- 1Department of Cerebrovascular Diseases, The Second Affiliated Hospital of Zhengzhou University, Zhengzhou, China
- 2Academy of Medical Science, Zhengzhou University, Zhengzhou, China
- 3Henan Medical Key Laboratory of Translational Cerebrovascular Diseases, Zhengzhou, China
- 4Department of Clinical Neurosciences, Hotchkiss Brain Institute, University of Calgary, Calgary, AB, Canada
Intracerebral hemorrhage (ICH) is a subtype of stroke that is characterized by high morbidity and mortality, for which clinical outcome remains poor. An extensive literature indicates that the release of ferrous iron from ruptured erythrocytes in the hematoma is a key pathogenic factor in ICH-induced brain injury. Deferoxamine is an FDA-approved iron chelator that has the capacity to penetrate the blood-brain barrier after systemic administration and binds to iron. Previous animal studies have shown that deferoxamine attenuates ICH-induced brain edema, neuronal death, and neurological deficits. This review summarizes recent progress of the mechanisms by which deferoxamine may alleviate ICH and discusses further studies on its clinical utility.
Introduction
Intracerebral hemorrhage (ICH) is caused by the rupture of blood vessels in the brain. It accounts for approximately 15% of all stroke types and affects about 2 million people worldwide each year (Gross et al., 2019). The prognosis of ICH is worse than that of the more common ischemic stroke; ICH has a 30-day mortality rate of 43–51%. Most patients that survive ICH have residual sequelae such as neurological dysfunctions (Krishnamurthi et al., 2020). The adverse outcomes associated with ICH are due to a combination of various pathophysiological processes, such as hematoma formation, space-occupying effects caused by the enlarging hematoma, local cerebral blood flow change, disruption of blood-brain barrier (BBB), and brain edema (Bai et al., 2020b; Chen et al., 2020; Zhang et al., 2021). The mechanism of brain injury after ICH can be broadly divided into primary injury caused by the mass effect of intraparenchymal hematoma, and secondary injury induced by neuroinflammatory responses and oxidative stress (OS) (Zhu et al., 2019; Xue and Yong, 2020; Holste et al., 2021).
Iron, as one of the main degradation products of hemoglobin catabolism, can cause secondary brain injury after ICH by promoting free radical formation and inflammatory responses (Zhu et al., 2021). Iron overload can lead to brain injury in several ways, such as lipid peroxidation and free radical formation (Stockwell et al., 2017). As an iron-chelating agent, deferoxamine can rapidly cross the blood-brain barrier (BBB) after systemic administration, and also effectively combine with iron ions to reduce the ferrous ion concentration in the hematoma area, thereby ameliorating the secondary neurological damage caused by ICH (Zeng et al., 2018). In ICH animal models, deferoxamine has been shown to be neuroprotective through several mechanisms, including reduction of hemoglobin-related edema and inhibition of neuronal death; it improves neurological deficits and brain atrophy after ICH (Cui et al., 2015; Zhang et al., 2022a). In this review, we summarize the mechanisms and advances of deferoxamine in the treatment of ICH.
Erythrocyte Lysis and Brain Edema Formation
Notably, hemoglobin and its degradation products are neurotoxic and contribute to delayed neuronal injury and edema formation after ICH (Wagner et al., 2003; Hua et al., 2007). At present, the mechanisms of brain edema formation following ICH has not been fully clarified and it may occur through a series of pathophysiological processes, including platelet aggregation, clot formation, activation of the coagulation cascade, iron overload and hemoglobin toxicity, complement activation, secondary injury after reperfusion, and disruption of the blood-brain barrier (Appelboom et al., 2013; Wang et al., 2018). Studies have shown that clots begin to dissolve on the first day after ICH, and their by-products, including free hemoglobin, heme and free iron, contribute to brain injury that may last days or weeks (An et al., 2017).
Erythrocytes lyse after ICH and release large amounts of ferrous ions, which contribute to brain edema formation (Perez De La Ossa et al., 2010; Zhang et al., 2022d). In the rat model of autologous blood-induced ICH, brain edema peaks on the third or 4th day after the onset of ICH, and then resorbs and gradually diminishes over time (Xi et al., 1998). In the thromboplastic-induced rat model, the earliest peak of brain edema occurs within 48 h (Yang et al., 1994; Zhang et al., 2021). The potential toxic effects of erythrocyte degradation products have been demonstrated, and results indicate that early erythrocyte lysis in the hematoma facilitates brain injury including disruption of BBB, brain iron overload, and neurological deficits within 24 h in aged rats (Xue and Del Bigio, 2005; Balami and Buchan, 2012; Figure 1).
