- 1LSU Neuroscience Center, Louisiana State University Health Science Center, New Orleans, LA, United States
- 2Department of Ophthalmology, Louisiana State University Health Science Center, New Orleans, LA, United States
- 3Department Neurology, Louisiana State University Health Science Center, New Orleans, LA, United States
Introduction
A small family of NF-kB (p50/p65)-regulated microRNAs (miRNAs) has been identified and partially characterized in Alzheimer's disease (AD) brain in the hippocampal CA1 and temporal lobe neocortical regions, and in reactive oxygen species (ROS)-, cytokine interleukin 1-beta (IL-1β)-, amyloid beta 42 (Aβ42) peptide- and/or lipopolysaccharide (LPS)-stressed human neuronal-glial (HNG) cells in primary co-culture. This microRNA family currently includes miRNA-9, miRNA-30b, miRNA-34a, miRNA-125b, miRNA-146a and miRNA-155. Other brain-enriched miRNA species may be involved. Experimental evidence suggests that in neurological diseases, the upregulation of this small pro-inflammatory miRNA family orchestrates a pathogenic gene expression program that can explain many aspects of AD onset, and propagation and severity of the disease including failure of microglial-mediated clearance of amyloid-beta (Aβ) and other end-stage peptides from brain cells, amyloidogenesis, astrogliosis, deficits in neurotropism, neuronal cell atrophy, and downregulation in the production of essential cytoskeletal components and synaptic signaling elements. This opinion article will contribute to the interpretation of the most recent findings in the current research area involving NF-kB-regulated miRNA-mediated signaling pathways, and how this information may aid in the advancement of therapeutic strategies for more effective clinical management of AD and other progressive age-related and lethal neurodegenerative disorders.
Alzheimer's Disease (AD): Epidemiology and Basic Features
AD is a slowly developing, irreversible, progressive, and inflammatory neurodegeneration of the human brain, central nervous system (CNS), and neural vasculature (Alzheimer et al., 1995; Lane et al., 2018; Trejo-Lopez et al., 2021). Due in part to the aging population and demographics, the global incidence and prevalence of AD are sharply increasing, and AD currently represents the largest single cause of age-related memory impairment and behavioral and cognitive decline in Westernized societies. Current estimates put the global incidence of AD at about ~55 million, and this number is expected to reach epidemic proportions and rise to about ~150 million cases by the year 2050 (Tahami Monfared et al., 2022; https://alz-journals.onlinelibrary.wiley.com/doi/full/10.1002/alz.12638; https://www.alz.org/media/documents/Alzheimers-facts-and-figures.pdf; https://www.Alzint.org/about/dementia-facts-figures/dementia-statistics/last accessed 13 June 2022). AD places a tremendous socioeconomic burden on caregivers and on the healthcare system as a whole. Despite ~115 years of intense and directed research, no effective treatment or cure for AD exists, although many insights into the molecular-genetic nature of this age-related disease have been established (Alzheimer et al., 1995; Kaur et al., 2015; Lane et al., 2018; Dong et al., 2021; Hermans et al., 2021; Trejo-Lopez et al., 2021; Liu et al., 2022; Olufunmilayo and Holsinger, 2022; Pogue et al., 2022; Rastegar-Moghaddam et al., 2022; Singh et al., 2022; Tahami Monfared et al., 2022; Yoon et al., 2022). As a devastating, inflammatory, and terminal neurodegenerative disorder, one molecular genetic component that has emerged at the forefront of current AD research is the DNA-binding element NF-kB, probably the single most important transcription factor yet identified in the complex neuropathology of AD. This opinion article will address some recently described effects of NF-kB on a small family of regulatory miRNAs observed to be significantly altered in abundance, complexity and speciation in AD-affected brain.
NF-κB-Sensitive microRNAs and Inflammatory Signaling in Alzheimer's Disease (AD)
Discovered about ~36 years ago, the heterodimeric transcription factor NF-kB (p50/p65) was first described as a rapidly inducible nuclear factor κ-light-chain transcription enhancer of activated B cell lymphocytes in part responsible for the humoral immunity component of the immune system (Sen and Baltimore, 1986; Kaltschmidt et al., 1993; Taganov et al., 2006). Since then, recognition sites for this redox-controlled transcriptional activator and DNA-binding protein have been found to occur in multiple gene promoters, and it is now known to drive a broad range of gene expression patterns in diverse cellular types involved in host innate- and adaptive-immune functions with important roles in the clearance of waste molecules from the cytoplasm, inflammatory signaling, differentiation, cell growth, tumorigenesis and