- 1Department of Psychology, SUNY Old Westbury, Old Westbury, NY, United States
- 2SUNY Neuroscience Research Institute, SUNY Old Westbury, Old Westbury, NY, United States
Behavioral neuroscience tests such as the Light/Dark Test, the Open Field Test, the Elevated Plus Maze Test, and the Three Chamber Social Interaction Test have become both essential and widely used behavioral tests for transgenic and pre-clinical models for drug screening and testing. However, as fast as the field has evolved and the contemporaneous involvement of technology, little assessment of the literature has been done to ensure that these behavioral neuroscience tests that are crucial to pre-clinical testing have well-controlled ethological motivation by the use of lighting (i.e., Lux). In the present review paper, N = 420 manuscripts were examined from 2015 to 2019 as a sample set (i.e., n = ~20–22 publications per year) and it was found that only a meager n = 50 publications (i.e., 11.9% of the publications sampled) met the criteria for proper anxiogenic and anxiolytic Lux reported. These findings illustrate a serious concern that behavioral neuroscience papers are not being vetted properly at the journal review level and are being released into the literature and public domain making it difficult to assess the quality of the science being reported. This creates a real need for standardizing the use of Lux in all publications on behavioral neuroscience techniques within the field to ensure that contributions are meaningful, avoid unnecessary duplication, and ultimately would serve to create a more efficient process within the pre-clinical screening/testing for drugs that serve as anxiolytic compounds that would prove more useful than what prior decades of work have produced. It is suggested that improving the standardization of the use and reporting of Lux in behavioral neuroscience tests and the standardization of peer-review processes overseeing the proper documentation of these methodological approaches in manuscripts could serve to advance pre-clinical testing for effective anxiolytic drugs. This report serves to highlight this concern and proposes strategies to proactively remedy them as the field moves forward for decades to come.
Introduction
Over the last decade, there has been a rapid advancement of a more integrative and interdisciplinary neuroscience that has sought to explore structure-function relationships either through brain mapping, (neuro) imaging, establishing and refining the connectome, and/or using cross-species comparisons to better understand the uniqueness of the human brain that orchestrate behavioral outputs (Koch et al., 2010; Goulas et al., 2014; Miranda-Dominquez et al., 2014; Wang et al., 2014; Snyder and Bauer, 2019). Despite these exceptional advancements, prior to this recent boom in the big-technology and big-data revolution in the field of neuroscience, there remained a series of challenges faced by behavioral neuroscientists in trying to address longstanding issues in: relating behavioral observations back to molecular biological targets and exercising caution that molecular data cannot replace the unique adaptive behavioral processes of organisms in response to changing environments (Lederhendler and Schulkin, 2000), mining large datasets (Akil et al., 2011) and in particular bridging data between psychology, ethology, and neuroscience (Marshall, 2009; Gomez-Marin et al., 2014), with long-term goals in understanding the subtleties of social behaviors (Adolphs, 2010; Insel, 2010) that can be complex in nature (Cacioppo and Decety, 2011), and to remain grounded in our understanding of reinforcement learning, conditioning, and its neural correlates (Maia, 2009). What is more concerning is that these challenges remain decades later with little advancement and standardization in their use within the field to address such a critical problem.
This situation may be partially explained by many non-traditionally trained behaviorists engaging in basic behavioral neuroscience tests in an effort to characterize and/or determine traits of specific locomotor activities (i.e., through the use of the Open Field) and anxiety-like behaviors (i.e., through the use of the Elevated Plus Maze, Zero Maze, Light/Dark Box or Light/Dark Test, and the Three Chamber Social Interaction Test). This situation has resulted in an unfortunate reduction in the quality of behavioral neuroscience research (Bespalov and Steckler, 2018) and efforts by other groups to try to make behavioral tests they design more accessible through open-source applications are noteworthy (White et al., 2019). Notably, without ensuring both generalizability and reliability training through open-source efforts to standardize the field, one must seriously question the behavioral neuroscience datasets they review as their interpretations can be quite variable. There seems to be a tendency that as advancements in (bio) technology are made, it inadvertently creates a widening gap between re-establishing important links of behavioral bioassays in neuroscience (Brown and Bolivar, 2018). Thus, researchers in the field that employ behavioral tests to better describe neurobiological phenomena associated with a broad range of animal model systems and associated ecological contexts to diversify the translations of their work (Mathuru et al., 2020) should undergo more training in traditional behaviorism (Thompson, 1994; Moore, 2011) to prevent creating barriers in integrating behavioral and neural datasets (Thompson, 1994; Moore, 2002; Carobrez and Bertoglio, 2005; Ortu and Vaidya, 2016).
One of the basic tenets of the Open Field Test, the Elevated Plus Maze, the Zero Maze, the Light/Dark Test, or the Light/Dark Box, is the use of an aversive bright light to serve as an establishing operation to induce anxiogenic behaviors, whereas, in contrast, the Three Chamber Social Interaction Test uses dim lighting to increase socialization to induce anxiolytic behaviors. These ethologically derived concepts surrounding the intentional utilization of light as the motivational stimuli serve as the foundational basis by which all interpreted anxiety-like behaviors in rodents are reliably confirmed through pre-clinical behavioral neuroscience testing procedures. Thus, the rodent’s sensitivity to lighting (i.e., changes in Lux) can serve to set the floor and ceiling thresholds for anxiogenic responses in both typical wild type, transgenic, and/or other mutant rodent models engineered to exhibit face and construct valid social and other associated anxiety-like behavioral traits. However, despite cases where revisiting standards for testing anxiety-like behaviors for the Elevated Plus Maze over a 20-year period have been reported in the literature to increase future face, construct, and predictive validity within an ethological context (Ortu and Vaidya, 2016), it unfortunately, failed to include lighting controls in its 2-decade review of the experimentation. These observations led to the following question: How many publications for these behavioral neuroscience tests actually report the Lux values as their ethological stimulus controls for inducing anxiogenic and/or anxiolytic responses? This question is important to consider, especially if the behavioral neuroscience field continues to produce large amounts of publications without the standardization of Lux through clearly defined controlled lighting as the main experimental control to be the anxiogenic/anxiolytic stimulus to motivate rodents. Furthermore, when such standardization is established, it, in turn, increases the integrity of the science, the internal and external validity, and translational meaning of the work that has and continues to be published and the work forthcoming. Thus, the present study sought to determine what proportion of research studies actually report the lighting/lux values in their reports in an effort to revisit the standards for behavioral neuroscience testing of anxiety-like behaviors in rodents.
Method
Database Keyword Search
In order to determine the frequency of publications that used the Open Field Test, the Elevated Plus Maze, the Zero Maze, the Light/Dark Box, or the Light/Dark Test, and the Three Chamber Social Interaction Test, a keyword search for these exact terms were done using Elsevier’s Science Direct for the years 2009–2019 covering a decade of research published through this outlet. The Elsevier’s Science Direct Article Database was used as it had to most journal subscriptions (i.e., 4,620 journals) to cover a broad range to obtain a representative sample of the behavioral neuroscience tests understudy. The years were truncated not to go beyond 2019 to avoid any publication data being skewed due to the coronavirus (COVID-19). However, some papers in 2019 at the time of the search were either in press/forthcoming or published online ahead of print and their actual publication dates occurred in 2020. The total number of the articles returned that met the inclusion criteria were then used to evaluate the trends of the use of each test in the behavioral neuroscience field over the last decade.
Refined Search and Inclusion/Exclusionary Criteria
Next, for each of the types of behavioral tests of interest (i.e., Light/Dark Box, the Open Field, the Elevated Plus Maze, and the Three Chamber Social Interaction Test) from 2015 to 2019, covering the latter half of the decade (i.e., since there was a consistent upward trend in these latter years). Using this time-period, the first 22–23 publications from each year (i.e., 105 publications per behavioral test that were sampled; totaling N = 420 publications of which only n = 351 met the criteria (Kochenborger et al., 2014; Allah Yar et al., 2015; Amos-Kroohs et al., 2015; Banaskowski et al., 2015; Bentea et al., 2015; Bernard et al., 2015; Bertolus et al., 2015; Brown et al., 2015; Bruining et al., 2015; Casarrubea et al., 2015, 2016; Chao et al., 2015; Colla et al., 2015; Cox et al., 2015; Daher and Mattioli, 2015; Dutra-Tavares et al., 2015; Finlay et al., 2015; Fowler and Muma, 2015; Gamberini et al., 2015; Goes et al., 2015; Gray and Hughes, 2015; Haleem et al., 2015; Hill et al., 2015; Horii and Kawaguchi, 2015; Iqbal et al., 2015; Kalouda and Pitsikas, 2015; Kawasaki et al., 2015, 2017; Ketcha Wanda et al., 2015; Kigar et al., 2015; Kumar et al., 2015; Langley et al., 2015; Lawther et al., 2015; Lecorps and Féron, 2015; Lee J. et al., 2015; Lee K. M. et al., 2015; Listowska et al., 2015; Liu et al., 2015; Livingston-Thomas et al., 2015; Mascarenhas et al., 2015; Mcneilly et al., 2015; Nakamura A. et al., 2015; Nakamura K. et al., 2015; Noguerón-Merino et al., 2015; Nunes et al., 2015; Pereda et al., 2015; Quines et al., 2015; Rafati et al., 2015; Reilly et al., 2015; Reimer et al., 2015; Rilett et al., 2015; Saito and Brandão, 2015; Saitoh et al., 2015; Sauce et al., 2015; Schroeder et al., 2015; Skupio et al., 2015; Słupski and Rutkowska, 2015; Štefánik et al., 2015; Telonis and Margarity, 2015; Thompson et al., 2015; Tothova et al., 2015; Washington et al., 2015; Wensheng et al., 2015; Xu et al., 2015, 2019; Yang et al., 2015; Yoshimi et al., 2015; Yu et al., 2015; Zagorácz et al., 2015; Zhan, 2015; Zhang C. et al., 2015; Zhang H. et al., 2015; Zhang M. et al., 2015; Zhang W. et al., 2015; Zhou et al., 2015; Zoubovsky et al., 2015; Acevedo et al., 2016; Bashiri et al., 2016; Biagioni et al., 2016; Buffington et al., 2016; Christensen et al., 2016; Cipriano et al., 2016; Dagan et al., 2016; Daniel and Hughes, 2016; de La Tremblaye et al., 2016; Dhediya et al., 2016; Diaz et al., 2016; Dos Anjos et al., 2016; Duarte et al., 2016; Estork et al., 2016; Farajdokht et al., 2016; Fedotova et al., 2016; Fernandez et al., 2016; Ferri et al., 2016; Figueiredo et al., 2016; Girard et al., 2016; Gomes et al., 2016; Gomez et al., 2016; Hegde et al., 2016; Hicks K. et al., 2016; Hicks J. A. et al., 2016; Horsley et al., 2016; Hughes and Hancock, 2016; Kang et al., 2016; Kerr et al., 2016; Komaki et al., 2016; Kratsman et al., 2016; Kumar and Sharma, 2016a, b; Labots et al., 2016; Lamontagne et al., 2016; Lawson et al., 2016; Lecorps et al., 2016; Lee and Green, 2016; Lee et al., 2016, 2018; Li J. et al., 2016; Li K. et al., 2016; Martins-Júnior et al., 2016; Matthews et al., 2016; Miller et al., 2016; Miranda-Morales and Pautassi, 2016; Mlyniec et al., 2016; Näslund et al., 2016; O’Connor et al., 2016; Onaolapo et al., 2016; Palotai and Telegdy, 2016; Psyrdellis et al., 2016a, b; Rico et al., 2016; Rodrigues Tavares et al., 2016; Rogers et al., 2017; Rojas et al., 2016; Ryan et al., 2016; Sakurai et al., 2016; Salari et al., 2016; Saré et al., 2016; Schambra et al., 2016; Scheinert et al., 2016; Serafim et al., 2016; Socala and Wlaz, 2016; Stohn et al., 2016; Torres et al., 2016; Tran and Keele, 2016; Verpeut et al., 2016; Vogt et al., 2016; Wang et al., 2016a, b, 2018b; Wscieklica et al., 2016; Yau et al., 2016; Yeung et al., 2016; Zhang et al., 2016; Abdellatif et al., 2017; Akbar et al., 2017; Alkhlaif et al., 2017; Bagosi et al., 2017a, b; Bahi, 2017a, b; Bartolomé et al., 2017; Bassi et al., 2017; Benoit et al., 2017; Blankenship et al., 2017; Borbely et al., 2017; Burke and Trang, 2017; Cai et al., 2017; Cao et al., 2017; Casarrubea et al., 2017; Chandra Sekhar et al., 2017; Demir Özkay et al., 2017; Djordjevic et al., 2017; Domonkos et al., 2017; Donatti et al., 2017; Ebihara et al., 2017; Fernandes et al., 2017a, b; Garcia et al., 2017; Gatica et al., 2017; Gillette et al., 2017; Hansen et al., 2017; Henbid et al., 2017; Hsieh et al., 2017; Jiménez-Ferrer et al., 2017; Karkaba et al., 2017; Kawabe, 2017; Kędzierska et al., 2017; Khalil and Fendt, 2017; Kuniishi et al., 2017; Kyriakou et al., 2017; López-Cruz et al., 2017; Lee et al., 2017; Leković et al., 2017; Machado et al., 2017; Makinson et al., 2017; Malikowska and Sałat, 2017; Mazur et al., 2017; Mihara et al., 2017; Narasingam et al., 2017; Orfanidou et al., 2017; Provenzano et al., 2017; Rangel-Barajas et al., 2017; Reinhart et al., 2017; Salari and Amani, 2017; Sanguedo et al., 2017; Sanna et al., 2017; Santangelo et al., 2017; Santos et al., 2017; Scheich et al., 2017; Schindler et al., 2017; Shafia et al., 2017; Sirohi et al., 2017; Speight et al., 2017; Sprowles et al., 2017; Stoppel and Anderson, 2017; Taherichadorneshin et al., 2017; Toma et al., 2017; Uemura et al., 2017; van Den Boom et al., 2017; Vázquez-León et al., 2017, 2018; Wei et al., 2017; Wen et al., 2017; Wu et al., 2017; Yamamoto et al., 2017; Yeshurun et al., 2017; Zilkha et al., 2017; Zimcikova et al., 2017; Alves et al., 2018; Amodeo et al., 2018; Arakawa, 2018; Batinić et al., 2018; Bausch et al., 2018; Benekareddy et al., 2018; Bialuk et al., 2018; Blume et al., 2018; Bodden et al., 2018; Bonuti and Morato, 2018; Borland et al., 2018; Boyette-Davis et al., 2018; Cazuza et al., 2018; Crestani et al., 2018; Cui et al., 2018; Dastamooz et al., 2018; Donaire et al., 2018; Dong et al., 2018; Evans et al., 2018; Ferreira de Araújo et al., 2018; Funck et al., 2018; Garcia-Gutierrez et al., 2018; Goñi-Balentziaga et al., 2018; He et al., 2018; Heinla et al., 2018; Hirano et al., 2018; Holman et al., 2018; Holubová et al., 2018; Hughes and Hamilton, 2018; Jacobskind et al., 2018; Keenan et al., 2018; Khalil et al., 2018; Kosari-Nasab et al., 2018; Leung et al., 2018; López Rivilli et al., 2018; Macedo et al., 2018; Mahmoudi et al., 2018; Melo et al., 2018; Melo-Thomas et al., 2018; Morley-Fletcher et al., 2018; Morud et al., 2018; Namvarpour et al., 2018; Nie et al., 2018; Noworyta-Sokołowska et al., 2018; Okamoto et al., 2018; Perea-Rodriguez et al., 2018; Purvis et al., 2018; Rauhut and Curran-Rauhut, 2018; Robinson et al., 2018; Rojas-Carvajal et al., 2018; Roohi-Azizi et al., 2018; Saitoh et al., 2018; Screven and Dent, 2018; Sheth et al., 2018; Shimizu et al., 2018; Smith et al., 2018; Sobolewski et al., 2018; Sorregotti et al., 2018; Sparling et al., 2018; Struntz and Siegel, 2018; Subramaniam et al., 2018; Tarland and Brosda, 2018; Tavares et al., 2018; Upadhyay et al., 2018; Van Camp et al., 2018; Varghese et al., 2018; Walia et al., 2018, 2019a, b; Wang S. et al., 2018; Wang et al., 2018a, b; Wille-Bille et al., 2018; Zahra et al., 2018; Zhang and Yao, 2018; Zhou et al., 2018; Al-Harrasi et al., 2019; Arnold et al., 2019; Atigari et al., 2019; Bahi and Dreyer, 2019; Basaure et al., 2019; Borrow et al., 2019; Burns et al., 2019; Caliskan et al., 2019; Dempsey et al., 2019; Dixon and Hughes, 2019; Dougherty et al., 2019; Ebrahimi-Ghiri et al., 2019; Elhady et al., 2019; Estrada-Camarena et al., 2019; Faure et al., 2019; Fisch et al., 2019; Freels et al., 2019; Garbarino et al., 2019; García-Díaz et al., 2019; García-Ríos et al., 2019; Gubert and Hannan, 2019; Hatcher et al., 2019; Herbst et al., 2019; Hetzler et al., 2019; Jalilzad et al., 2019; Kosel et al., 2019; Kruse et al., 2019; Kumar et al., 2019; Laureano-Melo et al., 2019; Lee et al., 2019; Lin T. et al., 2019; Lin Y. et al., 2019; Liu et al., 2019; Lopes Andrade et al., 2019; Lovelock and Deak, 2019; Malikowska-Racia et al., 2019; Marks et al., 2019; Matsuo et al., 2019; Medawar et al., 2019; Miao et al., 2019; Miguel et al., 2019; Moreira et al., 2019; Morgan et al., 2019; Munshi et al., 2019; Nakazawa et al., 2019; Neuwirth et al., 2019b; Peleh et al., 2019; Peng et al., 2019; Queiroz et al., 2019; Samad et al., 2019; Sapozhnikova et al., 2019; Scholl et al., 2019; Suleymanova et al., 2019; Tartaglione et al., 2019; Tillmann and Wegener, 2019; Tillmann et al., 2019; Trofimiuk et al., 2019; Tsatsakis et al., 2019; Victoriano et al., 2019; Vieira et al., 2019; Wąsik et al., 2019; Wang G. et al., 2019; Wang L. et al., 2019; Wang H. et al., 2019; Winther et al., 2019; Xiao et al., 2019; Yang et al., 2019; Yuan et al., 2019; Zaccarelli-Magalhães et al., 2019; Zare et al., 2019; Zoeram et al., 2019; Bond et al., 2020; Ng et al., 2020) in which a full PDF was freely accessible were downloaded and their methods section were examined for clear reporting of the ethological lighting used (i.e., ~ ranging from 300 to 1,000 Lux to ensure to induce an anxiogenic response to light as an aversive stimulus for all tests but the Three Chamber Social Interaction Test). The Three Chamber Social Interaction Test requires a low-light stimulus in order to promote social behaviors in approaching other rodents as an anxiolytic stimulus (i.e., ~ ranging from 0 to 30 Lux to prevent freezing and other immobility behaviors that would otherwise interfere with testing). Data were included if the publication reported lighting measures, whereas if the publication did not, it was excluded (i.e., this also excluded any other work that previously cited other work). The number of remaining articles that met the criteria for these animal behavioral testing purposes were: the