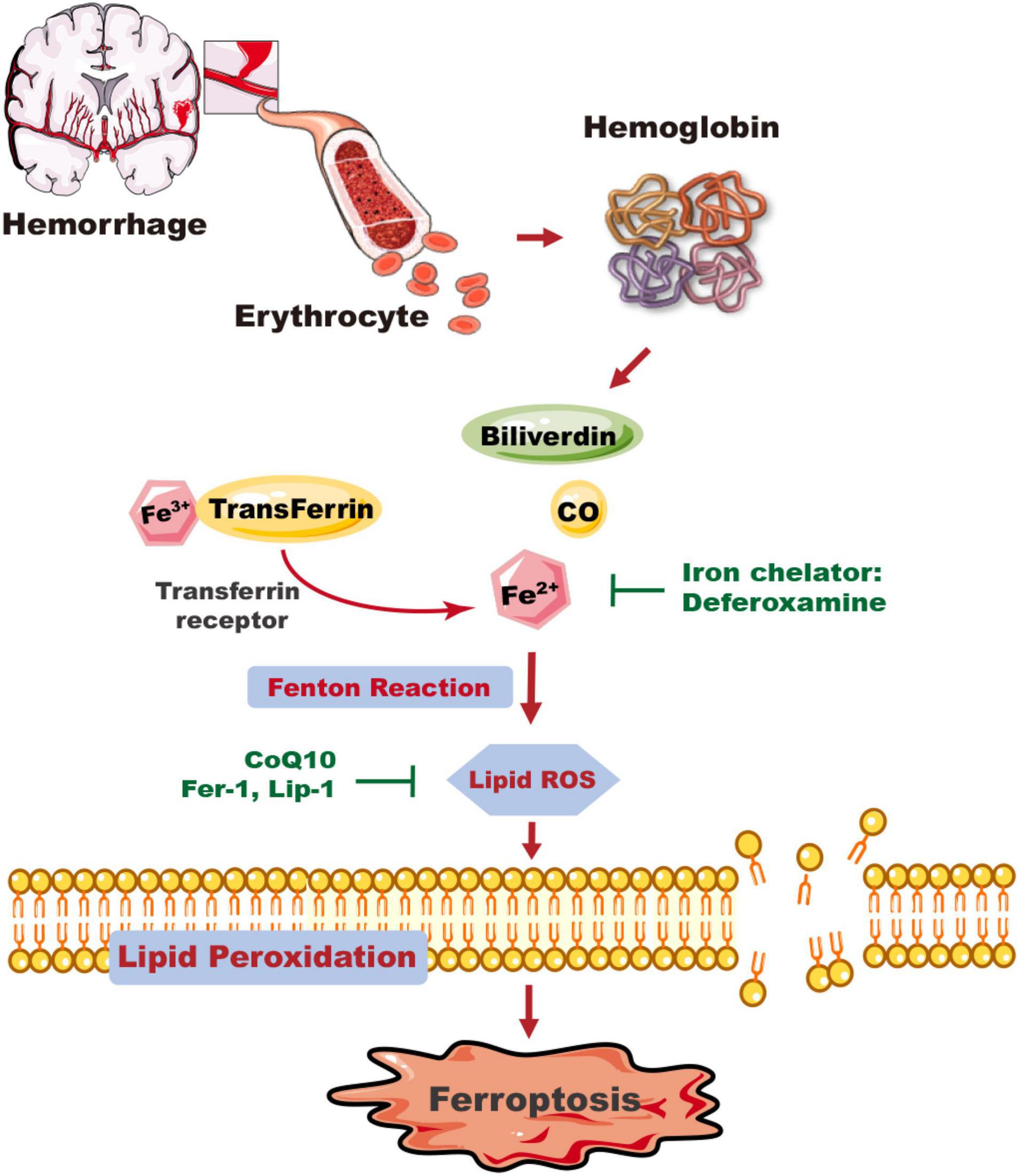
Figure 1. The mechanism of ICH-induced ferroptosis in the brain. Following ICH, erythrocytes release hemoglobin, which produces a degradation product, carbon monoxide (CO), biliverdin, and free iron. Excess Fe2+, the reactive form of iron, can generate ROS and cause membrane lipid peroxidation, and trigger ferroptosis. Deferoxamine can reduce the level of iron ions and inhibit the production of ROS and the occurrence of ferroptosis. The ferroptosis inhibitors CoQ10, Fer-1, and Lip-1 inhibit lipid ROS activity and ferroptosis. CO, carbon monoxide; CoQ10, coenzyme Q10; Fer-1, ferrostatin-1; Lip-1, liproxstatin-1.
Hemoglobin and Brain Injury
Hemoglobin and its degradation products are crucial factors in brain injury after ICH (Zhu et al., 2019). Heme oxygenase-1 (HO-1) is a cytoprotective enzyme that catalyzes the toxic heme into carbon monoxide (CO), biliverdin and ferrous iron (Wang and Dore, 2007). Biliverdin is further converted to bilirubin by biliverdin reductase, which possesses antioxidant activity. Heme oxygenase-1 is known to be upregulated in the brain (Schipper et al., 2019). In addition, zinc protoporphyrin (ZnPP), an inhibitor of HO-1, suppresses brain edema and neuronal damage (Zhou et al., 2014; Zhang et al., 2022d). Also, intraperitoneal injection of deferoxamine in vivo may minimize brain injury resulting from hemoglobin degradation. In conclusion, hemoglobin itself and its degradation products, especially iron, play a key role in secondary brain injury after ICH (Huang et al., 2002).
Iron Triggers Neuroinflammation Following Intracerebral Hemorrhage
Iron and its homeostasis have been described as a hallmark of neuroinflammation (Wu et al., 2011), and mounting evidence supports that iron accumulation may lead to inflammatory responses (Wessling-Resnick, 2010; Bai et al., 2020a). It has been recognized that iron triggers a cascade of deleterious events, including activating microglia/macrophages, and elevating the levels of nitric oxide (NO) and reactive oxygen species (ROS) (Zhang et al., 2022b), matrix metalloproteinases (MMPs), tumor necrosis factor-α (TNF-α) and other inflammatory molecules, exacerbating neuroinflammation (Wu et al., 2002; Yong et al., 2019; Xue and Yong, 2020; Zhu et al., 2021).
The role of macrophages in iron homeostasis has been studied. The results show that proinflammatory microglia/macrophages tend to sequester iron, whereas regulatory microglia/macrophages express genes that promote iron release (Yong et al., 2019). Thus, neuroinflammation and iron are tightly linked, as the inflammatory environment is associated with iron accumulation and neuroinflammation following ICH may affect normal function throughout the entire brain (Ke and Ming Qian, 2003; Zhang et al., 2015; Li et al., 2016).
