- 1Benephyt, Strasbourg, France
- 2Centre National de la Recherche Scientifique, University of Strasbourg, Institute of Cellular and Integrative Neuroscience, INCI UPR3212, Strasbourg, France
The activation of the transient receptor potential (TRP) channels expressed by sensory neurons is essential to the transduction of thermal and mechanical sensory information. In the setting of chronic inflammatory conditions, the activation of the melastatin family member 8 (TRPM8), the TRP vanilloid 1 (TRPV1), and the TRP ankyrin 1 (TRPA1) is correlated with pain hypersensitivity reactions. Monoterpenes, among which pulegone and menthol, a major class of phytocompounds present in essential oils of medicinal plants, are known modulators of those TRP channels activity. In the present review, we correlate the monoterpene content of plants with their historical therapeutic properties. We then describe how monoterpenes exert their anti-inflammatory and antihyperalgesia effects through modulation of TRP channels activity. Finally, we discuss the importance and the potential of characterizing new plant extracts and reassessing studied plant extracts for the development of ethnopharmacology-based innovative treatments for chronic pain. This review suggests that monoterpene solutions, based on composition from traditional healing herbs, offer an interesting avenue for the development of new phytotherapeutic treatments to alleviate chronic inflammatory pain conditions.
Introduction
Chronic inflammatory pain conditions such as osteoarthritis are debilitating diseases that impair the normal life of 14–36% of the population of the USA (Neogi, 2013; Treede et al., 2019). They are characterized by the presence of spontaneous pain, not evenly linked to an injury, and by hypersensitivity to painful stimuli (Raja et al., 2020). Despite the existence of several medications to alleviate chronic pain conditions, such as opioid treatment, which come with their share of unwanted side effects (Opioid Overdose Crisis | National Institute on Drug Abuse, 2022), most debilitating chronic inflammatory states remain uncured (Gilbert, 2021). Phytotherapy, or traditional herbalism, referring to the use of plant-derived preparation in the treatment and prevention of disease, might be a promising lead, with lower rates of adverse effects and efficiencies that might reach those of currently used conventional drugs (Dragos et al., 2017). Across the world, it remains the first intentional solution to diseases, or a preponderant complement to “conventional” medicine. Phytotherapy has been increasingly supported by the WHO (World Health Organization, 2013), and has been used to treat chronic joint inflammatory disorders such as osteoarthritis (Cameron and Chrubasik, 2014), rheumatoid arthritis (Dragos et al., 2017), and to alleviate chronic low back pain (Oltean et al., 2014).
Regarding their elaborate secondary metabolism, plants are indeed interesting reservoirs of phytocompounds with pharmacological activities (Yang et al., 2018). Among secondary metabolites, monoterpenes, such as menthol, are small volatile and fragrant molecules synthesized through the terpenoid biosynthesis pathway (Bergman and Phillips, 2021). Cyclohexanoids monoterpenes, also known as “p-menthanes,” are highly valuable molecules in industry and of important economical value, accounting for the majority of the compounds in essential oils (Tetali, 2018; Bergman et al., 2019). Widespread across the plant kingdom, menthol is, among this compound family, the most studied. It represents a reference molecule (Croteau et al., 2005), especially in the case of the modulation of pain conditions (Galeotti et al., 2002). Menthol is the naturally cyclic monoterpene alcohol found in the peppermint plant Mentha x piperita L. that gives the Mentha species their distinctive peppermint odor and flavor, and this essential oil triggers cold sensation through the activation of the transient receptor potential (TRP) melastatin family member 8 (TRPM8) channel (Peier et al., 2002). Menthol not only modulates TRPM8 but also several receptors and channels such as other TRP channels [for review (Oz et al., 2017)]; notably the TRP vanilloid 1 (TRPV1) (Takaishi et al., 2014) and the TRP ankyrin 1 (TRPA1) (Karashima et al., 2007) that are both transducers of noxious stimulation and therefore contribute to pain hypersensitivities during inflammation (Julius, 2013). Despite the fact that terpenes are the largest class of secondary metabolites, with over 50,000 compounds with interesting biological activities (such as antibacterial and anti-inflammatory) (Zielińska-Błajet and Feder-Kubis, 2020), less than thirty monoterpenes have been identified to promote analgesia and antihyperalgesia effects (Sousa and Pergentino, 2011; Guimarães et al., 2013; Gouveia et al., 2018). In a recent comparative study, menthol and its precursor, pulegone, have both been demonstrated to bear anti-inflammatory and antihyperalgesic properties in an inflammatory pain rodent model (Hilfiger et al., 2021) suggesting that plant extracts containing pulegone could help alleviate pain conditions, in a synergistic fashion.
In this review, first, we will list the plants containing menthol and pulegone, and enumerate their therapeutic properties mentioned in ethnobotanical data, to define a new plant group as matrices of interest. Second, we will summarize the evidence demonstrating the pain-killer properties of menthol and other monoterpenes. This will be further extended by the description of the current state of knowledge on the modulation of TRP channels by menthol and pulegone leading to reduced nociception. Finally, we will discuss the importance and the potential of characterizing new and reassessing studied plant extracts for the development of ethnopharmacology-based innovative treatments for chronic pain.
A historic standpoint: Lamiaceae, pulegone, and menthol
Menthol and pulegone are monoterpene derivatives synthesized through the conserved terpenoid backbone biosynthesis pathway (Figure 1A). They have been recently characterized and demonstrated to reduce the mechanical and thermal pain hypersensitivities in a rodent inflammatory pain model, thus reinforcing various previous results (de Sousa et al., 2007, 2011).
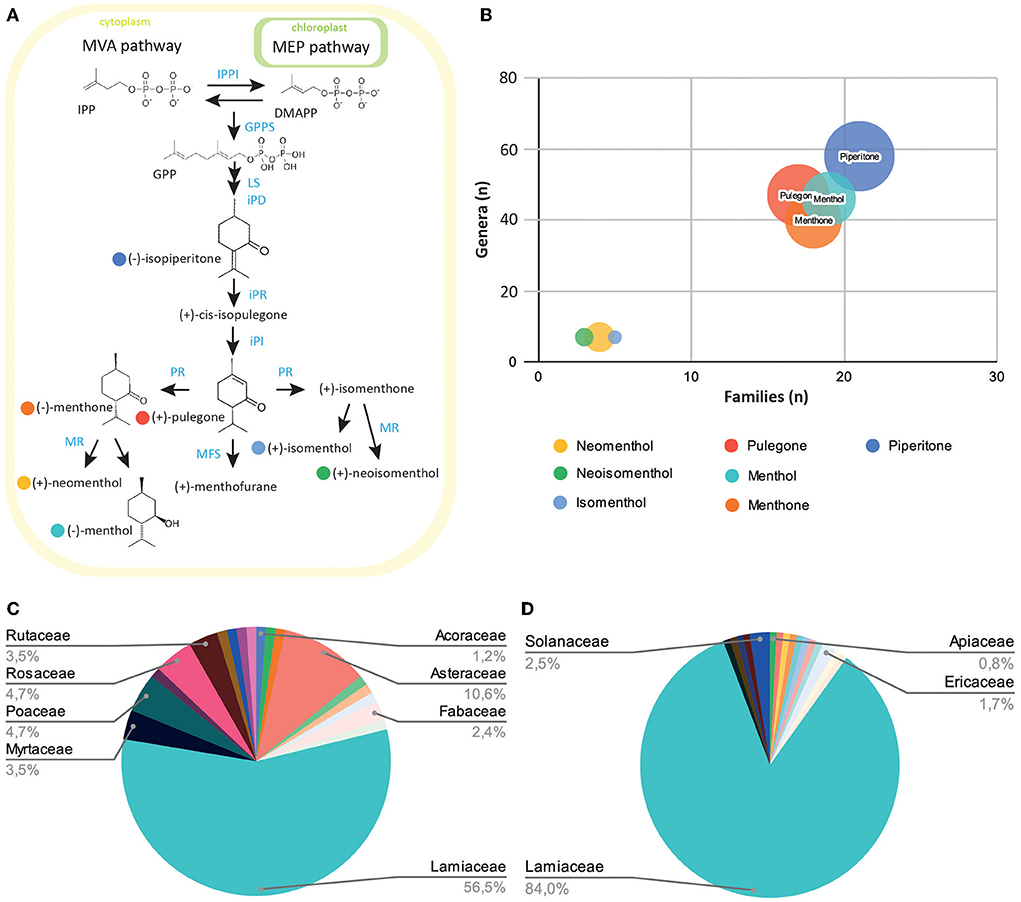
Figure 1. Exploration of the prevalence of menthol-like monoterpenes across the plant kingdom. (A) Schematic view of the monoterpene biosynthesis pathway leading to menthol, pulegone, and their derivatives. Plants produce the small precursor isopentenyl-pyrophosphate (IPP) through the mevalonate (MVA) pathway in the cytoplasm, or the methylerythritol (MEP) pathway in the plast. IPP can be isomerized into dimethylallyl-pyrophosphate (DMAPP) through the IPP isomerase activity (IPPI). Five-carbon units IPP and DMAPP can be converted into 10-unit geranyl-pyrophosphate (GPP) through the geranyl-pyrophosphate synthase (GPPS) activity. GPP is then converted into limonene, isopiperitone, and cis-isopulegone through limonene synthase (LS), trans-isopiperitenol dehydrogenase (iPD), and isopiperitone reductase (iPR) activity. Pulegone is then produced through cis-isopulegone isomerase (iPI) activity. Pulegone reductase (PR) converts pulegone into isomenthone or menthone, through different activities. These two compounds can then be converted into neomenthol and menthol, or isomenthol and neoisomenthol, respectively, through the menthone reductase activity (MR). The menthofurane synthase (MFS) can also convert pulegone into menthofurane, in an NADP-dependant reaction. (B) Bubble diagram representing the number of families (x-axis), genera (y-axis), and species (circle radius) containing monoterpenes precursors or derivatives. (C) Diagram of the distribution of menthol-containing plants within plant families. (D) Diagram of the distribution of pulegone-containing plants within plant families.
