- 1College of Forensic Medicine, Xi’an Jiaotong University Health Science Center, Xi’an, Shaanxi, China
- 2Institute of Forensic Injury, Institute of Forensic Bio-Evidence, Western China Science and Technology Innovation Harbor, Xi’an Jiaotong University, Xi’an, Shaanxi, China
- 3State Key Laboratory of Oncogenomics, School of Chemical Biology and Biotechnology, Peking University Shenzhen Graduate School, Shenzhen, China
- 4Shenzhen Bay Laboratory, Institute of Chemical Biology, Shenzhen, China
- 5NHC Key Laboratory of Forensic Science, College of Forensic Medicine, Xi’an Jiaotong University, Xi’an, Shaanxi, China
- 6Department of Psychiatry, University of Toronto, Toronto, ON, Canada
Background: Despite its role in inflammation and the redox system under hypoxia, the effects and molecular mechanisms of hypoxia-inducible factor (HIF) in neuroinflammation-associated depression are poorly explored. Furthermore, Prolyl hydroxylase domain-containing proteins (PHDs) regulate HIF-1; however, whether and how PHDs regulate depressive-like behaviors under Lipopolysaccharides (LPS)-induced stress conditions remain covered.
Methods: To highlight the roles and underlying mechanisms of PHDs-HIF-1 in depression, we employed behavioral, pharmacological, and biochemical analyses using the LPS-induced depression model.
Results: Lipopolysaccharides treatment induced depressive-like behaviors, as we found, increased immobility and decreased sucrose preference in the mice. Concurrently, we examined increased cytokine levels, HIF-1 expression, mRNA levels of PHD1/PHD2, and neuroinflammation upon LPS administration, which Roxadustat reduced. Furthermore, the PI3K inhibitor wortmannin reversed Roxadustat-induced changes. Additionally, Roxadustat treatment attenuated LPS-induced synaptic impairment and improved spine numbers, ameliorated by wortmannin.
Conclusion: Lipopolysaccharides-dysregulates HIF-PHDs signaling may contribute to neuroinflammation-coincides depression via PI3K signaling.
Introduction
Major depression disorder (MDD) is a global concern with increasing prevalence, expected to be a single leading cause among all disease burdens by 2030. Clinically, MMD is characterized by a lack of energy, persistent low mood, despairs, sleep disorders, and in severe cases, suicidal behaviors (Athira et al., 2020; Gutiérrez-Rojas et al., 2020; Abdoli et al., 2022). Unfortunately, due to its complexity and heterogeneity as determined by genetic and environmental factors, the molecular mechanisms of MDD are enigmatic (Athira et al., 2020). The current hypothesis of depression focuses on the hypothalamus-pituitary–adrenal (HPA) axis, neuroplasticity, and monoamine neurotransmitter depletion (Brigitta, 2002; Hasler, 2010). However, the delayed effect of available antidepressants and lack of focus on cells other than neurons demonstrate the limitation of these specific pathological mechanisms (Andrade and Rao, 2010; Malhi et al., 2020). Thus, around one-third of MDD patients do not respond well to the existing treatments that demand the revitalization of psychiatric therapeutics with novel intervention options that engage non-monoaminergic molecular targets. In recent years, the inflammatory hypothesis has been proposed for depressive symptoms (Madeeh Hashmi et al., 2013; Gałecki and Talarowska, 2018) as an activated immune system has been founded in MMD patients (Cassano et al., 2017; Zou et al., 2018), which opened new avenues for depression investigation. We reported previously that lipopolysaccharides (LPS) could induce neuroinflammation and depressive-like behavior in mice. Besides, it modulated inflammatory signaling molecules, including NLRP3, NF-кB, p-p38, etc., accompanied by depressive symptoms such as immobility, decreased sucrose preferences in the mice, and synaptic defects (Ali et al., 2020b; Li et al., 2021b).
Mechanistically, LPS administration dysregulates PI3K/Akt/NF-kB signaling, which may lead to neuroinflammation. Concurrently, the altered neuroinflammatory response can coincide with synaptic defect via different signaling, including HDAC1 and Sirt3/HO-1 signaling (Li et al., 2019; Zhao et al., 2019; Ali et al., 2020a; Jamali-Raeufy et al., 2021; Li et al., 2021b). Similarly, LPS can initiate the transcription factor HIF-1 signaling, which may be mediated through the NF-kB signaling (Frede et al., 2006; Palladino et al., 2018), as NF-kB has a binding site on the promoter of the HIF-1 gene as a transcription factor (van Uden et al., 2008). However, it has also been reported that hypoxia-activated HIF-1 is synergistic with LPS in macrophages (Mi et al., 2008). Similarly, PI3K signaling could contribute to the translation of HIF-1 via mTOR (Xu et al., 2020), while its role in neuroinflammation and synaptic defects is not highlighted yet.
Roxadustat (FG-4592) is the reversible inhibitor of hypoxia-inducible factor prolyl hydroxylase (PHDs), a hypoxia-inducible factor (HIF-1) stabilizer, orally available and approved by the FDA. Initially, this drug was approved for treating anemia, including in China. FG-4592 showed considerable protection against other hypoxia-related disorders, including cancer, fibrosis, and chronic inflammation. Besides, in the sepsis animal model, Roxadustat-treatment significantly reduced the cytokines, including IL-1β, IL-6, and TNF-α (Akizawa et al., 2019; Liu et al., 2021; Zhu et al., 2022). Furthermore, prolyl hydroxylases (PHDs) regulate HIF-1 under different conditions (Appelhoff et al., 2004), whose underlying mechanisms have not been explored in LPS-induced stress conditions. Similarly, the HIF-1 anti-inflammatory consensus has not been currently elucidated (Zhu et al., 2022). Therefore, we aimed to determine whether LPS-altered HIF-1 signaling can affect depressive symptoms coinciding with neuroinflammation and synaptic defects. Surprisingly, our data showed that LPS lead to HIF-1 signaling impairment, neuroinflammation, synaptic defects, spine number modification, and depressive symptom, which PI3K-signaling could alter.
Materials and methods
Animals
C57BL/6 J male mice (6–8 weeks) were obtained from the Guangdong Medical Laboratory Animal Centre, China. The experimental animals were housed at the Laboratory Animal Research Centre, Peking University Shenzhen Graduate School, under a 12 h light/12 h dark cycle at 18–22°C, ad libitum access to food and water throughout the study. The experimental procedures were set in such a way as to minimize mice suffering. The Animal Care and Use Committee of the Experimental Animal Center at Peking University, Shenzhen Graduate School, approved the animal experiments.
Lipopolysaccharides-induced depression is associated with inflammation (Ali et al., 2020a), and FG-4592 has shown anti-inflammatory action by reducing cytokine levels in the animal model (Akizawa et al., 2019; Liu et al., 2021; Zhu et al., 2022). Besides, the HIF-1 anti-inflammatory mechanism is largely unknown. Therefore, this was one reason for HIF-1 stabilizer selection for the LPS-induced neuroinflammation-coinciding depression. The rationale for the FG-4592 treatment for the depression model is unavailable; thus, we initially checked the dose-dependent action of the FG-4592 while performing OFT and SPT tests (Supplementary Figure S1A).
We performed the present study in two experiments. In the first experiment, animals were assigned to three groups (each group = 6–10): saline-treated group (Saline), LPS-treated group (1.5 mg/kg/day), Roxadustat: FG-4592 (Roxa; 5 mg/kg/day) plus LPS group (Roxa+LPS). LPS (0.1 ml/10 g mice) and Roxadustat (0.1 ml/10 g mice, were intraperitoneally administered to the mice. Besides, Roxadustat was treated 1 h before LPS administration. The drug treatment schedule is shown in Figure 1A. After 24 h of the final LPS administration, behavioral tests were performed. Finally, the mice were sacrificed, and tissues were collected and quickly stored at-80°C until further use. Notably, LPS (Sigma-Aldrich, #L2880) was dissolved with sterile water directly to its working concentration. Roxadustat was dissolved in 5% dimethyl-sulfoxide (DMSO) and was administrated 2 h before the behavior test.
