- 1Department of Psychiatry, Massachusetts General Hospital, Harvard Medical School, Charlestown, MA, United States
- 2Department of Radiology, The Second Affiliated Hospital of Guangzhou University of Chinese Medicine, Guangzhou, China
- 3Department of Neurology, The Second Affiliated Hospital of Guangzhou University of Chinese Medicine, Guangzhou, China
Background: Previous studies have shown a significant response to acute transcutaneous vagus nerve stimulation (taVNS) in regions of the vagus nerve pathway, including the nucleus tractus solitarius (NTS), raphe nucleus (RN) and locus coeruleus (LC) in both healthy human participants and migraine patients. This study aims to investigate the modulation effect of repeated taVNS on these brainstem regions by applying seed-based resting-state functional connectivity (rsFC) analysis.
Methods: 70 patients with migraine were recruited and randomized to receive real or sham taVNS treatments for 4 weeks. fMRI data were collected from each participant before and after 4 weeks of treatment. The rsFC analyses were performed using NTS, RN and LC as the seeds.
Results: 59 patients (real group: n = 33; sham group: n = 29) completed two fMRI scan sessions. Compared to sham taVNS, real taVNS was associated with a significant reduction in the number of migraine attack days (p = 0.024) and headache pain intensity (p = 0.008). The rsFC analysis showed repeated taVNS modulated the functional connectivity between the brain stem regions of the vagus nerve pathway and brain regions associated with the limbic system (bilateral hippocampus), pain processing and modulation (bilateral postcentral gyrus, thalamus, and mPFC), and basal ganglia (putamen/caudate). In addition, the rsFC change between the RN and putamen was significantly associated with the reduction in the number of migraine days.
Conclusion: Our findings suggest that taVNS can significantly modulate the vagus nerve central pathway, which may contribute to the potential treatment effects of taVNS for migraine.
Clinical Trial Registration: http://www.chictr.org.cn/hvshowproject.aspx?id=11101, identifier ChiCTR-INR-17010559.
Introduction
Vagus nerve stimulation has emerged as a potential treatment modality in the management of migraine (Straube et al., 2015; Grazzi et al., 2016; Tao et al., 2018; Zhang et al., 2021). Previous studies have demonstrated a significant response to acute transcutaneous vagus nerve stimulation (taVNS) in regions of the vagus nerve pathway, including the nucleus tractus solitarius (NTS), raphe nucleus (RN) and locus coeruleus (LC) in both healthy human participants and patients with migraine (Frangos et al., 2015; Yakunina et al., 2017, 2018; Sclocco et al., 2019, 2020; Zhang et al., 2019; Borgmann et al., 2021). However, the modulation effect of repeated taVNS on these key brain stem regions has yet to be examined.
Approximately 80% of vagus nerve (VN) fibers are sensory fibers that relay both somatic and general visceral signals (Yuan and Silberstein, 2016). The afferent vagal nerves primarily project to the nucleus tractus solitarius, which in turn transmits the signal to the other brain stem nodes such as the locus coeruleus, raphe nuclei, and other subcortical and cortical regions (Dorr and Debonnel, 2006; Manta et al., 2009, 2012; Sacca et al., 2022).
Literature suggests that the locus coeruleus and raphe nuclei are also associated with two neurotransmitter systems potentially important in regulating migraine (Saper, 2011; Obata, 2017). The first neurotransmitter system is the noradrenergic system, which plays a major role in arousal, attention, and the stress response (Berridge and Spencer, 2017), as well as long-term synaptic plasticity and pain modulation (Benarroch, 2009a,b). The locus coeruleus has the greatest amount of noradrenaline in the central nervous system and provides extremely widespread noradrenergic innervations of the entire cortex, diencephalon, and many other brain structures (Benarroch, 2009a,b). Noradrenergic neurons in the LC and its terminals in the dorsal reticular nucleus (DRt), medial prefrontal cortex (mPFC), spinal dorsal horn, and trigeminal spinal nucleus caudalis (spVc) have been shown to participate in the development and maintenance of allodynia and hyperalgesia after nerve injury (Taylor and Westlund, 2017), in addition to their well-described role in inhibiting spinal nociceptive transmissions. Thus, the LC may be a critical modulator for both pain inhibition and facilitation.
The second neurotransmitter system involved in regulating the pain experience is the serotonergic neuromodulatory system. Literature suggests that serotonin plays a major role in modulating pain perception. For instance, combined serotonin-norepinephrine reuptake inhibitors (SNRI) are increasingly considered to be the most effective treatment in the patients comorbid with depression and migraine (Goadsby et al., 2017; Burch, 2019). In the central nervous system, serotonergic innervation of the cerebral cortex, subcortical structures, and cerebellum mainly originate from the dorsal and median raphe nuclei (RN) (Ren et al., 2018).
The aim of this study is to explore the modulatory effect of repeated taVNS on the resting-state functional connectivity (rsFC) of the NTS, LC, and RN in migraine patients. We hypothesize that these brain stem targets of vagal afferents may be modulated by repeated taVNS.
Methods
Participants
70 patients with migraine were recruited and randomized in an equal ratio to receive real or sham taVNS treatments. Migraine was diagnosed by licensed neurobiologists based on the International Classification of Headache Disorders, 2nd Edition (ICHD-II). The study’s protocol was approved by the Institutional Review Board of the Second Affiliated Hospital of Guangzhou University of Chinese Medicine.
The dataset has been previously used to investigate how taVNS stimulation can modulate functional connectivity of subregions of the thalamus (Zhang et al., 2021). This study focuses on how taVNS can modulate the functional connectivity of key regions in the brain stem vagus nerve pathway (NTS, LC, and RN), which has not been published before. Please see supplementary materials or our previous publication (Zhang et al., 2021) for inclusion and exclusion material.
Experimental procedure
All patients underwent two MRI scans (before and after repeated taVNS). The entire study lasted for about 8 weeks, consisting of a baseline period of 4 weeks collecting migraine attack characteristics prior to treatment and the 4-week treatment period. At enrollment, patients were requested to maintain diary records of their headaches for the duration of the study. Each recorded headache entry included onset time, duration, pain intensity (using Visual Analog Scale (VAS) score), accompanying symptoms, and the use of rescue medication if any. Both real and sham taVNS treatments consisted of 12 sessions in a schedule of 3 sessions per week for 4 weeks, with each session lasting for 30 min. A 1 Hz continuous electrical stimulation was applied at the left cymba concha (Badran et al., 2018) for real taVNS treatment, while the sham stimulation site was located on the left tail of the helix (Badran et al., 2018). See supplementary materials for details in experimental procedures and data acquisition.