neurodegeneration (Taganov et al., 2006; Zhang et al., 2017; Baltimore, 2019). In resting cells the pre-formed NF-kB (p50/p65; 115 kDa) heterodimer is bound to a hydrophobic inhibitory kappa B protein (IkB, 36 kDa) that prevents free NF-kB from promoter-DNA-binding and gene transactivation. However NF-κB can be rapidly induced in all cell types by treating them with pro-inflammatory mediators that include interleukin-1beta (IL-1β), interleukin 6 (IL-6), tumor necrosis factor alpha (TNFα), and endotoxins such as lipopolysaccharides (LPS), an intensely inflammatory lipoprotein produced exclusively by Gram-negative bacteria, Aβ peptides, viral gene products, ultraviolet and other ionizing irradiation, and reactive oxygen species (ROS)-generating and/or oxidizing compounds. Exposure to these factors induces IkB decomposition triggered through a site-specific phosphorylation of an IκB kinase (IKK) complex, followed by degradation via the ubiquitin-proteasome system, and then rapid NF-kB-activated transcription by multiple pro-inflammatory NF-kB-sensitive gene promoters. The human brain and CNS contain a very active, pleiotropic NF-kB-signaling system that has a function in both neurological health and disease (Kaltschmidt and Kaltschmidt, 2009; Christian et al., 2016; Liu et al., 2022; Yoon et al., 2022). While the NF-kB (p50/p65; also known as p50/RelA) heterodimer is especially abundant in nervous tissues, other homo- or hetero-dimeric NF-kB transcription factor complexes containing at least 5 other molecular components that include RelA, RelB, c-Rel, p50, p52, and NFKB1 (p105) form at least 12 different identified NF-kB complexes. All share the conserved Rel homology domain (RHD) responsible for DNA binding, dimerization, and association with the repressor protein IκB (Zhang et al., 2017; Pires et al., 2018; Baltimore, 2019; https://www.genecards.org/cgi-bin/carddisp.pl?gene=RELA; last accessed 25 May 2022). It has been repeatedly demonstrated: (i) that activated NF-kB subsequently upregulates a select sub-group of disease-associated miRNAs comprising a well-characterized NF-kB-sensitive Homo sapiens-enriched family of potentially pathogenic miRNAs that include miRNA-9, miRNA-30b, miRNA-34a, miRNA-146a, and miRNA-155 normally involved in immunity, inflammation, and brain cell genetic function in the CNS (Lukiw, 2012, 2020; Brennan et al., 2019; Juźwik et al., 2019; Barnabei et al., 2021; Song et al., 2021; Yoon et al., 2022); (ii) that the same miRNAs are upregulated in AD brain; (iii) that this group of significantly over-expressed miRNAs are abundant in progressive and often lethal viral and prion-mediated and/or related neurological syndromes associated with progressive inflammatory neurodegeneration; and (iv) that all of these NF-kB-sensitive endotoxin-responsive miRNA genes have as many as three tandem, functional NF-kB binding sites stacked in their immediate 5'-promoter region, making this miRNA gene family exceptionally sensitive to NF-kB activation and NF-kB-mediated transcriptional upregulation (Taganov et al., 2006; Lukiw et al., 2008; Alexandrov et al., 2019; Jauhari et al., 2020; Table 1). Interestingly, there is also abundant recent evidence (i) that endotoxins such as LPS and other biophysical-barrier-penetrating glycolipids and lipoproteins specifically induce neurodegeneration by promoting neuro-inflammatory signaling by stimulation of Toll-like receptors (TLRs) present on the outer membranes of glial cells of the CNS (Kumar, 2019; Singh et al., 2022); (ii) that extremely potent pro-inflammatory species of LPSs have been found by many independent groups to be localized in human brain neurons in AD brain (Zhan et al., 2018, 2021; Alexandrov et al., 2019; Zhao et al., 2019a; Singh et al., 2022); and (iii) that a life-long supply of microbiome-abundant Gram-negative bacteria-derived LPS would be available over the long-term to chronically upregulate NF-kB in the brain and CNS, as is observed in LPS-treated human neurons in primary culture and in AD temporal lobe neocortex, the latter an anatomical area targeted by the AD process (Lukiw and Bazan, 1998; Zhang et al., 2017; Zhan et al., 2018, 2021; Zhao and Lukiw, 2018; Alexandrov et al., 2019; Zhao et al., 2019a; Singh et al., 2022).
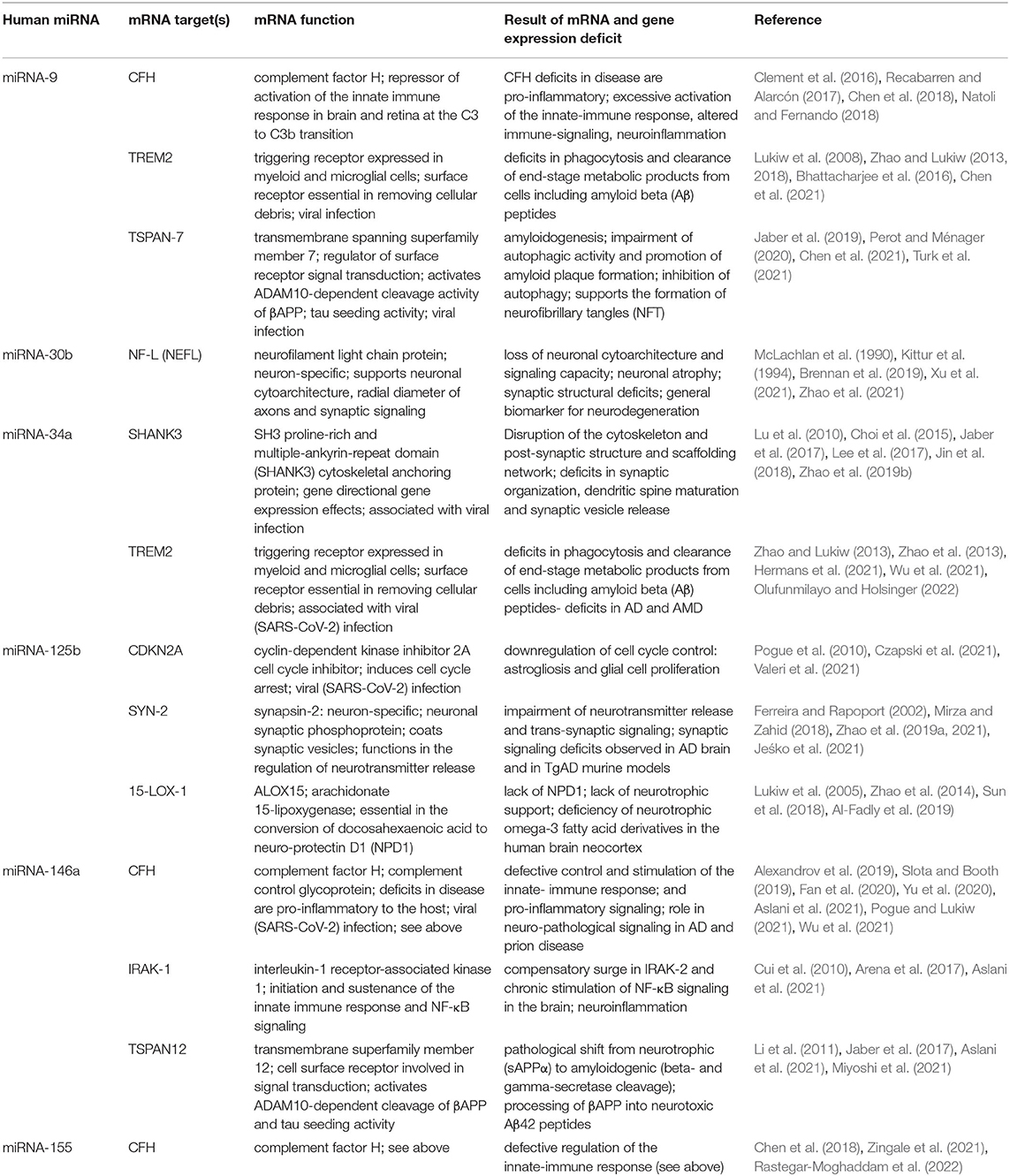
Table 1. NF-kB-induced microRNAs (miRNAs), their mRNA targets, functions, and pathways in Alzheimer's disease (AD)-affected brain; all miRNAs listed show: (i) significant upregulation following NF-kB (p50/p65) activation; (ii) are potentially pathogenic and are upregulated in AD brain, AD cell culture, and transgenic murine models of AD (TgAD); and (iii) have been shown to interactively contribute to the AD process; some closely related work on the functions these miRNAs in neuronal cell culture, TgAD models, and other human neurodegenerative diseases have also been included in the References.
