- 1Hospital of Chengdu University of Traditional Chinese Medicine, Chengdu, China
- 2The Affiliated Traditional Chinese Medicine Hospital of Southwest Medical University, Luzhou, China
- 3Guang'anmen Hospital, China Academy of Chinese Medical Sciences, Beijing, China
- 4Preventive Treatment Center, The Affiliated Traditional Chinese Medicine Hospital of Southwest Medical University, Luzhou, China
Millions of patients are suffering from ischemic stroke, it is urgent to figure out the pathogenesis of cerebral ischemia–reperfusion (I/R) injury in order to find an effective cure. After I/R injury, pro-inflammatory cytokines especially interleukin-1β (IL-1β) upregulates in ischemic brain cells, such as microglia and neuron. To ameliorate the inflammation after cerebral I/R injury, nucleotide-binding oligomerization domain (NOD), leucine-rich repeat (LRR), and pyrin domain-containing protein 3 (NLRP3) inflammasome is well-investigated. NLRP3 inflammasomes are complicated protein complexes that are activated by endogenous and exogenous danger signals to participate in the inflammatory response. The assembly and activation of the NLRP3 inflammasome lead to the caspase-1-dependent release of pro-inflammatory cytokines, such as interleukin (IL)-1β and IL-18. Furthermore, pyroptosis is a pro-inflammatory cell death that occurs in a dependent manner on NLRP3 inflammasomes after cerebral I/R injury. In this review, we summarized the assembly and activation of NLRP3 inflammasome; moreover, we also concluded the pivotal role of NLRP3 inflammasome and inhibitors, targeting the NLRP3 inflammasome in cerebral I/R injury.
Introduction
Ischemic stroke is the leading cause of disability in adults and has been a major health concern worldwide (Wang et al., 2017; Huang et al., 2022). Lack of understanding of the pathogenesis leads to limitations in the treatment of ischemic stroke. Currently, the immune response has shown both beneficial (Fernández-López et al., 2016) and detrimental effects (Jin et al., 2010) on the pathogenesis and prognosis of cerebral ischemia–reperfusion (I/R) injury. Oxidative stress, neuron death, and inflammation are involved in the pathogenesis of cerebral I/R injury (Pan et al., 2022a,b). Regarding neuroinflammation, the most definite mediator of inflammation after cerebral I/R injury is the cytokine interleukin-1 (IL-1) (Barrington et al., 2017). Nucleotide-binding oligomerization domain (NOD), leucine-rich repeat (LRR), and pyrin domain-containing protein 3 (NLRP3) inflammasome act as the upstream of IL-1, which regulates the mature and secretion of proinflammatory cytokines. After transient middle cerebral artery occlusion (MCAO) in mice, NLRP3 is the major contributor among the inflammasomes (Franke et al., 2021). It is confirmed that NLRP3 inflammasome has been a therapeutic target for cerebral I/R injury. As a new detective signaling platform, the NLRP3 inflammasome could be activated by a range of microbial infections, such as coronavirus (Zheng et al., 2020), Staphylococcus aureus (Mariathasan et al., 2006), and candida albicans hyphae (Joly et al., 2009). As shown in Figure 1 and Table 1, the activation of NLRP3 inflammasome is involved with the onset and progression of various diseases, and the research for medicines that inhibit the activation of NLRP3 inflammasome could be therapeutically beneficial. Therefore, numerous studies are pursuing to figure out the physiological structure, assembly, and activation of NLRP3 inflammasome and detect the potential pathogenic mechanisms.
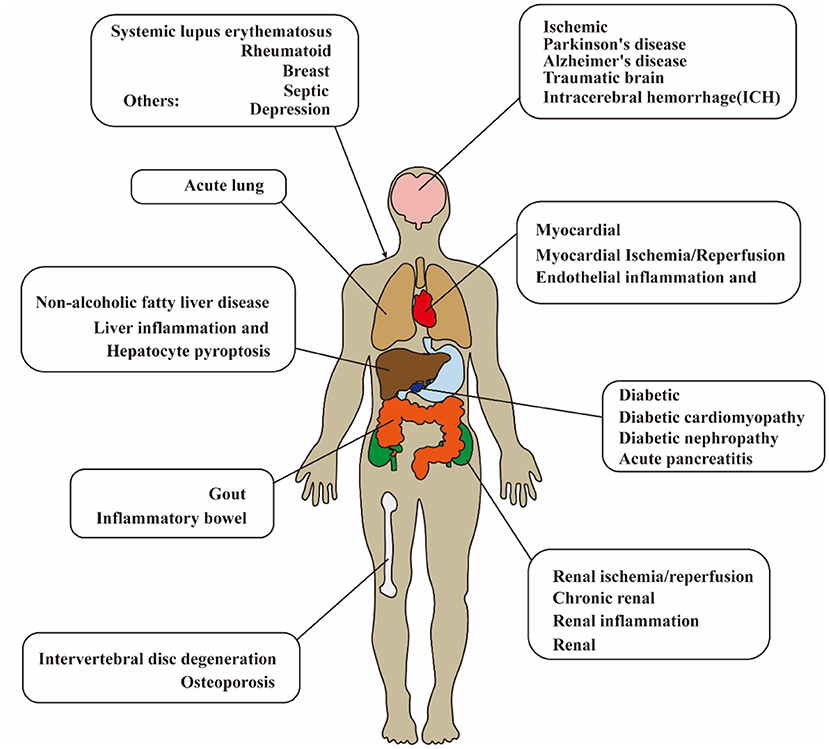
Figure 1. Diagram of NLRP3 inflammasome-related disease in human. The activation of NLRP3 inflammasome is extensively involved with the onset and progression of various diseases. NLRP3, pyrin domain-containing protein 3.
The Assembly of NLRP3 Inflammasome
Inflammasome was first described by Fabio Martinon in 2002, which was the identification of a caspase-activating complex that consisted of a sensor (PRRs), an adaptor (ASC), and an effector (caspase1) (Martinon et al., 2002; Swanson et al., 2019). The PRRs are expressed in innate immune system cells (such as macrophages and neutrophils cells) (Abderrazak et al., 2015), and PRRs sense pathogen-associated molecular patterns (PAMPs) or damage/danger-associated molecular patterns (DAMPs) (Matzinger, 2002; Karasawa and Takahashi, 2017) for the innate immune system to defend against these “danger signals.” PRRs can be divided into many subgroups, including members of Toll-like receptors (TLRs), C-type lectin receptors (CLRs), NOD-like receptors (NLRs), and absent-in-melanoma 2 receptors (ALRs) (Lamkanfi and Dixit, 2014; Kelley et al., 2019). NLRP3 inflammasome is the most well-characterized inflammasome and closely related to the cleavage of caspase-1 (Martinon et al., 2002). NLRP3 is composed of central NOD, a C-terminal LRR domain, and an N-terminal pyrin domain (PYD) (Menu and Vince, 2011; Alishahi et al., 2019). ASC (apoptosis speck protein) has a C-terminal pyrin domain (PYD) and a C-terminal caspase recruitment domain (CARD) (Alishahi et al., 2019). The CARD in ASC is homotypic with the CARD in pro-caspase-1. After the LRR domain is sensitized by stimuli, NLRP3 self-oligomerizes through the interaction of homotype NODs (Alishahi et al., 2019), then, the N-terminal PYD domain in oligomerized NLRP3 facilitates homotypic PYD–PYD interactions between NLRP and adapter protein ASC (Liepinsh et al., 2003), and assembled ASC recruits pro-caspase-1 via C-terminal CARD–CARD interactions (Srinivasula et al., 2002). The assembly and activation of NLRP3 inflammasome promote the cleavage of pro-caspase-1 to form active caspase-1, which leads to the maturation and cleaves of proinflammatory cytokines, such as IL-1β and IL-18.
The Activation of NLRP3 Inflammasome Under I/R Injury
Notably, the activation of NLRP3 inflammasome has been detected after I/R injury. It is confirmed that NLRP3 was upregulated significantly in 4 h after hypoxic-ischemic in rats, and the elevated levels of IL-1β were detected in 8 h after hypoxic-ischemic injury (Li et al., 2021b). A study of patients with acute ischemic stroke admitted <24 h showed that the level of serum concentration of NLRP3 was related to the increased risk of malignant brain edema (MBE) (Wang et al., 2021e). Therefore, elucidating the mechanism of NLRP3 activation and intervening early after the onset of ischemic stroke are of great importance for the treatment and prognosis of ischemic stroke. It is widely accepted that NLRP3 inflammasome activation is related to two signals: in signal I (priming), cytokines or PAMPs could lead to pro-IL-1β and NLRP3 upregulation in a nuclear factor-κ-gene binding (NF-κB)-dependent manner in response to the activation of proinflammatory cytokine receptors or transcription-modulating PRRs; in signal II (activation), various upstream DAMP and PAMP signaling events lead to the oligomerization of NLRP3 and the assembly of ASC and pro-caspase-1 to form NLRP3 inflammasome (Shao et al., 2015; Liu, Q. Y et al., 2018; Sho and Xu, 2019; Swanson et al., 2019). Recent studies demonstrate that there are mainly three models which activate the signal II in the activation of NLRP3 inflammasome, namely, ionic flux, mitochondrial destabilization, and lysosomal damage (Gong et al., 2018a; Kelley et al., 2019). All these models are shown in Figure 2. In this study, we described the activation of NLRP3 inflammasome after cerebral I/R injury.