Functional analysis
Functional data preprocessing and statistical analysis were performed in the SPM12-based toolbox CONN19.c (Whitfield-Gabrieli and Nieto-Castanon, 2012),1 SUIT (Diedrichsen, 2006),2 and AFNI software version 17.2.05 (Cox et al., 2017).3 See supplementary materials for data preprocessing procedures.
The bilateral NTS were defined based on a publicly available template provided by the team of Nikos Priovoulos (Priovoulos et al., 2019). The bilateral RN (combination of median and dorsal raphe) and LC seeds were defined based on the Automatic Anatomical Labeling 3 atlas 3 (AAL) template (Pollak Dorocic et al., 2014). In the first-level analysis, we produced a correlation map for each patient by extracting the BOLD time series from each brainstem seed and computing Pearson’s correlation coefficients between the time series in every seed and all other voxels of the whole brain, respectively. Correlation coefficients were Fisher transformed into ‘Z’ scores to increase normality.
Seed-to-voxel second-level analyses were performed using a mixed-designed ANOVA with treatment (real taVNS vs. sham taVNS) entered as the between-subject factor, time (pre-treatment vs. post-treatment) as the within-subject factor, and age and gender as covariates. We also performed a one-sample t-test to assess the baseline functional connectivity of each seed in all migraine patients. A threshold of voxel-wise p < 0.005 and cluster-level p < 0.05 FDR corrected was applied for second-level analyses.
Based on previous imaging studies, our a prior regions of interest included: (1) downstream targets of vagal afferents in the brain stem: LC and RN (Dorr and Debonnel, 2006; Manta et al., 2009, 2012) and (2) the pain perception and modulation network in migraine: hypothalamus, thalamus, hippocampus, amygdala, insula, anterior cingulate cortex (ACC), medial prefrontal cortex (mPFC) and postcentral gyrus (PoCG) (Frangos et al., 2015; Hachem et al., 2018; Kaniusas et al., 2019; Lee et al., 2019; Silberstein et al., 2020; Ashina et al., 2021). An initial threshold of voxel-wise p < 0.005 was used in all data analysis. To correct for multiple comparisons, Monte Carlo simulations using the 3dFWHMx and 3dClustSim implemented in AFNI were applied for these ROIs (i.e., brain regions listed above, where for each region, the minimum voxel size required for p < 0.05 cluster level p-value correction will be indicated as a k value in the results presented below). For the rest of the brain, a voxel-wise threshold of p < 0.005 with p < 0.05 cluster level FDR correction was applied. A similar analysis was performed in our previous study (Gollub et al., 2018).
To investigate the association between the rsFC change and the primary clinical outcome (number of migraine attack days), we created a sphere with a 2 mm radius using the peak coordinates of the brain regions that produced significant rsFC changes after taVNS and extracted the average z values. Then, we performed a correlation analysis between the rsFC z value changes and the corresponding clinical outcomes across all participants.
Results
Clinical outcome
59 patients (33 in the taVNS group, 26 in the sham taVNS group) completed two MRI scans before and after 4 weeks of treatment. No patients were excluded due to fMRI head motion correction based on the calculated mean frame displacement (FD) standard.
Compared to the sham taVNS group, patients in the real taVNS treatment group had a significant reduction in the number of migraine attack days ([F (1,57) =5.41, p = 0.024]), headache pain intensity ([F (1,57) = 7.52, p = 0.008]), and migraine attack times (numbers) ([F (1,57) = 6.29, p = 0.015]). There was no significant improvement in MSQ, SAS and SDS evaluation after real taVNS treatment. Detailed patients’ baseline characteristics and clinical outcome measurements were shown in Supplementary Table e1.
Functional connectivity results
NTS functional connectivity results
Among all migraine patients, baseline functional connectivity of the NTS showed positive connectivity with the bilateral angular gyrus (AG), superior/middle frontal gyrus, posterior cingulate gyrus, fusiform gyrus; left hippocampus/parahippocampus, thalamus, cerebellum, and right lingual gyrus; and negative connectivity with the bilateral dorsal lateral prefrontal cortex (dlPFC), putamen, middle/superior temporal gyrus, supramarginal gyrus, supplementary motor cortex, superior frontal gyrus (SFG), left inferior occipital gyrus (IOG), postcentral gyrus (PoCG), and right precentral gyrus (PreCG). Baseline functional connectivity of the NTS is shown in Supplementary Table e2.
Compared to the sham group, the real taVNS group showed decreased NTS functional connectivity with the bilateral medial prefrontal cortex (mPFC) and bilateral hippocampus (HPC); and increased functional connectivity with the bilateral raphe nuclei after treatment (Table 1 and Figure 1).