Light/Dark Box Test [n = 98 (Banaskowski et al., 2015; Bentea et al., 2015; Bertolus et al., 2015; Brown et al., 2015; Chao et al., 2015; Colla et al., 2015; Kalouda and Pitsikas, 2015; Lee K. M. et al., 2015; Lee et al., 2017; Liu et al., 2015; Livingston-Thomas et al., 2015; Pereda et al., 2015; Quines et al., 2015; Saitoh et al., 2015; Sauce et al., 2015; Skupio et al., 2015; Thompson et al., 2015; Tothova et al., 2015; Yu et al., 2015; Zhan, 2015; Zhang C. et al., 2015; Zhang et al., 2016; Acevedo et al., 2016; Christensen et al., 2016; Daniel and Hughes, 2016; Diaz et al., 2016; Farajdokht et al., 2016; Fedotova et al., 2016; Fernandez et al., 2016; Hicks J. A. et al., 2016; Hughes and Hancock, 2016; Labots et al., 2016; Li J. et al., 2016; Miranda-Morales and Pautassi, 2016; Mlyniec et al., 2016; Rojas et al., 2016; Salari et al., 2016; Scheinert et al., 2016; Socala and Wlaz, 2016; Stohn et al., 2016; Vogt et al., 2016; Abdellatif et al., 2017; Alkhlaif et al., 2017; Bahi, 2017b; Benoit et al., 2017; Borbely et al., 2017; Chandra Sekhar et al., 2017; Djordjevic et al., 2017; Domonkos et al., 2017; Khalil and Fendt, 2017; Makinson et al., 2017; Orfanidou et al., 2017; Rogers et al., 2017; Salari and Amani, 2017; Sanna et al., 2017; Scheich et al., 2017; Shafia et al., 2017; Sirohi et al., 2017; Toma et al., 2017; Wen et al., 2017; Alves et al., 2018; Amodeo et al., 2018; Cazuza et al., 2018; Dong et al., 2018; Ferreira de Araújo et al., 2018; Garcia-Gutierrez et al., 2018; Heinla et al., 2018; Hughes and Hamilton, 2018; Jacobskind et al., 2018; Keenan et al., 2018; Kosari-Nasab et al., 2018; Mahmoudi et al., 2018; Morley-Fletcher et al., 2018; Van Camp et al., 2018; Varghese et al., 2018; Walia et al., 2018, 2019a, b; Wille-Bille et al., 2018; Zhang and Yao, 2018; Zhou et al., 2018; Al-Harrasi et al., 2019; Bahi and Dreyer, 2019; Borrow et al., 2019; Dempsey et al., 2019; Dixon and Hughes, 2019; Freels et al., 2019; Laureano-Melo et al., 2019; Lovelock and Deak, 2019; Matsuo et al., 2019; Medawar et al., 2019; Morgan et al., 2019; Peng et al., 2019; Queiroz et al., 2019; Samad et al., 2019; Tillmann et al., 2019; Wang H. et al., 2019; Winther et al., 2019; Zaccarelli-Magalhães et al., 2019], the Open Field Test [n = 105 (Allah Yar et al., 2015; Amos-Kroohs et al., 2015; Colla et al., 2015; Dutra-Tavares et al., 2015; Fowler and Muma, 2015; Gray and Hughes, 2015; Haleem et al., 2015; Iqbal et al., 2015; Kalouda and Pitsikas, 2015; Kawasaki et al., 2015, 2017; Ketcha Wanda et al., 2015; Listowska et al., 2015; Liu et al., 2015, 2019; Nakamura A. et al., 2015; Nunes et al., 2015; Rilett et al., 2015; Thompson et al., 2015; Wensheng et al., 2015; Zagorácz et al., 2015; Biagioni et al., 2016; Dagan et al., 2016; Dos Anjos et al., 2016; Estork et al., 2016; Figueiredo et al., 2016; Girard et al., 2016; Gomez et al., 2016; Hicks K. et al., 2016; Horsley et al., 2016; Lecorps et al., 2016; Li K. et al., 2016; Martins-Júnior et al., 2016; Miller et al., 2016; Onaolapo et al., 2016; Psyrdellis et al., 2016a, b; Saré et al., 2016; Schambra et al., 2016; Tran and Keele, 2016; Wscieklica et al., 2016; Bahi, 2017b; Blankenship et al., 2017; Casarrubea et al., 2017; Donatti et al., 2017; Hansen et al., 2017; Kawabe, 2017; Khalil and Fendt, 2017; Kuniishi et al., 2017; Machado et al., 2017; Mazur et al., 2017; Rangel-Barajas et al., 2017; Reinhart et al., 2017; Sanguedo et al., 2017; Santangelo et al., 2017; Santos et al., 2017; Speight et al., 2017; Sprowles et al., 2017; Zimcikova et al., 2017; van Den Boom et al., 2017; Batinić et al., 2018; Blume et al., 2018; Bodden et al., 2018; Cui et al., 2018; Dastamooz et al., 2018; Evans et al., 2018; Holubová et al., 2018; Khalil et al., 2018; Melo-Thomas et al., 2018; Noworyta-Sokołowska et al., 2018; Perea-Rodriguez et al., 2018; Purvis et al., 2018; Rauhut and Curran-Rauhut, 2018; Robinson et al., 2018; Rojas-Carvajal et al., 2018; Roohi-Azizi et al., 2018; Saitoh et al., 2018; Struntz and Siegel, 2018; Wang et al., 2018a; Wang G. et al., 2019; Bonuti and Morato, 2018; Caliskan et al., 2019; Dougherty et al., 2019; Elhady et al., 2019; Fisch et al., 2019; Hetzler et al., 2019; Jalilzad et al., 2019; Kruse et al., 2019; Kumar et al., 2019; Lopes Andrade et al., 2019; Marks et al., 2019; Miguel et al., 2019; Neuwirth et al., 2019b; Sapozhnikova et al., 2019; Suleymanova et al., 2019; Trofimiuk et al., 2019; Tsatsakis et al., 2019; Yuan et al., 2019; Zare et al., 2019)], the Elevated Plus Maze [n = 99 (Kochenborger et al., 2014; Casarrubea et al., 2015, 2016; Colla et al., 2015; Daher and Mattioli, 2015; Gamberini et al., 2015; Goes et al., 2015; Hill et al., 2015; Horii and Kawaguchi, 2015; Ketcha Wanda et al., 2015; Lawther et al., 2015; Lecorps and Féron, 2015; Mascarenhas et al., 2015; Mcneilly et al., 2015; Noguerón-Merino et al., 2015; Rafati et al., 2015; Reimer et al., 2015; Słupski and Rutkowska, 2015; Saito and Brandão, 2015; Telonis and Margarity, 2015; Yang et al., 2015; Zhou et al., 2015; Bashiri et al., 2016; Cipriano et al., 2016; Dhediya et al., 2016; Duarte et al., 2016; Estork et al., 2016; Gomes et al., 2016; Kang et al., 2016; Komaki et al., 2016; Lamontagne et al., 2016; Lecorps et al., 2016; Li K. et al., 2016; Näslund et al., 2016; O’Connor et al., 2016; Palotai and Telegdy, 2016; Rico et al., 2016; Rodrigues Tavares et al., 2016; Serafim et al., 2016; Yeung et al., 2016; Akbar et al., 2017; Bartolomé et al., 2017; Bassi et al., 2017; Demir Özkay et al., 2017; Donatti et al., 2017; Fernandes et al., 2017a, b; Gatica et al., 2017; Gillette et al., 2017; Jiménez-Ferrer et al., 2017; Kędzierska et al., 2017; Kyriakou et al., 2017; Leković et al., 2017; Malikowska and Sałat, 2017; Mazur et al., 2017; Narasingam et al., 2017; Schindler et al., 2017; Taherichadorneshin et al., 2017; Vázquez-León et al., 2017, 2018; Bialuk et al., 2018; Boyette-Davis et al., 2018; Cazuza et al., 2018; Donaire et al., 2018; Funck et al., 2018; Hirano et al., 2018; López Rivilli et al., 2018; Macedo et al., 2018; Melo et al., 2018; Morud et al., 2018; Nie et al., 2018; Shimizu et al., 2018; Sorregotti et al., 2018; Sparling et al., 2018; Tavares et al., 2018; Upadhyay et al., 2018; Walia et al., 2018, 2019a; Wang S. et al., 2018; Arnold et al., 2019; Atigari et al., 2019; Caliskan et al., 2019; Ebrahimi-Ghiri et al., 2019; Estrada-Camarena et al., 2019; García-Ríos et al., 2019; Hatcher et al., 2019; Herbst et al., 2019; Kumar et al., 2019; Lee et al., 2019; Malikowska-Racia et al., 2019; Moreira et al., 2019; Munshi et al., 2019; Neuwirth et al., 2019b; Scholl et al., 2019; Tillmann and Wegener, 2019; Victoriano et al., 2019; Vieira et al., 2019; Wąsik et al., 2019; Zare et al., 2019)], and the Three Chamber Social Interaction Test [n = 49 (Bernard et al., 2015; Bruining et al., 2015; Cox et al., 2015; Finlay et al., 2015; Kigar et al., 2015; Kumar et al., 2015; Langley et al., 2015; Lee J. et al., 2015; Lee et al., 2016, 2018; Buffington et al., 2016; Ferri et al., 2016; Hegde et al., 2016; Kerr et al., 2016; Kratsman et al., 2016; Kumar and Sharma, 2016a, b; Lawson et al., 2016; Lee and Green, 2016; Li J. et al., 2016; de La Tremblaye et al., 2016; Bagosi et al., 2017a, b; Burke and Trang, 2017; Cai et al., 2017; Cao et al., 2017; Ebihara et al., 2017; Garcia et al., 2017; Henbid et al., 2017; Hsieh et al., 2017; Karkaba et al., 2017; Arakawa, 2018; Bausch et al., 2018; Benekareddy et al., 2018; Borland et al., 2018; Crestani et al., 2018; Goñi-Balentziaga et al., 2018; He et al., 2018; Holman et al., 2018; Leung et al., 2018; Basaure et al., 2019; Burns et al., 2019; Faure et al., 2019; Garbarino et al., 2019; García-Díaz et al., 2019; Gubert and Hannan, 2019; Kosel et al., 2019; Lin T. et al., 2019; Lin Y. et al., 2019; Bond et al., 2020)]. The following data indicate the included number of publications with adequate reporting of lighting from the starting n = 105 per behavioral test: the Light/Dark Box Test [n = 61; 62.25% (Banaskowski et al., 2015; Bentea et al., 2015; Bertolus et al., 2015; Kalouda and Pitsikas, 2015; Liu et al., 2015; Quines et al., 2015; Sauce et al., 2015; Skupio et al., 2015; Thompson et al., 2015; Yu et al., 2015; Zhang C. et al., 2015; Zhang et al., 2016; Acevedo et al., 2016; Christensen et al., 2016; Diaz et al., 2016; Farajdokht et al., 2016; Fedotova et al., 2016; Fernandez et al., 2016; Hicks J. A. et al., 2016; Labots et al., 2016; Miranda-Morales and Pautassi, 2016; Salari et al., 2016; Socala and Wlaz, 2016; Vogt et al., 2016; Bahi, 2017a; Benoit et al., 2017; Borbely et al., 2017; Chandra Sekhar et al., 2017; Domonkos et al., 2017; Khalil and Fendt, 2017; Lee et al., 2017; Makinson et al., 2017; Orfanidou et al., 2017; Rogers et al., 2017; Salari and Amani, 2017; Sanna et al., 2017; Sirohi et al., 2017; Amodeo et al., 2018; Cazuza et al., 2018; Dong et al., 2018; Ferreira de Araújo et al., 2018; Garcia-Gutierrez et al., 2018; Heinla et al., 2018; Keenan et al., 2018; Mahmoudi et al., 2018; Morley-Fletcher et al., 2018; Van Camp et al., 2018; Wille-Bille et al., 2018; Zhang and Yao, 2018; Al-Harrasi et al., 2019; Bahi and Dreyer, 2019; Borrow et al., 2019; Freels et al., 2019; Laureano-Melo et al., 2019; Lovelock and Deak, 2019; Matsuo et al., 2019; Morgan et al., 2019; Peng et al., 2019; Tillmann et al., 2019; Winther et al., 2019; Zaccarelli-Magalhães et al., 2019)], the Open Field Test [n = 40; 30.09% (Allah Yar et al., 2015; Fowler and Muma, 2015; Kalouda and Pitsikas, 2015; Liu et al., 2015; Nakamura A. et al., 2015; Rilett et al., 2015; Thompson et al., 2015; Wensheng et al., 2015; Dagan et al., 2016; Estork et al., 2016; Girard et al., 2016; Li K. et al., 2016; Psyrdellis et al., 2016a, b; Schambra et al., 2016; Wscieklica et al., 2016; Blankenship et al., 2017; Casarrubea et al., 2017; Hansen et al., 2017; Kawabe, 2017; Kawasaki et al., 2017; Khalil and Fendt, 2017; Kuniishi et al., 2017; Mazur et al., 2017; Speight et al., 2017; Batinić et al., 2018; Blume et al., 2018; Bodden et al., 2018; Holubová et al., 2018; Noworyta-Sokołowska et al., 2018; Perea-Rodriguez et al., 2018; Rauhut and Curran-Rauhut, 2018; Robinson et al., 2018; Rojas-Carvajal et al., 2018; Saitoh et al., 2018; Struntz and Siegel, 2018; Hetzler et al., 2019; Jalilzad et al., 2019; Kumar et al., 2019; Neuwirth et al., 2019b)], the Elevated Plus Maze [n = 41; 41.41% (Kochenborger et al., 2014; Casarrubea et al., 2015, 2016; Daher and Mattioli, 2015; Horii and Kawaguchi, 2015; Saito and Brandão, 2015; Yang et al., 2015; Zhou et al., 2015; Cipriano et al., 2016; Estork et al., 2016; Li K. et al., 2016; Näslund et al., 2016; Serafim et al., 2016; Akbar et al., 2017; Bartolomé et al., 2017; Fernandes et al., 2017a, b; Kyriakou et al., 2017; Mazur et al., 2017; Vázquez-León et al., 2017, 2018; Bialuk et al., 2018; Boyette-Davis et al., 2018; Cazuza et al., 2018; Funck et al., 2018; Hirano et al., 2018; López Rivilli et al., 2018; Shimizu et al., 2018; Sorregotti et al., 2018; Tavares et al., 2018; Wang S. et al., 2018; Caliskan et al., 2019; Ebrahimi-Ghiri et al., 2019; García-Ríos et al., 2019; Lee et al., 2019; Moreira et al., 2019; Munshi et al., 2019; Scholl et al., 2019; Victoriano et al., 2019; Wąsik et al., 2019)], and the Three Chamber Social Interaction Test [n = 17; 34.69% (Kumar et al., 2015; Langley et al., 2015; Lee J. et al., 2015; Nakamura K. et al., 2015; Ferri et al., 2016; Kerr et al., 2016; de La Tremblaye et al., 2016; Bagosi et al., 2017a, b; Cai et al., 2017; Mihara et al., 2017; Benekareddy et al., 2018; Crestani et al., 2018; He et al., 2018; Namvarpour et al., 2018; Faure et al., 2019; Nakazawa et al., 2019)]. The Zero Maze and the Light/Dark Test were excluded from the refined analyses as the Zero Maze showed very little use in the field compared to the Elevated Plus Maze and the Light/Dark Test showed equivalent use in the field compared to the Light/Dark Box Test. Figure 1 illustrates a flow chart diagram of the refined search and article sample selection method.
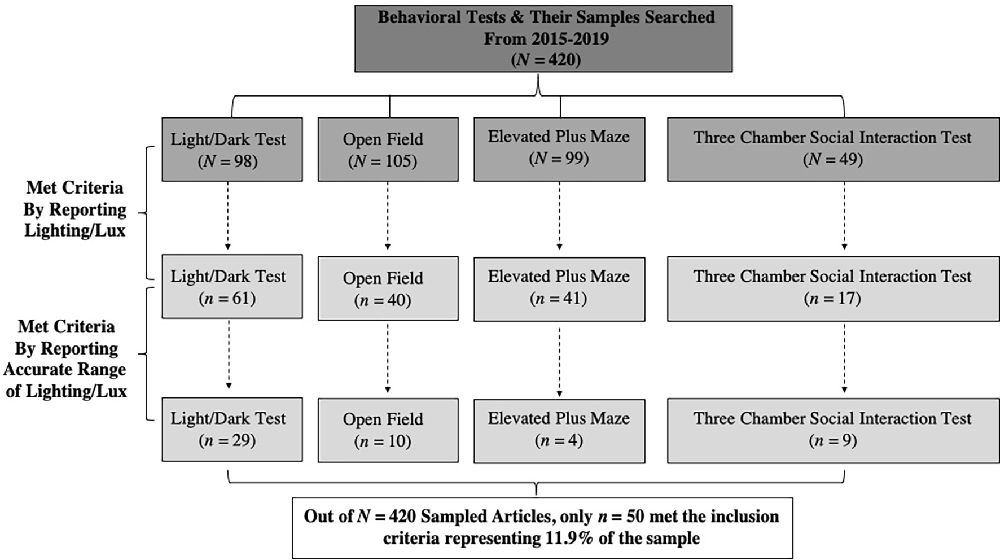
Figure 1. This figure illustrates the flow chart of how the 420 behavioral articles that were searched for to generate an equal representative sample (n = 105 articles of each behavioral test) comprising the Light/Dark Test, Open Field Test, Elevated Plus Maze Test, and the Three Chamber Social Interaction Test were sampled from 2015 to 2019 (n = 20–22 per year; upper dark gray rectangles). Of the N = 420 sampled, only n = 351 met the criteria for animal behavioral test relevance. The publications were then examined for meeting the inclusion criteria for reporting a lighting/Lux value for ethological controls for either anxiogenic or anxiolytic responses (middle light gray rectangles). Next, the refined number of articles were then examined for meeting the inclusion criteria for reporting accurate ranges of lighting/Lux for ethologically relevant stimuli motivation purposes that align with the test’s purpose in the field of behavioral neuroscience (lower light gray rectangles). Through the criteria used, 11.9% of the sampled articles across the behavioral tests were published using appropriate ethological motivational principles (white lowest rectangle) and only n = 50 met the full inclusion criteria, with the Light/Dark Test having better reporting (62.25%), followed by the Open Field (30.09%), then the Elevated Plus Maze (41.41%), and finally the Three Chamber Social Interaction Test with the worst (34.69%) of the respective samples.
Publications Meeting Criteria for Ethologically Controlled Lighting
Lastly, from the n = 351 selected publications, using the reporting of lighting as the next inclusion criteria, resulted in n = 159 publications returned across the behavioral tests (see Figure 1 middle light gray rectangles). Next, a new refinement criteria of whether the publications clearly noted the use of Lux for the evaluation of ethologically controlled lighting were determined. From this new refinement criteria (see Figure 1 lower gray rectangles) the following data indicate the included number of publications from the previously selected n = 159: the Light/Dark Box Test [n = 29 (Banaskowski et al., 2015; Bentea et al., 2015; Liu et al., 2015; Sauce et al., 2015; Zhang C. et al., 2015; Zhang et al., 2016; Acevedo et al., 2016; Christensen et al., 2016; Farajdokht et al., 2016; Fernandez et al., 2016; Hicks J. A. et al., 2016; Labots et al., 2016; Miranda-Morales and Pautassi, 2016; Vogt et al., 2016; Benoit et al., 2017; Borbely et al., 2017; Chandra Sekhar et al., 2017; Khalil and Fendt, 2017; Makinson et al., 2017; Rogers et al., 2017; Sirohi et al., 2017; Heinla et al., 2018; Keenan et al., 2018; Mahmoudi et al., 2018; Wille-Bille et al., 2018; Zhang and Yao, 2018; Laureano-Melo et al., 2019; Morgan et al., 2019; Tillmann et al., 2019)], the Open Field Test [n = 10 (Zhang C. et al., 2015; Estork et al., 2016; Casarrubea et al., 2017; Kawabe, 2017; Khalil and Fendt, 2017; Kuniishi et al., 2017; Blume et al., 2018; Perea-Rodriguez et al., 2018; Struntz and Siegel, 2018; Hetzler et al., 2019)], the Elevated Plus Maze [n = 4 (Zhang C. et al., 2015; Estork et al., 2016; Wang S. et al., 2018; Moreira et al., 2019)], and the Three Chamber Social Interaction Test [n = 9 (Langley et al., 2015; Lee J. et al., 2015; Nakamura K. et al., 2015; Ferri et al., 2016; Kerr et al., 2016; Mihara et al., 2017; Benekareddy et al., 2018; Crestani et al., 2018; He et al., 2018)]. Thus, from the original N = 420 publications sampled, only n = 50 met the criteria for ethologically controlled lighting across the behavioral tests evaluated herein, representing 11.9% of the sample studied (see Figure 1 white lowest rectangle).
Statistical Analyses
The descriptive statistics for the number of articles that met the aforementioned inclusion criterion were processed using SPSS version 24 (IBM®, Armonk, NY, United States). The data regarding the number of publications for the Light/Dark Test, Light/Dark Box Test, and the Open Field Test (Figure 2A) and the Elevated Plus Maze, Zero Maze Test, and the Three Chamber Social Interaction Test (Figure 2B) from 2009 to 2019 that were populated from Elsevier’s Science Direct search engine are depicted in Figure 2. The refined data that met the Lux criteria were depicted as Box and Whisker Plots showing the distribution of Lux used in each test (Figure 3). The mean is represented as (X) the median represented as the line within the boxes (-), the inter-quartile ranges (IQRs) were represented as the lower portion of the whisker to the box (IQR 1), the lower box to the median (IQR 2), the median to the upper box (IQR 3), and the upper box to the upper whisker (IQR 4; Figure 3).
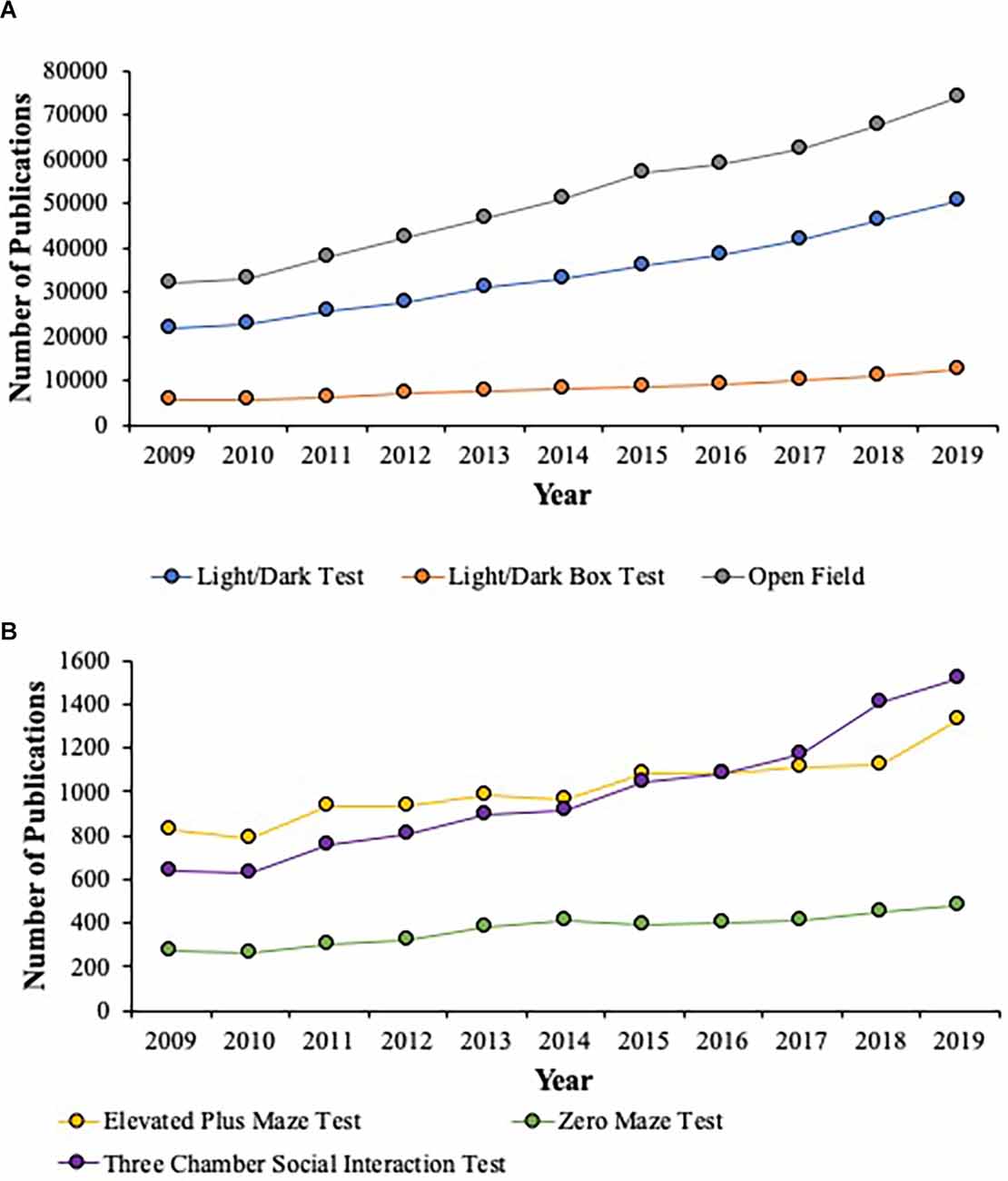
Figure 2. This figure illustrates the number of publications for the Light/Dark Test, Light/Dark Box Test, and the Open Field Test (A) and the Elevated Plus Maze, Zero Maze Test, and the Three Chamber Social Interaction Test (B) from 2009 to 2019 that populated from Elsevier’s Science Direct search engine. The data show that from 2009 to 2019, across all the behavioral tests noted above, there is a range of 43%–62% increase in their use across the last decade. The most popular behavioral tests used are the Open Field (Gray; A), the Light/Dark Test (Blue; A), followed by the Three Chamber Social Interaction Test (Purple; B), and the Elevated Plus Maze (Yellow; B). The Light/Dark Box Test (Orange; A) and the Zero Maze Test (Green; B) are used less than the Light/Dark Test (Blue; A) and the Elevated Plus Maze (Yellow; B). Across all behavioral tests, the Zero Maze Test was utilized the least (Green; B).
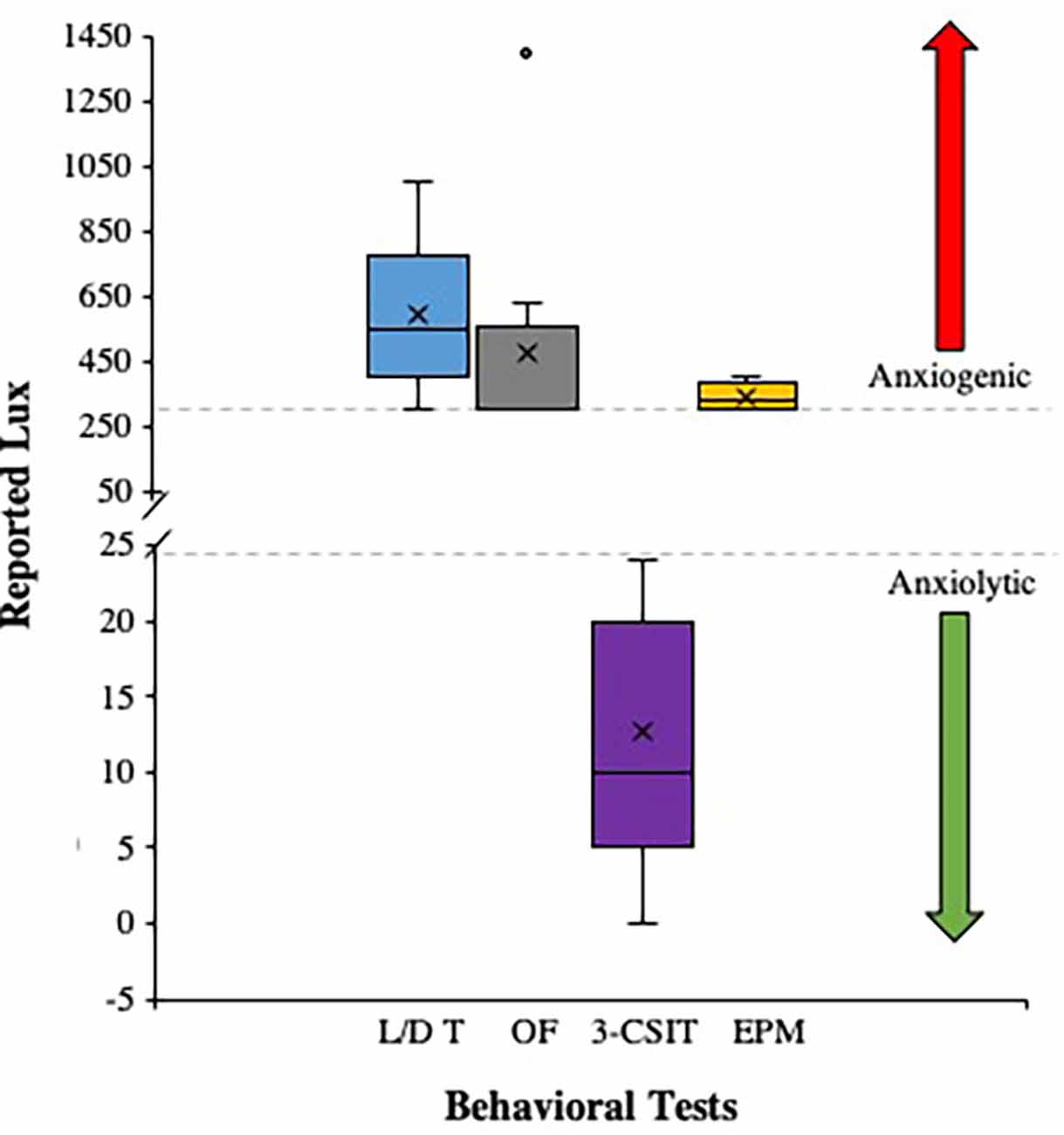
Figure 3. This figure illustrates the number of publications that met the criteria for ethologically relevant use of light stimuli (Lux) reported for the Light/Dark Test (L/D T), Open Field Test (OF), the Three Chamber Social Interaction Test (3-CSIT), and the Elevated Plus Maze Test (EPM). The data are presented as Box and Whisker Plots where the mean is represented as (X) the median represented as the line within the boxes (-), the inter-quartile ranges (IQRs) are represented as the lower portion of the whisker to the box (IQR 1), the lower box to the median (IQR 2), the median to the upper box (IQR 3), and the upper box to the upper whisker (IQR 4). The figure also shows a gray dashed line at 300 Lux indicating a threshold for anxiogenic behaviors that occur with light stimuli above this value (red arrow), whereas anxiolytic behaviors occur with light stimuli below the 25 Lux gray dashed line (green arrow). There was one reported outlier for the OF with a Lux of 1,400 reported, otherwise the L/D T, OF, and EPM for the studies that met criteria used comparable Lux as an anxiogenic stimulus range and the 3-CSIT Lux reported were within the anxiolytic stimulus range.