Therapeutic Strategies Directed Against NF-kB and/or Pro-Inflammatory miRNA Signaling
Because NF-kB signaling pathways appear to be centrally involved in a great many immune- and inflammation-linked human diseases from carcinogenesis to neurodegeneration, a large number of natural and synthetic NF-kB inhibitors have been designed and tested, and are under very active investigation to limit the extent of NF-kB activation in the cellular environment. The evolution of one single high-potency gene-activating transcription factor in overlapping signaling pathways has made it challenging to find biologically active inhibitory molecules that can interfere with and/or block specific signaling pathways that lead to NF-κB activation without multiple off-target effects or other complicating factors. Even so, a number of NF-kB modulator and blocking strategies have been adopted or are undergoing rigorous evaluation in both research laboratories and in clinical settings. In general, therapeutic NF-kB inhibition can occur via four basic mechanistic strategies: (i) by blockage of the original physiological stimulating signals that drive NF-kB activation; (ii) by targeting phosphorylation pathways associated with NF-kB activation because NF-κB phosphorylation controls transcription in a gene-specific manner; (iii) by modulation or activation of the IkB complex or other NF-kB subunits; and/or (iv) by blockage of NF-kB translocation, DNA sequence recognition, and binding and/or modification of NF-kB that affects its activity or target specificity. These approaches include but are not limited to the following strategies, most of which are under very active research and therapeutic development: (i) antioxidant approaches that neutralize the primary NF-kB activation signals such as quenching of reactive oxygen species (ROS) and other oxidizing and/or redox compounds (Kaur et al., 2015; Barnabei et al., 2021; Jover-Mengual et al., 2021; Pogue and Lukiw, 2021); (ii) alteration of multiple phosphorylation events that disrupt the activation of the multi-subunit NF-kB transcription complex and alter the interaction of these phosphorylation sites that ultimately determines the selectivity of NF-κB effects on transcriptional activity (by advanced investigation of these highly interactive phosphorylation sites and mechanisms it should be possible to modulate or block many aspects of phosphorylation-mediated NF-kB activation; Christian et al., 2016; https://360researchreports.com/global-nf-kb-inhibitors-market-20149725); (iii) as previously pointed out, because active NF-kB complexes are assembled from various combinations of RelA, RelB, c-Rel, p50, p52, NFKB1 (p105) etc., it may be possible to specifically block the generation and/or assembly of a single monomeric species of NF-kB by limiting the abundance of one of the subunits of NF-kB by genome editing, including knock-out technologies and/or the Cas9/CRISPR editing system (Wang et al., 2018; Dai et al., 2020; Katti et al., 2022); (iv) NF-kB is directed to bind to its genomic targets by topological features located in certain promoter DNA sequences; therefore, these highly specific promoter DNA binding sites can be masked, blocked, or modulated using genome blocking technologies involving small non-coding RNA (sncRNA), NF-kB decoy sequence, and/or Cas9/CRISPR editing strategies (Taganov et al., 2006; Christian et al., 2016; Zhang et al., 2017; Baltimore, 2019; Dai et al., 2020; Katti et al., 2022; Yoon et al., 2022); (v) specific upregulated miRNA abundance and speciation may be blocked or modulated using chemically stabilized anti-miRNA strategies or by targeting miRNA processing enzymes, thus preventing the creation of a fully active and/or biologically available miRNA species; (vi) directed delivery systems to the brain and/or CNS or other tissue and/or organ systems to minimize unwarranted off-target effects; (vii) long-term systemic ingestion of low-dose NF-κB inhibitors including dietary-administered flavonoids, lignans, diterpenes and sesquiterpenes, saponins, polysaccharides, polyphenols, biological fiber, and other natural products that may have general beneficial effects on reducing pro-inflammatory signaling, carcinogenesis, and neurodegeneration by chronic dampening of NF-κB activities. These have been used for millennia in the pharmacopeia associated with ethnic biomedical-treatment approaches (Gilmore and Herscovitch, 2006; Li et al., 2020a,b; Olajide and Sarker, 2020; Uddin et al., 2021; Al-Khayri et al., 2022; Das et al., 2022); and/or (viii) any combination of these therapeutic strategies. Regarding the creation and testing of synthetic NF-kB modulatory or blocking compounds, about ~80 pharmaceutical companies are currently in the pursuit of more effective, directed and specifically targeted NF-kB inhibitors for clinical application in human diseases involving excessive NF-kB activation and signaling (https://www.futuremarketinsights.com/reports/nf-kb-inhibitors-market; https://www.Globenewswire.com/news-release/2020/12/08/2141 093/0/en/NF-kappa-B-Inhibitors-Therapeutics-pipeline-analysis-of-80-Companies.html; https://360researchreports.com/global-nf-kb-inhibitors-market- 20149725; last accessed 25 May 2022).