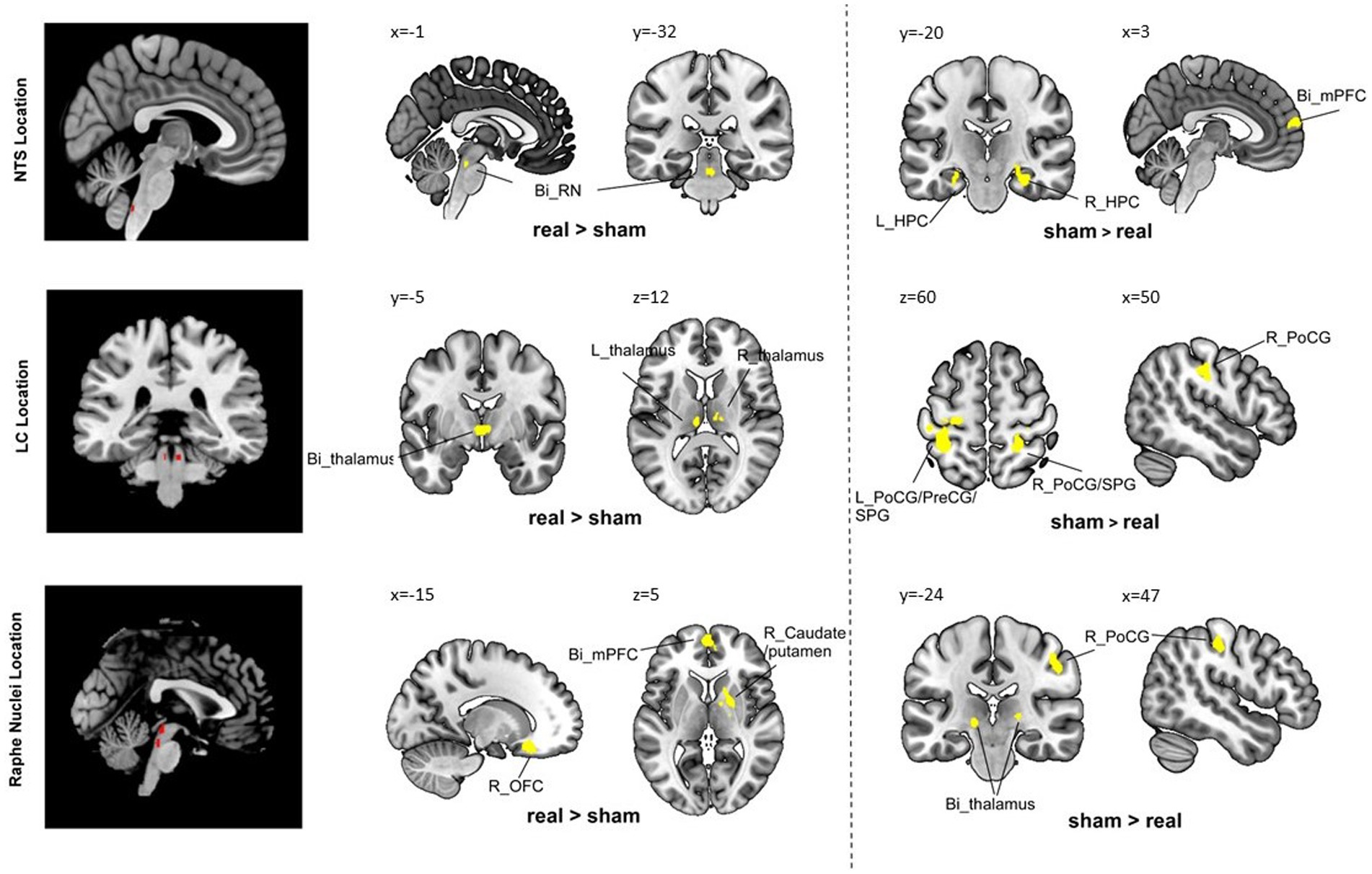
Figure 1. Group comparison results. Bi, bilateral; R, right; L, left; PoCG, postcentral gyrus; PreCG, precentral gyrus; SPG, superior parietal gyrus; AI, anterior insula; OFC, orbital frontal cortex; AG, angular gyrus; mPFC, medial prefrontal cortex; HPC, hippocampus; MCC, middle cingulate cortex.
LC functional connectivity results
Baseline functional connectivity of the LC showed positive connectivity with the bilateral cerebellum, lingual gyrus, and hippocampus/parahippocampus, and negative connectivity with right occipital fusiform gyrus (Supplementary Table e2).
Compared to the sham group, the real taVNS group showed decreased LC functional connectivity with the bilateral postcentral gyrus (PoCG), superior parietal gyrus (SPG), and left precentral gyrus (PreCG); and increased functional connectivity with the bilateral thalamus after treatment (Table 1 and Figure 1).
RN functional connectivity results
Baseline functional connectivity of the RN showed positive connectivity with the left thalamus and negative connectivity with the left caudate and right occipital pole (Supplementary Table e2).
Compared to the sham group, the real taVNS group showed decreased RN functional connectivity with the bilateral thalamus and right PoCG; and increased functional connectivity with the bilateral mPFC/anterior cingulate cortex (ACC), left occipital frontal cortex (OFC), left angular gyrus (AG), right caudate, and putamen after treatment (Table 1 and Figure 1).
Association with primary clinical outcome
Pearson’s correlation showed that the RN – right putamen rsFC change was significantly correlated with the primary outcome (reduction of the number of migraine days, R = 0.55, p < 0.001, p = 0.015 after Bonferroni correction) in the real taVNS group after treatment (Figure 2).
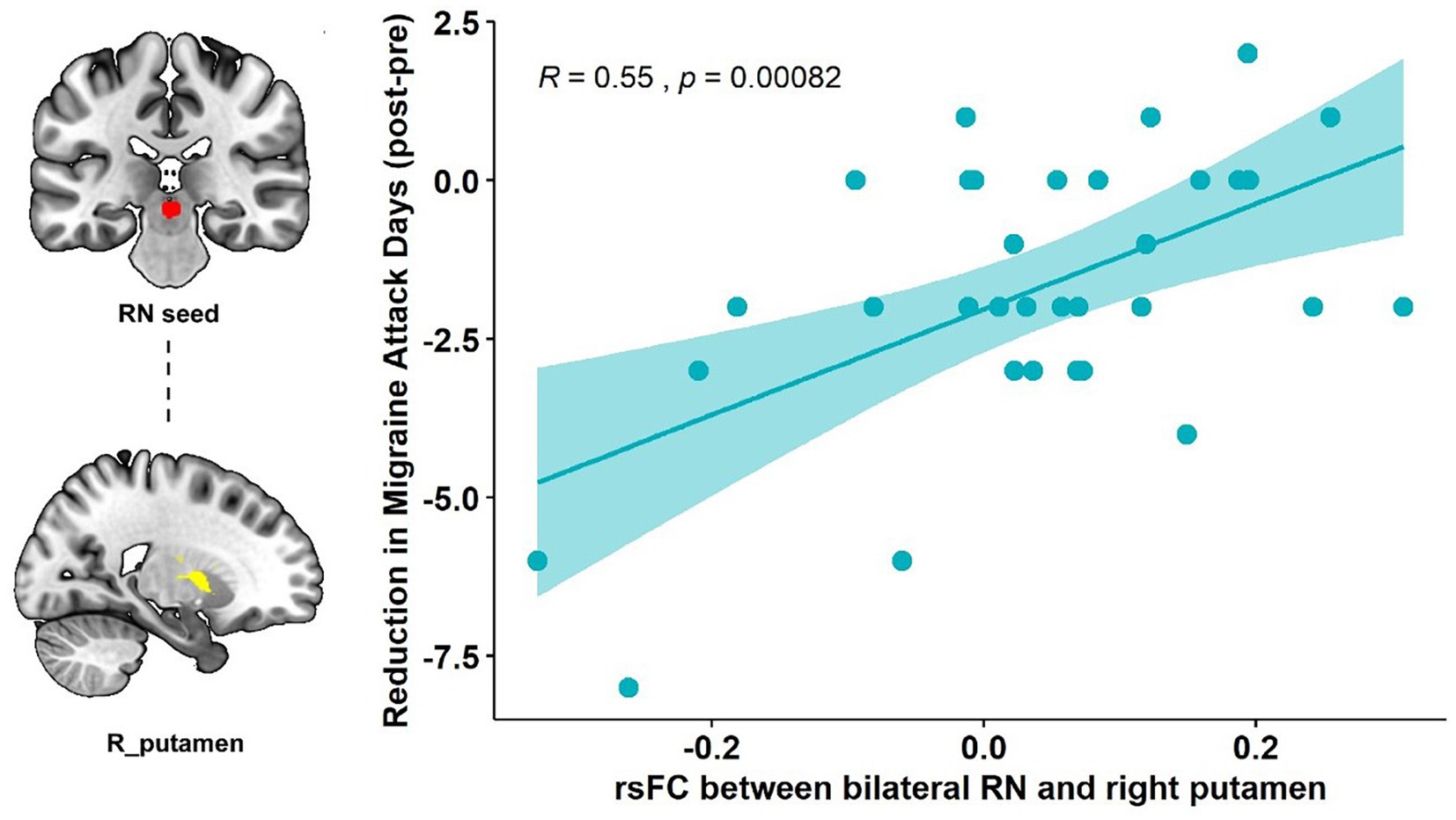
Figure 2. Correlation results of the RN-putamen rsFC change and clinical outcomes. The scatterplot indicates the significant correlation between the change in number of migraine attack days and the rsFC change of the bilateral RN and right putamen after real taVNS treatment (R = 0.55, p < 0.001, significant after Bonferroni correction).
There is no significant association between NTS/LC rsFC change and the corresponding primary clinical outcome changes.
Discussion
In the present study, we investigated the modulatory effects of repeated taVNS on functional connectivity of the NTS, LC and RN in patients with migraine. Comparisons of clinical measurements indicated repeated taVNS could significantly relieve patients’ symptoms. Functional analysis showed that repeated taVNS could modulate the connection between the NTS and key brain regions in the limbic system (bilateral hippocampus), and the functional connectivity of both the LC and RN with brain regions closely relating to pain processing and modulation (bilateral postcentral gyrus/bilateral thalamus/mPFC). Our findings also demonstrated that repeated taVNS could strengthen the functional connectivity between the RN and caudate/putamen, the main dopaminergic projecting targets. The RN-caudate/putamen rsFC change was significantly associated with the reduction in the number of migraine attack days. These findings suggests that repeated taVNS could significantly modulate the vagus nerve pathway in patients with migraine.
As the major target of vagal afferents, the NTS receives direct inputs from the vagus nerve and subsequently projects to other brainstem regions including the LC, RN and parabrachial nucleus, along with the forebrain limbic structures such as the periventricular nucleus of the hypothalamus, the thalamus, the central nucleus of the amygdala, the hippocampus, the bed nucleus of the stria terminalis, and the nucleus accumbens (Zoccal et al., 2014). In the current study, we observed a significantly decreased NTS rsFC with the bilateral mPFC and hippocampus and increased rsFC with the RN after the real taVNS treatments compared to the sham.
