- 1Nantong Laboratory of Development and Diseases, Key Laboratory of Neuroregeneration of Jiangsu and Ministry of Education, School of Life Science, Second Affiliated Hospital, Co-innovation Center of Neuroregeneration, Nantong University, Nantong, China
- 2Medical School, Nantong University, Nantong, China
Vessels and nerves are closely associated in anatomy as well as functions. Accumulating evidences have demonstrated that axon-guiding signals may affect endothelial cells migration and path finding, which is crucial for the patterning of both the complex vascular network and neural system. However, studies regarding the functional overlap between vascular and neuronal orchestrating are still incomplete. Semaphorin6D (Sema6D) belongs to the Semaphorin family and has been identified as an important regulating factor in diverse biological processes. Its roles in vascular development are still unclear. Here, we confirmed that sema6D is enriched in neural system and blood vessels of zebrafish embryos by in situ hybridization. Then, the deficiency of sema6D caused by specific antisense morpholino-oligonucleotides (MO) led to dramatic path finding defects in both intersegmental vessels (ISVs) and primary motor neurons (PMNs) of spinal cord in zebrafish embryos. Furthermore, these defective phenotypes were confirmed in F0 generation of sema6D knockouts and rescue experiments by overexpression of sema6D mRNA in sema6D morphants. These data collectively indicate that sema6D regulates zebrafish vascular patterning and motor neuronal axon growth in the spinal cord, which might be of great therapeutical use to regulate vessel and nerve guidance in the relevant diseases that affect both systems.
Introduction
Vascular formation is an early and essential process during the development of vertebrate embryos (Nikolova and Lammert, 2003). Vascular endothelial cells assemble into tubes and then couple into an initial vascular pattern. The stereotypical wiring pattern of vessels is essential for its functions and its abnormality is inextricably related to a wide range of diseases, including atherosclerosis, hypertension, aneurysms, diabetic retinopathy, aneurysms, and tumor (Carmeliet, 2003). Although studies on angiogenesis have made extraordinary progress, the detailed regulatory mechanisms of vascular patterning remain to be fully explored (Patan, 2000; Makanya et al., 2009; Uccelli et al., 2019). Vessels and nerves are closely associated in anatomy as well as functions. Increasing reports have demonstrated that axon-guiding signals may affect endothelial cells migration and path finding, which is crucial for the development of both the vascular and the nervous systems. However, studies regarding the functional overlap between vascular and neuronal pathways are still limited and need advanced research (Luo et al., 1993; Kolodkin and Tessier-Lavigne, 2011).
Semaphorins that are expressed in most organs and tissues were originally described as axon guidance in the hard wiring of the nervous systems (Bussolino et al., 2006; Suchting et al., 2006). However, increasing reports attested to the significance of Semaphorinsin the development and regulation outside the neuron system (Neufeld and Kessler, 2008; Capparuccia and Tamagnone, 2009). Members of Semaphorins have been found to regulate the cells motility during the development of nervous (Sema3A, 3F, 4D, 6C, 7A), immune (Sema4D), reproductive (Sema3), cancer (Sema3A, 4D) and vascular systems (Sema3A, 3E, 4D; Gherardi et al., 2004; Gu et al., 2005; Sakurai et al., 2012; Acker et al., 2018; Elder et al., 2018; Ferreira et al., 2018). Semaphorins6D (Sema6D) is a member of Semaphorin family and has been identified as an important regulating factor in the development of the spinal cord, optic nerve, heart, and tumor (Toyofuku et al., 2004a; Yazdani and Terman, 2006; Yoshida et al., 2006; Leslie et al., 2011; Peng et al., 2016). Additionally, Sema6D controls endothelial cell migration during heart development, which may imply its potential role in vascular development (Toyofuku et al., 2004a). However, there is so far no data proving the pro-angiogenic effects of Sema6D yet.
Zebrafish intersegmental vessels (ISVs) are an excellent system to trace cell behavior and morphology in vivo by expressing the fluorescent protein in transparent embryos (Ellertsdóttir et al., 2010). To further explore the function of sema6D in vertebrate development, we investigated the sequence homology, embryonic expression pattern, and function of sema6D in zebrafish. The results present in this study have shown that sema6D regulates zebrafish vascular patterning and motor neuronal axon growth in spinal cord. The findings might serve as a ground work for studying the molecular mechanism of neurovascular communication, and might be of therapeutically use to guide vessels and nerves in the relevant diseases that affect both systems.
Materials and Methods
Zebrafish Husbandry and Breeding
The study was conducted conforming to the local institutional laws and the Chinese law for the protection of animals. All adult zebrafish (Dario rerio) were maintained under standard conditions in accordance with our previous protocols (Huang et al., 2013; Xu et al., 2014). The AB/WT, Tg(kdrl:ras-mCherry) and Tg(mnx1:EGFP) zebrafish used in this article have been described previously (Nicoli et al., 2012; Jao et al., 2013). Zebrafish embryos after 24 hpf were treated with 0.2 mM 1-phenyl-2-thio-urea to prevent pigment formation.
Phylogenetic Analysis
The zebrafish sema6D protein sequences (NP_998164.3) and zebrafish sema6dl sequences (XP_005173705.1) together with other nine species, including Bos taurus (XP_024853302.1), Gallus gallus (XP_040562440.1), Homo sapiens (XP_024305841.1), Mus musculus (XP_030105194.1), Oryctolaguscuniculus (XP_017203544.1), Oryziaslatipes (XP_011486693.1), Rattus norvegicus (XP_038960910.1), Sus scrofa (XP_020951762.1), and Xenopus tropicalis (XP_031753477.1) were got from NCBI and used for phylogenetic analysis. The alignments of these sequences were constructed by MEGA7and the phylogenetic tree was constructed by ML.
RNA Isolation, Reverse Transcription (RT), Polymerase Chain Reaction (PCR), Quantitative RT-PCR, and RNA Probe Transcription
Total RNA of zebrafish embryos at various stages was extracted with TRizol according to the manufacturer’s instruction (Invitrogen, Waltham, MA, USA) and genomic contaminations were removed by DNaseI. Quantity of isolated RNA was verified using gel electrophoresis and Nanodrop, followed by cDNA synthesis using Transcriptor First Strand cDNA Synthesis Kit (Roche), and then was stored at −20°C.
Primers for PCR were designed by the Primer Premier six software and listed as following:
sema6D-QF: 5’-CCTCCTCCTATTCCTCTTCTGTT-3’;
sema6D-QR: 5’-ACTACGGTGCGGTTCTTATGA-3’;
ef1a–QF: 5’-GAGTTGTGCCGTACATCAG-3’;
ef1a–QR: 5’-CGTGAGAGTACATGGTCATG-3’.
Quantitative RT-PCR was conducted in a total 20 μl reaction volume with 10 μl SYBR premix (TIANGEN). The relative RNA amounts were calculated with the comparative CT (2-DDCT) method and normalized with elongation factor 1-alpha (ef1a) as the reference. Whole-mount in situ hybridization (WISH) with antisense RNA probes was synthesized as described previously (Wang et al., 2016). The cDNA fragments used for sema6D RNA probe transcription as templates were amplified using the forward primer 5’-CGACGGCTATCACTTCACTCT-3’and reverse primer 5’-TGGAACATTCTGACGGCTCTT-3’. Then a 548bp sequence of sema6D was inserted into pGEM-T-easy vector. Digxigenin (DIG)-labeled sense and antisense probes were performed from the linearized pGEM-T-easy plasmids using the DIG RNA Labeling Kit (Roche).
Whole Mount In situ Hybridization
Whole-mount in situ hybridization (WISH) was performed according to our previous procedures (Huang et al., 2013). Digoxigenin-labeled antisense probes were constructed as described above. Zebrafish embryos without pigment at different developmental stages were collected and fixed with 4% PFA overnight at 4°C. After incubated with the probe overnight, an alkaline phosphatase-conjugated antibody against digoxigenin and AP-substrate NBT/BCIP solution (Roche, Switzerland) was used to detect the digoxigenin-labeled RNA probe.