Iron Triggers Neuronal Death Following Intracerebral Hemorrhage
Iron, as a trace element, is essential for the maintenance of normal cellular physiological functions. It is also involved in the synthesis of myelin and various neurotransmitters in the central nervous system (Xiong et al., 2014), while the dysregulation of iron homeostasis in the brain (iron overload or deficiency) can contribute to multiple brain injuries, including hemorrhagic and ischemic stroke (Garton et al., 2016). Excess iron generates large amounts of ROS mainly through the Fenton reaction, triggering inflammation and neuronal death, which can lead to long-term cognitive dysfunction and early brain edema (Bai et al., 2020c; Zhang et al., 2022c). Upon erythrocyte lysis, the concentration of iron persists at a relatively high level. In a rat model of ICH, the concentration of non-heme iron increases threefold and is sustained at a high level for 28 days (Hua et al., 2006).
Iron overload contributes to brain edema and iron chelators can attenuate the degree of hematoma-induced brain edema (Qing et al., 2009). Countering the iron-induced phospholipid peroxidation and DNA damage (Gu et al., 2011), deferoxamine ameliorates neurological deficits, reduces the area of brain edema and inhibits neuronal apoptosis after ICH in rats (Nakamura et al., 2003).
Brain Iron Metabolism After Intracerebral Hemorrhage
The brain needs iron for its own metabolism and dysfunction occurs when iron homeostasis is imbalanced (Ke and Qian, 2007). The iron in the central nervous system is mainly transported through the BBB, while another part of iron is directly absorbed by brain cells. Iron transport across the BBB is mostly through the transferrin–transferrin receptor system (Tf-TfR) (Chiueh, 2001). As a plasma glycoprotein, transferrin binds to its receptor and plays an indispensable role in the transfer of iron. In addition, the blood cerebrospinal fluid (CSF) barrier has similar characteristics to the BBB regarding iron transport, which may be another important pathway for iron to enter the brain through the choroid plexus (Nnah and Wessling-Resnick, 2018). After being transported across the BBB or blood-CSF barrier, iron can rapidly bind to Tf secreted by oligodendrocytes and choroid plexus epithelial cells, thus facilitating uptake by brain cells (Bradbury, 1997). In the brain, ferritin is an iron storage-protein, expressed mainly in neurons and glial cells, consisting of a heavy chain (H-ferritin) and a light chain (L-ferritin), respectively (Connor et al., 2001). Ferritin plays a pivotal role in iron storage and maintenance of intracellular iron homeostasis (Yang et al., 2021).
Deferoxamine Reduces Brain Injury After Intracerebral Hemorrhage
Deferoxamine is an iron chelator used clinically to treat severe anemia and iron overload disorders caused by repeated blood transfusions (Selim, 2009). Deferoxamine has a high affinity for Fe3+ and binds directly to iron in plasma and tissues, contributing to the formation of a stable, non-toxic, water-soluble complex. The subsequent excretion of the complex from the body thereby protects brain against iron overload (Zeng et al., 2018).
After systemic administration, deferoxamine can penetrate the BBB, reduce iron content around the hematoma site, and thus attenuate secondary injury after ICH (Wan et al., 2019). As well, deferoxamine can catalyze the degradation of hemoglobin by increasing the expression of HO-1, thereby protecting against the toxic effects of free heme released during hemoglobin degradation mediated by glutamate (Schipper et al., 2019). Additionally, deferoxamine attenuated ICH-induced CD163 upregulation and brain cell death in vivo, and hemoglobin-induced CD163 elevation and neuronal death in vitro (Liu et al., 2017). Moreover, deferoxamine may reduce the production of reactive hydroxyl radical by inhibiting the Fenton and Haber—Weiss reactions (Matsuki et al., 1999). Our recent study found that the combination of deferoxamine with minocycline provided prominent neuroprotection after ICH (Sheng et al., 2018). Minocycline, a broad-spectrum tetracycline, can provide neuroprotection through its anti-inflammatory properties, including inhibiting microglia activity and reducing the expression of matrix metalloproteinases (Xue et al., 2010; Zhang et al., 2022a). Minocycline has also iron chelation capacity (Zhao et al., 2016; Liu et al., 2021). An obvious neurovascular protective effect by minocycline was observed in ICH models in rats (Lee et al., 2003). Our study revealed that the extent of brain damage, neuronal death, and the activation of microglia/macrophages were significantly reduced after ICH in minocycline and deferoxamine combined treatment. There was also decreased iron accumulation in the area around the hematoma in the combination treatment group (Figure 2), and animals recovered from their neurological deficits better (Li et al., 2021).