Results
Trends in the Frequency of Behavioral Tests Used in Publications Over the Last Decade
The publications that were sampled for the behavioral tests of interest over the last decade showed that a large number of publications used the Light/Dark Test (Mean = 34, 138.55; SD = 9, 489.16) and/or the Light/Dark Box Test (Mean = 8, 383.64; SD = 2, 194.19) along with the Open Field test (Mean = 51, 209.5; SD = 14, 107.1; see Figure 2A). Additionally, the use of these behavioral tests increased from 2009 to 2019 to the following levels: the Light/Dark Test (43.45%), the Light/Dark Box Test (45.37%), and the Open Field Test (43.13%). These three behavioral tests represented the most utilized in the neuroscience field. In contrast, the last decade showed a moderate number of publications that used the Elevated Plus Maze (Mean = 1, 013.82; SD = 151.85) and/or the Zero Maze (Mean = 372.55; SD = 70.54) along with the Three Chamber Social Interaction Test (Mean = 986.91; SD = 291.46; see Figure 2B), which had the lowest representation. This latter point is most likely due to this behavioral test being the more recent to be introduced to and adopted within the behavioral neuroscience field. Moreover, the use of these behavioral tests increased from 2009 to 2019 to the following levels: the Elevated Plus Maze (62.29%), the Zero Maze (56.73%), and the Three Chamber Social Interaction Test (42.07%).
Ethologically Relevant Findings From the Included Publications Reported Lux
The n = 50 publications that were included in the final analyses were compiled into a box and whisker plot to depict the distribution of Lux ranges reported for the Light/Dark Test, the Open Field, the Elevated Plus Maze, and the Three Chamber Social Interaction Tests, respectively. This was done to illustrate how researchers in the field are setting the lighting floor and ceiling threshold parameters for establishing operation for their behavioral tests, and when summarized in this illustrative way, can help facilitate the assessment of anxiogenic vs. anxiolytic stimuli parameters being properly used in the field under ethological motivational principles. The data showed a consist and ethologically relevant anxiogenic light stimuli (i.e., lighting was in the proper range to motivate the animal to respond appropriately) for the Light/Dark Test (Mean Lux = 593.41; Median = 550; SD = 245.82), the Open Field (Mean Lux = 476.5; Median = 300; SD = 345.32), the Elevated Plus Maze (Mean Lux = 337.5; Median = 325; SD = 47.87), and the Three Chamber Social Interaction Test (Mean Lux = 12.67; Median = 10; SD = 8.53; see Figure 3).
Discussion
The results from reviewing the literature on the proper use of lighting controls for ethological motivation in behavioral neuroscience testing revealed that as per this subsample, the majority of the publications did not report Lux or evidence of proper controls for lighting over the last half-decade. This highlights some rather serious concerns for the field, as current researchers using behavioral testing techniques, and prospective training of the next generation of behavioral neuroscientists will need to address this matter head-on. A main tenant in any science is the use of proper controls, minimizing threats to internal validity, and certainly having the foresight to limit and whenever possible, attempt to eliminate extraneous variables. In doing so, the behavioral work conducted will have an increased probability of external, face, construct, and predictive validity. This latter point prevents the unnecessary use of duplication of work, addressing ethical concerns with reducing the number of rodents required for testing research hypotheses, and ultimately serves to advance science in a more efficient and meaningful way; especially, in the behavioral neuroscience field (Russell and Burch, 1959; National Research Council of The National Academies, 2003; Committee for the Update of the Guide for the Care and Use of Laboratory Animals, Institute for Laboratory Animal Research, Division on Earth and Life Studies, & National Research Council, 2010; Cardon et al., 2012; Office of Laboratory Animal Welfare, 2015). It is important to state that a limitation of the research conducted herein is that the results are limited to an editorial group sampled from one type of journal database and it is possible that the same findings may occur when sampled from other journal databases, but this remains to be tested. Notably, the findings from this study indicate that 11.9% of the sampled papers reported proper use of the Lux in their behavioral testing and this would allow other researchers to evaluate the quality of ethological motivational principles within their tests for determining how it would influence their work. The remaining 88.1% of the papers either failed to mention lighting stimuli or used incorrect lighting measures (i.e., anxiogenic light stimuli in an anxiolytic test or anxiolytic light stimuli in an anxiogenic test). The consequences of not mentioning light conditions or using incorrect lighting are quite different and the proportion of each case remains unknown. Thus, authors are strongly encouraged to measure the lighting for each behavioral test they conduct on anxiety-like behaviors to address this issue. Further, knowing the lighting conditions and then considering the anxiety-like behaviors will serve to aid authors in confirming or disconfirming whether they have observed the animals’ responses to be consistent with what is expected for a given behavioral test when conducted within the appropriate Lux range consistent with prior reports.
From the publications that met the criterion (Figure 3), there seems to be a consistent range of Lux being used for the behavioral tests as follows: the Light/Dark Box Test (300–1,000 Lux), the Open Field Test (300–635 Lux), the Elevated Plus Maze (300–400 Lux), and the Three Chamber Social Interaction Test (0–24 Lux), respectively. In particular, the anxiogenic behavioral tests (i.e., the Light/Dark Box Test, the Open Field Test, and the Elevated Plus Maze) were shown to have publications reporting Lux within overlapping ranges (Garcia et al., 2005, 2011; Miller et al., 2021; Shoji and Miyakawa, 2021). This is a good sign that some researchers are conscientious of the ethological motivational factors and by using the same and/or approximate ranges of Lux that overlap, it permits the ability to have an external, face, construct, and predicative validity for these tests. In addition, the anxiolytic Three Chamber Social Interaction Test showed a broad low range of Lux to promote mobility as it is a key principle and motivating factor to ensure rodents are comfortable and will attempt to engage in movement related to the social operationally defined dependent measures for the respective test. Moreover, what can be extracted from this study is that when researchers use these behavioral tests, they should first determine their lighting stimuli in their respective behavioral testing rooms. If need be, a commercial Lux meter can be easily purchased from the internet (e.g., for $20-$25 USD from www.amazon.com). Thus, there is no cost-prohibitive factor in securing a simple, yet critical, piece of equipment for determining Lux prior to starting a behavioral research study.
However, even though the articles that met the inclusion criteria for each test show logical use of Lux for conducting each of the behavioral tests reviewed, there are still some concerns in variability that arise and should caution how future work should be reported and reviewed carefully. For example, the consistent reporting of species used and sex studied are paramount in understanding anxiety-like behaviors both within and between species as well as sex within a given species. In further review of the articles that met the inclusion criteria (see Table 1) illustrates the following variability in sex reporting that was found: Elevated Plus Maze (25% for males, 25% for females, and 50% for both males and females); Three Chamber Social Interaction Test (50% for males, 25% for both males and females, 12.5% for females, and 12.5% did not specify any sex); Open Field Test (60% for males and 40% for both males and females); and Light/Dark Test (62.07% for males, 10.34% for females, and 24.14% for both males and females, and 3.45% did not specify any sex). Moreover, the variability in species reporting that was found indicated a range of rats, mice, voles, and gerbils. To this end, there is also a need to be cautious of the diurnal/nocturnal biological rhythms, the age, generation, and whether or not the animals were subjected to drug compounds or other genetic manipulation prior to anxiety-like behavioral testing. These factors are equally important to consider whilst ensuring that any extrapolations from testing these particular animal species and manipulated models should include a clear and consistent practice of reporting the sex, testing both sexes, and noting the Lux used.
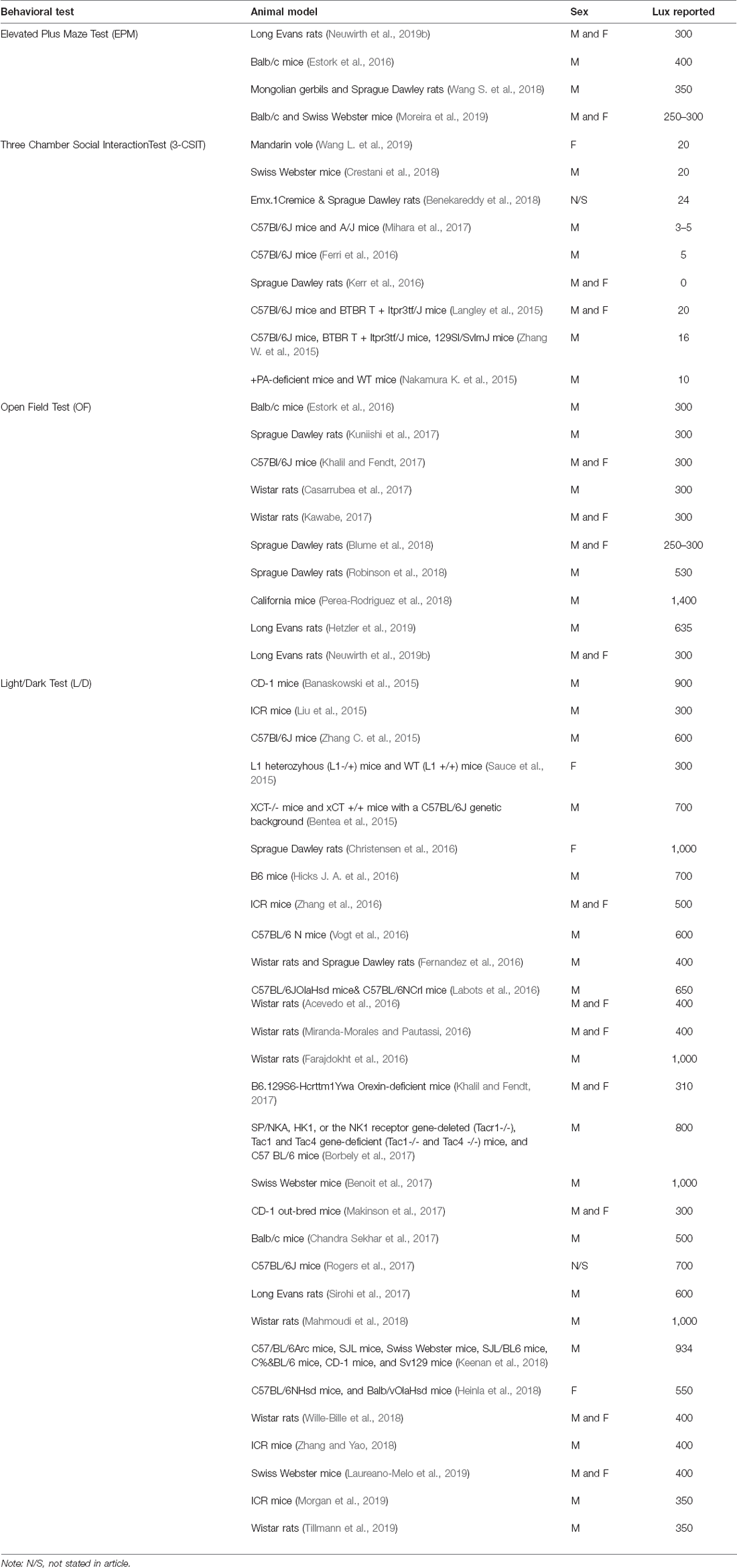
Table 1. Variations in behavioral tests that met criteria for the Lux reported to motivate anxiety-like behaviors.
Another factor for consideration is the issue of testing time (i.e., duration) of the anxiety-like behaviors in these species and sex used as pre-clinical models. In a review of the articles that met the inclusion criteria, it was also observed that variability ranging from as low as 4 min to as high as 30 min was used, but on average many studies reported use of 5 min to 10 min across all behavioral tests evaluated. Another concern arises that in the case of most animals, novelty to a new environment may induce natural neophobic traits that would cause the anxiogenic effect of just being exposed to a new testing environment. Thus, when constraining a behavioral test to provide an index or screening of anxiety-like behaviors, these tests may be skewed towards inflating the anxiogenic response profile as they do not offer ample time for habituation to a novel environment to be assessed carefully. In contrast, when prolonging a behavioral test of anxiety-like behaviors to run longer than 10 min, the inverse problem arises whereby the test may be skewed towards inflating the anxiolytic response profile as they offer too much time to habituate and remain uninterested in the novel environment to be assessed carefully. Thus, the 10 min behavioral testing time (i.e., duration) for a single test session for an animal is the recommended time (i.e., the first 5 min are used for screening anxiogenic responses and the last 5 min are used for screening anxiolytic responses).
Further, lighting becomes equally important when rodents are subjected to sequential behavioral tests that may compound or cause carryover anxiogenic effects [i.e., using the Open Field, then the Elevated Plus Maze, then the Two-Day Hole Board Test, or Context Fear Conditioning Tests, etc. (Neuwirth et al., 2013, 2015; Neuwirth et al., 2017; Neuwirth et al., 2019a; Neuwirth, 2014; Budylin et al., 2019)] and knowing the Lux measures can help to standardize such testing procedures to limit or as best limit artificially inflating the rodent’s anxiogenic neurobehavioral profiles. This is important as behavioral pharmacology or psychopharmacology that is used for such pre-clinical testing may then show either false-positives or false-negatives since they may represent more of an exaggerated behavioral phenotype than a well-intended pre-clinical animal model for screening new anxiolytic medications. In some cases, it may actually behoove researchers to employ the Open Field using an anxiolytic Lux stimulus (i.e., 0–24 Lux consistent with the Three Chamber Social Interaction Test) to promote behavioral movement in a baseline screening effort to assess for traits of hyperactivity or hypoactivity prior to being sequentially tested in the Elevated Plus Maze (Neuwirth et al., 2019a). This thoughtful and intentional behavioral testing methodology may help to better scrutinize whether immobility, freezing, or lack of exploration in the open arms of the Elevated Plus Maze were due to hypoactivity traits that were missed in a prior Open Field Test under anxiogenic lighting, in comparison to the rodent being hyperactive in the Open Field the prior day under anxiolytic lighting. The latter would provide insight into the clear environmental stimuli (e.g., the Elevated Plus Maze) and the lighting stimuli (i.e., anxiogenic effects), creating through the proper ethological motivational controls an actual anxiogenic behavioral response specific to the combined stimuli and not stimuli that were carried over. Additionally, through standardizing the anxiogenic and anxiolytic lighting stimuli for behavioral tests, such lighting parameters can be generalized over into novel or other behavioral tests to induce the same ethological motivational factors to make the rodents elicit specific and well-controlled behavioral responses, that in turn, can be treated with anxiolytic drugs for pre-clinical testing. Thus, with proper care and consideration for standardizing Lux as a measure in all behavioral neuroscience research design and then subsequent testing, better and more rapid advancements into anxiolytic drugs in the pre-clinical stage may become a reality for next-generation behavioral neuroscientists.
Conclusion
The field of behavioral neuroscience has had many challenges to overcome during the last few decades. One factor studied herein was the proper use of ethologically motivated Lux/lighting stimuli in the Light/Dark Test, the Open Field, the Elevated Plus Maze (i.e., for proper anxiogenic controls), and the Three Chamber Social Interaction Test (i.e., for proper anxiolytic controls). In a review of a sample set of N = 420 publications using these tests (i.e., n = 100–102 per test with approximately n = 20–22 sampled from each year from 2015 to 2019 prior to the pandemic), there were only n = 50 publications that were properly done and specified in their reports the use of Lux. This suggests that if this sample set were to be generalized back to the total publications in the literature that approximately 11%–12% of publications use proper ethologically motivated controls in their behavioral tests. This raises serious concerns for researchers in the area of behavioral neuroscience and perhaps the following suggestions may help to leverage better publishing controls for ensuring that the behavioral neuroscience community revisits this issue and sets a new standard to address this problem as the field moves forward. First, all journals that solicit and accept manuscript submissions that use behavioral testing, must be reviewed by an expert in the field with a behavioral neuroscience background. If journals are lacking such expertise in their editorial board or review board, they should solicit experts in the behavioral neuroscience area to serve to address this deficiency. Second, the journal’s editorial board should clearly identify such individuals for the public to be informed to confirm such expertise exists for the given journal. Third, in the requirements for the author’s manuscript guidelines, a subsection should include clearly stating the Lux/lighting for each behavioral test used as part of the submission requirements [i.e., this could also include the color of the light, Lux, and if using another measure (e.g., Watt), then the distance of the light from the behavioral test apparatus ought to be added along with the type of light (e.g., LED) so that a conversion to Lux can be conducted by the reader]. If the manuscript does not have this information, it should be returned to the authors to be corrected, and if it cannot be corrected, it should be rejected (i.e., this is also true if the specie, sex, age of the animals, and n-size used are not reported; especially, for the use of new mutant/genetically modified animals). Fourth, a re-evaluation of this report should occur in another decade to determine whether such standards have been actually approached or achieved in a direct effort to address one challenge in the behavioral neuroscience field so that other precious efforts and resources can be devoted to other challenges left unaddressed in the area. The goal should be for the field to reach 80%–85% of publications using behavioral neuroscience methods to consistently report Lux to interpret the ethologically motivated controls from each report. Additionally, researchers in the field should also seek to achieve an equal amount of reports on both male and female animal behaviors that should also reach 80%–85% of reports as the current situation shows largely a male animal model dominated literature. The latter is a critical point as the clinical literature reports greater susceptibility, vulnerability, and diagnoses of disorders in women over men. Moreover, males and females respond differently to drugs and drugs may need to be designed specifically to their differences in neurophysiological systems. Thus, the field needs to be more cognizant and intentional in increasing female animal models to be included in their pre-clinical studies. Lastly, researchers have the utmost responsibility that if they are to work in and employ behavioral neuroscience techniques and methods in the field then it is their duty to upskill and reskill themselves in fully understanding the importance of ethologically motivated controls for their behavioral tests. This would be no different for someone needing to know the basic principles of proteins to conduct proper Western blots, or reporting the degrees of freedom for any statistical test in a manuscript. So too, the Lux measure ought to be reported in all behavioral neuroscience manuscripts as they should not be an exception. In closing, researchers in this area can find invaluable information from seminal books on behavioral neuroscience testing from a range of classics to modern resources to help fill in any gaps that one might have (Green and Swets, 1966; Underwood, 1966; Gordon et al., 1968; Richelle and Lejune, 1980; Martin, 1997; Plomin et al., 2001; Harrington, 2011; Conn, 2017; Kolb and Whishaw, 2017; Commins, 2018).
Data Availability Statement
The original contributions presented in the study are included in the article, further inquiries can be directed to the corresponding author.
Author Contributions
MV (Open Field Test), ZH-M (Elevated Plus Maze/Zero Maze), JO (Light/Dark Test and/or Light/Dark box), and OL (Three Chamber Social Interaction Test) conducted the literature search, the sample collection method, and organized and tabulated the data from the respective publications of the behavioral tests reported herein. LN wrote the manuscript. MV, ZH-M, OL, and LN approved the final version of the manuscript to be submitted for publication. All authors contributed to the article and approved the submitted version.
Funding
The study was supported by a SUNY Old Westbury Faculty Development Grant (FDG) awarded to LN and a Collegiate Science Technology Entry Program (CSTEP)/LSAMP stipend awarded to OL.
Conflict of Interest
The authors declare that the research was conducted in the absence of any commercial or financial relationships that could be construed as a potential conflict of interest.
Publisher’s Note
All claims expressed in this article are solely those of the authors and do not necessarily represent those of their affiliated organizations, or those of the publisher, the editors and the reviewers. Any product that may be evaluated in this article, or claim that may be made by its manufacturer, is not guaranteed or endorsed by the publisher.
Acknowledgments
We would like to acknowledge the detailed and meticulous work of MV, ZH-M, JO, and OL for their dedication, labor, and effort in compiling the datasets used herein to make this publication possible.