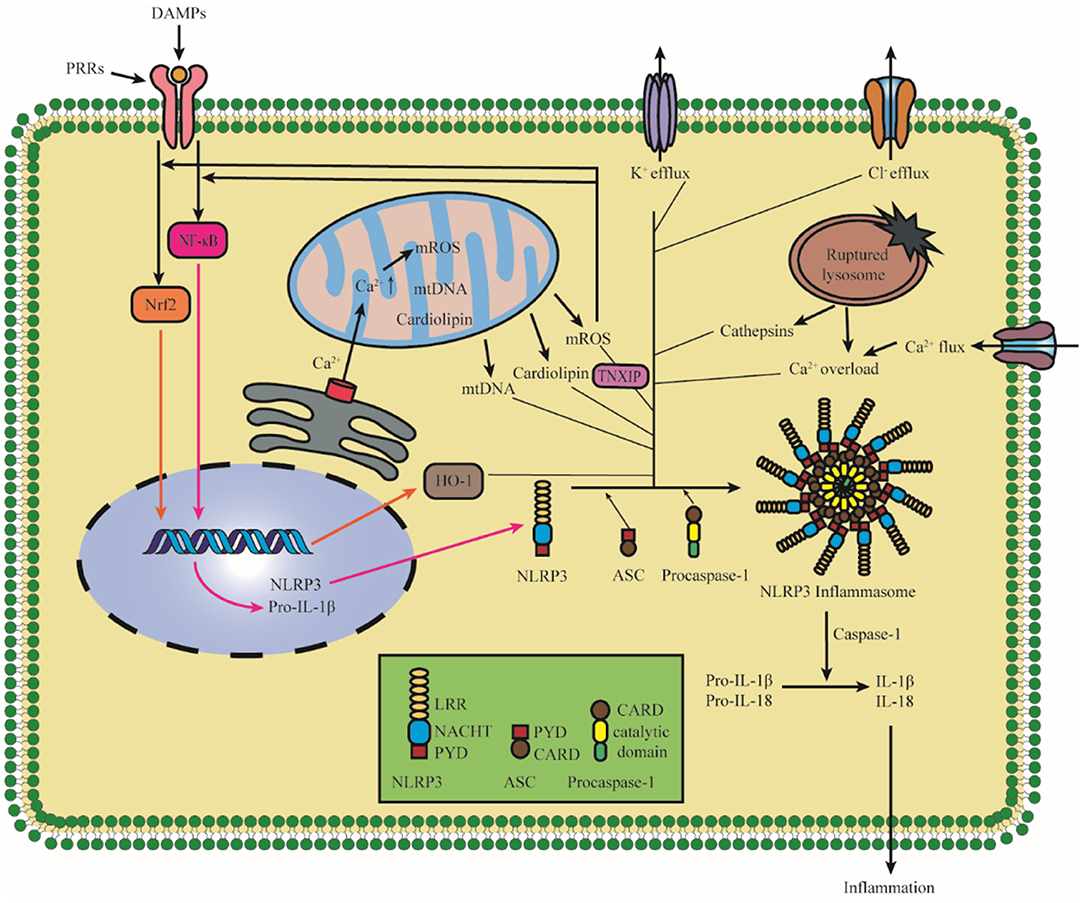
Figure 2. Diagram of NLRP3 inflammasome activation. The priming induces NLRP3 and pro-IL-1β upregulation in an NF-κB-dependent manner through translocating to the nucleus. The upstream DAMP and PAMP signaling events lead to the oligomerization of NLRP3 and the assembly of ASC and pro-caspase-1 to form NLRP3 inflammasome. There are mainly three models, which activate the activation of NLRP3 inflammasome: K+ efflux, ROS, and lysosomal damage, and specifically it also includes Cl− efflux, Ca2+ overload, mtDNA, cardiolipin, and the nuclear translocation of Nrf2. The assembly and activation of the NLRP3 inflammasome lead to the cleavage of Caspase-1, which promotes the maturation and cleaves proinflammatory cytokines IL-1β and IL-18. NLRP3, pyrin domain-containing protein 3; NF-κB, nuclear factor-k-gene binding; HO-1, Hemeoxygenase-1; TNXIP, thioredoxin interacting protein; PRR, pattern recognition receptor; DAMPs, damage/danger-associated molecular patterns; Nrf2, NF-E2-related factor 2; ROS, reactive oxygen species; mtDNA, mitochondrial DNA.
Ionic Flux
Intracellular ionic flux which includes K+ efflux, Ca2+ mobilization, and Cl− efflux acts as an upstream regulation role in the activation of NLRP3 inflammasome (Gong et al., 2018a). K+ efflux is the most investigated target, the main reason for the decrease in intracellular K+ concentration is related to K+ channel opening (Di et al., 2018), membrane remodeling (Gianfrancesco et al., 2019), and membrane permeabilization change (Franchi et al., 2014); at the same time, many stimuli can trigger K+ effluxes such as adenosine triphosphate (ATP), nigericin, and monosodium urate (MSU) crystals (Nomura et al., 2015). The activation of NLRP3 could be blocked by inhibiting K+ efflux; therefore, the low intracellular K+ concentration is a key trigger for NLRP3 inflammasome activation (Pétrilli et al., 2007). It has demonstrated that K+-ATP channel pore-forming subunit Kir6.1 is a bona fide negative regulator of the NLRP3 inflammasome, and the suppression of Kir6.1 increases the accumulation of damaged mitochondria and production of reactive oxygen species (ROS) (Du et al., 2019; Hu et al., 2019). K+ efflux is a well-accepted upstream regulating signal for NLRP3 inflammasome, but some studies have reported that other signals could also affect NLRP3 inflammasome activation in a K+ efflux-independent manner. Imiquimod affects ROS production by regulating the quinone oxidoreductases, namely, NQO2 and mitochondrial Complex I to participate in the activation of the NLRP3 inflammasome, and the activation of the NLRP3 inflammasome by imiquimod is K+ efflux-independent (Groß et al., 2016).
Mitochondrial Destabilization
After beingstimulated, the products derived from mitochondria and other mitochondrial signaling molecules contribute to the NLRP3 inflammasome activation, such as mitochondrial ROS (mROS), mitochondrial DNA (mtDNA), and cardiolipin (Gong et al., 2018b; Zhong et al., 2018; Dagvadorj et al., 2021). All these details are shown in Figure 3. It is well-agreed that inducing ROS is the common characteristic of most NLRP3 activators (Hornung and Latz, 2010), and the main source of cellular ROS is the mitochondria (Dan Dunn et al., 2015). mROS is critical for NLRP3 inflammasome activation (Tschopp and Schroder, 2010; Gurung et al., 2015; Minutoli et al., 2016; Yu and Lee, 2016). The overexpression of mROS would activate the NLRP3 inflammasome through mainly two-signal models, namely, NF-κB pathway and mitochondria/thioredoxin-interacting protein (TXNIP), which activates the NLRP3 inflammasome (Sho and Xu, 2019). Accumulating studies have shown that the inhibitors of mtDNA synthesis and mROS can alleviate diseases by suppressing NLRP3 inflammasome activation (Zhong et al., 2016; Guo et al., 2017; Lee et al., 2019). Cardiolipin could activate the NLRP3 inflammasome in a ROS-independent way during mitochondrial destabilization. In addition to mROS, mtDNA and cardiolipin also serve as the ultimate NLRP3 ligand for the activation of NLRP3 inflammasome (Shimada et al., 2012; Iyer et al., 2013).
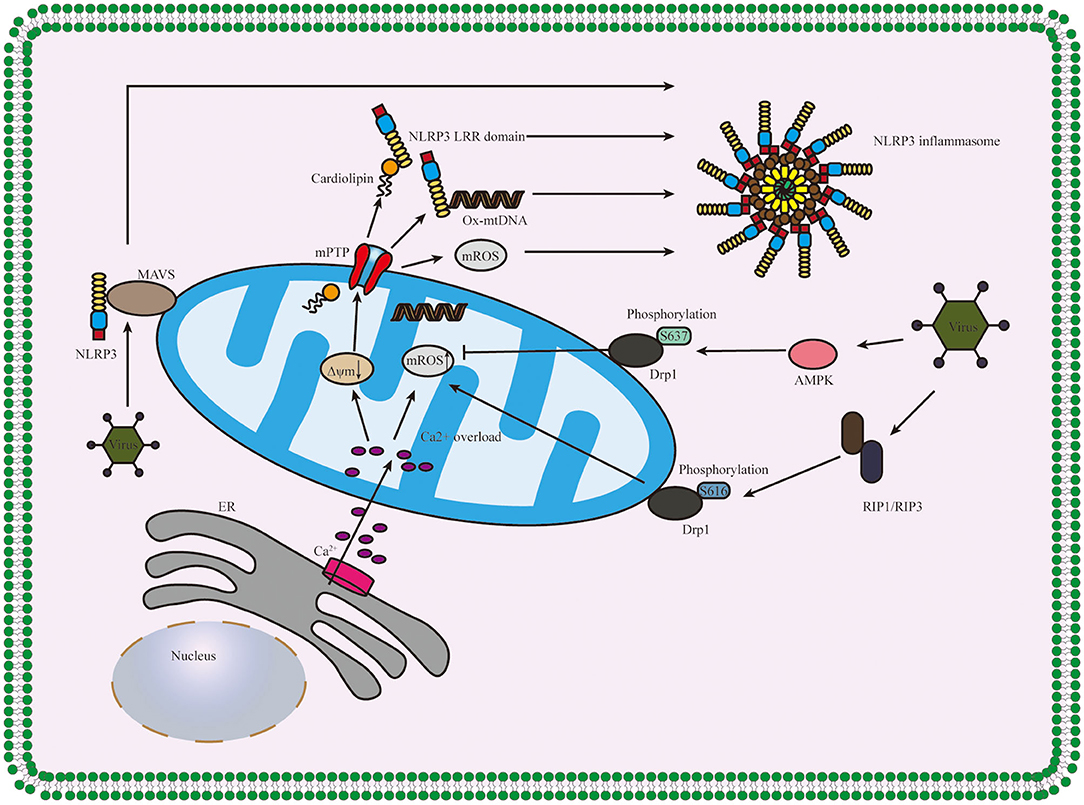
Figure 3. Diagram of the role of the mitochondrion in NLRP3 inflammasome activation. Ca2+ which released from ER influxes into mitochondria, and the overload of Ca2+ contributes to the high mitochondrial membrane potential (Δψm) depolarizes and the opening of mPTP, thus releasing mitochondria-derived molecules such as mROS, mtDNA and cardiolipin which produced during mitochondrial destabilization. The cardiolipin and ox-mtDNA release into cytosol and then bind to NLRP3, which might serve as NLRP3 ligands to activate the NLRP3 inflammasome. The infection of the virus accelerates the recruitment of NLRP3 to mitochondria and binds between NLRP3 and MAVS and regulates the mitochondrial destabilization and NLRP3 inflammasome activation in Drp1 phosphorylation-dependent ways. NLRP3, pyrin domain-containing protein 3; ER, endoplasmic reticulum; mPTP, mitochondrial permeability transition pore; MAVS, mitochondrial antiviral signaling protein; AMPK, AMP-activated protein kinase; RIP1/RIP3, receptor-interacting serine/threonine kinase 1 and RIP3; Δψm, mitochondrial membrane potential.
