterization of KATP-channels in rat basilar and middle cerebral arteries: studies of vasomotor responses and mRNA expression. Eur. J. Pharmacol. 523, 109–118. doi: 10.1016/j.ejphar.2005.08.028
Khan, S., Amin, F. M., Christensen, C. E., Ghanizada, H., Younis, S., Olinger, A. C. R., et al. (2019). Meningeal contribution to migraine pain: a magnetic resonance angiography study. Brain 142, 93–102. doi: 10.1093/brain/awy300
Kidney, J. C., Fuller, R. W., Worsdell, Y. M., Lavender, E. A., Chung, K. F., and Barnes, P. J. (1993). Effect of an oral potassium channel activator, BRL 38227, on airway function and responsiveness in asthmatic patients: comparison with oral salbutamol. Thorax 48, 130–133. doi: 10.1136/thx.48.2.130
Kogelman, L. J. A., Esserlind, A.-L., Francke Christensen, A., Awasthi, S., Ripke, S., Ingason, A., et al. (2019). Migraine polygenic risk score associates with efficacy of migraine-specific drugs. Neurol Genet. 5:e364. doi: 10.1212/NXG.0000000000000364
Koh, S. D., Bradley, K. K., Rae, M. G., Keef, K. D., Horowitz, B., and Sanders, K. M. (1998). Basal activation of ATP-sensitive potassium channels in murine colonic smooth muscle cell. Biophys. J. 75, 1793–1800. doi: 10.1016/S0006-3495(98)77621-0
Kokoti, L., al-Karagholi, M. A. M., Elbahi, F. A., Coskun, H., Ghanizada, H., Amin, F. M., et al. (2022). Effect of KATPchannel blocker glibenclamide on PACAP38-induced headache and hemodynamic. Cephalalgia 42, 846–858. doi: 10.1177/03331024221080574
Kuburas, A., Mason, B. N., Hing, B., Wattiez, A.-S., Reis, A. S., Sowers, L. P., et al. (2021). PACAP induces light aversion in mice by an inheritable mechanism independent of CGRP. J. Neurosci. 41, 4697–4715. doi: 10.1523/JNEUROSCI.2200-20.2021
Lassen, L. H., Ashina, M., Christiansen, I., Ulrich, V., and Olesen, J. (1997). Nitric oxide synthase inhibition in migraine. Lancet 349, 401–402. doi: 10.1016/S0140-6736(97)80021-9
Lassen, L. H., Haderslev, P. A., Jacobsen, V. B., Iversen, H. K., Sperling, B., and Olesen, J. (2002). CGRP may play a causative role in migraine. Cephalalgia 22, 54–61. doi: 10.1046/j.1468-2982.2002.00310.x
Lassen, L. H., Thomsen, L. L., Kruuse, C., Iversen, H. K., and Olesen, J. (1996). Histamine-1 receptor blockade does not prevent nitroglycerin induced migraine. Support for the NO-hypothesis of migraine. Eur. J. Clin. Pharmacol. 49, 335–339. doi: 10.1007/BF00203773
Lassen, L. H., Thomsen, L. L., and Olesen, J. (1995). Histamine induces migraine via the H1-receptor. Support for the NO hypothesis of migraine. Neuroreport 6, 1475–1479. doi: 10.1097/00001756-199507310-00003
Lee, K., Dixon, A. K., Rowe, I. C. M., Ashford, M. L. J., and Richardson, P. J. (1995). Direct demonstration of sulphonylurea-sensitive KATP channels on nerve terminals of the rat motor cortex. Br. J. Pharmacol. 115, 385–388. doi: 10.1111/j.1476-5381.1995.tb16344.x
Li, Y., Aziz, Q., Anderson, N., Ojake, L., and Tinker, A. (2020). Endothelial ATP-sensitive Potassium Channel protects against the development of hypertension and atherosclerosis. Hypertens 76, 776–784. doi: 10.1161/HYPERTENSIONAHA.120.15355
Li, L., Wu, J., and Jiang, C. (2003). Differential expression of Kir6.1 and SUR2B mRNAs in the vasculature of various tissues in rats. J. Membr. Biol. 196, 61–69. doi: 10.1007/s00232-003-0625-z
