- 1Department of Pediatrics, Xiangya Hospital, Central South University, Changsha, China
- 2Hunan Intellectual and Developmental Disabilities Research Center, Changsha, China
- 3Department of Neurology, Children’s Hospital Affiliated to Zhengzhou University, Henan Children’s Hospital, Zhengzhou Children’s Hospital, Zhengzhou, China
- 4Department of Anesthesiology and Pharmacology, University of British Columbia, Vancouver, BC, Canada
Background: Attention-deficit/hyperactive disorder (ADHD) is a neurodevelopmental disorder that commonly occurs in children with a prevalence ranging from 3.4 to 7.2%. It profoundly affects academic achievement, well-being, and social interactions. As a result, this disorder is of high cost to both individuals and society. Despite the availability of knowledge regarding the mechanisms of ADHD, the pathogenesis is not clear, hence, the existence of many challenges especially in making correct early diagnosis and provision of accurate management.
Objectives: We aimed to review the pathogenic pathways of ADHD in children. The major focus was to provide an update on the reported etiologies in humans, animal models, modulators, therapies, mechanisms, epigenetic changes, and the interaction between genetic and environmental factors.
Methods: References for this review were identified through a systematic search in PubMed by using special keywords for all years until January 2022.
Results: Several genes have been reported to associate with ADHD: DRD1, DRD2, DRD4, DAT1, TPH2, HTR1A, HTR1B, SLC6A4, HTR2A, DBH, NET1, ADRA2A, ADRA2C, CHRNA4, CHRNA7, GAD1, GRM1, GRM5, GRM7, GRM8, TARBP1, ADGRL3, FGF1, MAOA, BDNF, SNAP25, STX1A, ATXN7, and SORCS2. Some of these genes have evidence both from human beings and animal models, while others have evidence in either humans or animal models only. Notably, most of these animal models are knockout and do not generate the genetic alteration of the patients. Besides, some of the gene polymorphisms reported differ according to the ethnic groups. The majority of the available animal models are related to the dopaminergic pathway. Epigenetic changes including SUMOylation, methylation, and acetylation have been reported in genes related to the dopaminergic pathway.
Conclusion: The dopaminergic pathway remains to be crucial in the pathogenesis of ADHD. It can be affected by environmental factors and other pathways. Nevertheless, it is still unclear how environmental factors relate to all neurotransmitter pathways; thus, more studies are needed. Although several genes have been related to ADHD, there are few animal model studies on the majority of the genes, and they do not generate the genetic alteration of the patients. More animal models and epigenetic studies are required.
Introduction
Attention-deficit/hyperactive disorder (ADHD) is a neurodevelopmental disorder that is characterized by hyperactivity, inattention, impulsivity, and problems in social interaction and academic performance [Diagnostic and Statistical Manual of Mental Disorders, 5th edition (DSM-5)]. The predominantly hyperactive/impulsive, predominantly inattentive, and combined types are the major three forms that exist (DSM-5). It most commonly occurs in children with prevalence rates ranging from 3.4 to 7.2% (Polanczyk et al., 2015; Thomas et al., 2015). It affects children aged 6 to 17 years (Berger, 2011); however, it can persist into adulthood in about 60 to 80% (Childress and Berry, 2012). Males are more affected compared to females (Childress and Berry, 2012). This disorder profoundly affects the academic achievement, well-being, and social interactions of children (Klein et al., 2012; Groman and Barzman, 2014), as a result, it brings high costs for both individuals and society (Pelham et al., 2007).
The phenotype is complex and heterogeneous, presenting with variable clinical features, developmental course, and outcome. The diagnosis of ADHD is typically determined in the school-age years; however, it can occur from early childhood. It is very difficult to diagnose young children due to their inability to cooperate well, and this happens because diagnosis relies much on interviews and observations according to the rating scale. There is no available standardized protocol for the auxiliary examinations which can assist in diagnosis despite the presence of many studies. Moreover, ADHD is frequently comorbid with other conditions in about 75% of the cases (Pliszka, 1998; Fayyad et al., 2007), including anxiety disorders, oppositional defiant disorder, mood disorders, substance use disorders, and conduct disorders (Rizzo et al., 2010; Nogueira et al., 2014; Tokko et al., 2022). All the aforementioned issues make this disorder more complex (Dias et al., 2013).
Although the etiology and pathophysiology remain unclear, there is some evidence supporting it to occur due to an interaction between genetic and environmental factors (Sun et al., 2018). Neurotransmitters including dopamine and norepinephrine acting in conjunction with each other via multiple receptors influence the functioning of the prefrontal cortex, cerebellum, and caudate, which are responsible for the regulation of attention, thoughts, emotions, behavior, and actions (Pliszka, 2005; Arnsten and Pliszka, 2011). These areas have also emerged as primary areas showing deficits in ADHD (Arnsten and Pliszka, 2011). Polymorphisms of the genes that encode dopamine receptor D4 (DRD4), dopamine transporter 1 (DAT-1), and 5-hydroxytryptamine receptor 1 (HTR1) have been reported to cause dysfunction of the neurotransmission (Gizer et al., 2009; Akutagava-Martins et al., 2013; Bonvicini et al., 2018). Microelements deficiencies including zinc, magnesium, and iron can affect the production of neurotransmitters (Villagomez and Ramtekkar, 2014; Hariri and Azadbakht, 2015). Despite multiple studies on ADHD, the pathogenesis is not clear, hence, the existence of many challenges especially in making an early and correct diagnosis, and provision of accurate management.
Consequently, we aimed to review the pathogenic pathways of ADHD in children. The major focus was to provide an update of the reported etiologies in humans, animal models, modulators, mechanisms, therapies (pharmacological and dietary), and the interaction between genetic and environmental factors. This update will deepen the understanding of the pathogenesis, the interaction between genetic and environmental factors, and possibly provide molecular targets for the development of accurate therapies.
Methods
References for this review were identified through a systematic search in PubMed. Keywords used were the combination of Attention-Deficit/Hyperactivity Disorder or ADHD and gene or copy number variations or microRNAs or environmental factors or social factors or diet or epigenetics or neuroimaging or animal model or modulators, or pharmacology or mechanisms or pathways for all years until January 2022. This review included only papers published in English regarding ADHD, animal models, gene mutations, copy number variations, micro-RNAs, neuro-imaging changes, environmental factors, epigenetic changes, pathways, dietary, and pharmacological treatments. It excluded abstracts, patents, book chapters, and conference papers. The final reference list was generated based on the originality and relevance to the broad scope of this review.
Results
Several genes have been related to ADHD in human beings from different parts of the world as shown in Supplementary Table 1. There are some microRNAs reported to relate to ADHD (Supplementary Table 2). Environmental and social biomarkers reported to relate to ADHD can be found in Supplementary Table 3. Table 1 provides a summary of different neurotransmitters and neuroreceptors and the roles they play in the pathophysiology and pathogenesis of ADHD, as well as their interactions with environmental/social factors and their respective pharmacology. Genes reported to associate with ADHD in animal models and modulators have been summarized in Table 2. Detailed results and discussion of the common pathogenic pathways and the interaction between genetic and environmental factors can be found in the following sections.
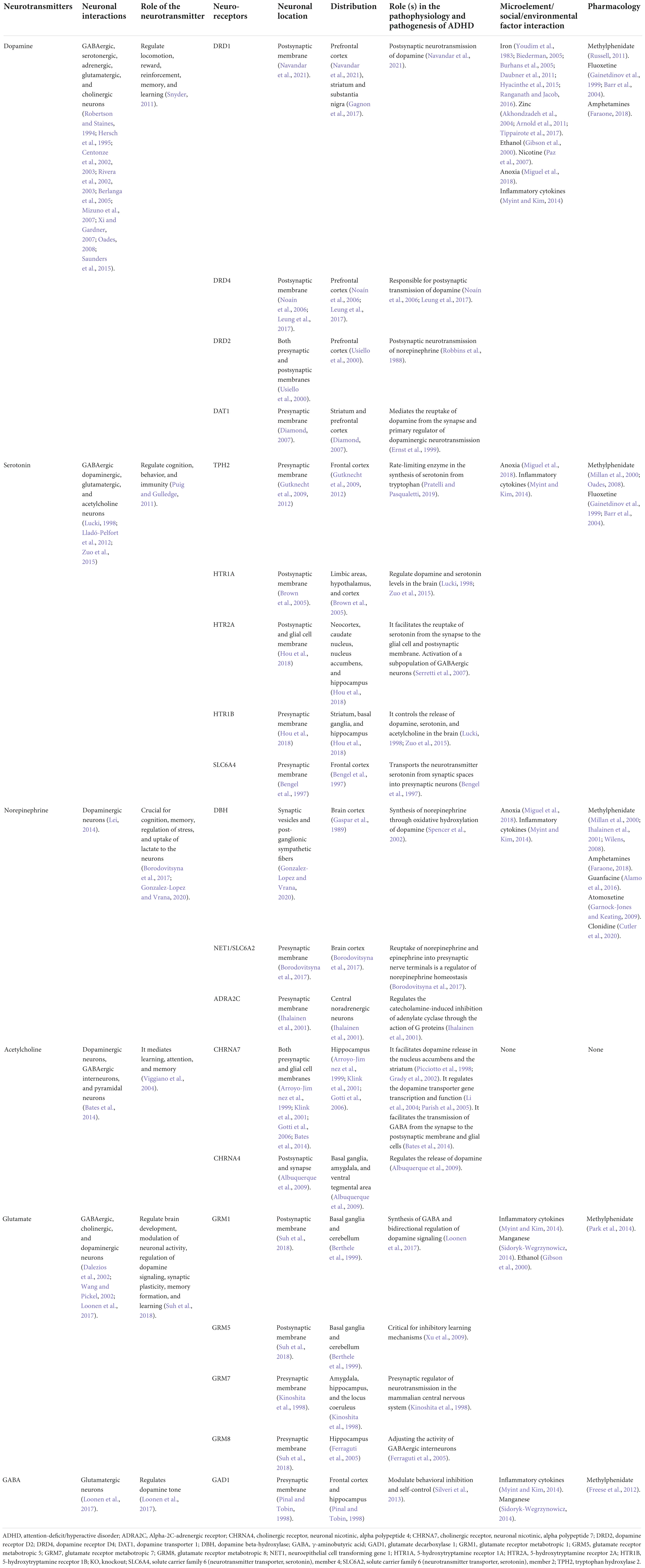
Table 1. A summary of different neurotransmitters and neuroreceptors and the roles they play in the pathophysiology and pathogenesis of ADHD.
Genetic mechanisms and their interactions with environmental factors
Attention-deficit/hyperactive disorder is a heterogeneous and highly heritable disorder resulting from complex gene–gene and gene–environment interactions (Smalley, 1997; Faraone, 2004; Faraone et al., 2005; Banaschewski et al., 2010). Multiple genetic studies support a strong genetic contribution to the disorder’s phenotype. Biological parents and siblings of patients with ADHD have a two- to eight-fold risk of having it too (Biederman et al., 1990, 1992). The heritability is estimated to be 0.76 for childhood ADHD (Faraone et al., 2005). The usage of genome-wide association studies has found many variants that are implicated in ADHD, including common and rare ones from a broad range of genes related to neurotransmission and neurodevelopment (Akutagava-Martins et al., 2013). The results from the copy number variations (CNVs) tests consolidate the idea that, alongside common variants, rare variants are involved in ADHD etiology (Williams et al., 2012; Martin et al., 2015). This includes rare recurrent variants in non-coding regions as revealed by the whole genome sequencing from the children of both African American and European American ancestry (Liu et al., 2021a). Furthermore, miRNA studies based on animal models, cell lines, or human subjects show the emerging trend of miRNA in ADHD (Srivastav et al., 2018).
Multiple genes have been reported to associate with ADHD in children. All reported genes have a significant association with neurotransmission and neurodevelopment which can explain ADHD etiology and pathophysiology (Figure 1). They fall under six pathways: dopaminergic, serotonergic, adrenergic, cholinergic, glutamatergic, and GABAergic, and can interact with environmental factors as shown in Table 1. Some of the reported genes in humans have evidence from animal models while others don’t (Table 2).
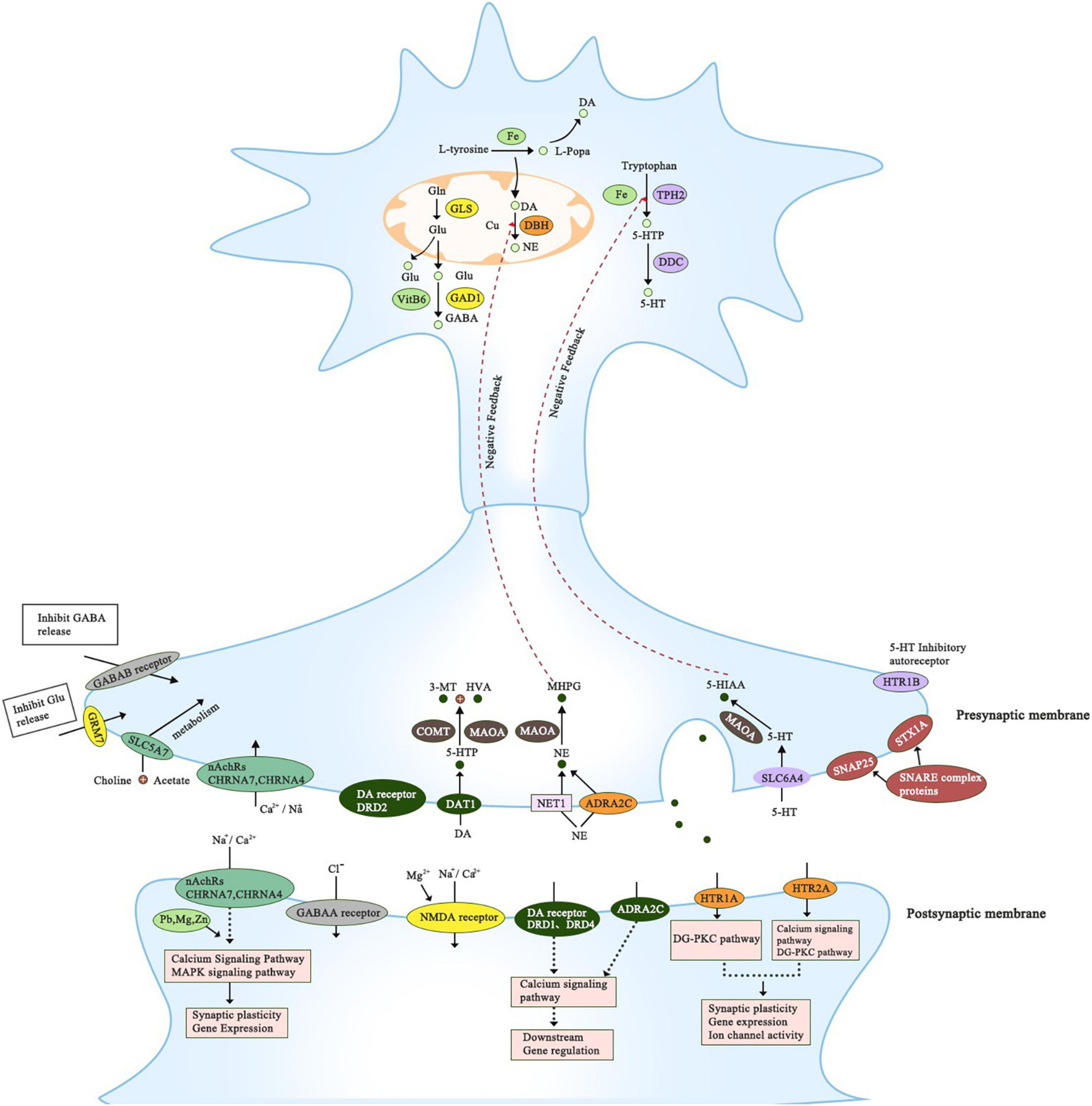
Figure 1. This figure summarizes all genes that have been reported to associate with ADHD. It includes genes that belong to the proposed pathways; dopaminergic, serotonergic, adrenergic, cholinergic, glutamatergic, and GABAergic. The precise location of the gene is presented; axon, presynaptic and postsynaptic compartments. All of the genes have a significant role in regulating dopamine neurotransmission directly or indirectly.
Genes and microRNAs related to dopaminergic pathway
Dopamine exerts its effects in the frontal cortex through GABAergic neurons (Saunders et al., 2015; Kramer et al., 2020), noradrenergic (Zhu, 2018), as well as serotonergic neurons (Di Matteo et al., 2008). Dopamine neurotransmission comprises numerous dopamine signaling pathways: mesolimbic (dopaminergic neurons projecting from the ventral tegmental area to the nucleus accumbens), neocortical (dopaminergic neurons projecting from the ventral tegmental area to the frontal cortex), and mesostriatal (dopaminergic neurons projecting from the ventral tegmental area to the caudate putamen) (Chen et al., 2016). Signals transmitted by dopamine regulate emotion, reward, locomotion, complex behavior, and cognition (Snyder, 2011). Dysfunction of dopamine signaling in the forebrain leads to inappropriate or deficient attention (Huang et al., 2015). Animal models of ADHD showed dysregulation of dopamine functions and abnormal behaviors which were normalized upon administration of stimulant medications (Russell, 2011). Some studies based on children with ADHD identified frontal cortical regions, which are rich in dopamine, as sites related to ADHD (McAlonan et al., 2007, 2009). Additionally, several studies have shown that the dopamine receptor density in several brain regions of ADHD patients is lower compared to healthy individuals (Jönsson et al., 1999; Medin et al., 2013). Notably, it has been revealed that it is the elevation of the frontal cortical dopamine levels and not norepinephrine or serotonin that acts as a convergent mechanism for the paradoxical effects of ADHD psychostimulants therapies (Harris et al., 2022). ADHD is associated with higher mortality and lowered estimated life expectancy by adulthood and some of the dopaminergic SNP has been reported to relate to 5- and 2-year reductions in estimated life expectancy (Barkley et al., 2019). Consequently, the dopaminergic pathway has a crucial role to play in the occurrence of ADHD. Genes reported in this pathway include DRD1, DRD2, DRD4, and DAT1.
DRD1
DRD1 gene encodes for DRD1 (dopamine receptor D1) receptor. DRD1 is found in the postsynaptic membrane where it regulates the postsynaptic transmission of dopamine. It is expressed in excitatory neurons in the prefrontal cortex (Navandar et al., 2021). It is a G-protein coupled receptor (GPCR) that activates adenylyl cyclase after dopamine binds to it (Undieh, 2010). After the activation of the adenylyl cyclase, there is a generation of the cyclic AMP (cAMP) which in turn activates protein kinase A and cAMP-mediated gene expression (Sassone-Corsi, 2012). There is an association between DRD1 polymorphisms and childhood ADHD according to the case-control study conducted in Spain (Ribasés et al., 2012). Another study which was conducted among 232 families revealed that DRD1 polymorphisms are more related to the inattention phenotype (Luca et al., 2007). Likewise, another case-control study revealed a relationship between DRD1 polymorphisms and ADHD (Bobb et al., 2005). It has been shown that the hypofunction of DRD1-mediated regulation of GABAergic inhibitory synaptic transmission onto layer V pyramidal cells of the anterior cingulate cortex might play a role in the pathophysiology of ADHD as demonstrated in spontaneously hypertensive rats (Satoh et al., 2018).
DRD2
DRD2 gene encodes for DRD2 (dopamine receptor D2) receptor. DRD2 is highly expressed in the prefrontal cortex, responsible for locomotion, reward, reinforcement, memory, and learning (Usiello et al., 2000). DRD2 is a GPCR that inhibits adenylyl cyclase, found in the dopaminergic synapse. DRD2 is found in both presynaptic and postsynaptic membranes, and facilitates the reuptake of dopamine from the synapse to the presynaptic membrane via DAT1. When dopamine binds to the DRD2, it inhibits adenylate cyclase and thus decreasing intracellular concentrations of cAMP, hence the change in intracellular and extracellular concentrations of ions causing hyperpolarization of cell membrane which affects the transmission of the impulse. Meanwhile, it can also activate mitogen-activated protein kinase (MAPK) which in turn activates the calcium signaling pathway which influences synaptic plasticity, affects downstream gene regulation, long- and short-excitability as well as cell death or survival. It is also responsible for postsynaptic neurotransmission of NE (Robbins et al., 1988).
There are some evidence from human beings that relate DRD2 polymorphisms and ADHD (Supplementary Table 1). DRD2 genetic predisposition is associated with aggressive behavior, memory dysfunction, and ADHD (Fernandez et al., 2022). DRD2 Taq1A (rs1800497) (Neville et al., 2004; Pan et al., 2015) has been reported to relate to DRD2 expression levels (Laakso et al., 2005). DRD2-12 (rs7131465) is related to a higher risk for ADHD and autism spectrum disorder overlap (Mariggiò et al., 2021). The intronic DRD2 SNPs (rs1079727, rs1079595, and rs1124491), and SNP in the 3’-UTR (rs1800497) have been linked with ADHD in males (Nyman et al., 2007). T allele of rs1800497 was also identified as a risk of ADHD in a meta-analysis (Pan et al., 2015). The interaction of ADORA2A and DRD2 genes has been reported to be responsible for anxiety disorders in ADHD children and adolescence (Fraporti et al., 2019). There is a significant interaction effect of DRD2 rs1800497 and lead exposure on the cortical thickness of the prefrontal cortex in ADHD according to the case-control study (Kim et al., 2018a). Currently, there is no DRD2 animal model for ADHD.
DRD4
DRD4 gene encodes DRD4 (dopamine receptor D4) receptor. This receptor is a GPCR that inhibits adenylyl cyclase, found in the dopaminergic synapse. DRD4 is expressed in the prefrontal cortex including the anterior cingulate and orbitofrontal cortex, which are regions predominantly affected in ADHD (Noaín et al., 2006; Leung et al., 2017). DRD4 is located in the postsynaptic membrane and is responsible for the postsynaptic transmission of dopamine. Similar to DRD2, when dopamine binds to the DRD4, it inhibits adenylate cyclase and thus decreases intracellular concentrations of cAMP, hence change in intracellular and extracellular concentrations of ions causing hyperpolarization of cell membrane which affects the transmission of the impulse. Meanwhile, it can also activate MAPK which in turn triggers the calcium signaling pathway which influences synaptic plasticity, affects downstream gene regulation, long-and-short excitability, as well as cell death or survival.