Discussion
The pleiotropic homo- or hetero-dimeric transcription factor NF-kB is a master regulator of the innate immune system, inflammatory responses, and neurotropic, cytoskeletal, synaptic, phagocytic, and synaptic deficit characteristics of the AD-affected brain and related neurodegenerative disorders. One critically important regulatory action of the NF-kB complex is to upregulate a small family of pathogenic miRNAs in the brain and CNS, which in turn targets and downregulates a group of mRNAs whose downregulation is decisive in driving the neuropathology of AD (Table 1). It is our opinion that these NF-kB-regulated miRNA-mRNA linked pathological interactions will provide a wealth of targets for therapeutics and disease intervention. The merging of experimental and human clinical trials will be decisive in the strategic design and application of NF-kB and NF-kB-miRNA-induced modulatory agents and drugs useful in the more efficacious treatment of the current AD epidemic.
Author Contributions
WL researched, compiled data, and wrote the paper. WL approved and submitted the final version.
Funding
Research involving small non-coding RNA (sncRNA) and miRNA signaling, including NF-kB (p50/p65)-mediated miRNA signaling, the innate-immune response, amyloidogenesis and neuro-inflammation in AD and prion disease, contributions of the microbiome-derived neurotoxins such as lipopolysaccharide (LPS), reactive oxygen species (ROS), anti-NF-kB and/or anti-miRNA therapeutic strategies was supported through the Louisiana Biotechnology Research Network (LBRN), an unrestricted grant to the LSU Eye Center from Research to Prevent Blindness (RPB), The Brown Foundation, Joe and Dorothy Dorsett Innovation in Science Healthy Aging Award; and National Institutes of Health (NIH) NIA Grants AG18031 and AG038834.
Conflict of Interest
The author declares that the research was conducted in the absence of any commercial or financial relationships that could be construed as a potential conflict of interest.
Publisher's Note
All claims expressed in this article are solely those of the authors and do not necessarily represent those of their affiliated organizations, or those of the publisher, the editors and the reviewers. Any product that may be evaluated in this article, or claim that may be made by its manufacturer, is not guaranteed or endorsed by the publisher.
References
Alexandrov, P., Zhao, Y., Li, W., and Lukiw, W. J. (2019). Lipopolysaccharide-stimulated, NF-kB-, miRNA-146a- and miRNA-155-mediated molecular-genetic communication between the human gastrointestinal tract microbiome and the brain. Folia Neuropathol. 57, 211–219. doi: 10.5114/fn.2019.88449
Al-Fadly, E. D., Elzahhar, P. A., Tramarin, A., Elkazaz, S., Shaltout, H., Abu-Serie, M. M., et al. (2019). Tackling neuroinflammation and cholinergic deficit in Alzheimer's disease: multi-target inhibitors of cholinesterases, cyclooxygenase-2 and 15-lipoxygenase. Eur. J. Med. Chem. 167, 161–186. doi: 10.1016/j.ejmech.2019.02.012
Al-Khayri, J. M., Sahana, G. R., Nagella, P., Joseph, B. V., Alessa, F. M., and Al-Mssallem, M. Q. (2022). Flavonoids as potential anti-inflammatory molecules: a review. Molecules. 27, 2901. doi: 10.3390/molecules27092901
Alzheimer, A., Stelzmann, R. A., Schnitzlein, H. N., and Murtagh, F. R. (1995). An English translation of Alzheimer's 1907 paper, “Uber eine eigenartige Erkankung der Hirnrinde”. Clin. Anat. 8, 429–431. doi: 10.1002/ca.980080612
Arena, A., Iyer, A. M., Milenkovic, I., Kovacs, G. G., Ferrer, I., Perluigi, M., et al. (2017). Developmental expression and dysregulation of miRNA-146a and miRNA-155 in Down's syndrome and mouse models of down's syndrome and Alzheimer's disease. Curr. Alzheimer's Res. 14, 1305–1317. doi: 10.2174/1567205014666170706112701
Aslani, M., Mortazavi-Jahromi, S. S., and Mirshafiey, A. (2021). Efficient roles of miRNA-146a in cellular and molecular mechanisms of neuroinflammatory disorders: An effectual review in neuroimmunology. Immunol. Lett. 238, 1–20. doi: 10.1016/j.imlet.2021.07.004
Baltimore, D. (2019). Sixty years of discovery. Annu. Rev. Immunol. 37, 1–17. doi: 10.1146/annurev-immunol-042718-041210
Barnabei, L., Laplantine, E., Mbongo, W., Rieux-Laucat, F., and Weil, R. (2021). NF-κB: at the borders of autoimmunity and inflammation. Front. Immunol. 12, 716469. doi: 10.3389/fimmu.2021.716469