What is more, mitochondrial antiviral signaling protein (MAVS) is a critical regulator in the recruitment of NLRP3 to mitochondria, promoting the production of IL-1β and the pathophysiological activity of the NLRP3 inflammasome (Subramanian et al., 2013). Park et al. further discovered that MAVS not only accelerated the recruitment of NLRP3 to the mitochondria and brought it close to mtROS to improve its activation but also was involved in the assembly of NLRP3 inflammasome (Park et al., 2013). During mitochondrial destabilization, the recruitment of dynamin-related protein 1 (Drp1) on mitochondria leads to excessive mitochondrial fission, ultimately activating the NLRP3 inflammasome. It was conducted that AMP-activated protein kinase (AMPK) activation inhibited mitochondrial fission by upregulating Drp1 phosphorylation at serine637 (Ser637) in an AMPK-dependent manner to protect mitochondrial integrity and then, suppressed ER stress to inhibit the activation of NLRP3 inflammasome (Li et al., 2015, 2016; Guo et al., 2018b). What is more, it was also shown that receptor-interacting serine/threonine kinase (RIP) 1/RIP3 (RIP1-RIP3) complex could phosphorylate Drp1 at serine616 (Ser616) to activate and promote Drp1 translocation to mitochondria and ultimately contributed to the activation of NLRP3 inflammasome via (RIP1–RIP3)—Drp1 pathway (Wang et al., 2014). It has also proven that RIP3 regulates potassium efflux-dependent NLRP3 inflammasome activation via mixed-lineage kinase domain-like protein (MLKL)-induced pores and can be inhibited by supplementing extracellular potassium (Conos et al., 2017).
Lysosomal Damage
Pyrin domain-containing protein 3 activators induce lysosomal damage, which is indirectly sensed by the NLRP3 inflammasome (Hornung and Latz, 2010). Several reports attribute the activation of the NLRP3 inflammasome to the leakage of lysosomal contents into the cytosol following phagocytosis of particulate stimuli that could damage their integrity (Hornung et al., 2008; Shimada et al., 2012). Lysosomal rupture can release cathepsins and ROS, which also significantly impacted mitochondria membrane integrity and lead to membrane permeabilization (Shimada et al., 2012). Leu-Leu-O-methyl ester (LLME) is a lysosome-damaging compound when LLME is transported to the lysosome, resulting in lysosomal membrane permeability (LMP) (Hornung and Latz, 2010). It is proved that low-dose LLME causes mild LMP and strongly activates the inflammasome (Schilling, 2016). What is more, lysosomes are emerging as intracellular Ca2+ stores (Zhong et al., 2017), and the activity of lysosomal ion channels and transporters maintains concentration gradients of K+, Ca2+, Na+, and Cl− across the lysosomal membrane. Once the lysosomal damage occurs, it would contribute to the Ca2+ overloading and the disorder of lysosomal ion channel activity, which stimulates the activation of the NLRP3 inflammasome (Kendall and Holian, 2021). It has been proven that the restoration of lysosomal dysfunction could augment neuroprotection against ischemic stroke in neurons (Zhang et al., 2022).
NLRP3 Inflammasome in Cerebral I/R Injury
The main pathogenesis of ischemic stroke includes inflammation, oxidative stress, and programmed cell death (PCD) (Jin et al., 2010; Ren et al., 2021). With the involvement of the innate immune system, the activation and expression site of NLRP3 inflammasome act as a critical role in the development of ischemic stroke. In this study, we explained in detail the involvement of NLRP3 inflammasome in the pathogenesis of cerebral I/R injury, and the details are shown in Figure 4.
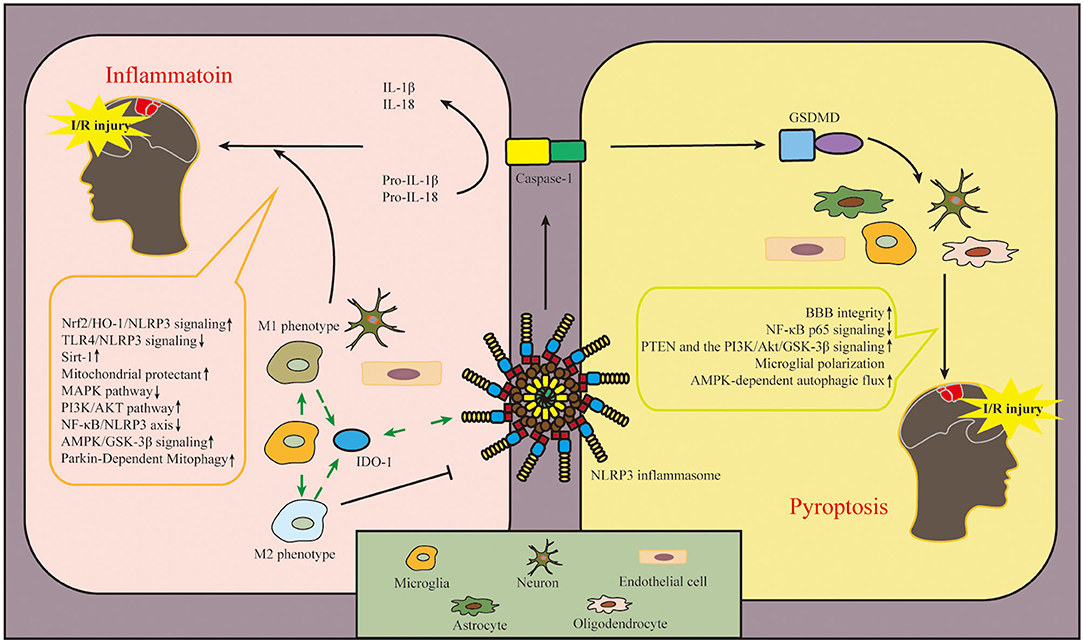
Figure 4. Diagram of NLRP3 inflammasome-related mechanisms after cerebral I/R injury. The involvement of NLRP3 inflammasome in the pathogenesis of cerebral I/R injury mainly includes two aspects: inflammation and pyroptosis. NLRP3 inflammasome is expressed in microglia, neuron, and endothelial cell to regulate inflammation after cerebral I/R injury. In addition, NLRP3 inflammasome is expressed in astrocytes, oligodendrocytes, microglia, neuron and endothelial cell involved with pyroptosis in ischemic tissue. In this figure, we also conclude the pathways in this review, which regulate the activation and inhibition of NLRP3 inflammasome in cerebral I/R injury. The upward arrow represents the suppression of NLRP3 inflammasome, and the downward arrow represents the activation of NLRP3 inflammasome. NLRP3, pyrin domain-containing protein 3; I/R, ischemia–reperfusion.
Inflammation
After cerebral I/R injury, various cellular responses are aroused, such as the activation of inflammatory cytokines and the accumulation of ROS and other oxygen free radicals (Fann et al., 2013; Guo et al., 2016), which exacerbate I/R injury by promoting brain oxidative stress, inflammation, and cerebral infarction volume (Jiang et al., 2019; Franke et al., 2021; Joaquim et al., 2021). The activation of the NLRP3 inflammasome plays an important role in the development of inflammation after cerebral I/R injury. NLRP3 inflammasome is first expressed in microglia and then in microvascular endothelial cells and neurons, but finally mainly in neurons at 24 h (Gong et al., 2018b).
Microglia
After ischemic I/R injury, the circulating macrophages were recruited to the ischemic tissue to be involved in cerebral I/R injury (Cai et al., 2018). As brain resident macrophages, microglia could be activated first after I/R injury, and macrophages/microglia change their M1 or M2 phenotype depending on the microenvironment of the central nervous system (CNS) (Hanisch and Kettenmann, 2007; Dong et al., 2021). M1 phenotype recognizes harmful stimuli and consequently generates inflammatory cytokines such as IL-1β, IL-6, and tumor necrosis factor-α (TNF-α) (Cherry et al., 2014). M2 phenotype shifts into an anti-inflammatory state where extracellular matrix deposition, debris clearance, and angiogenesis are promoted (Varin and Gordon, 2009). Regulating the polarization of macrophage/microglia could alleviate brain damage after ischemic stroke (Ye et al., 2019). Thirty minutes after modeling MCAO in mice, activated microglia were detected in the ischemic lesions (Rupalla et al., 1998). After ischemic stroke, DAMPs and PAMPs such as ischemia, hypoxia, and inflammatory factors could activate microglia (Gülke et al., 2018) and facilitate the proinflammatory role via hypoxia-inducible factor 1α (HIF-1α) in microglia (Yang et al., 2014). Additionally, anti-inflammatory factors, such as IL-10 and IL-4, could induce the M2 phenotype of microglia to protect against ischemic stroke (Xiong et al., 2015). As the monitoring of the microenvironment, immune system response after ischemic stroke can also affect the polarization of microglia, such as interferon regulatory factor (IRF) 4/5 signaling (Al Mamun et al., 2018). IRF5 is required for the M1 phenotype in microglia, and IRF4 was identified as a key transcription factor for M2 polarization in microglia (Al Mamun et al., 2018). In the mouse model of MCAO, the inhibition of NLRP3 inflammasome activation in activated microglia significantly improved functional neurological deficits (Sapkota and Choi, 2021). Additionally, the polarization of M2 microglia could protect against cerebral ischemic injury via the NF-E2–related factor 2 (Nrf2)/heme oxygenase-1 (HO-1)/NLRP3 pathway (Wang et al., 2021c). Therefore, it is therapeutic in ischemic stroke to inhibit inflammatory response via inhibiting phenotype switch of microglia and NLRP3 inflammasome activation.