References
Abdellatif, A., Omar, E. H., and Halima, G. (2017). The neuronal basis of copper induced modulation of anxiety state in rat. Acta Histochem. 119, 10–17. doi: 10.1016/j.acthis.2016.10.003
Acevedo, M. B., Fabio, M. C., Fernandez, M. S., and Pautassi, R. M. (2016). Anxiety response and restraint-induced stress differentially affect ethanol intake in female adolescent rats. Neuroscience 334, 259–274. doi: 10.1016/j.neuroscience.2016.08.011
Adolphs, R. (2010). Conceptual challenges and directions for social neuroscience. Neuron 65, 752–767. doi: 10.1016/j.neuron.2010.03.006
Akbar, S., Subhan, F., Karim, N., Aman, U., Ullah, S., Shahid, M., et al. (2017). Characterization of 6-methoxyflavanone as a novel anxiolytic agent: a behavioral and pharmacokinetic approach. Eur. J. Pharmacol. 801, 19–27. doi: 10.1016/j.ejphar.2017.02.047
Akil, H., Martone, M. E., and van Essen, D. C. (2011). Challenges and opportunities in mining neuroscience data. Science 331, 708–712. doi: 10.1126/science.1199305
Al-Harrasi, A., Khan, A., Rehman, N. U., Al-Shidhani, S., Karim, N., Khan, I., et al. (2019). Evidence for the involvement of a gabaergic mechanism in the effectiveness of natural and synthetically modified incensole derivatives in neuropharmacological disorders: a computational and pharmacological approach. Phytochemistry 163, 58–74. doi: 10.1016/j.phytochem.2019.04.007
Alkhlaif, Y., Bagdas, D., Jackson, A., Park, A. J., and Damaj, I. M. (2017). Assessment of nicotine withdrawal-induced changes in sucrose preference in mice. Pharmacol. Biochem. Behav. 161, 47–52. doi: 10.1016/j.pbb.2017.08.013
Allah Yar, R., Akbar, A., and Iqbal, F. (2015). Creatine monohydrate supplementation for 10 weeks mediates neuroprotection and improves learning/memory following neonatal hypoxia ischemia encephalopathy in female albino mice. Brain Res. 1595, 92–100. doi: 10.1016/j.pbb.2017.08.013
Alves, C. D. S., Frias, H. V., Kirsten, T. B., Cordeiro, F., Bernardi, M. M., and Suffredini, I. B. (2018). Luffa operculata fruit aqueous extract induces motor impairments, anxiety-like behavior and testis damage in rats. J. Ethnopharmacol. 222, 52–60. doi: 10.1016/j.jep.2018.04.044
Amodeo, L. R., Wills, D. N., Sanchez-Alavez, M., Nguyen, W., Conti, B., and Ehlers, C. L. (2018). Intermittent voluntary ethanol consumption combined with ethanol vapor exposure during adolescence increases drinking and alters other behaviors in adulthood in female and male rats. Alcohol 73, 57–66. doi: 10.1016/j.alcohol.2018.04.003
Amos-Kroohs, R. M., Bloor, C. P., Qureshi, M. A., Vorhees, C. V., and Williams, M. T. (2015). Effects of developmental exposure to manganese and/or low iron diet: changes to metal transporters, sucrose preference, elevated zero-maze, open-field and locomotion in response to fenfluramine, amphetamine and MK-801. Toxicol. Rep. 2, 1046–1056. doi: 10.1016/j.toxrep.2015.07.015
Arakawa, H. (2018). Analysis of social process in two inbred strains of male mice: a predominance of contact-based investigation in BALB/c mice. Neuroscience 369, 124–138. doi: 10.1016/j.neuroscience.2017.10.045
Arnold, M., Thallon, C., Pitkofsky, J., and Meerts, S. (2019). Sexual experience confers resilience to restraint stress in female rats. Horm. Behav. 107, 61–66. doi: 10.1016/j.yhbeh.2018.12.003
Atigari, D., Uprety, R., Pasternak, G., Majumdar, S., and Kivell, B. (2019). MP1104, a mixed kappa-delta opioid receptor agonist has anti-cocaine properties with reduced side-effects in rats. Neuropharmacology 150, 217–228. doi: 10.1016/j.neuropharm.2019.02.010
Bagosi, Z., Czébely-Lénárt, A., Karasz, G., Csabafi, K., Jászberényi, M., and Telegdy, G. (2017a). The effects of CRF and urocortins on the preference for social novelty of mice. Behav. Brain Res. 324, 146–154. doi: 10.1016/j.bbr.2017.02.009
Bagosi, Z., Karasz, G., Czébely-Lénárt, A., Csabafi, K., Jászberényi, M., and Telegdy, G. (2017b). The effects of CRF and urocortins on the sociability of mice. Brain Res. 1663, 114–122. doi: 10.1016/j.brainres.2017.03.003
Bahi, A. (2017a). Decreased anxiety, voluntary ethanol intake and ethanol-induced CPP acquisition following activation of the metabotropic glutamate receptor 8 “mGluR8.” Pharmacol. Biochem. Behav. 155, 32–42. doi: 10.1016/j.pbb.2017.03.004
Bahi, A. (2017b). Environmental enrichment reduces chronic psychosocial stress-induced anxiety and ethanol-related behaviors in mice. Prog. Neuropsychopharmacol. Biol. Psychiatry 77, 65–74. doi: 10.1016/j.pnpbp.2017.04.001
Bahi, A., and Dreyer, J. (2019). Dopamine transporter (DAT) knockdown in the nucleus accumbens improves anxiety- and depression-related behaviors in adult mice. Behav. Brain Res. 359, 104–115. doi: 10.1016/j.bbr.2018.10.028
Banaskowski, T. J., Cloutier, C. J., Ossenkopp, K., and Kavaliers, M. (2015). Repeated exposure of male mice to low doses of lipopolysaccharide: dose and time dependent development of behavioral sensitization and tolerance in an automated light-dark anxiety test. Behav. Brain Res. 286, 241–248. doi: 10.1016/j.bbr.2015.03.004
Bartolomé, I., Llidó, A., Darbra, S., and Pallarès, M. (2017). Effects of neonatal and adolescent neuroactive steroid manipulation on locomotor activity induced by ethanol in male wistar rats. Behav. Brain Res. 330, 68–74. doi: 10.1016/j.bbr.2017.05.009
Basaure, P., Guardia-Escote, L., Biosca-Brull, J., Blanco, J., Cabré, M., Peris-Sampedro, F., et al. (2019). Exposure to chlorpyrifos at different ages triggers APOE genotype-specific responses in social behavior, body weight and hypothalamic gene expression. Environ. Res. 178:108684. doi: 10.1016/j.envres.2019.108684
Bashiri, H., Rezayof, A., Sahebgharani, M., Tavangar, S., and Zarrindast, M. (2016). Modulatory effects of the basolateral amygdala α2-adrenoceptors on nicotine-induced anxiogenic-like behaviours of rats in the elevated plus maze. Neuropharmacology 105, 478–486. doi: 10.1016/j.neuropharm.2016.02.010
Bassi, G., Carvalho, M., Almada, R., and Brandão, M. (2017). Inhibition of substance P-induced defensive behavior via neurokinin-1 receptor antagonism in the central and medial but not basolateral nuclei of the amygdala in male Wistar rats. Prog. Neuropsychopharmacol. Biol. Psychiatry 77, 146–154. doi: 10.1016/j.pnpbp.2017.03.026
Batinić, B., Stanković, T., Stephen, M., Kodali, R., Tiruveedhula, V., Li, G., et al. (2018). Attaining in vivo selectivity of positive modulation of α3βγ2 GABAA receptors in rats: a hard task. Eur. Neuropsychopharmacol. 28, 903–914. doi: 10.1016/j.euroneuro.2018.05.014
Bausch, A. E., Ehinger, R., Straubinger, J., Zerfass, P., Nann, Y., and Lukowski, R. (2018). Loss of sodium-activated potassium channel slack and FMRP differentially affect social behavior in mice. Neuroscience 384, 361–374. doi: 10.1016/j.neuroscience.2018.05.040
Benekareddy, M., Stachniak, T., Bruns, A., Knoflach, F., Von Kienlin, M., Künnecke, B., et al. (2018). Identification of a corticohabenular circuit regulating socially directed behavior. Biol. Psychiatry 83, 607–617. doi: 10.1016/j.biopsych.2017.10.032
Benoit, S., Chaumontet, C., Schwarz, J., Cakir-Kiefer, C., and Tome, D. (2017). Mapping in mice the brain regions involved in the anxiolytic-like properties of a-casozepine, a tryptic peptide derived from bovine as1-casein. J. Funct. Foods 38, 464–473. doi: 10.1016/j.jff.2017.09.014
Bentea, E., Demuyser, T., Van Liefferinge, J., Albertini, G., Deneyer, L., Nys, J., et al. (2015). Absence of system xc- in mice decreases anxiety and depressive-like behavior without affecting sensorimotor function or spatial vision. Prog. NeuroPsychopharmacol. Biol. Psychiatry 59, 49–58. doi: 10.1016/j.pnpbp.2015.01.010
Bernard, P., Castano, A., Beitzel, C., Carlson, V., and Benke, T. (2015). Behavioral changes following a single episode of early-life seizures support the latent development of an autistic phenotype. Epilepsy Behav. 44, 78–85. doi: 10.1016/j.yebeh.2015.01.006
Bertolus, J. B., Nemeth, G., Makowska, I. J., and Weary, D. M. (2015). Rat aversion to sevoflurane and isoflurane. Appl. Anim. Behav. Sci. 164, 73–80. doi: 10.1016/j.applanim.2014.12.013
Bespalov, A., and Steckler, T. (2018). Lacking quality in research: Is behavioral neuroscience affected more than other areas of biomedical science? J. Neurosci. Methods 300, 4–9. doi: 10.1016/j.jneumeth.2017.10.018
Biagioni, A., Anjos-Garcia, T., Ullah, F., Fisher, I., Falconi-Sobrinho, L., Freitas, R., et al. (2016). Neuroethological validation of an experimental apparatus to evaluate oriented and non-oriented escape behaviours: comparison between the polygonal arena with a burrow and the circular enclosure of an open-field test. Behav. Brain Res. 298, 65–77. doi: 10.1016/j.bbr.2015.10.059
Bialuk, I., Taranta, A., and Winnicka, M. (2018). IL-6 deficiency alters spatial memory in 4- and 24-month-old mice. Neurobiol. Learn. Mem. 155, 21–29. doi: 10.1016/j.nlm.2018.06.006
Blankenship, P., Cherep, L., Donaldson, T., Brockman, S., Trainer, A., Yoder, R., et al. (2017). Otolith dysfunction alters exploratory movement in mice. Behav. Brain Res. 325, 1–11. doi: 10.1016/j.bbr.2017.02.031
Blume, S., Nam, H., Luz, S., Bangasser, D., and Bhatnagar, S. (2018). Sex- and age-dependent effects of orexin 1 receptor blockade on open-field behavior and neuronal activity. Neuroscience 381, 11–21. doi: 10.1016/j.neuroscience.2018.04.005
Bodden, C., Siestrup, S., Palme, R., Kaiser, S., Sachser, N., and Richter, S. (2018). Evidence-based severity assessment: impact of repeated versus single open-field testing on welfare in C57BL/6J mice. Behav. Brain Res. 336, 261–268. doi: 10.1016/j.bbr.2017.08.029
Bond, C. M., Johnson, J. C., Chaudhary, V., McCarthy, E. M., McWhorter, M. L., and Woehrle, N. S. (2020). Perinatal fluoxetine exposure results in social deficits and reduced monoamine oxidase gene expression in mice. Brain Res. 1727:146282. doi: 10.1016/j.brainres.2019.06.001
Bonuti, R., and Morato, S. (2018). Proximity as a predictor of social behavior in rats. J. Neurosci. Methods 293, 37–44. doi: 10.1016/j.jneumeth.2017.08.027
Borbely, E., Hajna, Z., Nabi, L., Scheich, B., Tekus, V., Laszlo, K., et al. (2017). Hemokinin-1 mediates anxiolytic and antidepressant-like actions in mice. Brain Behav. Immun. 59, 219–232. doi: 10.1016/j.bbi.2016.09.004
Borland, J. M., Grantham, K. N., Aiai, L. M., Frantz, K. J., and Albers, H. E. (2018). Role of oxytocin in the ventral tegmental area in social reinforcement. Psychoneuroendocrinology 95, 128–137. doi: 10.1016/j.psyneuen.2018.05.028
Borrow, A. P., Heck, A. L., Miller, A. M., Sheng, J. A., Stover, S. A., Daniels, R. M., et al. (2019). Chronic variable stress alters hypothalamic pituitary-adrenal axis function in the female mouse. Physiol. Behav. 209:112613. doi: 10.1016/j.physbeh.2019.112613
Boyette-Davis, J., Rice, H., Shoubaki, R., Gonzalez, C., Kunkel, M., Lucero, D., et al. (2018). A recreational dose of methylphenidate, but not methamphetamine, decreases anxiety-like behavior in female rats. Neurosci. Lett. 682, 21–26. doi: 10.1016/j.neulet.2018.06.005
Brown, R. E., and Bolivar, S. (2018). The importance of behavioural bioassays in neuroscience. J. Neurosci. Methods 300, 68–76. doi: 10.1016/j.jneumeth.2017.05.022
Brown, G. R., Kulbarsh, K. D., Spencer, K. A., and Duval, C. (2015). Peri-pubertal exposure to testicular hormones organizes response to novel environments and social behaviour in adult male rats. Horm. Behav. 73, 135–141. doi: 10.1016/j.yhbeh.2015.07.003
Bruining, H., Matsui, A., Oguro-Ando, A., Kahn, R. S., Van‘t Spijker, H. M., Akkermans, G., et al. (2015). Genetic mapping in mice reveals the involvement of Pcdh9 in long-term social and object recognition and sensorimotor development. Biol. Psychiatry 78, 485–495. doi: 10.1016/j.biopsych.2015.01.017
Budylin, T., Guariglia, S. R., Duran, L. I., Behring, B. M., Shaikh, Z., Neuwirth, L. S., et al. (2019). Ultrasonic vocalization sex differences in 5-HT1A-R deficient mouse pups: predictive phenotypes associated with later-life anxiety-like behaviors. Behav. Brain Res. 375:112062. doi: 10.1016/j.bbr.2019.112062
Buffington, S., Di Prisco, G., Auchtung, T., Ajami, N., Petrosino, J., and Costa-Mattioli, M. (2016). Microbial reconstitution reverses maternal diet-induced social and synaptic deficits in offspring. Cell 165, 1762–1775. doi: 10.1016/j.cell.2016.06.001
Burke, N. N., and Trang, T. (2017). Neonatal injury results in sex-dependent nociceptive hypersensitivity and social behavioral deficits during adolescence, without alterting morphine response. J. Pain 18, 1384–1396. doi: 10.1016/j.jpain.2017.07.003
Burns, P., Bowditch, J., McFadyen, J., Loiacono, R., Albiston, A. L., Pham, V., et al. (2019). Social behaviour is altered in the insulin-regulated aminopeptidase knockout mouse. Behav. Brain Res. 376:112150. doi: 10.1016/j.bbr.2019.112150
Cacioppo, J. T., and Decety, J. (2011). Social neuroscience: challenges and opportunities in the study of complex behavior. Ann. N Y Acad. Sci. 1224, 162–173. doi: 10.1111/j.1749-6632.2010.05858.x
Cai, Y., Wang, L., Xiao, R., Li, X., He, X., Gao, J., et al. (2017). Autism-like behavior in the BTBR mouse model of autism is improved by propofol. Neuropharmacology 118, 175–187. doi: 10.1016/j.neuropharm.2017.03.021
Caliskan, H., Akat, F., Tatar, Y., Zaloglu, N., Dursun, A., Bastug, M., et al. (2019). Effects of exercise training on anxiety in diabetic rats. Behav. Brain Res. 376:112084. doi: 10.1016/j.bbr.2019.112084
Cao, M., Pu, T., Wang, L., Marshall, C., He, H., Hu, G., et al. (2017). Early enriched physical environment reverses impairments of the hippocampus, but not medial prefrontal cortex, of socially-isolated mice. Brain Behav. Immun. 64, 232–243. doi: 10.1016/j.bbi.2017.04.009
Cardon, A. D., Bailey, M. R., and Bennett, B. T. (2012). The animal welfare act: from enactment to enforcement. J. Am. Assoc. Lab. Anim. Sci. 51, 301–305. Available online at: https://www.ncbi.nlm.nih.gov/pmc/articles/PMC3358977/.
Carobrez, A. P., and Bertoglio, L. J. (2005). Ethological and temporal analyses of anxiety-like behavior: the elevated plus-maze model 20 years on. Neurosci. Biobehav. Rev. 29, 1193–1205. doi: 10.1016/j.neubiorev.2005.04.017
Casarrubea, M., Faulisi, F., Caternicchia, F., Santangelo, A., Di Giovanni, G., Benigno, A., et al. (2016). Temporal patterns of rat behaviour in the central platform of the elevated plus maze. Comparative analysis between male subjects of strains with different basal levels of emotionality. J. Neurosci. Methods 268, 155–162. doi: 10.1016/j.jneumeth.2015.07.024
Casarrubea, M., Faulisi, F., Cudia, A., Cancemi, D., Cardaci, M., Magnusson, M., et al. (2017). Discovery of recurring behavioural sequences in Wistar rat social activity: possible support to studies on autism spectrum disorders. Neurosci. Lett. 653, 58–63. doi: 10.1016/j.neulet.2017.05.031
Casarrubea, M., Faulisi, F., Sorbera, F., and Crescimanno, G. (2015). The effects of different basal levels of anxiety on the behavioral shift analyzed in the central platform of the elevated plus maze. Behav. Brain Res. 281, 55–61. doi: 10.1016/j.bbr.2014.12.021
Cazuza, R. A., Pol, O., and Leite-Panissi, C. R. A. (2018). Enhanced expression of hemeoxygenase-1 in the locus coeruleus can be associated with anxiolytic-like effects. Behav. Brain Res. 336, 204–210. doi: 10.1016/j.bbr.2017.09.007
Chandra Sekhar, Y., Phani Kumar, G., and Anilakumar, K. R. (2017). Terminalia arjuna bark extract attenuates picrotoxin-induced behavioral changes by activation of serotonergic, dopaminergic, GABAergic and antioxidant systems. Chin. J. Nat. Med. 15, 584–596. doi: 10.1016/S1875-5364(17)30086-9
Chao, O. Y., Wang, A., Nikolaus, S., and Silva, M. A. (2015). NK3 receptor antagonism reinstates temporal order memory in the hemiparkinsonian rat. Behav. Brain Res. 285, 208–212. doi: 10.1016/j.bbr.2014.06.006
Christensen, S. L., Petersen, S., Sorensen, D. B., Olesen, J., and Jansen-Olesen, I. (2016). Infusion of low dose glyceryl trinitrate has no consistent effect on burrowing behavior, running wheel activity and light sensitivity in female rats. J. Pharmacol. Toxicol. Methods 80, 43–50. doi: 10.1016/j.vascn.2016.04.004
Cipriano, A., Gomes, K., and Nunes-de-Souza, R. (2016). CRF receptor type 1 (but not type 2) located within the amygdala plays a role in the modulation of anxiety in mice exposed to the elevated plus maze. Horm. Behav. 81, 59–67. doi: 10.1016/j.yhbeh.2016.03.002
Colla, A. R., Rosa, J. M., Cunha, M. P., and Rodrigues, A. S. (2015). Anxiolytic-like effects of ursolic acid in mice. Eur. J. Pharmacol. 758, 171–176. doi: 10.1016/j.ejphar.2015.03.077
Commins, S. (2018). Behavioural Neuroscience. Cambridge, United Kingdom: Cambridge University Press.
Committee for the Update of the Guide for the Care and Use of Laboratory Animals, Institute for Laboratory Animal Research, Division on Earth and Life Studies, & National Research Council. (2010). Guide for the Care and Use of Laboratory Animals. 8th Edn. Washington, DC: National Academies Press.
Cox, K., Quinnies, K., Eschendroeder, A., Didrick, P., Eugster, E., and Rissman, E. (2015). Number of X-chromosome genes influences social behavior and vasopressin gene expression in mice. Psychoneuroendocrinology 51, 271–281. doi: 10.1016/j.psyneuen.2014.10.010
Crestani, A. M., Cipriano, A. C., and Nunes-de-Souza, R. L. (2018). Single aggressive and non-aggressive social interactions elicit distinct behavioral patterns to the context in mice. Behav. Processes 157, 601–609. doi: 10.1016/j.beproc.2018.04.010
Cui, R., Wang, L., Liu, L., Ruan, H., and Li, X. (2018). Effects of noradrenergic and serotonergic systems on risk-based decision-making and center arena activity in open field in rats. Eur. J. Pharmacol. 841, 57–66. doi: 10.1016/j.ejphar.2018.09.026
Dagan, S., Tsoory, M., Fainzilber, M., and Panayotis, N. (2016). COLORcation: a new application to phenotype exploratory behavior models of anxiety in mice. J. Neurosci. Methods 270, 9–16. doi: 10.1016/j.jneumeth.2016.06.003
Daher, F., and Mattioli, R. (2015). Impairment in the aversive memory of mice in the inhibitory avoidance task but not in the elevated plus maze through intra-amygdala injections of histamine. Pharmacol. Biochem. Behav. 135, 237–245. doi: 10.1016/j.pbb.2015.05.023
Daniel, J. J., and Hughes, R. N. (2016). Increased anxiety and impaired spatial memory in young adult rats following adolescent exposure to methylone. Pharmacol. Biochem. Behav. 146–147, 44–49. doi: 10.1016/j.pbb.2016.05.003
Dastamooz, S., Tahmasebi Boroujeni, S., Shahbazi, M., and Vali, Y. (2018). Physical activity as an option to reduce adverse effect of EMF exposure during pregnancy. Int. J. Dev. Neurosci. 71, 10–17. doi: 10.1016/j.ijdevneu.2018.07.009
de La Tremblaye, P., Linares, N., Schock, S., and Plamondon, H. (2016). Activation of CRHR1 receptors regulates social and depressive-like behaviors and expression of BDNF and TrkB in mesocorticolimbic regions following global cerebral ischemia. Exp. Neurol. 284, 84–97. doi: 10.1016/j.expneurol.2016.07.019
Demir Özkay, Ü., Can, Ö., Sağlık, B., and Turan, N. (2017). A benzothiazole/piperazine derivative with acetylcholinesterase inhibitory activity: improvement in streptozotocin-induced cognitive deficits in rats. Pharmacol. Rep. 69, 1349–1356. doi: 10.1016/j.pharep.2017.06.009
Dempsey, E., Abautret-Daly, A., Docherty, N. G., Medina, C., and Harkin, A. (2019). Persistent central inflammation and region specific cellular activation accompany depression- and anxiety-like behaviours during the resolution phase of experimental colitis. Brain Behav. Immun. 80, 616–632. doi: 10.1016/j.bbi.2019.05.007
Dhediya, R., Joshi, S., Gajbhiye, S., Jalgaonkar, S., and Biswas, M. (2016). Evaluation of antiepileptic effect of S-adenosyl methionine and its role in memory impairment in pentylenetetrazole-induced kindling model in rats. Epilepsy Behav. 61, 153–157. doi: 10.1016/j.yebeh.2016.05.024
Diaz, M. R., Mooney, S. M., and Varlinskaya, E. I. (2016). Acute prenatal exposure to ethanol on gestational day 12 elicits opposing deficits in social behaviors and anxiety-like behaviors in Sprague Dawley rats. Behav. Brain Res. 310, 11–19. doi: 10.1016/j.bbr.2016.05.003
Dixon, E. I., and Hughes, R. N. (2019). Treatment with 1-benzylpiperazine (BZP) during adolescence of male and female hooded rats exposed to environmental enrichment: subsequent behavioral outcomes. Int. J. Dev. Neurosci. 73, 32–40. doi: 10.1016/j.ijdevneu.2018.12.005
Djordjevic, A., Bursac, B., Velickovic, N., Gligorovska, L., Ignjatovic, D., Tomic, M., et al. (2017). Disturbances of systemic and hippocampal insulin sensitivity in macrophage migration inhibitory factor (MIF) knockout male mice lead to behavioral changes associated with decreased PSA-NCAM levels. Horm. Behav. 96, 95–103. doi: 10.1016/j.yhbeh.2017.09.008
Domonkos, E., Borbelyova, V., Csongova, M., Bosy, M., Kacmarova, M., Ostatnikova, D., et al. (2017). Sex differences and sex hormones in anxiety-like behavior of aging rats. Horm. Behav. 93, 159–165. doi: 10.1016/j.yhbeh.2017.05.019
Donaire, R., Conrad, S., Thompson, J., Papini, M., and Torres, C. (2018). Augmented voluntary consumption of ethanol induced by reward downshift increases locomotor activity of male Wistar rats in the elevated plus maze. Behav. Processes 150, 59–65. doi: 10.1016/j.beproc.2018.02.013
Donatti, A., Soriano, R., Leite-Panissi, C., Branco, L., and de Souza, A. (2017). Anxiolytic-like effect of hydrogen sulfide (H2S) in rats exposed and re-exposed to the elevated plus-maze and open field tests. Neurosci. Lett. 642, 77–85. doi: 10.1016/j.neulet.2017.01.059
Dong, E., Guidotti, A., Zhang, H., and Pandey, S. C. (2018). Prenatal stress leads to chromatin and synaptic remodeling and excessive alcohol intake comorbid with anxiety-like behaviors in adult offspring. Neuropharmacology 140, 76–85. doi: 10.1016/j.neuropharm.2018.07.010
Dos Anjos, L., Gomes, F., Do Couto, L., Mourão, C., Moreira, K., Silva, L., et al. (2016). Anxiolytic activity and evaluation of potentially adverse effects of a bradykinin-related peptide isolated from a social wasp venom. Life Sci. 149, 153–159. doi: 10.1016/j.lfs.2016.02.063