Bhattacharjee, S., Zhao, Y., Dua, P., Rogaev, E. I., and Lukiw, W. J. (2016). microRNA-34a-mediated down-regulation of the microglial-enriched triggering receptor and phagocytosis-sensor TREM2 in age-related macular degeneration. PLoS One. 11, e0150211. doi: 10.1371/journal.pone.0150211
Brennan, S., Keon, M., Liu, B., Su, Z., and Saksena, N. K. (2019). Panoramic visualization of circulating microRNAs across neurodegenerative diseases in humans. Mol. Neurobiol. 56, 7380–7407. doi: 10.1007/s12035-019-1615-1
Chen, J., Qi, Y., Liu, C. F., Lu, J. M., Shi, J., and Shi, Y. (2018). microRNA expression data analysis to identify key miRNAs associated with Alzheimer's disease. J. Gene Med. 20, e3014. doi: 10.1002/jgm.3014
Chen, M. L., Hong, C. G., Yue, T., Li, H. M., Duan, R., Hu, W. B., et al. (2021). Inhibition of miR-331-3p and miR-9-5p ameliorates Alzheimer's disease by enhancing autophagy. Theranostics 11, 2395–2409. doi: 10.7150/thno.47408
Choi, S. Y., Pang, K., Kim, J. Y., Ryu, J. R., Kang, H., and Liu, Z. (2015). Post-transcriptional regulation of SHANK3 expression by microRNAs related to multiple neuropsychiatric disorders. Mol. Brain. 8, 74. doi: 10.1186/s13041-015-0165-3
Christian, F., Smith, E. L., and Carmody, R. J. (2016). The regulation of NF-κB subunits by phosphorylation. Cells 5, 12. doi: 10.3390/cells5010012
Clement, C., Hill, J. M., Dua, P., Culicchia, F., and Lukiw, W. J. (2016). Analysis of RNA from Alzheimer's disease post-mortem brain tissues. Mol. Neurobiol. 53, 1322–1328. doi: 10.1007/s12035-015-9105-6
Cui, J. G., Li, Y. Y., Zhao, Y., Bhattacharjee, S., and Lukiw, W. J. (2010). Differential regulation of interleukin-1 receptor-associated kinase-1 (IRAK-1) and IRAK-2 by microRNA-146a and NF-kB in stressed human astroglial cells and in Alzheimer disease. J. Biol. Chem. 285, 38951–38960. doi: 10.1074/jbc.M110.178848
Czapski, G. A., Cieślik, M., Białopiotrowicz, E., Lukiw, W. J., and Strosznajder, J. B. (2021). Down-regulation of cyclin D2 in amyloid β toxicity, inflammation, and Alzheimer's disease. PLoS ONE 16, e0259740. doi: 10.1371/journal.pone.0259740
Dai, W., Wu, J., Wang, D., and Wang, J. (2020). Cancer gene therapy by NF-κB-activated cancer cell-specific expression of CRISPR/Cas9 targeting telomeres. Gene Ther. 27, 266–280. doi: 10.1038/s41434-020-0128-x
Das, R., Mehta, D. K., and Dhanawat, M. (2022). Medicinal plants in cancer treatment: contribution of nuclear factor-kappa B (NF-kB) inhibitors. Mini. Rev. Med. Chem. doi: 10.2174/1389557522666220307170126
Dong, Z., Gu, H., Guo, Q., Liang, S., Xue, J., Yao, F., et al. (2021). Profiling of serum exosome miRNA reveals the potential of a miRNA panel as diagnostic biomarker for Alzheimer's disease. Mol. Neurobiol. 58, 3084–3094. doi: 10.1007/s12035-021-02323-y
Fan, W., Liang, C., Ou, M., Zou, T., Sun, F., Zhou, H., et al. (2020). microRNA-146a Is a wide-reaching neuroinflammatory regulator and potential treatment target in neurological diseases. Front. Mol. Neurosci. 13, 90. doi: 10.3389/fnmol.2020.00090
Ferreira, A., and Rapoport, M. (2002). The synapsins: beyond the regulation of neurotransmitter release. Cell. Mol. Life Sci. 59, 589–595. doi: 10.1007/s00018-002-8451-5
Gilmore, T. D., and Herscovitch, M. (2006). Inhibitors of NF-kappaB signaling: 785 and counting. Oncogene. 25, 6887–6899. doi: 10.1038/sj.onc
Hermans, S. J., Nero, T. L., Morton, C. J., Gooi, J. H., Crespi, G. A. N., Hancock, N. C., et al. (2021). Structural biology of cell surface receptors implicated in Alzheimer's disease. Biophys. Rev. 14, 233–255. doi: 10.1007/s12551-021-00903-9
Jaber, V., Zhao, Y., and Lukiw, W. J. (2017). Alterations in micro RNA-messenger RNA (miRNA-mRNA) coupled signaling networks in sporadic Alzheimer's disease (AD) hippocampal CA1. J. Alzheimer's Dis. Parkinsonism. 7, 312. doi: 10.4172/2161-0460.1000312
Jaber, V. R., Zhao, Y., Sharfman, N. M., Li, W., and Lukiw, W. J. (2019). Addressing Alzheimer's disease (AD) neuropathology using anti-microRNA (AM) strategies. Mol. Neurobiol. 56, 8101–8108. doi: 10.1007/s12035-019-1632-0
Jauhari, A., Singh, T., Mishra, S., Shankar, J., and Yadav, S. (2020). Coordinated action of miRNA-146a and parkin gene regulate rotenone-induced neurodegeneration. Toxicol. Sci. 176, 433–445. doi: 10.1093/toxsci/kfaa066
Jeśko, H., Wieczorek, I., Wencel, P. L., Gassowska-Dobrowolska, M., Lukiw, W. J., and Strosznajder, R. P. (2021). Age-related transcriptional deregulation of genes coding synaptic proteins in Alzheimer's disease murine model: potential neuroprotective effect of fingolimod. Front. Mol. Neurosci. 14, 660104. doi: 10.3389/fnmol.2021.660104