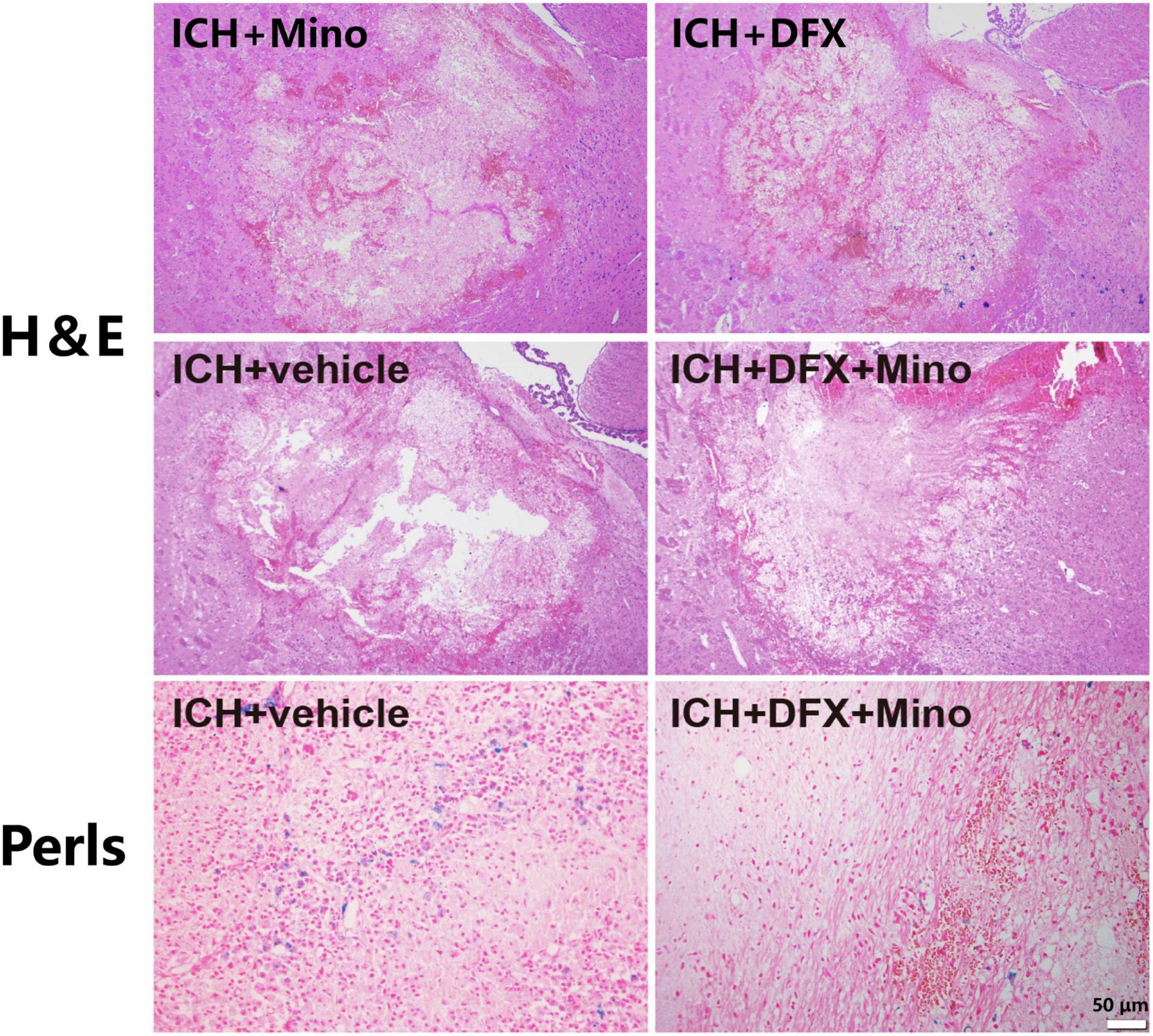
Figure 2. The combined therapy of deferoxamine and minocycline attenuated iron deposition and reduced the area of brain damage after ICH. Paraffin sections from rats at day 3 after ICH were analyzed, and representative images of Hematoxylin and Eosin staining showing the brain damage area, and Perls’ blue staining to reveal iron-labeled cells, are displayed. Scale bar, 50 μm.
Progress in the Translational Research of Deferoxamine in Intracerebral Hemorrhage
The efficacy of deferoxamine in treating hemorrhage-induced brain damage in animal models has been previously summarized (Selim, 2009; Cui et al., 2015; Zeng et al., 2018; Hu et al., 2019). Deferoxamine reduced the effects of ischemic and hypoxic brain injury, particularly in the cortex and hippocampus, and attenuated ICH-induced brain atrophy and neurological deficits (Okauchi et al., 2010; Hatakeyama et al., 2013). In the collagenase-induced ICH in mice, systemic administration of deferoxamine lowered local iron-deposition, inhibited secondary inflammation, reduced neuronal cell death, and improved recovery from neurofunctional deficits (Okauchi et al., 2009). Other studies have shown that deferoxamine diminished parenchymal iron levels but failed to attenuate functional impairment or lesion volume after ICH (Auriat et al., 2012). In the piglet model of ICH, deferoxamine treatment reduced the number of iron-positive cells, neuronal death, and iron accumulation around the hematoma (Gu et al., 2009; Hu et al., 2019). Similarly, Xie et al. showed that deferoxamine decreased white matter edema and TNF-α levels after ICH in piglets, both of which are supportive of deferoxamine as a potentially effective therapeutic agent for ICH patients (Xie et al., 2014). Our study demonstrated that deferoxamine reduced neuronal death, suppressed the activation of microglia/macrophages, decreased iron accumulation around the hematoma, lessened the area of brain injury, and improved neurological deficits in ICH (Li et al., 2021).
Deferoxamine is being tested in human clinical trials. A phase I multicenter, multi-quantitative trial was conducted to assess the tolerability and safety of deferoxamine in ICH (Selim et al., 2011). Deferoxamine was administered for 3 consecutive days by intravenous infusion starting within 18 h after the onset of ICH. The results found deferoxamine to be well tolerated in patients with ICH and was not associated with serious adverse events or mortality. A phase II clinical trial (NCT 01662895) was subsequently conducted (Selim et al., 2019). This placebo-controlled, randomized, double-blind trial was designed to investigate whether high-dose deferoxamine was effective in improving neurological function in patients with ICH, and to assess whether the drug should be investigated in a phase III trial. The results show that deferoxamine was safe and improved the chances of a good clinical outcome. The recent i-DEF trial (Intracerebral Hemorrhage Deferoxamine Trial) assessed modified Rankin Score (mRS) longitudinally by following ICH patients from day 7 to the end of the 6-month (Foster et al., 2022). The results revealed that a large proportion of patients continued to improve up to 6 months after ICH, signaling that deferoxamine may accelerate and alter the trajectory of recovery as assessed by mRS. Nevertheless, deferoxamine caused a series of adverse reactions, including allergic reactions, systemic allergies, and cardiovascular, hematologic, and neurotoxic effects (Yeatts et al., 2013). In addition, deferoxamine may increase cytotoxicity by inhibiting DNA synthesis (Liachenko et al., 2003).
Future Directions
Encouraging, over the past decade, an increasing body of knowledge gained from basic science research on ICH has advanced considerations of therapeutic options. Targeting iron is one of this translation, with the appreciation that iron plays an important role in the formation of hematoma, neuronal death, and behavioral deficits following ICH. Accordingly, deferoxamine has been scrutinized for the treatment of secondary brain injury in patients with ICH.