Dougherty, J., Springer, D., Cullen, M., and Gershengorn, M. (2019). Evaluation of the effects of chemotherapy-induced fatigue and pharmacological interventions in multiple mouse behavioral assays. Behav. Brain Res. 360, 255–261. doi: 10.1016/j.bbr.2018.12.011
Duarte, F., Duzzioni, M., Leme, L., Smith, S., and De Lima, T. (2016). Evidence for involvement of NK3 receptors in the anxiogenic-like effect of SP6-11 (C-terminal), a metabolite of substance P, in rats evaluated in the elevated plus-maze. Behav. Brain Res. 303, 168–175. doi: 10.1016/j.bbr.2016.02.003
Dutra-Tavares, A., Manhães, A., Silva, J., Nunes-Freitas, A., Conceição, E., Moura, E., et al. (2015). Locomotor response to acute nicotine in adolescent mice is altered by maternal undernutrition during lactation. Int. J. Dev. Neurosci. 47, 278–285. doi: 10.1016/j.ijdevneu.2015.10.002
Ebihara, K., Fujiwara, H., Awale, S., Dibwe, D., Araki, R., Yabe, T., et al. (2017). Decrease in endogenous brain allopregnanolone induces autism spectrum disorder (ASD)-like behavior in mice: a novel animal model of ASD. Behav. Brain Res. 334, 6–15. doi: 10.1016/j.bbr.2017.07.019
Ebrahimi-Ghiri, M., Nasehi, M., and Zarrindast, M. (2019). Anxiolytic and antidepressant effects of ACPA and harmaline co-treatment. Behav. Brain Res. 364, 296–302. doi: 10.1016/j.bbr.2019.02.034
Elhady, M., Khalaf, A., Kamel, M., and Noshy, P. (2019). Carvacrol ameliorates behavioral disturbances and DNA damage in the brain of rats exposed to propiconazole. Neurotoxicology 70, 19–25. doi: 10.1016/j.neuro.2018.10.008
Estork, D. M., Gusmão, D. F., Paciencia, M. L. B., Frana, S. A., Díaz, I. E. C., Varella, A. D., et al. (2016). Casinga-cheirosa organic extract impairment over Balb-c male mice behavioral phenotype. Rev. Br. Farmac. 26, 216–224. doi: 10.1016/j.bjp.2015.10.004
Estrada-Camarena, E., Sollozo-Dupont, I., Islas-Preciado, D., González-Trujano, M. E., Carro-Juárez, M., and López-Rubalcava, C. (2019). Anxiolytic- and anxiogenic-like effects of Montanoa tomentosa (Asteraceae): dependence on the endocrine condition. J. Ethnopharmacol. 241:112006. doi: 10.1016/j.jep.2019.112006
Evans, C., Hvoslef-Eide, M., Thomas, R., Kidd, E., and Good, M. (2018). A rapidly acquired foraging-based working memory task, sensitive to hippocampal lesions, reveals age-dependent and age-independent behavioural changes in a mouse model of amyloid pathology. Neurobiol. Learn. Mem. 149, 46–57. doi: 10.1016/j.nlm.2018.02.004
Farajdokht, F., Babri, S., Karimi, P., and Mohaddes, G. (2016). Ghrelin attenuates hyperalgesia and light aversion-induced by nitroglycerin in male rats. Neurosci. Lett. 630, 30–37. doi: 10.1016/j.neulet.2016.07.026
Faure, A., Nosjean, A., Pittaras, E., Duchêne, A., Andrieux, A., Granon, S., et al. (2019). Dissociated features of social cognition altered in mouse models of schizophrenia: focus on social dominance and acoustic communication. Neuropharmacology 159:107334. doi: 10.1016/j.neuropharm.2018.09.009
Fedotova, J., Soultanov, V., Nikitina, T., Roschin, V., Ordyan, N., Hritcu, L., et al. (2016). Ropren treatment reverses anxiety-like behavior and monoamines levels in gonadectomized rat model of Alzheimer’s disease. Biomed. Pharmacother. 83, 1444–1455. doi: 10.1016/j.biopha.2016.08.065
Fernandes, C., Serafim, K., Gianlorenço, A., and Mattioli, R. (2017a). Cholinergic agonist reverses H1-induced memory deficit in mice. Prog. Neuropsychopharmacol. Biol. Psychiatry 72, 16–22. doi: 10.1016/j.pnpbp.2016.08.007
Fernandes, C., Serafim, K., Gianlorenco, A., and Mattioli, R. (2017b). Intra-vermis H4 receptor agonist impairs performance in anxiety- and fear-mediated models. Brain Res. Bull. 135, 179–184. doi: 10.1016/j.brainresbull.2017.10.014
Fernandez, M. S., Fabio, M. C., Miranda-Morales, R. S., Virgolini, M. B., De Giovanni, L. N., Hansen, C., et al. (2016). Age-related effects of chronic restraint stress on ethanol drinking, ethanol-induced sedation and on basal and stress-induced anxiety response. Alcohol 51, 89–100. doi: 10.1016/j.alcohol.2015.11.009
Ferreira de Araújo, E. J., Rezende-Júnior, L. M., Lima, L. K. F., da Silva-Júnior, M. P., Silva, O. A., Neto, B. P. D. S., et al. (2018). Pathophysiological investigations, anxiolytic effects and interaction of a semisynthetic Riparin with benzodiazepine receptors. Biomed. Pharmacother. 103, 973–981. doi: 10.1016/j.biopha.2018.04.130
Ferri, S., Kreibich, A., Torre, M., Piccoli, C., Dow, H., Pallathra, A., et al. (2016). Activation of basolateral amygdala in juvenile C57BL/6J mice during social approach behavior. Neuroscience 335, 184–194. doi: 10.1016/j.neuroscience.2016.08.006
Figueiredo, D. A. F., Pordeus, L. C. M., Paulo, L. L., Braga, R. M., Fonsêca, D. V., Sousa, B. S., et al. (2016). Effects of bark flour of Passiflora edulis on food intake, body weight and behavioral response of rats. Rev. Br. Farmac. 26, 595–600. doi: 10.1016/j.bjp.2016.02.010
Finlay, J., Dunham, G., Isherwood, A., Newton, C., Nguyen, T., Reppar, P., et al. (2015). Effects of prefrontal cortex and hippocampal NMDA NR1-subunit deletion on complex cognitive and social behaviors. Brain Res. 1600, 70–83. doi: 10.1016/j.brainres.2014.10.037
Fisch, J., Feistauer, V., de Moura, A., Silva, A., Bollis, V., Porawski, M., et al. (2019). Maternal feeding associated to post-weaning diet affects metabolic and behavioral parameters in female offspring. Physiol. Behav. 204, 162–167. doi: 10.1016/j.physbeh.2019.02.026
Fowler, S. C., and Muma, N. A. (2015). Use of a force-sensing automated open field apparatus in a longitudinal study of multiple behavioral deficits in CAG140 Huntington’s disease model mice. Behav. Brain Res. 294, 7–16. doi: 10.1016/j.bbr.2015.07.036
Freels, T. G., Lester, D. B., and Cook, M. N. (2019). Arachidonoyl serotonin (AA-5-HT) modulates general fear-like behavior and inhibits mesolimbic dopamine release. Behav. Brain Res. 362, 140–151. doi: 10.1016/j.bbr.2019.01.010
Funck, V., Fracalossi, M., Vidigal, A., and Beijamini, V. (2018). Dorsal hippocampal galanin modulates anxiety-like behaviours in rats. Brain Res. 1687, 74–81. doi: 10.1016/j.brainres.2018.02.036
Gamberini, M., Rodrigues, D., Rodrigues, D., and Pontes, V. (2015). Effects of the aqueous extract of Pimpinella anisum L. seeds on exploratory activity and emotional behavior in rats using the open field and elevated plus maze tests. J. Ethnopharmacol. 168, 45–49. doi: 10.1016/j.jep.2015.03.053
Garbarino, V. R., Santos, T. A., Nelson, A. R., Zhang, W. Q., Smolik, C. M., Javors, M. A., et al. (2019). Prenatal metformin exposure or organic cation transporter 3 knock-out curbs social interaction preference in male mice. Pharmacol. Res. 140, 21–32. doi: 10.1016/j.phrs.2018.11.013
Garcia, A. N., Bezner, K., Depena, C., Yin, W., and Gore, A. C. (2017). The effects of long-term estradiol treatment on social behavior and gene expression in adult female rats. Horm. Behav. 87, 145–154. doi: 10.1016/j.yhbeh.2016.11.011
Garcia, A. M., Cardenas, F. P., and Morato, S. (2005). Effect of different illumination levels on rat behavior in the elevated plus-maze. Physiol. Behav. 85, 265–270. doi: 10.1016/j.physbeh.2005.04.007
Garcia, A. M., Cardenas, F. P., and Morato, S. (2011). The effects of pentylenetetrazol, chlordiazepoxide and caffeine in rats tested in the elevated plus-maze depend on the experimental illumination. Behav. Brain Res. 217, 171–177. doi: 10.1016/j.bbr.2010.09.032
García-Díaz, C., Sánchez-Catalán, M. J., Castro-Salazar, E., García-Avilés, A., Albert-Gascó, H., Sánchez-Sarasúa de la Bárcena, S., et al. (2019). Nucleus incertus ablation disrupted conspecific recognition and modified immediate early gene expression patterns in “social brain” circuits of rats. Behav. Brain Res. 356, 332–347. doi: 10.1016/j.bbr.2018.08.035
Garcia-Gutierrez, M. S., Navarrete, F., Laborda, J., and Manzanares, J. (2018). Deletion of DLK1 increases the vulnerability to developing anxiety-like behaviors and ethanol consumption in mice. Biochem. Pharmacol. 158, 37–44. doi: 10.1016/j.bcp.2018.09.029
García-Ríos, R., Mora-Pérez, A., González-Torres, D., Carpio-Reyes, R., and Soria-Fregozo, C. (2019). Anxiolytic-like effect of the aqueous extract of Justicia spicigera leaves on female rats: a comparison to diazepam. Phytomedicine 55, 9–13. doi: 10.1016/j.phymed.2018.07.007
Gatica, R., Pérez-Valenzuela, E., Sierra-Mercado, D., and Fuentealba, J. (2017). The expression of amphetamine sensitization is dissociable from anxiety and aversive memory: effect of an acute injection of amphetamine. Neurosci. Lett. 638, 21–26. doi: 10.1016/j.neulet.2016.12.009
Gillette, R., Reilly, M., Topper, V., Thompson, L., Crews, D., Gore, A., et al. (2017). Anxiety-like behaviors in adulthood are altered in male but not female rats exposed to low dosages of polychlorinated biphenyls in utero. Horm. Behav. 87, 8–15. doi: 10.1016/j.yhbeh.2016.10.011
Girard, S., Escoffier, G., Khrestchatisky, M., and Roman, F. (2016). The FVB/N mice: a well suited strain to study learning and memory processes using olfactory cues. Behav. Brain Res. 296, 254–259. doi: 10.1016/j.bbr.2015.09.004
Goes, T., Antunes, F., and Teixeira-Silva, F. (2015). Environmental enrichment for adult rats: effects on trait and state anxiety. Neurosci. Lett. 584, 93–96. doi: 10.1016/j.neulet.2014.10.004
Gomes, F., Paniago, C., Freire, D., Souza, A., Lima, M., Oliveira-Júnior, N., et al. (2016). Anxiolytic-like effect of a novel peptide isolated from the venom of the social wasp Synoeca surinama. Toxicon 122, 39–42. doi: 10.1016/j.toxicon.2016.09.015
Gomez, A., Petrucci, A., Dance, L., Morales-Valenzuela, J., Gibbs, N., Dahlhausen, C., et al. (2016). An acute, non-therapeutic dose of methylphenidate disrupts partner preference in female rats. Pharmacol. Biochem. Behav. 150, 100–107. doi: 10.1016/j.pbb.2016.09.005
Gomez-Marin, A., Paton, J. J., Kampff, A. R., Costa, R. M., and Mainen, Z. F. (2014). Big behavioral data: psychology, ethology and the foundations of neuroscience. Nat. Neurosci. 17, 1455–1462. doi: 10.1038/nn.3812
Goñi-Balentziaga, O., Perez-Tejada, J., Renteria-Dominguez, A., Lebeña, A., and Labaka, A. (2018). Social instability in female rodents as a model of stress related disorders: a systematic review. Physiol. Behav. 196, 190–199. doi: 10.1016/j.physbeh.2018.09.001
Gordon, M. S., Bartholomew, G. A., Grinnell, A. D., Baker-Jørgensen, C., and White, F. N. (1968). Animal Function: Principles and Adaptations. New York, NY: The Macmillian Company.
Goulas, A., Bastiani, M., Bezgin, G., Uylings, H. B. M., Roebroechk, A., Stiers, P., et al. (2014). Comparative analysis of the macroscale structural connectivity in the macaque and human brain. PLoS Comput. Biol. 10:e1003529. doi: 10.1371/journal.pcbi.1003529
Gray, V., and Hughes, R. (2015). Drug-, dose- and sex-dependent effects of chronic fluoxetine, reboxetine and venlafaxine on open-field behavior and spatial memory in rats. Behav. Brain Res. 281, 43–54. doi: 10.1016/j.bbr.2014.12.023
Green, D. M., and Swets, J. A. (1966). Signal Detection Theory and Psychophysics. New York, NY: John Wilely and Sons, Inc.
Gubert, C., and Hannan, A. J. (2019). Environmental enrichment as an experience-dependent modulator of social plasticity and cognition. Brain Res. 1717, 1–14. doi: 10.1016/j.brainres.2019.03.033
Haleem, D. J., Inam, Q., and Haleem, M. A. (2015). Effects of clinically relevant doses of methy-phenidate on spatial memory, behavioral sensitization and open field habituation: a time related study. Behav. Brain Res. 281, 208–214. doi: 10.1016/j.bbr.2014.12.031
Hansen, A., Almeida, F., Bandiera, S., Pulcinelli, R., Fragoso, A., Schneider, R., et al. (2017). Taurine restores the exploratory behavior following alcohol withdrawal and decreases BDNF mRNA expression in the frontal cortex of chronic alcohol-treated rats. Pharmacol. Biochem. Behav. 161, 6–12. doi: 10.1016/j.pbb.2017.09.001
Harrington, M. (2011). The Design of Experiments in Neuroscience, 2nd Edn. Thousand Oaks, CA: Sage Publishers.
Hatcher, K., Willing, J., Chiang, C., Rattan, S., Flaws, J., Mahoney, M., et al. (2019). Exposure to di-(2-ethylhexyl) phthalate transgenerationally alters anxiety-like behavior and amygdala gene expression in adult male and female mice. Physiol. Behav. 207, 7–14. doi: 10.1016/j.physbeh.2019.04.018
He, Z., Guo, Q., Yang, Y., Wang, L., Zhang, S., Yuan, W., et al. (2018). Pre-weaning paternal deprivation impairs social recognition and alters hippocampal neurogenesis and spine density in adult mandarin voles. Neurobiol. Learn. Mem. 155, 452–462. doi: 10.1016/j.nlm.2018.09.006
Hegde, S., Ji, H., Oliver, D., Patel, N., Poupore, N., Shtutman, M., et al. (2016). PDE11A regulates social behaviors and is a key mechanism by which social experience sculpts the brain. Neuroscience 335, 151–169. doi: 10.1016/j.neuroscience.2016.08.019
Heinla, I., Ahlgren, J., Vasar, E., and Voikar, V. (2018). Behavioural characterization of C57BL/6N and BALB/c female mice in social home cage - effect of mixed housing in complex environment. Physiol. Behav. 188, 32–41. doi: 10.1016/j.physbeh.2018.01.024
Henbid, M., Marks, W., Collins, M., Cain, S., Snutch, T., Howland, J., et al. (2017). Sociability impairments in genetic absence epilepsy rats from strasbourg: reversal by the t-type calcium channel antagonist Z944. Exp. Neurol. 296, 16–22. doi: 10.1016/j.expneurol.2017.06.022
Herbst, L., Gaigher, T., Siqueira, A., Joca, S., Sampaio, K., Beijamini, V., et al. (2019). New evidence for refinement of anesthetic choice in procedures preceding the forced swimming test and the elevated plus-maze. Behav. Brain Res. 368:111897. doi: 10.1016/j.bbr.2019.04.011
Hetzler, B. E., Mclester-Davis, L. W. Y., and Tenpas, S. E. (2019). Methylphenidate and alcohol effects on flash-evoked potentials, body temperature and behavior in Long-Evans rats. Alcohol 77, 79–89. doi: 10.1016/j.alcohol.2018.10.009
Hicks, J. A., Hatzidis, A., Arruda, N. L., Gelineau, R. R., Monteiro De Pina, I., Adams, K. W., et al. (2016). Voluntary wheel-running attenuates insulin and weight gain and affects anxiety-like behaviors in C57BL6/J mice exposed to a high-fat diet. Behav. Brain Res. 310, 1–10. doi: 10.1016/j.bbr.2016.04.051
Hicks, K., Sullivan, A., Cao, J., Sluzas, E., Rebuli, M., Patisaul, H., et al. (2016). Interaction of bisphenol a (BPA) and soy phytoestrogens on sexually dimorphic sociosexual behaviors in male and female rats. Horm. Behav. 84, 121–126. doi: 10.1016/j.yhbeh.2016.06.010
Hill, X., Richeri, A., and Scorza, C. (2015). Measure of anxiety-related behaviors and hippocampal BDNF levels associated to the amnesic effect induced by MK-801 evaluated in the modified elevated plus-maze in rats. Physiol. Behav. 147, 359–363. doi: 10.1016/j.physbeh.2015.05.013
Hirano, T., Yanai, S., Takada, T., Yoneda, N., Omotehara, T., Kubota, N., et al. (2018). NOAEL-dose of a neonicotinoid pesticide, clothianidin, acutely induce anxiety-related behavior with human-audible vocalizations in male mice in a novel environment. Toxicol. Lett. 282, 57–63. doi: 10.1016/j.toxlet.2017.10.010
Holman, P. J., Ellis, L., Morgan, E., and Weinberg, J. (2018). Prenatal alcohol exposure disrupts male adolescent social behavior and oxytocin receptor binding in rodents. Horm. Behav. 105, 115–127. doi: 10.1016/j.yhbeh.2018.08.004
Holubová, A., Mikulecká, A., Pometlová, M., Nohejlová, K., and Šlamberová, R. (2018). Long-term early life adverse experience impairs responsiveness to exteroceptive stimuli in adult rats. Behav. Processes 149, 59–64. doi: 10.1016/j.beproc.2018.02.005
Horii, Y., and Kawaguchi, M. (2015). Higher detection sensitivity of anxiolytic effects of diazepam by ledge-free open arm with opaque walled closed arm elevated plus maze in male rats. Behav. Brain Res. 294, 131–140. doi: 10.1016/j.bbr.2015.07.059
Horsley, R., Lhotkova, E., Hajkova, K., Jurasek, B., Kuchar, M., Palenicek, T., et al. (2016). Detailed pharmacological evaluation of methoxetamine (MXE), a novel psychoactive ketamine analogue—behavioural, pharmacokinetic and metabolic studies in the Wistar rat. Brain Res. Bull. 126, 102–110. doi: 10.1016/j.brainresbull.2016.05.002
Hsieh, L., Wen, J. H., Miyares, L., Lombroso, P. J., and Bordey, A. (2017). Outbred CD1 mice are as suitable as inbred C57BL/6J mice in performing social tasks. Neurosci. Lett. 637, 142–147. doi: 10.1016/j.neulet.2016.11.035
Hughes, R. N., and Hamilton, J. J. (2018). Sex-dependent modification by chronic caffeine of acute methamphetamine effects on anxiety-related behavior in rats. Behav. Brain Res. 345, 30–38. doi: 10.1016/j.bbr.2018.02.013
Hughes, R. N., and Hancock, N. J. (2016). Strain-dependent effects of acute caffeine on anxiety related behavior in PVG/c, long-evans and wistar rats. Pharmacol. Biochem. Behav. 140, 51–61. doi: 10.1016/j.pbb.2015.11.005
Insel, T. R. (2010). The challenge of translation in social neuroscience: a review of oxytocin, vasopressin and affiliative behavior. Neuron 65, 768–779. doi: 10.1016/j.neuron.2010.03.005
Iqbal, S., Ali, M., and Iqbal, F. (2015). Long term creatine monohydrate supplementation, following neonatal hypoxic ischemic insult, improves neuromuscular coordination and spatial learning in male albino mouse. Brain Res. 1603, 76–83. doi: 10.1016/j.brainres.2014.10.006
Jacobskind, J. S., Rosinger, Z. J., Gonzalez, T., Zuloaga, K. L., and Zuloaga, D. G. (2018). Chronic methamphetamine exposure attenuates neural activation in Hypothalamic-Pituitary Adrenal Axis-associated brain regions in a sex-specific manner. Neuroscience 380, 132–145. doi: 10.1016/j.neuroscience.2018.04.010
Jalilzad, M., Jafari, A., and Babaei, P. (2019). Neuregulin1β improves both spatial and associative learning and memory in Alzheimer model of rats possibly through signaling pathways other than Erk1/2. Neuropeptides 78:101963. doi: 10.1016/j.npep.2019.101963
Jiménez-Ferrer, E., Santillán-Urquiza, M. A., Alegría-Herrera, E., Zamilpa, A., Noguerón-Merino, C., Tortoriello, J., et al. (2017). Anxiolytic effect of fatty acids and terpenes fraction from Aloysia triphylla: serotoninergic, GABAergic and glutamatergic implications. Biomed. Pharmacother. 96, 320–327. doi: 10.1016/j.biopha.2017.10.024
Kalouda, T., and Pitsikas, N. (2015). The nitric oxide donor molsidomine induces anxiolytic-like behaviour in two different rat models of anxiety. Pharmacol. Biochem. Behav. 138, 111–116. doi: 10.1016/j.pbb.2015.09.004
Kang, S., Wu, M., Galvez, R., and Gulley, J. (2016). Timing of amphetamine exposure in relation to puberty onset determines its effects on anhedonia, exploratory behavior and dopamine D1 receptor expression in young adulthood. Neuroscience 339, 72–84. doi: 10.1016/j.neuroscience.2016.09.044
Karkaba, A., Soualeh, N., Soulimani, R., and Bouayed, J. (2017). Perinatal effects of exposure to PCBs on social preferences in young adult and middle-aged offspring mice. Horm. Behav. 96, 137–146. doi: 10.1016/j.yhbeh.2017.09.002
Kawabe, K. (2017). Effects of chronic forced-swim stress on behavioral properties in rats with neonatal repeated MK-801 treatment. Pharmacol. Biochem. Behav. 159, 48–54. doi: 10.1016/j.pbb.2017.06.009
Kawasaki, K., Annicchiarico, I., Glueck, A., Morón, I., and Papini, M. (2017). Reward loss and the basolateral amygdala: a function in reward comparisons. Behav. Brain Res. 331, 205–213. doi: 10.1016/j.bbr.2017.05.036
Kawasaki, K., Glueck, A. C., Annicchiarico, I., and Papini, M. R. (2015). Function of the centromedial amygdala in reward devaluation and open-field activity. Neuroscience 303, 73–81. doi: 10.1016/j.neuroscience.2015.06.053
Keenan, R. J., Chan, J., Donnelly, P. S., Barnham, K. J., and Jacobson, L. H. (2018). The social defeat/overcrowding murine psychosocial stress model results in a pharmacologically reversible body weight gain but not depression - related behaviours. Neurobiol. Stress 9, 176–187. doi: 10.1016/j.ynstr.2018.09.008
Kędzierska, E., Dudka, J., Poleszak, E., and Kotlińska, J. (2017). Antidepressant and anxiolytic-like activity of sodium selenite after acute treatment in mice. Pharmacol. Rep. 69, 276–280. doi: 10.1016/j.pharep.2016.11.005
Kerr, D. M., Gilmartin, A., and Roche, M. (2016). Pharmacological inhibition of fatty acid amide hydrolase attenuates social behavioural deficits in male rats prenatally exposed to valproic acid. Pharmacol. Res. 113, 228–235. doi: 10.1016/j.phrs.2016.08.033
Ketcha Wanda, G. J. M., Djiogue, S., Gamo, F. Z., Ngitedem, S. G., and Njamen, D. (2015). Anxiolytic and sedative activities of aqueous leaf extract of Dichrocephala integrifolia (Asteraceae) in mice. J. Ethnopharmacol. 176, 494–498. doi: 10.1016/j.jep.2015.11.035
Khalil, R., and Fendt, M. (2017). Increased anxiety but normal fear and safety learning in orexin deficient mice. Behav. Brain Res. 320, 210–218. doi: 10.1016/j.bbr.2016.12.007
Khalil, S., Khalifa, H., Abdel-Motal, S., Mohammed, H., Elewa, Y., Mahmoud, H., et al. (2018). Spirulina platensis attenuates the associated neurobehavioral and inflammatory response impairments in rats exposed to lead acetate. Ecotoxicol. Environ.Saf. 157, 255–265. doi: 10.1016/j.ecoenv.2018.03.068
Kigar, S. L., Chang, L., and Auger, A. P. (2015). Gadd45b is an epigenetic regulator of juvenile social behavior and alters local pro-inflammatory cytokine production in the rodent amygdala. Brain Behav. Immun. 46, 60–69. doi: 10.1016/j.bbi.2015.02.018
Koch, K., Wagner, G., Dahnke, R., Schachtzabel, C., Güllmar, D., Reichenbach, J. R., et al. (2010). Structure-function relationships in the context of reinforcement-related learning: a combined diffusion tensor imaging-functional magnetic resonance imaging study. Neuroscience 168, 190–199. doi: 10.1016/j.neuroscience.2010.03.026
Kochenborger, L., Levone, B., Da Silva, E., Taschetto, A., Terenzi, M., Paschoalini, M., et al. (2014). The microinjection of a cannabinoid agonist into the accumbens shell induces anxiogenesis in the elevated plus-maze. Pharmacol. Biochem. Behav. 124, 160–166. doi: 10.1016/j.pbb.2014.05.017
Kolb, B., and Whishaw, I. (2017). Brain Behaviour: Revisiting the Classic Studies. Thousand Oaks, CA: Sage Publications.