Jin, C., Kang, H., Ryu, J. R., Kim, S., Zhang, Y., Lee, Y., et al. (2018). Integrative brain transcriptome analysis reveals region-specific and broad molecular changes in SHANK3-overexpressing mice. Front. Mol. Neurosci. 11, 250. doi: 10.3389/fnmol.2018.00250
Jover-Mengual, T., Hwang, J. Y., Byun, H. R., Court-Vazquez, B. L., Centeno, J. M., Burguete, M. C., et al. (2021). The role of NF-κB triggered inflammation in cerebral ischemia. Front. Cell. Neurosci. 15, 633610. doi: 10.3389/fncel.2021.633610
Juźwik, C. A. S, Drake, S, Zhang, Y., Paradis-Isler, N., Sylvester, A., Amar-Zifkin, A., et al. (2019). microRNA dysregulation in neurodegenerative diseases: A systematic review. Prog. Neurobiol. 182, 101664. doi: 10.1016/j.pneurobio.2019.101664
Kaltschmidt, B., Baeuerle, P. A., and Kaltschmidt, C. (1993). Potential involvement of the transcription factor NF-kappa B in neurological disorders. Mol Aspects Med. 14, 171–190. doi: 10.1016/0098-2997(93)90004-w
Kaltschmidt, B., and Kaltschmidt, C. (2009). NF-kappaB in the nervous system. Cold Spring Harb. Perspect. Biol. 1, a001271. doi: 10.1101/cshperspect.a001271
Katti, A., Diaz, B. J., Caragine, C. M., Sanjana, N. E., and Dow, L. E. (2022). CRISPR in cancer biology and therapy. Nat. Rev. Cancer. 22, 259–279. doi: 10.1038/s41568-022-00441-w
Kaur, U., Banerjee, P., Bir, A., Sinha, M., Biswas, A., and Chakrabarti, S. (2015). Reactive oxygen species, redox signaling and neuroinflammation in Alzheimer's disease: the NF-κB connection. Curr. Top Med. Chem. 15, 446–457. doi: 10.2174/1568026615666150114160543
Kittur, S., Hoh, J., Endo, H., Tourtellotte, W., Weeks, B. S., Markesbery, W., et al. (1994). Cytoskeletal neurofilament gene expression in brain tissue from Alzheimer's disease patients. Decrease in NF-L and NF-M message. J. Geriatr. Psychiatry Neurol. 7, 153–158. doi: 10.1177/089198879400700305
Kumar, V. (2019). Toll-like receptors in the pathogenesis of neuroinflammation. J. Neuroimmunol. 332, 16–30. doi: 10.1016/j.jneuroim.2019.03.012
Lane, C. A., Hardy, J., and Schot, J. M. (2018). Alzheimer's disease. Eur. J. Neurol. 25, 59–70. doi: 10.1111/ene.13439
Lee, Y., Kang, H., Lee, B., Zhang, Y., Kim, Y., Kim, S., et al. (2017). Integrative analysis of brain region-specific SHANK3 interactomes for understanding the heterogeneity of neuronal pathophysiology related to SHANK3 mutations. Front. Mol. Neurosci. 10, 110. doi: 10.3389/fnmol.2017.00110
Li, C. L., Liu, X. H., Qiao, Y., Ning, L. N., Li, W. J., and Sun, Y. S. (2020a). Allicin alleviates inflammation of diabetic macroangiopathy via the Nrf2 and NF-kB pathway. Eur. J. Pharmacol. 876, 173052. doi: 10.1016/j.ejphar.2020.173052
Li, L., Xu, Y., Zhao, M., and Gao, Z. (2020b). Neuro-protective roles of long non-coding RNA MALAT1 in Alzheimer's disease with the involvement of the microRNA-30b/CNR1 network and the following PI3K/AKT activation. Exp. Mol. Pathol. 117, 104545. doi: 10.1016/j.yexmp.2020.104545
Li, Y. Y., Cui, J. G., Dua, P., Pogue, A. I., Bhattacharjee, S., and Lukiw, W. J. (2011). Differential expression of miRNA-146a-regulated inflammatory genes in human primary neural, astroglial and microglial cells. Neurosci. Lett. 499, 109–113. doi: 10.1016/j.neulet.2011.05.044
Liu, S., Fan, M., Zheng, Q., Hao, S., Yang, L., Xia, Q., et al. (2022). microRNAs in Alzheimer's disease: Potential diagnostic markers and therapeutic targets. Biomed. Pharmacother. 148, 112681. doi: 10.1016/j.biopha.2022.112681
Lu, B., Guo, H., Zhao, J., Wang, C., Wu, G., Pang, M., et al. (2010). Increased expression of iASPP, regulated by hepatitis B virus X protein-mediated NF-κB activation, in hepatocellular carcinoma. Gastroenterology. 139, 2183–2194.e5. doi: 10.1053/j.gastro.2010.06.049
Lukiw, W. J. (2012). NF-κB-regulated micro RNAs (miRNAs) in primary human brain cells. Exp. Neurol. 235, 484–490. doi: 10.1016/j.expneurol.2011.11.022
Lukiw, W. J. (2020). microRNA-146a signaling in Alzheimer's disease (AD) and prion disease (PrD). Front. Neurol. 11, 462. doi: 10.3389/fneur.2020.00462
Lukiw, W. J., and Bazan, N. G. (1998). Strong nuclear factor-kappaB-DNA binding parallels cyclooxygenase-2 gene transcription in aging and in sporadic Alzheimer's disease superior temporal lobe neocortex. J. Neurosci. Res. 53, 583–592. doi: 10.1002/(SICI)1097-4547(19980901)53:5<583:AID-JNR8>3.0.CO;2-5.