The hippocampus is a main structure of the limbic system. Accumulating evidence suggests that limbic system, and the hippocampus alone, is involved in pain processing, pain-related attention, anxiety, and the stress response (Liu and Chen, 2009; Mokhtari et al., 2019; Yu et al., 2020). Both structural and functional abnormalities of the hippocampus have been reported in migraine patients (Biggio et al., 2009). Literature suggests that headache frequency, cumulative number of migraine attacks, anxiety scores, depression scores, and genetic variants are related to hippocampal morphology and functional changes in individuals with migraine (Liu et al., 2018). A previous neuroimaging study found that migraineurs had greater pain-induced activation of the hippocampus, and the activation strength had a significant correlation with headache frequency in migraine patients (Schwedt et al., 2014). Our results suggest that taVNS could modulate the functional connection between the hippocampus and the NTS. This finding is also consistent with a previous study in which the authors found that plasticity within the hippocampus is an important contributor to the vagus nerve response (Biggio et al., 2009).
We found that taVNS could modulate the rsFC of both the LC and RN with brain regions closely relating to pain processing and modulation (PoCG/thalamus/mPFC). In particular, both the LC and RN is associated with a reduced functional connectivity with the PoCG after repeated taVNS treatment. Previous studies have identified involvement of the PoCG in chronic pain (Kong et al., 2013; Ossipov et al., 2014). Particularly, the majority of patients with migraine show somatosensory hypersensitivity (i.e., reduced cutaneous pain thresholds) and other symptoms of somatosensory hypersensitivity (i.e., cutaneous allodynia) during migraine attacks (Schwedt, 2013). The LC sends strong projections to the sensory cortex and has long been posited to play an important role in modulating sensory encoding (Bruinstroop et al., 2012; Vazey et al., 2018).
As the major source of serotonergic projections, the raphe nucleus plays a role in the regulation of multiple functional systems, including the somatosensory system (Hornung, 2003). Our results suggest that repeated taVNS may regulate the perception of nociceptive inputs in patients with migraine by interfering with the connection between LC/RN and PoCG. These connectivity changes may alter the projection of noradrenergic and serotonergic neurotransmitters in the brain stem to the somatosensory cortex.
The thalamus is a major center in the brain for processing nociceptive information and relays this information to cortical regions for further processing (Lenz et al., 2004). Evidence of activation of the thalamus during migraines is clear and well established (Bahra et al., 2001; Afridi et al., 2005; Tu et al., 2019). It is worth noting that our results showed that repeated taVNS could modulate the rsFC of both the LC and RN with the thalamus in patients with migraine. A previous study found that both noradrenergic and serotonergic fibers innervate the thalamus, and the origins of these projections are primarily located at the LC and RN (Westlund et al., 1991). We also found that taVNS can significantly modulate the connectivity between the thalamus and sensory-motor cortex (Zhang et al., 2021). Interestingly, we found that after repeated taVNS, the rsFC of the thalamus was increased with the LC but decreased with the RN. As the noradrenergic and serotonergic fibers project to multiple nuclei of the thalamus, involving both excitatory and inhibitory effects (Varela, 2014), future studies are needed to investigate more details on how taVNS may affect the LC and RN projections to the thalamus.