Komaki, A., Hoseini, F., Shahidi, S., and Baharlouei, N. (2016). Study of the effect of extract of Thymus vulgaris on anxiety in male rats. J. Tradit. Complement. Med. 6, 257–261. doi: 10.1016/j.jtcme.2015.01.001
Kosari-Nasab, M., Shokouhi, G., Ghorbanihaghjo, A., Abbasi, M. M., and Salari, A. (2018). Anxiolytic and antidepressant-like effects of silymarin compared to diazepam and fluoxetine in a mouse model of mild traumatic brain injury. Toxicol. Appl. Pharmacol. 338, 159–173. doi: 10.1016/j.taap.2017.11.012
Kosel, F., Munoz, P. T., Yang, J. R., Wong, A. A., and Franklin, T. B. (2019). Age-related changes in social behaviours in the 5xFAD mouse model of Alzheimer’s disease. Behav. Brain Res. 362, 160–172. doi: 10.1016/j.bbr.2019.01.029
Kratsman, N., Getselter, D., and Elliott, E. (2016). Sodium butyrate attenuates social behavior deficits and modifies the transcription of inhibitory/excitatory genes in the frontal cortex of an autism model. Neuropharmacology 102, 136–145. doi: 10.1016/j.neuropharm.2015.11.003
Kruse, M., Vadillo, M., Miguelez Fernández, A., Rey, M., Zanutto, B., and Coirini, H. (2019). Sucrose exposure in juvenile rats produces long-term changes in fear memory and anxiety-like behavior. Psychoneuroendocrinology 104, 300–307. doi: 10.1016/j.psyneuen.2019.03.016
Kumar, D., Gupta, S., Ganeshpurkar, A., Singh, R., Kumar, D., Das, N., et al. (2019). Biological profiling of piperazinediones for the management of anxiety. Pharmacol. Biochem. Behav. 176, 63–71. doi: 10.1016/j.pbb.2018.11.009
Kumar, H., and Sharma, B. (2016a). Memantine ameliorates autistic behavior, biochemistry blood brain barrier impairments in rats. Brain Res. Bull. 124, 27–39. doi: 10.1016/j.brainresbull.2016.03.013
Kumar, H., and Sharma, B. (2016b). Minocycline ameliorates prenatal valproic acid induced autistic behaviour, biochemistry and blood brain barrier impairments in rats. Brain Res. 1630, 83–97. doi: 10.1016/j.brainres.2015.10.052
Kumar, H., Sharma, B., and Sharma, B. (2015). Benefits of agomelatine in behavioral, neurochemical and blood brain barrier alterations in prenatal valproic acid induced autism spectrum disorder. Neurochem. Int. 91, 34–45. doi: 10.1016/j.neuint.2015.10.007
Kuniishi, H., Ichisaka, S., Yamamoto, M., Ikubo, N., Matsuda, S., Futora, E., et al. (2017). Early deprivation increases high-leaning behavior, a novel anxiety-like behavior, in the open field test in rats. Neurosci. Res. 123, 27–35. doi: 10.1016/j.neures.2017.04.012
Kyriakou, E., Manfré, G., Spadaro, J., Nguyen, H., Harst, J., and Homberg, J. (2017). Anxiety and risk assessment-related traits in a rat model of Spinocerebellar ataxia type 17. Behav. Brain Res. 321, 106–112. doi: 10.1016/j.bbr.2016.12.023
Labots, M., Zheng, X., Moattari, G., Lozeman-van’t Klooster, J. G., Baars, J. M., Hesseling, P., et al. (2016). Substrain and light regime effects on integrated anxiety-related behavioral z-scores in male C57BL/6 mice hypomagnesaemia has only a small effect on avoidance behavior. Behav. Brain Res. 306, 71–83. doi: 10.1016/j.bbr.2016.01.060
Lamontagne, S., Olmstead, M., and Menard, J. (2016). The lateral septum and anterior hypothalamus act in tandem to regulate burying in the shock-probe test but not open-arm avoidance in the elevated plus-maze. Behav. Brain Res. 314, 16–20. doi: 10.1016/j.bbr.2016.07.034
Langley, E., Krykbaeva, M., Blusztajn, J., and Mellott, T. (2015). High maternal choline consumption during pregnancy and nursing alleviates deficits in social interaction and improves anxiety-like behaviors in the BTBR T+Itpr3tf/J mouse model of autism. Behav. Brain Res. 278, 210–220. doi: 10.1016/j.bbr.2014.09.043
Laureano-Melo, R., Caldeira, R. F., Guerra, A. F., Rodrigues da Conceição, R., Sena de Souza, J., Giannocco, G., et al. (2019). Maternal supplementation with lactobacillus paracasei DTA 83 alters emotional behavior in Swiss mice offspring. PharmaNutrition 8:100148. doi: 10.1016/j.phanu.2019.100148
Lawson, S. K., Gray, A. C., and Woehrle, N. S. (2016). Effects of oxytocin on serotonin 1B agonist-induced autism-like behavior in mice. Behav. Brain Res. 314, 52–64. doi: 10.1016/j.bbr.2016.07.027
Lawther, A., Clissold, M., Ma, S., Kent, S., Lowry, C., Gundlach, A., et al. (2015). Anxiogenic drug administration and elevated plus-maze exposure in rats activate populations of relaxin-3 neurons in the nucleus incertus and serotonergic neurons in the dorsal raphe nucleus. Neuroscience 303, 270–284. doi: 10.1016/j.neuroscience.2015.06.052
Lecorps, B., and Féron, C. (2015). Correlates between ear postures and emotional reactivity in a wild type mouse species. Behav. Processes 120, 25–29. doi: 10.1016/j.beproc.2015.08.002
Lecorps, B., Rödel, H., and Féron, C. (2016). Assessment of anxiety in open field and elevated plus maze using infrared thermography. Physiol. Behav. 157, 209–216. doi: 10.1016/j.physbeh.2016.02.014
Lederhendler, I., and Schulkin, J. (2000). Behavioral neuroscience: challenges for the era of molecular biology. Trends Neurosci. 23, 451–545. doi: 10.1016/s0166-2236(00)01636-2
Lee, K. M., Coehlo, M., McGregor, H. A., Waltermire, R. S., and Szumlinski, K. K. (2015). Binge alcohol drinking elicits persistent negative affect in mice. Behav. Brain Res. 291, 385–398. doi: 10.1016/j.bbr.2015.05.055
Lee, A., Cunniff, M., See, J., Wilke, S., Luongo, F., Ellwood, I., et al. (2019). VIP interneurons contribute to avoidance behavior by regulating information flow across hippocampal-prefrontal networks. Neuron 102, 1223–1234.e4. doi: 10.1016/j.neuron.2019.04.001
Lee, J., and Green, M. F. (2016). Social preference and glutamatergic dysfunction: underappreciated prerequisites for social dysfunction in schizophrenia. Trends Neurosci. 39, 587–596. doi: 10.1016/j.tins.2016.06.005
Lee, H., Jang, M., and Noh, J. (2017). Oxytocin attenuates aversive response to nicotine and anxiety-like behavior in adolescent rats. Neurosci. Res. 115, 29–36. doi: 10.1016/j.neures.2016.11.007
Lee, Y., Kim, Y., and Goto, Y. (2016). Cognitive and affective alterations by prenatal and postnatal stress interaction. Physiol. Behav. 165, 146–153. doi: 10.1016/j.physbeh.2016.07.014
Lee, J., Kwak, M., and Lee, P. (2015). Impairment of social behavior and communication in mice lacking the Uba6-dependent ubiquitin activation system. Behav. Brain Res. 281, 78–85. doi: 10.1016/j.bbr.2014.12.019
Lee, J. H., Zhang, J. Y., Wei, Z. Z., and Yu, S. P. (2018). Impaired social behaviors and minimized oxytocin signaling of the adult mice deficient in the N-methyl-d-aspartate receptor GluN3A subunit. Exp. Neurol. 305, 1–12. doi: 10.1016/j.expneurol.2018.02.015
Leković, A., Ademović, A., Grubač, Ž., Šutulović, N., Knežević, B., Novaković, M., et al. (2017). Anxiety-like behavior in elevated plus maze upon sleep fragmentation of one light phase of rats circadian cycle. Porto Biomed. J. 2:204. doi: 10.1016/j.pbj.2017.07.070
Leung, C., Cao, F., Nguyen, R., Joshi, K., Aqrabawi, A., Xia, S., et al. (2018). Activation of entorhinal cortical projections to the dentate gyrus underlies social memory retrieval. Cell Rep. 23, 2379–2391. doi: 10.1016/j.celrep.2018.04.073
Li, J., Li, H., Shou, X., Xu, X., Song, T., Han, S., et al. (2016). Effects of chronic restraint stress on social behaviors and the number of hypothalamic oxytocin neurons in male rats. Neuropeptides 60, 21–28. doi: 10.1016/j.npep.2016.08.011
Li, K., Lund, E., and Voigt, J. (2016). The impact of early postnatal environmental enrichment on maternal care and offspring behaviour following weaning. Behav. Processes 122, 51–58. doi: 10.1016/j.beproc.2015.11.008
Lin, T., Lo, Y., Lin, H., Li, S., Lin, S., Chen, K. C., et al. (2019). MR imaging central thalamic deep brain stimulation restored autistic-like social deficits in the rat. Brain Stimul. 12, 1410–1420. doi: 10.1016/j.brs.2019.07.004
Lin, Y., Lin, Y., Chen, K. C., Yang, Y. K., and Hsiao, Y. (2019). Collapsin response mediator protein 5 (CRMP5) causes social deficits and accelerates memory loss in an animal model of Alzheimer’s disease. Neuropharmacology 157:107673. doi: 10.1016/j.neuropharm.2019.107673
Listowska, M., Glac, W., Grembecka, B., Grzybowska, M., and Wrona, D. (2015). Changes in blood CD4+T and CD8+T lymphocytes in stressed rats pretreated chronically with desipramine are more pronounced after chronic open field stress challenge. J. Neuroimmunol. 282, 54–62. doi: 10.1016/j.jneuroim.2015.02.015
Liu, J., Zhai, W., Yang, Y., Shi, J., Liu, Q., Liu, G., et al. (2015). GABA and 5-HT systems are implicated in the anxiolytic-like effect of spinosin in mice. Pharmacol. Biochem. Behav. 128, 41–49. doi: 10.1016/j.pbb.2014.11.003
Liu, L., Zhang, L., Wang, T., and Chen, L. (2019). Dopamine D1 receptor in the medial prefrontal cortex mediates anxiety-like behaviors induced by blocking glutamatergic activity of the ventral hippocampus in rats. Brain Res. 1704, 59–67. doi: 10.1016/j.brainres.2018.09.024
Livingston-Thomas, J. M., Jeffers, M. S., Nguemeni, C., Shoichet, M. S., Morshead, C. M., Corbett, D., et al. (2015). Assessing cognitive function following medial prefrontal stroke in the rat. Behav. Brain Res. 294, 102–110. doi: 10.1016/j.bbr.2015.07.053
Lopes Andrade, A., Dias Ribeiro Figueiredo, D., Torequl Islam, M., Viana Nunes, A., Da Conceição Machado, K., Da Conceição Machado, K., et al. (2019). Toxicological evaluation of the biflavonoid, agathisflavone in albino Swiss mice. Biomed. Pharmacother. 110, 68–73. doi: 10.1016/j.biopha.2018.11.050
López Rivilli, M., Turina, A., Bignante, E., Molina, V., Perillo, M., Briñon, M., et al. (2018). Synthesis and pharmacological evaluation of pyrazolo[4,3-c]quinolinones as high affinity GABAA-R ligands and potential anxiolytics. Bioor. Med. Chem. 26, 3967–3974. doi: 10.1016/j.bmc.2018.06.021
López-Cruz, L., Carbó-Gas, M., Pardo, M., Bayarri, P., Valverde, O., Ledent, C., et al. (2017). Adenosine A2A receptor deletion affects social behaviors and anxiety in mice: involvement of anterior cingulate cortex and amygdala. Behav. Brain Res. 321, 8–17. doi: 10.1016/j.bbr.2016.12.020
Lovelock, D. F., and Deak, T. (2019). Acute stress imposed during adolescence yields heightened anxiety in Sprague Dawley rats that persists into adulthood: sex differences and potential involvement of the medial amygdala. Brain Res. 1723:146392. doi: 10.1016/j.brainres.2019.146392
Macedo, G., Morita, G., Domingues, L., Favoretto, C., Suchecki, D., Quadros, I., et al. (2018). Consequences of continuous social defeat stress on anxiety- and depressive-like behaviors and ethanol reward in mice. Horm. Behav. 97, 154–161. doi: 10.1016/j.yhbeh.2017.10.007
Machado, T., Alves, G., Quinteiro-Filho, W., and Palermo-Neto, J. (2017). Cohabitation with an Ehrlich tumor-bearing cagemate induces immune but not behavioral changes in male mice. Physiol. Behav. 169, 82–89. doi: 10.1016/j.physbeh.2016.11.022
Mahmoudi, J., Mohaddes, G., Erfani, M., Sadigh-Eteghad, S., Karimi, P., Rajabi, M., et al. (2018). Cerebrolysin attenuates hyperalgesia, photophobia and neuroinflammation in a nitroglycerin-induced migraine model in rats. Brain Res. Bull. 140, 197–204. doi: 10.1016/j.brainresbull.2018.05.008
Maia, T. V. (2009). Reinforcement learning, conditioning and the brain: Successes and challenges. Cogn. Affect. Behav. Neurosci. 9, 343–364. doi: 10.3758/CABN.9.4.343
Makinson, R., Lloyd, K., Rayasam, A., McKee, S., Brown, A., Barila, G., et al. (2017). Intrauterine inflammation induces sex-specific effects on neuroinflammation, white matter and behavior. Brain Behav. Immun. 66, 277–288. doi: 10.1016/j.bbi.2017.07.016
Malikowska, N., and Sałat, K. (2017). Evaluation of the activity of bupropion in a mouse model of posttraumatic stress disorder using forced swim and elevated plus maze tests. Eur. Neuropsychopharmacol. 27, S768–S769. doi: 10.1016/S0924-977X(17)31403-7
Malikowska-Racia, N., Sałat, K., Nowaczyk, A., Fijałkowski, Ł., and Popik, P. (2019). Dopamine D2/D3 receptor agonists attenuate PTSD-like symptoms in mice exposed to single prolonged stress. Neuropharmacology 155, 1–9. doi: 10.1016/j.neuropharm.2019.05.012
Marks, W., Zabder, N., Cain, S., Snutch, T., and Howland, J. (2019). The t-type calcium channel antagonist, z944, alters social behavior in genetic absence epilepsy rats from strasbourg. Behav. Brain Res. 361, 54–64. doi: 10.1016/j.bbr.2018.12.021
Marshall, P. J. (2009). Relating psychology and neuroscience: taking up the challenges. Perspect. Psychol. Sci. 4, 113–125. doi: 10.1111/j.1745-6924.2009.01111.x
Martin, R. (1997). Neurosceince Methods: A Guide for Advanced Students. Canberra, Australia: Harwood Academic Publishers.
Martins-Júnior, J., Bernardi, M., and Bondan, E. (2016). Propentofylline treatment on open field behavior in rats with focal ethidium bromide-induced demyelination in the ventral surface of the brainstem. Life Sci. 148, 132–138. doi: 10.1016/j.lfs.2016.02.028
Mascarenhas, D., Gomes, K., and Nunes-de-Souza, R. (2015). Role of TRPV1 channels of the dorsal periaqueductal gray in the modulation of nociception and open elevated plus maze-induced antinociception in mice. Behav. Brain Res. 292, 547–554. doi: 10.1016/j.bbr.2015.07.023
Mathuru, A. S., Libersat, F., Vyas, A., and Teseo, S. (2020). Why behavioral neurosceicne still needs diversity?: a curious case of persistent need. Neurosci. Biobehav. Rev. 116, 130–141. doi: 10.1016/j.neubiorev.2020.06.021
Matsuo, T., Uchida, T., Yamashita, K., Takei, S., Ido, D., Fujiwara, A., et al. (2019). Vision evaluation by functional observational battery, operant behavior test and light/dark box test in retinal dystrophic RCS rats versus normal rats. Heliyon 5:e01936. doi: 10.1016/j.heliyon.2019.e01936
Matthews, G., Nieh, E., Vander weele, C., Halbert, S., Pradhan, R., Yosafat, A., et al. (2016). Dorsal raphe dopamine neurons represent the experience of social isolation. Cell 164, 617–631. doi: 10.1016/j.cell.2015.12.040
Mazur, F., Oliveira, L., Cunha, M., Rodrigues, A., Pértile, R., Vendruscolo, L., et al. (2017). Effects of physical exercise and social isolation on anxiety-related behaviors in two inbred rat strains. Behav. Processes 142, 70–78. doi: 10.1016/j.beproc.2017.06.001
Mcneilly, A., Stewart, C., Sutherland, C., and Balfour, D. (2015). High fat feeding is associated with stimulation of the hypothalamic-pituitary-adrenal axis and reduced anxiety in the rat. Psychoneuroendocrinology 52, 272–280. doi: 10.1016/j.psyneuen.2014.12.002
Medawar, E., Benway, T. A., Liu, W., Hanan, T. A., Haslehurst, P., James, O. T., et al. (2019). Effects of rising amyloid β levels on hippocampal synaptic transmission, microglial response and cognition in APPSWE/PSEN1M146V transgenic mice. EBioMedicine 39, 422–435. doi: 10.1016/j.ebiom.2018.12.006
Melo, A. C., Costa, S. C. A., Castro, A. F., Souza, A. N. V., Sato, S. W., Lívero, F. A. R., et al. (2018). Hydroethanolic extract of Tropaeolum majus promotes anxiolytic effects on rats. Revista Brasileira de Farmacognosia 28, 589–593. doi: 10.1016/j.bjp.2018.06.006
Melo-Thomas, L., Gil-Martínez, A., Cuenca, L., Estrada, C., Gonzalez-Cuello, A., Schwarting, R., et al. (2018). Electrical stimulation or MK-801 in the inferior colliculus improve motor deficits in MPTP-treated mice. Neurotoxicology 65, 38–43. doi: 10.1016/j.neuro.2018.01.004
Miao, Z., Zhang, J., Li, Y., Li, X., Song, W., Sun, Z., et al. (2019). Presence of the pregnant partner regulates microRNA-30a and BDNF levels and protects male mice from social defeat-induced abnormal behaviors. Neuropharmacology 159:107589. doi: 10.1016/j.neuropharm.2019.03.032
Miguel, P., Deniz, B., Confortim, H., Bronauth, L., de Oliveira, B., Alves, M., et al. (2019). Methylphenidate administration reverts attentional inflexibility in adolescent rats submitted to a model of neonatal hypoxia-ischemia: predictive validity for ADHD study. Exp. Neurol. 315, 88–99. doi: 10.1016/j.expneurol.2019.02.004
Mihara, T., Mensah-Brown, K., Sobota, R., Lin, R., Featherstone, R., and Siegel, S. (2017). Amygdala activity associated with social choice in mice. Behav. Brain Res. 332, 84–89. doi: 10.1016/j.bbr.2017.04.040
Miller, C. K., Halbing, A. A., Patisaul, H. B., and Meitzen, J. (2021). Interactions of the estrous cycle, novelty and light on female and male rat open field locomotor and anxiety-related behaviors. Physiol. Behav. 228:113203. doi: 10.1016/j.physbeh.2020.113203
Miller, R., Yan, Z., Maher, C., Zhang, H., Gudsnuk, K., Mcdonald, J., et al. (2016). Impact of prenatal polycyclic aromatic hydrocarbon exposure on behavior, cortical gene expression and DNA methylation of the BDNF gene. Neuroepigenetics 5, 11–18. doi: 10.1016/j.nepig.2016.02.001
Miranda-Dominquez, O., Mills, B. D., Grayson, D., Woodall, A., Grant, K. A., Kroenke, C. D., et al. (2014). Bridging the gap between the human and macaque Connectome: a quantitative comparison of global interspecies structure-function relationships and network topology. J. Neurosci. 34, 5552–5563. doi: 10.1523/JNEUROSCI.4229-13.2014
Miranda-Morales, R. S., and Pautassi, R. M. (2016). Pharmacological characterization of the nociceptin/orphanin FQ receptor on ethanol-mediated motivational effects in infant and adolescent rats. Behav. Brain Res. 298, 88–96. doi: 10.1016/j.bbr.2015.04.016
Mlyniec, K., Starowicz, G., Gawel, M., Frackiewicz, E., and Nowak, G. (2016). Potential antidepressant like properties of the TC G-1008, A GPR39 (zinc receptor) agonist. J. Affect. Disord. 201, 179–184. doi: 10.1016/j.jad.2016.05.007
Moore, J. (2002). Some thoughts on the relation between behavior analysis and behavioral neuroscience. Psychol. Rec. 52, 261–279. doi: 10.1007/BF03395429
Moreira, V., Mattaraia, V., Rodrigues, M., de Albuquerque, C., and Moura, A. S. (2019). Parental behavior and anxiety in isogenic and outbred mice given access to two types of nesting materials. Appl. Anim. Behav. Sci. 215, 68–76. doi: 10.1016/j.applanim.2019.03.012
Morgan, A., Kondev, V., Bedse, G., Baldi, R., Marcus, D., and Patel, S. (2019). Cyclooxygenase-2 inhibition reduces anxiety-like behavior and normalizes enhanced amygdala glutamatergic transmission following chronic oral corticosterone treatment. Neurobiol. Stress 11:100190. doi: 10.1016/j.ynstr.2019.100190
Morley-Fletcher, S., Zuena, A. R., Mairesse, J., Gatta, E., Van Camp, G., Bouwalerh, H., et al. (2018). The reduction in glutamate release is predictive of cognitive and emotional alterations that are corrected by the positive modulator of AMPA receptors S 47445 in perinatal stressed rats. Neuropharmacology 135, 284–296. doi: 10.1016/j.neuropharm.2018.03.018
Morud, J., Strandberg, J., Andrén, A., Ericson, M., Söderpalm, B., and Adermark, L. (2018). Progressive modulation of accumbal neurotransmission and anxiety-like behavior following protracted nicotine withdrawal. Neuropharmacology 128, 86–95. doi: 10.1016/j.neuropharm.2017.10.002
Munshi, S., Parrilli, V., and Rosenkranz, J. (2019). Peripheral anti-inflammatory cytokine Interleukin-10 treatment mitigates interleukin-1β - induced anxiety and sickness behaviors in adult male rats. Behav. Brain Res. 372:112024. doi: 10.1016/j.bbr.2019.112024
Nakamura, A., Funaya, H., Uezono, N., Nakashima, K., Ishida, Y., Suzuki, T., et al. (2015). Low-cost three-dimensional gait analysis system for mice with an infrared depth sensor. Neurosci. Res. 100, 55–62. doi: 10.1016/j.neures.2015.06.006
Nakamura, K., Takabe, A., Shimizu, F., Takahashi, M., Matsuo, O., and Mitsui, S. (2015). Tissue plasminogen activator modulates emotion in a social context. Behav. Brain Res. 281, 24–31. doi: 10.1016/j.bbr.2014.11.022
Nakazawa, H., Suzuki, Y., Bando, Y., Yoshida, S., and Shiosaka, S. (2019). Impaired social discrimination behavior despite normal social approach by kallikrein-related peptidase 8 knockout mouse. Neurobiol. Learn. Mem. 162, 47–58. doi: 10.1016/j.nlm.2019.04.014
Namvarpour, Z., Nasehi, M., Amini, A., and Zarrindast, M. (2018). Protective role of alpha-lipoic acid in impairments of social and stereotyped behaviors induced by early postnatal administration of thimerosal in male rat. Neurotoxicol. Teratol. 67, 1–9. doi: 10.1016/j.ntt.2018.02.002
Narasingam, M., Vijeepallam, K., Mohamed, Z., and Pandy, V. (2017). Anxiolytic- and antidepressant-like activities of a methanolic extract of Morinda citrifolia Linn. (noni) fruit in mice: involvement of benzodiazepine-GABAAergic, serotonergic and adrenergic systems. Biomed. Pharmacother. 96, 944–952. doi: 10.1016/j.biopha.2017.11.148
Näslund, J., Studer, E., Johansson, E., and Eriksson, E. (2016). Effects of gonadectomy and serotonin depletion on inter-individual differences in anxiety-like behaviour in male Wistar rats. Behav. Brain Res. 308, 160–165. doi: 10.1016/j.bbr.2016.04.015
National Research Council of The National Academies (2003). Guidelines for the Care and Use of Mammals in Neuroscience and Behavioral Research. Washington, DC: National Academies Press.
Neuwirth, L. S. (2014). The characterization of Pb2+ toxicity in rat neural development: an assessment of Pb2+ effects on the GABA shift in neural networks and implications for learning and memory disruption. Dissertations, Theses, and Capstone Projects. CUNY Academic Works. Available online at: https://academicworks.cuny.edu/gc_etds/82/.
Neuwirth, L. S., Emenike, B. U., Barrera, E. D., Hameed, N., Rubi, S., Dacius, T. F., Jr., et al. (2019a). Assessing the anxiolytic properties of taurine-derived compounds in rats following developmental lead exposure: a neurodevelopmental and behavioral pharmacological pilot study. Adv. Exp. Med. Biol. 1155, 801–819. doi: 10.1007/978-981-13-8023-5_69
Neuwirth, L. S., Masood, S., Anderson, D. W., and Schneider, J. S. (2019b). The attention set-shifting test is sensitive for revealing sex-based impairments in executive functions following developmental lead exposure in rats. Behav. Brain Res. 366, 126–134. doi: 10.1016/j.bbr.2019.03.022
Neuwirth, L. S., Volpe, N. P., Corwin, C., Ng, S., Madan, N., Ferraro, A. M., et al. (2017). “Taurine recovery of learning deficits induced by developmental Pb2+ exposure,” in Taurine 10, Advances in Experimental Medicine and Biology, Vol. 975, eds D. H. Lee, S. Shaffer, E. Park and H. W. Kim (Dordrecht: Springer), 39–55. doi: 10.1007/978-94-024-1079-2_4
Neuwirth, L. S., and Volpe, N. P., and El Idrissi, A. (2013). “Taurine effects on emotional learning and memory in aged mice: neurochemical alterations and differentiation in auditory cued fear and context conditioning,” in Taurine 8: Physiological Roles and Mechanisms of Action, Advances in Experimental Medicine and Biology, Vol. 775, eds A. El Idrissi and W. L’Amoreaux (New York, NY: Springer Press), 195–214.
Neuwirth, L. S., Volpe, N. P., Ng, S., Marsillo, A., Corwin, C., Madan, N., et al. (2015). “Taurine recovers mice emotional learning and memory disruptions associated with Fragile X Syndrome in context fear and auditory cued-conditioning,” in Taurine 9; Advances in Experimental Medicine and Biology, Vol. 803, eds J. Marcinkiewicz and S. W. Schaffer (New York, NY: Springer Press), 425–438.