Indoleamine 2,3-dioxygenase 1 (IDO-1) is an immunosuppressive metabolic enzyme and elicits neuroprotective effects on ischemic injury (Park et al., 2020). IDO-1 is mainly expressed in the macrophage/microglia of the perivascular but not the parenchymal microglia of the brain (Ji R. et al., 2021). As the downstream enzyme of the NLRP3 inflammasome, the inhibition of IDO-1 with curcumin decreased NLRP3 expression (Zhang W.-Y et al., 2019); in contrast, the inhibition of IDO-1 reduced NLRP3 expression, and inhibiting NLRP3 also increased IDO-1 expression, indicating that the relationship between NLRP3 and IDO-1 is bidirectional, and the direction depends on the activation status of macrophages/microglia (Ji R. et al., 2021). It is indicated that IDO-1 may decrease the expression of NLRP3 in macrophage/microglia of the perivascular space to inhibit inflammation and protect the integrity of the blood-brain barrier (BBB) against cerebral I/R injury.
Microvascular Endothelial Cell
As the important structure of BBB, microvascular endothelial cells play a vital role in cerebral I/R injury. With regard to I/R inflammation, the adhesion of neutrophils to vascular endothelial cells is fundamental to the development of I/R inflammation (Dong et al., 2019). It has been studied that endothelial cells can secrete inflammatory factors such as vascular cell adhesion molecule-1 (VCAM-1) to recruit neutrophils and/or lymphocytes, leading to the infiltration of inflammatory cells in the ischemic region (Gao et al., 2021). Lysophosphatidylcholine (LPC) is the main active component of oxidized low-density lipoproteins (ox-LDLs), and it has been proven that LPC could facilitate inflammatory response in brain microvascular endothelial cells (BMECs) via G protein-coupled receptor 4 (GPR4)-mediated activation of NLRP3 inflammasomes (Liu et al., 2021a). The expression inhibition of NLRP3 inflammasome in endothelial cells could improve the integrity of BBB and behavioral outcomes (Cao et al., 2016). The functional integrity of BMECs has shown a significant protective effect against brain I/R injury (Wang et al., 2021a).
Neuron
After the onset of cerebral I/R injury, cellular damage is mainly triggered by excitotoxicity, mitochondrial disturbances, dysfunction of the endoplasmic reticulum, ROS production, calcium toxicity, nitric oxide toxicity, zinc toxicity, and PCD (Lo et al., 2005; Hossmann, 2006). After the ischemic injury, neuron death occurs within minutes (Amantea et al., 2009), and the neuronal cell death after cerebral I/R injury included apoptosis, autophagy, pyroptosis, ferroptosis, parthanatos, phagoptosis, and necroptosis (Tuo et al., 2022). With the time prolongation, the neuron cell and nuclear membrane in the center of the ischemic region ruptured, and the cells were lysed (Gou et al., 2021). As mentioned earlier, the cellular damage could contribute to the activation of the NLRP3 inflammasome, ultimately exacerbating the inflammatory response. Based on the fact that NLRP3 inflammasome is ultimately mainly expressed in neurons after ischemic stroke, drugs that inhibit NLRP3 inflammasome to alleviate cerebral I/R injury and reduce inflammatory response must be studied.
Pyroptosis
Pyroptosis is one kind of pro-inflammatory PCD, which has been shown to be involved in cerebral I/R injury (Voet et al., 2019). Different from other PCD, pyroptosis is characterized by the rupture of the plasma membrane to form pores, increases in cell permeability, and the release of inflammatory cytokines (Fink and Cookson, 2005). The occurrence of pyroptosis relies on caspase-1/4/5/11, and caspase-1 and caspase-11 are the main activated inflammatory caspases during cerebral ischemia (Gou et al., 2021). The oxidative stress and inflammatory response activate the NLRP3 inflammasome and consequently induce the cleave of pro-caspase-1 to form active caspase-1, which ultimately promotes pyroptosis via the canonical inflammasome pathway (Broz, 2015). Caspase-1 cleaves pro-IL-1β and pro-IL-18 into a mature form of IL-1β and IL-18. Caspase-1 could also cleave Gasdermin D (GSDMD), expose Asp280 amino acid sites, and promote the recruitment of the N-domain of GSDMD to the cell membrane, leading to the cell membrane pore formation and pyroptosis (Zhang et al., 2019; Ji et al., 2021), thereby releasing IL-1β and IL-18, and leading to cascade inflammatory response and inflammatory cell recruitment (Vande Walle and Lamkanfi, 2016).
Pyroptosis has been shown to be expressed in various CNS cells, such as microglia, neuron, oligodendrocytes, and astrocytes (McKenzie et al., 2018; Zhou et al., 2019; Hu et al., 2021; Zhao et al., 2021). Additionally, it has been proven that the pyroptosis of endothelial cells participates in the pathogenesis of cerebral I/R injury (Wang et al., 2021d). Regarding the critical downstream effector of pyroptosis (Shi et al., 2015), the expression of GSDMD was found to increase after I/R and peak at 3–5 days in mice (Lu et al., 2021) and so aggravates I/R-induced cerebral infarction and brain injury. The ablation of GSDMD exerts a neuroprotective effect by inhibiting microglia pyroptosis in mice after cerebral I/R injury (Wang et al., 2020a). Moreover, the inhibition of NLRP3 inflammasome-dependent pyroptosis could reduce neuronal injury and cerebral infarct after I/R injury (Kang et al., 2021; Shi et al., 2022). Regarding the upstream of pyroptosis, the suppression of NLRP3 inflammasome is a critical target for the treatment of cerebral I/R injury.
Therapeutic Approaches Targeting NLRP3 Inflammasome for Cerebral I/R Injury
As a neurological disease with a high disability rate, ischemic stroke still lacks efficient therapeutic treatment in the clinic. As previously mentioned, NLRP3 inflammasome plays a major role in cerebral I/R injury, and many drugs that inhibit NLRP3 inflammasome activation have been studied for the treatment of ischemic stroke. In this study, we summarized the therapeutic approaches involving NLRP3 inflammasome and classified these drugs as follows: clinical treatment, herbal/natural component, and novel inhibitor.
Novel Inhibitor
To find out the potential pathogenesis and therapeutic drugs for cerebral I/R injury application, many novel inhibitors have been found to mitigate cerebral I/R injury by suppressing the activation of the NLRP3 inflammasome. The most well-studied inhibitor is MCC950, which is proven specificity in inhibiting NLRP3 inflammasome targeting for the assembly of NLRP3 inflammasome (Wu et al., 2020). MCC950 can modify the active conformation of NLRP3, and it prevents NLRP3 oligomerization in response to external stimulation (Tapia-Abellán et al., 2019). Experimental studies have found that the treatment of MCC950 could reduce the infarction and edema and improved neurological deficits and BBB integrity via inhibiting inflammatory cytokines, pyroptosis, and brain oxidative stress in the ischemic region after MCAO (Ismael et al., 2018; Bellut et al., 2021; Joaquim et al., 2021). CY-09 could inhibit ATPase activity and block NLRP3 oligomerization to inhibit the activation of NLRP3 inflammasome (Jiang et al., 2017). It has shown the therapeutic effect of CY-09 in cerebral I/R injury via inhibiting NLRP3 inflammasome-induced inflammation and pyroptosis (Sun et al., 2020; Franke et al., 2021). Oridonin could prevent NLRP3 inflammasome complex assembly against NLRP3 inflammasome activation (He et al., 2018). The study found oridonin prevented oxidative stress-induced endothelial injury via promoting the Nrf2 pathway and thereby repaired BBB integrity, alleviated neuroinflammation, and infarct volume after ischemic stroke (Li et al., 2021a).
Clinical Treatment
Although the pathogenesis of ischemic stroke remains unclear, many conventional medicines revealed the therapeutic effect on ischemic stroke function by inhibiting the activation of NLRP3 inflammasome. As an oxygen radical scavenger, Edaravone reduced neurotoxicity, oxidative stress, and inflammatory response after cerebral I/R injury (Xu et al., 2021a). Indobufen and Aspirin and their Combinations with Clopidogrel or Ticagrelor (IACT) could alleviate pyroptosis via NF-κB/NLRP3 pathway after cerebral I/R injury, which indicated that the combination of antiplatelet drugs is a promising strategy for the curation of cerebral I/R injury (Li et al., 2021c). In addition, idebenone, as a mitochondrial protectant, was found to decrease ROS and cytosolic oxidized mtDNA, suppress uncontrolled NLRP3 activation, and consequently alleviate cerebral inflammatory response after cerebral I/R injury (Peng et al., 2020). Hypothermia is used for clinical neuroprotective purposes after ischemic stroke. It was suggested that hypothermia downregulated the expression of NLRP3 and attenuated I/R-induced pyroptosis partially via the phosphatidylinositol-3-kinase (PI3K)/Akt/Glycogen synthase kinase-3β (GSK-3β) pathway (Diao et al., 2020). Electroacupuncture (EA) is commonly used to relieve chronic pain and stroke rehabilitation, and some studies have uncovered that EA pretreatment protects against transient cerebral I/R injury (Wang et al., 2012). EA stimulus has a neuroprotective effect through α7nAChR by modulating the inhibition of NLRP3 inflammasome-associated inflammatory response and cellular apoptosis (Jiang et al., 2019).