We also found that taVNS could significantly modulate the rsFC between the RN and bilateral mPFC / ACC in patients with migraine. The mPFC is a crucial brain region that integrates information from multiple cortical and subcortical areas and converges updated outputs to downstream structures. In addition, the mPFC / ACC is also a key region of the descending pain modulation system (Kong et al., 2010). The functional and structural alterations of the mPFC in patients with migraine have been reported (Jin et al., 2013; Soheili-Nezhad et al., 2019). It was considered to play an essential role in regulating pain-related emotion (depression and anxiety) and cognition in patients with migraine (Ma et al., 2018; Tu et al., 2019; Zhang et al., 2019). A previous study showed that migraine is associated with increased PAG – mPFC connectivity, and the increased connectivity decreased after acupuncture treatments (Li et al., 2016). We also found taVNS can modulate the PAG – ACC/mPFC connectivity in patient with migraine (Cao et al., 2021). Interestingly, we explored the association between the RN-mPFC rsFC change and changes in depression scores in the repeated taVNS treatment group. The results showed that these two factors are highly correlated, with a p value of 0.0038 (R = −0.49). Our result suggests that taVNS may hold the potential to relieve both headache and depression in patients comorbid with migraine and depression. This is consistent with our previous studies showing that taVNS can also reduce symptoms in patients with depression (Kong et al., 2018; Li et al., 2022).
Our results showed that repeated taVNS significantly altered the functional connectivity between the RN and basal ganglia subregions, specifically the right putamen and caudate. This connectivity change is associated with a reduction in the number of migraine attack days, i.e., the higher the functional strength between RN and right putamen, the greater the reduction in the number of attack days in migraine patients.
Literature has suggested that the basal ganglia may be involved in the sensory, emotional / cognitive, and endogenous/modulatory domains of pain processing (Borsook et al., 2010). In particular, the putamen is one of the major sites of cortical input into basal ganglia loops and is frequently activated during pain (Starr et al., 2011). Dysfunctions of the monoamine systems, especially the dopaminergic and serotonergic projections to the basal ganglia, were reported to correlate with individual ratings of the sensory and unpleasant qualities of the experience of pain(Scott et al., 2006) and might be the early result of chronic maladaptation to persistent pain (Sagheddu et al., 2015). Thus, we speculate that the repeated administration of taVNS might modulate pain perception attributed to the serotonin activation in the putamen projected from the RN in patients with migraine.
There are several limitations to this study. Firstly, the current study was conducted over a 4-week period and therefore the effects observed can only represent short-and mid-term effects; a study with a longer duration is needed to investigate long-term effects. Secondly, the sham stimulation was applied at the tail of the helix with the same electrical stimulation parameters, which might also activate the sensory related cortex. Caution needs to be taken in the interpretation of the comparison results of the rsFC change between the two groups, especially when it comes to the upregulation of somatosensory cortex. Future studies are needed to validate / replicate our findings.
In conclusion, we found that repeated taVNS can modulate the connection between the key nodes of the brain stem vagus nerve pathway with the limbic system and pain perception and modulation networks. These findings suggest that repeated taVNS may yield pain relief through modulating of the NTS, LC, and RN connections with the above regions, which may further affect their synaptic plasticity and neurotransmitter release associated with migraine.
Data availability statement
The raw data supporting the conclusions of this article will be made available per request.
Ethics statement
The studies involving human participants were reviewed and approved by the Institutional Review Board of the Second Affiliated Hospital of Guangzhou University of Chinese Medicine. The patients/participants provided their written informed consent to participate in this study.
Author contributions
YZ, BL, and JK: design and conceptualized study. YH and JK: analyze and interpret the data. YZ, HL, ZY, XL, XH, and WC: acquisition of data. YH, SH, TC-Z, and JK: manuscript preparation. YZ and BL: funding acquisition. All authors contributed to the article and approved the submitted version.
Funding
This study was supported by the Medical Scientific Research Foundation of Guangdong Province of China (A2017234) and the Administration of Traditional Chinese Medicine of Guangdong Province of China (20182047).
Conflict of interest
The authors declare that the research was conducted in the absence of any commercial or financial relationships that could be construed as a potential conflict of interest.
Publisher’s note
All claims expressed in this article are solely those of the authors and do not necessarily represent those of their affiliated organizations, or those of the publisher, the editors and the reviewers. Any product that may be evaluated in this article, or claim that may be made by its manufacturer, is not guaranteed or endorsed by the publisher.
Supplementary material
The Supplementary material for this article can be found online at: https://www.frontiersin.org/articles/10.3389/fnmol.2023.1160006/full#supplementary-material
Footnotes
References
Afridi, S. K., Giffin, N. J., Kaube, H., Friston, K. J., Ward, N. S., Frackowiak, R. S. J., et al. (2005). A positron emission tomographic study in spontaneous migraine. Arch. Neurol. 62, 1270–1275. doi: 10.1001/archneur.62.8.1270
Ashina, S., Bentivegna, E., Martelletti, P., and Eikermann-Haerter, K. (2021). Structural and functional brain changes in migraine. Pain Ther. 10, 211–223. doi: 10.1007/s40122-021-00240-5
Badran, B. W., Brown, J. C., Dowdle, L. T., Mithoefer, O. J., LaBate, N. T., Coatsworth, J., et al. (2018). Tragus or cymba conchae? Investigating the anatomical foundation of transcutaneous auricular vagus nerve stimulation (taVNS). Brain Stimul. 11, 947–948. doi: 10.1016/j.brs.2018.06.003
Bahra, A., Matharu, M. S., Buchel, C., Frackowiak, R. S. J., and Goadsby, P. J. (2001). Brainstem activation specific to migraine headache. Lancet 357, 1016–1017. doi: 10.1016/S0140-6736(00)04250-1
Benarroch, E. E. (2009a). The locus ceruleus norepinephrine system-functional organization and potential clinical significance. Neurology 73, 1699–1704. doi: 10.1212/WNL.0b013e3181c2937c
Benarroch, E. E. (2009b). The locus ceruleus norepinephrine system: functional organization and potential clinical significance. Neurology 73, 1699–1704. doi: 10.1212/WNL.0b013e3181c2937c
Berridge, C. W., and Spencer, R. C. (2017). “Chapter 4 - noradrenergic control of arousal and stress” in Stress: neuroendocrinology and neurobiology. ed. G. Fink (San Diego: Academic Press), 39–47.