Morpholino and mRNA Injections
Splicing-blocking Morpholino (5’- TGTGAGCTGAGTGAATGCAGACCT -3’) that was specific for sema6D gene was synthesized by Gene Tools. The Morpholino was diluted to 0.3 mM with RNase-free water. The single cell stage embryos of Tg(kdrl:ras-mCherry) and Tg(mnx1:EGFP) zebrafish were obtained for microinjections as described previously (Wang et al., 2016). Then, the embryos were raised in E3 medium at 28.5°C for following imaging.
sgRNA/Cas9 mRNA Synthesis and Injections
Cas9 mRNA was obtained by in vitro transcription with the linearized plasmid pXT7-Cas9 according to the procedure previously described (Nakayama et al., 2013). The sema6D guide RNA (gRNA; 5’-GGCGTGGCAGAAGTAATGAGTGG-3’) was designed and synthesized followed the previously reported (Chang et al., 2013). Transgenic zebrafish lines Tg(kdrl:ras-mCherry) and Tg(mnx1:EGFP) were natural mated to obtain embryos for microinjection. One to two-cell stage zebrafish embryos were injected with 2–3 nl of a solution containing 250 ng/μl Cas9 mRNA and 15 ng/μl sgRNA (Gong et al., 2017). At 72 hpf, 10 zebrafish embryos were randomly collected and mixed for genomic DNA extraction according to the previous methods (Gong et al., 2017). Then, the amplicons from the genomic DNA were cloned into pGEM-T-easy vector and after transformation, 50 clones were selected randomly for sequencing. The inserted fragments were sequenced by using the forward primer 5’-CCTGTGCATATAGATTGTTG-3’ and reverse primer 5’-AAGTCTACAGACAGTAACG-3’. The mutation efficiency of Crispr-Cas9 was then calculated based on the sequencing results (Wu et al., 2018).
Rescue Experiments
Full-length and truncated coding sequences of sema6D were synthesized and inserted into PCS2+ vector as templates for in vitro transcription. The mRNA synthesis was carried out by using the mMESSAGEmMACHINESp6 Ultra Kit (Ambion) and purified with the MEGAclearTM Transcription Clean-Up Kit (Ambion) before the injection. Finally, 2 nl capped mRNA was co-injected with sema6D Mo into one-cell stage embryos. The fli1a:sema6D plasmid was constructed by LR recombination as described in the LifetechMultiste Gateway Manual (Life Technologies, Carls-bad, CA, USA). Then, the construct was injected into one cell stage embryos of Tg(mnx1:EGFP::kdrl:ras-mcherry) zebrafish for tissue specific rescue experiments (1 ng per embryo).
Microscopy and Statistical Analysis
After being anesthetized with tricaine, the zebrafish embryos were mounted in 0.8% low melt agarose and then photographed by Leica TCS-SP5 LSM confocal microscope. For the in situ hybridization, Photographs were taken using an Olympus stereomicroscope MVX10. Statistical analyses were performed by one-way analysis of variance (ANOVA) and the Mann-Whitney test. Statistical differences were considered significant for P-values <0.05.
Results
sema6D Gene Is Evolutionarily Conserved in Vertebrates
To analyze the homology of zebrafish sema6D with other homologous genes, the multiple alignments and phylogenetic analyses of sema6D/sema6Dl were performed with species including Danio rerio, Bostaurus, Gallus gallus, Homo sapiens, Musmusculus, Oryctolaguscuniculus, Oryziaslatipes, Rattusnorvegicus, Sus scrofa, and Xenopus tropicalis. As shown in Figures 1A,B, the sema6D proteins are significantly conserved during evolution, especially for the sema domain, suggesting their important functions. In addition, zebrafish sema6D was clustered in a separate clade with Oryziaslatipes and was close to Xenopus tropicalis in the phylogenetic tree constructed by complete amino acid sequences of all the sema6D proteins above (Figure 1C). Interestingly, the sema6Dl, a sema6D isoform gene in zebrafish, was clustered with sema6D from Oryziaslatipes, suggesting it may not be functionally related to sema6D in zebrafish.
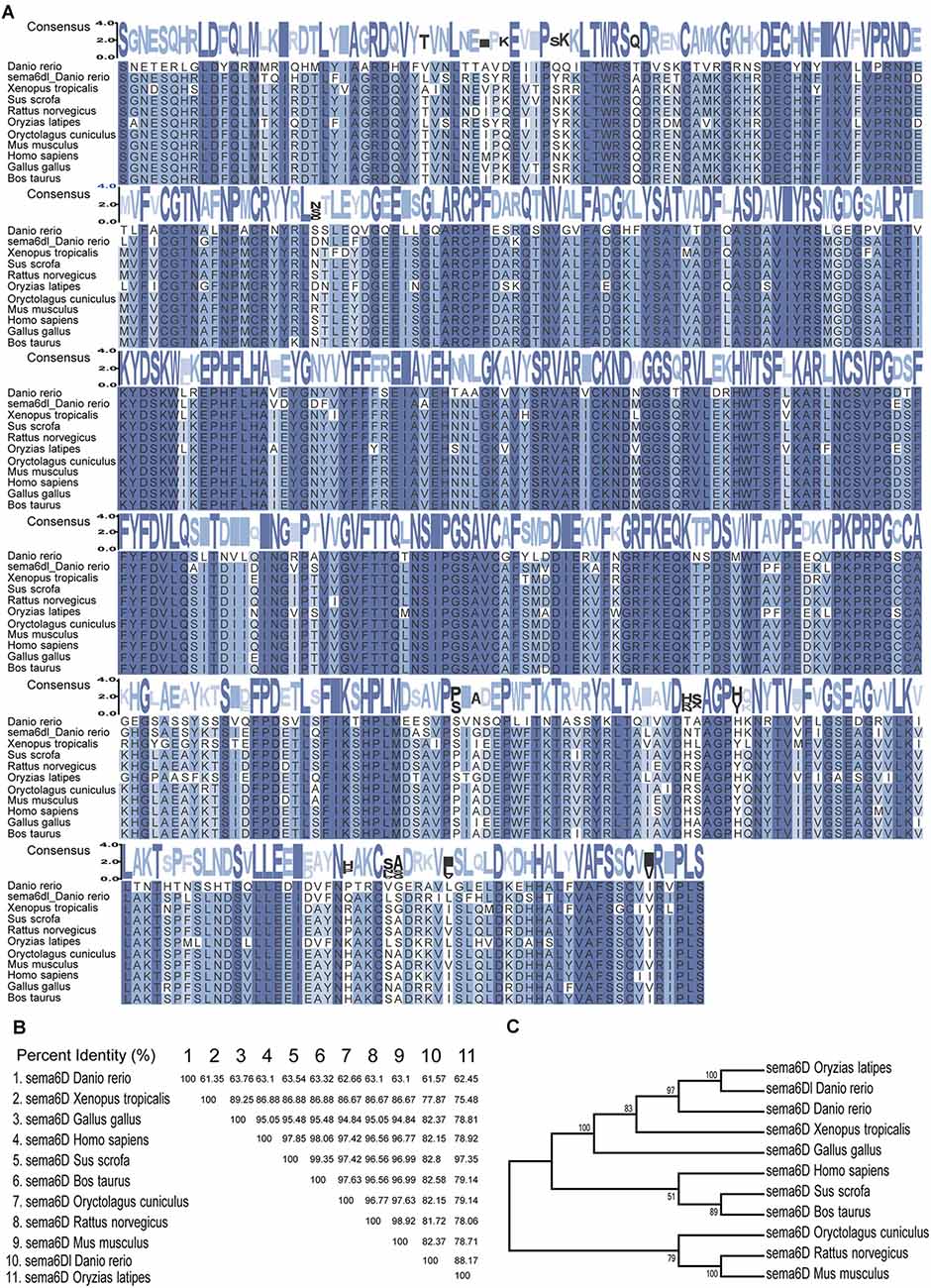
Figure 1. Sema6D Is highly conserved during evolution. (A) Alignment of the amino acid sequences of the sema domain of sema6D/sema6Dl in Danio rerio, Bostaurus, Gallus gallus, Homo sapiens, Musmusculus, Oryctolaguscuniculus, Oryziaslatipes, Rattusnorvegicus, Sus scrofa, and Xenopus tropicalis. Consensus values indicate the similarity of amino acid sequence, and the greater the similarity. These protein sequences were aligned using MEGA7 software and edited by TBtools. (B) The amino acid sequence similarity (%) of sema domain in above species. (C) Phylogenetic tree of the sema6D protein.