There are some evidence both from humans (Supplementary Table 1) and animal models (Table 2) to support the relationship between DRD4 gene polymorphisms and ADHD. However, it is worth noting that most of the animal models are knockouts of which the majority do not replicate the full manifestation of ADHD as seen in patients. The variable number of tandem repeats (VNTR) in exon 3 of the DRD4 gene has been implicated in the etiology of ADHD (Chang et al., 1996; Bidwell et al., 2011). Interestingly, different populations have different risk alleles, for instance, the 2-repeat allele (2R) has been noticed in the Han Chinese (Chang et al., 1996) and Koreans (Hong et al., 2018), 4-repeat allele (4R) for Asians while 7-repeat allele (7R) for white Europeans and Americans (Faraone et al., 1999; Hawi et al., 2000; Shahin et al., 2015; Tabatabaei et al., 2017). A haplotype of markers: allele 2 of the 120 bp duplication, 616 C/G substitutions, and 521 C/T substitutions of the C allele can confer a predisposition to ADHD (Mill et al., 2003). DRD4 7R allele is related to reduced prefrontal gyrification in children with ADHD (Palaniyappan et al., 2019). DRD4 2R allele can affect the default mode network, the executive control network, and the sensorimotor network in the prefrontal cortex circles (Qian et al., 2018a). According to functional magnetic resonance imaging, DRD4 polymorphisms are associated with abnormality in the frontal–striatal–cerebellar loop among ADHD children (Qian et al., 2018b). The sensitive allele repeats in the DRD4 gene have been suggested as a prognostic factor (Tabatabaei et al., 2017).
Besides, DRD4-rs916457 has been linked with abnormal neuropsychological tasks outlining working memory and perceptual organization in ADHD patients (Cervantes-Henriquez et al., 2021). The DRD4-521 C/T SNP regulates the transcription rate and expression for DRD4 which affects the level of dopamine in the brain (Zhang et al., 2021a). DRD4 and α2AR co-localize in cortical pyramidal neurons to form α2AR-D4R heteromers which play a pivotal role in catecholaminergic signaling in the brain cortex and are potential targets for ADHD pharmacotherapy (Casadó-Anguera et al., 2021). SNPs in DRD4 relate to distinct domains of ADHD severity (Cervantes-Henríquez et al., 2022). The 7R allele of the DRD4 gene is more prevalent in sluggish cognitive cases than in ADHD cases (Bolat et al., 2020). It has been reported that the interaction between the methylation of CpG sites of DRD4 (CpG26 and CpG28) and phthalate metabolite levels can affect the attention level in ADHD patients (Kim et al., 2021). It has been shown that Drd4 knockout mice demonstrate hyperactivity and impaired behavioral inhibition signifying that intact Drd4 signaling during development is essential for the development of ADHD phenotype (Avale et al., 2004). In another Drd4 knockout mice study, it has been revealed that a lack of DRD4 is not adequate to cause impulsivity (Helms et al., 2008). Viral expression of the DRD4 7R allele in the prefrontal cortex of Drd4 knockout mice exhibits augmented exploratory and novelty-seeking behaviors, mimicking human ADHD (Qin et al., 2016). This DRD4 7R allele causes over-suppression of NMDA receptor function in the prefrontal cortex (Qin et al., 2016). Drd4 knock-in mice demonstrate augmented DRD4-mediated dopaminergic regulators of corticostriatal transmission which is associated with ADHD (Bonaventura et al., 2017). Transgenic mice (DRD4–/– and DRD4±) showed that reduced DRD4 expression increases extracellular levels of glutamate neurotransmission in the striatum of DRD4–/– mice (Thomas et al., 2009).
In regard to pharmacology, children carrying DRD4 homozygous long 7-repeat allele showed better behavioral response to methylphenidate (Naumova et al., 2019). A recent meta-analysis suggested that DRD4 48 bp VNTR variants can be considered as ADHD biomarkers to support the diagnosis and to predict methylphenidate response in children (Bonvicini et al., 2020). Besides, the interaction between methylation of CpG7 of DRD4 and prenatal maternal stress has been suggested as a possible predictor of the methylphenidate response in youth with ADHD (Kim et al., 2018b). DRD4 4R showed a significant association with methylphenidate response according to one meta-analysis (Myer et al., 2018). Children homozygous for the DRD4 7R allele showed a better response to methylphenidate according to the placebo-controlled trial (Naumova et al., 2019). The interaction between genetic polymorphisms of DRD4 and organophosphate pesticide exposure as well as oxidative stress increases the risk of ADHD in children (Chang et al., 2018). It has been shown that fetal methylphenidate exposure prompts ADHD-like phenotypes and reduces Drd2 and Slc6a3 expression levels in mouse descendants (Aoki et al., 2021).
DAT1
DAT1 gene encodes for dopamine transporter 1 (DAT) which is located in the presynaptic membrane (Diamond, 2007). It is more expressed in the striatum and prefrontal cortex (Diamond, 2007). It mediates the reuptake of dopamine from the synapse and primary regulator of dopaminergic neurotransmission (Ernst et al., 1999). Reduction in expression of DAT1 can reduce reuptake and increase the metabolism of dopamine (Ernst et al., 1999). There are some evidence both from humans (Supplementary Table 1) and knockout animal models (Table 2) to support the relationship between DAT1 gene polymorphisms and ADHD or some of the ADHD phenotypes. DAT1 VNTR polymorphism can modulate working memory in ADHD children (Pineau et al., 2019). The 6R and 10R have been considered as risk alleles for childhood ADHD while 9R has been associated with adulthood ADHD (Chen et al., 2003; Mill et al., 2005a; Brown et al., 2011; Agudelo et al., 2015). The 10R/10R genotype of DAT1 VNTRs is related to ADHD in Korean children (Hong et al., 2018). The haplotype rs27048 (C)/rs429699 (T) has been suggested as a genetic marker for the inattentive ADHD subtype in the Chinese population (Shang et al., 2011). Likewise, rs27072 polymorphism is related to inattention and hyperactivity/impulsivity (Ouellet-Morin et al., 2008). It has been reported that there is no association between VNTR polymorphism of DAT1 and DRD4 genes and ADHD among Indonesian children based on case-control study (Thursina et al., 2020). DAT1 9 R carriers have a higher likelihood of high traffic risk behavior in case only they also have ADHD symptoms (Tokko et al., 2022).
DAT-knockout rats show impairment in learning the cognitive task, however, their hyperactivity does not prevent the capability to learn a non-spatial cognitive task in the appearance of novel stimuli (Kurzina et al., 2021). Importantly, DAT genetic hypofunction in mice produced alterations consistent with ADHD, but not with schizophrenia or bipolar disorders (Mereu et al., 2017) in one study, but it was shown in another study that DAT silencing in the rat produces abnormalities in the prefrontal-midbrain and in striato-cerebellar circuits leading to motor hyperactivity and compulsive-like behaviors applicable for ADHD, schizophrenia, and obsessive-compulsive disorder (Reinwald et al., 2022). DAT hypofunctional mice exhibit hyperactivity, attentional and impulsivity, and decreased expression of Homer1a in the prefrontal cortex, notably, amphetamine treatments rescued hyperactivity and cognitive deficits (Mereu et al., 2017). DAT knockout also exhibits anxiety, novelty seeking, and stereotypical-perseverative spectrum (Pogorelov et al., 2005). DAT-Cnr2 conditional knockout mice (mice lacking cannabinoid CB2 receptors) in midbrain dopaminergic neurons demonstrated a hyperactivity phenotype of ADHD (Canseco-Alba et al., 2021). P35 knockout mice (ADHD animal model) display reduced dopamine uptake and cell surface DAT expression levels in the striatum. DAT1 gene 5′-UTR methylation has been suggested as a new approach for the exploration of an epigenetic biomarker in ADHD diagnosis. Paternal nicotine exposure prompts hyperactivity in C57BL/6 mice next-generation through down-regulating the expression of DAT which results from hyper-methylation of DAT (Zhang et al., 2020).
There are several DAT postnatal epigenetic modifiers revealed in rat brain (Green et al., 2019). Dnmt1, Dnmt3a, Dnmt3b, and Hdac2 exhibit age-related decreases in DNA mRNA expression while Hdac5 and Hdac8 demonstrate increased mRNA expression with age (Green et al., 2019). Besides, there is a protein enhancement of acetylated histone 3 at lysines 9 and 14 as well as the transcription factors (dopaminergic) Nurr1 and Pitx3 within the DAT promoter according to age (Green et al., 2019). The loss of the Slc6a8 (creatine) gene in DAT (Slc6a3) expressing cells leads to hyperactivity but sparing motor function as shown in DAT-specific Slc6a8 knockouts (dCrt-/y) (Abdulla et al., 2020). SUMOylation plays a critical role in regulating DAT protein hemostasis, dopamine uptake, and dopamine signaling in neurons; therefore, DAT SUMOylation can be the potential therapeutic target in regulating DAT stability and dopamine clearance in ADHD (Cartier et al., 2019).
MicroRNAs and dopaminergic pathway
There is a close relationship between levels of circulating miRNAs and ADHD. Wu et al. (2010) studied spontaneously hypertensive rats whereby they discovered miRNA let-7d as an important regulator of galectin-3 expression in the prefrontal cortex which is the affected area in ADHD. They noticed low levels of galectin-3 expression in the prefrontal cortex, which is important for dopamine metabolism (Wu et al., 2010). Then, they investigated serum miRNA let-7d in children with ADHD in comparison with controls, whereby, they found high circulating miRNA let-7d in ADHD subjects than in controls (Wu et al., 2015). Kandemir et al. (2014) found decreased levels of miR18a-5p, miR22-3p, miR24-3p, miR106b-5p, and miR107 in ADHD subjects compared with controls. Additional reported miRNAs include miR-125b-5p, miR-155-5p, miR-138-1, miR-296, miR-34c, miR-34b-3p, miR-34c-3p, miR-let-7d, miR-96, miR-641, miR-30b-5p, miR-1301, miR-6070, and miR-5692b (Wu et al., 2010, 2015, 2017; Németh et al., 2013; Sánchez-Mora et al., 2013; Kandemir et al., 2014; Šerý et al., 2015; Garcia-Martínez et al., 2016; Aydin et al., 2019). MiR-384-5p overexpression increases phosphorylation of the cAMP response element-binding protein (CREB) and reduces DAT levels in the prefrontal cortex of spontaneously hypertensive rats, whereas, miR-384-5p suppression increases DRD1 and decreases DAT and CREB protein levels (Xu et al., 2019). High levels of miR-132-3p have been identified in ADHD children (Coskun et al., 2021). More details about microRNAs and their associated gene(s) are given in Supplementary Table 2.
Dopaminergic pathway and environmental/social interactions
Iron helps in the homeostasis of the hemoglobin structure, antioxidants, genetic repair, and in particular, central nervous system function (Chen et al., 2013). To date, four meta-analyses have evaluated peripheral iron levels in children with ADHD (Biederman, 2005; Bonvicini et al., 2016; Wang et al., 2017; Tseng et al., 2018). The most recent one indicated that children with iron deficiency have more severe symptoms as compared to those without iron deficiency. Lower serum ferritin levels and iron deficiency are the risk factor for ADHD which can be explained by the following possible pathophysiological mechanisms. First, iron deficiency may impair the production of dopamine which has a prominent role in the pathophysiology of ADHD (Biederman, 2005; Burhans et al., 2005; Daubner et al., 2011). Therefore, iron deficiency disrupts dopamine production as shown in several animal studies (Youdim et al., 1983; Hyacinthe et al., 2015; Ranganath and Jacob, 2016). This dysregulation of dopaminergic neurons may further result in multiple frontal dysfunctions that mimic the symptoms of ADHD (Ghorayeb et al., 2019). Second, lower ferritin levels may provide indirect evidence of elevated oxidative stress (Finazzi and Arosio, 2014), and oxidative stress has also been reported in patients with ADHD (Adisetiyo et al., 2014). This increased oxidative stress burden may disturb neurodevelopmental trajectories and gene functions potentially predisposing to the onset of ADHD (Adisetiyo et al., 2014). Despite these evidence, iron supplementation solely does not improve ADHD symptoms (Sever et al., 1997).
Zinc is a very important cofactor for more than 100 enzymes and is required for modulating melatonin and dopamine. Dopamine plays a major role in the pathogenesis of ADHD as has been described before (Lepping and Huber, 2010). Animal and human studies indicated that zinc deficiency is associated with an increased prevalence of hyperactivity (Bettger et al., 1979). Some studies have suggested that zinc is significantly deficient in children with ADHD as compared to controls (Gao et al., 2014). Furthermore, the hair zinc level was positively correlated with inattention and hyperactivity (Tippairote et al., 2017). Lower copper/zinc and lead/zinc ratios and marginally higher zinc and magnesium levels in the hair of ADHD children have been reported (Tippairote et al., 2017). Zinc increased the affinity of methylphenidate to the dopamine transporter, however, zinc supplementations solely could not improve ADHD (Akhondzadeh et al., 2004; Arnold et al., 2011; Tippairote et al., 2017).
Ethanol affects dopamine release and impairs the binding of glutamate to mGluRs in the postsynaptic membrane (Gibson et al., 2000). Parental alcoholism presents an increased risk of offspring with ADHD (Knopik et al., 2005; Eichler et al., 2018). Additionally, rats exposed to ethanol prenatally showed attention deficits that are similar to children with ADHD than controls (Hausknecht et al., 2005). Children whose mothers smoked during pregnancy had a higher incidence of ADHD than controls (Schmitz et al., 2006; Neuman et al., 2007). Animal studies also showed that prenatal nicotine exposure increases locomotor activity in mice (Paz et al., 2007). Neonatal anoxia/hypoxia is another environmental risk that has been reported (Lou, 1996). Neonatal anoxia caused a sequence of acute and persistent neurochemical changes in rat monoaminergic systems as well as transient hyperactivity and spatial memory impairment that persisted into adulthood (Miguel et al., 2018). Additionally, animals exposed to hypoxia presented with impaired executive function associated with tissue atrophy and dopaminergic disturbance in the prefrontal cortex (Miguel et al., 2018).
Exposure to pyrethroid pesticides has been linked with ADHD both in human beings and animals. There are associations between pyrethroid insecticide use and urinary 3-phenoxybenzoic acid concentrations among Korean preschool-age boys (Lee et al., 2020). Mice exposed to pyrethroids demonstrated ADHD symptoms (Richardson et al., 2015) and abnormal dopamine neurotransmission (Bloomquist et al., 2002; Elwan et al., 2006). Maltreatment and emotional trauma correlate significantly with childhood mental disturbances. Famularo et al. (1992) discovered that children who had suffered maltreatment exhibited significantly greater incidences of ADHD than the controls. McLeer et al. (1994) found the most frequent diagnosis of sexually abused children to be ADHD in about 46% of cases. Noteworthy, among children without a strong genetic vulnerability, environmental risk factors become important, however, among children with a substantial genetic vulnerability, the impact of environmental risk factors becomes less important (Rowland et al., 2018). Nevertheless, more studies are needed to evaluate whether other environmental risk factors and parental history can independently predict ADHD occurrence. Rat pups reared in social isolation displayed a variety of behavioral changes, including hyperactivity, impulsivity, aggression, anxiety, and learning and memory deficits (Dalley et al., 2002; Koike et al., 2009).
Genes related to serotonergic pathway
Serotonin exerts its effects in the frontal cortex through GABAergic neurons (Lladó-Pelfort et al., 2012). Serotonin can also interact with dopaminergic, glutamatergic, and acetylcholine neurons (Lucki, 1998; Lladó-Pelfort et al., 2012; Zuo et al., 2015). Signals transmitted by serotonin regulate cognition, behavior, and immunity (Puig and Gulledge, 2011). A disruption in serotonergic development can change the brain’s function, which results in behavioral changes such as depression, anxiety, impulsivity, violence, and irregular appetite (Lucki, 1998; Sheehan et al., 2007). Reduction in serotonergic function has been linked to the impulsive subtype, which is supported by biochemical and pharmacological evidence. Relatively low platelet serotonin levels have been reported in patients with ADHD (Rapoport et al., 1974; Gainetdinov et al., 1999). Furthermore, the hyperactive state of DAT-KO mice was attenuated when fluoxetine (a selective serotonin re-uptake inhibitor) was administered (Gainetdinov et al., 1999; Barr et al., 2004). The reported genes in this pathway include TPH2, HTR1A, HTR1B, SLC6A4, and HTR2A.
TPH2
TPH2 gene encodes for TPH2 (Tryptophan hydroxylase) which is a rate-limiting enzyme in the synthesis of serotonin from tryptophan. Tryptophan hydroxylase is found in the cytosol of the presynaptic membrane and is highly expressed in the frontal cortex. Park T. W. et al. (2013) reported rs11179027 and rs1843809 as risk alleles for this disorder. Sheehan et al. (2005) also identified that there was an association between ADHD and the T allele of marker rs1843809; however, this finding was not replicated in another study (Sheehan et al., 2007). In addition, TPH2-rs4570625 and TPH2-rs11178997 were reported as risk alleles in a family study (Walitza et al., 2005), but another study performed by Shim et al. could not replicate the former (Shim et al., 2010). TPH2 rs11179027 and TPH2 rs1843809 alleles are linked with the genetic predisposition to ADHD in Egyptian children (Abo El Fotoh et al., 2020). The T allele (rs1843809), A allele (rs1386493), and G allele (rs1007023) have been associated with ADHD in the United Kingdom population (Brookes et al., 2006). TPH2 (rs17110747) is related to abnormal behaviors and impaired cognition in female ADHD children according to the family based association tests (Fageera et al., 2021). TPH2-deficient mice show anxiety-like behavior (Waider et al., 2011). The Tph2 null mutant mouse mimics the TPH2 G-703T phenotype in humans (Akhrif et al., 2021). High tyrosine levels can decrease TPH2 activity and tyrosinemia type 1 presents with ADHD symptoms as shown in 8 Norwegian children (Barone et al., 2020). TPH2 methylation has been correlated with ADHD in boys at early school age in a German longitudinal cohort study (Heinrich et al., 2017). As a result, there are some conflicting results regarding TPH2 alleles which makes it difficult to draw a conclusion. Despite these contradicting results, TPH2 has a role to play in ADHD but needs more studies.
5-hydroxytryptamine receptor 1A
HTR1A (5-hydroxytryptamine receptor 1A) encodes the 5-HT1A receptor that binds to the endogenous neurotransmitter serotonin. This receptor is a GPCR that is found in the postsynaptic membrane and is highly expressed in limbic areas, hypothalamus, and cortex. It plays a role in the regulation of dopamine and 5-HT levels in the brain. Activation of 5-HT1A receptors stimulates the opening of G-protein-coupled inwardly rectifying K+ (GIRK) channels in CA1 pyramidal cells of the hippocampus (Brown et al., 2005), followed by the outflow of K+ ions from the intracellular to the extracellular space which hyperpolarizes the neuron (Araragi and Lesch, 2013). The activation of the 5-HT1A receptor may increase dopamine release in the medial prefrontal cortex, striatum, and hippocampus, subsequently, inhibition of the release of glutamate and acetylcholine occurs in various areas of the brain (Lucki, 1998; Zuo et al., 2015). Shim et al. (2010) reported an association between allele frequencies of HTR1A C1019G and ADHD. The homozygous allele C frequency was significantly higher in ADHD patients than in controls (Shim et al., 2010). Park Y. H. et al. (2013) identified rs10042486, rs1423691, and rs878567 as risk alleles of HTR1A for ADHD but they have not been replicated.
HTR2A
The HTR2A gene encodes HTR2A (5-hydroxytryptamine receptor 2A) which is also a GPCR for serotonin. It is found in the postsynaptic and glial cell membranes, highly expressed in the neocortex, caudate nucleus, nucleus accumbens, and hippocampus. It facilitates the reuptake of serotonin from the synapse to the glial cell and postsynaptic membrane. HTR2A plays a role in the frontal cortex, where it mediates the activation of a subpopulation of GABAergic neurons (Serretti et al., 2007). Stimulation of HTR2A activates cAMP-response element-binding protein and/or brain-derived neurotrophic factor through calcium-dependent protein kinases in the cortex, leading to complex cell modifications depending on the second messenger cascade. Few studies with a small sample size have focused on HTR2A, whereby in one study, HTR2A 452Tyr allele was found to associate weakly with this disorder (Quist et al., 2000). Inattention subtype of ADHD was reported to have a link with SNP rs7984966 (Pinto et al., 2016). Levitan et al. (2002) found that the CC genotype of the T102C variant was associated with childhood ADHD in women who later developed seasonal affective disorder.
HTR1B
The HTR1B gene encodes HTR1B (5-hydroxytryptamine receptor 1B) which is also found in the presynaptic membrane and highly expressed in the striatum, basal ganglia, and hippocampus. It inhibits the activity of adenylate cyclase and controls the release of dopamine, serotonin, and acetylcholine in the brain. Two serotonergic genes: HTR1B and SLC6A4 were found to associate with ADHD according to the review (Faraone et al., 2005). Multiple SNPs that were associated with the inattentive subtype of ADHD including rs6296, rs6297, rs130060, rs6298, rs130058, and rs11568817 (Levitan et al., 2002) were once reported; however, they were not replicated in a subsequent study (Banerjee et al., 2012). Additional evidence showed that 5HT1B knockout mice exhibit behavioral disinhibition, hyperactivity, and increased aggression (Bouwknecht et al., 2001).
Solute carrier family 6 member 4
Solute carrier family 6 member 4 (SLC6A4) is encoded by the SLC6A4 gene. SLC6A4 is an integral membrane protein that transports the neurotransmitter serotonin from synaptic spaces into presynaptic neurons. Functional 5HTTLPR 44-bp insertion/deletion ‘long’ allele was identified as a risk allele for ADHD according to the meta-analysis (Gizer et al., 2009). The concurrence of the SLC6A4 rs6354 GG/GT and ADRA2A rs553668GG/GA genotypes is associated with a 6.15-fold increased risk of ADHD in comparison to cases carrying the combination of ADRA2A rs553668 AA and ANKK1 rs1800497 AA genotypes highlighting the role of the gene-gene interactions and polygenic effects in the occurrence of the ADHD as shown in a case-control study (Wang et al., 2021).