Herbal/Natural Component
Herbs andtheir extracts have been an important source of approach for the treatment of ischemic stroke, many herbal medicines have shown neuroprotection after cerebral I/R injury via inhibiting NLRP3 inflammasome activation, and the detailed information is summarized in Table 2. Tongxinluo (TXL) is a common Chinese patent drug used clinically in the treatment of stroke, and TXL could protect ischemic brain tissues against pyroptosis in astrocytic by inactivating caspase-11/GSDMD (Wang et al., 2021b). Xingnaojing injection (XNJ) is isolated from famous traditional Chinese medicine prescriptions named An-Gong-Niu-Huang Wan, which is well-accepted in the clinic due to its significant therapeutic effect (Lai et al., 2017). XNJ ameliorated neurological deficits and BBB disruption following I/R injury in a manner of NLRP3 inflammasome suppression (Qu et al., 2019). The effective compounds extracted from Buyang Huanwu Decoction (BYHWD) can alleviate neuronal damage and inhibit NLRP3 inflammasome-mediated neuronal pyroptosis (She et al., 2019). Hispidulin is a component widely existing in traditional Chinese medicine and could inhibit I/R-induced pyroptosis in the ischemic cortex by modulating AMPK/GSK3β signaling (An et al., 2019). Resveratrol, anthocyanin, melodinhenine B, and 6-Gingerol could alleviate cerebral I/R injury by inhibiting NLRP3 inflammasome activation (He et al., 2017; Cui et al., 2018; Li et al., 2020a; Luo et al., 2021). Icariin, a flavonol glycoside extracted from Epimedium brevicornum Maxim (Berberidaceae), has shown an anti-inflammatory effect against Oxygen and Glucose Deprivation/Reoxygenation (OGD/R) through the inositol-requiring enzyme-1 (IRE1)/X-box binding protein 1 (XBP1) pathway in microglia (Mo et al., 2021). Moreover, the pretreatment of sulforaphane (SFN) in the ischemic stroke model could protect neurovascular and alleviate neurological deficits and BBB disruption via the Nrf2/HO-1 defense pathway (Alfieri et al., 2013; Warpsinski et al., 2020). Herbal medicines especially traditional Chinese medicines have been an essential approach for the recovery of ischemic stroke, identifying natural herbs which ameliorate I/R injury via NLRP3 inflammasome that has prospective value.
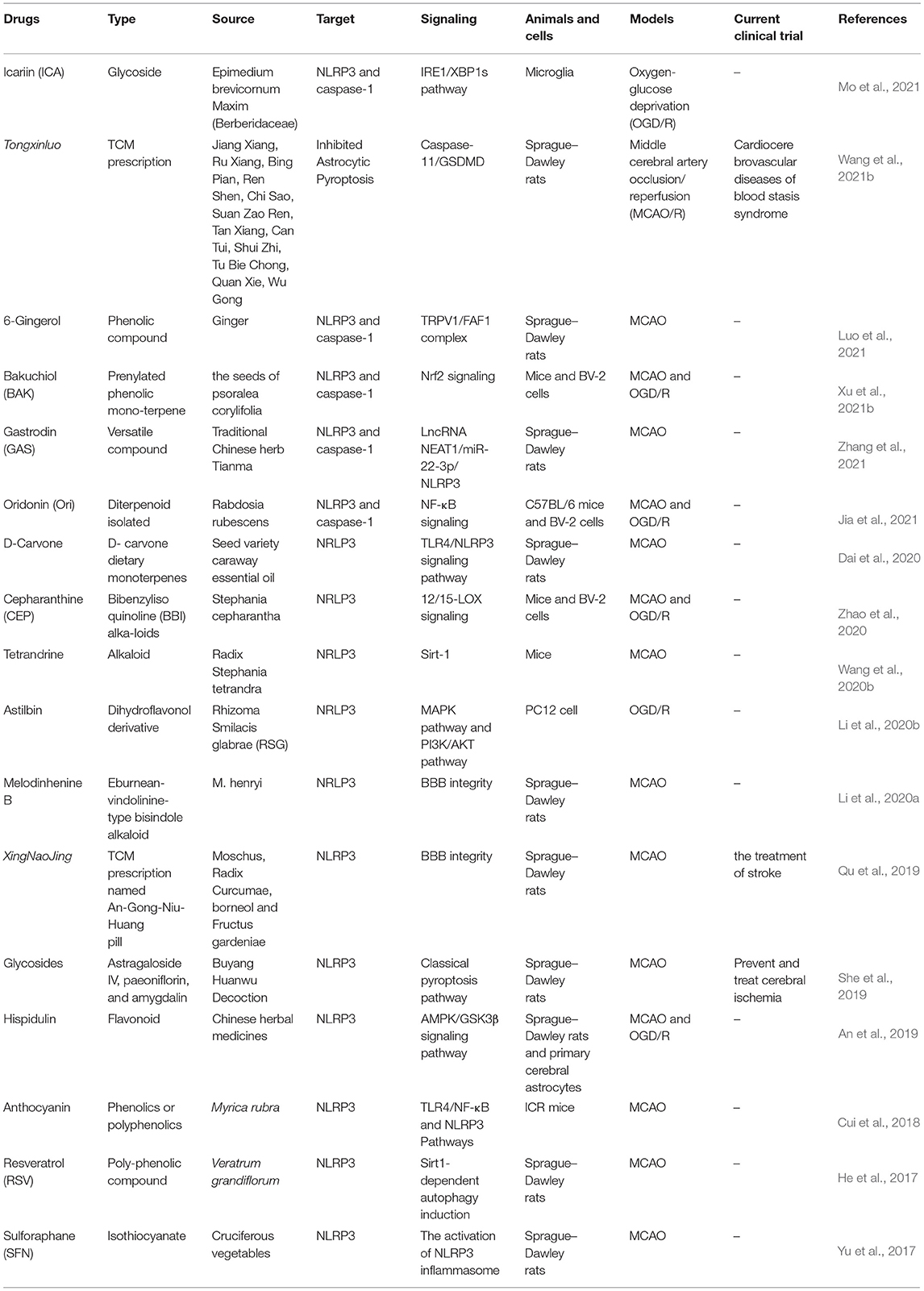
Table 2. Herbal drugs that target NLRP3 inflammasome after cerebral ischemia–reperfusion (I/R) injury.
Others
Intriguingly, non-coding RNA (ncRNA), including long non-coding RNA and microRNA, is involved in the pyroptosis, inflammatory response, oxidative stress, apoptosis, and BBB permeability in a manner of NLRP3 inflammasome after cerebral I/R injury (Ghafouri-Fard et al., 2020), and all the information is shown in Table 3. It is of great importance to develop drugs that inhibit NLRP3 by targeting ncRNAs.
Bone marrow mesenchymal stem cell-derived exosomes (BMSC-Exos) can attenuate the activation of NLRP3 inflammasome and NLRP3 inflammasome-mediated pyroptosis via promoting AMPK-dependent autophagic flux in OGD/R injury (Zeng et al., 2020), by a mechanism that switches microglial phenotypes from M1 to M2, so as to ameliorate cerebral I/R injury (Liu et al., 2021b). Moreover, intermittent fasting can attenuate the inflammation and neuronal damage following cerebral I/R injury through the suppression of NLRP3 inflammasome activation (Fann et al., 2014). Additionally, an enriched environment (EE) could rescue neurological deficits after I/R injury via inhibiting the activities of NLRP3 inflammasome and attenuating neuronal pyroptosis (Liu et al., 2021c).
The continuous studies for NLRP3 inflammasome will promote the new drug research and development for cerebral I/R injury clinical treatment, but further research is needed for the clinical application of new compounds.
Discussion
Despite efforts to understand the pathophysiology of ischemic stroke, no effective neuroprotective drugs have been identified to modulate brain damage following ischemic stroke in human due to the complexity of ischemic stroke. With a fall of cerebral blood flow in ischemic stroke, the ischemic region contains two aspects, namely, ischemic core and penumbra, the damage to the brain in the penumbra is reversible based on the ionic homeostasis and transmembrane electrical potentials (Astrup et al., 1981), and how to restore blood flow in penumbra is a therapeutic target for the ischemic stroke clinic treatment (Ramos-Cabrer et al., 2011). Due to the sudden and rapid onset, ischemic stroke is often treated out of the therapeutic time window and, therefore, produces irreversible neuronal death (Sommer, 2017). As the most well-characterized inflammasome, NLRP3 inflammasome is closely related to the inflammatory response and pyroptosis; therefore, the blocking of NLRP3 inflammasome becomes a significant therapeutic target for ischemic stroke. Therefore, it is meaningful to downregulate the expression of NLRP3 inflammasome after cerebral I/R injury. The abnormal expression of NLRP3 inflammasome is not only detected in lab experiments but also is exactly confirmed in burgeoning clinical evidence (Chen et al., 2013; Zheng et al., 2013). It is shown that the neuronal upregulation of NLRP3 is an early event within the first 24 h of cerebral I/R injury which corresponds to the hyperacute and acute phase of human stroke (Franke et al., 2021). After the onset of ischemic stroke, the inhibition of NLRP3 inflammasome according to its expression time sequence could be considered as a future therapeutic target. Within 24 h of the occurrence of ischemic stroke, the early activation of NLRP3 inflammasome in microglia and subsequent activation in neurons should be effectively targeted according to the cell type, thereby, reducing cerebral I/R injury (Chumboatong et al., 2022; Wang et al., 2022), but the exact time needs further experimental studies. The NLRP3 inflammasome is a critical part of the innate immune system which regulates the cleaves and secretion of proinflammatory cytokines, such as IL-1β and IL-18 in response to the DAMP and PAMP signaling. Inflammatory cytokines are involved with the secondary brain injury in cerebral I/R, especially IL-1β and IL-18. It is influential for the treatment of cerebral I/R to regulate the expression of inflammatory cytokines under control, thus the involvement with inflammatory response makes NLRP3 inflammasome extensively investigated for assembly and activation. In addition to inflammation, the activation of NLRP3 inflammasome in ischemic brain tissue promotes the activities of pyroptosis and eventually aggravates brain injury. According to the activation and assembly of the NLRP3 inflammasome, molecules and compounds interfering with NLRP3 activation can alleviate cerebral I/R injury by downregulating the expression of the NLRP3 inflammasome in animal experiments. Importantly, these inhibitors have not been conducted in clinical trials due to their limited pharmacokinetic profiles and safety. Moreover, it is proven that the impact of the unspecific medications (SFN, Genipin) on the inflammasomes besides NLRP3 is negligible in the treatment of ischemic stroke (Franke et al., 2021). Therefore, highly specific and efficient inhibitors that focus on inhibiting the NLRP3 inflammasome and can effectively permeate the cell membrane and BBB will be an important topic for their research and clinical application.