Biggio, F., Gorini, G., Utzeri, C., Olla, P., Marrosu, F., Mocchetti, I., et al. (2009). Chronic vagus nerve stimulation induces neuronal plasticity in the rat hippocampus. Int. J. Neuropsychopharmacol. 12, 1209–1221. doi: 10.1017/S1461145709000200
Borgmann, D., Rigoux, L., Kuzmanovic, B., Edwin Thanarajah, S., Münte, T. F., Fenselau, H., et al. (2021). Technical note: modulation of fMRI brainstem responses by transcutaneous vagus nerve stimulation. NeuroImage 244:118566. doi: 10.1016/j.neuroimage.2021.118566
Borsook, D., Upadhyay, J., Chudler, E. H., and Becerra, L. (2010). A key role of the basal ganglia in pain and analgesia--insights gained through human functional imaging. Mol. Pain 6:27. doi: 10.1186/1744-8069-6-27
Bruinstroop, E., Cano, G., Vanderhorst, V. G. J. M., Cavalcante, J. C., Wirth, J., Sena-Esteves, M., et al. (2012). Spinal projections of the A5, A6 (locus coeruleus), and A7 noradrenergic cell groups in rats. J. Comp. Neurol. 520, 1985–2001. doi: 10.1002/cne.23024
Burch, R. (2019). Antidepressants for preventive treatment of migraine. Curr. Treat. Options Neurol. 21:18. doi: 10.1007/s11940-019-0557-2
Cao, J., Zhang, Y., Li, H., Yan, Z., Liu, X., Hou, X., et al. (2021). Different modulation effects of 1 Hz and 20 Hz transcutaneous auricular vagus nerve stimulation on the functional connectivity of the periaqueductal gray in patients with migraine. J. Transl. Med. 19:354. doi: 10.1186/s12967-021-03024-9
Cox, R. W., Chen, G., Glen, D. R., Reynolds, R. C., and Taylor, P. A. (2017). fMRI clustering and false-positive rates. Proc. Natl. Acad. Sci. U. S. A. 114, E3370–e3371. doi: 10.1073/pnas.1614961114
Diedrichsen, J. (2006). A spatially unbiased atlas template of the human cerebellum. NeuroImage 33, 127–138. doi: 10.1016/j.neuroimage.2006.05.056
Dorr, A. E., and Debonnel, G. (2006). Effect of vagus nerve stimulation on serotonergic and noradrenergic transmission. J. Pharmacol. Exp. Ther. 318, 890–898. doi: 10.1124/jpet.106.104166
Frangos, E., Ellrich, J., and Komisaruk, B. R. (2015). Non-invasive access to the Vagus nerve central projections via electrical stimulation of the external ear: fMRI evidence in humans. Brain Stimul. 8, 624–636. doi: 10.1016/j.brs.2014.11.018
Goadsby, P. J., Holland, P. R., Martins-Oliveira, M., Hoffmann, J., Schankin, C., and Akerman, S. (2017). Pathophysiology of migraine: a disorder of sensory processing. Physiol. Rev. 97, 553–622. doi: 10.1152/physrev.00034.2015
Gollub, R. L., Kirsch, I., Maleki, N., Wasan, A. D., Edwards, R. R., Tu, Y., et al. (2018). A functional neuroimaging study of expectancy effects on pain response in patients with knee osteoarthritis. J. Pain 19, 515–527. doi: 10.1016/j.jpain.2017.12.260
Grazzi, L., Egeo, G., Calhoun, A. H., McClure, C. K., Liebler, E., and Barbanti, P. (2016). Non-invasive Vagus nerve stimulation (nVNS) as mini-prophylaxis for menstrual/menstrually related migraine: an open-label study. J. Headache Pain 17:91. doi: 10.1186/s10194-016-0684-z
Hachem, L. D., Wong, S. M., and Ibrahim, G. M. (2018). The vagus afferent network: emerging role in translational connectomics. Neurosurg. Focus. 45:E2. doi: 10.3171/2018.6.FOCUS18216
Hornung, J. P. (2003). The human raphe nuclei and the serotonergic system. J. Chem. Neuroanat. 26, 331–343. doi: 10.1016/j.jchemneu.2003.10.002
Jin, C., Yuan, K., Zhao, L., Zhao, L., Yu, D., von Deneen, K. M., et al. (2013). Structural and functional abnormalities in migraine patients without aura. NMR Biomed. 26, 58–64. doi: 10.1002/nbm.2819
Kaniusas, E., Kampusch, S., Tittgemeyer, M., Panetsos, F., Gines, R. F., Papa, M., et al. (2019). Current directions in the auricular Vagus nerve stimulation I – a physiological perspective. Front. Neurosci. 13:854. doi: 10.3389/fnins.2019.00854
Kong, J., Fang, J., Park, J., Li, S., and Rong, P. (2018). Treating depression with transcutaneous auricular Vagus nerve stimulation: state of the art and future perspectives. Front. Psych. 9:20. doi: 10.3389/fpsyt.2018.00020
Kong, J., Spaeth, B., Wey, H. Y., Cheetham, A., Cook, A. H., Jensen, K., et al. (2013). S1 is associated with chronic low back pain: a functional and structural MRI study. Mol. Pain 9:43. doi: 10.1186/1744-8069-9-43
Kong, J., Tu, P. C., Zyloney, C., and Su, T. P. (2010). Intrinsic functional connectivity of the periaqueductal gray, a resting fMRI study. Behav. Brain Res. 211, 215–219. doi: 10.1016/j.bbr.2010.03.042
Lee, M. J., Park, B. Y., Cho, S., Kim, S. T., Park, H., and Chung, C. S. (2019). Increased connectivity of pain matrix in chronic migraine: a resting-state functional MRI study. J. Headache Pain 20:29. doi: 10.1186/s10194-019-0986-z
Lenz, F. A., Weiss, N., Ohara, S., Lawson, C., and Greenspan, J. D. (2004). “Chapter 6 the role of the thalamus in pain” in Supplements to clinical neurophysiology. eds. M. Hallett, L. H. Phillips, D. L. Schomer, and J. M. Massey, vol. 57 (Radarweg, Amsterdam: Elsevier), 50–61.