Genes related to adrenergic pathway
Norepinephrine is important for cognition, memory, regulation of stress, and uptake of lactate to the neurons. Excessive reuptake to the presynaptic membrane by transporters leads to depletion in synaptic junction hence the occurrence of ADHD clinical features. This can explain the role of norepinephrine reuptake inhibitors in patients with ADHD. Noradrenergic gene polymorphisms can affect the efficacy of methylphenidate and atomoxetine in children with ADHD according to the meta-analysis (Gul et al., 2021; Yuan et al., 2021). The reported genes include DBH, DRD4, NET1/SLC6A2, ADRA2A, and ADRA2C.
Dopamine beta-hydroxylase
Dopamine beta-hydroxylase (DBH) is responsible for the synthesis of norepinephrine through oxidative hydroxylation of dopamine and it is released into the circulation during synaptic transmissions from sympathetic neurons (Spencer et al., 2002). It is encoded by the DBH gene. Lower activities of DBH in the serum and urine of patients with ADHD have been reported (Pliszka et al., 1994), and decreased DBH levels correlated with the symptoms of this disorder in children (Rogeness et al., 1989). DBH KO mouse has decreased norepinephrine levels in the central neural system, which suggests the importance of this enzyme in the maintenance of normal norepinephrine functions (Cryan et al., 2001). DBH is highly associated with ADHD. Multiple DBH gene polymorphisms have been reported including rs1611115 (Kieling et al., 2008; Bhaduri et al., 2010), rs1108580 (Bhaduri et al., 2010) as well as rs6271 and rs2519152 (Tang et al., 2006). Moreover, paternal over-transmission for rs2519152 and a strong correlation between rs1611115, rs1108580, and rs2519152 and DBH enzyme activity have been identified (Bhaduri et al., 2010). The DBH rs1611115 is related to verbal aggression while DRD2 rs4274224 is associated with executive functions in ADHD among adult prisoners (Fernandez et al., 2022). Nevertheless, rs1611115 and rs1108580 had no significant association with childhood ADHD according to the recent meta-analysis (Gizer et al., 2009).
NET1/SLC6A2
NET1/SLC6A2 (neuroepithelial cell transforming gene 1) encodes for the norepinephrine transporter. It is found in the presynaptic membrane and is highly expressed in the brain cortex, responsible for the reuptake of norepinephrine and epinephrine into presynaptic nerve terminals, and is a regulator of norepinephrine homeostasis. This gene regulated intrinsic brain activity, visual memory, and attention in ADHD children (Shang et al., 2021). Biederman et al. (2008) found variants in NET1/SLC6A2 associated more with ADHD in females, whereas Anney et al. (2008) reported about paternal over the transmission of risk alleles to affected individuals.
Alpha-2C-adrenergic receptor
Alpha-2C-adrenergic receptor (ADRA2C) is encoded by the ADRA2C gene and it is found in the presynaptic membrane and highly expressed in heart and central noradrenergic neurons. It regulates the catecholamine-induced inhibition of adenylate cyclase through the action of G proteins. Cho et al. (2008) found that homozygous carriers of the C allele of the Dral polymorphism in ADRA2C have a trend toward increased response time variability while individuals homozygous for the G allele at the Mspl polymorphism has a trend toward decreased response time variability. Guan et al. (2009) reported a significant association of ADRA2C variants with combined subtype of ADHD, but the study done by Barr et al. (2001) revealed no association between the alleles of ADRA2C genes with ADHD. Larger sample size analyses are needed to verify this.
ADRA2A
According to the case-control study, neurocognitive functions are affected by the interaction between blood lead levels and alpha-2A-adrenergic receptor (ADRA2A) (Choi et al., 2020). ADRA2A rs553668GG/GA and SLC6A4 rs6354 GG/GT genotypes display a 6.15-fold increase in the risk of ADHD in comparison to the cases carrying the combination of ADRA2A rs553668 AA and ANKK1 rs1800497 AA genotypes emphasizing the importance of the gene-gene interactions in the occurrence of the ADHD (Wang et al., 2021). Gene-environment interactions have been observed between prenatal tobacco smoking exposure and ADRA2A rs553668 in relation to ADHD (Wang et al., 2019). Notably, exposure to tobacco smoke has been linked with ADHD and learning disabilities in Korean children (Cho et al., 2013).
Genes and copy number variations related to cholinergic pathway
The neurotransmitter acetylcholine (ACh) plays a critical role in brain circuits mediating motor control, learning, attention, and memory. Cholinergic dysfunction is associated with multiple brain disorders including ADHD (English et al., 2009). There are few studies that focused on genes impacting ACh signaling as determinants of ADHD risk. Nicotinic acetylcholine receptors (nAChRs) are expressed in regions densely innervated by dopaminergic neurons (Arroyo-Jim nez et al., 1999; Klink et al., 2001; Gotti et al., 2006). The activation of presynaptic nAChRs is known to facilitate dopamine release in the nucleus accumbens and in the striatum (Picciotto et al., 1998; Grady et al., 2002). Additionally, nAChRs signaling was shown to regulate the dopamine transporter gene transcription and function, potentially affecting dopamine uptake (Li et al., 2004; Parish et al., 2005). Developmental nicotine exposure augments nicotine preference, provokes hyperactivity and risk-taking behaviors, impairs nAChR expression and function, impairs DAT function, and leads to DNA hypomethylation in frontal cortex and striatum of both first and second-generation adolescent offspring of the developmental nicotine exposure mice (Buck et al., 2019).
Two cholinergic genes (CHRNA4 and CHNRA7) that encode α4 and α7 subunits of nicotinic acetylcholine receptors, respectively, have been reported (Kent et al., 2001a,b; Wallis et al., 2009; Mastronardi et al., 2016). CHRNA4 gene (cholinergic receptor nicotinic alpha 4 subunit) encodes for the nAChR α4 subunit, which is found in the postsynaptic membrane and highly expressed in the central nervous system. CHRNA4 is a ligand-gated ion channel that mediates fast signal transmission at synapses. Several genetic studies have focused on the CHRNA4 gene as a candidate gene for ADHD due to its involvement in the nicotinic acetylcholine system; however, they have conflicting results. Comings et al. found a dinucleotide repeat in intron 1 as a risk for ADHD, Todd et al. identified polymorphism in the exon 2–intron 2 junction as a risk for severe inattention problems, Brookes et al. found the association between the 5’ flanking regions of CHRNA4 with combined subtype of ADHD; nevertheless, Kent et al. and Bobb et al. found no significant evidence of association in their studies (Kent et al., 2001b; Todd et al., 2003; Bobb et al., 2005; Brookes et al., 2006; Lee et al., 2008; Wallis et al., 2009).
Cholinergic receptor nicotinic alpha 7 subunits (CHRNA7) is a gene coding for the a7 subunit of the neuronal acetylcholine receptor (CHRNA7). CHRNA7 is also ligand-gated ion channels that mediate fast signal transmission at synapses and is found in both presynaptic and glial cell membranes. It is more prominent in the hippocampus and GABAergic interneurons of stratum oriens, stratum radiatum, and on pyramidal neurons (Bates et al., 2014). It facilitates the transmission of GABA from the synapse to the postsynaptic membrane and glial cells. The study by Kent et al. (2001a) showed that there was no association between CHRNA7 microsatellite markers and ADHD. Deletion at 15q13 spanning KLF13 and CHRNA7 has been reported to relate to ADHD (Valbonesi et al., 2015). Although this CNV spans two genes, CHRNA7 is mostly likely the cause as CHRNA7 duplications at 15q13.3 has also been linked with ADHD (Gillentine et al., 2017). There are few association studies representing CHRNA4 and CHRNA7 genes as candidate genes for ADHD. Further analyses should be done to clarify the possible effects of the cholinergic neurotransmitter system on the pathogenesis of childhood ADHD.
Genes and copy number variations related to glutamatergic and GABAergic pathway
Glutamate is the major excitatory neurotransmitter in the brain and is involved in multiple functions relevant to ADHD: brain development, modulation of neuronal activity, regulation of dopamine signaling, synaptic plasticity, memory formation, and learning. GABA is an inhibitory neurotransmitter in the brain which is produced from the conversion of glutamate via glutamic acid decarboxylase (GAD) enzyme. Glutamate plays a role in ADHD through bidirectional regulation of dopamine signaling while GABA modulates dopamine metabolism. A study done based on proton magnetic resonance spectroscopy revealed higher levels of glutamate and low levels of GABA in children with ADHD than in controls (Courvoisie et al., 2004). This imbalance leads to symptoms of ADHD such as inattention, hyperactivity, and impulsivity hence showing the biological basis for ADHD. Reported genes include GAD1, glutamate receptor genes (GRM7, GRM5, GRM8, and GRM1). GAD1 (Glutamate decarboxylase 1) encodes a key enzyme of GABA biosynthesis (GAD67). GAD67 catalyzes the conversion of glutamic acid to gamma-aminobutyric acid (GABA) and is found in the presynaptic membrane and highly expressed in the frontal cortex. Bruxel et al. (2016) performed the first study that showed a positive association between the GAD1 gene and ADHD.
Glutamate receptor metabotropic (GRMs) are GPCRs involved in the modulation of excitatory synaptic transmission (Taniura et al., 2006). There are three receptor groups that are based on putative signal transduction mechanisms, sequence homology, and pharmacologic properties (Conn and Pin, 1997). GRM1 and GRM5 are members of group 1 and are expressed in the basal ganglia and cerebellum (Berthele et al., 1999). GRM1 knockout mice demonstrated the involvement of this receptor in associative learning due to reduced hippocampal long-term potentiation (Aiba et al., 1994a; Gil-Sanz et al., 2008) as well as in motor learning due to deficient cerebellar long-term depression (Aiba et al., 1994b). Impaired GRM5 receptor function results in inappropriate retention of aversive memories leading to anxiety disorders; therefore, it seems to be critical for inhibitory learning mechanisms (Xu et al., 2009). GRM7 and GRM8 are members of group 3 that inhibit the cyclic AMP cascade. GRM7 is an important presynaptic regulator of neurotransmission in the mammalian central nervous system. It has been linked to anxiety (Cryan et al., 2003) and is the most highly conserved GRM member across multiple species (Makoff et al., 1996). GRM4 rs1906953 and GRM7 rs9826579 are associated with ADHD in the Chinese population (Zhang et al., 2021b). GRM7 rs37952452 polymorphism was reported to play a role in the treatment response to methylphenidate in children with ADHD (Park S. et al., 2013), and it was replicated in another study (Park et al., 2014). GRID2 (Glutamate Ionotropic Receptor Delta Type Subunit 2) increases the risk of ADHD too (Zhang et al., 2021c). The GRM8 null mutant mice showed novelty-induced hyperactivity and altered fear responses (Gerlai et al., 2002; Fendt et al., 2010). Anxiety disorders, motor coordination problems, and learning disorders are common features found in ADHD cases (Rommelse et al., 2009).
Approximately 10% of the children with ADHD carried CNVs in the glutamate metabotropic genes (GRM5, GRM7, GRM8, and GRM1) or in genes known to interact with them (Elia et al., 2011). A high rate of copy number variations in children with ADHD compared to controls has been reported (Williams et al., 2010) and replicated (Yang et al., 2013). Most of the identified CNVs in children with ADHD carry glutamate metabotropic genes (Elia et al., 2011; Liu et al., 2021b).
Glutamatergic and GABAergic pathway and environmental interactions
Manganese is an important cofactor in the synthesis of anti-oxidative enzymes (Bresciani et al., 2015) and is an essential metal that plays a fundamental role in brain development and functioning. Hence, its deficiency can lead to oxidative stress. Although an excessive amount of manganese can also damage the nerves and disturb the glutamine-glutamate-GABAergic cycle which is vital for proper brain functioning, especially in the frontal cortex (Sidoryk-Wegrzynowicz, 2014). Manganese hair levels have been associated with ADHD in children (Collipp et al., 1983). Moreover, developmental exposure to manganese in laboratory animals led to hyperactivity (Banerjee et al., 2007).
Genes and copy number variations related to other pathways
TAR RNA Binding Protein 1 (TARBP1) DNA methylation is related to ADHD symptoms in adulthood and childhood (Weiß et al., 2021). ADGRL3-rs1565902 is linked with ADHD in the Caribbean population (Cervantes-Henriquez et al., 2021). Atrophilin-3 [LPHN3; or ADGRL (3)] is a synaptic adhesion G protein-coupled receptor that binds to fibronectin leucine rich transmembrane protein 3 and teneurin-3 (FLRT3 and TEN-3) (Regan et al., 2019). The rs2122642-ADGRL3 (C allele) and ADGRL3 haplotype CCC (markers rs1565902-rs10001410-rs2122642) are associated with ADHD in the Caribbean community (Puentes-Rozo et al., 2019). Mice null for Lphn3 exhibit high levels of dopamine and serotonin in the dorsal striatum and present with a hyperactive phenotype suggesting its role in the modulation of monoamine signaling (Wallis et al., 2012). A null mutation of Lphn3 (KO) in Sprague-Dawley rats was related to hyperactivity and striatal changes in dopamine markers (Regan et al., 2019). Fibroblast Growth Factor 1 (FGF1) -rs2282794 has been reported to relate to ADHD among the Caribbean population too (Cervantes-Henriquez et al., 2021). Monoamine Oxidase A (MAO) is encoded by the MAOA gene and is highly expressed in the frontal cortex, responsible for the oxidation of neurotransmitters and dietary amines including serotonin, norepinephrine, and dopamine. Das et al. (2006) identified that the short 3.5 repeat allele of the MAOA-u VNTR, is associated with ADHD in children. Moreover, the 6-repeat allele of the CA microsatellite and the G-allele of the 941G/T SNP of MAOA have been reported (Domschke et al., 2005). Brain-derived neurotrophic factor (BDNF) is important for the development of GABAergic neurons (Yamada et al., 2002; Hong et al., 2008). Patients with ADHD have been reported to have BDNF mutations (Kwon et al., 2015). Syntaxin1, SNAP25, and VAMP2 form a SNARE complex that is responsible for the regulation of neurotransmitter release (Rizo and Südhof, 2012). SNAP25 and STX1A polymorphisms have been reported to associate with ADHD (Gao et al., 2015).
Other acknowledged CNVs include deletions at 2p16.3 spanning NRXN1, 15q11.2, 15q13.3 spanning BP4 and BP4.5-BP5 and 22q11.21, and duplications at 1q21, 16p11.2, 16p13.11, and 22q11.21 (Gudmundsson et al., 2019). Deletions and duplications at the 15q13 and 16p11.2 and deletion and duplication of PARK2 locus at 6q25.2-q27 (Jarick et al., 2014). Additionally, 16p13.11 and 15q13.3, 3p26 deletion or duplication spanning the CNTN6 gene have been reported (Williams et al., 2010, 2012; Jarick et al., 2014; Hu et al., 2015; Zarrei et al., 2019). De novo aberrations at four CNVs: 15q13.1-13.2 duplication, 16p13.11 duplication, 16p12.2 deletion, and 22q11.21 duplication have been implicated in ADHD studies too (Martin et al., 2020).
Other microelement dysfunction related to unknown pathways
Nutritional deficiencies have been increasingly implicated as possible risk factors for ADHD (Sinn and Bryan, 2007; Verlaet et al., 2014). Low levels of copper, iron, zinc, magnesium, and omega-3 fatty acids have been detected in children with this disorder while sugar, artificial food colorants, and preservatives have been associated with an increased risk of ADHD (Holtkamp et al., 2004; Cormier and Elder, 2007; Mahmoud et al., 2011; San Mauro Martín et al., 2018). Iron, zinc, and magnesium supplements act as novel therapies for certain aspects of ADHD (Konofal et al., 2008).
Hair mercury and manganese are considered to be the other developmental toxicants related to the development of ADHD symptomatology (Banerjee et al., 2007; Boucher et al., 2012). Mercury is a potent neurodevelopmental toxicant commonly encountered in the environment in dietary or non-dietary form. Small prenatal exposures resulting from maternal consumption of contaminated fish adversely affected intelligence, language development, gross motor skills, visual-spatial skills, memory, and attention in offspring (Anderson et al., 1981; Collipp et al., 1983). Duration and dosage of mercury exposure determine the incidence and prevalence of ADHD according to one meta-analysis (Yoshimasu et al., 2014).
Lead is known as an environmental toxin that negatively affects brain development (Foltinová et al., 2007). Low levels of lead exposure can result to inattention, low intelligence, and behavioral problems (Lanphear et al., 2005; Nevin et al., 2008). High serum lead levels have an association with ADHD (Boucher et al., 2012). A recent meta-analysis of children and adolescents demonstrated a significant association between lead exposure and symptoms of both inattention and hyperactivity/impulsivity (Goodlad et al., 2013).
Treatments
Pharmacological treatments
The treatment options include pharmacological and non-pharmacological. Pharmacological treatments are helpful as they restore the balance of the neurotransmitters in the prefrontal cortex (Seixas et al., 2012; Burcu et al., 2016). Commonly used drugs include stimulants such as methylphenidate and amphetamines as well as non-stimulants such as atomoxetine and α-2 agonists (guanfacine and clonidine) (Reddy, 2013; Sharma and Couture, 2014; Tarver et al., 2014; Catalá-López et al., 2015). These drugs have many side effects ranging from mild to severe ones (Tobaiqy et al., 2011; Clavenna and Bonati, 2017). Despite the availability of pharmacotherapy, the outcomes differ according to the individuals; about 80% of the patients respond well to the psychostimulant drugs while approximately 20% have a poor response (Kendall et al., 2008; Wolraich et al., 2019). Therefore, there is a need to explore and develop more efficacious drugs with less side effects.
Dietary treatments
Low levels of copper, iron, zinc, magnesium, and omega-3 fatty acids have been detected in children with this disorder while sugar, artificial food colorants, and preservatives have been associated with an increased risk of ADHD (Holtkamp et al., 2004; Konofal et al., 2004; Cormier and Elder, 2007; Lahat et al., 2011; San Mauro Martín et al., 2018). Iron, zinc, and magnesium supplements act as novel therapies for certain aspects of ADHD (Konofal et al., 2008). Low levels of omega-3 fatty acids and the utilization of sugar, artificial food colorants, and preservatives have been reported to associate with an increased risk of ADHD (Millichap and Yee, 2012). Several dietary treatments have been suggested including sugar-restricted, additive, and salicylate-free (Feingold diet), oligoantigenic, ketogenic, megavitamin, and polyunsaturated fatty acid supplements (PUFA) (Howard et al., 2011; Millichap and Yee, 2012). Nevertheless, the role of diet and dietary supplements in the cause and treatment of ADHD in children is still controversial (Millichap and Yee, 2012).
Conclusion
By using a systematic search that combines results from different studies, we provide evidence that the etiology of ADHD in children is multifactorial. Genetic and environmental/social factors have been reported to play a role by altering neurotransmission. SNPs, copy number variations, and microRNAs have been linked with ADHD. The imbalance of the neurotransmitters leads to ADHD. DRD1, DRD2, DRD4, DAT1, DBH, NET1/SLC6A2, ADRA2C, ADRA2A, CHRNA4, CHRNA7, and MAO is involved in dopaminergic pathways directly or indirectly. TPH2, HTR1A, HTR1B, and HTR2A are involved in the serotonergic pathway. SNAP-25 and BDNF are involved in neurotransmission and neuronal plasticity. GAD1 is involved in the GABAergic pathway. SNPs that fall under the dopaminergic and serotonergic pathways appear to contribute more to the possible etiology. Dysregulation/exposure to environmental factors including nicotine, ethanol, copper, iron, zinc, magnesium, lead, and manganese can also interfere with neurotransmission. The reported SNPs differ according to ethnic groups. MicroRNAs are responsible for post-transcription modulation of the genes, and studies have shown their possible roles in the regulation of some of the ADHD-related genes. For instance, miR22-3p can regulate BDNF, HTR2C, and MAO, miR-138-1 and miR-296 can regulate BDNF, miR-96 can regulate HTR1B, miR-30b-5p, miR-6070, miR-1301, and miR-384-5p can regulate DAT1 (Supplementary Table 2). Although there are some animal model studies, most of them are KO and do not generate the genetic alteration of the patients. The majority of the available animal models are those related to the dopaminergic pathway. Epigenetic changes such as SUMOylation, methylation, and acetylation have been reported in genes related to the dopaminergic pathway.
Consequently, the dopaminergic pathway remains to be crucial in the pathogenesis of ADHD. It can be affected by environmental factors and other proposed pathways including adrenergic, GABAergic, glutamatergic, serotonergic, and cholinergic, directly or indirectly. At least one of the five dopaminergic receptors is located in adrenergic, GABAergic, glutamatergic, and cholinergic neurons: DRD1, DRD2, dopamine D3 receptor (DRD3), DRD4, and dopamine D5 receptor (DRD5) (Robertson and Staines, 1994; Hersch et al., 1995; Centonze et al., 2002, 2003; Rivera et al., 2002, 2003; Berlanga et al., 2005; Mizuno et al., 2007; Xi and Gardner, 2007). Animal models of ADHD showed dysregulation of dopamine functions and abnormal behaviors of which were normalized upon administration of stimulant medications (Russell, 2011). Some studies based on children with ADHD identified frontal cortical regions, which are rich in dopamine, as sites related to ADHD (McAlonan et al., 2007, 2009). Additionally, several studies have shown that the dopamine receptor density in several brain regions of ADHD patients is lower compared to healthy individuals (Jönsson et al., 1999; Medin et al., 2013). Methylphenidate is the first-line stimulant drug for ADHD. It improves the symptoms of ADHD in about 70% of the patients (Hodgkins et al., 2012; Bolea-Alamañac et al., 2014). It acts through several pathways including dopaminergic, noradrenergic, serotonergic, glutamatergic, and GABAergic to increase the levels of dopamine in synaptic cleft as it was reported by recent studies on human and animal models (Millan et al., 2000; Oades, 2008; Wilens, 2008; Freese et al., 2012; Park et al., 2014). Preclinical studies, as well as the dissection of the mechanism of action of available stimulant drugs, support the view that dopaminergic, serotonergic, glutamatergic and GABAergic systems are functionally interconnected. These observations open the field for the exploration of the physical interconnections between receptors and neurotransmitters. Table 1 summarizes different neurotransmitters and neuroreceptors and the roles they play in the pathophysiology and pathogenesis of ADHD.