We first wanted to explore natural products databases to establish a list of plants currently known to contain menthol or pulegone. Because there are many natural products repositories (Sorokina and Steinbeck, 2020), we chose to investigate the LOTUS database, as it is one of the latest intents to harmonize, curate, and validate structure-organism pairs, in an open access fashion (Rutz et al., 2021). Dr. Duke's Phytochemical and Ethnobotanical Database (1992–2016), hosted on the USDA website (https://data.nal.usda.gov/dataset/dr-dukes-phytochemical-and-ethnobotanical-databases), was also consulted for its richness in ethnobotanical mentions for each plant. We started by extracting all plant names mentioned to contain pulegone and/or menthol, and also their precursor piperitone and their associated derivatives: neomenthol, isomenthol, and neoisomenthol (Figure 1B). To avoid any duplicates, their names were converted to their accurate scientific names and botanical families according to the Angiosperm Phylogeny Group IV (2016) and listed on “Plants of the World Online” (POWO, 2022 facilitated by the Royal Botanic Gardens; http://www.plantsoftheworldonline.org/---Retrieved 10 May 2022). Results show that piperitone appears in the highest number of species inventoried in the databases (over 158 species listed), throughout most of the plant families registered, whereas the derivatives of pulegone: (neomenthol, neoisomenthol, and isomenthol) present the lowest occurrence in the catalog (24, 13, and 12 species, respectively) (Figure 1B). The list of all plants recorded for the presence of menthol and pulegone forms a group of 85 and 110 species of plants, respectively (Supplementary Table 1). Among them, the Lamiaceae subgroup is best represented, with pulegone and menthol present in 84 and 56.5% of the Lamiaceae, respectively (Figures 1C,D).
Because of their aromatic properties, plants within this family have been extensively used across the world and for all kinds of purposes: food, aromatics, cosmetics, and healing. A trend to investigate plant properties based on their traditional use has emerged, embodied in the vast discipline of ethnopharmacology and showing promising results (Bruhn and Rivier, 2019). The re-examination of historical texts and traditional pharmacopeias will help to re-evaluate plant species' potential toward new indications that were discarded in the modern pharmacopeias (Heinrich et al., 2006).
During the Greek Antiquity (Leonti and Verpoorte, 2017), as referred to in Theophrastus' Historia Plantarum (Totelin, 2006), Origanum majorana and Origanum sipylaeum are mentioned as aromatics. Mentha pulegium is also mentioned with properties useful for pain treatment that were allegedly “known by all”: endemic from Crete and imported to Athens, it had a great reputation in ancient Greece (Totelin, 2006). In Mattioli's translation of Dioscorides' original texts from AD 50 to 70 (Mattioli, 1590), Thymbrium was cited as Menta romana for the Italians, and it was stated that leaves could relieve head pain when topically applied as a cataplasm. Origanum was described to relieve ear pain, when applied with milk. As for the “Pulegium,” probably referring to M. pulegium L., he stated that when applied with dried sour cherry, it relieved all inflammation and helped patients to support gout (Mattioli, 1590). A cross-observation between the following plants: M. pulegium, Origanum, and M. romana, reveals that they are all reported to contain menthol and pulegone; (Supplementary Table 1) eventually supporting a shared use based on their aromatic properties (Ntalli et al., 2010). Interestingly, 38 plants of the Lamiaceae family are present on the A list of the French Pharmacopeia, among which 22 are sold over the counter. Eight species are on the B list, due to their putative undesirable effects outnumbering the benefits they provide. Very few of them still have an indication for pain alleviation. As plant uses and medical paradigms fluctuate across history, sometimes resulting in loss of knowledge or transformations in the indications, it is important to re-assess plant compositions and their effects on a scientific basis.
Among those, M. x piperita L. is a textbook example, having been used for centuries in traditional medicine to reduce numerous ailments such as infections, insomnia, irritable bowel syndrome, and also pain (Farco and Grundmann, 2013). It has been demonstrated that the large quantity of menthol in this plant acts as the major active principle to alleviate joint pain (Topp et al., 2013). Another example is Clinopodium nepeta (L.) Kuntze, a plant of the Lamiaceae family, which has been mentioned since Greek Antiquity and in at least two of the most renowned herbals of the 16th and 17th centuries for the treatment of rheumatism (Adams et al., 2009): in Mattioli (1590), it is advised to boil and drink a C. nepeta (L.) Kuntze-based broth against gout and slime, while FUCHS (1543) recommends an external application of leaves against hip pain. In this case, the major active substance associated with beneficial effects is pulegone, which is found in large quantities in C. nepeta (L.) (BoŽović and Ragno, 2017). Recent evidence points out that pulegone, like menthol, acts as an antihyperalgesic phytocompound in an inflammatory pain animal model (Hilfiger et al., 2021).
Phytotherapy based on M. x piperita L. (Mentha x officinalis Hull) and C. nepeta (L.), Kuntze preparations rich in menthol or pulegone, as a major active compound, shows the potential for studying other members of the Lamiaceae family described throughout history of medicine for pain relief to reveal other analgesic monoterpenes. Chemotaxonomy suggests that other plants, which did not make it to the European traditional pharmacopeia, might also be interesting due to their composition. In our analysis, we found out, for instance, that Clinopodium serpylifollium subsp. fruticosum (L.) Bräuchler's [formally Micromeria fruticosa (L.) Druce] essential oil contains over 80% of pulegone, along with 36 other compounds. It might therefore be an interesting European plant to investigate (Kinmer et al., 1993). This idea is supported by the fact that essential oils from Lamiaceae do not exclusively contain pulegone or menthol but also other monoterpenes which, although present in low quantities, could act potentially in synergy on different molecular targets to alleviate pain (Maroon et al., 2010). However, as in the case of any molecule under investigation, it is crucial to assess toxicity effects (Wojtunik-Kulesza, 2022). Although pulegone's use as a food additive has been restricted by the FDA, there is no conclusive evidence of its carcinogenicity, and it is still authorized when extracted from natural sources (Food Drug Administration, 2018).
Pulegone and menthol as potent analgesic and anti-inflammatory phytocompounds
Pulegone and menthol are both reported to have analgesic properties, along with 26 other monoterpenes and some of their derivatives (Guimarães et al., 2013; Wang et al., 2017; Dos Santos et al., 2021). To understand their putative pain relief properties, we reduced this group to monoterpenes associated with anti-inflammatory activity (de Cássia da Silveira e Sá et al., 2013) that is mediated at least through an anti-TNF-α activity (Chen et al., 2021; Hilfiger et al., 2021). We combined data reporting a modulation of the TRPV1, TRPA1, or TRPM8 activity to define a group of anti-inflammatory phytocompound modulators of TRP channels (Xu et al., 2005; de Sousa et al., 2010; Ortar et al., 2012; de Cássia da Silveira e Sá et al., 2013; Guimarães et al., 2013; Rufino et al., 2014; Takaishi et al., 2014; Mihara and Shibamoto, 2015; Ohtsubo et al., 2015; Dai, 2016; Kaimoto et al., 2016; Oz et al., 2017; Wang et al., 2017; Chamanara et al., 2019; de Christo Scherer et al., 2019; Quintans et al., 2019; Soleimani et al., 2019; Ghosh et al., 2020; Heblinski et al., 2020; Hilfiger et al., 2020; Islam et al., 2020; Zhu et al., 2020; Chen et al., 2021; Dos Santos et al., 2021; Kashiwadani et al., 2021) (Table 1). Both menthol and pulegone, among other monoterpenes, match these properties (Table 1). Interestingly, M. pulegium L. and Origanum dictamnus L. have been used in traditional pain-relief preparations and their essential oil analysis revealed that their essential oils contain 5 and 6 of those monoterpenes, respectively (Ntalli et al., 2010) (see Table 1).