Li, Z., Liu, M., Lan, L., Zeng, F., Makris, N., Liang, Y., et al. (2016). Altered periaqueductal gray resting state functional connectivity in migraine and the modulation effect of treatment. Sci. Rep. 6:20298. doi: 10.1038/srep20298
Li, S., Rong, P., Wang, Y., Jin, G., Hou, X., Li, S., et al. (2022). Comparative effectiveness of transcutaneous auricular Vagus nerve stimulation vs citalopram for major depressive disorder: a randomized trial. Neuromodulation 25, 450–460. doi: 10.1016/j.neurom.2021.10.021
Liu, M.-G., and Chen, J. (2009). Roles of the hippocampal formation in pain information processing. Neurosci. Bull. 25, 237–266. doi: 10.1007/s12264-009-0905-4
Liu, H.-Y., Chou, K.-H., and Chen, W.-T. (2018). Migraine and the Hippocampus. Curr. Pain Headache Rep. 22:13. doi: 10.1007/s11916-018-0668-6
Ma, M., Zhang, J., Chen, N., Guo, J., Zhang, Y., and He, L. (2018). Exploration of intrinsic brain activity in migraine with and without comorbid depression. J. Headache Pain 19:48. doi: 10.1186/s10194-018-0876-9
Manta, S., Dong, J., Debonnel, G., and Blier, P. (2009). Enhancement of the function of rat serotonin and norepinephrine neurons by sustained vagus nerve stimulation. J. Psychiatry Neurosci. 34, 272–280.
Manta, S., El Mansari, M., Debonnel, G., and Blier, P. (2012). Electrophysiological and neurochemical effects of long-term vagus nerve stimulation on the rat monoaminergic systems. Int. J. Neuropsychopharmacol. 16, 459–470. doi: 10.1017/S1461145712000387
Mokhtari, T., Tu, Y., and Hu, L. (2019). Involvement of the hippocampus in chronic pain and depression. Brain Sci. Adv. 5, 288–298. doi: 10.26599/BSA.2019.9050025
Obata, H. (2017). Analgesic mechanisms of antidepressants for neuropathic pain. Int. J. Mol. Sci. 18:2483. doi: 10.3390/ijms18112483
Ossipov, M. H., Morimura, K., and Porreca, F. (2014). Descending pain modulation and chronification of pain. Curr. Opin. Support. Palliat. Care 8, 143–151. doi: 10.1097/SPC.0000000000000055
Pollak Dorocic, I., Fürth, D., Xuan, Y., Johansson, Y., Pozzi, L., Silberberg, G., et al. (2014). A whole-brain atlas of inputs to serotonergic neurons of the dorsal and median raphe nuclei. Neuron 83, 663–678. doi: 10.1016/j.neuron.2014.07.002
Priovoulos, N., Poser, B. A., Ivanov, D., Verhey, F. R. J., and Jacobs, H. I. L. (2019). In vivo imaging of the nucleus of the solitary tract with magnetization transfer at 7 tesla. NeuroImage 201:116071. doi: 10.1016/j.neuroimage.2019.116071
Ren, J., Friedmann, D., Xiong, J., Liu, C. D., Ferguson, B. R., Weerakkody, T., et al. (2018). Anatomically defined and functionally distinct dorsal raphe serotonin sub-systems. Cells 175, 472–487.e20. doi: 10.1016/j.cell.2018.07.043
Sacca, V., Zhang, Y., Cao, J., Li, H., Yan, Z., Ye, Y., et al. (2022). Evaluation of the modulation effects evoked by different transcutaneous auricular Vagus nerve stimulation frequencies along the central Vagus nerve pathway in migraines: a functional magnetic resonance imaging study. Neuromodulation: journal of the international Neuromodulation. Society 26, 620–628. doi: 10.1016/j.neurom.2022.08.459
Sagheddu, C., Aroni, S., De Felice, M., Lecca, S., Luchicchi, A., Melis, M., et al. (2015). Enhanced serotonin and mesolimbic dopamine transmissions in a rat model of neuropathic pain. Neuropharmacology 97, 383–393. doi: 10.1016/j.neuropharm.2015.06.003
Saper, C. B. (2011). Diffuse cortical projection systems: anatomical organization and role in cortical function. Compr. Physiol. 1, 169–210.
Schwedt, T. J. (2013). Multisensory integration in migraine. Curr. Opin. Neurol. 26, 248–253. doi: 10.1097/WCO.0b013e328360edb1
Schwedt, T. J., Chong, C. D., Chiang, C. C., Baxter, L., Schlaggar, B. L., and Dodick, D. W. (2014). Enhanced pain-induced activity of pain-processing regions in a case-control study of episodic migraine. Cephalalgia 34, 947–958. doi: 10.1177/0333102414526069
Sclocco, R., Garcia, R. G., Kettner, N. W., Fisher, H. P., Isenburg, K., Makarovsky, M., et al. (2020). Stimulus frequency modulates brainstem response to respiratory-gated transcutaneous auricular vagus nerve stimulation. Brain Stimul. 13, 970–978. doi: 10.1016/j.brs.2020.03.011
Sclocco, R., Garcia, R. G., Kettner, N. W., Isenburg, K., Fisher, H. P., Hubbard, C. S., et al. (2019). The influence of respiration on brainstem and cardiovagal response to auricular vagus nerve stimulation: a multimodal ultrahigh-field (7T) fMRI study. Brain Stimul. 12, 911–921. doi: 10.1016/j.brs.2019.02.003
Scott, D. J., Heitzeg, M. M., Koeppe, R. A., Stohler, C. S., and Zubieta, J. K. (2006). Variations in the human pain stress experience mediated by ventral and dorsal basal ganglia dopamine activity. J. Neurosci. 26, 10789–10795. doi: 10.1523/JNEUROSCI.2577-06.2006
Silberstein, S. D., Yuan, H., Najib, U., Ailani, J., Morais, A. L., Mathew, P. G., et al. (2020). Non-invasive vagus nerve stimulation for primary headache: a clinical update. Cephalalgia 40, 1370–1384. doi: 10.1177/0333102420941864
Soheili-Nezhad, S., Sedghi, A., Schweser, F., Eslami Shahr Babaki, A., Jahanshad, N., Thompson, P. M., et al. (2019). Structural and functional reorganization of the brain in migraine without Aura. Front. Neurol. 10:442. doi: 10.3389/fneur.2019.00442