The limitations and strengths of this review
This is a comprehensive review covering genetics, neurotransmitters as well as neuroanatomical structures involved in ADHD. Nevertheless, ADHD being a heterogeneous illness with a varying number of comorbidities, it is still unclear how environmental factors relate to all neurotransmitter pathways. Future studies should explore more the associations between environmental factors and neurotransmitter pathways. Although several genes have been related to ADHD, there are few animal model studies on the majority of the genes. More epigenetic studies are required for other genes on top of those related to the dopaminergic pathway.
Author contributions
MK, HD, and JX performed a literature review, drafted the article, and gave final approval of the version to be published. BC and YM assisted in the preparation of the table, revised the manuscript, and gave final approval of the version to be published. LY, FH, OB, and JP revised the manuscript critically for important intellectual content and gave final approval of the version to be published. FY conceptualized, designed, and revised the manuscript critically for important intellectual content and gave final approval of the version to be published. All authors contributed to the article and approved the submitted version.
Funding
This work was supported by the National Natural Science Foundation of China (Grant no. 81771408), the Hunan Key Research and Development Program (Grant no. 2019SK208), and the open project of Key Laboratory of Children’s Genetic and Metabolic Diseases in Henan Province (SS201905).
Conflict of interest
The authors declare that the research was conducted in the absence of any commercial or financial relationships that could be construed as a potential conflict of interest.
Publisher’s note
All claims expressed in this article are solely those of the authors and do not necessarily represent those of their affiliated organizations, or those of the publisher, the editors and the reviewers. Any product that may be evaluated in this article, or claim that may be made by its manufacturer, is not guaranteed or endorsed by the publisher.
Supplementary material
The Supplementary Material for this article can be found online at: https://www.frontiersin.org/articles/10.3389/fnmol.2022.925049/full#supplementary-material
References
Abdulla, Z. I., Pahlevani, B., Lundgren, K. H., Pennington, J. L., Udobi, K. C., Seroogy, K. B., et al. (2020). Deletion of the creatine transporter (Slc6a8) in dopaminergic neurons leads to hyperactivity in mice. J. Mol. Neurosci. 70, 102–111. doi: 10.1007/s12031-019-01405-w
Abo El Fotoh, W. M. M., Bayomy, N. R., Kasemy, Z. A., Barain, A. M., Shalaby, B. M., and Abd El Naby, S. A. (2020). Genetic variants and haplotypes of tryptophan hydroxylase 2 and reelin genes may be linked with attention deficit hyperactivity disorder in egyptian children. ACS Chem. Neurosci. 11, 2094–2103. doi: 10.1021/acschemneuro.0c00136
Adisetiyo, V., Jensen, J. H., Tabesh, A., Deardorff, R. L., Fieremans, E., Di Martino, A., et al. (2014). Multimodal MR imaging of brain iron in attention deficit hyperactivity disorder: a noninvasive biomarker that responds to psychostimulant treatment? Radiology 272, 524–532. doi: 10.1148/radiol.14140047
Agudelo, J. A., Gálvez, J. M., Fonseca, D. J., Mateus, H. E., Talero-Gutiérrez, C., and Velez-Van-Meerbeke, A. (2015). Evidence of an association between 10/10 genotype of DAT1 and endophenotypes of attention deficit/hyperactivity disorder. Neurologia 30, 137–143. doi: 10.1016/j.nrl.2013.12.005
Aiba, A., Chen, C., Herrup, K., Rosenmund, C., Stevens, C. F., and Tonegawa, S. (1994a). Reduced hippocampal long-term potentiation and context-specific deficit in associative learning in mGluR1 mutant mice. Cell 79, 365–375. doi: 10.1016/0092-8674(94)90204-6
Aiba, A., Kano, M., Chen, C., Stanton, M. E., Fox, G. D., Herrup, K., et al. (1994b). Deficient cerebellar long-term depression and impaired motor learning in mGluR1 mutant mice. Cell 79, 377–388.
Akhondzadeh, S., Mohammadi, M.-R., and Khademi, M. (2004). Zinc sulfate as an adjunct to methylphenidate for the treatment of attention deficit hyperactivity disorder in children: a double blind and randomized trial [ISRCTN64132371]. BMC Psychiatry 4:9. doi: 10.1186/1471-244X-4-9
Akhrif, A., Roy, A., Peters, K., Lesch, K.-P., Romanos, M., Schmitt-Böhrer, A., et al. (2021). REVERSE phenotyping-Can the phenotype following constitutive Tph2 gene inactivation in mice be transferred to children and adolescents with and without adhd? Brain Behav. 11:e02054. doi: 10.1002/brb3.2054
Akutagava-Martins, G. C., Salatino-Oliveira, A., Kieling, C. C., Rohde, L. A., and Hutz, M. H. (2013). Genetics of attention-deficit/hyperactivity disorder: current findings and future directions. Expert Rev. Neurother. 13, 435–445. doi: 10.1586/ern.13.30
Alamo, C., López-Muñoz, F., and Sánchez-García, J. (2016). Mechanism of action of guanfacine: a postsynaptic differential approach to the treatment of attention deficit hyperactivity disorder (adhd). Actas Esp. Psiquiatr. 44, 107–112.
Albuquerque, E. X., Pereira, E. F. R., Alkondon, M., and Rogers, S. W. (2009). Mammalian nicotinic acetylcholine receptors: from structure to function. Physiol. Rev. 89, 73–120. doi: 10.1152/physrev.00015.2008
Anderson, R. J., Hart, G. R., Crumpler, C. P., and Lerman, M. J. (1981). Clonidine overdose: report of six cases and review of the literature. Ann. Emerg. Med. 10, 107–112. doi: 10.1016/s0196-0644(81)80350-2
Anney, R. J. L., Hawi, Z., Sheehan, K., Mulligan, A., Pinto, C., Brookes, K. J., et al. (2008). Parent of origin effects in attention/deficit hyperactivity disorder (ADHD): analysis of data from the international multicenter ADHD genetics (IMAGE) program. Am. J. Med. Genet. B Neuropsychiatr. Genet. Off. Publ. Int. Soc. Psychiatr. Genet. 147B, 1495–1500. doi: 10.1002/ajmg.b.30659
Aoki, S., Kaizaki-Mitsumoto, A., Hattori, N., and Numazawa, S. (2021). Fetal methylphenidate exposure induced ADHD-like phenotypes and decreased Drd2 and Slc6a3 expression levels in mouse offspring. Toxicol. Lett. 344, 1–10. doi: 10.1016/j.toxlet.2021.02.016
Araragi, N., and Lesch, K.-P. (2013). Serotonin (5-HT) in the regulation of depression-related emotionality: insight from 5-HT transporter and tryptophan hydroxylase-2 knockout mouse models. Curr. Drug Targets 14, 549–570. doi: 10.2174/1389450111314050005
Arnold, L. E., Disilvestro, R. A., Bozzolo, D., Bozzolo, H., Crowl, L., Fernandez, S., et al. (2011). Zinc for attention-deficit/hyperactivity disorder: placebo-controlled double-blind pilot trial alone and combined with amphetamine. J. Child Adolesc. Psychopharmacol. 21, 1–19. doi: 10.1089/cap.2010.0073
Arnsten, A. F. T., and Pliszka, S. R. (2011). Catecholamine influences on prefrontal cortical function: relevance to treatment of attention deficit/hyperactivity disorder and related disorders. Pharmacol. Biochem. Behav. 99, 211–216. doi: 10.1016/j.pbb.2011.01.020
Arroyo-Jim nez, M. M., Bourgeois, J. P., Marubio, L. M., Le Sourd, A. M., Ottersen, O. P., Rinvik, E., et al. (1999). Ultrastructural localization of the alpha4-subunit of the neuronal acetylcholine nicotinic receptor in the rat substantia nigra. J. Neurosci. 19, 6475–6487. doi: 10.1523/JNEUROSCI.19-15-06475.1999
Avale, M. E., Falzone, T. L., Gelman, D. M., Low, M. J., Grandy, D. K., and Rubinstein, M. (2004). The dopamine D4 receptor is essential for hyperactivity and impaired behavioral inhibition in a mouse model of attention deficit/hyperactivity disorder. Mol. Psychiatry 9, 718–726. doi: 10.1038/sj.mp.4001474
Aydin, S. U., Kabukcu Basay, B., Cetin, G. O., Gungor Aydin, A., and Tepeli, E. (2019). Altered microRNA 5692b and microRNA let-7d expression levels in children and adolescents with attention deficit hyperactivity disorder. J. Psychiatr. Res. 115, 158–164. doi: 10.1016/j.jpsychires.2019.05.021
Banaschewski, T., Becker, K., Scherag, S., Franke, B., and Coghill, D. (2010). Molecular genetics of attention-deficit/hyperactivity disorder: an overview. Eur. Child Adolesc. Psychiatry 19, 237–257. doi: 10.1007/s00787-010-0090-z
Banerjee, E., Banerjee, D., Chatterjee, A., Sinha, S., and Nandagopal, K. (2012). Selective maternal inheritance of risk alleles and genetic interaction between serotonin receptor-1B (5-HTR1B) and serotonin transporter (SLC6A4) in ADHD. Psychiatry Res. 200, 1083–1085. doi: 10.1016/j.psychres.2012.04.003
Banerjee, T. D., Middleton, F., and Faraone, S. V. (2007). Environmental risk factors for attention-deficit hyperactivity disorder. Acta Paediatr. 96, 1269–1274. doi: 10.1111/j.1651-2227.2007.00430.x
Barkley, R. A., Smith, K. M., and Fischer, M. (2019). ADHD risk genes involved in dopamine signaling and metabolism are associated with reduced estimated life expectancy at young adult follow-up in hyperactive and control children. Am. J. Med. Genet. B Neuropsychiatr. Genet. Off. Publ. Int. Soc. Psychiatr. Genet. 180, 175–185. doi: 10.1002/ajmg.b.32711
Barone, H., Bliksrud, Y. T., Elgen, I. B., Szigetvari, P. D., Kleppe, R., Ghorbani, S., et al. (2020). Tyrosinemia Type 1 and symptoms of ADHD: biochemical mechanisms and implications for treatment and prognosis. Am. J. Med. Genet. B Neuropsychiatr. Genet. Off. Publ. Int. Soc. Psychiatr. Genet. 183, 95–105. doi: 10.1002/ajmg.b.32764
Barr, A. M., Lehmann-Masten, V., Paulus, M., Gainetdinov, R. R., Caron, M. G., and Geyer, M. A. (2004). The selective serotonin-2A receptor antagonist M100907 reverses behavioral deficits in dopamine transporter knockout mice. Neuropsychopharmacol. Off. Publ. Am. Coll. Neuropsychopharmacol. 29, 221–228. doi: 10.1038/sj.npp.1300343
Barr, C. L., Wigg, K., Zai, G., Roberts, W., Malone, M., Schachar, R., et al. (2001). Attention-deficit hyperactivity disorder and the adrenergic receptors alpha 1C and alpha 2C. Mol. Psychiatry 6, 334–337. doi: 10.1038/sj.mp.4000863
Bates, R. C., Stith, B. J., Stevens, K. E., and Adams, C. E. (2014). Reduced CHRNA7 expression in C3H mice is associated with increases in hippocampal parvalbumin and glutamate decarboxylase-67 (GAD67) as well as altered levels of GABA(A) receptor subunits. Neuroscience 273, 52–64. doi: 10.1016/j.neuroscience.2014.05.004
Bengel, D., Jöhren, O., Andrews, A. M., Heils, A., Mössner, R., Sanvitto, G. L., et al. (1997). Cellular localization and expression of the serotonin transporter in mouse brain. Brain Res. 778, 338–345. doi: 10.1016/s0006-8993(97)01080-9
Berger, I. (2011). Diagnosis of attention deficit hyperactivity disorder: much ado about something. Isr. Med. Assoc. J. 13, 571–574.
Berlanga, M. L., Simpson, T. K., and Alcantara, A. A. (2005). Dopamine D5 receptor localization on cholinergic neurons of the rat forebrain and diencephalon: a potential neuroanatomical substrate involved in mediating dopaminergic influences on acetylcholine release. J. Comp. Neurol. 492, 34–49. doi: 10.1002/cne.20684
Berthele, A., Platzer, S., Laurie, D. J., Weis, S., Sommer, B., Zieglgänsberger, W., et al. (1999). Expression of metabotropic glutamate receptor subtype mRNA (mGluR1-8) in human cerebellum. Neuroreport 10, 3861–3867. doi: 10.1097/00001756-199912160-00026
Bettger, W. J., Reeves, P. G., Moscatelli, E. A., Reynolds, G., and O’Dell, B. L. (1979). Interaction of Zinc and essential fatty acids in the rat. J. Nutr. 109, 480–488. doi: 10.1093/jn/109.3.480
Bhaduri, N., Sarkar, K., Sinha, S., Chattopadhyay, A., and Mukhopadhyay, K. (2010). Study on DBH genetic polymorphisms and plasma activity in attention deficit hyperactivity disorder patients from Eastern India. Cell. Mol. Neurobiol. 30, 265–274. doi: 10.1007/s10571-009-9448-5
Bidwell, L. C., Willcutt, E. G., McQueen, M. B., DeFries, J. C., Olson, R. K., Smith, S. D., et al. (2011). A family based association study of DRD4, DAT1, and 5HTT and continuous traits of attention-deficit hyperactivity disorder. Behav. Genet. 41, 165–174. doi: 10.1007/s10519-010-9437-y
Biederman, J. (2005). Attention-deficit/hyperactivity disorder: a selective overview. Biol. Psychiatry 57, 1215–1220. doi: 10.1016/j.biopsych.2004.10.020
Biederman, J., Faraone, S. V., Keenan, K., Benjamin, J., Krifcher, B., Moore, C., et al. (1992). Further evidence for family-genetic risk factors in attention deficit hyperactivity disorder. Patterns of comorbidity in probands and relatives psychiatrically and pediatrically referred samples. Arch. Gen. Psychiatry 49, 728–738. doi: 10.1001/archpsyc.1992.01820090056010
Biederman, J., Keenan, K., and Faraone, S. V. (1990). Parent-based diagnosis of attention deficit disorder predicts a diagnosis based on teacher report. J. Am. Acad. Child Adolesc. Psychiatry 29, 698–701. doi: 10.1097/00004583-199009000-00004
Biederman, J., Kim, J. W., Doyle, A. E., Mick, E., Fagerness, J., Smoller, J. W., et al. (2008). Sexually dimorphic effects of four genes (COMT, SLC6A2, MAOA, SLC6A4) in genetic associations of ADHD: a preliminary study. Am. J. Med. Genet. B Neuropsychiatr. Genet. Off. Publ. Int. Soc. Psychiatr. Genet. 147B, 1511–1518. doi: 10.1002/ajmg.b.30874
Bloomquist, J. R., Barlow, R. L., Gillette, J. S., Li, W., and Kirby, M. L. (2002). Selective effects of insecticides on nigrostriatal dopaminergic nerve pathways. Neurotoxicology 23, 537–544. doi: 10.1016/s0161-813x(02)00031-1
Bobb, A. J., Addington, A. M., Sidransky, E., Gornick, M. C., Lerch, J. P., Greenstein, D. K., et al. (2005). Support for association between ADHD and two candidate genes: NET1 and DRD1. Am. J. Med. Genet. B Neuropsychiatr. Genet. Off. Publ. Int. Soc. Psychiatr. Genet. 134B, 67–72. doi: 10.1002/ajmg.b.30142
Bolat, H., Ercan, E. S., Ünsel-Bolat, G., Tahillioðlu, A., Yazici, K. U., Bacanli, A., et al. (2020). DRD4 genotyping may differentiate symptoms of attention-deficit/hyperactivity disorder and sluggish cognitive tempo. Rev. Bras. Psiquiatr. 42, 630–637. doi: 10.1590/1516-4446-2019-0630
Bolea-Alamañac, B., Nutt, D. J., Adamou, M., Asherson, P., Bazire, S., Coghill, D., et al. (2014). Evidence-based guidelines for the pharmacological management of attention deficit hyperactivity disorder: update on recommendations from the British Association for Psychopharmacology. J. Psychopharmacol. 28, 179–203. doi: 10.1177/0269881113519509
Bonaventura, J., Quiroz, C., Cai, N.-S., Rubinstein, M., Tanda, G., and Ferré, S. (2017). Key role of the dopamine D(4) receptor in the modulation of corticostriatal glutamatergic neurotransmission. Sci. Adv. 3:e1601631. doi: 10.1126/sciadv.1601631
Bonvicini, C., Cortese, S., Maj, C., Baune, B. T., Faraone, S. V., and Scassellati, C. (2020). DRD4 48?bp multiallelic variants as age-population-specific biomarkers in attention-deficit/hyperactivity disorder. Transl. Psychiatry 10:70. doi: 10.1038/s41398-020-0755-4
Bonvicini, C., Faraone, S. V., and Scassellati, C. (2016). Attention-deficit hyperactivity disorder in adults: a systematic review and meta-analysis of genetic, pharmacogenetic and biochemical studies. Mol. Psychiatry 21, 872–884. doi: 10.1038/mp.2016.74
Bonvicini, C., Faraone, S. V., and Scassellati, C. (2018). Common and specific genes and peripheral biomarkers in children and adults with attention-deficit/hyperactivity disorder. World J. Biol. Psychiatry Off. J. World Fed. Soc. Biol. Psychiatry 19, 80–100. doi: 10.1080/15622975.2017.1282175
Borodovitsyna, O., Flamini, M., and Chandler, D. (2017). Noradrenergic modulation of cognition in health and disease. Neural Plast. 2017:6031478. doi: 10.1155/2017/6031478
Boucher, O., Jacobson, S. W., Plusquellec, P., Dewailly, E., Ayotte, P., Forget-Dubois, N., et al. (2012). Prenatal methylmercury, postnatal lead exposure, and evidence of attention deficit/hyperactivity disorder among Inuit children in Arctic Québec. Environ. Health Perspect. 120, 1456–1461. doi: 10.1289/ehp.1204976
Bouwknecht, J. A., Hijzen, T. H., van der Gugten, J., Maes, R. A., Hen, R., and Olivier, B. (2001). Absence of 5-HT(1B) receptors is associated with impaired impulse control in male 5-HT(1B) knockout mice. Biol. Psychiatry 49, 557–568. doi: 10.1016/s0006-3223(00)01018-0
Bresciani, G., da Cruz, I. B. M., and González-Gallego, J. (2015). Manganese superoxide dismutase and oxidative stress modulation. Adv. Clin. Chem. 68, 87–130. doi: 10.1016/bs.acc.2014.11.001
Brookes, K., Xu, X., Chen, W., Zhou, K., Neale, B., Lowe, N., et al. (2006). The analysis of 51 genes in DSM-IV combined type attention deficit hyperactivity disorder: association signals in DRD4, DAT1 and 16 other genes. Mol. Psychiatry 11, 934–953. doi: 10.1038/sj.mp.4001869
Brown, A. B., Biederman, J., Valera, E., Makris, N., Doyle, A., Whitfield-Gabrieli, S., et al. (2011). Relationship of DAT1 and adult ADHD to task-positive and task-negative working memory networks. Psychiatry Res. 193, 7–16. doi: 10.1016/j.pscychresns.2011.01.006
Brown, S. M., Peet, E., Manuck, S. B., Williamson, D. E., Dahl, R. E., Ferrell, R. E., et al. (2005). A regulatory variant of the human tryptophan hydroxylase-2 gene biases amygdala reactivity. Mol. Psychiatry 10, 884–888. doi: 10.1038/sj.mp.4001716
Bruxel, E. M., Akutagava-Martins, G. C., Salatino-Oliveira, A., Genro, J. P., Zeni, C. P., Polanczyk, G. V., et al. (2016). GAD1 gene polymorphisms are associated with hyperactivity in attention-deficit/hyperactivity disorder. Am. J. Med. Genet. B Neuropsychiatr. Genet. Off. Publ. Int. Soc. Psychiatr. Genet. 171, 1099–1104. doi: 10.1002/ajmg.b.32489
Buck, J. M., Sanders, K. N., Wageman, C. R., Knopik, V. S., Stitzel, J. A., and O’Neill, H. C. (2019). Developmental nicotine exposure precipitates multigenerational maternal transmission of nicotine preference and ADHD-like behavioral, rhythmometric, neuropharmacological, and epigenetic anomalies in adolescent mice. Neuropharmacology 149, 66–82. doi: 10.1016/j.neuropharm.2019.02.006
Burcu, M., Zito, J. M., Metcalfe, L., Underwood, H., and Safer, D. J. (2016). Trends in stimulant medication use in commercially insured youths and adults, 2010-2014. JAMA Psychiatry 73, 992–993. doi: 10.1001/jamapsychiatry.2016.1182
Burhans, M. S., Dailey, C., Beard, Z., Wiesinger, J., Murray-Kolb, L., Jones, B. C., et al. (2005). Iron deficiency: differential effects on monoamine transporters. Nutr. Neurosci. 8, 31–38. doi: 10.1080/10284150500047070
Canseco-Alba, A., Sanabria, B., Hammouda, M., Bernadin, R., Mina, M., Liu, Q.-R., et al. (2021). Cell-type specific deletion of CB2 cannabinoid receptors in dopamine neurons induced hyperactivity phenotype: possible relevance to attention-deficit hyperactivity disorder. Front. Psychiatry 12:803394. doi: 10.3389/fpsyt.2021.803394
Cartier, E., Garcia-Olivares, J., Janezic, E., Viana, J., Moore, M., Lin, M. L., et al. (2019). The SUMO-Conjugase Ubc9 prevents the degradation of the dopamine transporter, enhancing its cell surface level and dopamine uptake. Front. Cell. Neurosci. 13:35. doi: 10.3389/fncel.2019.00035
Casadó-Anguera, V., Moreno, E., Sánchez-Soto, M., Cai, N. S., Bonaventura, J., Homar-Ruano, P., et al. (2021). Heteromerization between α(2A) adrenoceptors and different polymorphic variants of the dopamine D(4) receptor determines pharmacological and functional differences. Implications for impulsive-control disorders. Pharmacol. Res. 170:105745. doi: 10.1016/j.phrs.2021.105745
Catalá-López, F., Hutton, B., Núñez-Beltrán, A., Mayhew, A. D., Page, M. J., Ridao, M., et al. (2015). The pharmacological and non-pharmacological treatment of attention deficit hyperactivity disorder in children and adolescents: protocol for a systematic review and network meta-analysis of randomized controlled trials. Syst. Rev. 4:19. doi: 10.1186/s13643-015-0005-7
Centonze, D., Bracci, E., Pisani, A., Gubellini, P., Bernardi, G., and Calabresi, P. (2002). Activation of dopamine D1-like receptors excites LTS interneurons of the striatum. Eur. J. Neurosci. 15, 2049–2052. doi: 10.1046/j.1460-9568.2002.02052.x
Centonze, D., Grande, C., Usiello, A., Gubellini, P., Erbs, E., Martin, A. B., et al. (2003). Receptor subtypes involved in the presynaptic and postsynaptic actions of dopamine on striatal interneurons. J. Neurosci. 23, 6245–6254. doi: 10.1523/JNEUROSCI.23-15-06245.2003
Cervantes-Henriquez, M. L., Acosta-López, J. E., Ahmad, M., Sánchez-Rojas, M., Jiménez-Figueroa, G., Pineda-Alhucema, W., et al. (2021). ADGRL3, FGF1 and DRD4: linkage and association with working memory and perceptual organization candidate endophenotypes in ADHD. Brain Sci. 11:854. doi: 10.3390/brainsci11070854
Cervantes-Henríquez, M. L., Acosta-López, J. E., Martinez, A. F., Arcos-Burgos, M., Puentes-Rozo, P. J., and Vélez, J. I. (2022). Machine learning prediction of ADHD severity: association and linkage to ADGRL3, DRD4, and SNAP25. J. Atten. Disord. 26, 587–605. doi: 10.1177/10870547211015426
Chang, C.-H., Yu, C.-J., Du, J.-C., Chiou, H.-C., Chen, H.-C., Yang, W., et al. (2018). The interactions among organophosphate pesticide exposure, oxidative stress, and genetic polymorphisms of dopamine receptor D4 increase the risk of attention deficit/hyperactivity disorder in children. Environ. Res. 160, 339–346. doi: 10.1016/j.envres.2017.10.011
Chang, F. M., Kidd, J. R., Livak, K. J., Pakstis, A. J., and Kidd, K. K. (1996). The world-wide distribution of allele frequencies at the human dopamine D4 receptor locus. Hum. Genet. 98, 91–101. doi: 10.1007/s004390050166
Chen, C.-K., Chen, S.-L., Mill, J., Huang, Y.-S., Lin, S.-K., Curran, S., et al. (2003). The dopamine transporter gene is associated with attention deficit hyperactivity disorder in a Taiwanese sample. Mol. Psychiatry 8, 393–396. doi: 10.1038/sj.mp.4001238
Chen, M.-H., Su, T.-P., Chen, Y.-S., Hsu, J.-W., Huang, K.-L., Chang, W.-H., et al. (2013). Association between psychiatric disorders and iron deficiency anemia among children and adolescents: a nationwide population-based study. BMC Psychiatry 13:161. doi: 10.1186/1471-244X-13-161
Chen, X., Xing, J., Jiang, L., Qian, W., Wang, Y., Sun, H., et al. (2016). Involvement of calcium/calmodulin-dependent protein kinase II in methamphetamine-induced neural damage. J. Appl. Toxicol. 36, 1460–1467. doi: 10.1002/jat.3301
Childress, A. C., and Berry, S. A. (2012). Pharmacotherapy of attention-deficit hyperactivity disorder in adolescents. Drugs 72, 309–325. doi: 10.2165/11599580-000000000-00000
Cho, S. C., Hong, Y. C., Kim, J. W., Park, S., Park, M. H., Hur, J., et al. (2013). Association between urine cotinine levels, continuous performance test variables, and attention deficit hyperactivity disorder and learning disability symptoms in school-aged children. Psychol. Med. 43, 209–219. doi: 10.1017/S0033291712001109
Cho, S.-C., Kim, J.-W., Kim, B.-N., Hwang, J.-W., Shin, M.-S., Park, M., et al. (2008). Association between the alpha-2C-adrenergic receptor gene and attention deficit hyperactivity disorder in a Korean sample. Neurosci. Lett. 446, 108–111. doi: 10.1016/j.neulet.2008.09.058
Choi, J.-W., Jung, A.-H., Nam, S., Kim, K. M., Kim, J. W., Kim, S. Y., et al. (2020). Interaction between lead and noradrenergic genotypes affects neurocognitive functions in attention-deficit/hyperactivity disorder: a case control study. BMC Psychiatry 20:407. doi: 10.1186/s12888-020-02799-3
Clavenna, A., and Bonati, M. (2017). Pediatric pharmacoepidemiology - safety and effectiveness of medicines for ADHD. Expert Opin. Drug Saf. 16, 1335–1345. doi: 10.1080/14740338.2017.1389894
Collipp, P. J., Chen, S. Y., and Maitinsky, S. (1983). Manganese in infant formulas and learning disability. Ann. Nutr. Metab. 27, 488–494. doi: 10.1159/000176724
Conn, P. J., and Pin, J. P. (1997). Pharmacology and functions of metabotropic glutamate receptors. Annu. Rev. Pharmacol. Toxicol. 37, 205–237. doi: 10.1146/annurev.pharmtox.37.1.205
Cormier, E., and Elder, J. H. (2007). Diet and child behavior problems: fact or fiction? Pediatr. Nurs. 33, 138–143.