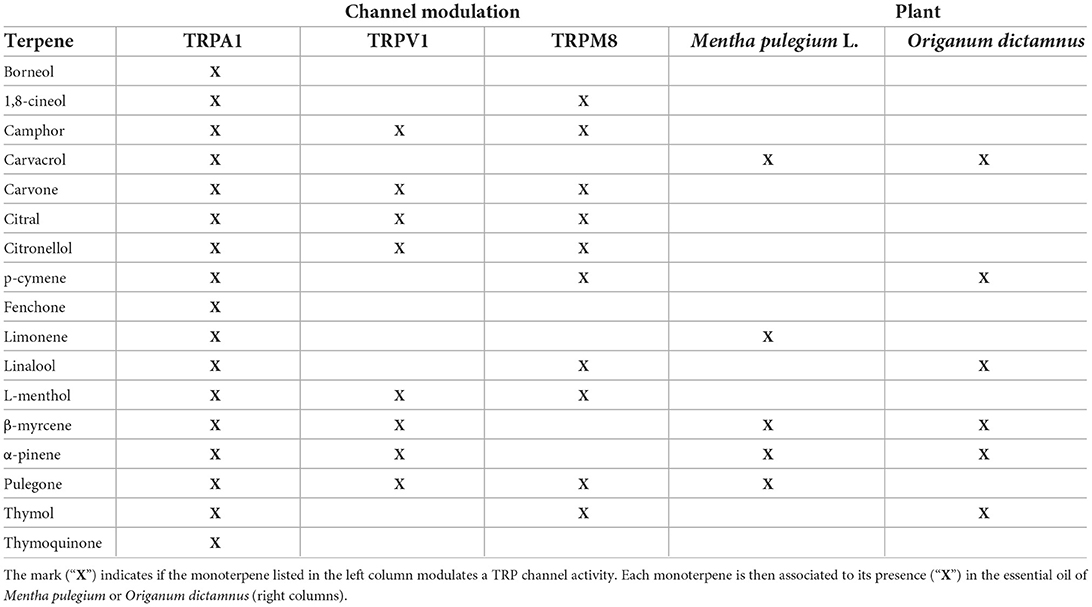
Table 1. Anti-inflammatory monoterpenes modulating TRP channel activity compared to the essential oil of Mentha pulegium L. and Origanum dictamnus L.
However, how monoterpenes, particularly based on menthol and pulegone reports, promote analgesic properties through the modulation of the activities of TRPV1, TRPA1, or TRPM8 channels in the setting of inflammatory pain conditions remains to be explored.
TRP channels in pain, physiologic, and chronic inflammatory conditions
Under normal conditions, nociceptors aim to detect potentially painful stimuli, and the detection and transduction of neuronal messages are less involving: TRPV1, for temperatures > 42°C; TRPM8, for temperatures around 18°C, and TRPA1, for various noxious and mechanical stimuli (Dai, 2016). Aside from these functional arrangements exists an anatomical differentiation: TRPA1-expressing nociceptors are a subset of TRPV1-expressing nociceptors in rodents, whereas TRPM8 remains more expressed in a different set of specific sensory neurons (Dhaka et al., 2008; Jankowski et al., 2017; Kupari et al., 2021). In addition to their sensor roles, it is important to note that TRP channels are also expressed in immune cells [for review (Khalil et al., 2018)]: TRPM8 activity regulates the TNF-α production by macrophage cells (Khalil et al., 2016). These observations allow considering TRP channels not only as peripheral transducers but also as ionic receptors for endogenous ligands and major actors in pain hypersensitivities: TRPM8, TRPA1, and TRPV1 have a fundamental role in the initiation, regulation, and maintenance of hyperalgesia conditions (Schumacher, 2010).
Moreover, they may have a role in pain-central sensitization due to their expression in different regions and/or cellular subtypes of the central nervous system. Indeed, TRPV1 was reported to be expressed in a subset of sensory neurons (Cavanaugh et al., 2011) and central nervous cells (González-Ramírez et al., 2017). Therefore, rodent TRPV1 is present in brain regions such as the sensory, prefrontal, entorhinal, insular, and anterior cingulate cortices, and also the hippocampus, amygdala, and thalamus (for review see Duitama et al., 2020). Interestingly, those regions are involved in pain integration and/or modulation (Ordás et al., 2021; Wahis et al., 2021). However, it is important to note that human TRPV1 was, up to now, only detected in cortical regions (Morelli et al., 2013). The functional contribution of the central expression of TRPV1 is still debated and its contribution to central synaptic plasticity, especially as part of the endocannabinoid system regulation, is under scrutiny (Hakimizadeh et al., 2012; Li et al., 2021). Functional investigations have demonstrated that TRPV1 expressed in hippocampal neurons (Sun et al., 2013) contributes to strengthening spontaneous synaptic transmission (Anstötz et al., 2018).
Functional TRPA1 is described in the hippocampus and somatosensory cortex (Menigoz and Boudes, 2011; Shigetomi et al., 2011; Kheradpezhouh et al., 2017). In the hippocampus, TRPA1 activation increases extracellular GABA concentration, leading to a downregulation of the astrocyte GABA transport GAT-3 activity and a decreased efficiency of inhibitory synapses (Shigetomi et al., 2011). Finally, TRPM8 is present at least in the hypothalamus, septum, and thalamic reticular nucleus (for review Ordás et al., 2021). Interestingly, TRPM8 expressing neurons are innervating the hypothalamus, the periaqueductal gray, and the amygdala regions (Ordás et al., 2021), which are major actors of pain regulation (Eliava et al., 2016; Ordás et al., 2021; Iwasaki et al., 2022).
Under inflammatory conditions, TRP channels are overexpressed at the membrane of sensory nerve endings innervating the inflamed joint. In addition, their intrinsic properties, namely, conductance or sensibility to noxious cues, change and contribute directly to abnormal pain symptoms such as hyperalgesia (Schumacher, 2010). Such conclusions are supported through experimental investigation using inflammatory animal pain models based on the administration of the complete Freund's adjuvant (CFA). Consisting of an injection of CFA into the joint or into the plantar surface of the hind paw, this model is characterized by the presence of exaggerated sensitivity to heat and cold stimulations and heightened sensitivity to mechanical tactile stimulations (Hilfiger et al., 2020, 2021). At the inflammation site, the free endings of the nociceptors are in contact with several inflammatory mediators such as proinflammatory cytokines and protein kinases [for review: (Dai, 2016)]. These interactions lead to sensitization of the nociceptors through different molecular pathways, which contribute directly to pain hypersensitivities and are referred to as “peripheral sensitization” (Mizumura, 1997). Peripheral sensitization allows, for example, the overexpression of TRPV1 in nociceptors (Yu et al., 2008). Such condition was investigated, using complementary pharmacological and genetic approaches. It was demonstrated that TRPV1 is essential to thermal hyperalgesia but not to mechanical hypersensitivity of nociceptors (for review (Dai, 2016). Nonetheless, their activation still recruits mechanical allodynia neuronal circuits (Petitjean et al., 2014). Based on the same approaches, it was clearly shown that TRPM8 is involved in cold hyperalgesia (Colburn et al., 2007; Gong and Jasmin, 2017) while TRPA1 is responsible for mechanical hypersensitivity and nocifensive reactions (Kwan et al., 2006; Eid et al., 2008; Kerstein et al., 2009; Vilceanu and Stucky, 2010; Lennertz et al., 2012; Asgar et al., 2015).