Lukiw, W. J., Cui, J. G., Marcheselli, V. L., Bodker, M., Botkjaer, A., Gotlinger, K., et al. (2005). A role for docosahexaenoic acid-derived neuroprotectin D1 in neural cell survival and Alzheimer disease. J. Clin. Invest. 115, 2774–2783. doi: 10.1172/JCI25420
Lukiw, W. J., Zhao, Y., and Cui, J. G. (2008). An NF-kB-sensitive micro RNA-146a-mediated inflammatory circuit in Alzheimer disease and in stressed human brain cells. J. Biol. Chem. 283, 31315–31322. doi: 10.1074/jbc.M805371200
McLachlan, D. R. C., Lukiw, W. J., and Kruck, T. P. (1990). Aluminum, altered transcription, and the pathogenesis of Alzheimer's disease. Environ. Geochem. Health. 12, 103–114. doi: 10.1007/BF01734059
Mirza, F. J., and Zahid, S. (2018). The role of synapsins in neurological disorders. Neurosci. Bull. 34, 349–358. doi: 10.1007/s12264-017-0201-7
Miyoshi, E., Bilousova, T., Melnik, M., Fakhrutdinov, D., Poon, W. W., Vinters, H. V., et al. (2021). Exosomal tau with seeding activity is released from Alzheimer's disease synapses, and seeding potential is associated with amyloid beta. Lab Invest. 101, 1605–1617. doi: 10.1038/s41374-021-00644-z
Natoli, R., and Fernando, N. (2018). microRNA as therapeutics for age-related macular degeneration. Adv. Exp. Med. Biol. 1074, 37–43. doi: 10.1007/978-3-319-75402-4_5
Olajide, O. A., and Sarker, S. D. (2020). Alzheimer's disease: natural products as inhibitors of neuroinflammation. Inflammopharmacology. 28, 1439–1455. doi: 10.1007/s10787-020-00751-1
Olufunmilayo, E. O., and Holsinger, R. M. D. (2022). Variant TREM2 signaling in Alzheimer's disease. J. Mol. Biol. 434, 167470. doi: 10.1016/j.jmb.2022.167470
Perot, B. P., and Ménager, M. M. (2020). Tetraspanin 7 and its closest paralog tetraspanin 6: membrane organizers with key functions in brain development, viral infection, innate immunity, diabetes and cancer. Med. Microbiol. Immunol. 209, 427–436. doi: 10.1007/s00430-020-00681-3
Pires, B. R. B., Binato, R., Ferreira, G. M., Cecchini, R., Panis, C., and Abdelhay, E. (2018). NF-kappaB regulates redox status in breast cancer subtypes. Genes (Basel).9, 320. doi: 10.3390/genes9070320
Pogue, A. I., Cui, J. G., Li, Y. Y., Zhao, Y., Culicchia, F., and Lukiw, W. J. (2010). Micro RNA-125b (miRNA-125b) function in astrogliosis and glial cell proliferation. Neurosci. Lett. 476, 18–22. doi: 10.1016/j.neulet.2010.03.054
Pogue, A. I., and Lukiw, W. J. (2021). microRNA-146a-5p, neurotropic viral infection and prion disease (PrD). Int. J. Mol. Sci. 22, 9198. doi: 10.3390/ijms22179198
Pogue, A. I., Zhao, Y., and Lukiw, W. J. (2022). microRNA-146a as a biomarker for transmissible spongiform encephalopathy. Folia Neuropathol. 60, 24–34. doi: 10.5114/fn.2022.113561
Rastegar-Moghaddam, S. H., Ebrahimzadeh-Bideskan, A., Shahba, S., Malvandi, A. M., and Mohammadipour, A. (2022). Roles of the miR-155 in neuroinflammation and neurological disorders: a potent biological and therapeutic target. Cell. Mol. Neurobiol. doi: 10.1007/s10571-022-01200-z
Recabarren, D., and Alarcón, M. (2017). Gene networks in neurodegenerative disorders. Life Sci. 183, 83–97. doi: 10.1016/j.lfs.2017.06.009
Sen, R., and Baltimore, D. (1986). Multiple nuclear factors interact with the immunoglobulin enhancer sequences. Cell. 46, 705–716. doi: 10.1016/0092-8674(86)90346-6
Singh, S., Sahu, K., Singh, C., and Singh, A. (2022). Lipopolysaccharide induced altered signaling pathways in various neurological disorders. Naunyn Schmiedebergs Arch. Pharmacol. 395, 285–294. doi: 10.1007/s00210-021-02198-9
Slota, J. A., and Booth, S. A. (2019). MicroRNAs in neuroinflammation: implications in disease pathogenesis, biomarker discovery and therapeutic applications. Noncoding RNA. 5, 35. doi: 10.3390/ncrna5020035
Song, Y., Hu, M., Zhang, J., Teng, Z. Q., and Chen, C. (2021). A novel mechanism of synaptic and cognitive impairments mediated via microRNA-30b in Alzheimer's disease. EBioMedicine. 39, 409–421. doi: 10.1016/j.ebiom.2018.11.059
Sun, G. Y., Simonyi, A., Fritsche, K. L., Chuang, D. Y., Hannink, M., Gu, Z., et al. (2018). Docosahexaenoic acid (DHA): an essential nutrient and a nutraceutical for brain health and diseases. Prostaglandins Leukot. Essent. Fatty Acids. 136, 3–13. doi: 10.1016/j.plefa.2017.03.006
Taganov, K. D., Boldin, M. P., Chang, K. J., and Baltimore, D. (2006). NF-kappaB-dependent induction of microRNA miRNA-146a, an inhibitor targeted to signaling proteins of innate immune responses. Proc. Natl. Acad. Sci. USA. 103, 12481–12486. doi: 10.1073/pnas.0605298103
Tahami Monfared, A. A., Byrnes, M. J., White, L. A., and Zhang, Q. (2022). Alzheimer's disease: epidemiology and clinical progression. Neurol. Ther. 11, 553–569. doi: 10.1007/s40120-022-00338-8
Trejo-Lopez, J. A., Yachnis, A. T., and Prokop, S. (2021). Neuropathology of Alzheimer's disease. Neurotherapeutics. doi: 10.1007/s13311-021-01146-y
Turk, A., Kunej, T., and Peterlin, B. (2021). microRNA-target interaction regulatory network in Alzheimer's disease. J. Pers. Med. 11, 1275. doi: 10.3390/jpm11121275
Uddin, M. S., Hasana, S., Ahmad, J., Hossain, M. F., Rahman, M. M., Behl, T., et al. (2021). Anti-Neuroinflammatory potential of polyphenols by inhibiting NF-κB to halt Alzheimer's disease. Curr. Pharm. Des. 27, 402–414. doi: 10.2174/1381612826666201118092422