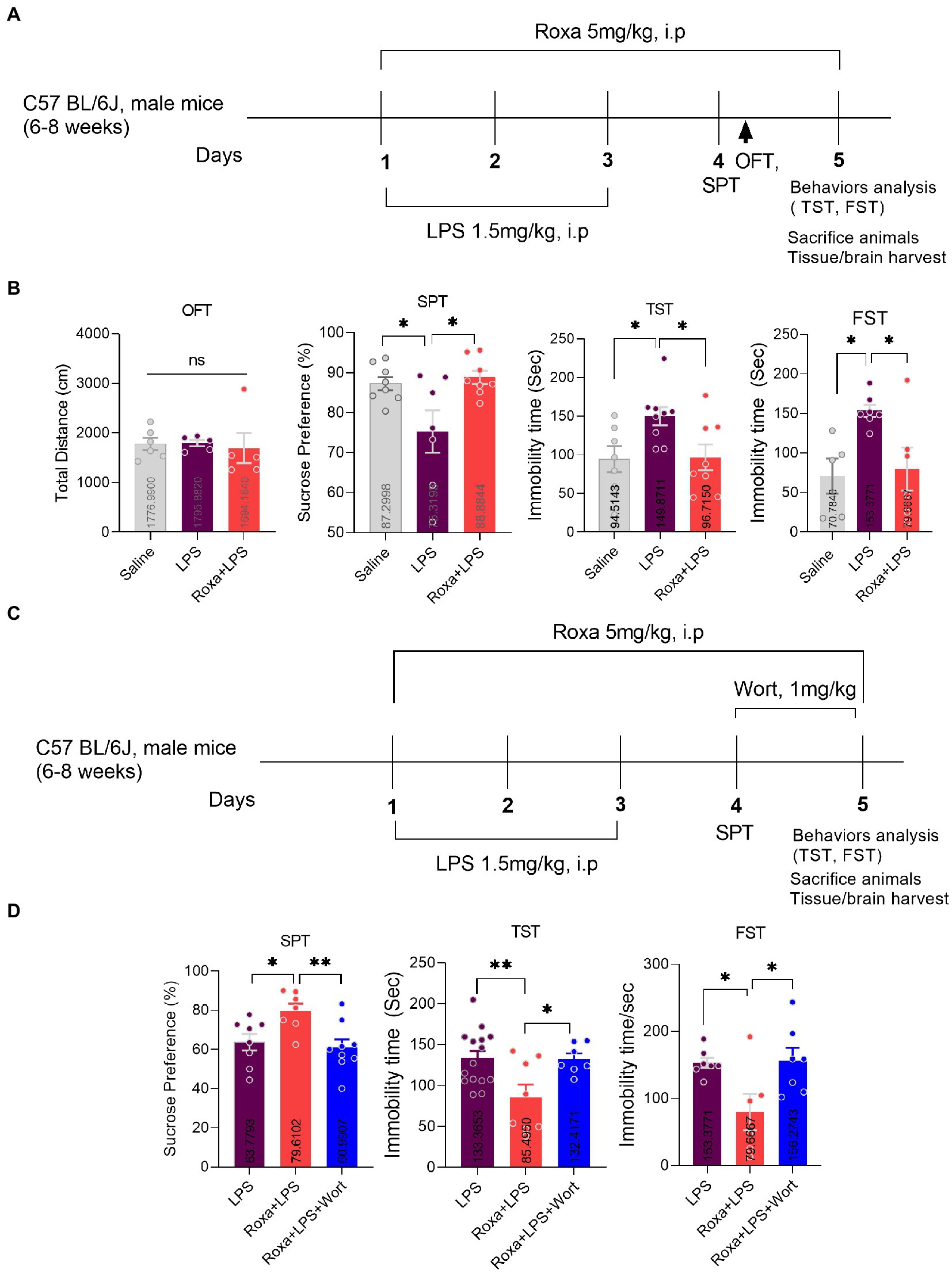
Figure 1. Roxadustat treatment reduced LPS-induced depressive-like behaviors via PI3K signaling. (A) Drugs treatment schedule, (B) open field test (OFT), forced swimming test (FST), and a sucrose preference test (SPT). (C) Drugs treatment schedule, (D) Sucrose preference Test (SPT), and tail suspension Test (TST). All the values are expressed as mean ± SEM: ANOVA followed by post hoc analysis. p = <0.05 were considered significant *p < 0.05, **p < 0.01. ns: non-significant.
In the second experiment, animals were again divided into three groups (8–15 mice/group): LPS group, Roxa+LPS group, and Roxa+LPS + Wort group (Wortmannin,1 mg/kg). Wortmannin (wort) was administered intraperitoneally for 2 days, before 2 h behavior tests (Figure 1C). Wortmannin was dissolved in 5% DMSO. The behavior and organ/tissues collection process were the same as above.
Open field test
Open field test (OFT) was performed according to previous protocols (Ali et al., 2020b). Briefly, mice were adapted to the experimental room for 1 h and then placed in a 45 × 45 × 30 cm chamber. Each mouse was started in the center of the chamber and was allowed to move freely for 5 min. The total distance traveled was recorded and analyzed using Smart v3.0 software.
Sucrose preference test
A sucrose preference test was performed using a two-bottle free-choice paradigm (Liu et al., 2018). Initially, the mice were habituated for 48 h hrs with two drinking bottles (one containing 1% sucrose and the other water) in their home cage. Next, on the third day of the drug administration, the mice were deprived of water and food for 24 h. The next day, each mouse had free access to two bottles of either 1% sucrose solution or standard drinking water. The positions of the bottles were switched halfway through testing to prevent the possible effects of side preference on drinking behavior. Percentage preference for sucrose was calculated at the end of the test using the following formula: Sucrose Preference = Sucrose consumption/ (Sucrose consumption + Water consumption) × 100%.
Tail suspension test
The tail suspension test was performed as described previously (Li et al., 2022). Mice were suspended by the tails to a rod about 45 cm above the floor by placing adhesive tape 1 cm from the tail tip. By the commonly accepted criteria, immobility was defined as the absence of movement of the animals’ heads and bodies. The immobility time was recorded for 6 min in a dark room. Etho Vision XT software was used for TST recording and analysis.
Forced swimming test
The forced swimming test (FST) was performed as described previously. Animals were forced to swim in a Plexiglas cylinder (height: 70 cm, diameter: 30 cm) filled with water (23 ± 1°C) to a height of 30 cm. The video was recorded for 6 min, and the immobility time was analyzed during the last 5 min of the test. Mice were immobile when they remained motionless or only made movements necessary to keep their heads above the water’s surface.
ELISA
According to the manufacturer’s protocols, cytokines expression was quantified using ELISA kits (Elabscience). Briefly, a 100 μL standard or sample was added to the wells and incubated for 90 min at 37°C. The plates were then washed, and a Biotinylated Detection Ab working solution was added to each well. The plates were incubated for 1 h at 37°C. Next, 100ul HRP conjugate working solution was added for 30 min at 37°C. Finally, the reaction was stopped, and the optical density was measured.
Immunofluorescence
Immunofluorescence staining was performed according to described previously (Li et al., 2022). Briefly, mice were perfusion-fixed with 4% paraformaldehyde and soaked in PFA for 48 h, then brian was replaced with sucrose, and 30um brain tissue sections were prepared. Sections were washed with PBS for 15 min (5 min × 3). After washing, the sections were blocked with blocking buffer (10% normal goat serum in 0.3% Triton X-100 in PBS) for 1 h at room temperature and then incubated with primary antibodies overnight at 4°C, Rabbit anti-Iba1 (1: 1000, Wako,#019–19,741); Mouse anti-GFAP (1,1,000, Sigma-Aldrich, #MAB360). The next day, sections were incubated with secondary antibodies (Alexa Flour secondary antibodies, Thermo Fisher) at room temperature for 1 h; the hoechst was applied in the last 10 min. The sections were washed with PBS for 15 min (5 min × 3). After washing, sections were transferred onto glass slides, and glass coverslips were mounted using a mounting medium. Images were captured using a confocal microscope and were analyzed by ImageJ software.
Golgi staining
Golgi staining was performed according to Sami Zaqout’s protocols (Li et al., 2021a). Impregnation step: the mice brain sample was kept in Golgi-Cox solution at room temperature in the dark; after 24 h, the sample was transferred into a new Golgi solution-containing bottle with the help of a histological cassette and kept settling at room temperature in the dark for 7–10 days. Tissue protection step: the brain sample is transferred from the Golgi-Cox impregnation solution to a new bottle with the tissue-protectant solution and kept at 4°C in the dark. After 24 h, the tissue-protectant solution is replaced by a new solution in a new bottle for 4–7 days. Sectioning step: the brain sample is embedded in 4% low melting point agarose. 150–200 um sections were prepared using a sliding microtome and mounted to gelatin-coated microscope slides. Then, the brain tissue was placed in a staining solution for 10 min and rinsed with double distilled water, followed by dehydration (sequential rinse 50,75, and 95% ethanol) and xylene treatment finally, examined under an inverted fluorescence microscope IX73 Olympus.