Expression of sema6D Genes in Zebrafish
To explore the roles of sema6D during zebrafish embryonic development, the expression level of sema6D was studied using QRT-PCR and WISH. According to QRT-PCR results, sema6D exhibited steady expression from 24 hpf to 96 hpf, with the highest level at 72 hpf, followed by 96 hpf (Figure 2A). Then, the spatial expression pattern of sema6D in early embryonic development was further studied by WISH. From 24 hpf to 48 hpf, sema6D was mainly expressed in the nervous system and also in the blood vessels (Figures 2B–D). Its expression in the nervous system is much restricted to the brain and head structures, being very weak in the spinal cord. Its expression in the vascular system was mainly observed in the ISVs. To further analyze the expression of sema6D in the zebrafish vessels, the endothelial cells from Tg(fli1a:EGFP) were sorted for RT-PCR (Figure 2E). The results showed that both fli1a and sema6D were detected in the selected endothelial cells, which was consistent with the previously reported single-cell RNA sequencing data of zebrafish endothelial cells (Shi et al., 2020; Figure 2F; Supplementary Table S1). Besides, mef2aa, which is specifically expressed in somite and heart, was chosen as a negative control to validate the purity of selected cells (Lv et al., 2017). The results showed that no mef2aa signals were detected in the sorted EGFP-positive cells (Figure 2F). Taken together, these results suggested that sema6D might participate in the development of zebrafish vessels and nerves.
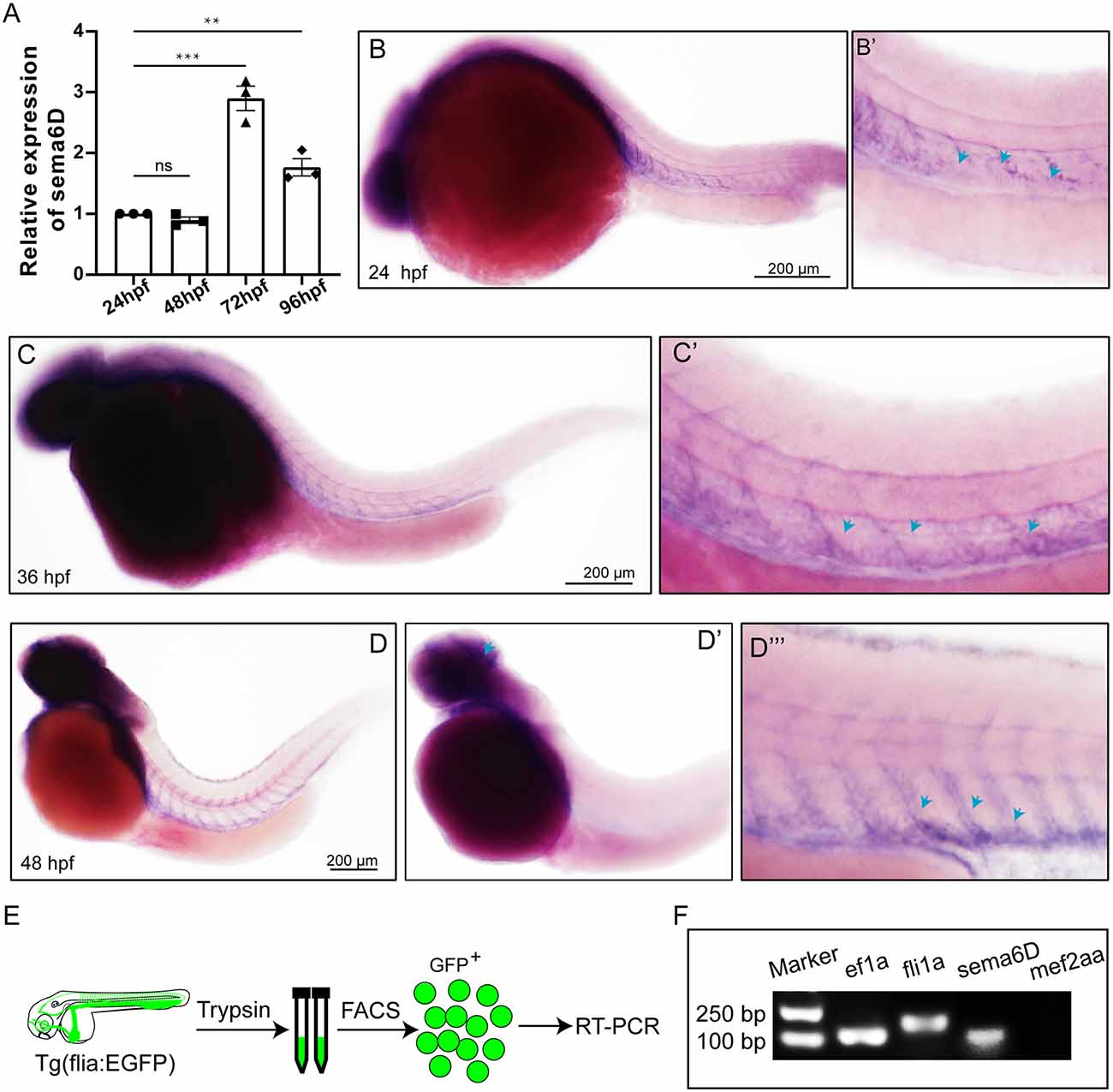
Figure 2. Expression of sema6D gene in embryonic zebrafish at different stages. (A) QRT-PCR analysis of sema6D expression in embryonic zebrafish at different stages (n = 3). One-way ANOVA, values with ** and *** above the bars are significantly different (P < 0.01 and P < 0.001, respectively); value with “ns” above the bars indicated no significantly different. (B–D) Whole mount in situ hybridization analysis of sema6D in zebrafish embryos at different stages, blue arrowheads indicate blood vessels. (E) The procedure of the endothelial cells sorting and RT-PCR. (F) The agarose gel electrophoresis results of RT-PCR on fli1a-EGFP sorted cells.
Deficiency of sema6D Caused Developmental Defect of PMNs
Considering the significant expression of sema6D in the zebrafish brain, it is rational to speculate it might modulate the development of neural system. To validate the hypothesis, specific morpholino antisense oligonucleotide (sema6D-MO) was used to knockdown the expression of sema4D in Tg(mnx1:EGFP) transgenic zebrafish. The results of cDNA amplification provided evidence that the injection of sema6D-MO efficiently altered the transcription pattern of sema6D (Supplementary Figure S1). Themorphology of PMNs was examined by confocal microscopy at 48 and 72 hpf. Although the morphant zebrafish embryos grossly appeared normal, the deficiency of sema6D caused dramatic developmental defects of PMNs (Figure 3A). Compared with the controls, the development of caudal primary motor neurons (Caps) was significantly inhibited at 48 hpf, where the average length of CaPs in the sema6D morphants was particularly shorter than that of the control groups (Figure 3B). At 72 hpf, although the length of CaP in the morphants were similar to the controls, the axonal trajectories of PMNs were significantly misled and many of the truncated axons in the sema6D deficient zebrafish could not recover completely (Figure 3C). Moreover, the number of CaP branches in the morphants decreased obviously at 72 hpf (Figure 3D). Taken together, these results suggest that sema6D is required for the development and navigation of neural networks.