Valeri, A., Chiricosta, L., Calcaterra, V., Biasin, M., Cappelletti, G., Carelli, S., et al. (2021). Transcriptomic analysis of HCN-2 cells suggests connection among oxidative stress, senescence, and neuron death after SARS-CoV-2 infection. Cells. 10, 2189. doi: 10.3390/cells10092189
Wang, P., Liu, Z., Zhang, X., Li, J., Sun, L., Ju, Z., et al. (2018). CRISPR/Cas9-mediated gene knockout reveals a guardian role of NF-κB/RelA in maintaining the homeostasis of human vascular cells. Protein Cell. 9, 945–965. doi: 10.1007/s13238-018-0560-5
Wu, Y., Wang, M., Yin, H., Ming, S., Li, X., Jiang, G., et al. (2021). TREM-2 is a sensor and activator of T cell response in SARS-CoV-2 infection. Sci Adv. 7, eabi6802. doi: 10.1126/sciadv.abi6802
Xu, H., Zhang, J., Shi, X., Li, X., and Zheng, C. (2021). NF-κB inducible miR-30b-5p aggravates joint pain and loss of articular cartilage via targeting SIRT1-FoxO3a-mediated NLRP3 inflammasome. Aging. 13, 20774–20792. doi: 10.18632/aging.203466
Yoon, S., Kim, S. E., Ko, Y., Jeong, G. H., Lee, K. H., Lee, J., et al. (2022). Differential expression of microRNAs in Alzheimer's disease: a systematic review and meta-analysis. Mol. Psychiatry. 27, 2405–2413. doi: 10.1038/s41380-022-01476-z
Yu, J., Yuan, X., Chen, H., Chaturvedi, S., Braunstein, E. M., and Brodsky, R. A. (2020). Direct activation of the alternative complement pathway by SARS-CoV-2 spike proteins is blocked by factor D inhibition. Blood. 136, 2080–2089. doi: 10.1182/blood.2020008248
Zhan, X., Hakoupian, M., Jin, L. W., and Sharp, F. R. (2021). Lipopolysaccharide, identified using an antibody and by PAS Staining, is associated with corpora amylacea and white matter injury in Alzheimer's disease and aging brain. Front. Aging Neurosci. 13, 705594. doi: 10.3389/fnagi.2021.705594
Zhan, X., Stamova, B., and Sharp, F. R. (2018). Lipopolysaccharide associates with amyloid plaques, neurons and oligodendrocytes in Alzheimer's disease brain: a review. Front. Aging Neurosci. 10, 42. doi: 10.3389/fnagi.2018.00042
Zhang, Q., Lenardo, M. J., and Baltimore, D. (2017). 30 Years of NF-κB: a blossoming of relevance to human pathobiology. Cell. 168, 37–57. doi: 10.1016/j.cell.2016.12.012
Zhao, Y., Arceneaux, L., Culicchia, F., and Lukiw, W. J. (2021). Neurofilament Light (NF-L) chain protein from a highly polymerized structural component of the neuronal cytoskeleton to a neurodegenerative disease biomarker in the periphery. HSOA J. Alzheimer's Neurodegener Dis. 7, 056. doi: 10.24966/AND-9608/100056 Epub 2021 Oct 13.
Zhao, Y., Bhattacharjee, S., Jones, B. M., Dua, P., Alexandrov, P. N., Hill, J. M., et al. (2013). Regulation of TREM2 expression by an NF-κB-sensitive miRNA-34a. Neuroreport 24, 318–323. doi: 10.1097/WNR.0b013e32835fb6b0
Zhao, Y., Bhattacharjee, S., Jones, B. M., Hill, J. M., Dua, P., and Lukiw, W. J. (2014). Regulation of neurotropic signaling by the inducible, NF-kB-sensitive miRNA-125b in Alzheimer's disease (AD) and in primary human neuronal-glial (HNG) cells. Mol. Neurobiol. 50, 97–106. doi: 10.1007/s12035-013-8595-3
Zhao, Y., Jaber, V. R., LeBeauf, A., Sharfman, N. M., and Lukiw, W. J. (2019b). microRNA-34a (miRNA-34a) mediated down-regulation of the post-synaptic cytoskeletal element SHANK3 in sporadic Alzheimer's disease (AD). Front. Neurol. 10:28. doi: 10.3389/fneur.2019.00028
Zhao, Y., and Lukiw, W. J. (2013). TREM2 signaling, miRNA-34a and the extinction of phagocytosis. Front. Cell. Neurosci. 7, 131. doi: 10.3389/fncel.2013.00131
Zhao, Y., and Lukiw, W. J. (2018). Bacteroidetes neurotoxins and inflammatory neurodegeneration. Mol. Neurobiol. 55, 9100–9107. doi: 10.1007/s12035-018-1015-y
Zhao, Y., Sharfman, N. M., Jaber, V. R., and Lukiw, W. J. (2019a). Down-regulation of essential synaptic components by GI-tract microbiome-derived lipopolysaccharide (LPS) in LPS-treated human neuronal-glial (HNG) cells in primary culture: relevance to Alzheimer's disease (AD). Front. Cell. Neurosci. 13, 314. doi: 10.3389/fncel.2019.00314
Keywords: Alzheimer's disease (AD), microRNA, NF-kB (p50/p65), reactive oxygen species (ROS), lipopolysaccharide, miRNA-based therapy, miRNA-9/miRNA-30b/miRNA-34a/miRNA-146a/miRNA-155, anti-NF-kB therapy
Citation: Lukiw WJ (2022) NF-kB (p50/p65)-Mediated Pro-Inflammatory microRNA (miRNA) Signaling in Alzheimer's Disease (AD). Front. Mol. Neurosci. 15:943492. doi: 10.3389/fnmol.2022.943492
Received: 13 May 2022; Accepted: 27 May 2022;
Published: 28 June 2022.
Edited by:
Jun Yan, The University of Queensland, AustraliaReviewed by:
Uwe Beffert, Boston University, United StatesCopyright © 2022 Lukiw. This is an open-access article distributed under the terms of the Creative Commons Attribution License (CC BY). The use, distribution or reproduction in other forums is permitted, provided the original author(s) and the copyright owner(s) are credited and that the original publication in this journal is cited, in accordance with accepted academic practice. No use, distribution or reproduction is permitted which does not comply with these terms.
*Correspondence: Walter J. Lukiw, d2x1a2l3JiN4MDAwNDA7bHN1aHNjLmVkdQ==