Real-time quantitative RT-PCR
Total RNA was isolated from the hippocampus with Trizol (Invitrogen, Germany) and reversed to cDNA using the Reverse Transcription System (Promega). Primers ordered with the following sequences: HIF-1, 5′-GAAACGACCACTGCTAAGGCA-3′(forward) and 5′-GGCAGACAGCTTAAGGCTCCT-3′(reverse); prolyl hydroxylase (PHD)1, 5′-GGCCAGTGGTAGCCAACATC-3′(forward) and 5′-GTGGCATAGGCTGGCTTCAC-3′(reverse); PHD2, 5′- TGACCACACCTCTCCAGCAA-3′ (forward) and 5′- CTGCCAACAATGCCAAACAG-3′ (reverse); and PHD3, 5′-GGTGGCTTGCTATCCAGGAA-3′(forward) and 5′-ATACAGCGGCCATCACCATT-3′(reverse).
Western blotting
Western blotting was also performed according to the standard protocol. Briefly, the protein sample was denatured by boiling at 95°C for 5 min and separated via SDS-PAGE. The separated protein was then transferred onto a nitrocellulose membrane. The membrane was blocked with non-fat milk in TBST (Tris-buffered saline, 0.1% Tween 20) and then incubated with primary antibody (1:1000) (Table 1) overnight at 4°C. The next day, the membranes were incubated with a secondary antibody (1:10000) for 1 h at room temperature. For detection, the ECL Super signal chemiluminescence kit was used according to the manufacturer’s protocol. Blots were developed using ChemiDoc MP BIO-RAD. Densitometric analysis of the bands was performed using the Image Lab software. The stripping buffer was purchased from Thermo Scientific (LOT: WE32238 Scientific) blot stripping. Briefly, blots were washed with TBST 3 times. Immerse blot in stripping buffer, followed by incubating for 15-30 min at room temperature. Finally, removed the stripping buffer by washing it with TBST three times, followed by re-blocking the membrane for 1 h.
Statistical analysis
All the statistical analyses were performed using the GraphPad Prism 8 software. Data are presented as mean ± SEM. One-way analysis of variance (ANOVA) followed by post hoc Tukey/Bonferroni Multiple Comparison tests was performed to compare different groups. p < 0.05 was regarded as statistically significant.
Results
Roxadustat (FG-4592) reversed lipopolysaccharides-induced depressive symptoms
Lipopolysaccharides is a well-known inflammatory agent that can induce depressive-like behaviors in mice (Ali et al., 2020a). Similarly, our LPS-treated mice displayed depressive symptoms, as demonstrated by increased immobility during TST and FST, while decreased sucrose preference for 1% sucrose solution over normal water. However, Roxadustat (FG-4592) treatment significantly attenuated these LPS-induced depressive-like behaviors (Figures 1A,B).
PI3K Signaling mediated the effects of Roxadustat
Previous studies evidenced LPS-altered PI3K signaling (Saponaro et al., 2012; Zheng et al., 2018). However, its association with HIF-1 is enigmatic. Herein, we treated mice with wortmannin to determine the interplay among LPS, HIF-1, and PI3K signaling in depression. Surprisingly, PI3K antagonism significantly reversed Roxadustat anti-depressive effects in the presence of LPS, as it found that increased mice immobility decreased sucrose preference (Figures 1C,D). These findings suggested the involvement of HIF-1 and PI3K signaling in LPS-induced depressive symptoms. Moreover, we found an increase in HIF-1 expression, PHD1,2 (mRNA), and PI3K phosphorylation upon LPS administration, which was reduced by Roxadustat treatment (Figure 2; Supplementary Figures S1B,C). As the PI3K-Akt signaling could be activated by LPS stimulus (Hemmings and Restuccia, 2012), we examined Akt and its downstream signaling changes in the hippocampus of LPS-treated mice. Notably, LPS administration significantly enhanced Akt/GSK3β phosphorylation which was reduced by Roxadustat treatment.
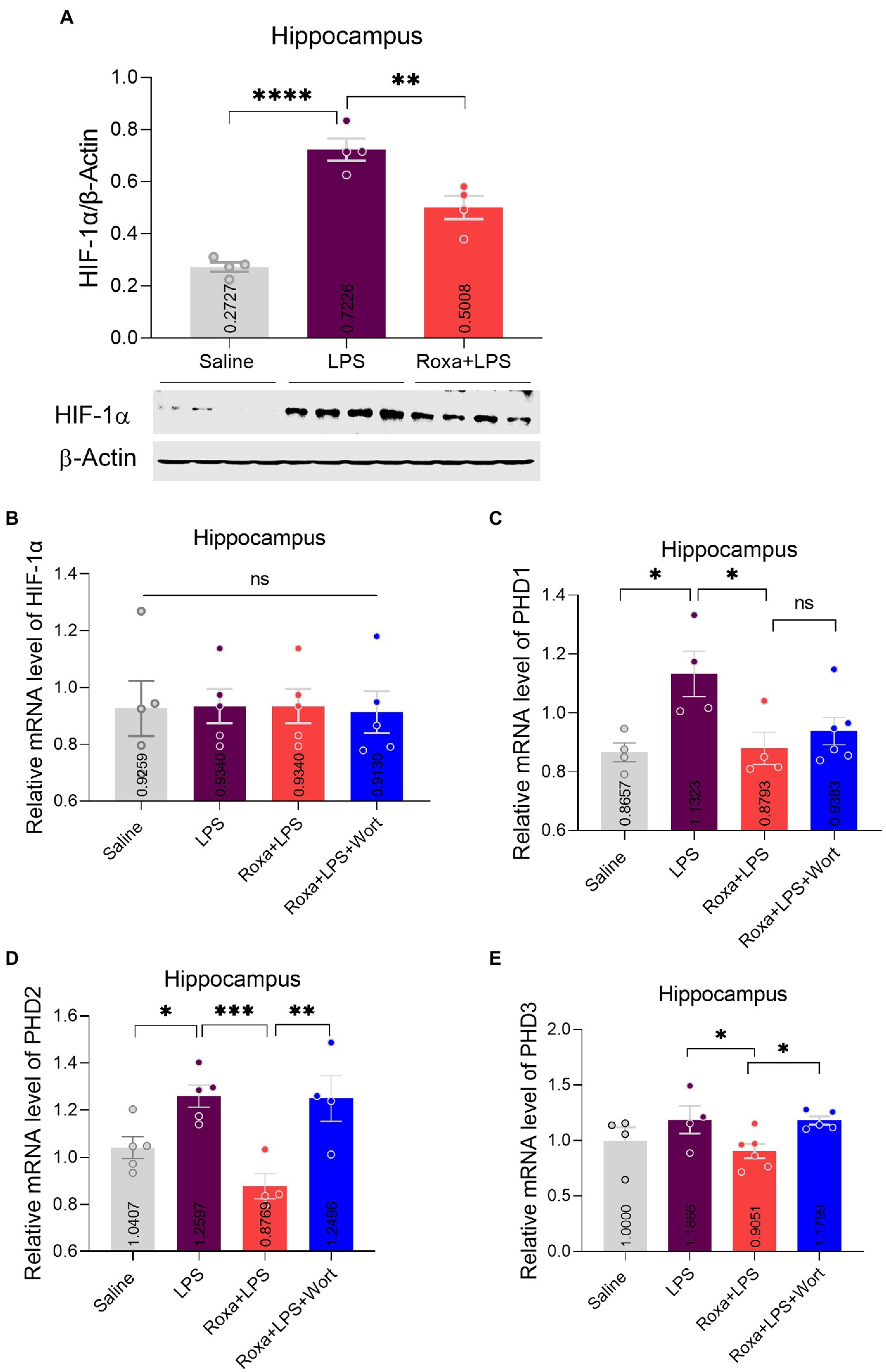
Figure 2. Roxadustat effects on HIF-1a and PHDs expression. (A) Representative immune blot images and average protein levels of HIF-1 levels were normalized with β-Actin. (B–E) Showing mRNA levels of HIF-1, PHD1, PHD2, and PHD3. Image lab software was used for quantitative blot analysis and was analyzed via GraphPad prism. Data were expressed as mean ± SEM, One-way ANOVA, followed by post hoc analysis. p = <0.05 were considered significant. *p < 0.05, **p < 0.01, ***p < 0.001, ****p < 0.0001. ns: non-significant.