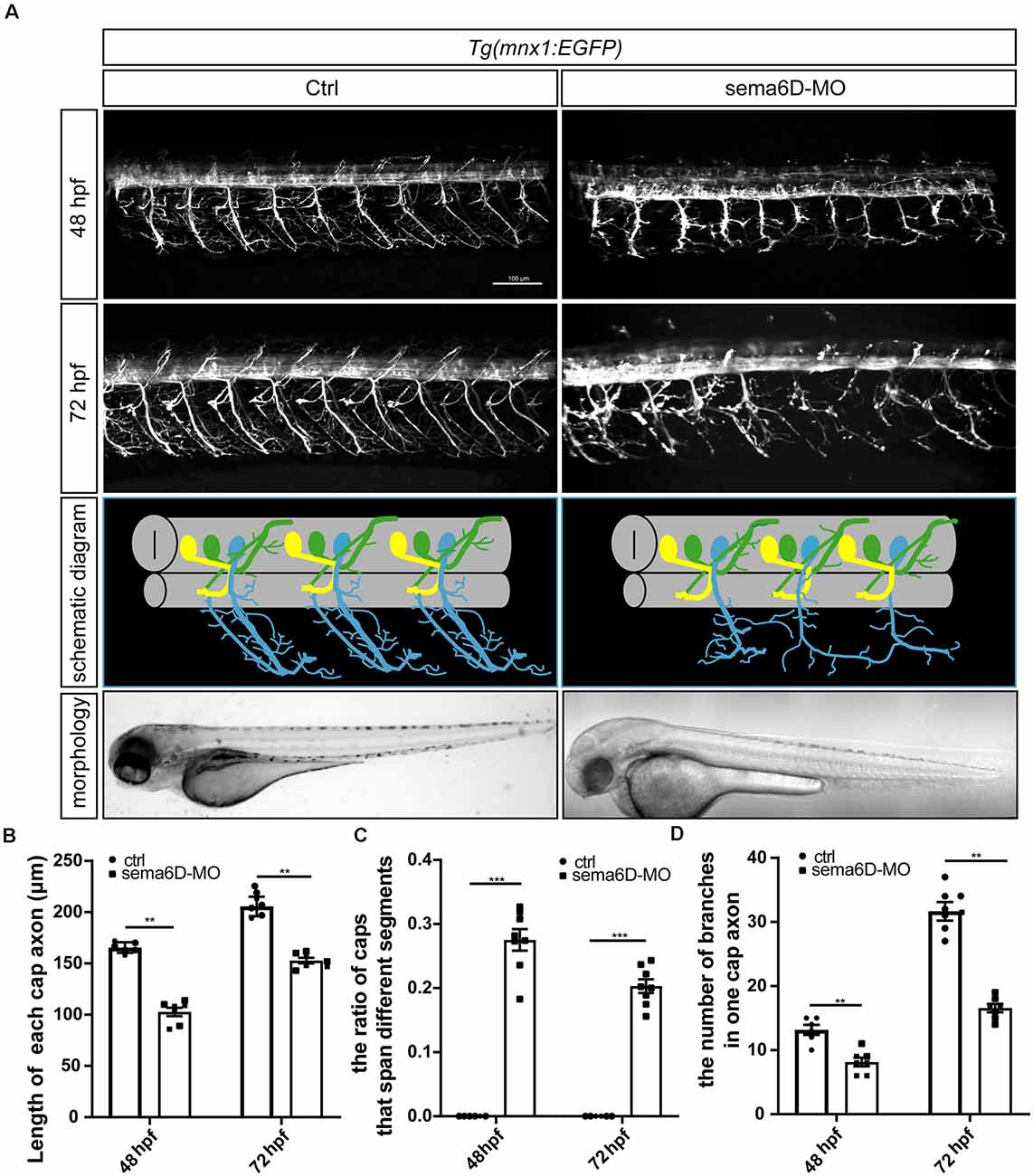
Figure 3. Primary motor neuron morphogenesis defects in the sema6D knockout zebrafish. (A) Confocal imaging analysis of PMNs in control and sema6D knockout groups at 48 and 72 hpf. (B) The statistical analysis of the length of each cap axon in the control and sema6D morphants at 48 and 72 hpf (n = 7). The length of five cap axon was measured in each zebrafish and the average was used. Mann-Whitney test, P = 0.0012. Values with ** above the bars are significantly different (P < 0.01). (C) The statistical analysis of the ratio of Caps across different segments in the control and sema6D morphants at 48 and 72 hpf (n = 8). Mann-Whitney test, P = 0.0007. Values with *** above the bars are significantly different (P < 0.001). (D) The statistical analysis of the number of branches in one cap axon in the control and sema6D morphants at 48 and 72 hpf. The number of branches in each cap axon was measured in five axon of each zebrafish and the average was used. Mann-Whitney test, 48 hpf: P = 0.0017; 72 hpf: P = 0.0012. Values with ** above the bars are significantly different (P < 0.01).
Deficiency of sema6D Caused Developmental Defects of Vascular Pattern
Since sema6D was found to express in zebrafish vessels, we speculated that it might participate in the development of blood vessel. To further explore the roles of sema6D in blood vessel formation, the morphology of ISVs in sema6D knockdown zebrafish was observed by confocal microscopy at different stages. The results showed that the deficiency of sema6D resulted in significant path finding defects of zebrafish ISVs, which was consistent with observation in the development of motor neuron. In control groups, ISVs grew from the dorsal aorta and reached the dorsal roof in an orderly manner to form dorsal anastomotic vessels (DLAV). In contrast, the ISVsin sema6D deficiency zebrafish grew upwards halfway, then turned to connect with adjacent ISVs disorderly, and could not form complete DLAV (Figures 4A,B). In addition, a small number of ISVs which grew only halfway or even less usually failed to cross the horizontal myoseptum in the sema6D knockdown zebrafish (Figure 4C). These results indicated that sema6D seems not necessary for the initial stages of ISVs sprouting, but rather regulates the vascular patterning.
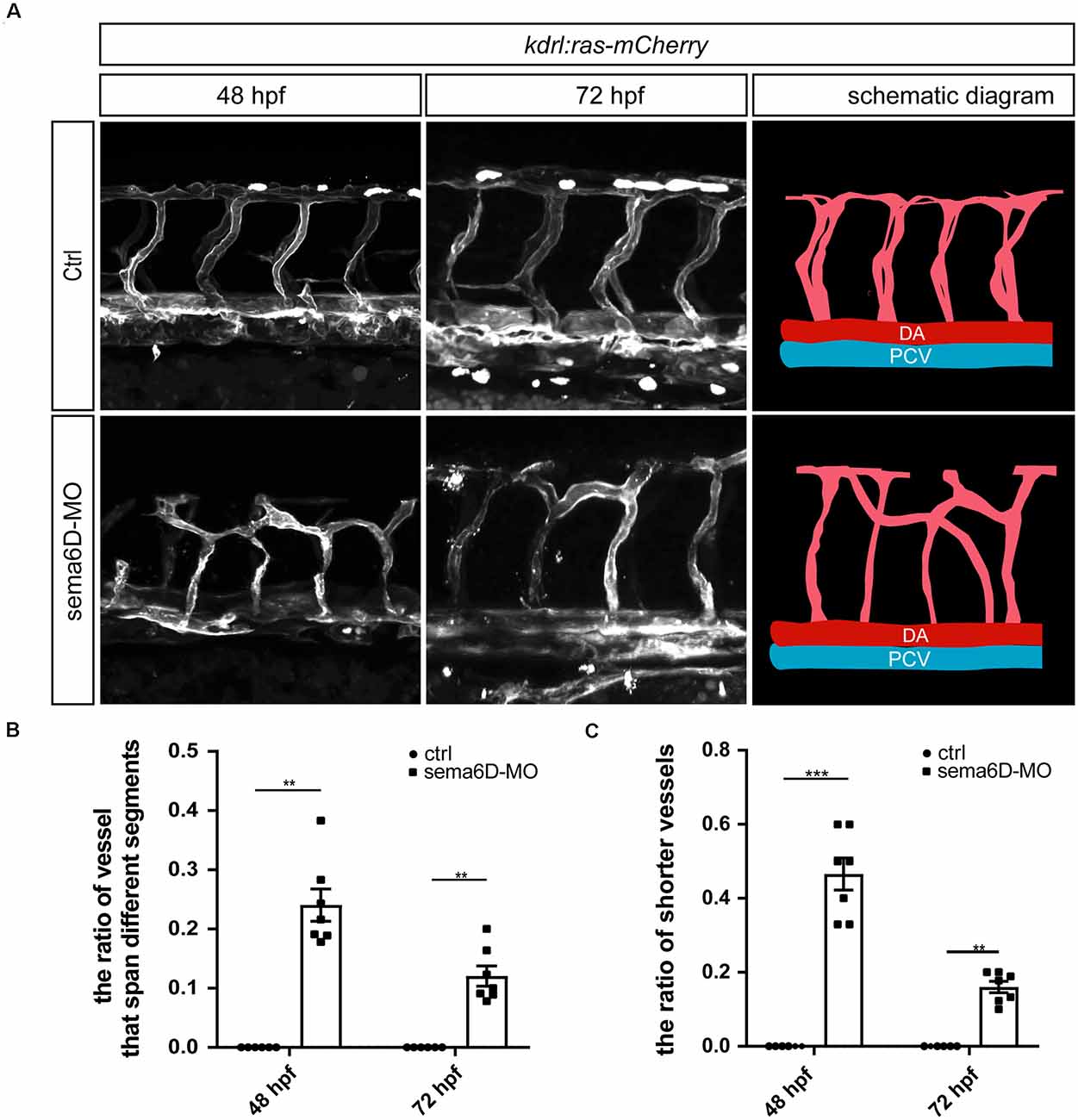
Figure 4. Deficiency of sema6D caused aberrant vascular networks. (A) Confocal imaging analysis of ISVs in the control and sema6D-MO embryos at 48 and 72 hpf. (B) The statistical analysis of the ratio of vessels that span different segments in the control and sema6D morphants at 48 and 72 hpf (n = 7). About 9–12 ISVs were used for statistics per zebrafish. Mann-Whitney test, P = 0.0012.Values with ** above the bars are significantly different (P < 0.01).(C) The statistical analysis of the ratio of shorter vessels in the control and sema6D morphants at 48 and 72 hpf (n = 7). About 9–12 ISVs were used for statistics per zebrafish. Mann-Whitney test, 48 hpf: P = 0.0006; 72 hpf: P = 0.0012. Values with ** and *** above the bars are significantly different (P < 0.01 and P < 0.001, respectively).