Lipton, R. B., and Dodick, D. W. (2004). CGRP antagonists in the acute treatment of migraine. Lancet Neurol. 3:332. doi: 10.1016/S1474-4422(04)00764-1
Loder, E. (2010). Triptan therapy in migraine. N. Engl. J. Med. 363, 63–70. doi: 10.1056/NEJMct0910887
Lückhoff, A., and Busse, R. (1990). Activators of potassium channels enhance calcium influx into endothelial cells as a consequence of potassium currents. Naunyn Schmiedeberg's Arch. Pharmacol. 342, 94–99. doi: 10.1007/BF00178979
Mannhold, R. (2004). KATP channel openers: structure-activity relationships and therapeutic potential. Med. Res. Rev. 24, 213–266. doi: 10.1002/med.10060
Mele, A., Camerino, G. M., Calzolaro, S., Cannone, M., Conte, D., and Tricarico, D. (2014). Dual response of the KATP channels to staurosporine: a novel role of SUR2B, SUR1 and Kir6.2 subunits in the regulation of the atrophy in different skeletal muscle phenotypes. Biochem. Pharmacol. 91, 266–275. doi: 10.1016/j.bcp.2014.06.023
Miki, T., Suzuki, M., Shibasaki, T., Uemura, H., Sato, T., Yamaguchi, K., et al. (2002). Mouse model of Prinzmetal angina by disruption of the inward rectifier Kir6.1. Nat. Med. 8, 466–472. doi: 10.1038/nm0502-466
Munro, G., Petersen, S., Jansen-Olesen, I., and Olesen, J. (2018). A unique inbred rat strain with sustained cephalic hypersensitivity as a model of chronic migraine-like pain. Sci. Rep. 8:1836. doi: 10.1038/s41598-018-19901-1
Olesen, J., Friberg, L., Olsen, T. S., Iversen, H. K., Lassen, N. A., Andersen, A. R., et al. (1990). Timing and topography of cerebral blood flow, aura, and headache during migraine attacks. Ann. Neurol. 28, 791–798. doi: 10.1002/ana.410280610
Olesen, J., Larsen, B., and Lauritzen, M. (1981). Focal hyperemia followed by spreading oligemia and impaired activation of rCBF in classic migraine. Ann. Neurol. 9, 344–352. doi: 10.1002/ana.410090406
Oshinsky, M. L., Sanghvi, M. M., Maxwell, C. R., Gonzalez, D., Spangenberg, R. J., Cooper, M., et al. (2012). Spontaneous trigeminal allodynia in rats: a model of primary headache. Headache 52, 1336–1349. doi: 10.1111/j.1526-4610.2012.02247.x
Ploug, K. B., Amrutkar, D. V., Baun, M., Ramachandran, R., Iversen, A., Lund, T. M., et al. (2012). K ATP channel openers in the trigeminovascular system. Cephalalgia 32, 55–65. doi: 10.1177/0333102411430266
Ploug, K. B., Baun, M., Hay-Schmidt, A., Olesen, J., and Jansen-Olesen, I. (2010). Presence and vascular pharmacology of KATP channel subtypes in rat central and peripheral tissues. Eur. J. Pharmacol. 637, 109–117. doi: 10.1016/j.ejphar.2010.03.027
Ploug, K. B., Edvinsson, L., Olesen, J., and Jansen-Olesen, I. (2006). Pharmacological and molecular comparison of KATP channels in rat basilar and middle cerebral arteries. Eur. J. Pharmacol. 553, 254–262. doi: 10.1016/j.ejphar.2006.09.053
Ploug, K. B., Sørensen, M. A., Strøbech, L., Klaerke, D. A., Hay-Schmidt, A., Sheykhzade, M., et al. (2008). KATP channels in pig and human intracranial arteries. Eur. J. Pharmacol. 601, 43–49. doi: 10.1016/j.ejphar.2008.10.041