Contrarily, Roxadustat treatment significantly increased AMPKα phosphorylation in the presence of LPS (Figure 3A), indicating a link between HIF-1 and AMPK singling under LPS-induced stress conditions. As reported, AMPK is involved in the Akt signaling activation (Han et al., 2018); its link to PI3K is largely unknown, particularly in LPS-initiated stress conditions. Thus, we examined AMPKα/Akt/GSK3b signaling changes upon PI3K antagonism in the presence of LPS. Except for Akt, wortmannin treatment significantly attenuated Roxadustat-induced p-AMPKα, GSK3b, and p-p38 expressional changes in the mice hippocampal tissues in the presence of LPS (Figure 3B). These findings indicated the interplay among AMPK/PI3K and HIF-1 signaling under LPS-induced stress conditions.
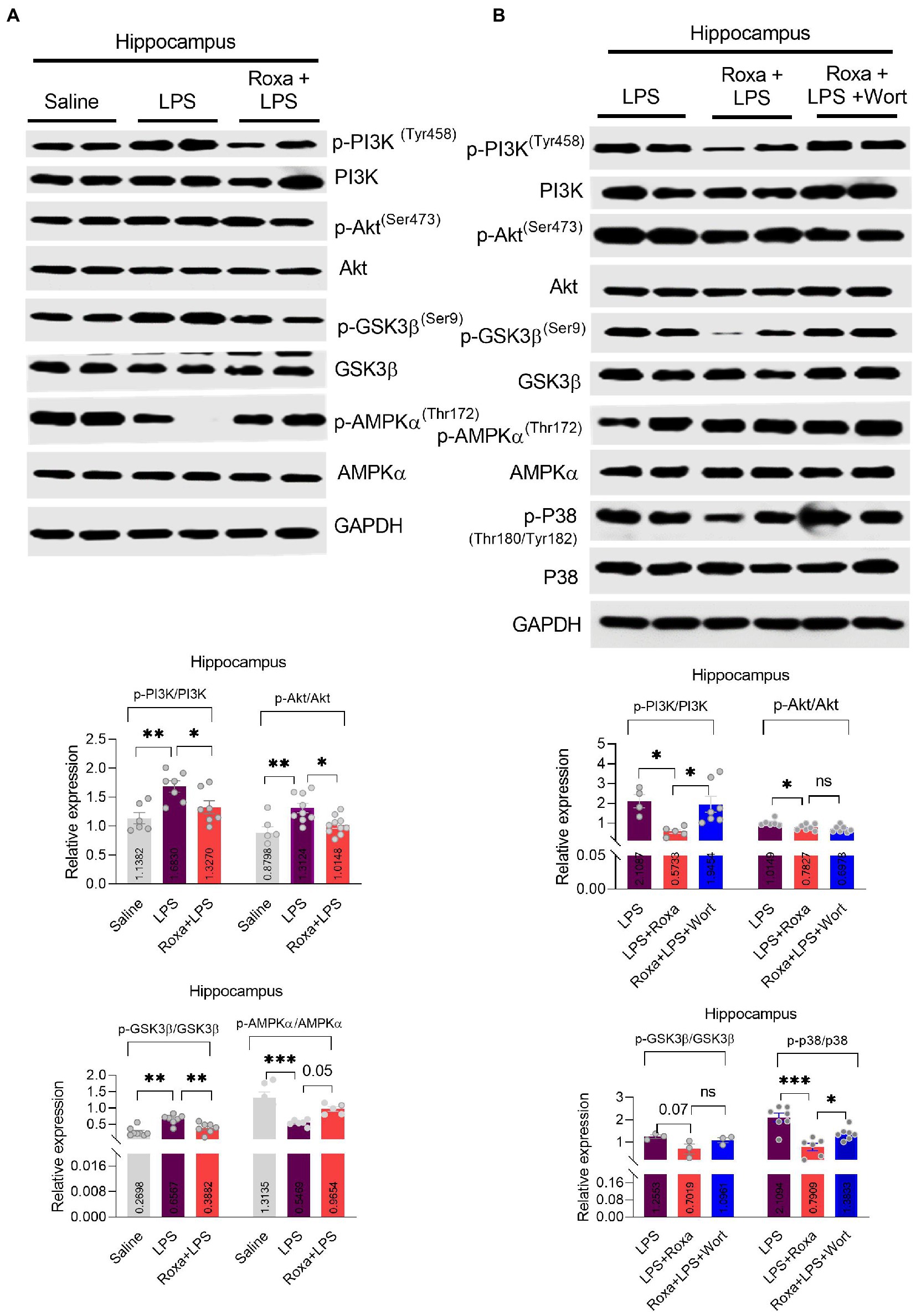
Figure 3. Roxadustat reduced LPS-induced PI3K and its associated signaling. (A,B) representative western blots of PI3K, Akt, GSK3β, APMKα, and P38 and quantitative column graphs for mice treated with LPS, Roxadustat and wortmannin. All the values were normalized with GAPDH. Image lab software was used for blots quantitative analysis and was analyzed via GraphPad prism. Data were expressed as mean ± SEM, One-way ANOVA, followed by post hoc analysis. p = <0.05 were considered significant. *p < 0.05, **p < 0.01), ***p < 0.001. ns: non-significant.
Roxadustat treatment attenuated lipopolysaccharides-induced neuroinflammation
Lipopolysaccharides-induced neuroinflammation played an integral role of depressive symptoms in mice (Li et al., 2017, 2021a). Here, we sought to determine whether LPS-induced neuroinflammation is linked to HIF-1 signaling. Our results indicated that Roxadustat significantly reduced IBA-1 and GFAP expression in the hippocampus (DG region) of the LPS-treated mice (Figures 4A,B). Similarly, Roxadustat treatment decreased cytokines, including IL-1b, IL-6, and TNF-a levels in the hippocampal tissue of the brain (Figure 4D). However, the anti-inflammatory effects of Roxadustat could be attenuated by wortmannin treatment (Figures 4A,C,D), suggesting PI3K signaling was involved in HIF-1 modulation of LPS-induced neuroinflammation. The cellular signaling changes associated with neuroinflammation were examined to validate these results further. Surprisingly, Roxadustat treatment significantly reduced LPS-elevated expression of NLRP3 in mice brains (Figure 5A), which could be increased by wortmannin (Figure 5B). As LPS-induced inflammation accompanies oxidative stress, we thus measured the expressions of anti-oxidative markers, including Nrf2, SOD2, and HO-1. However, no significant changes in NRF2, SOD2, and HO-1 Roxadustat and Wortmannin treatment could be defined (Figures 5A,B).