Knockout of sema6D Caused Aberrant Patterns of Both Nerves and Vascular System
In order to confirm that sema6D is required for the development of PMNs and ISVs, the CRISPR/Cas9 system was utilized to knockout sema6D in Tg(mnx1:EGFP::kdrl:ras-mCherry) transgenic zebrafish line. In order to ensure complete disruption of functional proteins, the target sites near and downstream of the translation start codon (ATG) of sema6D coding sequence were selected for gRNAs design (Figure 5A). The editing efficiency and the knockout patterns of the selected gRNA-Cas9 system were identified by sequencing (Figures 5B,C). It was found that the phenotypes of PMNs and ISVs in the F0 generation of sema6D knockouts were consistent with the deficient morphants by confocal imaging analysis (Figure 5D). The PMNs were shorter and the axonal trajectories were apparently misled in the F0 knockouts (Figure 5E). Besides, the disorganized vasculature was also observed (Figure 5F). Furthermore, injection of sema6D gRNA without cas9 caused no obvious developmental defects, confirming the phenotype was a specific consequence of sema6D knockdown.
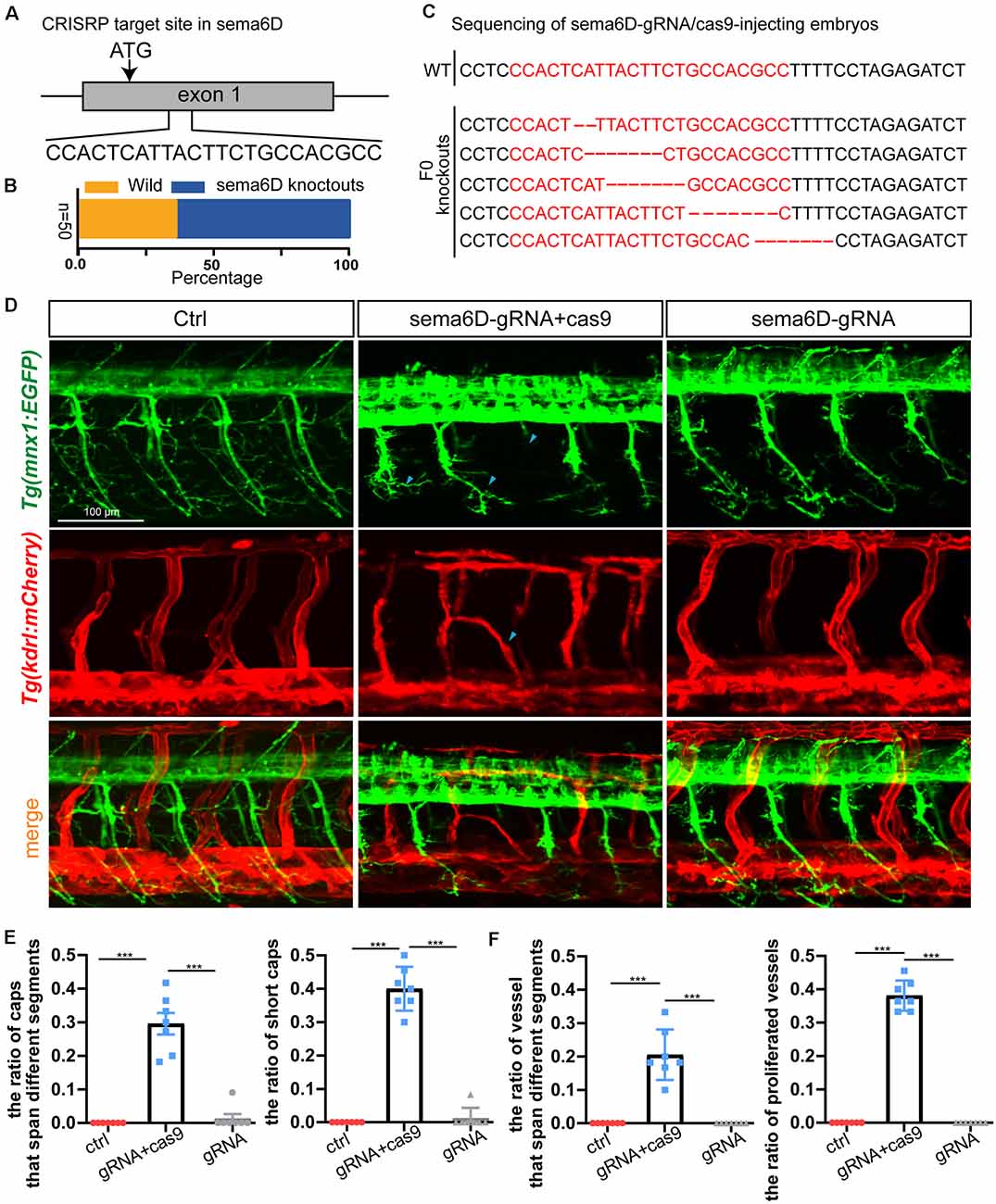
Figure 5. Knockout of sema6D caused both aberrant phenotypes in PMNs and ISVs. (A) Schematic diagram showing the targeting site of the gRNA on the sema6D gene. Starting codon (ATG) site is indicated by arrow. (B) The statistics of the ratio of wildtype to mutant sequences (n = 50). (C) Knockout patterns of sema6D-gRNA/cas9-injecting embryos by sequencing. (D) Confocal imaging analysis of primary motor neurons and intersegmental vessels in control and the F0 generation of the injected Tg(mnx1:EGFP::kdrl:ras-mCherry) zebrafish at 48 hpf, blue arrowheads indicate aberrant PMN and ISV. (E) The statistical analysis of the ratio of aberrant axonal projection of Caps (P = 0.0006) and short Caps (P = 0.0006) in the wild, F0 knockouts, and cas9 negative control at 48 hpf (n = 7). About 9–11caps were used for statistics per zebrafish. Mann-Whitney test. Values with *** above the bars are significantly different (P < 0.001). (F) The statistical analysis of the ratio of vessels that span different segments (P = 0.0006) and the ratio of proliferated vessels (P = 0.006) in the wild, F0 knockouts, and cas9 negative control at 48 hpf (n = 7). About 9–12 ISVs were used for statistics per zebrafish. Mann-Whitney test. Values with *** above the bars are significantly different(P < 0.001).
Overexpressing sema6D Partially Restored the Defects of ISVs and PMNs in sema6D Deficient Embryos
In order to confirm the defects of vascular and neuronal development were specifically caused by sema6D deficiency, the in vitro synthesized sema6D mRNA and sema6D-Mo were co-injected into one cell stage zebrafish embryos. Confocal imaging analysis revealed that the overexpression of sema6D mRNA could greatly rescue the defective phenotypes of PMNs and ISVs (Figures 6A–C) in sema6D deficient embryos. In addition, single sema6D mRNA injection also caused phenotypes of PMNs and ISVs, which are similar to those in deficient morphant (Figures 6A–C). This result validated that sema6D could regulate growing guidance of vessels and neurons in zebrafish. To further investigate the consequences of tissue specific rescue, sema6D was over-expressed in morphants with the fli1a promoter. Comparing with the morphant, embryos co-injected with sema6D-MO and fli1a:sema6D plasmid exhibited rescue phenotypes (Figures 6D–F). Taken together, these results suggested that endothelial-derived sema6D were involved in both neural and vascular development.