Coskun, S., Karadag, M., Gokcen, C., and Oztuzcu, S. (2021). miR-132 and miR-942 expression levels in children with attention deficit and hyperactivity disorder: a controlled study. Clin. Psychopharmacol. Neurosci. Off. Sci. J. Korean Coll. Neuropsychopharmacol. 19, 262–268. doi: 10.9758/cpn.2021.19.2.262
Courvoisie, H., Hooper, S. R., Fine, C., Kwock, L., and Castillo, M. (2004). Neurometabolic functioning and neuropsychological correlates in children with ADHD-H: preliminary findings. J. Neuropsychiatry Clin. Neurosci. 16, 63–69. doi: 10.1176/jnp.16.1.63
Cryan, J. F., Dalvi, A., Jin, S. H., Hirsch, B. R., Lucki, I., and Thomas, S. A. (2001). Use of dopamine-beta-hydroxylase-deficient mice to determine the role of norepinephrine in the mechanism of action of antidepressant drugs. J. Pharmacol. Exp. Ther. 298, 651–657.
Cryan, J. F., Kelly, P. H., Neijt, H. C., Sansig, G., Flor, P. J., and van Der Putten, H. (2003). Antidepressant and anxiolytic-like effects in mice lacking the group III metabotropic glutamate receptor mGluR7. Eur. J. Neurosci. 17, 2409–2417. doi: 10.1046/j.1460-9568.2003.02667.x
Cutler, A. J., Mattingly, G. W., Jain, R., and O’Neal, W. (2020). Current and future nonstimulants in the treatment of pediatric ADHD: monoamine reuptake inhibitors, receptor modulators, and multimodal agents. CNS Spectr. 27, 199–207. doi: 10.1017/S1092852920001984
Dalezios, Y., Luján, R., Shigemoto, R., Roberts, J. D. B., and Somogyi, P. (2002). Enrichment of mGluR7a in the presynaptic active zones of GABAergic and non-GABAergic terminals on interneurons in the rat somatosensory cortex. Cereb. Cortex 12, 961–974. doi: 10.1093/cercor/12.9.961
Dalley, J. W., Theobald, D. E., Pereira, E. A. C., Li, P. M. M. C., and Robbins, T. W. (2002). Specific abnormalities in serotonin release in the prefrontal cortex of isolation-reared rats measured during behavioural performance of a task assessing visuospatial attention and impulsivity. Psychopharmacology 164, 329–340. doi: 10.1007/s00213-002-1215-y
Das, M., Bhowmik, A. D., Sinha, S., Chattopadhyay, A., Chaudhuri, K., Singh, M., et al. (2006). MAOA promoter polymorphism and attention deficit hyperactivity disorder (ADHD) in indian children. Am. J. Med. Genet. B Neuropsychiatr. Genet. Off. Publ. Int. Soc. Psychiatr. Genet. 141B, 637–642. doi: 10.1002/ajmg.b.30385
Daubner, S. C., Le, T., and Wang, S. (2011). Tyrosine hydroxylase and regulation of dopamine synthesis. Arch. Biochem. Biophys. 508, 1–12. doi: 10.1016/j.abb.2010.12.017
Dela Peña, I. J. I, Botanas, C. J., de la Peña, J. B., Custodio, R. J., Dela Peña, I., Ryoo, Z. Y., et al. (2019). The Atxn7-overexpressing mice showed hyperactivity and impulsivity which were ameliorated by atomoxetine treatment: a possible animal model of the hyperactive-impulsive phenotype of ADHD. Prog. Neuropsychopharmacol. Biol. Psychiatry 88, 311–319. doi: 10.1016/j.pnpbp.2018.08.012
Di Matteo, V., Di Giovanni, G., Pierucci, M., and Esposito, E. (2008). Serotonin control of central dopaminergic function: focus on in vivo microdialysis studies. Prog. Brain Res. 172, 7–44. doi: 10.1016/S0079-6123(08)00902-3
Diamond, A. (2007). Consequences of variations in genes that affect dopamine in prefrontal cortex. Cereb. Cortex 17, (Suppl. 1), i161–i170. doi: 10.1093/cercor/bhm082
Dias, T. G. C., Kieling, C., Graeff-Martins, A. S., Moriyama, T. S., Rohde, L. A., and Polanczyk, G. V. (2013). Developments and challenges in the diagnosis and treatment of ADHD. Rev. Bras. Psiquiatr. 35, (Suppl. 1), S40–S50. doi: 10.1590/1516-4446-2013-S103
Domschke, K., Sheehan, K., Lowe, N., Kirley, A., Mullins, C., O’sullivan, R., et al. (2005). Association analysis of the monoamine oxidase A and B genes with attention deficit hyperactivity disorder (ADHD) in an Irish sample: preferential transmission of the MAO-A 941G allele to affected children. Am. J. Med. Genet. B Neuropsychiatr. Genet. Off. Publ. Int. Soc. Psychiatr. Genet. 134B, 110–114. doi: 10.1002/ajmg.b.30158
Eagle, D. M., Wong, J. C. K., Allan, M. E., Mar, A. C., Theobald, D. E., and Robbins, T. W. (2011). Contrasting roles for dopamine D1 and D2 receptor subtypes in the dorsomedial striatum but not the nucleus accumbens core during behavioral inhibition in the stop-signal task in rats. J. Neurosci. Off. J. Soc. Neurosci. 31, 7349–7356. doi: 10.1523/JNEUROSCI.6182-10.2011
Eichler, A., Hudler, L., Grunitz, J., Grimm, J., Raabe, E., Goecke, T. W., et al. (2018). Effects of prenatal alcohol consumption on cognitive development and ADHD-related behaviour in primary-school age: a multilevel study based on meconium ethyl glucuronide. J. Child Psychol. Psychiatry 59, 110–118. doi: 10.1111/jcpp.12794
Elia, J., Glessner, J. T., Wang, K., Takahashi, N., Shtir, C. J., Hadley, D., et al. (2011). Genome-wide copy number variation study associates metabotropic glutamate receptor gene networks with attention deficit hyperactivity disorder. Nat. Genet. 44, 78–84. doi: 10.1038/ng.1013
Elwan, M. A., Richardson, J. R., Guillot, T. S., Caudle, W. M., and Miller, G. W. (2006). Pyrethroid pesticide-induced alterations in dopamine transporter function. Toxicol. Appl. Pharmacol. 211, 188–197. doi: 10.1016/j.taap.2005.06.003
English, B. A., Hahn, M. K., Gizer, I. R., Mazei-Robison, M., Steele, A., Kurnik, D. M., et al. (2009). Choline transporter gene variation is associated with attention-deficit hyperactivity disorder. J. Neurodev. Disord. 1, 252–263. doi: 10.1007/s11689-009-9033-8
Ernst, M., Zametkin, A. J., Matochik, J. A., Pascualvaca, D., Jons, P. H., and Cohen, R. M. (1999). High midbrain [18F]DOPA accumulation in children with attention deficit hyperactivity disorder. Am. J. Psychiatry 156, 1209–1215. doi: 10.1176/ajp.156.8.1209
Fageera, W., Sengupta, S. M., Fortier, M. -È, Grizenko, N., Babienco, S., Labbe, A., et al. (2021). Sex-dependent complex association of TPH2 with multiple dimensions of ADHD. Prog. Neuropsychopharmacol. Biol. Psychiatry 110:110296. doi: 10.1016/j.pnpbp.2021.110296
Famularo, R., Kinscherff, R., and Fenton, T. (1992). Psychiatric diagnoses of maltreated children: preliminary findings. J. Am. Acad. Child Adolesc. Psychiatry 31, 863–867. doi: 10.1097/00004583-199209000-00013
Faraone, S. V. (2004). Genetics of adult attention-deficit/hyperactivity disorder. Psychiatr. Clin. North Am. 27, 303–321. doi: 10.1016/S0193-953X(03)00090-X
Faraone, S. V. (2018). The pharmacology of amphetamine and methylphenidate: relevance to the neurobiology of attention-deficit/hyperactivity disorder and other psychiatric comorbidities. Neurosci. Biobehav. Rev. 87, 255–270. doi: 10.1016/j.neubiorev.2018.02.001
Faraone, S. V., Biederman, J., Weiffenbach, B., Keith, T., Chu, M. P., Weaver, A., et al. (1999). Dopamine D4 gene 7-repeat allele and attention deficit hyperactivity disorder. Am. J. Psychiatry 156, 768–770. doi: 10.1176/ajp.156.5.768
Faraone, S. V., Perlis, R. H., Doyle, A. E., Smoller, J. W., Goralnick, J. J., Holmgren, M. A., et al. (2005). Molecular genetics of attention-deficit/hyperactivity disorder. Biol. Psychiatry 57, 1313–1323. doi: 10.1016/j.biopsych.2004.11.024
Fayyad, J., De Graaf, R., Kessler, R., Alonso, J., Angermeyer, M., Demyttenaere, K., et al. (2007). Cross-national prevalence and correlates of adult attention-deficit hyperactivity disorder. Br. J. Psychiatry 190, 402–409. doi: 10.1192/bjp.bp.106.034389
Fendt, M., Bürki, H., Imobersteg, S., van der Putten, H., McAllister, K., Leslie, J. C., et al. (2010). The effect of mGlu8 deficiency in animal models of psychiatric diseases. Genes Brain Behav. 9, 33–44. doi: 10.1111/j.1601-183X.2009.00532.x
Fernandez, F., Byrne, M. K., Batterham, M., Grant, L., and Meyer, B. J. (2022). Associations between Omega-3 index, dopaminergic genetic variants and aggressive and metacognitive traits: a study in adult male prisoners. Nutrients 14:1379. doi: 10.3390/nu14071379
Ferraguti, F., Klausberger, T., Cobden, P., Baude, A., Roberts, J. D. B., Szucs, P., et al. (2005). Metabotropic glutamate receptor 8-expressing nerve terminals target subsets of GABAergic neurons in the hippocampus. J. Neurosci. 25, 10520–10536. doi: 10.1523/JNEUROSCI.2547-05.2005
Finazzi, D., and Arosio, P. (2014). Biology of ferritin in mammals: an update on iron storage, oxidative damage and neurodegeneration. Arch. Toxicol. 88, 1787–1802. doi: 10.1007/s00204-014-1329-0
Foltinová, J., Foltin, V., and Neu, E. (2007). Occurrence of lead in placenta–important information for prenatal and postnatal development of child. Neuro Endocrinol. Lett. 28, 335–340.
Fraporti, T. T., Contini, V., Tovo-Rodrigues, L., Recamonde-Mendoza, M., Rovaris, D. L., Rohde, L. A., et al. (2019). Synergistic effects between ADORA2A and DRD2 genes on anxiety disorders in children with ADHD. Prog. Neuropsychopharmacol. Biol. Psychiatry 93, 214–220. doi: 10.1016/j.pnpbp.2019.03.021
Freese, L., Muller, E. J., Souza, M. F., Couto-Pereira, N. S., Tosca, C. F., Ferigolo, M., et al. (2012). GABA system changes in methylphenidate sensitized female rats. Behav. Brain Res. 231, 181–186. doi: 10.1016/j.bbr.2012.03.017
Gagnon, D., Petryszyn, S., Sanchez, M. G., Bories, C., Beaulieu, J. M., De Koninck, Y., et al. (2017). Striatal neurons expressing D(1) and D(2) receptors are morphologically distinct and differently affected by dopamine denervation in mice. Sci. Rep. 7:41432. doi: 10.1038/srep41432
Gainetdinov, R. R., Wetsel, W. C., Jones, S. R., Levin, E. D., Jaber, M., and Caron, M. G. (1999). Role of serotonin in the paradoxical calming effect of psychostimulants on hyperactivity. Science 283, 397–401. doi: 10.1126/science.283.5400.397
Gao, Q., Liu, L., Chen, Y., Li, H., Yang, L., Wang, Y., et al. (2015). Synaptosome-related (SNARE) genes and their interactions contribute to the susceptibility and working memory of attention-deficit/hyperactivity disorder in males. Prog. Neuropsychopharmacol. Biol. Psychiatry 57, 132–139. doi: 10.1016/j.pnpbp.2014.11.001
Gao, Q., Liu, L., Qian, Q., and Wang, Y. (2014). Advances in molecular genetic studies of attention deficit hyperactivity disorder in China. Shanghai Arch. Psychiatry 26, 194–206. doi: 10.3969/j.issn.1002-0829.2014.04.003
Garcia-Martínez, I., Sánchez-Mora, C., Pagerols, M., Richarte, V., Corrales, M., Fadeuilhe, C., et al. (2016). Preliminary evidence for association of genetic variants in pri-miR-34b/c and abnormal miR-34c expression with attention deficit and hyperactivity disorder. Transl. Psychiatry 6:e879. doi: 10.1038/tp.2016.151
Garnock-Jones, K. P., and Keating, G. M. (2009). Atomoxetine: a review of its use in attention-deficit hyperactivity disorder in children and adolescents. Paediatr. Drugs 11, 203–226. doi: 10.2165/00148581-200911030-00005
Gaspar, P., Berger, B., Febvret, A., Vigny, A., and Henry, J. P. (1989). Catecholamine innervation of the human cerebral cortex as revealed by comparative immunohistochemistry of tyrosine hydroxylase and dopamine-beta-hydroxylase. J. Comp. Neurol. 279, 249–271. doi: 10.1002/cne.902790208
Gerlai, R., Adams, B., Fitch, T., Chaney, S., and Baez, M. (2002). Performance deficits of mGluR8 knockout mice in learning tasks: the effects of null mutation and the background genotype. Neuropharmacology 43, 235–249. doi: 10.1016/s0028-3908(02)00078-3
Ghorayeb, I., Gamas, A., Mazurie, Z., and Mayo, W. (2019). Attention-deficit hyperactivity and obsessive-compulsive symptoms in adult patients with primary restless legs syndrome: different phenotypes of the same disease? Behav. Sleep Med. 17, 246–253. doi: 10.1080/15402002.2017.1326919
Gibson, M. A., Butters, N. S., Reynolds, J. N., and Brien, J. F. (2000). Effects of chronic prenatal ethanol exposure on locomotor activity, and hippocampal weight, neurons, and nitric oxide synthase activity of the young postnatal guinea pig. Neurotoxicol. Teratol. 22, 183–192. doi: 10.1016/s0892-0362(99)00074-4
Gillentine, M. A., Berry, L. N., Goin-Kochel, R. P., Ali, M. A., Ge, J., Guffey, D., et al. (2017). The cognitive and behavioral phenotypes of individuals with CHRNA7 duplications. J. Autism Dev. Disord. 47, 549–562. doi: 10.1007/s10803-016-2961-8
Gil-Sanz, C., Delgado-García, J. M., Fairén, A., and Gruart, A. (2008). Involvement of the mGluR1 receptor in hippocampal synaptic plasticity and associative learning in behaving mice. Cereb. Cortex 18, 1653–1663. doi: 10.1093/cercor/bhm193
Gizer, I. R., Ficks, C., and Waldman, I. D. (2009). Candidate gene studies of ADHD: a meta-analytic review. Hum. Genet. 126, 51–90. doi: 10.1007/s00439-009-0694-x
Gonzalez-Lopez, E., and Vrana, K. E. (2020). Dopamine beta-hydroxylase and its genetic variants in human health and disease. J. Neurochem. 152, 157–181. doi: 10.1111/jnc.14893
Goodlad, J. K., Marcus, D. K., and Fulton, J. J. (2013). Lead and attention-deficit/hyperactivity disorder (ADHD) symptoms: a meta-analysis. Clin. Psychol. Rev. 33, 417–425. doi: 10.1016/j.cpr.2013.01.009
Gotti, C., Zoli, M., and Clementi, F. (2006). Brain nicotinic acetylcholine receptors: native subtypes and their relevance. Trends Pharmacol. Sci. 27, 482–491. doi: 10.1016/j.tips.2006.07.004
Grady, S. R., Murphy, K. L., Cao, J., Marks, M. J., McIntosh, J. M., and Collins, A. C. (2002). Characterization of nicotinic agonist-induced [(3)H]dopamine release from synaptosomes prepared from four mouse brain regions. J. Pharmacol. Exp. Ther. 301, 651–660. doi: 10.1124/jpet.301.2.651
Green, A. L., Eid, A., Zhan, L., Zarbl, H., Guo, G. L., and Richardson, J. R. (2019). Epigenetic regulation of the ontogenic expression of the dopamine transporter. Front. Genet. 10:1099. doi: 10.3389/fgene.2019.01099
Groman, C. M. J., and Barzman, D. H. (2014). The impact of ADHD on morality development. Atten. Defic. Hyperact. Disord. 6, 67–71. doi: 10.1007/s12402-014-0131-4
Guan, L., Wang, B., Chen, Y., Yang, L., Li, J., Qian, Q., et al. (2009). A high-density single-nucleotide polymorphism screen of 23 candidate genes in attention deficit hyperactivity disorder: suggesting multiple susceptibility genes among Chinese Han population. Mol. Psychiatry 14, 546–554. doi: 10.1038/sj.mp.4002139
Gudmundsson, O. O., Walters, G. B., Ingason, A., Johansson, S., Zayats, T., Athanasiu, L., et al. (2019). Attention-deficit hyperactivity disorder shares copy number variant risk with schizophrenia and autism spectrum disorder. Transl. Psychiatry 9:258. doi: 10.1038/s41398-019-0599-y
Gul, M. K., Sener, E. F., Onal, M. G., and Demirci, E. (2021). Role of the norepinephrine transporter polymorphisms in atomoxetine treatment: from response to side effects in children with ADHD. J. Psychopharmacol. 36, 715–722. doi: 10.1177/02698811211015245
Gutknecht, L., Araragi, N., Merker, S., Waider, J., Sommerlandt, F. M. J., Mlinar, B., et al. (2012). Impacts of brain serotonin deficiency following Tph2 inactivation on development and raphe neuron serotonergic specification. PLoS One 7:e43157. doi: 10.1371/journal.pone.0043157
Gutknecht, L., Kriegebaum, C., Waider, J., Schmitt, A., and Lesch, K.-P. (2009). Spatio-temporal expression of tryptophan hydroxylase isoforms in murine and human brain: convergent data from Tph2 knockout mice. Eur. Neuropsychopharmacol. J. Eur. Coll. Neuropsychopharmacol. 19, 266–282. doi: 10.1016/j.euroneuro.2008.12.005
Hariri, M., and Azadbakht, L. (2015). Magnesium, iron, and Zinc supplementation for the treatment of attention deficit hyperactivity disorder: a systematic review on the recent literature. Int. J. Prev. Med. 6:83. doi: 10.4103/2008-7802.164313
Harris, S. S., Green, S. M., Kumar, M., and Urs, N. M. (2022). A role for cortical dopamine in the paradoxical calming effects of psychostimulants. Sci. Rep. 12:3129. doi: 10.1038/s41598-022-07029-2
Hausknecht, K. A., Acheson, A., Farrar, A. M., Kieres, A. K., Shen, R.-Y., Richards, J. B., et al. (2005). Prenatal alcohol exposure causes attention deficits in male rats. Behav. Neurosci. 119, 302–310. doi: 10.1037/0735-7044.119.1.302
Hawi, Z., McCarron, M., Kirley, A., Daly, G., Fitzgerald, M., and Gill, M. (2000). No association of the dopamine DRD4 receptor (DRD4) gene polymorphism with attention deficit hyperactivity disorder (ADHD) in the Irish population. Am. J. Med. Genet. 96, 268–272. doi: 10.1002/1096-8628(20000612)96:3<268::aid-ajmg6>3.0.co;2-#
Heinrich, H., Grunitz, J., Stonawski, V., Frey, S., Wahl, S., Albrecht, B., et al. (2017). Attention, cognitive control and motivation in ADHD: linking event-related brain potentials and DNA methylation patterns in boys at early school age. Sci. Rep. 7:3823. doi: 10.1038/s41598-017-03326-3
Helms, C. M., Gubner, N. R., Wilhelm, C. J., Mitchell, S. H., and Grandy, D. K. (2008). D4 receptor deficiency in mice has limited effects on impulsivity and novelty seeking. Pharmacol. Biochem. Behav. 90, 387–393. doi: 10.1016/j.pbb.2008.03.013
Hersch, S. M., Ciliax, B. J., Gutekunst, C. A., Rees, H. D., Heilman, C. J., Yung, K. K., et al. (1995). Electron microscopic analysis of D1 and D2 dopamine receptor proteins in the dorsal striatum and their synaptic relationships with motor corticostriatal afferents. J. Neurosci. 15, 5222–5237. doi: 10.1523/JNEUROSCI.15-07-05222.1995
Hodgkins, P., Shaw, M., Coghill, D., and Hechtman, L. (2012). Amfetamine and methylphenidate medications for attention-deficit/hyperactivity disorder: complementary treatment options. Eur. Child Adolesc. Psychiatry 21, 477–492. doi: 10.1007/s00787-012-0286-5
Holtkamp, K., Konrad, K., Müller, B., Heussen, N., Herpertz, S., Herpertz-Dahlmann, B., et al. (2004). Overweight and obesity in children with attention-deficit/hyperactivity disorder. Int. J. Obes. Relat. Metab. Disord. J. Int. Assoc. Study Obes. 28, 685–689. doi: 10.1038/sj.ijo.0802623
Hong, E. J., McCord, A. E., and Greenberg, M. E. (2008). A biological function for the neuronal activity-dependent component of Bdnf transcription in the development of cortical inhibition. Neuron 60, 610–624. doi: 10.1016/j.neuron.2008.09.024
Hong, J. H., Hwang, I. W., Lim, M. H., Kwon, H. J., and Jin, H. J. (2018). Genetic associations between ADHD and dopaminergic genes (DAT1 and DRD4) VNTRs in Korean children. Genes Genom. 40, 1309–1317. doi: 10.1007/s13258-018-0726-9
Hou, Y.-W., Xiong, P., Gu, X., Huang, X., Wang, M., and Wu, J. (2018). Association of serotonin receptors with attention deficit hyperactivity disorder: a systematic review and meta-analysis. Curr. Med. Sci. 38, 538–551. doi: 10.1007/s11596-018-1912-3