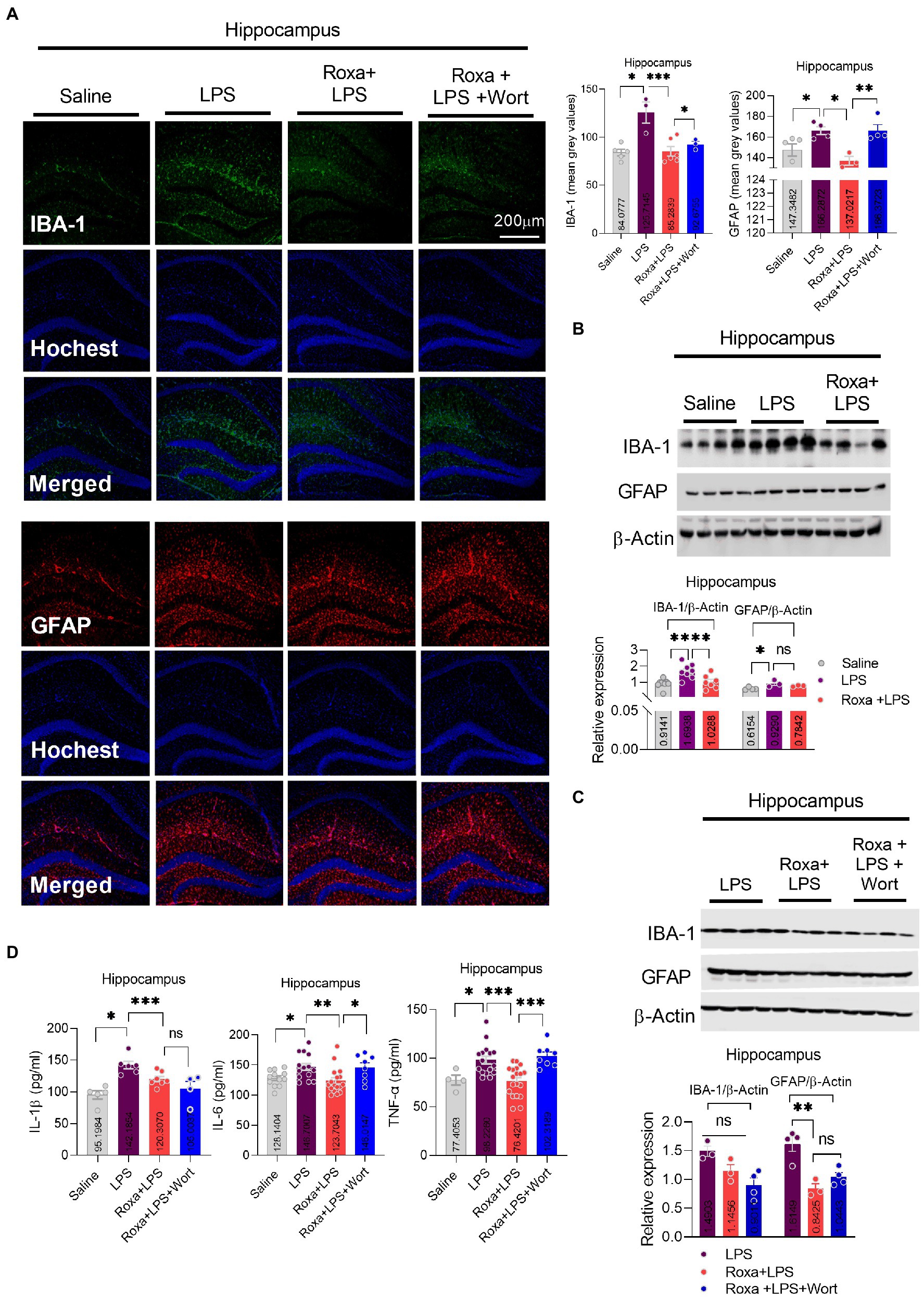
Figure 4. Roxadustat reduced LPS-induced neuroinflammation. (A) Microscopy results of Iba-1 expression in the different experimental groups of brain tissues, with respective bar graphs (n = 6). Magnification 10×. The image data were collected from three independent experiments and were analyzed by ImageJ software. The differences are shown in the graphs. (B,C) Representative immune blots with individual level column graphs showing Iba-1 and GFAP expression n = 3–6. All the values were normalized with β-Actin. (D) Bar graphs showing the expression level of IL-1β, IL-6, and TNF-α. Data were expressed as mean ± SEM, One-way ANOVA, followed by post hoc analysis. p = <0.05 were considered significant. *p < 0.05, **p < 0.01, ***p < 0.001, ****p < 0.0001. ns: non-significant.
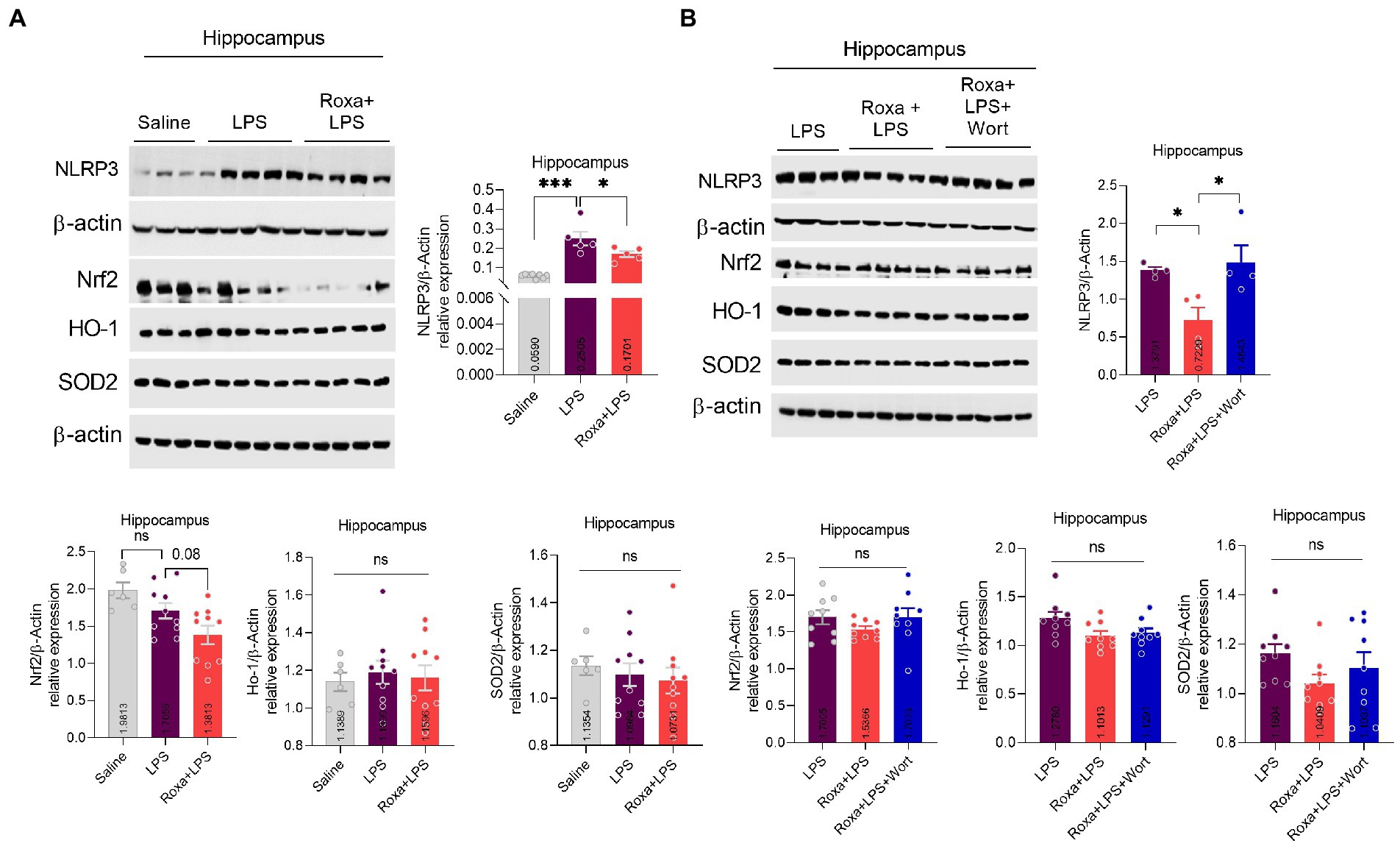
Figure 5. Roxadustat reduced NLRP3 level in the presence of LPS. (A,B) Representative immune blots images and bar graphs show the expression of NLRP3, Nrf2, HO-1, and SOD2. All the values were normalized with β-Actin. Image lab software was used for blots quantitative analysis and was analyzed via GraphPad prism. Data were expressed as mean ± SEM, One-way ANOVA, followed by post hoc analysis. p = <0.05 were considered significant, *p < 0.05, ***p < 0.001. ns: non-significant.
HIF-1-PHD antagonism by Roxadustat attenuated lipopolysaccharides-induced synaptic defects
Previous studies, including ours, showed that LPS administration could induce synaptic defects, which may underly the pathological processes of depression symptoms (Postnikova et al., 2020; Li et al., 2021a). Our results revealed dysregulated synaptic factors and reduced spins in hippocampal tissues (Figure 6C). Besides, the eEF2 phosphorylation level, which regulates synaptic plasticity, was also increased in LPS-treated mice but reversed by Roxadustat treatment (Figure 6A; Verpelli et al., 2010). Moreover, wortmannin attenuated the effects of Roxadustat and enhanced synaptic proteins, including PSD95, SNAP25, and Synapsin-1 (Figure 6B). These findings suggest that Roxadustat could alleviate dysregulated synaptic proteins induced by LPS via HIF-1/PI3K signaling.