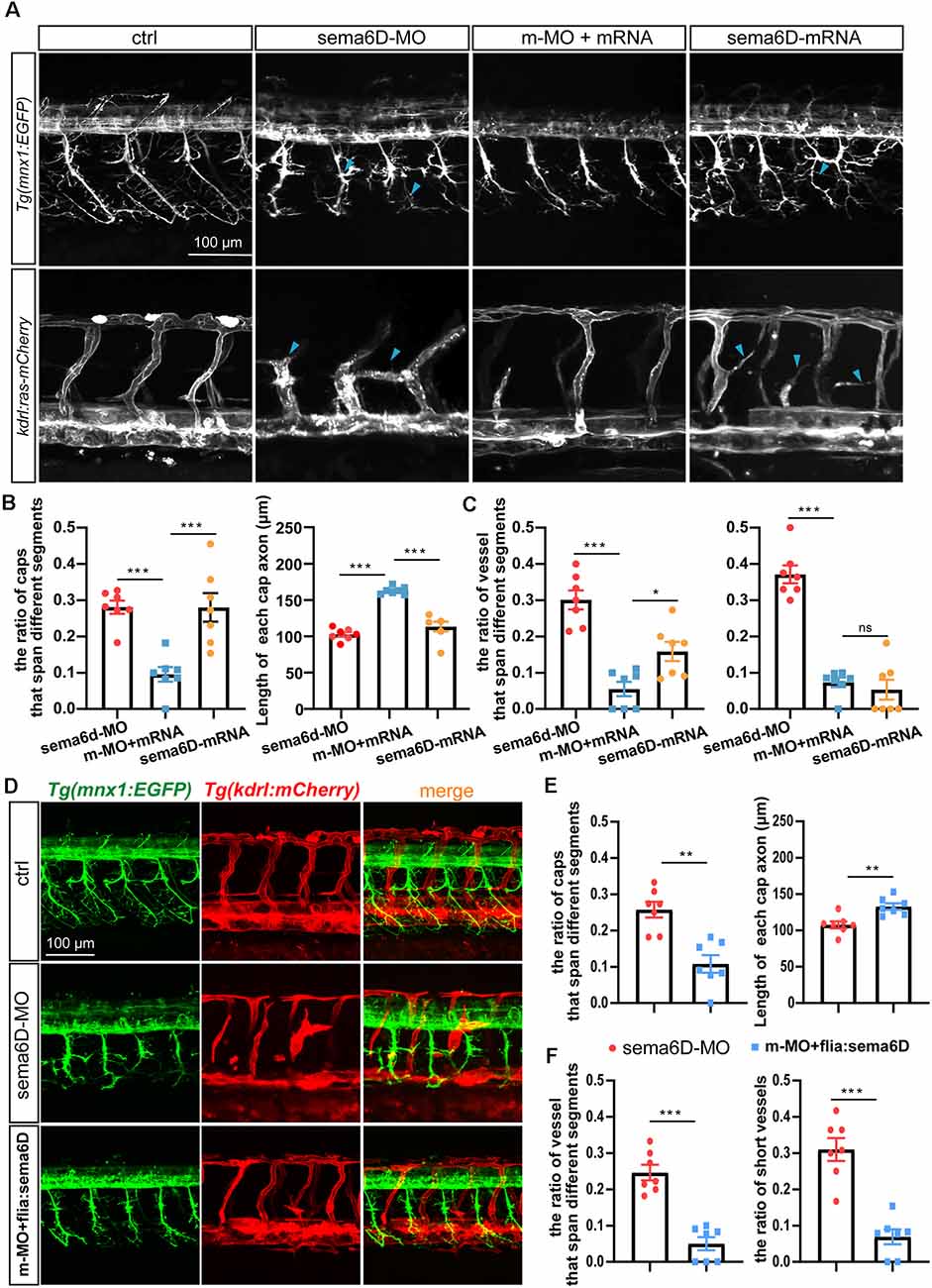
Figure 6. Overexpressing sema6D partially restored the defects of PMNs and ISVs in sema6D-deficient embryos. (A) Confocal imaging analysis of PMNs and ISVs in control, sema6D-MO, and sema6D-mRNA injected embryos at 48 hpf. Blue arrowheads indicate aberrant PMNs and ISVs. (B) The statistical analysis of the ratio of aberrant axonal projection of Caps (P = 0.0006) and short Caps (P = 0.0006) in sema6D-MO, m-MO+sema6D-mRNA, and sema6D-mRNA injected embryos at 48 hpf (n = 7). About 9–11 caps were used for statistics per zebrafish. Mann-Whitney test. Values with *** above the bars are significantly different (P < 0.001). (C) The statistical analysis of the ratio of vessel that span different segments (***P = 0.0006; *P = 0.0291) and the ratio of ectopic vessels (P = 0.0006) in the sema6D-MO, m-MO+sema6D-mRNA, and sema6D-mRNA injected embryos at 48 hpf (n = 7). About 9–12 ISVs were used for statistics per zebrafish. Mann-Whitney test. Values with *** above the bars are significantly different (P < 0.001). Value with “ns” above the bars indicated no significantly different. (D) Confocal imaging analysis of PMNs and ISVs in control, sema6D morphants, and sema6D morphants with fli1a:sema6D plasmid at 48 hpf. (E) The statistical analysis of the ratio of aberrant axonal projection of Caps (P = 0.0012) and the length of Caps (P = 0.0047) in the sema6D morphants and sema6D morphants with fli1a:sema6D plasmid at 48 hpf (n = 7). About 9–11 caps were used for statistics per zebrafish. Mann-Whitney test. Values with ** above the bars are significantly different (P < 0.01). (F) The statistical analysis of the ratio of vessels that span different segments (P = 0.0006) and the ratio of ectopic vessels (P = 0.0006) in the sema6D morphants and sema6D morphants with fli1a:sema6D plasmid at 48 hpf (n = 7). About 9–12 ISVs were used for statistics per zebrafish. Mann-Whitney test. Values with *** above the bars are significantly different (P < 0.001).
Discussion
In this study, we found sema6D played a dual role in regulating vascular and neuronal patterning for the first time by using unique advantages of the zebrafish model. First, detailed expression analysis confirmed that sema6D is enriched in the neural system as well as blood vessels. Then, the knockdown and knockout of sema6D disturbed the pattern of both ISVs and PMNs, suggesting a requirement for sema6D in guiding endothelial cells and neurons during zebrafish embryonic development. Furthermore, overexpression of sema6D in whole embryos and endothelial cells both significantly relieved the aberrant phenotypes of vessels and nerves in sema6D morphants, validating that endothelial-derived sema6D is of great importance to the development and patterning of embryonic nervous and vascular system in zebrafish.
Semaphoring signaling was initially recognized in guiding axon growth (Chisholm and Tessier-Lavigne, 1999; Goodman et al., 1999). However, recent studies have focused its roles outside the neural system. Sema6D belongs to the semaphore in family, which exerts its function in either cell–cell interaction or a long distances manner by releasing its extracellular domains (SEMA+PSI domains) from the cell surface as a secreted cytokine (Toyofuku et al., 2004a, b; Peng et al., 2016). Thus, sema6D may perform diverse important functions during development processes. Furthermore, sema6D and its receptor Plexin-A1 were found to promote endocardial cells migration during heart development in combination with VEGFR2, suggesting it may function as a proangiogenic factor (Toyofuku et al., 2004a; Sun et al., 2019). However, there is so far no data on the regulation of vascular development by sema6D. Here, our WISH results showed that sema6D was not only expressed in the nervous system, but also in the ISVs, suggesting sema6D may participate in the vascular development of zebrafish. Our knock-down study provides first in vivo evidence supporting the expectation. The deficiency of sema6D resulted in abnormal ISVs patterning in zebrafish embryo. This result is consistent with previous studies, which have suggested that the organization of vascular system share various common guiding factors involved in nerves network (Sakurai et al., 2012; Zhang et al., 2020). However, there is another sema6D related gene in zebrafish, sema6Dl, whose function is unclear. Interestingly, our evolutionary analysis showed that sema6Dl did not cluster with sema6D in zebrafish but with other species, suggesting it may not be functionally related to sema6D in zebrafish. Furthermore, previous reports have found that sema6Dl was mainly expressed in the brain, lens, vagal ganglion, and retinal ganglion cells, but not in the primary motor neurons or the vascular system (Supplementary Table S1, Ebert et al., 2012; Shi et al., 2020). It is rational to speculate there is no redundant function between sema6D and sema6Dl in PMNs and ISVs development. Therefore, our data and previous studies remind us that sema6D might contribute to the endothelial cell formation and path finding.