Currently, while deferoxamine is listed as a promising treatment for ICH, there is no validated clinical trial data to confirm the clinical effectiveness of deferoxamine. At present, the following open questions remain and should be interrogated: (1) whether deferoxamine is indeed efficacious in Phase III trials in ICH; (2) although deferoxamine can slow the formation of brain edema and improve resorption of hematoma after ICH (Nakamura et al., 2003), the mechanisms underlying the relationship between the rate of hematoma resorption and the degree of brain edema require further investigation; (3) whether deferoxamine could improve neurological function in patients with ICH when tested at different doses and at earlier treatment initiation times; and (4) whether patients can accept the potential toxic effects of deferoxamine, such as growth retardation or abnormal bone growth, hearing impairment, nephrotoxicity, and other treatment-related adverse events. Further clinical trials should be conducted to control the occurrence of adverse events and to investigate the best therapeutic options that are safe and well tolerated.
Author Contributions
All authors listed have made a substantial, direct, and intellectual contribution to the work, and approved it for publication.
Funding
The authors acknowledged operating grant support from the National Key Research and Development Program of China (grant no. 2018YFC1312200), National Natural Science Foundation of China (grant nos. 82071331, 81870942, and 81520108011), China Postdoctoral Science Foundation (grant nos. 2020TQ0289 and 2020M672291), and from the Canadian Institutes of Health Sciences (VY).
Conflict of Interest
The authors declare that the research was conducted in the absence of any commercial or financial relationships that could be construed as a potential conflict of interest.
Publisher’s Note
All claims expressed in this article are solely those of the authors and do not necessarily represent those of their affiliated organizations, or those of the publisher, the editors and the reviewers. Any product that may be evaluated in this article, or claim that may be made by its manufacturer, is not guaranteed or endorsed by the publisher.
References
An, S. J., Kim, T. J., and Yoon, B. W. (2017). Epidemiology, risk factors, and clinical features of intracerebral hemorrhage: an update. J. Stroke 19, 3–10. doi: 10.5853/jos.2016.00864
Appelboom, G., Bruce, S. S., Hickman, Z. L., Zacharia, B. E., Carpenter, A. M., Vaughan, K. A., et al. (2013). Volume-dependent effect of perihaematomal oedema on outcome for spontaneous intracerebral haemorrhages. J. Neurol. Neurosurg. Psychiatry 84, 488–493. doi: 10.1136/jnnp-2012-303160
Auriat, A. M., Silasi, G., Wei, Z. P., Paquette, R., Paterson, P., Nichol, H., et al. (2012). Ferric iron chelation lowers brain iron levels after intracerebral hemorrhage in rats but does not improve outcome. Exp. Neurol. 234, 136–143. doi: 10.1016/j.expneurol.2011.12.030
Bai, Q., Xue, M., and Yong, V. W. (2020b). Microglia and macrophage phenotypes in intracerebral haemorrhage injury: therapeutic opportunities. Brain J. Neurol. 143, 1297–1314. doi: 10.1093/brain/awz393
Bai, Q., Sheng, Z., Liu, Y., Zhang, R., Yong, V. W., and Xue, M. (2020a). Intracerebral haemorrhage: from clinical settings to animal models. Stroke Vasc. Neurol. 5, 388–395. doi: 10.1136/svn-2020-000334
Bai, Q. Q., Liu, J. C., and Wang, G. Q. (2020c). Ferroptosis, a regulated neuronal cell death type after intracerebral hemorrhage. Front. Cell. Neurosci. 14:591874. doi: 10.3389/fncel.2020.591874
Balami, J. S., and Buchan, A. M. (2012). Complications of intracerebral haemorrhage. Lancet Neurol. 11, 101–118.
Bradbury, M. W. (1997). Transport of iron in the blood-brain-cerebrospinal fluid system. J. Neurochem. 69, 443–454. doi: 10.1046/j.1471-4159.1997.69020443.x
Chen, L., Chen, T., Mao, G. S., Chen, B. D., Li, M. C., Zhang, H. B., et al. (2020). Clinical neurorestorative therapeutic guideline for brainstem hemorrhage (2020 China version). J. Neurorestoratol. 8, 232–240.