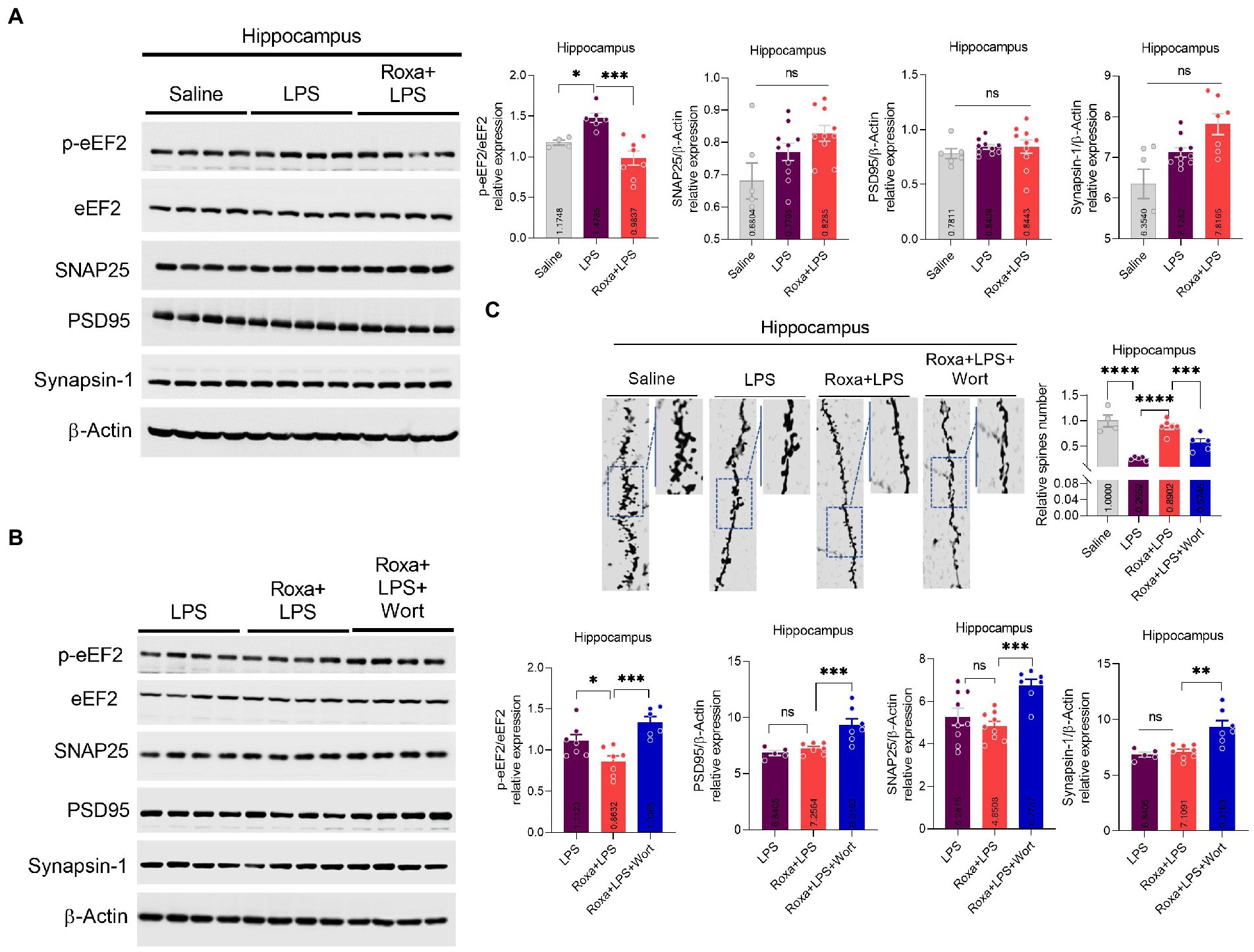
Figure 6. Changes in the expression of eEF2, SNAP25, PSD95, and Synapsin-1, and spine numbers after Roxa and LSP treatment. (A,B) Representative immune blots show the expression of p-eEF2, eEF2, SNAP25, PSD95, and Synapsin-1 levels in the hippocampus of subjects. All the values were normalized with β-Actin. (C) Golgi staining showing spine density and column graph showing spine numbers n = 3–5. Image lab software was used for blots quantitative analysis and was analyzed via GraphPad prism. Data were expressed as mean ± SEM, One-way ANOVA, followed by post hoc analysis. p = <0.05 were considered significant. *p < 0.05, **p < 0.01), ***p < 0.001, ****p < 0.0001. ns: non-significant.
Discussion
Results from our previous studies and other groups have documented the interplay between neuroinflammation and depressive-like behaviors (Li et al., 2017; Postnikova et al., 2020; Ali et al., 2020b; Li et al., 2021b), but its mechanistic link to HIF-1/PI3K signaling remains poorly explored. Here, we determined that LPS administration dysregulated HIF-1 via the PI3K pathway in the mice hippocampus, which underlined LPS elicited neuroinflammation and depressive-like behaviors. HIF-1-PHD inhibitor (Roxadustat) treatment attenuated LPS-mediated changes, which could be reversed by wortmannin, suggesting an etiological role of HIF-1 in neuroinflammation-associated depressive-like behaviors. However, conventional antidepressants show their effects slowly and may take about 2–3 weeks (Szegedi et al., 2009), immediate/quick antidepressant effects of several compounds/drugs (including Roxadustat treated here for 3 days) have also been documented in the LPS-induced model of depression (O’Connor et al., 2009; Cordeiro et al., 2019; Zhang et al., 2019).
As a transcriptional factor, HIF-1 controls the immune cell metabolism and function. Additionally, it plays a crucial role in regulating inflammatory functions in dendritic, mast, and epithelial cells (Imtiyaz and Simon, 2010). Besides, TNF-α and IL-1β can activate and increase HIF-1 expression/transcriptional via NF-kB (Jung et al., 2003; Zhou et al., 2003), indicating that HIF-1 could play an essential role in inflammation. Furthermore, it has also been reported that LPS can stimulate HIF-1 activities via several pathways, including NF-kB, ROS, and p42/p44 mitogen-activated protein kinases (MAPKs; Frede et al., 2006). In addition, in macrophages, LPS accumulates HIF-1 via decreasing PHD2 and PHD3 levels in a Toll-like receptor-4 (TLR-4) dependent manner (Peyssonnaux et al., 2007; Imtiyaz and Simon, 2010). This evidence supports our findings that LPS-induced neuroinflammation was reduced in the hippocampus of mice after Roxadustat treatment. However, it was more interesting to be identified here that PI3K inhibition reversed the effects of Roxadustat, indicating a link between HIF-1 and PI3K signaling in the presence of LPS. Previous studies have also demonstrated that PI3K/Akt signaling could regulate HIF-1 levels via mTOR signaling at the posttranslational level but not at the mRNA level (under hypoxic conditions; Zhang et al., 2018). Because PI3K inhibitor (LY294002) and Dual PI3K/mTOR inhibitor NVP-BEZ235 treatment suppressed Akt and HIF-1 activation and expression (Karar et al., 2012), respectively. These results indicated that the PI3K-Akt cascade is a highly conserved intracellular signaling pathway involved in the immune system’s growth, motility, survival, metabolism, and coordinating defense mechanisms by transducing extracellular stimuli (Hemmings and Restuccia, 2012).
Studies demonstrate the beneficial effects of intermittent hypoxia on neurological disorders, including depression, by promoting neurogenesis via BDNF signaling (Ferrari et al., 2017; Meng et al., 2020); however, the mechanisms are not fully explored as for the potential beneficial effects through the HIF-1 hypoxia responding factor. Our results showed that Roxadustat treatment rescued LPS-altered synaptic protein level and spin-altered morphology. Interestingly, similar neuroprotective roles of Roxadustat have been recently reported, while its link to LPS/PI3K signaling has not been explored. Our results further demonstrated that PI3K signalling-antagonism reversed the protective effects of Roxadustat, indicating that HIF-1/PI3K signaling mediated the LPS-induced neuroinflammation, synaptic deficits, and depressive symptoms.
In conclusion, HIF-1 contributed to inflammation and the redox system under hypoxia conditions; it also played a role in neuroinflammation-induced depression, which might be associated with PI3K-related signalings. Furthermore, Roxadustat showed potent anti-depressive effects via reducing neuroinflammation by stabilizing HIF-1 expression; however, the proper LPS-stimulated signaling cross-talk to HIF-1 under depression conditions is enigmatic, which is the limitation of the present study, and it needs further investigation.
Data availability statement
The original contributions presented in the study are included in the article/Supplementary material, further inquiries can be directed to the corresponding authors.
Ethics statement
The animal study was reviewed and approved by Institutional Animal Care and Use Committee of Peking University, Shenzhen Graduate School.