Starr, C. J., Sawaki, L., Wittenberg, G. F., Burdette, J. H., Oshiro, Y., Quevedo, A. S., et al. (2011). The contribution of the putamen to sensory aspects of pain: insights from structural connectivity and brain lesions. Brain 134, 1987–2004. doi: 10.1093/brain/awr117
Straube, A., Ellrich, J., Eren, O., Blum, B., and Ruscheweyh, R. (2015). Treatment of chronic migraine with transcutaneous stimulation of the auricular branch of the vagal nerve (auricular t-VNS): a randomized, monocentric clinical trial. J. Headache Pain 16:543. doi: 10.1186/s10194-015-0543-3
Tao, H., Wang, T., Dong, X., Guo, Q., Xu, H., and Wan, Q. (2018). Effectiveness of transcutaneous electrical nerve stimulation for the treatment of migraine: a meta-analysis of randomized controlled trials. J. Headache Pain 19:42. doi: 10.1186/s10194-018-0868-9
Taylor, B. K., and Westlund, K. N. (2017). The noradrenergic locus coeruleus as a chronic pain generator. J. Neurosci. Res. 95, 1336–1346. doi: 10.1002/jnr.23956
Tu, Y., Fu, Z., Zeng, F., Maleki, N., Lan, L., Li, Z., et al. (2019). Abnormal thalamocortical network dynamics in migraine. Neurology 92, e2706–e2716. doi: 10.1212/WNL.0000000000007607
Tu, Y., Jung, M., Gollub, R. L., Napadow, V., Gerber, J., Ortiz, A., et al. (2019). Abnormal medial prefrontal cortex functional connectivity and its association with clinical symptoms in chronic low back pain. Pain 160, 1308–1318. doi: 10.1097/j.pain.0000000000001507
Varela, C. (2014). Thalamic neuromodulation and its implications for executive networks. Front Neural Circuits 8:69. doi: 10.3389/fncir.2014.00069
Vazey, E. M., Moorman, D. E., and Aston-Jones, G. (2018). Phasic locus coeruleus activity regulates cortical encoding of salience information. Proc. Natl. Acad. Sci. 115, E9439–E9448. doi: 10.1073/pnas.1803716115
Westlund, K. N., Zhang, D., Carlton, S. M., Sorkin, L. S., and Willis, W. D. (1991). “Chapter 5 - noradrenergic innervation of somatosensory thalamus and spinal cord” in Progress in brain research. eds. C. D. Barnes and O. Pompeiano, vol. 88 (Radarweg, Amsterdam: Elsevier), 77–88.
Whitfield-Gabrieli, S., and Nieto-Castanon, A. (2012). Conn: a functional connectivity toolbox for correlated and Anticorrelated brain networks. Brain Connect. 2, 125–141. doi: 10.1089/brain.2012.0073
Yakunina, N., Kim, S. S., and Nam, E. C. (2017). Optimization of transcutaneous Vagus nerve stimulation using functional MRI. Neuromodulation 20, 290–300. doi: 10.1111/ner.12541
Yakunina, N., Kim, S. S., and Nam, E. C. (2018). BOLD fMRI effects of transcutaneous vagus nerve stimulation in patients with chronic tinnitus. PLoS One 13:e0207281. doi: 10.1371/journal.pone.0207281
Yu, S., Li, W., Shen, W., Edwards, R. R., Gollub, R. L., Wilson, G., et al. (2020). Impaired mesocorticolimbic connectivity underlies increased pain sensitivity in chronic low back pain. NeuroImage 218:116969. doi: 10.1016/j.neuroimage.2020.116969
Yuan, H., and Silberstein, S. D. (2016). Vagus nerve and Vagus nerve stimulation, a comprehensive review: part I. Headache 56, 71–78. doi: 10.1111/head.12647
Zhang, Y., Huang, Y., Li, H., Yan, Z., Zhang, Y., Liu, X., et al. (2021). Transcutaneous auricular vagus nerve stimulation (taVNS) for migraine: an fMRI study. Reg. Anesth. Pain Med. 46, 145–150. doi: 10.1136/rapm-2020-102088
Zhang, B., Jung, M., Tu, Y., Gollub, R., Lang, C., Ortiz, A., et al. (2019). Identifying brain regions associated with the neuropathology of chronic low back pain: a resting-state amplitude of low-frequency fluctuation study. Br. J. Anaesth. 123, e303–e311. doi: 10.1016/j.bja.2019.02.021
Zhang, Y., Liu, J., Li, H., Yan, Z., Liu, X., Cao, J., et al. (2019). Transcutaneous auricular vagus nerve stimulation at 1 Hz modulates locus coeruleus activity and resting state functional connectivity in patients with migraine: an fMRI study. NeuroImage Clin. 24:101971. doi: 10.1016/j.nicl.2019.101971
Keywords: taVNS, migraine, serotonergic, noradrenergic, NTS
Citation: Huang Y, Zhang Y, Hodges S, Li H, Yan Z, Liu X, Hou X, Chen W, Chai-Zhang T, Kong J and Liu B (2023) The modulation effects of repeated transcutaneous auricular vagus nerve stimulation on the functional connectivity of key brainstem regions along the vagus nerve pathway in migraine patients. Front. Mol. Neurosci. 16:1160006. doi: 10.3389/fnmol.2023.1160006
Edited by:
Ningbo Xu, Southern Medical University, ChinaReviewed by:
Ling Zhao, Chengdu University of Traditional Chinese Medicine, ChinaArtem Trufanov, Kirov Military Medical Academy, Russia
Copyright © 2023 Huang, Zhang, Hodges, Li, Yan, Liu, Hou, Chen, Chai-Zhang, Kong and Liu. This is an open-access article distributed under the terms of the Creative Commons Attribution License (CC BY). The use, distribution or reproduction in other forums is permitted, provided the original author(s) and the copyright owner(s) are credited and that the original publication in this journal is cited, in accordance with accepted academic practice. No use, distribution or reproduction is permitted which does not comply with these terms.
*Correspondence: Jian Kong, amtvbmcyQG1naC5oYXJ2YXJkLmVkdQ==; Bo Liu, bGl1Ym9nemNtQDE2My5jb20=
†These authors have contributed equally to this work