Chiueh, C. C. (2001). Iron overload, oxidative stress, and axonal dystrophy in brain disorders. Pediatr. Neurol. 25, 138–147. doi: 10.1016/s0887-8994(01)00266-1
Connor, J. R., Menzies, S. L., Burdo, J. R., and Boyer, P. J. (2001). Iron and iron management proteins in neurobiology. Pediatr. Neurol. 25, 118–129. doi: 10.1016/s0887-8994(01)00303-4
Cui, H. J., He, H. Y., Yang, A. L., Zhou, H. J., Wang, C., Luo, J. K., et al. (2015). Efficacy of deferoxamine in animal models of intracerebral hemorrhage: a systematic review and stratified meta-analysis. PLoS One 10:e0127256. doi: 10.1371/journal.pone.0127256
Foster, L., Robinson, L., Yeatts, S. D., Conwit, R. A., Shehadah, A., Lioutas, V., et al. (2022). Effect of deferoxamine on trajectory of recovery after intracerebral hemorrhage: a post hoc analysis of the i-DEF Trial. Stroke [Epub ahead of print]. doi: 10.1161/STROKEAHA.121.037298
Garton, T., Keep, R. F., Hua, Y., and Xi, G. (2016). Brain iron overload following intracranial haemorrhage. Stroke Vasc. Neurol. 1, 172–184. doi: 10.1136/svn-2016-000042
Gross, B. A., Jankowitz, B. T., and Friedlander, R. M. (2019). Cerebral intraparenchymal hemorrhage: a review. JAMA 321, 1295–1303. doi: 10.1001/jama.2019.2413
Gu, Y., Hua, Y., He, Y., Wang, L., Hu, H., Keep, R. F., et al. (2011). Iron accumulation and DNA damage in a pig model of intracerebral hemorrhage. Acta Neurochir. Suppl. 111, 123–128. doi: 10.1007/978-3-7091-0693-8_20
Gu, Y., Hua, Y., Keep, R. F., Morgenstern, L. B., and Xi, G. (2009). Deferoxamine reduces intracerebral hematoma-induced iron accumulation and neuronal death in piglets. Stroke 40, 2241–2243. doi: 10.1161/STROKEAHA.108.539536
Hatakeyama, T., Okauchi, M., Hua, Y., Keep, R. F., and Xi, G. (2013). Deferoxamine reduces neuronal death and hematoma lysis after intracerebral hemorrhage in aged rats. Transl. Stroke Res. 4, 546–553. doi: 10.1007/s12975-013-0270-5
Holste, K., Xia, F., Garton, H. J. L., Wan, S., Hua, Y., Keep, R. F., et al. (2021). The role of complement in brain injury following intracerebral hemorrhage: a review. Exp. Neurol. 340:113654. doi: 10.1016/j.expneurol.2021.113654
Hu, S., Hua, Y., Keep, R. F., Feng, H., and Xi, G. (2019). Deferoxamine therapy reduces brain hemin accumulation after intracerebral hemorrhage in piglets. Exp. Neurol. 318, 244–250. doi: 10.1016/j.expneurol.2019.05.003
Hua, Y., Keep, R. F., Hoff, J. T., and Xi, G. (2007). Brain injury after intracerebral hemorrhage: the role of thrombin and iron. Stroke 38, 759–762. doi: 10.1161/01.STR.0000247868.97078.10
Hua, Y., Nakamura, T., Keep, R. F., Wu, J. M., Schallert, T., Hoff, J. T., et al. (2006). Long-term effects of experimental intracerebral hemorrhage: the role of iron. J. Neurosurg. 104, 305–312. doi: 10.3171/jns.2006.104.2.305
Huang, F. P., Xi, G., Keep, R. F., Hua, Y., Nemoianu, A., and Hoff, J. T. (2002). Brain edema after experimental intracerebral hemorrhage: role of hemoglobin degradation products. J. Neurosurg. 96, 287–293. doi: 10.3171/jns.2002.96.2.0287
Ke, Y., and Ming Qian, Z. (2003). Iron misregulation in the brain: a primary cause of neurodegenerative disorders. Lancet Neurol. 2, 246–253. doi: 10.1016/s1474-4422(03)00353-3
Ke, Y., and Qian, Z. M. (2007). Brain iron metabolism: neurobiology and neurochemistry. Prog. Neurobiol. 83, 149–173. doi: 10.1016/j.pneurobio.2007.07.009
Krishnamurthi, R. V., Ikeda, T., and Feigin, V. L. (2020). Global, regional and country-specific burden of ischaemic stroke, intracerebral haemorrhage and subarachnoid haemorrhage: a systematic analysis of the Global Burden of Disease Study 2017. Neuroepidemiology 54, 171–179. doi: 10.1159/000506396
Lee, S. M., Yune, T. Y., Kim, S. J., Park, D. W., Lee, Y. K., Kim, Y. C., et al. (2003). Minocycline reduces cell death and improves functional recovery after traumatic spinal cord injury in the rat. J. Neurotrauma 20, 1017–1027.
Li, Y., Pan, K., Chen, L., Ning, J. L., Li, X., Yang, T., et al. (2016). Deferoxamine regulates neuroinflammation and iron homeostasis in a mouse model of postoperative cognitive dysfunction. J. Neuroinflammation 13:268. doi: 10.1186/s12974-016-0740-2
Li, Z., Liu, Y., Wei, R., Khan, S., Xue, M., and Yong, V. W. (2021). The combination of deferoxamine and minocycline strengthens neuroprotective effect on acute intracerebral hemorrhage in rats. Neurol. Res. 43, 854–864. doi: 10.1080/01616412.2021.1939487
Liachenko, S., Tang, P., and Xu, Y. (2003). Deferoxamine improves early postresuscitation reperfusion after prolonged cardiac arrest in rats. J. Cereb. Blood Flow Metab. 23, 574–581. doi: 10.1097/01.WCB.0000057742.00152.3F
Liu, R., Cao, S., Hua, Y., Keep, R. F., Huang, Y., and Xi, G. (2017). CD163 expression in neurons after experimental intracerebral hemorrhage. Stroke 48, 1369–1375. doi: 10.1161/STROKEAHA.117.016850
Liu, Y., Li, Z., Khan, S., Zhang, R., Wei, R., Zhang, Y., et al. (2021). Neuroprotection of minocycline by inhibition of extracellular matrix metalloproteinase inducer expression following intracerebral hemorrhage in mice. Neurosci. Lett. 764:136297. doi: 10.1016/j.neulet.2021.136297
Matsuki, N., Takanohashi, A., Boffi, F. M., Inanami, O., Kuwabara, M., and Ono, K. (1999). Hydroxyl radical generation and lipid peroxidation in C2C12 myotube treated with iodoacetate and cyanide. Free Radic. Res. 31, 1–8. doi: 10.1080/10715769900300551
Nakamura, T., Keep, R. F., Hua, Y., Schallert, T., Hoff, J. T., and Xi, G. (2003). Deferoxamine-induced attenuation of brain edema and neurological deficits in a rat model of intracerebral hemorrhage. Neurosurg. Focus 15:ECP4.