Howard, A. L., Robinson, M., Smith, G. J., Ambrosini, G. L., Piek, J. P., and Oddy, W. H. (2011). ADHD is associated with a “Western” dietary pattern in adolescents. J. Atten. Disord. 15, 403–411. doi: 10.1177/1087054710365990
Hu, J., Liao, J., Sathanoori, M., Kochmar, S., Sebastian, J., Yatsenko, S. A., et al. (2015). CNTN6 copy number variations in 14 patients: a possible candidate gene for neurodevelopmental and neuropsychiatric disorders. J. Neurodev. Disord. 7:26. doi: 10.1186/s11689-015-9122-9
Huang, J., Zhong, Z., Wang, M., Chen, X., Tan, Y., Zhang, S., et al. (2015). Circadian modulation of dopamine levels and dopaminergic neuron development contributes to attention deficiency and hyperactive behavior. J. Neurosci. 35, 2572–2587. doi: 10.1523/JNEUROSCI.2551-14.2015
Hyacinthe, C., De Deurwaerdere, P., Thiollier, T., Li, Q., Bezard, E., and Ghorayeb, I. (2015). Blood withdrawal affects iron store dynamics in primates with consequences on monoaminergic system function. Neuroscience 290, 621–635. doi: 10.1016/j.neuroscience.2015.01.057
Ihalainen, J. A., Tanila, H., Scheinin, M., and Riekkinen, P. J. (2001). alpha(2C)-Adrenoceptors modulate the effect of methylphenidate on response rate and discrimination accuracy in an operant test. Brain Res. Bull. 54, 553–557. doi: 10.1016/s0361-9230(01)00449-x
Jarick, I., Volckmar, A.-L., Pütter, C., Pechlivanis, S., Nguyen, T. T., Dauvermann, M. R., et al. (2014). Genome-wide analysis of rare copy number variations reveals PARK2 as a candidate gene for attention-deficit/hyperactivity disorder. Mol. Psychiatry 19, 115–121. doi: 10.1038/mp.2012.161
Jönsson, E. G., Nöthen, M. M., Grünhage, F., Farde, L., Nakashima, Y., Propping, P., et al. (1999). Polymorphisms in the dopamine D2 receptor gene and their relationships to striatal dopamine receptor density of healthy volunteers. Mol. Psychiatry 4, 290–296. doi: 10.1038/sj.mp.4000532
Kandemir, H., Erdal, M. E., Selek, S., Ay, ÖÝ, Karababa, I. F., Kandemir, S. B., et al. (2014). Evaluation of several micro RNA (miRNA) levels in children and adolescents with attention deficit hyperactivity disorder. Neurosci. Lett. 580, 158–162. doi: 10.1016/j.neulet.2014.07.060
Kendall, T., Taylor, E., Perez, A., and Taylor, C. (2008). Diagnosis and management of attention-deficit/hyperactivity disorder in children, young people, and adults: summary of NICE guidance. BMJ 337:a1239. doi: 10.1136/bmj.a1239
Kent, L., Green, E., Holmes, J., Thapar, A., Gill, M., Hawi, Z., et al. (2001a). No association between CHRNA7 microsatellite markers and attention-deficit hyperactivity disorder. Am. J. Med. Genet. 105, 686–689. doi: 10.1002/ajmg.1553
Kent, L., Middle, F., Hawi, Z., Fitzgerald, M., Gill, M., Feehan, C., et al. (2001b). Nicotinic acetylcholine receptor alpha4 subunit gene polymorphism and attention deficit hyperactivity disorder. Psychiatr. Genet. 11, 37–40. doi: 10.1097/00041444-200103000-00007
Kieling, C., Genro, J. P., Hutz, M. H., and Rohde, L. A. (2008). The -1021 C/T DBH polymorphism is associated with neuropsychological performance among children and adolescents with ADHD. Am. J. Med. Genet. B Neuropsychiatr. Genet. Off. Publ. Int. Soc. Psychiatr. Genet. 147B, 485–490. doi: 10.1002/ajmg.b.30636
Kim, J. I., Kim, J.-W., Lee, J.-M., Yun, H. J., Sohn, C.-H., Shin, M.-S., et al. (2018a). Interaction between DRD2 and lead exposure on the cortical thickness of the frontal lobe in youth with attention-deficit/hyperactivity disorder. Prog. Neuropsychopharmacol. Biol. Psychiatry 82, 169–176. doi: 10.1016/j.pnpbp.2017.11.018
Kim, J. I., Kim, J.-W., Shin, I., and Kim, B.-N. (2018b). Effects of interaction between DRD4 methylation and prenatal maternal stress on methylphenidate-induced changes in continuous performance test performance in youth with attention-deficit/hyperactivity disorder. J. Child Adolesc. Psychopharmacol. 28, 562–570. doi: 10.1089/cap.2018.0054
Kim, J. I., Kim, J.-W., Shin, I., and Kim, B.-N. (2021). Interaction of DRD4 methylation and phthalate metabolites affects continuous performance test performance in ADHD. J. Atten. Disord. 25, 161–170. doi: 10.1177/1087054718776466
Kinoshita, A., Shigemoto, R., Ohishi, H., van der Putten, H., and Mizuno, N. (1998). Immunohistochemical localization of metabotropic glutamate receptors, mGluR7a and mGluR7b, in the central nervous system of the adult rat and mouse: a light and electron microscopic study. J. Comp. Neurol. 393, 332–352.
Klein, R. G., Mannuzza, S., Olazagasti, M. A. R., Roizen, E., Hutchison, J. A., Lashua, E. C., et al. (2012). Clinical and functional outcome of childhood attention-deficit/hyperactivity disorder 33 years later. Arch. Gen. Psychiatry 69, 1295–1303. doi: 10.1001/archgenpsychiatry.2012.271
Klink, R., de Kerchove d’Exaerde, A., Zoli, M., and Changeux, J. P. (2001). Molecular and physiological diversity of nicotinic acetylcholine receptors in the midbrain dopaminergic nuclei. J. Neurosci. 21, 1452–1463. doi: 10.1523/JNEUROSCI.21-05-01452.2001
Knopik, V. S., Sparrow, E. P., Madden, P. A. F., Bucholz, K. K., Hudziak, J. J., Reich, W., et al. (2005). Contributions of parental alcoholism, prenatal substance exposure, and genetic transmission to child ADHD risk: a female twin study. Psychol. Med. 35, 625–635. doi: 10.1017/s0033291704004155
Koike, H., Ibi, D., Mizoguchi, H., Nagai, T., Nitta, A., Takuma, K., et al. (2009). Behavioral abnormality and pharmacologic response in social isolation-reared mice. Behav. Brain Res. 202, 114–121. doi: 10.1016/j.bbr.2009.03.028
Konofal, E., Lecendreux, M., Arnulf, I., and Mouren, M.-C. (2004). Iron deficiency in children with attention-deficit/hyperactivity disorder. Arch. Pediatr. Adolesc. Med. 158, 1113–1115. doi: 10.1001/archpedi.158.12.1113
Konofal, E., Lecendreux, M., Deron, J., Marchand, M., Cortese, S., Zaïm, M., et al. (2008). Effects of iron supplementation on attention deficit hyperactivity disorder in children. Pediatr. Neurol. 38, 20–26. doi: 10.1016/j.pediatrneurol.2007.08.014
Kramer, P. F., Twedell, E. L., Shin, J. H., Zhang, R., and Khaliq, Z. M. (2020). Axonal mechanisms mediating γ-aminobutyric acid receptor type A (GABA-A) inhibition of striatal dopamine release. eLife 9:e55729. doi: 10.7554/eLife.55729
Kurzina, N. P., Volnova, A. B., Aristova, I. Y., and Gainetdinov, R. R. (2021). A new paradigm for training hyperactive dopamine transporter knockout rats: influence of novel stimuli on object recognition. Front. Behav. Neurosci. 15:654469. doi: 10.3389/fnbeh.2021.654469
Kwon, H. J., Ha, M., Jin, H. J., Hyun, J. K., Shim, S. H., Paik, K. C., et al. (2015). Association between BDNF gene polymorphisms and attention deficit hyperactivity disorder in Korean children. Genet. Test. Mol. Biomarkers 19, 366–371. doi: 10.1089/gtmb.2015.0029
Laakso, A., Pohjalainen, T., Bergman, J., Kajander, J., Haaparanta, M., Solin, O., et al. (2005). The A1 allele of the human D2 dopamine receptor gene is associated with increased activity of striatal L-amino acid decarboxylase in healthy subjects. Pharmacogenet. Genomics 15, 387–391. doi: 10.1097/01213011-200506000-00003
Lahat, E., Heyman, E., Livne, A., Goldman, M., Berkovitch, M., and Zachor, D. (2011). Iron deficiency in children with attention deficit hyperactivity disorder. Isr. Med. Assoc. J. 13, 530–533.
Lanphear, B. P., Hornung, R., Khoury, J., Yolton, K., Baghurst, P., Bellinger, D. C., et al. (2005). Low-level environmental lead exposure and children’s intellectual function: an international pooled analysis. Environ. Health Perspect. 113, 894–899. doi: 10.1289/ehp.7688
Lee, J., Laurin, N., Crosbie, J., Ickowicz, A., Pathare, T., Malone, M., et al. (2008). Association study of the nicotinic acetylcholine receptor alpha4 subunit gene, CHRNA4, in attention-deficit hyperactivity disorder. Genes Brain Behav. 7, 53–60. doi: 10.1111/j.1601-183X.2007.00325.x
Lee, W.-S., Lim, Y.-H., Kim, B.-N., Shin, C. H., Lee, Y. A., Kim, J. I., et al. (2020). Residential pyrethroid insecticide use, urinary 3-phenoxybenzoic acid levels, and attention-deficit/hyperactivity disorder-like symptoms in preschool-age children: the environment and development of children study. Environ. Res. 188:109739. doi: 10.1016/j.envres.2020.109739
Lei, S. (2014). Cross interaction of dopaminergic and adrenergic systems in neural modulation. Int. J. Physiol. Pathophysiol. Pharmacol. 6, 137–142.
Lepping, P., and Huber, M. (2010). Role of Zinc in the pathogenesis of attention-deficit hyperactivity disorder: implications for research and treatment. CNS Drugs 24, 721–728. doi: 10.2165/11537610-000000000-00000
Leung, P. W.-L., Chan, J. K. Y., Chen, L. H., Lee, C. C., Hung, S. F., Ho, T. P., et al. (2017). Family-based association study of DRD4 gene in methylphenidate-responded attention deficit/hyperactivity disorder. PLoS One 12:e0173748. doi: 10.1371/journal.pone.0173748
Levitan, R. D., Masellis, M., Basile, V. S., Lam, R. W., Jain, U., Kaplan, A. S., et al. (2002). Polymorphism of the serotonin-2A receptor gene (HTR2A) associated with childhood attention deficit hyperactivity disorder (ADHD) in adult women with seasonal affective disorder. J. Affect. Disord. 71, 229–233. doi: 10.1016/s0165-0327(01)00372-x
Li, S., Kim, K. Y., Kim, J. H., Kim, J. H., Park, M. S., Bahk, J. Y., et al. (2004). Chronic nicotine and smoking treatment increases dopamine transporter mRNA expression in the rat midbrain. Neurosci. Lett. 363, 29–32. doi: 10.1016/j.neulet.2004.03.053
Liu, Y., Chang, X., Qu, H.-Q., Tian, L., Glessner, J., Qu, J., et al. (2021a). Rare recurrent variants in noncoding regions impact attention-deficit hyperactivity disorder (ADHD) gene networks in children of both african american and european american ancestry. Genes 12:310. doi: 10.3390/genes12020310
Liu, Y., Qu, H.-Q., Chang, X., Nguyen, K., Qu, J., Tian, L., et al. (2021b). Deep learning prediction of attention-deficit hyperactivity disorder in African Americans by copy number variation. Exp. Biol. Med. 246, 2317–2323. doi: 10.1177/15353702211018970
Lladó-Pelfort, L., Santana, N., Ghisi, V., Artigas, F., and Celada, P. (2012). 5-HT1A receptor agonists enhance pyramidal cell firing in prefrontal cortex through a preferential action on GABA interneurons. Cereb. Cortex 22, 1487–1497. doi: 10.1093/cercor/bhr220
Loonen, A. J. M., Kupka, R. W., and Ivanova, S. A. (2017). Circuits regulating pleasure and happiness in bipolar disorder. Front. Neural Circ. 11:35. doi: 10.3389/fncir.2017.00035
Lou, H. C. (1996). Etiology and pathogenesis of attention-deficit hyperactivity disorder (ADHD): significance of prematurity and perinatal hypoxic-haemodynamic encephalopathy. Acta Paediatr. 85, 1266–1271. doi: 10.1111/j.1651-2227.1996.tb13909.x
Luca, P., Laurin, N., Misener, V. L., Wigg, K. G., Anderson, B., Cate-Carter, T., et al. (2007). Association of the dopamine receptor D1 gene, DRD1, with inattention symptoms in families selected for reading problems. Mol. Psychiatry 12, 776–785. doi: 10.1038/sj.mp.4001972
Lucki, I. (1998). The spectrum of behaviors influenced by serotonin. Biol. Psychiatry 44, 151–162. doi: 10.1016/s0006-3223(98)00139-5
Mahmoud, M. M., El-Mazary, A.-A. M., Maher, R. M., and Saber, M. M. (2011). Zinc, ferritin, magnesium and copper in a group of Egyptian children with attention deficit hyperactivity disorder. Ital. J. Pediatr. 37:60. doi: 10.1186/1824-7288-37-60
Makoff, A., Pilling, C., Harrington, K., and Emson, P. (1996). Human metabotropic glutamate receptor type 7: molecular cloning and mRNA distribution in the CNS. Brain Res. Mol. Brain Res. 40, 165–170. doi: 10.1016/0169-328x(96)00110-6
Mariggiò, M. A., Palumbi, R., Vinella, A., Laterza, R., Petruzzelli, M. G., Peschechera, A., et al. (2021). DRD1 and DRD2 receptor polymorphisms: genetic neuromodulation of the dopaminergic system as a risk factor for ASD, ADHD and ASD/ADHD overlap. Front. Neurosci. 15:705890. doi: 10.3389/fnins.2021.705890
Martin, J., Hosking, G., Wadon, M., Agha, S. S., Langley, K., Rees, E., et al. (2020). A brief report: de novo copy number variants in children with attention deficit hyperactivity disorder. Transl. Psychiatry 10:135. doi: 10.1038/s41398-020-0821-y
Martin, J., O’Donovan, M. C., Thapar, A., Langley, K., and Williams, N. (2015). The relative contribution of common and rare genetic variants to ADHD. Transl. Psychiatry 5:e506. doi: 10.1038/tp.2015.5
Martin, L. F., Kem, W. R., and Freedman, R. (2004). Alpha-7 nicotinic receptor agonists: potential new candidates for the treatment of schizophrenia. Psychopharmacology 174, 54–64. doi: 10.1007/s00213-003-1750-1
Mastronardi, C. A., Pillai, E., Pineda, D. A., Martinez, A. F., Lopera, F., Velez, J. I., et al. (2016). Linkage and association analysis of ADHD endophenotypes in extended and multigenerational pedigrees from a genetic isolate. Mol. Psychiatry 21, 1434–1440. doi: 10.1038/mp.2015.172
McAlonan, G. M., Cheung, V., Cheung, C., Chua, S. E., Murphy, D. G. M., Suckling, J., et al. (2007). Mapping brain structure in attention deficit-hyperactivity disorder: a voxel-based MRI study of regional grey and white matter volume. Psychiatry Res. 154, 171–180. doi: 10.1016/j.pscychresns.2006.09.006
McAlonan, G. M., Cheung, V., Chua, S. E., Oosterlaan, J., Hung, S., Tang, C., et al. (2009). Age-related grey matter volume correlates of response inhibition and shifting in attention-deficit hyperactivity disorder. Br. J. Psychiatry 194, 123–129. doi: 10.1192/bjp.bp.108.051359
McLeer, S. V., Callaghan, M., Henry, D., and Wallen, J. (1994). Psychiatric disorders in sexually abused children. J. Am. Acad. Child Adolesc. Psychiatry 33, 313–319. doi: 10.1097/00004583-199403000-00003
Medin, T., Rinholm, J. E., Owe, S. G., Sagvolden, T., Gjedde, A., Storm-Mathisen, J., et al. (2013). Low dopamine D5 receptor density in hippocampus in an animal model of attention-deficit/hyperactivity disorder (ADHD). Neuroscience 242, 11–20. doi: 10.1016/j.neuroscience.2013.03.036
Mereu, M., Contarini, G., Buonaguro, E. F., Latte, G., Managò, F., Iasevoli, F., et al. (2017). Dopamine transporter (DAT) genetic hypofunction in mice produces alterations consistent with ADHD but not schizophrenia or bipolar disorder. Neuropharmacology 121, 179–194. doi: 10.1016/j.neuropharm.2017.04.037
Miguel, P. M., Deniz, B. F., Deckmann, I., Confortim, H. D., Diaz, R., Laureano, D. P., et al. (2018). Prefrontal cortex dysfunction in hypoxic-ischaemic encephalopathy contributes to executive function impairments in rats: potential contribution for attention-deficit/hyperactivity disorder. World J. Biol. Psychiatry Off. J. World Fed. Soc. Biol. Psychiatry 19, 547–560. doi: 10.1080/15622975.2016.1273551
Mill, J., Asherson, P., Craig, I., and D’Souza, U. M. (2005a). Transient expression analysis of allelic variants of a VNTR in the dopamine transporter gene (DAT1). BMC Genet. 6:3. doi: 10.1186/1471-2156-6-3
Mill, J., Sagvolden, T., and Asherson, P. (2005b). Sequence analysis of Drd2, Drd4, and Dat1 in SHR and WKY rat strains. Behav. Brain Funct. 1:24. doi: 10.1186/1744-9081-1-24
Mill, J., Fisher, N., Curran, S., Richards, S., Taylor, E., and Asherson, P. (2003). Polymorphisms in the dopamine D4 receptor gene and attention-deficit hyperactivity disorder. Neuroreport 14, 1463–1466. doi: 10.1097/00001756-200308060-00011
Millan, M. J., Lejeune, F., and Gobert, A. (2000). Reciprocal autoreceptor and heteroreceptor control of serotonergic, dopaminergic and noradrenergic transmission in the frontal cortex: relevance to the actions of antidepressant agents. J. Psychopharmacol. 14, 114–138. doi: 10.1177/026988110001400202
Millichap, J. G., and Yee, M. M. (2012). The diet factor in attention-deficit/hyperactivity disorder. Pediatrics 129, 330–337. doi: 10.1542/peds.2011-2199
Mizuno, T., Schmauss, C., and Rayport, S. (2007). Distinct roles of presynaptic dopamine receptors in the differential modulation of the intrinsic synapses of medium-spiny neurons in the nucleus accumbens. BMC Neurosci. 8:8. doi: 10.1186/1471-2202-8-8
Myer, N. M., Boland, J. R., and Faraone, S. V. (2018). Pharmacogenetics predictors of methylphenidate efficacy in childhood ADHD. Mol. Psychiatry 23, 1929–1936. doi: 10.1038/mp.2017.234
Myint, A.-M., and Kim, Y.-K. (2014). Network beyond IDO in psychiatric disorders: revisiting neurodegeneration hypothesis. Prog. Neuropsychopharmacol. Biol. Psychiatry 48, 304–313. doi: 10.1016/j.pnpbp.2013.08.008
Naumova, D., Grizenko, N., Sengupta, S. M., and Joober, R. (2019). DRD4 exon 3 genotype and ADHD: randomised pharmacodynamic investigation of treatment response to methylphenidate. World J. Biol. Psychiatry Off. J. World Fed. Soc. Biol. Psychiatry 20, 486–495. doi: 10.1080/15622975.2017.1410221
Navandar, M., Martín-García, E., Maldonado, R., Lutz, B., Gerber, S., and Ruiz de Azua, I. (2021). Transcriptional signatures in prefrontal cortex confer vulnerability versus resilience to food and cocaine addiction-like behavior. Sci. Rep. 11:9076. doi: 10.1038/s41598-021-88363-9
Nayak, S., and Cassaday, H. J. (2003). The novel dopamine D4 receptor agonist (PD 168,077 maleate): doses with different effects on locomotor activity are without effect in classical conditioning. Prog. Neuropsychopharmacol. Biol. Psychiatry 27, 441–449. doi: 10.1016/S0278-5846(03)00031-9
Németh, N., Kovács-Nagy, R., Székely, A., Sasvári-Székely, M., and Rónai, Z. (2013). Association of impulsivity and polymorphic microRNA-641 target sites in the SNAP-25 gene. PLoS One 8:e84207. doi: 10.1371/journal.pone.0084207
Neuman, R. J., Lobos, E., Reich, W., Henderson, C. A., Sun, L.-W., and Todd, R. D. (2007). Prenatal smoking exposure and dopaminergic genotypes interact to cause a severe ADHD subtype. Biol. Psychiatry 61, 1320–1328. doi: 10.1016/j.biopsych.2006.08.049
Neville, M. J., Johnstone, E. C., and Walton, R. T. (2004). Identification and characterization of ANKK1: a novel kinase gene closely linked to DRD2 on chromosome band 11q23.1. Hum. Mutat. 23, 540–545. doi: 10.1002/humu.20039
Nevin, R., Jacobs, D. E., Berg, M., and Cohen, J. (2008). Monetary benefits of preventing childhood lead poisoning with lead-safe window replacement. Environ. Res. 106, 410–419. doi: 10.1016/j.envres.2007.09.003
Noaín, D., Avale, M. E., Wedemeyer, C., Calvo, D., Peper, M., and Rubinstein, M. (2006). Identification of brain neurons expressing the dopamine D4 receptor gene using BAC transgenic mice. Eur. J. Neurosci. 24, 2429–2438. doi: 10.1111/j.1460-9568.2006.05148.x