Interestingly, it is a hot spot where mitochondria are closely related to NLRP3 inflammasome activation. Mitochondria are the central hub in innate and adaptive immune cells (Breda et al., 2019), and at the same time, mitochondria also participate in pyroptosis, a kind of cell death, which is related to cerebral I/R injury (Gurung et al., 2015). NLRP3 inflammasome triggers caspase-1-dependent mitochondrial damage. Caspase-1 activates multiple pathways to precipitate mitochondrial disassembly, which leads to the mROS production and dissipation of mitochondrial membrane potential, mitochondrial permeabilization, and mitochondrial network fragmentation (Yu et al., 2014). The molecules derived from mitochondria such as mROS, mtDNA, and cardiolipin are important regulators for the activation of the inflammasome, and mtDNA and cardiolipin are found to bind to NLRP3 and serve as a ligand for the activation of NLRP3 inflammasome. Now that the proven studies conducted that mitochondria are essential for NLRP3 inflammasome activation, the further studies are kindly suggested to focus on the specific mechanism by which mitochondria regulate the activation of NLRP3 inflammasomes.
Conclusion and Future Perspective
The role of NLRP3 inflammasome in cerebral I/R injury is mainly concentrated on NLRP3 inflammasome-dependent cytokine release and pyroptosis, which makes NLRP3 inflammasome a target therapeutic protein in cerebral I/R injury. Although the essential of NLRP3 inflammasome activation in ischemic stroke has been proved, its specific role needs to be further explored. It is expected that the more effective pharmacokinetic profiles and safe medicines for NLRP3 inflammasome could be used for cerebral I/R injury treatments in clinical.
Author Contributions
This study is finished under the guidance of SY. LW and WR proposed the idea of this manuscript. LW wrote the original draft. WR critically edited and revised the manuscript. QW, HX, and TL are responsible for the editing. YW, JD, and CZ investigated the literature. All authors contributed to the article and approved the submitted version.
Funding
This study was supported by the Science and Technology Department of Sichuan Province (Grant No. 2019YFS0543); Sichuan Administration of Traditional Chinese Medicine (2020ZD002, 2021ZD015, and 2020JC0150); the strategic cooperation project between Luzhou Municipal People's Government (2019LZXNYDC02); China Postdoctoral Science Foundation (Grant No. 2020M683365); and the National Natural Science Foundation of China (Grant No. 82074378).
Conflict of Interest
The authors declare that the research was conducted in the absence of any commercial or financial relationships that could be construed as a potential conflict of interest.
Publisher's Note
All claims expressed in this article are solely those of the authors and do not necessarily represent those of their affiliated organizations, or those of the publisher, the editors and the reviewers. Any product that may be evaluated in this article, or claim that may be made by its manufacturer, is not guaranteed or endorsed by the publisher.
Acknowledgments
We acknowledge the support of supervisor SY and appreciate the support of the National Natural Science Foundation of China, Science and Technology Department of Sichuan Province. The authors are sincerely thankful to all individuals who were involved in this study.
References
Abderrazak, A., Syrovets, T., Couchie, D., El Hadri, K., Friguet, B., Simmet, T., et al. (2015). NLRP3 inflammasome: from a danger signal sensor to a regulatory node of oxidative stress and inflammatory diseases. Redox Biol. 4, 296–307. doi: 10.1016/j.redox.2015.01.008
Al Mamun, A., Chauhan, A., Yu, H., Xu, Y., Sharmeen, R., and Liu, F. (2018). Interferon regulatory factor 4/5 signaling impacts on microglial activation after ischemic stroke in mice. Eur. J. Neurosci. 47, 140–149. doi: 10.1111/ejn.13778
Alfieri, A., Srivastava, S., Siow, R. C. M., Cash, D., Modo, M., Duchen, M. R., et al. (2013). Sulforaphane preconditioning of the Nrf2/HO-1 defense pathway protects the cerebral vasculature against blood-brain barrier disruption and neurological deficits in stroke. Free Radic. Biol. Med. 65, 1012–1022. doi: 10.1016/j.freeradbiomed.2013.08.190
Alishahi, M., Farzaneh, M., Ghaedrahmati, F., Nejabatdoust, A., Sarkaki, A., and Khoshnam, S. E. (2019). NLRP3 inflammasome in ischemic stroke: as possible therapeutic target. Int. J. Stroke. 14, 574–591. doi: 10.1177/1747493019841242
Amantea, D., Nappi, G., Bernardi, G., Bagetta, G., and Corasaniti, M. T. (2009). Post-ischemic brain damage: pathophysiology and role of inflammatory mediators. FEBS J. 276, 13–26. doi: 10.1111/j.1742-4658.2008.06766.x
An, P., Xie, J., Qiu, S., Liu, Y., Wang, J., Xiu, X., et al. (2019). Hispidulin exhibits neuroprotective activities against cerebral ischemia reperfusion injury through suppressing NLRP3-mediated pyroptosis. Life Sci. 232, 116599. doi: 10.1016/j.lfs.2019.116599
Astrup, J., Siesjö, B. K., and Symon, L. (1981). Thresholds in cerebral ischemia - the ischemic penumbra. Stroke 12, 723–725. doi: 10.1161/01.STR.12.6.723
Barrington, J., Lemarchand, E., and Allan, S. M. (2017). A brain in flame; do inflammasomes and pyroptosis influence stroke pathology? Brain Pathol. 27, 205–212. doi: 10.1111/bpa.12476
Bellut, M., Papp, L., Bieber, M., Kraft, P., Stoll, G., and Schuhmann, M. K. (2021). NLPR3 inflammasome inhibition alleviates hypoxic endothelial cell death in vitro and protects blood-brain barrier integrity in murine stroke. Cell Death Dis. 13, 20. doi: 10.1038/s41419-021-04379-z
Breda, C. N. D. S., Davanzo, G. G., Basso, P. J., Saraiva Câmara, N. O., and Moraes-Vieira, P. M. M. (2019). Mitochondria as central hub of the immune system. Redox Biol. 26, 101255. doi: 10.1016/j.redox.2019.101255
Broz, P. (2015). Immunology: Caspase target drives pyroptosis. Nature 526, 642–643. doi: 10.1038/nature15632
Cai, W., Liu, S., Hu, M., Sun, X., Qiu, W., Zheng, S., et al. (2018). Post-stroke DHA treatment protects against acute ischemic brain injury by skewing macrophage polarity toward the M2 phenotype. Transl. Stroke Res. 9, 669–680. doi: 10.1007/s12975-018-0662-7
Cao, G., Jiang, N., Hu, Y., Zhang, Y., Wang, G., Yin, M., et al. (2016). Ruscogenin attenuates cerebral ischemia-induced blood-brain barrier dysfunction by suppressing TXNIP/NLRP3 inflammasome activation and the MAPK pathway. Int. J. Mol. Sci. 17, 1418. doi: 10.3390/ijms17091418
Chen, D., Xiong, X. Q., Zang, Y. H., Tong, Y., Zhou, B., Chen, Q., et al. (2017). BCL6 attenuates renal inflammation via negative regulation of NLRP3 transcription. Cell Death Dis. 8, e3156. doi: 10.1038/cddis.2017.567
Chen, F., Jiang, G. W., Liu, H., Li, Z. M., Pei, Y. X., Wang, H., et al. (2020). Melatonin alleviates intervertebral disc degeneration by disrupting the IL-1β/NF-κB-NLRP3 inflammasome positive feedback loop. Bone Res. 8, 10. doi: 10.1038/s41413-020-0087-2
Chen, K. H., Feng, L., Hu, W., Chen, J., Wang, X. Y., Wang, L. M., et al. (2019a). Optineurin inhibits NLRP3 inflammasome activation by enhancing mitophagy of renal tubular cells in diabetic nephropathy. FASEB J. 33, 4571–85. doi: 10.1096/fj.201801749RRR
Chen, S., Ma, Q., Krafft, P. R., Hu, Q., Rolland, W., Sherchan, P., et al. (2013). P2X7R/cryopyrin inflammasome axis inhibition reduces neuroinflammation after SAH. Neurobiol Dis. 58, 296–307. doi: 10.1016/j.nbd.2013.06.011
Chen, Y. H., Meng, J., Bi, F. F., Li, H., Chang, C. C., Ji, C., et al. (2019b). EK7 regulates NLRP3 inflammasome activation and neuroinflammation post-traumatic brain injury. Front Mol Neurosci. 12, 202. doi: 10.3389/fnmol.2019.00202
Cherry, J. D., Olschowka, J. A., and O'Banion, M. K. (2014). Neuroinflammation and M2 microglia: the good, the bad, and the inflamed. J. Neuroinflammation. 11, 98. doi: 10.1186/1742-2094-11-98
Chumboatong, W., Khamchai, S., Tocharus, C., Govitrapong, P., and Tocharus, J. (2022). Agomelatine exerts an anti-inflammatory effect by inhibiting microglial activation through TLR4/NLRP3 pathway in pMCAO Rats. Neurotox. Res. 40, 259–266. doi: 10.1007/s12640-021-00447-6