Nnah, I. C., and Wessling-Resnick, M. (2018). Brain iron homeostasis: a focus on microglial iron. Pharmaceuticals 11:129. doi: 10.3390/ph11040129
Okauchi, M., Hua, Y., Keep, R. F., Morgenstern, L. B., Schallert, T., and Xi, G. (2010). Deferoxamine treatment for intracerebral hemorrhage in aged rats: therapeutic time window and optimal duration. Stroke 41, 375–382. doi: 10.1161/STROKEAHA.109.569830
Okauchi, M., Hua, Y., Keep, R. F., Morgenstern, L. B., and Xi, G. (2009). Effects of deferoxamine on intracerebral hemorrhage-induced brain injury in aged rats. Stroke 40, 1858–1863. doi: 10.1161/STROKEAHA.108.535765
Perez, De La Ossa, N., Sobrino, T., Silva, Y., Blanco, M., Millan, M., et al. (2010). Iron-related brain damage in patients with intracerebral hemorrhage. Stroke 41, 810–813. doi: 10.1161/STROKEAHA.109.570168
Qing, W. G., Dong, Y. Q., Ping, T. Q., Lai, L. G., Fang, L. D., Min, H. W., et al. (2009). Brain edema after intracerebral hemorrhage in rats: the role of iron overload and aquaporin 4 Laboratory investigation. J. Neurosurg. 110, 462–468. doi: 10.3171/2008.4.JNS17512
Schipper, H. M., Song, W., Tavitian, A., and Cressatti, M. (2019). The sinister face of heme oxygenase-1 in brain aging and disease. Prog. Neurobiol. 172, 40–70. doi: 10.1016/j.pneurobio.2018.06.008
Selim, M. (2009). Deferoxamine mesylate: a new hope for intracerebral hemorrhage: from bench to clinical trials. Stroke 40, S90–S91. doi: 10.1161/STROKEAHA.108.533125
Selim, M., Foster, L. D., Moy, C. S., Xi, G. H., Hill, M. D., Morgenstern, L. B., et al. (2019). Deferoxamine mesylate in patients with intracerebral haemorrhage (i-DEF): a multicentre, randomised, placebo-controlled, double-blind phase 2 trial. Lancet Neurol. 18, 428–438. doi: 10.1016/S1474-4422(19)30069-9
Selim, M., Yeatts, S., Goldstein, J. N., Gomes, J., Greenberg, S., Morgenstern, L. B., et al. (2011). Safety and tolerability of deferoxamine mesylate in patients with acute intracerebral hemorrhage. Stroke 42, 3067–U3237. doi: 10.1161/STROKEAHA.111.617589
Sheng, Z., Liu, Y., Li, H., Zheng, W., Xia, B., Zhang, X., et al. (2018). Efficacy of minocycline in acute ischemic stroke: a systematic review and meta-analysis of rodent and clinical studies. Front. Neurol. 9:1103. doi: 10.3389/fneur.2018.01103
Stockwell, B. R., Friedmann Angeli, J. P., Bayir, H., Bush, A. I., Conrad, M., Dixon, S. J., et al. (2017). Ferroptosis: a regulated cell death nexus linking metabolism, redox biology, and disease. Cell 171, 273–285. doi: 10.1016/j.cell.2017.09.021
Wagner, K. R., Sharp, F. R., Ardizzone, T. D., Lu, A., and Clark, J. F. (2003). Heme and iron metabolism: role in cerebral hemorrhage. J. Cereb. Blood Flow Metab. 23, 629–652. doi: 10.1097/01.WCB.0000073905.87928.6D
Wan, J., Ren, H., and Wang, J. (2019). Iron toxicity, lipid peroxidation and ferroptosis after intracerebral haemorrhage. Stroke Vasc Neurol 4, 93–95. doi: 10.1136/svn-2018-000205
Wang, G., Wang, L., Sun, X. G., and Tang, J. (2018). Haematoma scavenging in intracerebral haemorrhage: from mechanisms to the clinic. J. Cell. Mol. Med. 22, 768–777. doi: 10.1111/jcmm.13441
Wang, J., and Dore, S. (2007). Heme oxygenase-1 exacerbates early brain injury after intracerebral haemorrhage. Brain 130, 1643–1652. doi: 10.1093/brain/awm095
Wessling-Resnick, M. (2010). Iron homeostasis and the inflammatory response. Annu. Rev. Nutr. 30, 105–122.