Nogueira, M., Bosch, R., Valero, S., Gómez-Barros, N., Palomar, G., Richarte, V., et al. (2014). Early-age clinical and developmental features associated to substance use disorders in attention-deficit/hyperactivity disorder in adults. Compr. Psychiatry 55, 639–649. doi: 10.1016/j.comppsych.2013.12.002
Nyman, E. S., Ogdie, M. N., Loukola, A., Varilo, T., Taanila, A., Hurtig, T., et al. (2007). ADHD candidate gene study in a population-based birth cohort: association with DBH and DRD2. J. Am. Acad. Child Adolesc. Psychiatry 46, 1614–1621. doi: 10.1097/chi.0b013e3181579682
Oades, R. D. (2008). Dopamine-serotonin interactions in attention-deficit hyperactivity disorder (ADHD). Prog. Brain Res. 172, 543–565. doi: 10.1016/S0079-6123(08)00926-6
Olsen, D., Wellner, N., Kaas, M., de Jong, I. E. M., Sotty, F., Didriksen, M., et al. (2021). Altered dopaminergic firing pattern and novelty response underlie ADHD-like behavior of SorCS2-deficient mice. Transl. Psychiatry 11:74. doi: 10.1038/s41398-021-01199-9
Ouellet-Morin, I., Wigg, K. G., Feng, Y., Dionne, G., Robaey, P., Brendgen, M., et al. (2008). Association of the dopamine transporter gene and ADHD symptoms in a Canadian population-based sample of same-age twins. Am. J. Med. Genet. B Neuropsychiatr. Genet. Off. Publ. Int. Soc. Psychiatr. Genet. 147B, 1442–1449. doi: 10.1002/ajmg.b.30677
Palaniyappan, L., Batty, M. J., Liddle, P. F., Liddle, E. B., Groom, M. J., Hollis, C., et al. (2019). Reduced prefrontal gyrification in carriers of the dopamine D4 receptor 7-repeat allele with attention deficit/hyperactivity disorder: a preliminary report. Front. Psychiatry 10:235. doi: 10.3389/fpsyt.2019.00235
Pan, Y.-Q., Qiao, L., Xue, X.-D., and Fu, J.-H. (2015). Association between ANKK1 (rs1800497) polymorphism of DRD2 gene and attention deficit hyperactivity disorder: a meta-analysis. Neurosci. Lett. 590, 101–105. doi: 10.1016/j.neulet.2015.01.076
Parish, C. L., Nunan, J., Finkelstein, D. I., McNamara, F. N., Wong, J. Y., Waddington, J. L., et al. (2005). Mice lacking the alpha4 nicotinic receptor subunit fail to modulate dopaminergic neuronal arbors and possess impaired dopamine transporter function. Mol. Pharmacol. 68, 1376–1386. doi: 10.1124/mol.104.004820
Park, S., Jung, S.-W., Kim, B.-N., Cho, S.-C., Shin, M.-S., Kim, J.-W., et al. (2013). Association between the GRM7 rs3792452 polymorphism and attention deficit hyperacitiveity disorder in a Korean sample. Behav. Brain Funct. 9:1. doi: 10.1186/1744-9081-9-1
Park, S., Kim, B.-N., Cho, S.-C., Kim, J.-W., Kim, J. I., Shin, M.-S., et al. (2014). The metabotropic glutamate receptor subtype 7 rs3792452 polymorphism is associated with the response to methylphenidate in children with attention-deficit/hyperactivity disorder. J. Child Adolesc. Psychopharmacol. 24, 223–227. doi: 10.1089/cap.2013.0079
Park, T. W., Park, Y. H., Kwon, H. J., and Lim, M. H. (2013). Association between TPH2 gene polymorphisms and attention deficit hyperactivity disorder in Korean children. Genet. Test. Mol. Biomarkers 17, 301–306. doi: 10.1089/gtmb.2012.0376
Park, Y. H., Lee, K. K., Kwon, H. J., Ha, M., Kim, E. J., Yoo, S. J., et al. (2013). Association between HTR1A gene polymorphisms and attention deficit hyperactivity disorder in Korean children. Genet. Test. Mol. Biomarkers 17, 178–182. doi: 10.1089/gtmb.2012.0214
Paz, R., Barsness, B., Martenson, T., Tanner, D., and Allan, A. M. (2007). Behavioral teratogenicity induced by nonforced maternal nicotine consumption. Neuropsychopharmacol. Off. Publ. Am. Coll. Neuropsychopharmacol. 32, 693–699. doi: 10.1038/sj.npp.1301066
Pelham, W. E., Foster, E. M., and Robb, J. A. (2007). The economic impact of attention-deficit/hyperactivity disorder in children and adolescents. Ambul. Pediatr. 7, 121–131. doi: 10.1016/j.ambp.2006.08.002
Picciotto, M. R., Zoli, M., Rimondini, R., Léna, C., Marubio, L. M., Pich, E. M., et al. (1998). Acetylcholine receptors containing the beta2 subunit are involved in the reinforcing properties of nicotine. Nature 391, 173–177. doi: 10.1038/34413
Pinal, C. S., and Tobin, A. J. (1998). Uniqueness and redundancy in GABA production. Perspect. Dev. Neurobiol. 5, 109–118.
Pineau, G., Villemonteix, T., Slama, H., Kavec, M., Balériaux, D., Metens, T., et al. (2019). Dopamine transporter genotype modulates brain activity during a working memory task in children with ADHD. Res. Dev. Disabil. 92:103430. doi: 10.1016/j.ridd.2019.103430
Pinto, R., Asherson, P., Ilott, N., Cheung, C. H. M., and Kuntsi, J. (2016). Testing for the mediating role of endophenotypes using molecular genetic data in a twin study of ADHD traits. Am. J. Med. Genet. B Neuropsychiatr. Genet. Off. Publ. Int. Soc. Psychiatr. Genet. 171, 982–992. doi: 10.1002/ajmg.b.32463
Pliszka, S. R. (1998). Comorbidity of attention-deficit/hyperactivity disorder with psychiatric disorder: an overview. J. Clin. Psychiatry 59, (Suppl. 7), 50–58.
Pliszka, S. R. (2005). The neuropsychopharmacology of attention-deficit/hyperactivity disorder. Biol. Psychiatry 57, 1385–1390. doi: 10.1016/j.biopsych.2004.08.026
Pliszka, S. R., Maas, J. W., Javors, M. A., Rogeness, G. A., and Baker, J. (1994). Urinary catecholamines in attention-deficit hyperactivity disorder with and without comorbid anxiety. J. Am. Acad. Child Adolesc. Psychiatry 33, 1165–1173. doi: 10.1097/00004583-199410000-00012
Pogorelov, V. M., Rodriguiz, R. M., Insco, M. L., Caron, M. G., and Wetsel, W. C. (2005). Novelty seeking and stereotypic activation of behavior in mice with disruption of the Dat1 gene. Neuropsychopharmacol. Off. Publ. Am. Coll. Neuropsychopharmacol. 30, 1818–1831. doi: 10.1038/sj.npp.1300724
Polanczyk, G. V., Salum, G. A., Sugaya, L. S., Caye, A., and Rohde, L. A. (2015). Annual research review: a meta-analysis of the worldwide prevalence of mental disorders in children and adolescents. J. Child Psychol. Psychiatry. 56, 345–365. doi: 10.1111/jcpp.12381
Powell, S. B., Paulus, M. P., Hartman, D. S., Godel, T., and Geyer, M. A. (2003). RO-10-5824 is a selective dopamine D4 receptor agonist that increases novel object exploration in C57 mice. Neuropharmacology 44, 473–481. doi: 10.1016/s0028-3908(02)00412-4
Pratelli, M., and Pasqualetti, M. (2019). Serotonergic neurotransmission manipulation for the understanding of brain development and function: learning from Tph2 genetic models. Biochimie 161, 3–14. doi: 10.1016/j.biochi.2018.11.016
Puentes-Rozo, P. J., Acosta-López, J. E., Cervantes-Henríquez, M. L., Martínez-Banfi, M. L., Mejia-Segura, E., Sánchez-Rojas, M., et al. (2019). Genetic variation underpinning ADHD risk in a caribbean community. Cells 8:907. doi: 10.3390/cells8080907
Puig, M. V., and Gulledge, A. T. (2011). Serotonin and prefrontal cortex function: neurons, networks, and circuits. Mol. Neurobiol. 44, 449–464. doi: 10.1007/s12035-011-8214-0
Qian, A., Tao, J., Wang, X., Liu, H., Ji, L., Yang, C., et al. (2018a). Effects of the 2-repeat allele of the DRD4 gene on neural networks associated with the prefrontal cortex in children with ADHD. Front. Hum. Neurosci. 12:279. doi: 10.3389/fnhum.2018.00279
Qian, A., Wang, X., Liu, H., Tao, J., Zhou, J., Ye, Q., et al. (2018b). Dopamine D4 receptor gene associated with the frontal-striatal-cerebellar loop in children with ADHD: a resting-state fMRI study. Neurosci. Bull. 34, 497–506. doi: 10.1007/s12264-018-0217-7
Qin, L., Liu, W., Ma, K., Wei, J., Zhong, P., Cho, K., et al. (2016). The ADHD-linked human dopamine D4 receptor variant D4.7 induces over-suppression of NMDA receptor function in prefrontal cortex. Neurobiol. Dis. 95, 194–203. doi: 10.1016/j.nbd.2016.07.024
Quist, J. F., Barr, C. L., Schachar, R., Roberts, W., Malone, M., Tannock, R., et al. (2000). Evidence for the serotonin HTR2A receptor gene as a susceptibility factor in attention deficit hyperactivity disorder (ADHD). Mol. Psychiatry 5, 537–541. doi: 10.1038/sj.mp.4000779
Ranganath, A., and Jacob, S. N. (2016). Doping the mind: dopaminergic modulation of prefrontal cortical cognition. Neurosci. Rev. J. Bringing Neurobiol. Neurol. Psychiatry 22, 593–603. doi: 10.1177/1073858415602850
Rapoport, J., Quinn, P., Scribanu, N., and Murphy, D. L. (1974). Platelet serotonin of hyperactive school age boys. Br. J. Psychiatry 125, 138–140. doi: 10.1192/bjp.125.2.138
Reddy, D. S. (2013). Current pharmacotherapy of attention deficit hyperactivity disorder. Drugs Today 49, 647–665. doi: 10.1358/dot.2013.49.10.2008996
Regan, S. L., Hufgard, J. R., Pitzer, E. M., Sugimoto, C., Hu, Y.-C., Williams, M. T., et al. (2019). Knockout of latrophilin-3 in Sprague-Dawley rats causes hyperactivity, hyper-reactivity, under-response to amphetamine, and disrupted dopamine markers. Neurobiol. Dis. 130:104494. doi: 10.1016/j.nbd.2019.104494
Reinwald, J. R., Gass, N., Mallien, A. S., Sartorius, A., Becker, R., Sack, M., et al. (2022). Dopamine transporter silencing in the rat: systems-level alterations in striato-cerebellar and prefrontal-midbrain circuits. Mol. Psychiatry 27, 2329–2339. doi: 10.1038/s41380-022-01471-4
Ribasés, M., Ramos-Quiroga, J. A., Hervás, A., Sánchez-Mora, C., Bosch, R., Bielsa, A., et al. (2012). Candidate system analysis in ADHD: evaluation of nine genes involved in dopaminergic neurotransmission identifies association with DRD1. World J. Biol. Psychiatry Off. J. World Fed. Soc. Biol. Psychiatry 13, 281–292. doi: 10.3109/15622975.2011.584905
Richardson, J. R., Taylor, M. M., Shalat, S. L., Guillot, T. S. III, Caudle, W. M., Hossain, M. M., et al. (2015). Developmental pesticide exposure reproduces features of attention deficit hyperactivity disorder. FASEB J. Off. Publ. Fed. Am. Soc. Exp. Biol. 29, 1960–1972. doi: 10.1096/fj.14-260901
Rivera, A., Alberti, I., Martín, A. B., Narváez, J. A., de la Calle, A., and Moratalla, R. (2002). Molecular phenotype of rat striatal neurons expressing the dopamine D5 receptor subtype. Eur. J. Neurosci. 16, 2049–2058. doi: 10.1046/j.1460-9568.2002.02280.x
Rivera, A., Trías, S., Peñafiel, A., Angel Narváez, J., Díaz-Cabiale, Z., Moratalla, R., et al. (2003). Expression of D4 dopamine receptors in striatonigral and striatopallidal neurons in the rat striatum. Brain Res. 989, 35–41. doi: 10.1016/s0006-8993(03)03328-6
Rizo, J., and Südhof, T. C. (2012). The membrane fusion enigma: SNAREs, Sec1/Munc18 proteins, and their accomplices–guilty as charged? Annu. Rev. Cell Dev. Biol. 28, 279–308. doi: 10.1146/annurev-cellbio-101011-155818
Rizzo, R., Gulisano, M., Calì, P. V., and Curatolo, P. (2010). ADHD and epilepsy in children with Tourette syndrome: a triple comorbidity? Acta Paediatr. 99, 1894–1896. doi: 10.1111/j.1651-2227.2010.01951.x
Robbins, J., Wakakuwa, K., and Ikeda, H. (1988). Noradrenaline action on cat retinal ganglion cells is mediated by dopamine (D2) receptors. Brain Res. 438, 52–60. doi: 10.1016/0006-8993(88)91322-4
Robertson, G. S., and Staines, W. A. (1994). D1 dopamine receptor agonist-induced Fos-like immunoreactivity occurs in basal forebrain and mesopontine tegmentum cholinergic neurons and striatal neurons immunoreactive for neuropeptide Y. Neuroscience 59, 375–387. doi: 10.1016/0306-4522(94)90603-3
Rodriguiz, R. M., Chu, R., Caron, M. G., and Wetsel, W. C. (2004). Aberrant responses in social interaction of dopamine transporter knockout mice. Behav. Brain Res. 148, 185–198. doi: 10.1016/s0166-4328(03)00187-6
Rogeness, G. A., Maas, J. W., Javors, M. A., Macedo, C. A., Fischer, C., and Harris, W. R. (1989). Attention deficit disorder symptoms and urine catecholamines. Psychiatry Res. 27, 241–251. doi: 10.1016/0165-1781(89)90140-6
Rokem, A., Landau, A. N., Prinzmetal, W., Wallace, D. L., Silver, M. A., and D’Esposito, M. (2012). Modulation of inhibition of return by the dopamine D2 receptor agonist bromocriptine depends on individual DAT1 genotype. Cereb. Cortex 22, 1133–1138. doi: 10.1093/cercor/bhr185
Rommelse, N. N. J., Altink, M. E., Fliers, E. A., Martin, N. C., Buschgens, C. J. M., Hartman, C. A., et al. (2009). Comorbid problems in ADHD: degree of association, shared endophenotypes, and formation of distinct subtypes. Implications for a future DSM. J. Abnorm. Child Psychol. 37, 793–804. doi: 10.1007/s10802-009-9312-6
Rowland, A. S., Skipper, B. J., Rabiner, D. L., Qeadan, F., Campbell, R. A., Naftel, A. J., et al. (2018). Attention-deficit/hyperactivity disorder (ADHD): interaction between socioeconomic status and parental history of ADHD determines prevalence. J. Child Psychol. Psychiatry. 59, 213–222. doi: 10.1111/jcpp.12775
Russell, V. A. (2011). Overview of animal models of attention deficit hyperactivity disorder (ADHD). Curr. Protoc. Neurosci. 9:935. doi: 10.1002/0471142301.ns0935s54
San Mauro Martín, I., Blumenfeld Olivares, J. A., Garicano Vilar, E., Echeverry López, M., García Bernat, M., Quevedo Santos, Y., et al. (2018). Nutritional and environmental factors in attention-deficit hyperactivity disorder (ADHD): a cross-sectional study. Nutr. Neurosci. 21, 641–647. doi: 10.1080/1028415X.2017.1331952
Sánchez-Mora, C., Ramos-Quiroga, J.-A., Garcia-Martínez, I., Fernàndez-Castillo, N., Bosch, R., Richarte, V., et al. (2013). Evaluation of single nucleotide polymorphisms in the miR-183-96-182 cluster in adulthood attention-deficit and hyperactivity disorder (ADHD) and substance use disorders (SUDs). Eur. Neuropsychopharmacol. J. Eur. Coll. Neuropsychopharmacol. 23, 1463–1473. doi: 10.1016/j.euroneuro.2013.07.002
Sassone-Corsi, P. (2012). The cyclic AMP pathway. Cold Spring Harb. Perspect. Biol. 4:a011148. doi: 10.1101/cshperspect.a011148
Satoh, H., Suzuki, H., and Saitow, F. (2018). Downregulation of dopamine D1-like receptor pathways of GABAergic interneurons in the anterior cingulate cortex of spontaneously hypertensive rats. Neuroscience 394, 267–285. doi: 10.1016/j.neuroscience.2018.10.039
Saunders, A., Oldenburg, I. A., Berezovskii, V. K., Johnson, C. A., Kingery, N. D., Elliott, H. L., et al. (2015). A direct GABAergic output from the basal ganglia to frontal cortex. Nature 521, 85–89. doi: 10.1038/nature14179
Schmitz, M., Denardin, D., Laufer Silva, T., Pianca, T., Hutz, M. H., Faraone, S., et al. (2006). Smoking during pregnancy and attention-deficit/hyperactivity disorder, predominantly inattentive type: a case-control study. J. Am. Acad. Child Adolesc. Psychiatry 45, 1338–1345. doi: 10.1097/S0890-8567(09)61916-X
Seixas, M., Weiss, M., and Müller, U. (2012). Systematic review of national and international guidelines on attention-deficit hyperactivity disorder. J. Psychopharmacol. 26, 753–765. doi: 10.1177/0269881111412095
Serretti, A., Drago, A., and De Ronchi, D. (2007). HTR2A gene variants and psychiatric disorders: a review of current literature and selection of SNPs for future studies. Curr. Med. Chem. 14, 2053–2069. doi: 10.2174/092986707781368450
Šerý, O., Paclt, I., Drtílková, I., Theiner, P., Kopečková, M., Zvolský, P., et al. (2015). A 40-bp VNTR polymorphism in the 3’-untranslated region of DAT1/SLC6A3 is associated with ADHD but not with alcoholism. Behav. Brain Funct. 11:21. doi: 10.1186/s12993-015-0066-8
Sever, Y., Ashkenazi, A., Tyano, S., and Weizman, A. (1997). Iron treatment in children with attention deficit hyperactivity disorder. A preliminary report. Neuropsychobiology 35, 178–180. doi: 10.1159/000119341
Shahin, O., Meguid, N. A., Raafat, O., Dawood, R. M., Doss, M., Bader El Din, N. G., et al. (2015). Polymorphism in variable number of tandem repeats of dopamine d4 gene is a genetic risk factor in attention deficit hyperactive egyptian children: pilot study. Biomark. Insights 10, 33–38. doi: 10.4137/BMI.S18519
Shang, C.-Y., Gau, S. S.-F., Liu, C.-M., and Hwu, H.-G. (2011). Association between the dopamine transporter gene and the inattentive subtype of attention deficit hyperactivity disorder in Taiwan. Prog. Neuropsychopharmacol. Biol. Psychiatry 35, 421–428. doi: 10.1016/j.pnpbp.2010.08.016
Shang, C.-Y., Lin, H.-Y., and Gau, S. S.-F. (2021). The norepinephrine transporter gene modulates intrinsic brain activity, visual memory, and visual attention in children with attention-deficit/hyperactivity disorder. Mol. Psychiatry 26, 4026–4035. doi: 10.1038/s41380-019-0545-7
Sharma, A., and Couture, J. (2014). A review of the pathophysiology, etiology, and treatment of attention-deficit hyperactivity disorder (ADHD). Ann. Pharmacother. 48, 209–225. doi: 10.1177/1060028013510699
Sheehan, K., Hawi, Z., Gill, M., and Kent, L. (2007). No association between TPH2 gene polymorphisms and ADHD in a UK sample. Neurosci. Lett. 412, 105–107. doi: 10.1016/j.neulet.2006.10.043
Sheehan, K., Lowe, N., Kirley, A., Mullins, C., Fitzgerald, M., Gill, M., et al. (2005). Tryptophan hydroxylase 2 (TPH2) gene variants associated with ADHD. Mol. Psychiatry 10, 944–949. doi: 10.1038/sj.mp.4001698
Shim, S.-H., Hwangbo, Y., Kwon, Y.-J., Jeong, H.-Y., Lee, B.-H., Hwang, J.-A., et al. (2010). A case-control association study of serotonin 1A receptor gene and tryptophan hydroxylase 2 gene in attention deficit hyperactivity disorder. Prog. Neuropsychopharmacol. Biol. Psychiatry 34, 974–979. doi: 10.1016/j.pnpbp.2010.05.006
Sidoryk-Wegrzynowicz, M. (2014). Impairment of glutamine/glutamate-γ-aminobutyric acid cycle in manganese toxicity in the central nervous system. Folia Neuropathol. 52, 377–382. doi: 10.5114/fn.2014.47838
Silveri, M. M., Sneider, J. T., Crowley, D. J., Covell, M. J., Acharya, D., Rosso, I. M., et al. (2013). Frontal lobe γ-aminobutyric acid levels during adolescence: associations with impulsivity and response inhibition. Biol. Psychiatry 74, 296–304. doi: 10.1016/j.biopsych.2013.01.033
Sinn, N., and Bryan, J. (2007). Effect of supplementation with polyunsaturated fatty acids and micronutrients on learning and behavior problems associated with child ADHD. J. Dev. Behav. Pediatr. 28, 82–91. doi: 10.1097/01.DBP.0000267558.88457.a5
Smalley, S. L. (1997). Genetic influences in childhood-onset psychiatric disorders: autism and attention-deficit/hyperactivity disorder. Am. J. Hum. Genet. 60, 1276–1282. doi: 10.1086/515485
Snyder, S. H. (2011). What dopamine does in the brain. Proc. Natl. Acad. Sci. U.S.A. 108, 18869–18871. doi: 10.1073/pnas.1114346108
Spencer, T. J., Biederman, J., Wilens, T. E., and Faraone, S. V. (2002). Overview and neurobiology of attention-deficit/hyperactivity disorder. J. Clin. Psychiatry 63, (Suppl. 1), 3–9.