Thus, the three following TRP channels act as a complex molecular system that participates in pain hypersensitivities: TRPV1 contributes to thermal hyperalgesia (Caterina et al., 1997; Petitjean et al., 2014; Koivisto et al., 2022), TRPA1 contributes to thermal, mechanical, and chemical hyperalgesia (Koivisto et al., 2022), and TRPM8 contributes to cold allodynia (Koivisto et al., 2022). Expressed by nociceptors, those channels are easily accessible for exogenous molecules and two paths are generally proposed: a blockage of the channel activity through effective antagonists (Dai, 2016) or, paradoxically, overstimulation leading to long-term desensitization. Therefore, TRPV1, TRPM8, and TRPA1 are ideal targets for analgesic molecules.
Monoterpenes and TRP channels: An analgesic combination?
Interestingly, both pulegone and menthol act as strong anti-inflammatory molecules in in vitro essay with EC50 values of 1.2 ± 0.2 and 1.5 ± 0.1 mM, respectively (Hilfiger et al., 2021). In addition, a single intraperitoneal injection of 100 mg/kg pulegone or menthol in CFA rats exerts a transient antihyperalgesic effect on both mechanical, thermal heat and cold hyperalgesia (Hilfiger et al., 2021). Few studies have focused on pulegone-dependent mechanisms that reduce pain reactions. In chicks, intraplantar injection of pulegone causes nocifensive reactions which are attenuated by TRPA1 antagonists (Majikina et al., 2018). In humans and rodents, pulegone appears as a partial agonist of TRPV1 and an inhibitor of the menthol-induced TRPM8 activity (Jabba and Jordt, 2020) (Table 1). These suggest that pulegone is a strong agonist of TRPA1, and a partial TRPV1 agonist, and inhibits the TRPM8 activity. Interestingly, it is proposed that TRPA1 can be desensitized through different cellular pathways which are regulated by the activation of TRPV1 (Akopian et al., 2007; Ruparel et al., 2011; Kistner et al., 2016).
Molecular targets of menthol have been better investigated [for review (Oz et al., 2017)]. In humans and mouse pain models alike, TRPV1 currents activated by heat and capsaicin were inhibited by menthol, a phenomenon that might partially explain its antinociceptive properties (Takaishi et al., 2014); it also activates the TRPM8 to promote an antihyperalgesic effect (Liu et al., 2013). This activation induces a “cooling” sensation, followed by a TRPM8 channel shift into an inactive state that can be reversed only after the wash-out of the agonist compounds: a phenomenon called transient desensitization (Diver et al., 2019; Perri et al., 2020). Furthermore, menthol exerts in murine cells (in vitro) a bimodal modulation of TRPA1, activating it at low concentration (0.3–0.7 mM) and blocking it at high concentration (>3 mM) (Lemon et al., 2019). However, in humans, only the activation of TRPA1 by methanol has been observed (Xiao et al., 2008). This suggests that menthol is a strong agonist of TRPM8, and a partial TRPA1 agonist or blocker, and that it inhibits the TRPV1 activity.
In conclusion, both pulegone and menthol strongly interact with TRPM8, TRPA1, and TRPV1 (Table 1) (Takaishi et al., 2014; Oz et al., 2017; Jabba and Jordt, 2020). These pharmacological properties remain to be explored in the setting of TRPV1/TRPA1 channel heterodimers (Fischer et al., 2014). These heterodimers contribute also to pain symptoms (Galindo et al., 2018; Souza Monteiro de Araujo et al., 2020). Based on those properties, monoterpene activating the TRP channel with antihyperalgesic properties may be explained by an initial activation and then desensitization of TRP channels expressed in sensory neurons.
Prospects of new phytotherapy treatment to alleviate pain conditions
In prospect, beyond plants extracts or essential oils preparations, new treatments relying on specific monoterpenes mixes open an interesting avenue to treat pain. Monoterpenes are very volatile and have the capacity to cross the skin and the blood–brain barrier—thus enabling the modulation of peripheral and central neuronal, and immune cell receptors (Zhang et al., 2015; Weston-Green, 2019).
Based on these properties, commercial topical creams for pain relief were developed, for example, containing a mix of 3 monoterpenes: camphor, 1,8-cineole, and menthol (product NPN 02248563 RUB-A533 Antiphlogistine). To define the efficiency of a new putative mix, for new topical preparations, for instance, the reassessment of compounds found in traditional/popular medicine treatments and investigation of other bioactive principles that could alleviate chronic pain conditions seems a promising path. With an aim to develop a local and effective treatment to alleviate chronic conditions, this work intends to demonstrate the potential for the discovery of new antinociceptive drugs: there are already 50,000 terpenes identified (Breitmaier, 2006; Yamada et al., 2015) and their putative synergistic analgesic effects remain to be explored. This approach will further enable us to discover new uses for local plant species and to consider meaningful actions toward their valorization on environmental and patrimonial levels. Therefore, efforts toward the translation, annotation, and promotion of old folk books to a large audience are still worthy enterprises [for review (UNESCO World Heritage Center, 2010)]. Although ethnobotanical studies have been booming since the 1980s, few initiatives managed to share the results with traditional communities or raise awareness of local plant materials (Reyes-García et al., 2021; Schultz et al., 2021).
In the era of open science and open data sharing and within the logic of the “one health” theory, it is of the utmost importance to share this knowledge with citizens and professionals and to protect our plant heritage increasing our global commitment to biodiversity, as advised by international organizations (UNESCO, 2018; Mackenzie and Jeggo, 2019). We believe that this approach will bring new virtuous and resilient cycles of innovation, while increasing citizens' commitment and engagement toward their ecosystems.
Author contributions
EH performed the plant databases analysis. HP and LH performed the monoterpene databases analysis. EH, HP, and AC coordinated and produced the review. All authors discussed and elaborated the ideas, wrote the first draft, corrected it until the final version was obtained, and approved the manuscript.
Funding
This work was supported by the Centre National de la Recherche Scientifique contract UPR3212, the Université de Strasbourg contract UPR3212, the Graduate School of Pain EURIDOL, ANR-17-EURE-0022, and the ANRT CIFRE Grants No. 2018/1140 (to AC and HP) and no. 2016/047 (to HP).
Conflict of interest
HP, EH, LH, and GR are salaried employees at the private company Benephyt.
The remaining authors declare that the research was conducted in the absence of any commercial or financial relationships that could be construed as a potential conflict of interest.
Publisher's note
All claims expressed in this article are solely those of the authors and do not necessarily represent those of their affiliated organizations, or those of the publisher, the editors and the reviewers. Any product that may be evaluated in this article, or claim that may be made by its manufacturer, is not guaranteed or endorsed by the publisher.
Supplementary material
The Supplementary Material for this article can be found online at: https://www.frontiersin.org/articles/10.3389/fnmol.2022.945450/full#supplementary-material
References
Adams, M., Berset, C., Kessler, M., and Hamburger, M. (2009). Medicinal herbs for the treatment of rheumatic disorders–a survey of European herbals from the 16th and 17th century. J. Ethnopharmacol. 121, 343–359. doi: 10.1016/j.jep.2008.11.010
Akopian, A. N., Ruparel, N. B., Jeske, N. A., and Hargreaves, K. M. (2007). Transient receptor potential TRPA1 channel desensitization in sensory neurons is agonist dependent and regulated by TRPV1-directed internalization. J. Physiol. 583, 175–193. doi: 10.1113/jphysiol.2007.133231
Angiosperm Phylogeny Group IV (2016). An Update of the Angiosperm Phylogeny Group Classification for the Orders and Families of Flowering Plants. APG IV. Botanical Journal of the Linnean Society (Wiley Online Library). Available online at: https://onlinelibrary.wiley.com/doi/abs/10.1111/boj.12385 (accessed May 10, 2022).