Wu, H., Wu, T., Xu, X., Wang, J., and Wang, J. (2011). Iron toxicity in mice with collagenase-induced intracerebral hemorrhage. J. Cereb. Blood Flow Metab. 31, 1243–1250. doi: 10.1038/jcbfm.2010.209
Wu, J., Hua, Y., Keep, R. F., Schallert, T., Hoff, J. T., and Xi, G. (2002). Oxidative brain injury from extravasated erythrocytes after intracerebral hemorrhage. Brain Res. 953, 45–52. doi: 10.1016/s0006-8993(02)03268-7
Xi, G., Keep, R. F., and Hoff, J. T. (1998). Erythrocytes and delayed brain edema formation following intracerebral hemorrhage in rats. J. Neurosurg. 89, 991–996. doi: 10.3171/jns.1998.89.6.0991
Xie, Q., Gu, Y., Hua, Y., Liu, W., Keep, R. F., and Xi, G. (2014). Deferoxamine attenuates white matter injury in a piglet intracerebral hemorrhage model. Stroke 45, 290–292. doi: 10.1161/STROKEAHA.113.003033
Xiong, X. Y., Wang, J., Qian, Z. M., and Yang, Q. W. (2014). Iron and intracerebral hemorrhage: from mechanism to translation. Transl. Stroke Res. 5, 429–441. doi: 10.1007/s12975-013-0317-7
Xue, M., and Yong, V. W. (2020). Neuroinflammation in intracerebral haemorrhage: immunotherapies with potential for translation. Lancet Neurol. 19, 1023–1032. doi: 10.1016/S1474-4422(20)30364-1
Xue, M. Z., and Del Bigio, M. R. (2005). Injections of blood, thrombin, and plasminogen more severely damage neonatal mouse brain than mature mouse brain. Brain Pathol. 15, 273–280. doi: 10.1111/j.1750-3639.2005.tb00111.x
Xue, M. Z., Mikliaeva, E. I., Casha, S., Zygun, D., Demchuk, A., and Yong, V. W. (2010). Improving outcomes of neuroprotection by minocycline guides from cell culture and intracerebral hemorrhage in Mice. Am. J. Pathol. 176, 1193–1202. doi: 10.2353/ajpath.2010.090361
Yang, G., Qian, C., Zhang, C., Bao, Y., Liu, M. Y., Jiang, F., et al. (2021). Hepcidin attenuates the iron-mediated secondary neuronal injury after intracerebral hemorrhage in rats. Transl. Res. 229, 53–68. doi: 10.1016/j.trsl.2020.09.002
Yang, G. Y., Betz, A. L., Chenevert, T. L., Brunberg, J. A., and Hoff, J. T. (1994). Experimental intracerebral hemorrhage - relationship between brain edema, blood-flow, and blood-brain-barrier permeability in rats. J. Neurosurg. 81, 93–102. doi: 10.3171/jns.1994.81.1.0093
Yeatts, S. D., Palesch, Y. Y., Moy, C. S., and Selim, M. (2013). High dose deferoxamine in intracerebral hemorrhage (Hi-Def) trial: rationale, Design, and Methods. Neurocrit. Care 19, 257–266. doi: 10.1007/s12028-013-9861-y
Yong, H. Y. F., Rawji, K. S., Ghorbani, S., Xue, M., and Yong, V. W. (2019). The benefits of neuroinflammation for the repair of the injured central nervous system. Cell Mol. Immunol. 16, 540–546. doi: 10.1038/s41423-019-0223-3
Zeng, L., Tan, L., Li, H., Zhang, Q., Li, Y., and Guo, J. (2018). Deferoxamine therapy for intracerebral hemorrhage: a systematic review. PLoS One 13:e0193615. doi: 10.1371/journal.pone.0193615
Zhang, R., Xue, M., and Yong, V. W. (2021). Central nervous system tissue regeneration after intracerebral hemorrhage: the next frontier. Cells 10:2513. doi: 10.3390/cells10102513
Zhang, R., Yong, V. W., and Xue, M. (2022a). Revisiting minocycline in intracerebral hemorrhage: mechanisms and clinical translation. Front. Immunol. 13:844163. doi: 10.3389/fimmu.2022.844163
Zhang, Y., Khan, S., Liu, Y., Wu, G., Yong, V. W., and Xue, M. (2022b). Oxidative stress following intracerebral hemorrhage: from molecular mechanisms to therapeutic targets. Front. Immunol. 13:847246. doi: 10.3389/fimmu.2022.847246
Zhang, Y., Zhang, X., Wee Yong, V., and Xue, M. (2022c). Vildagliptin improves neurological function by inhibiting apoptosis and ferroptosis following intracerebral hemorrhage in mice. Neurosci. Lett. 776:136579. doi: 10.1016/j.neulet.2022.136579
Zhang, X. Y., Cao, J. B., Zhang, L. M., Li, Y. F., and Mi, W. D. (2015). Deferoxamine attenuates lipopolysaccharide-induced neuroinflammation and memory impairment in mice. J. Neuroinflammation 12:20. doi: 10.1186/s12974-015-0238-3
Zhang, Y., Khan, S., Liu, Y., Wu, G., Tang, Z., and Xue, M. (2022d). Modes of Brain Cell Death Following Intracerebral Hemorrhage. Front. Cell Neurosci. 16:799753. doi: 10.3389/fncel.2022.799753
Zhao, F., Xi, G. H., Liu, W. Q., Keep, R. F., and Hua, Y. (2016). Minocycline attenuates iron-induced brain injury. Acta. Neurochir. Suppl. 121, 361–365. doi: 10.1007/978-3-319-18497-5_62
Zhou, Y., Wang, Y., Wang, J., Anne Stetler, R., and Yang, Q. W. (2014). Inflammation in intracerebral hemorrhage: from mechanisms to clinical translation. Prog. Neurobiol. 115, 25–44. doi: 10.1016/j.pneurobio.2013.11.003
Zhu, F., Zi, L., Yang, P., Wei, Y., Zhong, R., Wang, Y., et al. (2021). Efficient Iron and ROS Nanoscavengers for Brain Protection after Intracerebral Hemorrhage. ACS Appl. Mater. Interfaces 13, 9729–9738. doi: 10.1021/acsami.1c00491
Keywords: intracerebral hemorrhage, brain edema, deferoxamine, iron overload, neuronal death
Citation: Li Z, Liu Y, Wei R, Khan S, Zhang R, Zhang Y, Yong VW and Xue M (2022) Iron Neurotoxicity and Protection by Deferoxamine in Intracerebral Hemorrhage. Front. Mol. Neurosci. 15:927334. doi: 10.3389/fnmol.2022.927334
Received: 24 April 2022; Accepted: 20 May 2022;
Published: 16 June 2022.
Edited by:
Qiang Liu, Tianjin Medical University General Hospital, ChinaReviewed by:
Mingming Liu, Tianjin Medical University General Hospital, ChinaWenyan He, Capital Medical University, China
Copyright © 2022 Li, Liu, Wei, Khan, Zhang, Zhang, Yong and Xue. This is an open-access article distributed under the terms of the Creative Commons Attribution License (CC BY). The use, distribution or reproduction in other forums is permitted, provided the original author(s) and the copyright owner(s) are credited and that the original publication in this journal is cited, in accordance with accepted academic practice. No use, distribution or reproduction is permitted which does not comply with these terms.
*Correspondence: Voon Wee Yong, vyong@ucalgary.ca; Mengzhou Xue, xuemengzhou@zzu.edu.cn