Conos, S. A., Chen, K. W., De Nardo, D., Hara, H., Whitehead, L., Núñez, G., et al. (2017). Active MLKL triggers the NLRP3 inflammasome in a cell-intrinsic manner. Proc. Natl. Acad. Sci. U.S.A. 114, E961–E9. doi: 10.1073/pnas.1613305114
Cui, D. L, Liu, S. Y, Tang, M. H, Lu, Y. Z, Zhao, M., Mao, R. W., et al. (2020). Phloretin ameliorates hyperuricemia-induced chronic renal dysfunction through inhibiting NLRP3 inflammasome and uric acid reabsorption. Phytomedicine 66, 153111. doi: 10.1016/j.phymed.2019.153111
Cui, H.-X., Chen, J.-H., Li, J.-W., Cheng, F.-R., and Yuan, K. (2018). Protection of anthocyanin from against cerebral ischemia-reperfusion injury via modulation of the TLR4/NF-κB and NLRP3 pathways. Molecules 23, 1788. doi: 10.3390/molecules23071788
Dagvadorj, J., Mikulska-Ruminska, K., Tumurkhuu, G., Ratsimandresy, R. A., Carriere, J., Andres, A. M., et al. (2021). Recruitment of pro-IL-1α to mitochondrial cardiolipin, via shared LC3 binding domain, inhibits mitophagy and drives maximal NLRP3 activation. Proc. Natl. Acad. Sci. U.S.A. 118, e2015632118. doi: 10.1073/pnas.2015632118
Dai, M., Wu, L., Yu, K., Xu, R., Wei, Y., Chinnathambi, A., et al. (2020). D-Carvone inhibit cerebral ischemia/reperfusion induced inflammatory response TLR4/NLRP3 signaling pathway. Biomed. Pharmacother. 132, 110870. doi: 10.1016/j.biopha.2020.110870
Dan Dunn, J., Alvarez, L. A., Zhang, X. Z., and Soldati, T. (2015). Reactive oxygen species and mitochondria: a nexus of cellular homeostasis. Redox Biol. 6, 472–485. doi: 10.1016/j.redox.2015.09.005
Dempsey, C., Rubio Araiz, A., Bryson, K. J., Finucane, O., Larkin, C., Mills, E. L., et al. (2017). Inhibiting the NLRP3 inflammasome with MCC950 promotes non-phlogistic clearance of amyloid-β and cognitive function in APP/PS1 mice. Brain Behav. Immun. 61, 306–316. doi: 10.1016/j.bbi.2016.12.014
Di, A., Xiong, S. Q., Ye, Z. M., Malireddi, R. K. S., Kometani, S., Zhong, M., et al. (2018). The TWIK2 potassium efflux channel in macrophages mediates nlrp3 inflammasome-induced inflammation. Immunity 49, 56–65.e4. doi: 10.1016/j.immuni.2018.04.032
Diao, M.-Y., Zhu, Y., Yang, J., Xi, S.-S., Wen, X., Gu, Q., et al. (2020). Hypothermia protects neurons against ischemia/reperfusion-induced pyroptosis via m6A-mediated activation of PTEN and the PI3K/Akt/GSK-3β signaling pathway. Brain Res. Bull. 159, 25–31. doi: 10.1016/j.brainresbull.2020.03.011
Dong, R., Huang, R., Wang, J., Liu, H., and Xu, Z. (2021). Effects of microglial activation and polarization on brain injury after stroke. Front. Neurol. 12, 620948. doi: 10.3389/fneur.2021.620948
Dong, X., Gao, J., Zhang, C. Y., Hayworth, C., Frank, M., and Wang, Z. (2019). Neutrophil membrane-derived nanovesicles alleviate inflammation to protect mouse brain injury from ischemic stroke. ACS Nano. 13, 1272–1283. doi: 10.1021/acsnano.8b06572
Du, R. H., Lu, M., Wang, C., Ding, J. H., Wu, G.y, and Hu, G. (2019). The pore-forming subunit Kir6.1 of the K-ATP channel negatively regulates the NLRP3 inflammasome to control insulin resistance by interacting with NLRP3. Exp. Mol. Med. 51, 1–13. doi: 10.1038/s12276-019-0291-6
Du, R. H., Tan, J., Sun, X. Y., Lu, M., Ding, J. H., and Hu, G. (2016). Fluoxetine inhibits NLRP3 inflammasome activation: implication in depression. Int. J. Neuropsychopharmacol. 19, pyw037. doi: 10.1093/ijnp/pyw037
Fann, D. Y., Lee, S. Y., Manzanero, S., Tang, S. C., Gelderblom, M., Chunduri, P., et al. (2013). Intravenous immunoglobulin suppresses NLRP1 and NLRP3 inflammasome-mediated neuronal death in ischemic stroke. Cell Death Dis. 4, e790. doi: 10.1038/cddis.2013.326
Fann, D. Y.-W., Santro, T., Manzanero, S., Widiapradja, A., Cheng, Y.-L., Lee, S.-Y., et al. (2014). Intermittent fasting attenuates inflammasome activity in ischemic stroke. Exp. Neurol. 257, 114–119. doi: 10.1016/j.expneurol.2014.04.017
Fernández-López, D., Faustino, J., Klibanov, A. L., Derugin, N., Blanchard, E., Simon, F., et al. (2016). Microglial cells prevent hemorrhage in neonatal focal arterial stroke. J. Neurosci. 36, 2881–2893. doi: 10.1523/JNEUROSCI.0140-15.2016
Fink, S. L., and Cookson, B. T. (2005). Apoptosis, pyroptosis, and necrosis: mechanistic description of dead and dying eukaryotic cells. Infect. Immun. 73, 1907–1916. doi: 10.1128/IAI.73.4.1907-1916.2005
Franchi, L., Eigenbrod, T., Muñoz-Planillo, R., Ozkurede, U., Kim, Y. G., Arindam, C., et al. (2014). Cytosolic double-stranded RNA activates the NLRP3 inflammasome via MAVS-induced membrane permeabilization and K+ efflux. J. Immunol. 193, 4214–4222. doi: 10.4049/jimmunol.1400582
Franke, M., Bieber, M., Kraft, P., Weber, A. N. R., Stoll, G., and Schuhmann, M. K. (2021). The NLRP3 inflammasome drives inflammation in ischemia/reperfusion injury after transient middle cerebral artery occlusion in mice. Brain Behav. Immun. 92, 223–233. doi: 10.1016/j.bbi.2020.12.009
Fu, Q., Zhai, Z. S., Wang, Y. Z., Xu, L. X., Jia, P. C., Xia, P., et al. (2018). NLRP3 deficiency alleviates severe acute pancreatitis and pancreatitis-associated lung injury in a mouse model. Biomed Res. Int. 2018, 1294951. doi: 10.1155/2018/1294951
Fujisue, K., Sugamura, K., Kurokawa, H., Matsubara, J., Ishii, M., Izumiya, Y., et al. (2017). Colchicine improves survival, left ventricular remodeling, and chronic cardiac function after acute myocardial infarction. Circ. J. 81, 1174–1182. doi: 10.1253/circj.CJ-16-0949
Gao, C., Jia, W., Xu, W., Wu, Q., and Wu, J. (2021). Downregulation of CD151 restricts VCAM-1 mediated leukocyte infiltration to reduce neurobiological injuries after experimental stroke. J. Neuroinflammation 18, 118. doi: 10.1186/s12974-021-02171-6
Ghafouri-Fard, S., Shoorei, H., and Taheri, M. (2020). Non-coding RNAs participate in the ischemia-reperfusion injury. Biomed. Pharmacother. 129, 110419. doi: 10.1016/j.biopha.2020.110419
Gianfrancesco, M. A., Dehairs, J., L'Homme, L., Herinckx, G., Esser, N., Jansen, O., et al. (2019). Saturated fatty acids induce NLRP3 activation in human macrophages through K efflux resulting from phospholipid saturation and Na, K-ATPase disruption. Biochim. Biophys. Acta Mol. Cell Biol. Lipids 1864, 1017–1030. doi: 10.1016/j.bbalip.2019.04.001
Goldberg, E. L., Asher, J. L., Molony, R. D., Shaw, A. C., Zeiss, C. J., Wang, C., et al. (2017). β-Hydroxybutyrate deactivates neutrophil NLRP3 inflammasome to relieve gout flares. Cell Rep. 18, 2077–2087. doi: 10.1016/j.celrep.2017.02.004
Gong, T., Yang, Y. Q., Jin, T. C., Jiang, W., and Zhou, R. B. (2018a). Orchestration of NLRP3 inflammasome activation by ion fluxes. Trends Immunol. 39, 393–406. doi: 10.1016/j.it.2018.01.009
Gong, Z., Pan, J., Shen, Q. Y., Li, M., and Peng, Y. (2018b). Mitochondrial dysfunction induces NLRP3 inflammasome activation during cerebral ischemia/reperfusion injury. J. Neuroinflammation 15, 242. doi: 10.1186/s12974-018-1282-6
Gou, X., Xu, D., Li, F., Hou, K., Fang, W., and Li, Y. (2021). Pyroptosis in stroke-new insights into disease mechanisms and therapeutic strategies. J. Physiol. Biochem. 77, 511–529. doi: 10.1007/s13105-021-00817-w
Groß, C. J., Mishra, R., Schneider, K. S., Médard, G., Wettmarshausen, J., Dittlein, D. C., et al. (2016). K efflux-independent NLRP3 inflammasome activation by small molecules targeting mitochondria. Immunity 45, 761–773. doi: 10.1016/j.immuni.2016.08.010
Gülke, E., Gelderblom, M., and Magnus, T. (2018). Danger signals in stroke and their role on microglia activation after ischemia. Ther. Adv. Neurol. Disord. 11, 1756286418774254. doi: 10.1177/1756286418774254
Guo, C., Fu, R., Wang, S., Huang, Y., Li, X., Zhou, M., et al. (2018a). NLRP3 inflammasome activation contributes to the pathogenesis of rheumatoid arthritis. Clin. Exp. Immunol. 194, 231–243. doi: 10.1111/cei.13167
Guo, M., Wang, X., Zhao, Y. X., Yang, Q., Ding, H. Y., Dong, Q., et al. (2018b). Ketogenic diet improves brain ischemic tolerance and inhibits NLRP3 inflammasome activation by preventing Drp1-mediated mitochondrial fission and endoplasmic reticulum stress. Front. Mol. Neurosci. 11, 86. doi: 10.3389/fnmol.2018.00086