Author contributions
AL, TA, and SL: conceptualization. AL, ZL, RG, YL, QG, and HG: methodology. AL, ZL, CZ, WL, XL, and TL: investigation, analysis and funding support. TA and SL: writing. SL and TL: supervision. All authors reviewed and approved the manuscript.
Funding
This work was supported by the National Natural Science Foundation of CHINA (NSFC grant numbers: 82072112 and 81871530).
Acknowledgments
We thank Hong Kong Institute of Brain Science, Shenzhen and Shenzhen Fundamental Research Institutions, Shenzhen, China.
Conflict of interest
The authors declare that the research was conducted in the absence of any commercial or financial relationships that could be construed as a potential conflict of interest.
Publisher’s note
All claims expressed in this article are solely those of the authors and do not necessarily represent those of their affiliated organizations, or those of the publisher, the editors and the reviewers. Any product that may be evaluated in this article, or claim that may be made by its manufacturer, is not guaranteed or endorsed by the publisher.
Supplementary material
The Supplementary material for this article can be found online at: https://www.frontiersin.org/articles/10.3389/fnmol.2023.1048985/full#supplementary-material
References
Abdoli, N., Salari, N., Darvishi, N., Jafarpour, S., Solaymani, M., Mohammadi, M., et al. (2022). The global prevalence of major depressive disorder (MDD) among the elderly: a systematic review and meta-analysis. Neurosci. Biobehav. Rev. 132, 1067–1073. doi: 10.1016/j.neubiorev.2021.10.041
Akizawa, T., Iwasaki, M., Otsuka, T., Reusch, M., and Misumi, T. (2019). Roxadustat treatment of chronic kidney disease-associated anemia in Japanese patients not on dialysis: a phase 2, randomized, double-blind, placebo-controlled trial. Adv. Ther. 36, 1438–1454. doi: 10.1007/s12325-019-00943-4
Ali, T., Hao, Q., Ullah, N., Rahman, S. U., Shah, F. A., He, K., et al. (2020a). Melatonin act as an antidepressant via attenuation of Neuroinflammation by targeting Sirt1/Nrf2/HO-1 signaling. Front. Mol. Neurosci. 13:96. doi: 10.3389/fnmol.2020.00096
Ali, T., Rahman, S. U., Hao, Q., Li, W., Liu, Z., Ali Shah, F., et al. (2020b). Melatonin prevents neuroinflammation and relieves depression by attenuating autophagy impairment through FOXO3a regulation. J. Pineal Res. 69:e12667. doi: 10.1111/jpi.12667
Andrade, C., and Rao, N. S. (2010). How antidepressant drugs act: a primer on neuroplasticity as the eventual mediator of antidepressant efficacy. Indian J. Psychiatry 52, 378–386. doi: 10.4103/0019-5545.74318
Appelhoff, R. J., Tian, Y.-M., Raval, R. R., Turley, H., Harris, A. L., Pugh, C. W., et al. (2004). Differential function of the prolyl hydroxylases PHD1, PHD2, and PHD3 in the regulation of hypoxia-inducible factor*. J. Biol. Chem. 279, 38458–38465. doi: 10.1074/jbc.M406026200
Athira, K. V., Bandopadhyay, S., Samudrala, P. K., Naidu, V. G. M., Lahkar, M., and Chakravarty, S. (2020). An overview of the heterogeneity of major depressive disorder: current knowledge and future prospective. Curr. Neuropharmacol. 18, 168–187. doi: 10.2174/1570159X17666191001142934
Brigitta, B. (2002). Pathophysiology of depression and mechanisms of treatment. Dialogues Clin. Neurosci. 4, 7–20. doi: 10.31887/DCNS.2002.4.1/bbondy
Cassano, P., Bui, E., Rogers, A. H., Walton, Z. E., Ross, R., Zeng, M., et al. (2017). Inflammatory cytokines in major depressive disorder: a case-control study. Aust. N. Z. J. Psychiatry 51, 23–31. doi: 10.1177/0004867416652736
Cordeiro, R. C., Chaves Filho, A. J. M., Gomes, N. S., Tomaz, V. D. S., Medeiros, C. D., Queiroz, A. I. D. G., et al. (2019). Leptin prevents lipopolysaccharide-induced depressive-like behaviors in mice: involvement of dopamine receptors. Front. Psych. 10:125. doi: 10.3389/fpsyt.2019.00125
Ferrari, M., Jain, I. H., Goldberger, O., Rezoagli, E., Thoonen, R., Cheng, K. H., et al. (2017). Hypoxia treatment reverses neurodegenerative disease in a mouse model of Leigh syndrome. Proc. Natl. Acad. Sci. U. S. A. 114, E4241–E4250. doi: 10.1073/pnas.1621511114
Frede, S., Stockmann, C., Freitag, P., and Fandrey, J. (2006). Bacterial lipopolysaccharide induces HIF-1 activation in human monocytes via p44/42 MAPK and NF-kappaB. Biochem. J. 396, 517–527. doi: 10.1042/BJ20051839
Gałecki, P., and Talarowska, M. (2018). Inflammatory theory of depression. Psychiatr. Pol. 52, 437–447. doi: 10.12740/PP/76863
Gutiérrez-Rojas, L., Porras-Segovia, A., Dunne, H., Andrade-González, N., and Cervilla, J. A. (2020). Prevalence and correlates of major depressive disorder: a systematic review. Braz. J Psychiatry 42, 657–672. doi: 10.1590/1516-4446-2020-0650
Han, F., Li, C.-F., Cai, Z., Zhang, X., Jin, G., Zhang, W.-N., et al. (2018). The critical role of AMPK in driving Akt activation under stress, tumorigenesis and drug resistance. Nat. Commun. 9:4728. doi: 10.1038/s41467-018-07188-9
Hasler, G. (2010). Pathophysiology of depression: do we have any solid evidence of interest to clinicians? World Psychiatry 9, 155–161. doi: 10.1002/j.2051-5545.2010.tb00298.x
Hemmings, B. A., and Restuccia, D. F. (2012). PI3K-PKB/Akt pathway. Cold Spring Harb. Perspect. Biol. 4:a011189. doi: 10.1101/cshperspect.a011189
Imtiyaz, H. Z., and Simon, M. C. (2010). Hypoxia-inducible factors as essential regulators of inflammation. Curr. Top. Microbiol. Immunol. 345, 105–120. doi: 10.1007/82_2010_74
Jamali-Raeufy, N., Alizadeh, F., Mehrabi, Z., Mehrabi, S., and Goudarzi, M. (2021). Acetyl-L-carnitine confers neuroprotection against lipopolysaccharide (LPS) -induced neuroinflammation by targeting TLR4/NFκB, autophagy, inflammation and oxidative stress. Metab. Brain Dis. 36, 1391–1401. doi: 10.1007/s11011-021-00715-6
Jung, Y. J., Isaacs, J. S., Lee, S., Trepel, J., and Neckers, L. (2003). IL-1beta-mediated up-regulation of HIF-1alpha via an NFkappaB/COX-2 pathway identifies HIF-1 as a critical link between inflammation and oncogenesis. FASEB J. 17, 2115–2117. doi: 10.1096/fj.03-0329fje
Karar, J., Cerniglia, G. J., Lindsten, T., Koumenis, C., and Maity, A. (2012). Dual PI3K/mTOR inhibitor NVP-BEZ235 suppresses hypoxia-inducible factor (HIF)-1α expression by blocking protein translation and increases cell death under hypoxia. Cancer Biol. Ther. 13, 1102–1111. doi: 10.4161/cbt.21144
Li, W., Ali, T., He, K., Liu, Z., Shah, F. A., Ren, Q., et al. (2021a). Ibrutinib alleviates LPS-induced neuroinflammation and synaptic defects in a mouse model of depression. Brain Behav. Immun. 92, 10–24. doi: 10.1016/j.bbi.2020.11.008
Li, W., Ali, T., Zheng, C., He, K., Liu, Z., Shah, F. A., et al. (2022). Anti-depressive-like behaviors of APN KO mice involve Trkb/BDNF signaling related neuroinflammatory changes. Mol. Psychiatry 27, 1047–1058. doi: 10.1038/s41380-021-01327-3