Srivastav, S., Walitza, S., and Grünblatt, E. (2018). Emerging role of miRNA in attention deficit hyperactivity disorder: a systematic review. Atten. Defic. Hyperact. Disord. 10, 49–63. doi: 10.1007/s12402-017-0232-y
Suh, Y. H., Chang, K., and Roche, K. W. (2018). Metabotropic glutamate receptor trafficking. Mol. Cell. Neurosci. 91, 10–24. doi: 10.1016/j.mcn.2018.03.014
Sun, H., Chen, Y., Huang, Q., Lui, S., Huang, X., Shi, Y., et al. (2018). Psychoradiologic utility of MR imaging for diagnosis of attention deficit hyperactivity disorder: a radiomics analysis. Radiology 287, 620–630. doi: 10.1148/radiol.2017170226
Tabatabaei, S. M., Amiri, S., Faghfouri, S., Noorazar, S. G., AbdollahiFakhim, S., and Fakhari, A. (2017). DRD4 gene polymorphisms as a risk factor for children with attention deficit hyperactivity disorder in iranian population. Int. Sch. Res. Not. 2017:2494537. doi: 10.1155/2017/2494537
Tang, Y., Buxbaum, S. G., Waldman, I., Anderson, G. M., Zabetian, C. P., Köhnke, M. D., et al. (2006). A single nucleotide polymorphism at DBH, possibly associated with attention-deficit/hyperactivity disorder, associates with lower plasma dopamine beta-hydroxylase activity and is in linkage disequilibrium with two putative functional single nucleotide po. Biol. Psychiatry 60, 1034–1038. doi: 10.1016/j.biopsych.2006.02.017
Taniura, H., Sanada, N., Kuramoto, N., and Yoneda, Y. (2006). A metabotropic glutamate receptor family gene in Dictyostelium discoideum. J. Biol. Chem. 281, 12336–12343. doi: 10.1074/jbc.M512723200
Tarver, J., Daley, D., and Sayal, K. (2014). Attention-deficit hyperactivity disorder (ADHD): an updated review of the essential facts. Child Care Health Dev. 40, 762–774. doi: 10.1111/cch.12139
Thomas, R., Sanders, S., Doust, J., Beller, E., and Glasziou, P. (2015). Prevalence of attention-deficit/hyperactivity disorder: a systematic review and meta-analysis. Pediatrics 135, e994–e1001. doi: 10.1542/peds.2014-3482
Thomas, T. C., Grandy, D. K., Gerhardt, G. A., and Glaser, P. E. A. (2009). Decreased dopamine D4 receptor expression increases extracellular glutamate and alters its regulation in mouse striatum. Neuropsychopharmacol. Off. Publ. Am. Coll. Neuropsychopharmacol. 34, 436–445. doi: 10.1038/npp.2008.74
Thursina, C., Nurputra, D. K., Harahap, I. S. K., Harahap, N. I. F., Sa’adah, N., Wibowo, S., et al. (2020). Determining the association between polymorphisms of the DAT1 and DRD4 genes with attention deficit hyperactivity disorder in children from Java Island. Neurol. Int. 12:8292. doi: 10.4081/ni.2020.8292
Tippairote, T., Temviriyanukul, P., Benjapong, W., and Trachootham, D. (2017). Hair Zinc and severity of symptoms are increased in children with attention deficit and hyperactivity disorder: a hair multi-element profile study. Biol. Trace Elem. Res. 179, 185–194. doi: 10.1007/s12011-017-0978-2
Tobaiqy, M., Stewart, D., Helms, P. J., Williams, J., Crum, J., Steer, C., et al. (2011). Parental reporting of adverse drug reactions associated with attention-deficit hyperactivity disorder (ADHD) medications in children attending specialist paediatric clinics in the UK. Drug Saf. 34, 211–219. doi: 10.2165/11586050-000000000-00000
Todd, R. D., Lobos, E. A., Sun, L.-W., and Neuman, R. J. (2003). Mutational analysis of the nicotinic acetylcholine receptor alpha 4 subunit gene in attention deficit/hyperactivity disorder: evidence for association of an intronic polymorphism with attention problems. Mol. Psychiatry 8, 103–108. doi: 10.1038/sj.mp.4001257
Tokko, T., Miškinyte, G., Eensoo, D., and Harro, J. (2022). Driving risks of young drivers with symptoms of attention deficit hyperactivity disorder: association with the dopamine transporter gene VNTR polymorphism. Nord. J. Psychiatry [Epub ahead of print]. doi: 10.1080/08039488.2022.2032330
Tseng, P.-T., Cheng, Y.-S., Yen, C.-F., Chen, Y.-W., Stubbs, B., Whiteley, P., et al. (2018). Peripheral iron levels in children with attention-deficit hyperactivity disorder: a systematic review and meta-analysis. Sci. Rep. 8:788. doi: 10.1038/s41598-017-19096-x
Undieh, A. S. (2010). Pharmacology of signaling induced by dopamine D(1)-like receptor activation. Pharmacol. Ther. 128, 37–60. doi: 10.1016/j.pharmthera.2010.05.003
Usiello, A., Baik, J. H., Rougé-Pont, F., Picetti, R., Dierich, A., LeMeur, M., et al. (2000). Distinct functions of the two isoforms of dopamine D2 receptors. Nature 408, 199–203. doi: 10.1038/35041572
Valbonesi, S., Magri, C., Traversa, M., Faraone, S. V., Cattaneo, A., Milanesi, E., et al. (2015). Copy number variants in attention-deficit hyperactive disorder: identification of the 15q13 deletion and its functional role. Psychiatr. Genet. 25, 59–70. doi: 10.1097/YPG.0000000000000056
Verlaet, A. A. J., Noriega, D. B., Hermans, N., and Savelkoul, H. F. J. (2014). Nutrition, immunological mechanisms and dietary immunomodulation in ADHD. Eur. Child Adolesc. Psychiatry 23, 519–529. doi: 10.1007/s00787-014-0522-2
Viggiano, D., Ruocco, L. A., Arcieri, S., and Sadile, A. G. (2004). Involvement of norepinephrine in the control of activity and attentive processes in animal models of attention deficit hyperactivity disorder. Neural Plast. 11, 133–149. doi: 10.1155/NP.2004.133
Villagomez, A., and Ramtekkar, U. (2014). Iron, Magnesium, Vitamin D, and Zinc deficiencies in children presenting with symptoms of attention-deficit/hyperactivity disorder. Child 1, 261–279. doi: 10.3390/children1030261
Waider, J., Araragi, N., Gutknecht, L., and Lesch, K.-P. (2011). Tryptophan hydroxylase-2 (TPH2) in disorders of cognitive control and emotion regulation: a perspective. Psychoneuroendocrinology 36, 393–405. doi: 10.1016/j.psyneuen.2010.12.012
Walitza, S., Renner, T. J., Dempfle, A., Konrad, K., Wewetzer, C., Halbach, A., et al. (2005). Transmission disequilibrium of polymorphic variants in the tryptophan hydroxylase-2 gene in attention-deficit/hyperactivity disorder. Mol. Psychiatry 10, 1126–1132. doi: 10.1038/sj.mp.4001734
Wallis, D., Arcos-Burgos, M., Jain, M., Castellanos, F. X., Palacio, J. D., Pineda, D., et al. (2009). Polymorphisms in the neural nicotinic acetylcholine receptor α4 subunit (CHRNA4) are associated with ADHD in a genetic isolate. Atten. Defic. Hyperact. Disord. 1, 19–24. doi: 10.1007/s12402-009-0003-5
Wallis, D., Hill, D. S., Mendez, I. A., Abbott, L. C., Finnell, R. H., Wellman, P. J., et al. (2012). Initial characterization of mice null for Lphn3, a gene implicated in ADHD and addiction. Brain Res. 1463, 85–92. doi: 10.1016/j.brainres.2012.04.053
Wang, H., and Pickel, V. M. (2002). Dopamine D2 receptors are present in prefrontal cortical afferents and their targets in patches of the rat caudate-putamen nucleus. J. Comp. Neurol. 442, 392–404. doi: 10.1002/cne.10086
Wang, Y., Hu, D., Chen, W., Xue, H., and Du, Y. (2019). Prenatal tobacco exposure modulated the association of genetic variants with diagnosed ADHD and its symptom domain in children: a community based case-control study. Sci. Rep. 9:4274. doi: 10.1038/s41598-019-40850-w
Wang, Y., Huang, L., Zhang, L., Qu, Y., and Mu, D. (2017). Iron status in attention-deficit/hyperactivity disorder: a systematic review and meta-analysis. PLoS One 12:e0169145. doi: 10.1371/journal.pone.0169145
Wang, Y., Wang, T., Du, Y., Hu, D., Zhang, Y., Li, H., et al. (2021). Polygenic risk of genes involved in the catecholamine and serotonin pathways for ADHD in children. Neurosci. Lett. 760:136086. doi: 10.1016/j.neulet.2021.136086
Weiß, A. L., Meijer, M., Budeus, B., Pauper, M., Hakobjan, M., Groothuismink, J., et al. (2021). DNA methylation associated with persistent ADHD suggests TARBP1 as novel candidate. Neuropharmacology 184:108370. doi: 10.1016/j.neuropharm.2020.108370
Wilens, T. E. (2008). Effects of methylphenidate on the catecholaminergic system in attention-deficit/hyperactivity disorder. J. Clin. Psychopharmacol. 28, S46–S53. doi: 10.1097/JCP.0b013e318173312f
Williams, N. M., Franke, B., Mick, E., Anney, R. J. L., Freitag, C. M., Gill, M., et al. (2012). Genome-wide analysis of copy number variants in attention deficit hyperactivity disorder: the role of rare variants and duplications at 15q13.3. Am. J. Psychiatry 169, 195–204. doi: 10.1176/appi.ajp.2011.11060822
Williams, N. M., Zaharieva, I., Martin, A., Langley, K., Mantripragada, K., Fossdal, R., et al. (2010). Rare chromosomal deletions and duplications in attention-deficit hyperactivity disorder: a genome-wide analysis. Lancet 376, 1401–1408. doi: 10.1016/S0140-6736(10)61109-9
Wolraich, M. L., Hagan, J. F. J., Allan, C., Chan, E., Davison, D., Earls, M., et al. (2019). Clinical practice guideline for the diagnosis, evaluation, and treatment of attention-deficit/hyperactivity disorder in children and adolescents. Pediatrics 144:e20192528. doi: 10.1542/peds.2019-2528
Wu, L., Zhao, Q., Zhu, X., Peng, M., Jia, C., Wu, W., et al. (2010). A novel function of microRNA let-7d in regulation of galectin-3 expression in attention deficit hyperactivity disorder rat brain. Brain Pathol. 20, 1042–1054. doi: 10.1111/j.1750-3639.2010.00410.x
Wu, L. H., Cheng, W., Yu, M., He, B. M., Sun, H., Chen, Q., et al. (2017). Nr3C1-Bhlhb2 axis dysregulation is involved in the development of attention deficit hyperactivity. Mol. Neurobiol. 54, 1196–1212. doi: 10.1007/s12035-015-9679-z
Wu, L. H., Peng, M., Yu, M., Zhao, Q. L., Li, C., Jin, Y. T., et al. (2015). Circulating MicroRNA Let-7d in attention-deficit/hyperactivity disorder. Neuromolecular Med. 17, 137–146. doi: 10.1007/s12017-015-8345-y
Xi, Z.-X., and Gardner, E. L. (2007). Pharmacological actions of NGB 2904, a selective dopamine D3 receptor antagonist, in animal models of drug addiction. CNS Drug Rev. 13, 240–259. doi: 10.1111/j.1527-3458.2007.00013.x
Xu, J., Zhu, Y., Contractor, A., and Heinemann, S. F. (2009). mGluR5 has a critical role in inhibitory learning. J. Neurosci. 29, 3676–3684. doi: 10.1523/JNEUROSCI.5716-08.2009
Xu, Q., Ou, J., Zhang, Q., Tang, R., Wang, J., Hong, Q., et al. (2019). Effects of aberrant miR-384-5p expression on learning and memory in a rat model of attention deficit hyperactivity disorder. Front. Neurol. 10:1414. doi: 10.3389/fneur.2019.01414
Yamada, M. K., Nakanishi, K., Ohba, S., Nakamura, T., Ikegaya, Y., Nishiyama, N., et al. (2002). Brain-derived neurotrophic factor promotes the maturation of GABAergic mechanisms in cultured hippocampal neurons. J. Neurosci. 22, 7580–7585. doi: 10.1523/JNEUROSCI.22-17-07580.2002
Yang, L., Neale, B. M., Liu, L., Lee, S. H., Wray, N. R., Ji, N., et al. (2013). Polygenic transmission and complex neuro developmental network for attention deficit hyperactivity disorder: genome-wide association study of both common and rare variants. Am. J. Med. Genet. Part B, Neuropsychiatr. Genet. Off. Publ. Int. Soc. Psychiatr. Genet. 162B, 419–430. doi: 10.1002/ajmg.b.32169
Yoshimasu, K., Kiyohara, C., Takemura, S., and Nakai, K. (2014). A meta-analysis of the evidence on the impact of prenatal and early infancy exposures to mercury on autism and attention deficit/hyperactivity disorder in the childhood. Neurotoxicology 44, 121–131. doi: 10.1016/j.neuro.2014.06.007
Youdim, M. B., Ben-Shachar, D., Ashkenazi, R., and Yehuda, S. (1983). Brain iron and dopamine receptor function. Adv. Biochem. Psychopharmacol. 37, 309–321.
Yuan, D., Zhang, M., Huang, Y., Wang, X., Jiao, J., and Huang, Y. (2021). Noradrenergic genes polymorphisms and response to methylphenidate in children with ADHD: a systematic review and meta-analysis. Medicine 100:e27858. doi: 10.1097/MD.0000000000027858
Zarrei, M., Burton, C. L., Engchuan, W., Young, E. J., Higginbotham, E. J., MacDonald, J. R., et al. (2019). A large data resource of genomic copy number variation across neurodevelopmental disorders. NPJ Genomic Med. 4:26. doi: 10.1038/s41525-019-0098-3
Zhang, H., Yang, B., Peng, G., Zhang, L., and Fang, D. (2021a). Effects of the DRD4 -521 C/T SNP on local neural activity and functional connectivity in children with ADHD. Front. Psychiatry 12:785464. doi: 10.3389/fpsyt.2021.785464
Zhang, Q., Chen, X., Li, S., Yao, T., and Wu, J. (2021b). Association between the group III metabotropic glutamate receptor gene polymorphisms and attention-deficit/hyperactivity disorder and functional exploration of risk loci. J. Psychiatr. Res. 132, 65–71. doi: 10.1016/j.jpsychires.2020.09.035
Zhang, Q., Huang, X., Chen, X.-Z., Li, S.-Y.-W., Yao, T., and Wu, J. (2021c). Association of gene variations in ionotropic glutamate receptor and attention-deficit/hyperactivity disorder in the chinese population: a two-stage case-control study. J. Atten. Disord. 25, 1362–1373. doi: 10.1177/1087054720905089
Zhang, K., Davids, E., Tarazi, F. I., and Baldessarini, R. J. (2002). Effects of dopamine D4 receptor-selective antagonists on motor hyperactivity in rats with neonatal 6-hydroxydopamine lesions. Psychopharmacology 161, 100–106. doi: 10.1007/s00213-002-1018-1
Zhang, M., Zhang, D., Dai, J., Cao, Y., Xu, W., He, G., et al. (2020). Paternal nicotine exposure induces hyperactivity in next-generation via down-regulating the expression of DAT. Toxicology 431:152367. doi: 10.1016/j.tox.2020.152367
Zhou, M., Gresack, J., Cheng, J., Uryu, K., Brichta, L., Greengard, P., et al. (2020). CK1δ over-expressing mice display ADHD-like behaviors, frontostriatal neuronal abnormalities and altered expressions of ADHD-candidate genes. Mol. Psychiatry 25, 3322–3336. doi: 10.1038/s41380-018-0233-z
Zhu, M.-Y. (2018). Noradrenergic modulation on dopaminergic neurons. Neurotox. Res. 34, 848–859. doi: 10.1007/s12640-018-9889-z
Zuo, L., Saba, L., Lin, X., Tan, Y., Wang, K., Krystal, J. H., et al. (2015). Significant association between rare IPO11-HTR1A variants and attention deficit hyperactivity disorder in Caucasians. Am. J. Med. Genet. B Neuropsychiatr. Genet. Off. Publ. Int. Soc. Psychiatr. Genet. 168, 544–556. doi: 10.1002/ajmg.b.32329
Keywords: attention-deficit/hyperactive disorder, pathogenic pathways, environmental factors, genes, copy number variations, microRNAs (miRNAs)
Citation: Kessi M, Duan H, Xiong J, Chen B, He F, Yang L, Ma Y, Bamgbade OA, Peng J and Yin F (2022) Attention-deficit/hyperactive disorder updates. Front. Mol. Neurosci. 15:925049. doi: 10.3389/fnmol.2022.925049
Received: 21 April 2022; Accepted: 26 August 2022;
Published: 21 September 2022.
Edited by:
Michel J. Simonneau, École Normale Supérieure Paris-Saclay, FranceReviewed by:
Matias Morin, Centro de Investigación Biomédica en Red de Enfermedades Raras (CIBERER), SpainJitendra Kumar Sinha, GloNeuro Academy, India
Copyright © 2022 Kessi, Duan, Xiong, Chen, He, Yang, Ma, Bamgbade, Peng and Yin. This is an open-access article distributed under the terms of the Creative Commons Attribution License (CC BY). The use, distribution or reproduction in other forums is permitted, provided the original author(s) and the copyright owner(s) are credited and that the original publication in this journal is cited, in accordance with accepted academic practice. No use, distribution or reproduction is permitted which does not comply with these terms.
*Correspondence: Fei Yin, eWYyMzIzQGhvdG1haWwuY29t
†These authors have contributed equally to this work