Ng, E., Geogiou, J., Avila, A., Trought, K., Mun, H., Hodgson, M., et al. (2020). Mice lacking Neuronal Calcium Sensor-1 show social and cognitive deficits. Behav. Brain Res. 381:112420. doi: 10.1016/j.bbr.2019.112420
Nie, L., Di, T., Li, Y., Cheng, P., Li, M., and Gao, J. (2018). Blockade of serotonin 5-HT2A receptors potentiates dopamine D2 activation-induced disruption of pup retrieval on an elevated plus maze, but has no effect on D2 blockade-induced one. Pharmacol. Biochem. Behav. 171, 74–84. doi: 10.1016/j.pbb.2018.06.004
Noguerón-Merino, M., Jiménez-Ferrer, E., Román-Ramos, R., Zamilpa, A., Tortoriello, J., and Herrera-Ruiz, M. (2015). Interactions of a standardized flavonoid fraction from Tilia americana with Serotoninergic drugs in elevated plus maze. J. Ethnopharmacol. 164, 319–327. doi: 10.1016/j.jep.2015.01.029
Noworyta-Sokołowska, K., Górska, A., and Gołembiowska, K. (2018). The effect of repeated-intermittent exposure to 5-methoxy-N,N-diisopropyltryptamine (5-MeO-DIPT) during adolescence on learning and memory in adult rats. Pharmacol. Rep. 70, 890–895. doi: 10.1016/j.pharep.2018.04.001
Nunes, G., Costa, L., Gutierrez, S., Satyal, P., and de Freitas, R. (2015). Behavioral tests and oxidative stress evaluation in mitochondria isolated from the brain and liver of mice treated with riparin A. Life Sci. 121, 57–64. doi: 10.1016/j.lfs.2014.11.018
O’Connor, K. A., Feustel, P. J., Ramirez-Zamora, A., Molho, E., Pilitsis, J., and Shin, D. (2016). Investigation of diazepam efficacy on anxiety-like behavior in hemiparkinsonian rats. Behav. Brain Res. 301, 226–237. doi: 10.1016/j.bbr.2015.12.045
Office of Laboratory Animal Welfare (2015). PHS policy on humane care and use of laboratory animals. Available online at: Olaw.nih.gov/policies-laws/phspolicy.htm. Accessed March 30, 2022.
Okamoto, K., Hitora-Imamura, N., Hioki, H., and Ikegaya, Y. (2018). GABAergic malfunction in the anterior cingulate cortex underlying maternal immune activation-induced social deficits. J. Neuroimmunol. 321, 92–96. doi: 10.1016/j.jneuroim.2018.06.006
Onaolapo, J., Onaolapo, Y., Akanmu, A., and Olayiwola, G. (2016). Caffeine and sleep-deprivation mediated changes in open-field behaviours, stress response and antioxidant status in mice. Sleep Sci. 9, 236–243. doi: 10.1016/j.slsci.2016.10.008
Orfanidou, M. A., Lafioniatis, A., Trevlopoulou, A., Touzlatzi, N., and Pitsikas, N. (2017). Acute and repeated exposure with the nitric oxide (NO) donor sodium nitroprusside (SNP) differentially modulate responses in a rat model of anxiety. Nitric Oxide 69, 56–60. doi: 10.1016/j.niox.2017.05.002
Ortu, D., and Vaidya, M. (2016). The challenges of integrating behavioral and neural data: bridging and breaking boundaries across levels of analysis. Behav. Anal. 40, 209–224. doi: 10.1007/s40614-016-0074-5
Palotai, M., and Telegdy, G. (2016). Anxiolytic effect of the GPR103 receptor agonist peptide P550 (homolog of neuropeptide 26RFa) in mice. Involvement of neurotransmitters. Peptides 82, 20–25. doi: 10.1016/j.peptides.2016.05.004
Peleh, T., Ike, K. G. O., Wams, E. J., Lebois, E. P., and Hengerer, B. (2019). The reverse translation of a quantitative neuropsychiatric framework into preclinical studies: focus on social interaction and behavior. Neurosci. Biobehav. Rev. 97, 96–111. doi: 10.1016/j.neubiorev.2018.07.018
Peng, H., Tsai, T., Huang, W., Wu, H., and Hsu, K. (2019). Probiotic treatment restores normal developmental trajectories of fear memory retention in maternally separated infant rats. Neuropharmacology 153, 53–62. doi: 10.1016/j.neuropharm.2019.04.026
Perea-Rodriguez, J., Zhao, M., Harris, B., Raqueno, J., and Saltzman, W. (2018). Behavioral and endocrine consequences of placentophagia in male California mice (Peromyscus californicus). Physiol. Behav. 188, 283–290. doi: 10.1016/j.physbeh.2018.02.022
Pereda, D., Pardo, M. R., Morales, Y., Dominguez, N., Arnau, M. R., and Borges, R. (2015). Mice lacking chromogranins exhibit increased aggressive and depression-like behaviour. Behav. Brain Res. 278, 98–106. doi: 10.1016/j.bbr.2014.09.022
Plomin, R., DeFries, J. C., McClearn, G. E., and McGuffin, P. (2001). Behavioral Genetics. 4th Edn. New York, NY: Worth Publishers.
Provenzano, G., Chelini, G., and Bozzi, Y. (2017). Genetic control of social behavior: Lessons from mutant mice. Behav. Brain Res. 325, 237–250. doi: 10.1016/j.bbr.2016.11.005
Psyrdellis, M., Pautassi, R., and Justel, N. (2016a). Open field exposure facilitates recovery from an aversive emotional event: involvement of adrenergic and cholinergic transmitter systems. Neurosci. Lett. 633, 202–209. doi: 10.1016/j.neulet.2016.09.015
Psyrdellis, M., Pautassi, R., Mustaca, A., and Justel, N. (2016b). Cholinergic transmission underlies modulation of frustration by open field exposure. Pharmacol. Biochem. Behav. 140, 8–16. doi: 10.1016/j.pbb.2015.10.017
Purvis, E., Klein, A., and Ettenberg, A. (2018). Lateral habenular norepinephrine contributes to states of arousal and anxiety in male rats. Behav. Brain Res. 347, 108–115. doi: 10.1016/j.bbr.2018.03.012
Queiroz, M. P., da Silva Lima, M., Tavares de Melo, M. F. F., Bertozzo, C. C. M. S., Fernandes de Araújod, D., Guerra, G. C. B., et al. (2019). Maternal suppplementation with conjugated linoleic acid reduce anxiety and lipid peroxidation in the offspring brain. J. Affect. Disord. 243, 75–82. doi: 10.1016/j.jad.2018.09.020
Quines, C. B., Da Rocha, J. T., Sampaio, T. B., Pesarico, A. P., Neto, J. S., Zeni, G., et al. (2015). Involvement of the serotonergic system in the anxiolytic-like effect of 2 phenylethynyl butyltellurium in mice. Behav. Brain Res. 277, 221–227. doi: 10.1016/j.bbr.2014.05.071
Rafati, A., Erfanizadeh, M., Noorafshan, A., and Karbalay-Doust, S. (2015). Effect of benzene on the cerebellar structure and behavioral characteristics in rats. Asian Pac. J. Trop. Biomed. 5, 568–573. doi: 10.1016/j.apjtb.2015.05.002
Rangel-Barajas, C., Estrada-Sánchez, A., Barton, S., Luedtke, R., and Rebec, G. (2017). Dysregulated corticostriatal activity in open-field behavior and the head-twitch response induced by the hallucinogen 2,5-dimethoxy-4-iodoamphetamine. Neuropharmacology 113, 502–510. doi: 10.1016/j.neuropharm.2016.11.001
Rauhut, A., and Curran-Rauhut, M. (2018). 17 β-Estradiol exacerbates methamphetamine-induced anxiety-like behavior in female mice. Neurosci. Lett. 681, 44–49. doi: 10.1016/j.neulet.2018.05.025
Reilly, M., Weeks, C., Topper, V., Thompson, L., Crews, D., and Gore, A. (2015). The effects of prenatal PCBs on adult social behavior in rats. Horm. Behav. 73, 47–55. doi: 10.1016/j.yhbeh.2015.06.002
Reimer, A., de Oliveira, A., Diniz, J., Hoexter, M., Chiavegatto, S., and Brandão, M. (2015). Rats with differential self-grooming expression in the elevated plus-maze do not differ in anxiety-related behaviors. Behav. Brain Res. 292, 370–380. doi: 10.1016/j.bbr.2015.06.036
Reinhart, F., Massri, N., Torres, N., Chabrol, C., Molet, J., Johnstone, D., et al. (2017). The behavioural and neuroprotective outcomes when 670nm and 810nm near infrared light are applied together in MPTP-treated mice. Neurosci. Res. 117, 42–47. doi: 10.1016/j.neures.2016.11.006
Rico, J., Penagos-Gil, M., Castañeda, A., and Corredor, K. (2016). Gerbils exhibit stable open-arms exploration across repeated testing on the elevated plus-maze. Behav. Processes 122, 104–109. doi: 10.1016/j.beproc.2015.11.017
Rilett, K., Friedel, M., Ellegood, J., Mackenzie, R., Lerch, J., and Foster, J. (2015). Loss of T cells influences sex differences in behavior and brain structure. Brain Behav. Immun. 46, 249–260. doi: 10.1016/j.bbi.2015.02.016
Robinson, L., Spruijt, B., and Riedel, G. (2018). Between and within laboratory reliability of mouse behaviour recorded in home-cage and open-field. J. Neurosci. Methods 300, 10–19. doi: 10.1016/j.jneumeth.2017.11.019
Rodrigues Tavares, L., Baptista-de-Souza, D., and Canto-de-Souza, A. (2016). (333) Fluoxetine revert the effect of MK-212 intra-amygdala injections but does not change the 8-OH-DPAT effects on antinociception induced in mice confined to open arms the elevated plus-maze. J. Pain 17:S59. doi: 10.1016/j.jpain.2016.01.240
Rogers, J., Li, S., Lanfumey, L., Hannan, A., and Renoir, T. (2017). Environmental enrichment reduces innate anxiety with no effect on depression-like behaviour in mice lacking the serotonin transporter. Behav. Brain Res. 332, 355–361. doi: 10.1016/j.bbr.2017.06.009
Rojas, A., Ganesh, T., Manji, Z., O’neill, T., and Dingledine, R. (2016). Inhibition of the prostaglandin E2 receptor EP2 prevents status epilepticus-induced deficits in the novel object recognition task in rats. Neuropharmacology 110, 419–430. doi: 10.1016/j.neuropharm.2016.07.028
Rojas-Carvajal, M., Fornaguera, J., Mora-Gallegos, A., and Brenes, J. (2018). Testing experience and environmental enrichment potentiated open-field habituation and grooming behaviour in rats. Anim. Behav. 137, 225–235. doi: 10.1016/j.anbehav.2018.01.018
Roohi-Azizi, M., Torkaman-Boutorabi, A., Akhondzadeh, S., Nejatisafa, A., Sadat-Shirazi, M., and Zarrindast, M. (2018). Influence of citicoline on citalopram-induced antidepressant activity in depressive-like symptoms in male mice. Physiol. Behav. 195, 151–157. doi: 10.1016/j.physbeh.2018.08.002
Russell, W. M. S., and Burch, R. L. (1959). The Principles of Humane Experimental Technique. London: Methuen.
Ryan, N., Catroppa, C., Godfrey, C., Noble-Haeusslein, L., Shultz, S., O’brien, T., et al. (2016). Social dysfunction after pediatric traumatic brain injury: a translational perspective. Neurosci. Biobehav. Rev. 64, 196–214. doi: 10.1016/j.neubiorev.2016.02.020
Saito, V., and Brandão, M. (2015). The benzodiazepine midazolam acts on the expression of the defensive behavior, but not on the processing of aversive information, produced by exposure to the elevated plus maze and electrical stimulations applied to the inferior colliculus of rats. Neuropharmacology 88, 180–186. doi: 10.1016/j.neuropharm.2014.07.018
Saitoh, A., Makino, Y., Hashimoto, T., Yamada, M., Gotoh, L., Sugiyama, A., et al. (2015). The voltage-gated sodium channel activator veratrine induces anxiogenic-like behaviors in rats. Behav. Brain Res. 292, 316–322. doi: 10.1016/j.bbr.2015.06.022
Saitoh, A., Soda, A., Kayashima, S., Yoshizawa, K., Oka, J., Nagase, H., et al. (2018). A delta opioid receptor agonist, KNT-127, in the prelimbic medial prefrontal cortex attenuates glial glutamate transporter blocker-induced anxiety-like behavior in mice. J. Pharmacol. Sci. 138, 176–183. doi: 10.1016/j.jphs.2018.09.009
Sakurai, K., Zhao, S., Takatoh, J., Rodriguez, E., Lu, J., Leavitt, A., et al. (2016). Capturing and manipulating activated neuronal ensembles with CANE delineates a hypothalamic social-fear circuit. Neuron 92, 739–753. doi: 10.1016/j.neuron.2016.10.015
Salari, A., and Amani, M. (2017). Neonatal blockade of GABA-A receptors alters behavioral and physiological phenotypes in adult mice. Int. J. Dev. Neurosci. 57, 62–71. doi: 10.1016/j.ijdevneu.2017.01.007
Salari, A., Fatehi-Gharehlar, L., Motayagheni, N., and Homberg, J. R. (2016). Fluoxetine normalizes the effects of prenatal maternal stress on depression- and anxiety-like behaviors in mouse dams and male offspring. Behav. Brain Res. 311, 354–367. doi: 10.1016/j.bbr.2016.05.062
Samad, N., Imran, I., Zulfiqar, I., and Bilal, K. (2019). Ameliorative effect of lithium chloride against D-Galactose induced behavioral and memory impairment, oxidative stress and alteration in serotonin function in rats. Pharmacol. Rep. 71, 909–916. doi: 10.1016/j.pharep.2019.04.022
Sanguedo, F., Figueiredo, A., Carey, R., Samuels, R., and Carrera, M. (2017). ERK activation in the prefrontal cortex by acute apomorphine and apomorphine conditioned contextual stimuli. Pharmacol. Biochem. Behav. 159, 76–83. doi: 10.1016/j.pbb.2017.07.011
Sanna, M. D., Ghelardini, C., Thurmond, R. L., Masini, E., and Galeotti, N. (2017). Behavioural phenotype of histamine H4 receptor knockout mice: focus on central neuronal functions. Neuropharmacology 114, 48–57. doi: 10.1016/j.neuropharm.2016.11.023
Santangelo, A., Provensi, G., Costa, A., Blandina, P., Ricca, V., Crescimanno, G., et al. (2017). Brain histamine depletion enhances the behavioural sequences complexity of mice tested in the open-field: partial reversal effect of the dopamine D2/D3 antagonist sulpiride. Neuropharmacology 113, 533–542. doi: 10.1016/j.neuropharm.2016.11.007
Santos, P., Herrmann, A., Benvenutti, R., Noetzold, G., Giongo, F., Gama, C., et al. (2017). Anxiolytic properties of N-acetylcysteine in mice. Behav. Brain Res. 317, 461–469. doi: 10.1016/j.bbr.2016.10.010
Sapozhnikova, T., Borisevich, S., Kireeva, D., Gabdrakhmanova, S., Khisamutdinova, R., Makara, N., et al. (2019). Effects of novel hexahydropyrimidine derivatives as potential ligands of M1 muscarinic acetylcholine receptor on cognitive function, hypoxia-induced lethality and oxidative stress in rodents. Behav. Brain Res. 373:112109. doi: 10.1016/j.bbr.2019.112109
Saré, R., Levine, M., Hildreth, C., Picchioni, D., and Smith, C. (2016). Chronic sleep restriction during development can lead to long-lasting behavioral effects. Physiol. Behav. 155, 208–217. doi: 10.1016/j.physbeh.2015.12.019
Sauce, B., Wass, C., Netrakanti, M., Saylor, J., Schachner, M., and Matzel, L. D. (2015). Heterozygous L1 deficient mice express an autisum-like phenotype. Behav. Brain Res. 292, 432–442. doi: 10.1016/j.bbr.2015.05.040
Schambra, U., Nunley, K., Harrison, T., and Lewis, C. (2016). Consequences of low or moderate prenatal ethanol exposures during gastrulation or neurulation for open field activity and emotionality in mice. Neurotoxicol. Teratol. 57, 39–53. doi: 10.1016/j.ntt.2017.05.001
Scheich, B., Cseko, K., Borbely, E., Abraham, I., Csernus, V., Gaszner, B., et al. (2017). Higher susceptibility of somatostatin 4 receptor gene-deleted mice to chronic stress induced behavioral and neuroendocrine alterations. Neuroscience 346, 320–336. doi: 10.1016/j.neuroscience.2017.01.039
Scheinert, R. B., Haeri, M. H., Lehmann, M. L., and Herkenham, M. (2016). Therapeutic effects of stress programmed lymphocytes transferred to chronically stressed mice. Prog. Neuropsychopharmacol. Biol. Psychiatry 70, 1–7. doi: 10.1016/j.pnpbp.2016.04.010
Schindler, N., Mayer, J., Saenger, S., Gimsa, U., Walz, C., Brenmoehl, J., et al. (2017). Phenotype analysis of male transgenic mice overexpressing mutant IGFBP-2 lacking the Cardin-Weintraub sequence motif: reduced expression of synaptic markers and myelin basic protein in the brain and a lower degree of anxiety-like behaviour. Growth Horm. IGF Res. 33, 1–8. doi: 10.1016/j.ghir.2016.11.003
Scholl, J., Afzal, A., Fox, L., Watt, M., Forster, G., and Scholl, J. (2019). Sex differences in anxiety-like behaviors in rats. Physiol. Behav. 211, 112670–112670. doi: 10.1016/j.physbeh.2019.112670
Schroeder, A., Buret, L., Hill, R., and van Den Buuse, M. (2015). Gene-environment interaction of reelin and stress in cognitive behaviours in mice: implications for schizophrenia. Behav. Brain Res. 287, 304–314. doi: 10.1016/j.bbr.2015.03.063
Screven, L. A., and Dent, M. L. (2018). Preference in female laboratory mice is influenced by social experience. Behav. Processes 157, 171–179. doi: 10.1016/j.beproc.2018.09.011
Serafim, K., Russo, P., Fernandes, C., Gianlorenço, A., and Mattioli, R. (2016). Intra-amygdala microinjections of chlorpheniramine impair memory formation or memory retrieval in anxiety- and fear-mediated models. Brain Res. Bull. 125, 127–133. doi: 10.1016/j.brainresbull.2016.06.006
Shafia, S., Vafaei, A. A., Samaei, S. A., Bandegi, A. R., Rafiei, A., Valadan, R., et al. (2017). Effects of moderate treadmill exercise and fluoxetine on behavioural and cognitive deficits, Hypothalamic-Pituitary-Adrenal Axis dysfunction and alternations in hippocampal BDNF and mRNA expression of apoptosis related proteins in a rat model of post-traumatic stress disorder. Neurobiol. Learn. Memory 139, 165–178. doi: 10.1016/j.nlm.2017.01.009
Sheth, S. K. S., Li, Y., and Shaw, C. A. (2018). Is exposure to aluminium adjuvants associated with social impairments in mice? A pilot study. J. Inorg. Biochem. 181, 96–103. doi: 10.1016/j.jinorgbio.2017.11.012
Shimizu, T., Minami, C., and Mitani, A. (2018). Effect of electrical stimulation of the infralimbic and prelimbic cortices on anxiolytic-like behavior of rats during the elevated plus-maze test, with particular reference to multiunit recording of the behavior-associated neural activity. Behav. Brain Res. 353, 168–175. doi: 10.1016/j.bbr.2018.07.005
Shoji, H., and Miyakawa, T. (2021). Effects of test experience, closed-arm wall color and illumination level on behavior and plasma corticosterone response in an elevated plus maze in male C57BL/6J mice: a challenge against conventional interpretation of the test. Mol. Brain 14:34. doi: 10.1186/s13041-020-00721-2
Sirohi, S., Van Cleef, A., and Davis, J. F. (2017). Binge-like intake of HFD attenuates alcohol intake in rats. Physiol. Behav. 178, 187–195. doi: 10.1016/j.physbeh.2016.10.006
Skupio, U., Tertil, M., Sikora, M., Golda, S., Wawrzczak-Bargiela, A., and Przewlocki, R. (2015). Behavioral and molecular alterations in mice resulting from chronic treatment with dexamethasone: relevance to depression. Neuroscience 286, 141–150. doi: 10.1016/j.neuroscience.2014.11.035
Słupski, W., and Rutkowska, M. (2015). Influence of simvastatin on the anxiolytic effect of diazepam in the elevated plus maze test in rats. Pharmacol. Rep. 67, 39–40. doi: 10.1016/j.pharep.2015.06.121
Smith, C. J. W., Wilkins, K. B., Li, S., Tulimieri, M. T., and Veenema, A. H. (2018). Nucleus accumbens mu opioid receptors regulate context-specific social preferences in the juvenile rat. Psychoneuroendocrinology 89, 56–68. doi: 10.1016/j.psyneuen.2017.12.017
Snyder, A. Z., and Bauer, A. Q. (2019). Mapping structure-function relationships in the brain. Biol. Psychiatry Cogn. Neurosci. Neuroimaging 4, 510–521. doi: 10.1016/j.bpsc.2018.10.005
Sobolewski, M., Anderson, T., Conrad, K., Marvin, E., Klocke, C., Morris-Schaffer, K., et al. (2018). Developmental exposures to ultrafine particle air pollution reduces early testosterone levels and adult male social novelty preference: risk for children’s sex-biased neurobehavioral disorders. Neurotoxicology 68, 203–211. doi: 10.1016/j.neuro.2018.08.009
Socala, K., and Wlaz, P. (2016). Evaluation of the antidepressant- and anxiolytic-like activity of a spinasterol, a plant derivative with TRPV1 antagonistic effects, in mice. Behav. Brain Res. 303, 19–25. doi: 10.1016/j.bbr.2016.01.048
Sorregotti, T., Cipriano, A., Cruz, F., Mascarenhas, D., Rodgers, R., and Nunes-de-Souza, R. (2018). Amygdaloid involvement in the defensive behavior of mice exposed to the open elevated plus-maze. Behav. Brain Res. 338, 159–165. doi: 10.1016/j.bbr.2017.10.022
Sparling, J., Baker, S., and Bielajew, C. (2018). Effects of combined pre- and post-natal enrichment on anxiety-like, social and cognitive behaviours in juvenile and adult rat offspring. Behav. Brain Res. 353, 40–50. doi: 10.1016/j.bbr.2018.06.033
Speight, A., Davey, W., Mckenna, E., and Voigt, J. (2017). Exposure to a maternal cafeteria diet changes open-field behaviour in the developing offspring. Int. J. Dev. Neurosci. 57, 34–40. doi: 10.1016/j.ijdevneu.2016.12.005
Sprowles, J., Hufgard, J., Gutierrez, A., Bailey, R., Jablonski, S., Williams, M., et al. (2017). Differential effects of perinatal exposure to antidepressants on learning and memory, acoustic startle, anxiety and open-field activity in Sprague-Dawley rats. Int. J. Dev. Neurosci. 61, 92–111. doi: 10.1016/j.ijdevneu.2017.06.004
Štefánik, P., Olexová, L., and Kršková, L. (2015). Increased sociability and gene expression of oxytocin and its receptor in the brains of rats affected prenatally by valproic acid. Pharmacol. Biochem. Behav. 131, 42–50. doi: 10.1016/j.pbb.2015.01.021
Stohn, J. P., Martinez, M. E., and Hernandez, A. (2016). Decreased anxiety- and depression-like behaviors and hyperactivity in a type 3 deiodinase-deficient mouse showing brain thyrotoxicosis and peripheral hypothyroidism. Psychoneuroendocrinology 74, 46–56. doi: 10.1016/j.psyneuen.2016.08.021
Stoppel, D. C., and Anderson, M. P. (2017). Hypersociability in the Angelman syndrome mouse model. Exp. Neurol. 293, 137–143. doi: 10.1016/j.expneurol.2017.04.002
Struntz, K., and Siegel, J. (2018). Effects of methamphetamine exposure on anxiety-like behavior in the open field test, corticosterone and hippocampal tyrosine hydroxylase in adolescent and adult mice. Behav. Brain Res. 348, 211–218. doi: 10.1016/j.bbr.2018.04.019
Subramaniam, S., Magen, I., Bove, N., Zhu, C., Lemesre, V., Dutta, G., et al. (2018). Chronic nicotine improves cognitive and social impairment in mice overexpressing wild type α-synuclein. Neurobiol. Dis. 117, 170–180. doi: 10.1016/j.nbd.2018.05.018
Suleymanova, E., Borisova, M., and Vinogradova, L. (2019). Early endocannabinoid system activation attenuates behavioral impairments induced by initial impact but does not prevent epileptogenesis in lithium-pilocarpine status epilepticus model. Epilepsy Behav. 92, 71–78. doi: 10.1016/j.yebeh.2018.12.001
Taherichadorneshin, H., Cheragh-Birjandi, S., Ramezani, S., and Abtahi-Eivary, S. (2017). Comparing sprint and endurance training on anxiety, depression and its relation with brain-derived neurotrophic factor in rats. Behav. Brain Res. 329, 1–5. doi: 10.1016/j.bbr.2017.04.034
Tarland, E., and Brosda, J. (2018). Male rats treated with subchronic PCP show intact olfaction and enhanced interest for a social odour in the olfactory habituation/dishabituation test. Behav. Brain Res. 345, 13–20. doi: 10.1016/j.bbr.2018.02.023
Tartaglione, A. M., Schiavi, S., Calamandrei, G., and Trezza, V. (2019). Prenatal valproate in rodents as a tool to understand the neural underpinnings of social dysfunctions in autism spectrum disorder. Neuropharmacology 159:107477. doi: 10.1016/j.neuropharm.2018.12.024
Tavares, L., Baptista-de-Souza, D., and Canto-de-Souza, A. (2018). Activation of 5-HT2C (but not 5-HT1A) receptors in the amygdala enhances fear-induced antinociception: blockade with local 5-HT2C antagonist or systemic fluoxetine. Neuropharmacology 135, 376–385. doi: 10.1016/j.neuropharm.2018.03.008
Telonis, A., and Margarity, M. (2015). Phobos: a novel software for recording rodents’ behavior during the thigmotaxis and the elevated plus-maze test. Neurosci. Lett. 599, 81–85. doi: 10.1016/j.neulet.2015.05.045
Thompson, R. F. (1994). Behaviorism and neuroscience. Psychol. Rev. 101, 259–265. doi: 10.1037/0033-295x.101.2.259
Thompson, T., Grabowski-Boase, L., and Tarantino, L. M. (2015). Prototypical anxiolytics do not reduce anxiety-like behavior in the open field in C57BL/6J mice. Pharmacol. Biochem. Behav. 133, 7–17. doi: 10.1016/j.pbb.2015.03.011
Tillmann, S., Skibdal, H. E., Christiansen, S. H., Gotzsche, C. R., Hassan, M., Mathe, A. A., et al. (2019). Sustained overexpression of neuropeptide S in the amygdala reduces anxiety-like behavior in rats. Behav. Brain Res. 367, 28–34. doi: 10.1016/j.bbr.2019.03.039
Tillmann, S., and Wegener, G. (2019). Probiotics reduce risk-taking behavior in the Elevated Plus Maze in the Flinders Sensitive Line rat model of depression. Behav. Brain Res. 359, 755–762. doi: 10.1016/j.bbr.2018.08.025
Toma, W., Kyte, S. L., Bagdas, D., Alkhlaif, Y., Alsharari, S. D., Lichtman, A. H., et al. (2017). Effects of paclitaxel on the development of neuropathy and affective behaviors in the mouse. Neuropharmacology 117, 305–315. doi: 10.1016/j.neuropharm.2017.02.020
Torres, L., Danver, J., Ji, K., Miyauchi, J., Chen, D., Anderson, M., et al. (2016). Dynamic microglial modulation of spatial learning and social behavior. Brain Behav. Immun. 55, 6–16. doi: 10.1016/j.bbi.2015.09.001
Tothova, L., Babickova, J., Borbelyova, V., Filova, B., Sebekova, K., and Hodosy, J. (2015). Chronic renal insufficiency does not induce behavioral and cognitive alteration in rats. Physiol. Behav. 138, 133–140. doi: 10.1016/j.physbeh.2014.10.027
Tran, L., and Keele, N. (2016). CaMKIIα knockdown decreases anxiety in the open field and low serotonin-induced upregulation of GluA1 in the basolateral amygdala. Behav. Brain Res. 303, 152–159. doi: 10.1016/j.bbr.2016.01.053
Trofimiuk, E., Wielgat, P., Braszko, J., and Car, H. (2019). Stress and ketamine, bimodal influence on cognitive functions. Behav. Brain Res. 360, 354–364. doi: 10.1016/j.bbr.2018.12.030
Tsatsakis, A., Tyshko, N., Docea, A., Shestakova, S., Sidorova, Y., Petrov, N., et al. (2019). The effect of chronic vitamin deficiency and long term very low dose exposure to 6 pesticides mixture on neurological outcomes—a real-life risk simulation approach. Toxicol. Lett. 315, 96–106. doi: 10.1016/j.toxlet.2019.07.026
Uemura, M., Asano, T., Hikawa, R., Yamakado, H., and Takahashi, R. (2017). Zonisamide inhibits monoamine oxidase and enhances motor performance and social activity. Neurosci. Res. 124, 25–32. doi: 10.1016/j.neures.2017.05.008
Underwood, B. J. (1966). Problems in Experimental Design and Inference: Woorkbook for the First Course in Experimental Psychology, New York, NY: Meredith Publishing Compay.