Anstötz, M., Lee, S. K., and Maccaferri, G. (2018). Expression of TRPV1 channels by Cajal-Retzius cells and layer-specific modulation of synaptic transmission by capsaicin in the mouse hippocampus. J. Physiol. 596, 3739–3758. doi: 10.1113/JP275685
Asgar, J., Zhang, Y., Saloman, J. L., Wang, S., Chung, M.-K., and Ro, J. Y. (2015). The role of TRPA1 in muscle pain and mechanical hypersensitivity under inflammatory conditions in rats. Neuroscience 310, 206–215. doi: 10.1016/j.neuroscience.2015.09.042
Bergman, M. E., Davis, B., and Phillips, M. A. (2019). Medically useful plant terpenoids: biosynthesis, occurrence, and mechanism of action. Molecules 24, 3961. doi: 10.3390/molecules24213961
Bergman, M. E., and Phillips, M. A. (2021). Structural diversity and biosynthesis of plant derived p-menthane monoterpenes. Phytochem. Rev. 20, 433–459. doi: 10.1007/s11101-020-09726-0
BoŽović, M., and Ragno, R. (2017). Calamintha nepeta (L.) savi and its main essential oil constituent pulegone: biological activities and chemistry. Molecules 22, 290. doi: 10.3390/molecules22020290
Breitmaier, E. (2006). “Terpenes: Importance, general structure, and biosynthesis,” in Terpenes (John Wiley and Sons Ltd), 1–9. doi: 10.1002/9783527609949.ch1
Bruhn, J. G., and Rivier, L. (2019). Ethnopharmacology - a journal, a definition and a society. J. Ethnopharmacol. 242, 112005. doi: 10.1016/j.jep.2019.112005
Cameron, M., and Chrubasik, S. (2014). Oral herbal therapies for treating osteoarthritis. Cochrane Database Syst. Rev. 2014, CD002947. doi: 10.1002/14651858.CD002947.pub2
Caterina, M. J., Schumacher, M. A., Tominaga, M., Rosen, T. A., Levine, J. D., and Julius, D. (1997). The capsaicin receptor: a heat-activated ion channel in the pain pathway. Nature 389, 816–824. doi: 10.1038/39807
Cavanaugh, D. J., Chesler, A. T., Bráz, J. M., Shah, N. M., Julius, D., and Basbaum, A. I. (2011). Restriction of transient receptor potential vanilloid-1 to the peptidergic subset of primary afferent neurons follows its developmental downregulation in nonpeptidergic neurons. J. Neurosci. 31, 10119–10127. doi: 10.1523/JNEUROSCI.1299-11.2011
Chamanara, M., Abdollahi, A., Rezayat, S. M., Ghazi-Khansari, M., Dehpour, A., Nassireslami, E., et al. (2019). Thymol reduces acetic acid-induced inflammatory response through inhibition of NF-kB signaling pathway in rat colon tissue. Inflammopharmacology 27, 1275–1283. doi: 10.1007/s10787-019-00583-8
Chen, M., Zheng, J., Zou, X., Ye, C., Xia, H., Yang, M., et al. (2021). Ligustrum robustum (Roxb.) blume extract modulates gut microbiota and prevents metabolic syndrome in high-fat diet-fed mice. J. Ethnopharmacol. 268, 113695. doi: 10.1016/j.jep.2020.113695
Colburn, R. W., Lubin, M. L., Stone, D. J., Wang, Y., Lawrence, D., D'Andrea, M. R., et al. (2007). Attenuated cold sensitivity in TRPM8 null mice. Neuron 54, 379–386. doi: 10.1016/j.neuron.2007.04.017
Croteau, R. B., Davis, E. M., Ringer, K. L., and Wildung, M. R. (2005). (-)-Menthol biosynthesis and molecular genetics. Naturwissenschaften 92, 562–577. doi: 10.1007/s00114-005-0055-0
de Cássia da Silveira e Sá, R., Andrade, L. N., and de Sousa, D. P. (2013). A review on anti-inflammatory activity of monoterpenes. Mol. Basel Switz. 18, 1227–1254. doi: 10.3390/molecules18011227
de Christo Scherer, M. M., Marques, F. M., Figueira, M. M., Peisino, M. C. O., Schmitt, E. F. P., Kondratyuk, T. P., et al. (2019). Wound healing activity of terpinolene and α-phellandrene by attenuating inflammation and oxidative stress in vitro. J. Tissue Viability 28, 94–99. doi: 10.1016/j.jtv.2019.02.003
de Sousa, D. P., Camargo, E. A., Oliveira, F. S., and de Almeida, R. N. (2010). Anti-inflammatory activity of hydroxydihydrocarvone. Z. Naturforschung C J. Biosci. 65, 543–550. doi: 10.1515/znc-2010-9-1003
de Sousa, D. P., Júnior, E. V. M., Oliveira, F. S., de Almeida, R. N., Nunes, X. P., and Barbosa-Filho, J. M. (2007). Antinociceptive activity of structural analogues of rotundifolone: structure-activity relationship. Z. Naturforschung C J. Biosci. 62, 39–42. doi: 10.1515/znc-2007-1-207
de Sousa, D. P., Nóbrega, F. F. F., de Lima, M. R. V., and de Almeida, R. N. (2011). Pharmacological activity of (R)-(+)-pulegone, a chemical constituent of essential oils. Z. Naturforschung C J. Biosci. 66, 353–359. doi: 10.1515/znc-2011-7-806
Dhaka, A., Earley, T. J., Watson, J., and Patapoutian, A. (2008). Visualizing cold spots: TRPM8-expressing sensory neurons and their projections. J. Neurosci. 28, 566–575. doi: 10.1523/JNEUROSCI.3976-07.2008
Diver, M. M., Cheng, Y., and Julius, D. (2019). Structural insights into TRPM8 inhibition and desensitization. Science 365, 1434–1440. doi: 10.1126/science.aax6672
Dos Santos, E., Leitão, M. M., Aguero Ito, C. N., Silva-Filho, S. E., Arena, A. C., Silva-Comar, F. M., et al. (2021). Analgesic and anti-inflammatory articular effects of essential oil and camphor isolated from Ocimum kilimandscharicum Gürke leaves. J. Ethnopharmacol. 269, 113697. doi: 10.1016/j.jep.2020.113697
Dragos, D., Gilca, M., Gaman, L., Vlad, A., Iosif, L., Stoian, I., et al. (2017). Phytomedicine in joint disorders. Nutrients 9, E70. doi: 10.3390/nu9010070
Dr. Duke's Phytochemical Ethnobotanical Database (1992–2016). Dr. Duke's Phytochemical and Ethnobotanical Databases (U.S. Department of Agriculture, Agricultural Research Service). Home Page. Available online at: http://phytochem.nal.usda.gov/ (accessed June 30, 2022).
Duitama, M., Vargas-López, V., Casas, Z., Albarracin, S. L., Sutachan, J.-J., and Torres, Y. P. (2020). TRP channels role in pain associated with neurodegenerative diseases. Front. Neurosci. 14, 782. doi: 10.3389/fnins.2020.00782
Eid, S. R., Crown, E. D., Moore, E. L., Liang, H. A., Choong, K.-C., Dima, S., et al. (2008). HC-030031, a TRPA1 selective antagonist, attenuates inflammatory- and neuropathy-induced mechanical hypersensitivity. Mol. Pain 4, 1744-8069-4–48. doi: 10.1186/1744-8069-4-48
Eliava, M., Melchior, M., Knobloch-Bollmann, H. S., Wahis, J., Gouveia, M., da, S., et al. (2016). A new population of parvocellular oxytocin neurons controlling magnocellular neuron activity and inflammatory pain processing. Neuron 89, 1291–1304. doi: 10.1016/j.neuron.2016.01.041
Farco, J. A., and Grundmann, O. (2013). Menthol – pharmacology of an important naturally medicinal “cool.” Mini-Rev. Med. Chem. 13, 124–131. doi: 10.2174/138955713804484686
Fischer, M. J. M., Balasuriya, D., Jeggle, P., Goetze, T. A., McNaughton, P. A., Reeh, P. W., et al. (2014). Direct evidence for functional TRPV1/TRPA1 heteromers. Pflugers Arch. 466, 2229–2241. doi: 10.1007/s00424-014-1497-z
Food and Drug Administration (2018). Food Additive Regulations; Synthetic Flavoring Agents and Adjuvants. Fed. Regist. Available online at: https://www.federalregister.gov/documents/2018/10/09/2018-21807/food-additive-regulations-synthetic-flavoring-agents-and-adjuvants (accessed May 10, 2022).
FUCHS, L. (1543). New Kreüterbuch. Available online at: https://docnum.unistra.fr/digital/collection/coll13/id/219002/rec/555 (accessed June 30, 2022).
Galeotti, N., Di Cesare Mannelli, L., Mazzanti, G., Bartolini, A., and Ghelardini, C. (2002). Menthol: a natural analgesic compound. Neurosci. Lett. 322, 145–148. doi: 10.1016/S0304-3940(01)02527-7
Galindo, T., Reyna, J., and Weyer, A. (2018). Evidence for transient receptor potential (TRP) channel contribution to arthritis pain and pathogenesis. Pharm. Basel Switz. 11, E105. doi: 10.3390/ph11040105
Ghosh, M., Schepetkin, I. A., Özek, G., Özek, T., Khlebnikov, A. I., Damron, D. S., et al. (2020). Essential oils from monarda fistulosa: chemical composition and activation of transient receptor potential A1 (TRPA1) channels. Molecules 25, 4873. doi: 10.3390/molecules25214873
Gilbert, S. (2021). “Chronic pain and dependence,” in Textbook of Addiction Treatment: International Perspectives, eds N. el-Guebaly, G. Carrà, M. Galanter, and A. M. Baldacchino (Cham: Springer International Publishing), 1255–1267.