Although sema6D has been validated in regulating specific axons projection as a guidance cue, its functional roles in animal motor neurons development remain unclear (Kimura et al., 2007; Kuwajima et al., 2012; Key et al., 2013). Here, our study demonstrated that sema6D is of great importance to the development of embryonic motor neurons in zebrafish. The deficiency of sema6D could lead to obvious motor neuron defects, including the inhibition of Caps growth and reduced branching of CaP axons. Meanwhile, the absence of sema6D caused dramatic aberrant patterning of PMNs, suggesting sema6D could regulate motor neurons path finding as a guidance signal. Interestingly, the previous report and our in situ hybridization results demonstrated that the expression of sema6D was not significant in the spinal cord (Kucenas et al., 2009). An important question is how does sema6D participate in motor neurons especially Cap axon guidance? From our results, the deficient phenotypes of PMNs are not always accompanied by abnormal ISVs, indicating the motor neurons defects are not the consequence of the aberrant vascular patterning. Another hypothesis is that sema6D acts as a guidance cue, which requires the receptors on motor neurons. Accordingly, a recent report demonstrated knockdown of plexin A1 leads to axons defect in somites, which is similar to our phenotypes (Dworschak et al., 2021). Furthermore, previous study in mouse also showed that developing embryonic but not mature adult blood vessels expressed Plexin-A1 andPlexin-A1 injected led a significant number of abnormal angiogenic spouts in zebrafish ISVs (Jacob et al., 2016). Therefore, our data and previous studies remind us that sema6D might contribute to the PMNs and ISVs patterning by binding to plexin A1 on motor neurons as well as endothelial cells. To further explore the relationship between the vascular and PMNs phenotypes, the tissue-specific rescue experiments were performed. The results showed that overexpressing sema6D in endothelial cells partially rescued the deficient phenotypes in the morphants, indicating endothelial-derived sema6D contributes to the development and patterning of embryonic nervous and vascular systems in zebrafish.
In summary, the present study identified the essential and multifunctional roles of sema6D during the embryonic nervous and vascular development. Deficiency of sema6D could cause deficient neuronal and vascular navigation. Our findings here provided new clues to the synergistically functional and molecular mechanism of sema6D underlying the nerves and blood vascular development. It is of great significance to completely dissect the axon guidance signaling network to understand how the nervous and blood vessel system are built up.
Data Availability Statement
The original contributions presented in the study are included in the article/Supplementary Material, further inquiries can be directed to the corresponding author.
Ethics Statement
The animal study was reviewed and approved by Administration Committee of Experimental Animals, Jiangsu Province, China.
Author Contributions
DL supervised and designed this project. JS and DL wrote the manuscript and analyzed the data. JS, JX, and KG performed the experiments. All authors contributed to the article and approved the submitted version.
Funding
This study was supported by grants from the National Natural Science Foundation of China (81870359, 2018YFA0801004) and Natural Science Foundation of Jiangsu Province (BK20180048).
Conflict of Interest
The authors declare that the research was conducted in the absence of any commercial or financial relationships that could be construed as a potential conflict of interest.
Publisher’s Note
All claims expressed in this article are solely those of the authors and do not necessarily represent those of their affiliated organizations, or those of the publisher, the editors and the reviewers. Any product that may be evaluated in this article, or claim that may be made by its manufacturer, is not guaranteed or endorsed by the publisher.
Supplementary Material
The Supplementary Material for this article can be found online at: https://www.frontiersin.org/articles/10.3389/fnmol.2022.854556/full#supplementary-material.
References
Acker, D. W., Wong, I., Kang, M., and Paradis, S. (2018). Semaphorin 4D promotes inhibitory synapse formation and suppresses seizures in vivo. Epilepsia 59, 1257–1268. doi: 10.1111/epi.14429
Bussolino, F., Valdembri, D., Caccavari, F., and Serini, G. (2006). Semaphoring vascular morphogenesis. Endothelium 13, 81–91. doi: 10.1080/10623320600698003
Capparuccia, L., and Tamagnone, L. (2009). Semaphorin signaling in cancer cells and in cells of the tumor microenvironment-two sides of a coin. J. Cell Sci. 122, 1723–1736. doi: 10.1242/jcs.030197
Carmeliet, P. (2003). Angiogenesis in health and disease. Nat. Med. 9, 653–660. doi: 10.1038/nm0603-653
Chang, N., Sun, C., Gao, L., Zhu, D., Xu, X., Zhu, X., et al. (2013). Genome editing with RNA-guided Cas9 nuclease in zebrafish embryos. Cell Res. 23, 465–472. doi: 10.1038/cr.2013.45
Chisholm, A., and Tessier-Lavigne, M. (1999). Conservation and divergence of axon guidance mechanisms. Curr. Opin. Neurobiol. 9, 603–615. doi: 10.1016/S0959-4388(99)00021-5
Dworschak, G. C., Punetha, J., Kalanithy, J. C., Mingardo, E., Erdem, H. B., Akdemir, Z. C., et al. (2021). Biallelic and monoallelic variants in PLXNA1 are implicated in a novel neurodevelopmental disorder with variable cerebral and eye anomalies. Genet. Med. 23, 1715–1725. doi: 10.1038/s41436-021-01196-9
Ebert, A. M., Lamont, R. E., Childs, S. J., and Mcfarlane, S. (2012). Neuronal expression of class 6 semaphorins in zebrafish. Gene Expr. Patterns 12, 117–122. doi: 10.1016/j.gep.2012.01.007
Elder, A. M., Tamburini, B. A., Crump, L. S., Black, S. A., Wessells, V. M., Schedin, P. J., et al. (2018). Semaphorin 7A promotes macrophage-mediated lymphatic remodeling during postpartum mammary gland involution and in breast cancer. Cancer Res. 78, 6473–6485. doi: 10.1158/0008-5472.CAN-18-1642
Ellertsdóttir, E., Lenard, A., Blum, Y., Krudewig, A., Herwig, L., Affolter, M., et al. (2010). Vascular morphogenesis in the zebrafish embryo. Dev. Biol. 341, 56–65. doi: 10.1016/j.ydbio.2009.10.035
Ferreira, G. D., Capp, E., Jauckus, J., Strowitzki, T., and Germeyer, A. (2018). Expression of semaphorin class 3 is higher in the proliferative phase on the human endometrium. Arch. Gynecol. Obstet. 297, 1175–1179. doi: 10.1007/s00404-018-4719-3
Gherardi, E., Love, C. A., Esnouf, R. M., and Jones, E. Y. (2004). The sema domain. Curr. Opin. Struct. Biol. 14, 669–678. doi: 10.1016/j.sbi.2004.10.010
Gong, J., Wang, X., Zhu, C., Dong, X., Zhang, Q., Wang, X., et al. (2017). Insm1a regulates motor neuron development in zebrafish. Front. Mol. Neurosci. 10:274. doi: 10.3389/fnmol.2017.00274
Goodman, C., Kolodkin, A., Luo, Y., Püschel, A., and Raper, J. (1999). Unified nomenclature for the semaphorins/collapsins. Cell 97, 551–552. doi: 10.1016/s0092-8674(00)80766-7
Gu, C., Yoshida, Y., Livet, J., Reimert, D. V., Mann, F., Merte, J., et al. (2005). Semaphorin 3E and plexin-D1 control vascular pattern independently of neuropilins. Science 307, 265–268. doi: 10.1126/science.1105416
Huang, Y., Wang, X., Wang, X., Xu, M., Liu, M., and Liu, D. (2013). Nonmuscle myosin II-B (myh10) expression analysis during zebrafish embryonic development. Gene Expr. Patterns 13, 265–270. doi: 10.1016/j.gep.2013.04.005