Pradhan, A. A., Smith, M. L., McGuire, B., Tarash, I., Evans, C. J., and Charles, A. (2014). Characterization of a novel model of chronic migraine. Pain 155, 269–274. doi: 10.1016/j.pain.2013.10.004
Quast, U. (1996). ATP-sensitive K+ channels in the kidney. Naunyn Schmiedeberg's Arch. Pharmacol. 354, 213–225. doi: 10.1007/BF00171051
Rea, B. J., Wattiez, A.-S., Waite, J. S., Castonguay, W. C., Schmidt, C. M., Fairbanks, A. M., et al. (2018). Peripherally administered calcitonin gene-related peptide induces spontaneous pain in mice: implications for migraine. Pain 159, 2306–2317. doi: 10.1097/j.pain.0000000000001337
Russell, M. B., and Ducros, A. (2011). Sporadic and familial hemiplegic migraine: pathophysiological mechanisms, clinical characteristics, diagnosis, and management. Lancet Neurol. 10, 457–470. doi: 10.1016/S1474-4422(11)70048-5
Sanabria, B. D., Palmegiani, E., Seron, A. F., Perdomo, Y. C., Miot, H. A., and Müller, R. P. (2022). Prospective cardiovascular evaluation with 24-hour Holter and 24-hour ambulatory blood pressure monitoring in men using 5-mg oral minoxidil for androgenetic alopecia. J. Am. Acad. Dermatol. 88, 436–437. doi: 10.1016/j.jaad.2022.05.026
Seino, S., and Miki, T. (2003). Physiological and pathophysiological roles of ATP-sensitive K+ channels. Prog. Biophys. Mol. Biol. 81, 133–176. doi: 10.1016/S0079-6107(02)00053-6
Simpson, D., and Wellington, K. (2004). Nicorandil: a review of its use in the management of stable angina pectoris, including high-risk patients. Drugs 64, 1941–1955. doi: 10.2165/00003495-200464170-00012
Skatchkov, S. N., Rojas, L., Eaton, M. J., Orkand, R. K., Biedermann, B., Bringmann, A., et al. (2002). Functional expression of Kir 6.1/SUR1-KATP channels in frog retinal Müller glial cells. Glia 38, 256–267. doi: 10.1002/glia.10073
Soundarapandian, M. M., Zhong, X., Peng, L., Wu, D., and Lu, Y. (2007). Role of K ATP channels in protection against neuronal excitatory insults. J. Neurochem. 103, 1721–1729. doi: 10.1111/j.1471-4159.2007.04963.x
Steiner, T. J., Stovner, L. J., Jensen, R., Uluduz, D., and Katsarava, Z. (2020). Migraine remains second among the world’s causes of disability, and first among young women: findings from GBD2019. J. Headache Pain 21, 4–7. doi: 10.1186/s10194-020-01208-0
Stovner, L. J., Nichols, E., Steiner, T. J., Abd-Allah, F., Abdelalim, A., al-Raddadi, R. M., et al. (2018). Global, regional, and national burden of migraine and tension-type headache, 1990-2016: a systematic analysis for the global burden of disease study 2016. Lancet Neurol. 17, 954–976. doi: 10.1016/S1474-4422(18)30322-3
Sun, H., Dodick, D. W., Silberstein, S., Goadsby, P. J., Reuter, U., Ashina, M., et al. (2016). Safety and efficacy of AMG 334 for prevention of episodic migraine: a randomised, double-blind, placebo-controlled, phase 2 trial. Lancet Neurol. 15, 382–390. doi: 10.1016/S1474-4422(16)00019-3
Tfelt-Hansen, P., and Loder, E. (2019). The Emperor’s new Gepants: are the effects of the new Oral CGRP antagonists clinically meaningful? Headache 59, 113–117. doi: 10.1111/head.13444
Tfelt-Hansen, P., and Olesen, J. (2012). Taking the negative view of current migraine treatments: the unmet needs. CNS Drugs 26, 375–382. doi: 10.2165/11630590-000000000-00000