Gong, K., and Jasmin, L. (2017). Sustained morphine administration induces TRPM8-dependent cold hyperalgesia. J. Pain 18, 212–221. doi: 10.1016/j.jpain.2016.10.015
González-Ramírez, R., Chen, Y., Liedtke, W. B., and Morales-Lázaro, S. L. (2017). “TRP channels and pain,” in Neurobiology of TRP Channels Frontiers in Neuroscience, ed T. L. R. Emir (Boca Raton, FL: CRC Press/Taylor & Francis). Available online at: http://www.ncbi.nlm.nih.gov/books/NBK476120/ (accessed July 1, 2022).
Gouveia, D. N., Pina, L. T. S., Rabelo, T. K., da Rocha Santos, W. B., Quintans, J. S. S., and Guimaraes, A. G. (2018). Monoterpenes as perspective to chronic pain management: a systematic review. Curr. Drug Targets 19, 960–972. doi: 10.2174/1389450118666170711145308
Guimarães, A. G., Quintans, J. S. S., and Quintans, L. J. (2013). Monoterpenes with analgesic activity–a systematic review. Phytother. Res. PTR 27, 1–15. doi: 10.1002/ptr.4686
Hakimizadeh, E., Oryan, S., Hajizadeh Moghaddam, A., Shamsizadeh, A., and Roohbakhsh, A. (2012). Endocannabinoid system and TRPV1 receptors in the dorsal hippocampus of the rats modulate anxiety-like behaviors. Iran. J. Basic Med. Sci. 15, 795–802.
Heblinski, M., Santiago, M., Fletcher, C., Stuart, J., Connor, M., McGregor, I. S., et al. (2020). Terpenoids commonly found in cannabis sativa do not modulate the actions of phytocannabinoids or endocannabinoids on TRPA1 and TRPV1 channels. Cannabis Cannabinoid Res. 5, 305–317. doi: 10.1089/can.2019.0099
Heinrich, M., Kufer, J., Leonti, M., and Pardo-de-Santayana, M. (2006). Ethnobotany and ethnopharmacology–interdisciplinary links with the historical sciences. J. Ethnopharmacol. 107, 157–160. doi: 10.1016/j.jep.2006.05.035
Hilfiger, L., Triaux, Z., Marcic, C., Héberl,é, E., Emhemmed, F., Darbon, P., et al. (2021). Anti-hyperalgesic properties of menthol and pulegone. Front. Pharmacol. 12, 3248. doi: 10.3389/fphar.2021.753873
Hilfiger, L., Zhao, Q., Kerspern, D., Inquimbert, P., Andry, V., Goumon, Y., et al. (2020). A nonpeptide oxytocin receptor agonist for a durable relief of inflammatory pain. Sci. Rep. 10, 3017. doi: 10.1038/s41598-020-59929-w
Islam, A. U. S., Hellman, B., Nyberg, F., Amir, N., Jayaraj, R. L., Petroianu, G., et al. (2020). Myrcene attenuates renal inflammation and oxidative stress in the adrenalectomized rat model. Mol. Basel Switz. 25, E4492. doi: 10.3390/molecules25194492
Iwasaki, M., Lefevre, A., Althammer, F., Łapieś, O., Hilfiger, L., Kerspern, D., et al. (2022). A novel analgesic pathway from parvocellular oxytocin neurons to the periaqueductal gray. BioRxiv [Preprint]. doi: 10.1101/2022.02.23.481531
Jabba, S. V., and Jordt, S. E. (2020). “Activation of respiratory irritant receptors by pulegone, a toxic flavor chemical in mint- or menthol-flavored electronic cigarettes,” in B18. E-Cigarette and Vaping Induced Respiratory Health Outcomes - It Is Not That Simple American Thoracic Society International Conference Abstracts (American Thoracic Society), A2767.
Jankowski, M. P., Rau, K. K., and Koerber, H. R. (2017). Cutaneous TRPM8-expressing sensory afferents are a small population of neurons with unique firing properties. Physiol. Rep. 5, e13234. doi: 10.14814/phy2.13234
Julius, D. (2013). TRP channels and pain. Annu. Rev. Cell Dev. Biol. 29, 355–384. doi: 10.1146/annurev-cellbio-101011-155833
Kaimoto, T., Hatakeyama, Y., Takahashi, K., Imagawa, T., Tominaga, M., and Ohta, T. (2016). Involvement of transient receptor potential A1 channel in algesic and analgesic actions of the organic compound limonene. Eur. J. Pain Lond. Engl. 20, 1155–1165. doi: 10.1002/ejp.840
Karashima, Y., Damann, N., Prenen, J., Talavera, K., Segal, A., Voets, T., et al. (2007). Bimodal action of menthol on the transient receptor potential channel TRPA1. J. Neurosci. 27, 9874–9884. doi: 10.1523/JNEUROSCI.2221-07.2007
Kashiwadani, H., Higa, Y., Sugimura, M., and Kuwaki, T. (2021). Linalool odor-induced analgesia is triggered by TRPA1-independent pathway in mice. Behav. Brain Funct. BBF 17, 3. doi: 10.1186/s12993-021-00176-y
Kerstein, P. C., del Camino, D., Moran, M. M., and Stucky, C. L. (2009). Pharmacological blockade of TRPA1 inhibits mechanical firing in nociceptors. Mol. Pain 5, 19. doi: 10.1186/1744-8069-5-19
Khalil, M., Alliger, K., Weidinger, C., Yerinde, C., Wirtz, S., Becker, C., et al. (2018). Functional role of transient receptor potential channels in immune cells and epithelia. Front. Immunol. 9, 174. doi: 10.3389/fimmu.2018.00174
Khalil, M., Babes, A., Lakra, R., Försch, S., Reeh, P. W., Wirtz, S., et al. (2016). Transient receptor potential melastatin 8 ion channel in macrophages modulates colitis through a balance-shift in TNF-alpha and interleukin-10 production. Mucosal Immunol. 9, 1500–1513. doi: 10.1038/mi.2016.16
Kheradpezhouh, E., Choy, J. M. C., Daria, V. R., and Arabzadeh, E. (2017). TRPA1 expression and its functional activation in rodent cortex. Open Biol. 7, 160314. doi: 10.1098/rsob.160314
Kinmer, N., Özek, T., Baser, K. H. C., and Tümen, G. (1993). The essential oil of Micromeria fruticosa (L.) Druce subsp. barbata (Boiss. et Kotschy) P.H. Davis of Turkish origin. Acta Hortic. 5, 239–244. doi: 10.17660/ActaHortic.1993.333.28
Kistner, K., Siklosi, N., Babes, A., Khalil, M., Selescu, T., Zimmermann, K., et al. (2016). Systemic desensitization through TRPA1 channels by capsazepine and mustard oil - a novel strategy against inflammation and pain. Sci. Rep. 6, 28621. doi: 10.1038/srep28621
Koivisto, A.-P., Belvisi, M. G., Gaudet, R., and Szallasi, A. (2022). Advances in TRP channel drug discovery: from target validation to clinical studies. Nat. Rev. Drug Discov. 21, 41–59. doi: 10.1038/s41573-021-00268-4
Kupari, J., Usoskin, D., Parisien, M., Lou, D., Hu, Y., Fatt, M., et al. (2021). Single cell transcriptomics of primate sensory neurons identifies cell types associated with chronic pain. Nat. Commun. 12, 1510. doi: 10.1038/s41467-021-21725-z
Kwan, K. Y., Allchorne, A. J., Vollrath, M. A., Christensen, A. P., Zhang, D.-S., Woolf, C. J., et al. (2006). TRPA1 contributes to cold, mechanical, and chemical nociception but is not essential for hair-cell transduction. Neuron 50, 277–289. doi: 10.1016/j.neuron.2006.03.042
Lemon, C. H., Norris, J. E., and Heldmann, B. A. (2019). The TRPA1 ion channel contributes to sensory-guided avoidance of menthol in mice. eNeuro 6, ENEURO.0304-19.2019. doi: 10.1523/ENEURO.0304-19.2019
Lennertz, R. C., Kossyreva, E. A., Smith, A. K., and Stucky, C. L. (2012). TRPA1 Mediates Mechanical Sensitization in Nociceptors during Inflammation. PLoS ONE 7, e43597. doi: 10.1371/journal.pone.0043597
Leonti, M., and Verpoorte, R. (2017). Traditional Mediterranean and European herbal medicines. J. Ethnopharmacol. 199, 161–167. doi: 10.1016/j.jep.2017.01.052
Li, Y., Chen, X., Nie, Y., Tian, Y., Xiao, X., and Yang, F. (2021). Endocannabinoid activation of the TRPV1 ion channel is distinct from activation by capsaicin. J. Biol. Chem. 297, 101022. doi: 10.1016/j.jbc.2021.101022
Liu, B., Fan, L., Balakrishna, S., Sui, A., Morris, J. B., and Jordt, S.-E. (2013). TRPM8 is the principal mediator of menthol-induced analgesia of acute and inflammatory pain. Pain 154, 2169–2177. doi: 10.1016/j.pain.2013.06.043
Mackenzie, J. S., and Jeggo, M. (2019). The one health approach—why is it so important? Trop. Med. Infect. Dis. 4, 88. doi: 10.3390/tropicalmed4020088
Majikina, A., Takahashi, K., Saito, S., Tominaga, M., and Ohta, T. (2018). Involvement of nociceptive transient receptor potential channels in repellent action of pulegone. Biochem. Pharmacol. 151, 89–95. doi: 10.1016/j.bcp.2018.02.032
Maroon, J. C., Bost, J. W., and Maroon, A. (2010). Natural anti-inflammatory agents for pain relief. Surg. Neurol. Int. 1, 80. doi: 10.4103/2152-7806.73804
Mattioli, P. A. (1590). Kreutterbuch. Available online at: https://docnum.unistra.fr/digital/collection/coll13/id/4668/rec/436 (accessed February 11, 2022).