Jacob, L., Sawma, P., Garnier, N., Meyer, L. A., Fritz, J., Hussenet, T., et al. (2016). Inhibition of PlexA1-mediated brain tumor growth and tumor-associated angiogenesis using a transmembrane domain targeting peptide. Oncotarget 7, 57851–57865. doi: 10.18632/oncotarget.11072
Jao, L.-E., Wente, S. R., and Chen, W. (2013). Efficient multiplex biallelic zebrafish genome editing using a CRISPR nuclease system. Proc. Natl. Acad. Sci. U S A 110, 13904–13909. doi: 10.1073/pnas.1308335110
Key, B., Matsuoka, R. L., Sun, L. O., Katayama, K.-I., Yoshida, Y., and Kolodkin, A. L. (2013). Sema6B, sema6C and sema6D expression and function during mammalian retinal development. PLoS One 8:e63207. doi: 10.1371/journal.pone.0063207
Kimura, M., Taniguchi, M., Mikami, Y., Masuda, T., Yoshida, T., Mishina, M., et al. (2007). Identification and characterization of zebrafish semaphorin 6D. Biochem. Biophys. Res. Commun. 363, 762–768. doi: 10.1016/j.bbrc.2007.09.038
Kolodkin, A. L., and Tessier-Lavigne, M. (2011). Mechanisms and molecules of neuronal wiring: a primer. Cold Spring Harb. Perspect. Biol. 3:a001727. doi: 10.1101/cshperspect.a001727
Kucenas, S., Wang, W.-D., Knapik, E. W., and Appel, B. (2009). A selective glial barrier at motor axon exit points prevents oligodendrocyte migration from the spinal cord. J. Neurosci. 29, 15187–15194. doi: 10.1523/JNEUROSCI.4193-09.2009
Kuwajima, T., Yoshida, Y., Takegahara, N., Petros, T. J., Kumanogoh, A., Jessell, T. M., et al. (2012). Optic chiasm presentation of semaphorin6D in the context of plexin-A1 and Nr-CAM promotes retinal axon midline crossing. Neuron 74, 676–690. doi: 10.1016/j.neuron.2012.03.025
Leslie, J. R., Imai, F., Fukuhara, K., Takegahara, N., Rizvi, T. A., Friedel, R. H., et al. (2011). Ectopic myelinating oligodendrocytes in the dorsal spinal cord as a consequence of altered semaphorin 6D signaling inhibit synapse formation. Development 138, 4085–4095. doi: 10.1242/dev.066076
Luo, Y., Raible, D., and Raper, J. A. (1993). Collapsin: a protein in brain that induces the collapse and paralysis of neuronal growth cones. Cell 75, 217–227. doi: 10.1016/0092-8674(93)80064-l
Lv, F., Zhu, C., Yan, X., Wang, X., and Liu, D. (2017). Generation of a mef2aa:EGFP transgenic zebrafish line that expresses EGFP in muscle cells. Fish Physiol. Biochem. 43, 287–294. doi: 10.1007/s10695-016-0286-3
Makanya, A. N., Hlushchuk, R., and Djonov, V. G. (2009). Intussusceptive angiogenesis and its role in vascular morphogenesis, patterning and remodeling. Angiogenesis 12, 113–123. doi: 10.1007/s10456-009-9129-5
Nakayama, T., Fish, M. B., Fisher, M., Oomen-Hajagos, J., Thomsen, G. H., and Grainger, R. M. (2013). Simple and efficient CRISPR/Cas9-mediated targeted mutagenesis in Xenopus tropicalis. Genesis 51, 835–843. doi: 10.1002/dvg.22720
Neufeld, G., and Kessler, O. (2008). The semaphorins: versatile regulators of tumour progression and tumour angiogenesis. Nat. Rev. Cancer 8, 632–645. doi: 10.1038/nrc2404
Nicoli, S., Knyphausen, C.-P., Zhu, L. J., Lakshmanan, A., and Lawson, N. D. (2012). miR-221 is required for endothelial tip cell behaviors during vascular development. Dev. Cell 22, 418–429. doi: 10.1016/j.devcel.2012.01.008
Nikolova, G., and Lammert, E. (2003). Interdependent development of blood vessels and organs. Cell Tissue Res. 314, 33–42. doi: 10.1007/s00441-003-0739-8
Patan, S. (2000). Vasculogenesis and angiogenesis as mechanisms of vascular network formation, growth and remodeling. J. Neurooncol. 50, 1–15. doi: 10.1023/a:1006493130855
Peng, Y., Song, L., Li, D., Kesterson, R., Wang, J., Wang, L., et al. (2016). Sema6D acts downstream of bone morphogenetic protein signalling to promote atrioventricular cushion development in mice. Cardiovasc. Res. 112, 532–542. doi: 10.1093/cvr/cvw200
Sakurai, A., Doci, C., and Gutkind, J. S. (2012). Semaphorin signaling in angiogenesis, lymphangiogenesis and cancer. Cell Res. 22, 23–32. doi: 10.1038/cr.2011.198
Shi, Y., Qian, P., Sheng, J., Zhang, X., Wang, X., Zhao, J., et al. (2020). Single-cell RNA-seq reveals endoimmune cells in zebrafish. bioRxiv [Preprint]. doi: 10.1101/2019.12.31.892240
Suchting, S., Bicknell, R., and Eichmann, A. (2006). Neuronal clues to vascular guidance. Exp. Cell Res. 312, 668–675. doi: 10.1016/j.yexcr.2005.11.009
Sun, Q., Peng, Y., Zhao, Q., Yan, S., Liu, S., Yang, Q., et al. (2019). SEMA6D regulates perinatal cardiomyocyte proliferation and maturation in mice. Dev. Biol. 452, 1–7. doi: 10.1016/j.ydbio.2019.04.013
Toyofuku, T., Zhang, H., Kumanogoh, A., Takegahara, N., Suto, F., Kamei, J., et al. (2004a). Dual roles of Sema6D in cardiac morphogenesis through region-specific association of its receptor, plexin-A1, with off-track and vascular endothelial growth factor receptor type 2. Genes Dev. 18, 435–447. doi: 10.1101/gad.1167304
Toyofuku, T., Zhang, H., Kumanogoh, A., Takegahara, N., Yabuki, M., Harada, K., et al. (2004b). Guidance of myocardial patterning in cardiac development by Sema6D reverse signalling. Nat. Cell Biol. 6, 1204–1211. doi: 10.1038/ncb1193
Uccelli, A., Wolff, T., Valente, P., Di Maggio, N., Pellegrino, M., Gürke, L., et al. (2019). Vascular endothelial growth factor biology for regenerative angiogenesis. Swiss Med. Wkly. 149:w20011. doi: 10.4414/smw.2019.20011
Wang, X., Ling, C. C., Li, L., Qin, Y., Qi, J., Liu, X., et al. (2016). MicroRNA-10a/10b represses a novel target gene mib1 to regulate angiogenesis. Cardiovasc. Res. 110, 140–150. doi: 10.1093/cvr/cvw023
Wu, R. S., Lam, I. I., Clay, H., Duong, D. N., Deo, R. C., and Coughlin, S. R. (2018). A rapid method for directed gene knockout for screening in G0 zebrafish. Dev. Cell 46, 112–125.e4. doi: 10.1016/j.devcel.2018.06.003
Xu, M., Liu, D., Dong, Z., Wang, X., Wang, X., Liu, Y., et al. (2014). Kinesin-12 influences axonal growth during zebrafish neural development. Cytoskeleton (Hoboken) 71, 555–563. doi: 10.1002/cm.21193
Yazdani, U., and Terman, J. R. (2006). The semaphorins. Genome Biol. 7:211. doi: 10.1186/gb-2006-7-3-211
Yoshida, Y., Han, B., Mendelsohn, M., and Jessell, T. M. (2006). PlexinA1 signaling directs the segregation of proprioceptive sensory axons in the developing spinal cord. Neuron 52, 775–788. doi: 10.1016/j.neuron.2006.10.032
Keywords: endothelial cells, motor neuronal axon, guidance cues, path finding, zebrafish
Citation: Sheng J, Xu J, Geng K and Liu D (2022) Sema6D Regulates Zebrafish Vascular Patterning and Motor Neuronal Axon Growth in Spinal Cord. Front. Mol. Neurosci. 15:854556. doi: 10.3389/fnmol.2022.854556
Received: 14 January 2022; Accepted: 02 March 2022;
Published: 07 April 2022.
Edited by:
Feng Rao, Peking University People’s Hospital, ChinaReviewed by:
Zhen Zhong, Zhejiang University, ChinaMatthew B. Veldman, Medical College of Wisconsin, United States
Copyright © 2022 Sheng, Xu, Geng and Liu. This is an open-access article distributed under the terms of the Creative Commons Attribution License (CC BY). The use, distribution or reproduction in other forums is permitted, provided the original author(s) and the copyright owner(s) are credited and that the original publication in this journal is cited, in accordance with accepted academic practice. No use, distribution or reproduction is permitted which does not comply with these terms.
*Correspondence: Dong Liu, bGl1ZG9uZ3RvbUBnbWFpbC5jb20=; dG9tQG50dS5lZHUuY24=
† These authors have contributed equally to this work