Li, W., Ali, T., Zheng, C., Liu, Z., He, K., Shah, F. A., et al. (2021b). Fluoxetine regulates eEF2 activity (phosphorylation) via HDAC1 inhibitory mechanism in an LPS-induced mouse model of depression. J. Neuroinflammation 18:38. doi: 10.1186/s12974-021-02091-5
Li, M., Li, C., Yu, H., Cai, X., Shen, X., Sun, X., et al. (2017). Lentivirus-mediated interleukin-1β (IL-1β) knock-down in the hippocampus alleviates lipopolysaccharide (LPS)-induced memory deficits and anxiety-and depression-like behaviors in mice. J. Neuroinflammation 14:190. doi: 10.1186/s12974-017-0964-9
Li, C., Zhao, B., Lin, C., Gong, Z., and An, X. (2019). TREM2 inhibits inflammatory responses in mouse microglia by suppressing the PI3K/NF-κB signaling. Cell Biol. Int. 43, 360–372. doi: 10.1002/cbin.10975
Liu, F., Wang, J., Ye, Q., Fu, H., and Mao, J. (2021). Roxadustat for renal anemia in ESRD from PKD patients: is it safe enough? J. Am. Soc. Nephrol. 32:1005. doi: 10.1681/ASN.2020111664
Liu, M. Y., Yin, C. Y., Zhu, L. J., Zhu, X. H., Xu, C., Luo, C. X., et al. (2018). Sucrose preference test for measurement of stress-induced anhedonia in mice. Nat. Protoc. 13, 1686–1698. doi: 10.1038/s41596-018-0011-z
Madeeh Hashmi, A., Awais Aftab, M., Mazhar, N., Umair, M., and Butt, Z. (2013). The fiery landscape of depression: a review of the inflammatory hypothesis. Pak. J. Med. Sci. 29, 877–884. doi: 10.12669/pjms.293.3357
Malhi, G. S., Bell, E., Morris, G., and Hamilton, A. (2020). The delay in response to antidepressant therapy: a window of opportunity? Aust. N. Z. J. Psychiatry 54, 127–129. doi: 10.1177/0004867419900313
Meng, S. X., Wang, B., and Li, W. T. (2020). Intermittent hypoxia improves cognition and reduces anxiety-related behavior in APP/PS1 mice. Brain Behav. 10:e01513. doi: 10.1002/brb3.1513
Mi, Z., Rapisarda, A., Taylor, L., Brooks, A., Creighton-Gutteridge, M., Melillo, G., et al. (2008). Synergystic induction of HIF-1alpha transcriptional activity by hypoxia and lipopolysaccharide in macrophages. Cell Cycle 7, 232–241. doi: 10.4161/cc.7.2.5193
O’connor, J. C., Lawson, M. A., André, C., Moreau, M., Lestage, J., Castanon, N., et al. (2009). Lipopolysaccharide-induced depressive-like behavior is mediated by indoleamine 2,3-dioxygenase activation in mice. Mol. Psychiatry 14, 511–522. doi: 10.1038/sj.mp.4002148
Palladino, M. A., Fasano, G. A., Patel, D., Dugan, C., and London, M. (2018). Effects of lipopolysaccharide-induced inflammation on hypoxia and inflammatory gene expression pathways of the rat testis. Basic Clin. Androl. 28:14. doi: 10.1186/s12610-018-0079-x
Peyssonnaux, C., Cejudo-Martin, P., Doedens, A., Zinkernagel, A. S., Johnson, R. S., and Nizet, V. (2007). Cutting edge: essential role of hypoxia inducible factor-1alpha in development of lipopolysaccharide-induced sepsis. J. Immunol. 178, 7516–7519. doi: 10.4049/jimmunol.178.12.7516
Postnikova, T. Y., Griflyuk, A. V., Ergina, J. L., Zubareva, O. E., and Zaitsev, A. V. (2020). Administration of Bacterial Lipopolysaccharide during early postnatal ontogenesis induces transient impairment of Long-term synaptic plasticity associated with behavioral abnormalities in young rats. Pharmaceuticals 13:48. doi: 10.3390/ph13030048
Saponaro, C., Cianciulli, A., Calvello, R., Dragone, T., Iacobazzi, F., and Panaro, M. A. (2012). The PI3K/Akt pathway is required for LPS activation of microglial cells. Immunopharmacol. Immunotoxicol. 34, 858–865. doi: 10.3109/08923973.2012.665461
Szegedi, A., Jansen, W. T., Van Willigenburg, A. P., Van Der Meulen, E., Stassen, H. H., and Thase, M. E. (2009). Early improvement in the first 2 weeks as a predictor of treatment outcome in patients with major depressive disorder: a meta-analysis including 6562 patients. J. Clin. Psychiatry 70, 344–353. doi: 10.4088/JCP.07m03780
Van Uden, P., Kenneth, N. S., and Rocha, S. (2008). Regulation of hypoxia-inducible factor-1alpha by NF-kappaB. Biochem. J. 412, 477–484. doi: 10.1042/BJ20080476
Verpelli, C., Piccoli, G., Zibetti, C., Zanchi, A., Gardoni, F., Huang, K., et al. (2010). Synaptic activity controls dendritic spine morphology by modulating eEF2-dependent BDNF synthesis. J. Neurosci. 30, 5830–5842. doi: 10.1523/JNEUROSCI.0119-10.2010
Xu, F., Na, L., Li, Y., and Chen, L. (2020). Roles of the PI3K/AKT/mTOR signalling pathways in neurodegenerative diseases and tumours. Cell Biosci. 10:54. doi: 10.1186/s13578-020-00416-0
Zhang, B., Wang, P. P., Hu, K. L., Li, L. N., Yu, X., Lu, Y., et al. (2019). Antidepressant-like effect and mechanism of action of Honokiol on the mouse lipopolysaccharide (LPS) depression model. Molecules 24:2035. doi: 10.3390/molecules24112035
Zhang, Z., Yao, L., Yang, J., Wang, Z., and Du, G. (2018). PI3K/Akt and HIF-1 signaling pathway in hypoxia-ischemia (review). Mol. Med. Rep. 18, 3547–3554. doi: 10.3892/mmr.2018.9375
Zhao, J., Bi, W., Xiao, S., Lan, X., Cheng, X., Zhang, J., et al. (2019). Neuroinflammation induced by lipopolysaccharide causes cognitive impairment in mice. Sci. Rep. 9:5790. doi: 10.1038/s41598-019-42286-8
Zheng, X., Zhang, W., and Hu, X. (2018). Different concentrations of lipopolysaccharide regulate barrier function through the PI3K/Akt signalling pathway in human pulmonary microvascular endothelial cells. Sci. Rep. 8:9963. doi: 10.1038/s41598-018-28089-3
Zhou, J., Schmid, T., and Brüne, B. (2003). Tumor necrosis factor-alpha causes accumulation of a ubiquitinated form of hypoxia inducible factor-1alpha through a nuclear factor-kappaB-dependent pathway. Mol. Biol. Cell 14, 2216–2225. doi: 10.1091/mbc.e02-09-0598
Zhu, X., Jiang, L., Wei, X., Long, M., and Du, Y. (2022). Roxadustat: not just for anemia. Front. Pharmacol. 13:971795. doi: 10.3389/fphar.2022.971795
Keywords: lipopolysaccharides, depression, Neuroinflammation, HIF-PHD/PI3K, roxadustat (FG-4592)
Citation: Li A, Liu Z, Ali T, Gao R, Luo Y, Gong Q, Zheng C, Li W, Guo H, Liu X, Li S and Li T (2023) Roxadustat (FG-4592) abated lipopolysaccharides-induced depressive-like symptoms via PI3K signaling. Front. Mol. Neurosci. 16:1048985. doi: 10.3389/fnmol.2023.1048985
Edited by:
Muddanna Sakkattu Rao, Kuwait University, KuwaitReviewed by:
Manikandan Samidurai, SENS Research Foundation, United StatesBharathi Hattiangady, Texas A&M University College of Medicine, United States
Copyright © 2023 Li, Liu, Ali, Gao, Luo, Gong, Zheng, Li, Guo, Li and Li. This is an open-access article distributed under the terms of the Creative Commons Attribution License (CC BY). The use, distribution or reproduction in other forums is permitted, provided the original author(s) and the copyright owner(s) are credited and that the original publication in this journal is cited, in accordance with accepted academic practice. No use, distribution or reproduction is permitted which does not comply with these terms.
*Correspondence: Shupeng Li, bGlzcEBwa3UuZWR1LmNu; Tao Li, TGl0YW8wNTA0MjhAbWFpbC54anR1LmVkdS5jbg==,