Menigoz, A., and Boudes, M. (2011). The expression pattern of TRPV1 in brain. J. Neurosci. 31, 13025–13027. doi: 10.1523/JNEUROSCI.2589-11.2011
Mihara, S., and Shibamoto, T. (2015). The role of flavor and fragrance chemicals in TRPA1 (transient receptor potential cation channel, member A1) activity associated with allergies. Allergy Asthma Clin. Immunol. 11, 11. doi: 10.1186/s13223-015-0074-0
Mizumura, K. (1997). Peripheral mechanism of hyperalgesia–sensitization of nociceptors. Nagoya J. Med. Sci. 60, 69–87.
Morelli, M. B., Amantini, C., Liberati, S., Santoni, M., and Nabissi, M. (2013). TRP channels: new potential therapeutic approaches in CNS neuropathies. CNS Neurol. Disord. Drug Targets 12, 274–293. doi: 10.2174/18715273113129990056
Neogi, T. (2013). The epidemiology and impact of pain in osteoarthritis. Osteoarthritis Cartilage 21, 1145–1153. doi: 10.1016/j.joca.2013.03.018
Ntalli, N. G., Ferrari, F., Giannakou, I., and Menkissoglu-Spiroudi, U. (2010). Phytochemistry and nematicidal activity of the essential oils from 8 Greek Lamiaceae aromatic plants and 13 terpene components. J. Agric. Food Chem. 58, 7856–7863. doi: 10.1021/jf100797m
Ohtsubo, S., Fujita, T., Matsushita, A., and Kumamoto, E. (2015). Inhibition of the compound action potentials of frog sciatic nerves by aroma oil compounds having various chemical structures. Pharmacol. Res. Perspect. 3, e00127. doi: 10.1002/prp2.127
Oltean, H., Robbins, C., van Tulder, M. W., Berman, B. M., Bombardier, C., and Gagnier, J. J. (2014). Herbal medicine for low-back pain. Cochrane Database Syst. Rev. 2014, CD004504. doi: 10.1002/14651858.CD004504.pub4
Opioid Overdose Crisis | National Institute on Drug Abuse (2022). NIDA. Available online at: https://nida.nih.gov/drug-topics/opioids/opioid-overdose-crisis (accessed April 27, 2022).
Ordás, P., Hernández-Ortego, P., Vara, H., Fernández-Peña, C., Reimúndez, A., Morenilla-Palao, C., et al. (2021). Expression of the cold thermoreceptor TRPM8 in rodent brain thermoregulatory circuits. J. Comp. Neurol. 529, 234–256. doi: 10.1002/cne.24694
Ortar, G., Morera, L., Moriello, A. S., Morera, E., Nalli, M., Di Marzo, V., et al. (2012). Modulation of thermo-transient receptor potential (thermo-TRP) channels by thymol-based compounds. Bioorg. Med. Chem. Lett. 22, 3535–3539. doi: 10.1016/j.bmcl.2012.03.055
Oz, M., El Nebrisi, E. G., Yang, K.-H. S., Howarth, F. C., and Al Kury, L. T. (2017). Cellular and molecular targets of menthol actions. Front. Pharmacol. 8, 472. doi: 10.3389/fphar.2017.00472
Peier, A. M., Moqrich, A., Hergarden, A. C., Reeve, A. J., Andersson, D. A., Story, G. M., et al. (2002). A TRP channel that senses cold stimuli and menthol. Cell 108, 705–715. doi: 10.1016/S0092-8674(02)00652-9
Perri, F., Coricello, A., and Adams, J. D. (2020). Monoterpenoids: the next frontier in the treatment of chronic pain? Journals 3, 195–214. doi: 10.3390/j3020016
Petitjean, H., Hugel, S., Barthas, F., Bohren, Y., Barrot, M., Yalcin, I., et al. (2014). Activation of transient receptor potential vanilloid 2-expressing primary afferents stimulates synaptic transmission in the deep dorsal horn of the rat spinal cord and elicits mechanical hyperalgesia. Eur. J. Neurosci. 40, 3189–3201. doi: 10.1111/ejn.12688
POWO (2022). Plants of the World Online. Facilitated by the Royal Botanic Gardens, Kew. Available online at: http://www.plantsoftheworldonline.org/ (accessed May 10, 2022).