Toulorge, D., Guerreiro, S., Hirsch, E. C., and Michel, P. P. (2010). KATP channel blockade protects midbrain dopamine neurons by repressing a glia-to-neuron signaling cascade that ultimately disrupts mitochondrial calcium homeostasis. J. Neurochem. 114, 553–564. doi: 10.1111/j.1471-4159.2010.06785.x
Vetvik, K. G., and MacGregor, E. A. (2021). Menstrual migraine: a distinct disorder needing greater recognition. Lancet Neurol. 20, 304–315. doi: 10.1016/S1474-4422(20)30482-8
Vollesen, L. H., Guo, S., Andersen, M. R., and Ashina, M. (2019). Effect of the H 1-antihistamine clemastine on PACAP38 induced migraine. Cephalalgia 39, 597–607. doi: 10.1177/0333102418798611
Wu, X. F., Liu, W. T., Liu, Y. P., Huang, Z. J., Zhang, Y. K., and Song, X. J. (2011). Reopening of ATP-sensitive potassium channels reduces neuropathic pain and regulates astroglial gap junctions in the rat spinal cord. Pain 152, 2605–2615. doi: 10.1016/j.pain.2011.08.003
Wu, S., Ren, X., Zhu, C., Wang, W., Zhang, K., Li, Z., et al. (2022). A c-Fos activation map in nitroglycerin/levcromakalim-induced models of migraine. J. Headache Pain 23:128. doi: 10.1186/s10194-022-01496-8
Yamada, M., Isomoto, S., Matsumoto, S., Kondo, C., Shindo, T., Horio, Y., et al. (1997). Sulphonylurea receptor 2B and Kir6.1 form a sulphonylurea-sensitive but ATP-insensitive K+ channel. J. Physiol. 499, 715–720. doi: 10.1113/jphysiol.1997.sp021963
Yang, L., Xu, M., Bhuiyan, S. A., Li, J., Zhao, J., Cohrs, R. J., et al. (2022). Human and mouse trigeminal ganglia cell atlas implicates multiple cell types in migraine. Neuron 110, 1806–1821.e8. doi: 10.1016/j.neuron.2022.03.003
Yildirim, C., Özkaya, B., and Bal, R. (2021). KATP and TRPM2-like channels couple metabolic status to resting membrane potential of octopus neurons in the mouse ventral cochlear nucleus. Brain Res. Bull. 170, 115–128. doi: 10.1016/j.brainresbull.2021.02.009
Zdravkovic, M., Kruse, M., Rost, K. L., Møss, J., and Kecskes, A. (2007). The effects of NN414, a SUR1/Kir6.2 selective potassium channel opener in subjects with type 2 diabetes. Exp. Clin. Endocrinol. Diabetes 115, 405–406. doi: 10.1055/s-2007-973062
Keywords: KATP antagonist, potassium channel, migraine, headache, levcromakalim, drug target
Citation: Clement A, Christensen SL, Jansen-Olesen I, Olesen J and Guo S (2023) The ATP sensitive potassium channel (KATP) is a novel target for migraine drug development. Front. Mol. Neurosci. 16:1182515. doi: 10.3389/fnmol.2023.1182515
Edited by:
Anan Srikiatkhachorn, King Mongkut’s Institute of Technology Ladkrabang, ThailandReviewed by:
Daniela Pietrobon, University of Padua, ItalyCopyright © 2023 Clement, Christensen, Jansen-Olesen, Olesen and Guo. This is an open-access article distributed under the terms of the Creative Commons Attribution License (CC BY). The use, distribution or reproduction in other forums is permitted, provided the original author(s) and the copyright owner(s) are credited and that the original publication in this journal is cited, in accordance with accepted academic practice. No use, distribution or reproduction is permitted which does not comply with these terms.
*Correspondence: Song Guo, c29uZy5ndW9AcmVnaW9uaC5kaw==