Upadhyay, P., Sadhu, A., Singh, P., Agrawal, A., Ilango, K., Purohit, S., et al. (2018). Revalidation of the neuroprotective effects of a United States patented polyherbal formulation on scopolamine induced learning and memory impairment in rats. Biomed. Pharmacother. 97, 1046–1052. doi: 10.1016/j.biopha.2017.11.008
Vázquez-León, P., Campos-Rodríguez, C., Gonzalez-Pliego, C., and Miranda-Páez, A. (2018). Differential effects of cholecystokinin (CCK-8) microinjection into the ventrolateral and dorsolateral periaqueductal gray on anxiety models in Wistar rats. Horm. Behav. 106, 105–111. doi: 10.1016/j.yhbeh.2018.10.003
Vázquez-León, P., Mendoza-Ruiz, L., Juan, E., Chamorro-Cevallos, G., and Miranda-Páez, A. (2017). Analgesic and anxiolytic effects of [Leu31,Pro34]-neuropeptide Y microinjected into the periaqueductal gray in rats. Neuropeptides 66, 81–89. doi: 10.1016/j.npep.2017.10.001
Van Camp, G., Cigalotti, J., Bouwalerh, H., Mairesse, J., Gatta, E., Palanza, P., et al. (2018). Consequences of a double hit of stress during the perinatal period and midlife in female rats: mismatch or cumulative effect. Psychoneuroendocrinology 93, 45–55. doi: 10.1016/j.psyneuen.2018.04.004
van Den Boom, B., Pavlidi, P., Wolf, C., Mooij, A., and Willuhn, I. (2017). Automated classification of self-grooming in mice using open-source software. J. Neurosci. Methods 289, 48–56. doi: 10.1016/j.jneumeth.2017.05.026
Varghese, R., Majumdar, A., Kumar, G., and Shukla, A. (2018). Rats exposed to 2.45 GHZ of non ionizing radiation exhibit behavioral changes with increased brain expression of apoptotic caspase 3. Pathophysiology 25, 19–30. doi: 10.1016/j.pathophys.2017.11.001
Verpeut, J. L., DiCicco-Bloom, E., and Bello, N. T. (2016). Ketogenic diet exposure during the juvenile period increases social behaviors and forebrain neural activation in adult Engrailed 2 null mice. Physiol. Behav. 161, 90–98. doi: 10.1016/j.physbeh.2016.04.001
Victoriano, G., Santos-Costa, N., Mascarenhas, D., Nunes-de-Souza, R., and Victoriano, G. (2019). Inhibition of the left medial prefrontal cortex (mPFC) prolongs the social defeat-induced anxiogenesis in mice: attenuation by NMDA receptor blockade in the right mPFC. Behav. Brain Res. 378:112312. doi: 10.1016/j.bbr.2019.112312
Vieira, J., Bassani, T., Santiago, R., de O. Guaita, G., Zanoveli, J., Da Cunha, C., et al. (2019). Anxiety-like behavior induced by 6-OHDA animal model of Parkinson’s disease may be related to a dysregulation of neurotransmitter systems in brain areas related to anxiety. Behav. Brain Res. 371:111981. doi: 10.1016/j.bbr.2019.111981
Vogt, M. A., Mallien, A. S., Pfeiffer, N., Inta, I., Gass, P., and Inta, D. (2016). Minocycline does not evoke anxiolytic and antidepressant-like effects in C57BL/6 mice. Behav. Brain Res. 301, 96–101. doi: 10.1016/j.bbr.2015.12.015
Wąsik, A., Białoń, M., Żarnowska, M., and Antkiewicz-Michaluk, L. (2019). Comparison of the effects of 1MeTIQ and olanzapine on performance in the elevated plus maze test and monoamine metabolism in the brain after ketamine treatment. Pharmacol. Biochem. Behav. 181, 17–27. doi: 10.1016/j.pbb.2019.04.002
Walia, V., Garg, C., and Garg, M. (2018). Anxiolytic-like effect of pyridoxine in mice by elevated plus maze and light and dark box: evidence for the involvement of GABAergic and no SGCCGMP pathway. Pharmacol. Biochem. Behav. 173, 96–106. doi: 10.1016/j.pbb.2018.06.001
Walia, V., Garg, C., and Garg, M. (2019a). Lithium potentiated, pyridoxine abolished and fluoxetine attenuated the anxiolytic effect of diazepam in mice. Brain Res. Bull. 150, 343–353. doi: 10.1016/j.brainresbull.2019.06.008
Walia, V., Garg, C., and Garg, M. (2019b). NO-SGC-CGMP signaling influence the anxiolytic like effect of lithium in mice in light and dark box and elevated plus maze. Brain Res. 1704, 114–126. doi: 10.1016/j.brainres.2018.10.002
Wang, G., Cao, J., and Wang, X. (2019). Effects of ethanol extract from Bidens pilosa L. on spontaneous activity, learning and memory in aged rats. Exp. Gerontol. 125:110651. doi: 10.1016/j.exger.2019.110651
Wang, A., Dai, Z., Gong, G., Zhou, C., and He, Y. (2014). Understanding structural-functional relationships in the human brain: a large-scale network perspective. Neuroscientist 21, 290–305. doi: 10.1177/1073858414537560
Wang, S., Feng, D., Li, Y., Wang, Y., Sun, X., Li, X., et al. (2018). The different baseline characteristics of cognitive behavior test between Mongolian gerbils and rats. Behav. Brain Res. 352, 28–34. doi: 10.1016/j.bbr.2017.09.042
Wang, L., He, Z., Zhu, Z., Yuan, W., Cai, W., Li, L., et al. (2019). The serotonin system in the hippocampus CA3 involves in effects of CSDS on social recognition in adult female mandarin voles (Microtus mandarinus). Prog. Neuro-Psychopharmacol. Biol. Psychiatry 95:109704. doi: 10.1016/j.pnpbp.2019.109704
Wang, H., Pei, D., Yang, R., Wan, C., Ye, Y., Peng, S., et al. (2019). Prenatal maternal vaginal inflammation increases anxiety and alters HPA axis signalling in adult male mice. Int. J. Dev. Neurosci. 75, 27–35. doi: 10.1016/j.ijdevneu.2019.04.001
Wang, R., Xu, X., Weng, H., Yan, S., and Sun, Y. (2016a). Effects of early pubertal exposure to di-(2-ethylhexyl) phthalate on social behavior of mice. Horm. Behav. 80, 117–124. doi: 10.1016/j.yhbeh.2016.01.012
Wang, R., Xu, X., and Zhu, Q. (2016b). Pubertal exposure to di-(2-ethylhexyl) phthalate influences social behavior and dopamine receptor D2 of adult female mice. Chemosphere 144, 1771–1779. doi: 10.1016/j.chemosphere.2015.10.062
Wang, Y., Yu, Y., Tsai, M., and Huang, A. (2018a). Motor function in an animal model with ouabain-induced bipolar disorder and comorbid anxiety behavior. Psychiatry Res. 268, 508–513. doi: 10.1016/j.psychres.2018.07.031
Wang, Y., Zhao, S., Liu, X., Zheng, Y., Li, L., and Meng, S. (2018b). Oxytocin improves animal behaviors and ameliorates oxidative stress and inflammation in autistic mice. Biomed. Pharmacother. 107, 262–269. doi: 10.1016/j.biopha.2018.07.148
Washington, J., Kumar, U., Medel-Matus, J., Shin, D., Sankar, R., and Mazarati, A. (2015). Cytokine-dependent bidirectional connection between impaired social behavior and susceptibility to seizures associated with maternal immune activation in mice. Epilepsy Behav. 50, 40–45. doi: 10.1016/j.yebeh.2015.05.040
Wei, D., Allsop, S., Tye, K., and Piomelli, D. (2017). Endocannabinoid signaling in the control of social behavior. Trends Neurosci. 40, 385–396. doi: 10.1016/j.tins.2017.04.005
Wen, D., Zhao, P., Hui, R., Wang, J., Shen, Q., Gong, M., et al. (2017). Hydrogen-rich saline attenuates anxiety-like behaviors in morphine-withdrawn mice. Neuropharmacology 118, 199–208. doi: 10.1016/j.neuropharm.2017.03.029
Wensheng, Y., Jie, K., Guoliang, Z., Shuangcheng, L., Yunxiao, K., Lei, W., et al. (2015). The effects of gonadectomy and binge-like ethanol exposure during adolescence on open field behaviour in adult male rats. Neurosci. Lett. 604, 52–57. doi: 10.1016/j.neulet.2015.07.039
White, S. R., Amarante, L. M., Kravitz, A. V., and Laubach, M. (2019). The future is open: open-source tools for behavioral neuroscience research. eNeuro 6:ENEURO.0223–19.2019. doi: 10.1523/ENEURO.0223-19.2019
Wille-Bille, A., Miranda-Morales, R. S., Pucci, M., Bellia, F., D’Addario, C., and Pautassi, R. M. (2018). Prenatal ethanol induces an anxiety phenotype and alters expression of dynorphin & nociceptin/orphanin FQ genes. Prog. Neuropsychopharmacol. Biol. Psychiatry 85, 77–88. doi: 10.1016/j.pnpbp.2018.04.005
Winther, G., Eskelund, A., Bay-Richter, C., Elfving, B., Muller, H. K., Lund, S., et al. (2019). Grandmaternal high-fat diet primed anxiety-like behaviour in the second generation female offspring. Behav. Brain Res. 359, 47–55. doi: 10.1016/j.bbr.2018.10.017
Wscieklica, T., de Barros Viana, M., Le Sueur Maluf, L., Pouza, K., Spadari, R., and Céspedes, I. (2016). Alcohol consumption increases locomotion in an open field and induces Fos-immunoreactivity in reward and approach/withdrawal-related neurocircuitries. Alcohol 50, 73–82. doi: 10.1016/j.alcohol.2015.11.005
Wu, H., Wang, X., Gao, J., Liang, S., Hao, Y., Sun, C., et al. (2017). Fingolimod (FTY720) attenuates social deficits, learning and memory impairments, neuronal loss and neuroinflammation in the rat model of autism. Life Sci. 173, 43–54. doi: 10.1016/j.lfs.2017.01.012
Xiao, X., Xu, X., Li, F., Xie, G., and Zhang, T. (2019). Anti-inflammatory treatment with β- asarone improves impairments in social interaction and cognition in MK-801 treated mice. Brain Res. Bull. 150, 150–159. doi: 10.1016/j.brainresbull.2019.05.017
Xu, H., Liu, L., Tian, Y., Wang, J., Li, J., Zheng, J., et al. (2019). A disinhibitory microcircuit mediates conditioned social fear in the prefrontal cortex. Neuron 102, 668–682.e5. doi: 10.1016/j.neuron.2019.02.026
Xu, X., Zhang, H., Shou, X., Li, J., Jing, W., Zhou, Y., et al. (2015). Prenatal hyperandrogenic environment induced autistic-like behavior in rat offspring. Physiol. Behav. 138, 13–20. doi: 10.1016/j.physbeh.2014.09.014
Yamamoto, H., Kamegaya, E., Hagino, Y., Takamatsu, Y., Sawada, W., Matsuzawa, M., et al. (2017). Loss of GluN2D subunit results in social recognition deficit, social stress, 5-HT2C receptor dysfunction and anhedonia in mice. Neuropharmacology 112, 188–197. doi: 10.1016/j.neuropharm.2016.07.036
Yang, Y., Qin, J., Chen, W., Sui, N., Chen, H., and Li, M. (2015). Behavioral and pharmacological investigation of anxiety and maternal responsiveness of postpartum female rats in a pup elevated plus maze. Behav. Brain Res. 292, 414–427. doi: 10.1016/j.bbr.2015.07.010
Yang, Z., Zhao, Y., Li, Q., Shao, Y., Yu, X., Cong, W., et al. (2019). Developmental exposure to mercury chloride impairs social behavior in male offspring dependent on genetic background and maternal autoimmune environment. Toxicol. Appl. Pharmacol. 370, 1–13. doi: 10.1016/j.taap.2019.03.006
Yau, S. Y., Chiu, C., Vetrici, M., and Christie, B. R. (2016). Chronic minocycline treatment improves social recognition memory in adult male Fmr1 knockout mice. Behav. Brain Res. 312, 77–83. doi: 10.1016/j.bbr.2016.06.015
Yeshurun, S., Rogers, J., Short, A., Renoir, T., Pang, T., and Hannan, A. (2017). Elevated paternal glucocorticoid exposure modifies memory retention in female offspring. Psychoneuroendocrinology 83, 9–18. doi: 10.1016/j.psyneuen.2017.05.014
Yeung, M., Treit, D., and Dickson, C. (2016). Ventral hippocampal histamine increases the frequency of evoked theta rhythm but produces anxiolytic-like effects in the elevated plus maze. Neuropharmacology 106, 146–155. doi: 10.1016/j.neuropharm.2015.09.024
Yoshimi, N., Futamura, T., and Hashimoto, K. (2015). Improvement of dizocilpine-induced social recognition deficits in mice by brexpiprazole, a novel serotonin-dopamine activity modulator. Eur. Neuropsychopharmacol. 25, 356–364. doi: 10.1016/j.euroneuro.2014.12.014
Yu, C., Mei, X., Zheng, Y., and Xu, D. (2015). Taurine zinc solid dispersions protect against cold restraint stress-induced gastric ulceration by upregulating HSP70 and exerting an anxiolytic effect. Eur. J. Pharmacol. 762, 63–71. doi: 10.1016/j.ejphar.2015.05.033
Yuan, H., Ni, X., Zheng, M., Han, X., Song, Y., and Yu, M. (2019). Effect of catalpol on behavior and neurodevelopment in an ADHD rat model. Biomed. Pharmacother. 118:109033. doi: 10.1016/j.biopha.2019.109033
Zaccarelli-Magalhães, J., Sandinib, T. M., Ramos de Abreu, G., Petrocelli, B. M., Moreira, N., Reis-Silva, T. M., et al. (2019). Prolonged exposure of rats to varenicline increases anxiety and alters serotonergic system, but has no effect on memory. Pharmacol. Biochem. Behav. 181, 1–8. doi: 10.1016/j.pbb.2019.03.009
Zagorácz, O., Kovács, A., László, K., Ollmann, T., Péczely, L., and Lénárd, L. (2015). Effects of direct QRFP-26 administration into the medial hypothalamic area on food intake in rats. Brain Res. Bull. 118, 58–64. doi: 10.1016/j.brainresbull.2015.09.004
Zahra, A., Jiang, J., Chen, Y., Long, C., and Yang, L. (2018). Memantine rescues prenatal citalopram exposure-induced striatal and social abnormalities in mice. Exp. Neurol. 307, 145–154. doi: 10.1016/j.expneurol.2018.06.003
Zare, Z., Tehrani, M., Rezaei, N., Dana Ghalebarzand, B., and Mohammadi, M. (2019). Anxiolytic activity of paraoxon is associated with alterations in rat brain glutamatergic system. Neurotoxicol. Teratol. 71, 32–40. doi: 10.1016/j.ntt.2018.12.001
Zhan, Y. (2015). Theta frequency prefrontal-hippocampal driving relationship during free exploration in mice. Neuroscience 300, 554–565. doi: 10.1016/j.neuroscience.2015.05.063
Zhang, C., Chua, B. E., Yang, A., Shabanpoor, F., Hossain, M. A., Wade, J. D., et al. (2015). Central relaxin-3 receptor (RXFP3) activation reduces elevated, but not basal, anxiety-like behaviour in C57BL/6J mice. Behav. Brain Res. 292, 125–132. doi: 10.1016/j.bbr.2015.06.010
Zhang, H., Li, H., Dai, Y., Xu, X., Han, S., Zhang, R., et al. (2015). Electro-acupuncture improves the social interaction behavior of rats. Physiol. Behav. 151, 485–493. doi: 10.1016/j.physbeh.2015.08.014
Zhang, M., Ji, M., Zhao, Q., Jia, M., Qiu, L., Yang, J., et al. (2015). Neurobehavioural abnormalities induced by repeated exposure of neonatal rats to sevoflurane can be aggravated by social isolation and enrichment deprivation initiated after exposure to the anaesthetic. Br. J. Anaesth. 115, 752–760. doi: 10.1093/bja/aev339
Zhang, W., Smolik, C., Barba-Escobedo, P., Gamez, M., Sanchez, J., Javors, M., et al. (2015). Acute dietary tryptophan manipulation differentially alters social behavior, brain serotonin and plasma corticosterone in three inbred mouse strains. Neuropharmacology 90, 1–8. doi: 10.1016/j.neuropharm.2014.10.024
Zhang, K., and Yao, L. (2018). The anxiolytic effect of Juniperus virginiana l. essential oil and determination of its active constituents. Physiol. Behav. 189, 50–58. doi: 10.1016/j.physbeh.2018.01.004
Zhang, N., Zhang, L., Feng, L., and Yao, L. (2016). The anxiolytic effect of essential oil of cananga odorata exposure on mice and determination of its major active constituents. Phytomedicine 23, 1727–1734. doi: 10.1016/j.phymed.2016.10.017
Zhou, H., Yu, C., Wang, L., Yang, Y., Mao, R., Zhou, Q., et al. (2015). NMDA and D1 receptors are involved in one-trial tolerance to the anxiolytic-like effects of diazepam in the elevated plus maze test in rats. Pharmacol. Biochem. Behav. 135, 40–45. doi: 10.1016/j.pbb.2015.05.009
Zhou, H., Zhao, W., Ye, L., Chen, Z., and Cui, Y. (2018). Postnatal low-concentration arsenic exposure induces autism-like behavior and affects frontal cortex neurogenesis in rats. Environ. Toxicol. Pharmacol. 62, 188–198. doi: 10.1016/j.etap.2018.07.012
Zilkha, N., Kuperman, Y., and Kimchi, T. (2017). High-fat diet exacerbates cognitive rigidity and social deficiency in the BTBR mouse model of autism. Neuroscience 345, 142–154. doi: 10.1016/j.neuroscience.2016.01.070
Zimcikova, E., Simko, J., Karesova, I., Kremlacek, J., and Malakova, J. (2017). Behavioral effects of antiepileptic drugs in rats: are the effects on mood and behavior detectable in open-field test. Seizure 52, 35–40. doi: 10.1016/j.seizure.2017.09.015
Zoeram, S. M., Salmani, M. E., Lashkarbolouki, T., and Goudarzi, I. (2019). Hippocampal orexin receptor blocking prevented the stress induced social learning and memory deficits. Neurobiol. Learn. Memory 157, 12–23. doi: 10.1016/j.nlm.2018.11.009
Keywords: light/dark test, open field, elevated plus maze, three chamber social interaction test, Lux/lighting, behavioral neuroscience tests, rodent pre-clinical screening/testing, ethologically motivated controls
Citation: Neuwirth LS, Verrengia MT, Harikinish-Murrary ZI, Orens JE and Lopez OE (2022) Under or Absent Reporting of Light Stimuli in Testing of Anxiety-Like Behaviors in Rodents: The Need For Standardization. Front. Mol. Neurosci. 15:912146. doi: 10.3389/fnmol.2022.912146
Received: 04 April 2022; Accepted: 21 June 2022;
Published: 17 August 2022.
Edited by:
Stephan C. F. Neuhauss, University of Zurich, SwitzerlandReviewed by:
Jonathan Cueto-Escobedo, Universidad Veracruzana, MexicoMeritxell Llorca-Torralba, University of Cádiz, Spain
Copyright © 2022 Neuwirth, Verrengia, Harikinish-Murrary, Orens and Lopez. This is an open-access article distributed under the terms of the Creative Commons Attribution License (CC BY). The use, distribution or reproduction in other forums is permitted, provided the original author(s) and the copyright owner(s) are credited and that the original publication in this journal is cited, in accordance with accepted academic practice. No use, distribution or reproduction is permitted which does not comply with these terms.
*Correspondence: Lorenz S. Neuwirth, neuwirthl@oldwestbury.edu