Quintans, J. S. S., Shanmugam, S., Heimfarth, L., Araújo, A. A. S., Almeida, J. R. G., da, S., et al. (2019). Monoterpenes modulating cytokines - a review. Food Chem. Toxicol. Int. J. Publ. Br. Ind. Biol. Res. Assoc. 123, 233–257. doi: 10.1016/j.fct.2018.10.058
Raja, S. N., Carr, D. B., Cohen, M., Finnerup, N. B., Flor, H., Gibson, S., et al. (2020). The revised International Association for the Study of Pain definition of pain: concepts, challenges, and compromises. Pain 161, 1976–1982. doi: 10.1097/j.pain.0000000000001939
Reyes-García, V., Benyei, P., Aceituno-Mata, L., Gras, A., Molina, M., Tardío, J., et al. (2021). Documenting and protecting traditional knowledge in the era of open science: Insights from two Spanish initiatives. J. Ethnopharmacol. 278, 114295. doi: 10.1016/j.jep.2021.114295
Rufino, A. T., Ribeiro, M., Judas, F., Salgueiro, L., Lopes, M. C., Cavaleiro, C., et al. (2014). Anti-inflammatory and chondroprotective activity of (+)-α-pinene: structural and enantiomeric selectivity. J. Nat. Prod. 77, 264–269. doi: 10.1021/np400828x
Ruparel, N. B., Patwardhan, A. M., Akopian, A. N., and Hargreaves, K. M. (2011). Desensitization of transient receptor potential ankyrin 1 (TRPA1) by the TRP vanilloid 1-selective cannabinoid arachidonoyl-2 chloroethanolamine. Mol. Pharmacol. 80, 117–123. doi: 10.1124/mol.110.068940
Rutz, A., Sorokina, M., Galgonek, J., Mietchen, D., Willighagen, E., Gaudry, A., et al. (2021). The lotus initiative for open natural products research: Knowledge management through wikidata. BioRxiv [Preprint]. doi: 10.1101/2021.02.28.433265
Schultz, F., Dworak-Schultz, I., Olengo, A., Anywar, G., and Garbe, L.-A. (2021). Transferring ethnopharmacological results back to traditional healers in rural indigenous communities – the Ugandan greater Mpigi region example: research translation. Video J. Educ. Pedagogy 6, 1–15. doi: 10.1163/23644583-bja10018
Schumacher, M. A. (2010). Transient receptor potential channels in pain and inflammation: therapeutic opportunities. Pain Pract. 10, 185–200. doi: 10.1111/j.1533-2500.2010.00358.x
Shigetomi, E., Tong, X., Kwan, K. Y., Corey, D. P., and Khakh, B. S. (2011). TRPA1 channels regulate astrocyte resting calcium and inhibitory synapse efficacy through GAT-3. Nat. Neurosci. 15, 70–80. doi: 10.1038/nn.3000
Soleimani, M., Sheikholeslami, M. A., Ghafghazi, S., Pouriran, R., and Parvardeh, S. (2019). Analgesic effect of α-terpineol on neuropathic pain induced by chronic constriction injury in rat sciatic nerve: involvement of spinal microglial cells and inflammatory cytokines. Iran. J. Basic Med. Sci. 22, 1445–1451. doi: 10.22038/IJBMS.2019.14028
Sorokina, M., and Steinbeck, C. (2020). Review on natural products databases: where to find data in 2020. J. Cheminformatics 12, 20. doi: 10.1186/s13321-020-00424-9
Sousa, D., and Pergentino, D. (2011). Analgesic-like activity of essential oils constituents. Molecules 16, 2233–2252. doi: 10.3390/molecules16032233
Souza Monteiro de Araujo, D., Nassini, R., Geppetti, P., and De Logu, F. (2020). TRPA1 as a therapeutic target for nociceptive pain. Expert Opin. Ther. Targets 24, 997–1008. doi: 10.1080/14728222.2020.1815191
Sun, F.-J., Guo, W., Zheng, D.-H., Zhang, C.-Q., Li, S., Liu, S.-Y., et al. (2013). Increased expression of TRPV1 in the cortex and hippocampus from patients with mesial temporal lobe epilepsy. J. Mol. Neurosci. 49, 182–193. doi: 10.1007/s12031-012-9878-2
Takaishi, M., Uchida, K., Fujita, F., and Tominaga, M. (2014). Inhibitory effects of monoterpenes on human TRPA1 and the structural basis of their activity. J. Physiol. Sci. 64, 47–57. doi: 10.1007/s12576-013-0289-0
Tetali, S. D. (2018). Terpenes and isoprenoids: a wealth of compounds for global use. Planta 249, 1–8. doi: 10.1007/s00425-018-3056-x
Topp, R., Brosky, J. A., and Pieschel, D. (2013). The effect of either topical menthol or a placebo on functioning and knee pain among patients with knee OA. J. Geriatr. Phys. Ther. 36, 92–99. doi: 10.1519/JPT.0b013e318268dde1
Totelin, L. M. V. (2006). Review of: Théophraste. Recherches sur les plantes. Tome V. Livre IX. Bryn Mawr Class. Rev. Available online at: https://bmcr.brynmawr.edu/2006/2006.07.24/ (accessed January 8 2022).
Treede, R.-D., Rief, W., Barke, A., Aziz, Q., Bennett, M., Benoliel, R., et al. (2019). Chronic pain as a symptom or a disease: the IASP classification of chronic pain for the international classification of diseases (ICD-11). Pain 160, 19–27. doi: 10.1097/j.pain.0000000000001384
UNESCO World Heritage Center (2010). n°56 - World Heritage Review n°56 < br /> Biodiversity. UNESCO World Herit. Cent. Available online at: https://whc.unesco.org/en/review/56/ (accessed May 12, 2022).
UNESCO. (2018). UNESCO's Commitment to Biodiversity: Connecting People and Nature For An Inspiring Future. Available online at: https://unesdoc.unesco.org/ark:/48223/pf0000265200 (accessed May 12, 2022).
Vilceanu, D., and Stucky, C. L. (2010). TRPA1 mediates mechanical currents in the plasma membrane of mouse sensory neurons. PLoS ONE 5, e12177. doi: 10.1371/journal.pone.0012177
Wahis, J., Baudon, A., Althammer, F., Kerspern, D., Goyon, S., Hagiwara, D., et al. (2021). Astrocytes mediate the effect of oxytocin in the central amygdala on neuronal activity and affective states in rodents. Nat. Neurosci. 24, 529–541. doi: 10.1038/s41593-021-00800-0
Wang, S., Zhang, D., Hu, J., Jia, Q., Xu, W., Su, D., et al. (2017). A clinical and mechanistic study of topical borneol-induced analgesia. EMBO Mol. Med. 9, 802–815. doi: 10.15252/emmm.201607300
Weston-Green, K. (2019). “The united chemicals of cannabis: beneficial effects of cannabis phytochemicals on the brain and cognition,” in Recent Advances in Cannabinoid Research, eds W. J. Costain and R. B. Laprairie (Wollongong, NSW: IntechOpen).
Wojtunik-Kulesza, K. A. (2022). Toxicity of selected monoterpenes and essential oils rich in these compounds. Mol. Basel Switz. 27, 1716. doi: 10.3390/molecules27051716
World Health Organization (2013). WHO Traditional Medicine Strategy: 2014-2023. World Health Organization. Available online at: https://apps.who.int/iris/handle/10665/92455 (accessed May 10, 2022).
Xiao, B., Dubin, A. E., Bursulaya, B., Viswanath, V., Jegla, T. J., and Patapoutian, A. (2008). Identification of transmembrane domain 5 as a critical molecular determinant of menthol sensitivity in mammalian TRPA1 channels. J. Neurosci. 28, 9640–9651. doi: 10.1523/JNEUROSCI.2772-08.2008
Xu, H., Blair, N. T., and Clapham, D. E. (2005). Camphor activates and strongly desensitizes the transient receptor potential vanilloid subtype 1 channel in a vanilloid-independent mechanism. J. Neurosci. 25, 8924–8937. doi: 10.1523/JNEUROSCI.2574-05.2005
Yamada, Y., Kuzuyama, T., Komatsu, M., Shin-Ya, K., Omura, S., Cane, D. E., et al. (2015). Terpene synthases are widely distributed in bacteria. Proc. Natl. Acad. Sci. U. S. A. 112, 857–862. doi: 10.1073/pnas.1422108112
Yu, L., Yang, F., Luo, H., Liu, F.-Y., Han, J.-S., Xing, G.-G., et al. (2008). The role of TRPV1 in different subtypes of dorsal root ganglion neurons in rat chronic inflammatory nociception induced by complete Freund's adjuvant. Mol. Pain 4, 61. doi: 10.1186/1744-8069-4-61
Zhang, Q., Wu, D., Wu, J., Ou, Y., Mu, C., Han, B., et al. (2015). Improved blood-brain barrier distribution: effect of borneol on the brain pharmacokinetics of kaempferol in rats by in vivo microdialysis sampling. J. Ethnopharmacol. 162, 270–277. doi: 10.1016/j.jep.2015.01.003
Zhu, X., Wang, G., Wu, S., and Li, C. (2020). Protective effect of D-carvone against dextran sulfate sodium induced ulcerative colitis in Balb/c mice and LPS induced RAW cells via the inhibition of COX-2 and TNF-α. J. Environ. Pathol. Toxicol. Oncol. 39, 235–245. doi: 10.1615/JEnvironPatholToxicolOncol.2020031860
Keywords: monoterpenes, pain, inflammation, TRP, pulegone, menthol, hyperalgesia
Citation: Petitjean H, Héberlé E, Hilfiger L, Łapieś O, Rodrigue G and Charlet A (2022) TRP channels and monoterpenes: Past and current leads on analgesic properties. Front. Mol. Neurosci. 15:945450. doi: 10.3389/fnmol.2022.945450
Received: 16 May 2022; Accepted: 04 July 2022;
Published: 29 July 2022.
Edited by:
Cyril Goudet, INSERM U1191 Institut de Génomique Fonctionnelle (IGF), FranceReviewed by:
Livio Luongo, University of Campania Luigi Vanvitelli, ItalyCopyright © 2022 Petitjean, Héberlé, Hilfiger, Łapieś, Rodrigue and Charlet. This is an open-access article distributed under the terms of the Creative Commons Attribution License (CC BY). The use, distribution or reproduction in other forums is permitted, provided the original author(s) and the copyright owner(s) are credited and that the original publication in this journal is cited, in accordance with accepted academic practice. No use, distribution or reproduction is permitted which does not comply with these terms.
*Correspondence: Alexandre Charlet, YWNoYXJsZXRAdW5pc3RyYS5mcg==; Hugues Petitjean, aHVndWVzLnBldGl0amVhbkBiZW5lcGh5dC5mcg==
†These authors have contributed equally to this work