Guo, W. J., Liu, W., Chen, Z., Gu, Y. H., Peng, S., Shen, L. H., et al. (2017). Tyrosine phosphatase SHP2 negatively regulates NLRP3 inflammasome activation via ANT1-dependent mitochondrial homeostasis. Nat. Commun. 8, 2168. doi: 10.1038/s41467-017-02351-0
Guo, Z., Yu, S., Chen, X., Ye, R., Zhu, W., and Liu, X. (2016). NLRP3 is involved in ischemia/reperfusion injury. CNS Neurol. Disord. Drug Targets 15, 699–712. doi: 10.2174/1871527315666160321111829
Gurung, P., Lukens, J. R., and Kanneganti, T.-D. (2015). Mitochondria: diversity in the regulation of the NLRP3 inflammasome. Trends Mol. Med. 21, 193–201. doi: 10.1016/j.molmed.2014.11.008
Hanisch, U.-K., and Kettenmann, H. (2007). Microglia: active sensor and versatile effector cells in the normal and pathologic brain. Nat. Neurosci. 10, 1387–1394. doi: 10.1038/nn1997
He, H., Jiang, H., Chen, Y., Ye, J., Wang, A., Wang, C., et al. (2018). Oridonin is a covalent NLRP3 inhibitor with strong anti-inflammasome activity. Nat. Commun. 9, 2550. doi: 10.1038/s41467-018-04947-6
He, J., and Zhang, X. (2020). miR-668 inhibitor attenuates mitochondrial membrane potential and protects against neuronal apoptosis in cerebral ischemic stroke. Folia Neuropathol. 58, 22–29. doi: 10.5114/fn.2020.94003
He, Q., Li, Z., Wang, Y., Hou, Y., Li, L., and Zhao, J. (2017). Resveratrol alleviates cerebral ischemia/reperfusion injury in rats by inhibiting NLRP3 inflammasome activation through Sirt1-dependent autophagy induction. Int. Immunopharmacol. 50, 208–215. doi: 10.1016/j.intimp.2017.06.029
Hornung, V., Bauernfeind, F., Halle, A., Samstad, E. O., Kono, H., Rock, K. L., et al. (2008). Silica crystals and aluminum salts activate the NALP3 inflammasome through phagosomal destabilization. Nat. Immunol. 9, 847–856. doi: 10.1038/ni.1631
Hornung, V., and Latz, E. (2010). Critical functions of priming and lysosomal damage for NLRP3 activation. Eur. J. Immunol. 40, 620–623. doi: 10.1002/eji.200940185
Hossmann, K.-A. (2006). Pathophysiology and therapy of experimental stroke. Cell. Mol. Neurobiol. 26, 1057–1083. doi: 10.1007/s10571-006-9008-1
Hu, Z., Yuan, Y., Zhang, X., Lu, Y., Dong, N., Jiang, X., et al. (2021). Human umbilical cord mesenchymal stem cell-derived exosomes attenuate oxygen-glucose deprivation/reperfusion-induced microglial pyroptosis by promoting FOXO3a-dependent mitophagy. Oxid. Med. Cell. Longev. 2021, 6219715. doi: 10.1155/2021/6219715
Hu, Z. L., Sun, T., Lu, M., Ding, J. H., Du, R. H., and Hu, G. (2019). Kir6.1/K-ATP channel on astrocytes protects against dopaminergic neurodegeneration in the MPTP mouse model of Parkinson's disease via promoting mitophagy. Brain Behav. Immun. 81, 509–522. doi: 10.1016/j.bbi.2019.07.009
Huang, K.-S., He, D.-X., Tao, Q., Wang, Y.-Y., Yang, Y.-Q., Zhang, B., et al. (2022). Changes in the incidence and prevalence of ischemic stroke and associations with natural disasters: an ecological study in 193 countries. Sci. Rep. 12, 1808. doi: 10.1038/s41598-022-05288-7
Ismael, S., Zhao, L., Nasoohi, S., and Ishrat, T. (2018). Inhibition of the NLRP3-inflammasome as a potential approach for neuroprotection after stroke. Sci. Rep. 8, 5971. doi: 10.1038/s41598-018-24350-x
Iyer, S. S., He, Q., Janczy, J. R., Elliott, E. I., Zhong, Z. Y, Olivier, A. K., et al. (2013). Mitochondrial cardiolipin is required for Nlrp3 inflammasome activation. Immunity. 39, 311–323. doi: 10.1016/j.immuni.2013.08.001
Ji, N., Qi, Z., Wang, Y., Yang, X., Yan, Z., Li, M., et al. (2021). Pyroptosis: a new regulating mechanism in cardiovascular disease. J. Inflamm. Res. 14, 2647–2666. doi: 10.2147/JIR.S308177
Ji, R., Ma, L., Chen, X., Sun, R., Zhang, L., Saiyin, H., et al. (2021). Characterizing the distributions of IDO-1 expressing macrophages/microglia in human and murine brains and evaluating the immunological and physiological roles of IDO-1 in RAW264.7/BV-2 cells. PLoS ONE 16, e0258204. doi: 10.1371/journal.pone.0258204
Jia, Y., Tong, Y., Min, L., Li, Y., and Cheng, Y. (2021). Protective effects of oridonin against cerebral ischemia/reperfusion injury by inhibiting the NLRP3 inflammasome activation. Tissue Cell 71, 101514. doi: 10.1016/j.tice.2021.101514
Jiang, H., He, H., Chen, Y., Huang, W., Cheng, J., Ye, J., et al. (2017). Identification of a selective and direct NLRP3 inhibitor to treat inflammatory disorders. J. Exp. Med. 214, 3219–3238. doi: 10.1084/jem.20171419
Jiang, T., Wu, M., Zhang, Z., Yan, C., Ma, Z., He, S., et al. (2019). Electroacupuncture attenuated cerebral ischemic injury and neuroinflammation through α7nAChR-mediated inhibition of NLRP3 inflammasome in stroke rats. Mol. Med. 25, 22. doi: 10.1186/s10020-019-0091-4
Jin, R., Yang, G., and Li, G. (2010). Inflammatory mechanisms in ischemic stroke: role of inflammatory cells. J. Leukoc. Biol. 87, 779–789. doi: 10.1189/jlb.1109766
Joaquim, L. S., Danielski, L. G., Bonfante, S., Biehl, E., Mathias, K., Denicol, T., et al. (2021). NLRP3 inflammasome activation increases brain oxidative stress after transient global cerebral ischemia in rats. Int. J. Neurosci. 20, 1–14. doi: 10.1080/00207454.2021.1922402
Joly, S., Ma, N., Sadler, J. J., Soll, D. R., Cassel, S. L., and Sutterwala, F. S. (2009). Cutting edge: Candida albicans hyphae formation triggers activation of the Nlrp3 inflammasome. J. Immunol. 183, 3578–3581. doi: 10.4049/jimmunol.0901323
Kang, X., Jiang, L., Chen, X., Wang, X., Gu, S., Wang, J., et al. (2021). Exosomes derived from hypoxic bone marrow mesenchymal stem cells rescue OGD-induced injury in neural cells by suppressing NLRP3 inflammasome-mediated pyroptosis. Exp. Cell Res. 405, 112635. doi: 10.1016/j.yexcr.2021.112635
Karasawa, T., and Takahashi, M. (2017). Role of NLRP3 inflammasomes in atherosclerosis. J. Atheroscler. Thromb. 24, 443–451. doi: 10.5551/jat.RV17001
Kelley, N., Jeltema, D., Duan, Y., and He, Y. (2019). The NLRP3 inflammasome: an overview of mechanisms of activation and regulation. Int. J. Mol. Sci. 20, 3328. doi: 10.3390/ijms20133328
Kendall, R. L., and Holian, A. (2021). The role of lysosomal ion channels in lysosome dysfunction. Inhal. Toxicol. 33, 41–54. doi: 10.1080/08958378.2021.1876188
Lai, X., Cao, K., Kong, L., Liu, Q., and Gao, Y. (2017). Xingnaojing for moderate-to-severe acute ischemic stroke (XMAS): study protocol for a randomized controlled trial. Trials 18, 479. doi: 10.1186/s13063-017-2222-y
Lamkanfi, M., and Dixit, V. M. (2014). Mechanisms and functions of inflammasomes. Cell 157, 1013–1022. doi: 10.1016/j.cell.2014.04.007
Lee, H. E., Yang, G., Park, Y. B., Kang, H. C., Cho, Y. Y., Lee, H. S., et al. (2019). Epigallocatechin-3-gallate prevents acute gout by suppressing NLRP3 inflammasome activation and mitochondrial DNA synthesis. Molecules 24, 2138. doi: 10.3390/molecules24112138
Li, A. Y., Zhang, S. H., Li, J., Liu, K., Huang, F., and Liu, B. L. (2016). Metformin and resveratrol inhibit Drp1-mediated mitochondrial fission and prevent ER stress-associated NLRP3 inflammasome activation in the adipose tissue of diabetic mice. Mol. Cell. Endocrinol. 434, 36–47. doi: 10.1016/j.mce.2016.06.008
Li, F., Xu, D., Hou, K., Gou, X., Lv, N., Fang, W., et al. (2021c). Pretreatment of indobufen and aspirin and their combinations with clopidogrel or ticagrelor alleviates inflammasome mediated pyroptosis via inhibiting NF-κB/NLRP3 pathway in ischemic stroke. J Neuroimmune Pharmacol. 16, 835–853. doi: 10.1007/s11481-020-09978-9
Li, J., Wang, Y. L., Wang, Y. P., Wen, X. D., Ma, X. N., Chen, W. J., et al. (2015). Pharmacological activation of AMPK prevents Drp1-mediated mitochondrial fission and alleviates endoplasmic reticulum stress-associated endothelial dysfunction. J. Mol. Cell. Cardiol. 86, 62–74. doi: 10.1016/j.yjmcc.2015.07.010
Li, L., Cheng, S.-Q., Guo, W., Cai, Z.-Y., Sun, Y.-Q., Huang, X.-X., et al. (2021a). Oridonin prevents oxidative stress-induced endothelial injury via promoting Nrf-2 pathway in ischaemic stroke. J. Cell. Mol. Med. 25, 9753–9766. doi: 10.1111/jcmm.16923
Li, N., Liu, C., Wang, C., Chen, R., Li, X., Wang, Y., et al. (2021b). Early changes of NLRP3 inflammasome activation after hypoxic-ischemic brain injury in neonatal rats. Int. J. Clin. Exp. Pathol. 14, 209–220.
Li, S., Lin, Q. S., Shao, X. H., Mou, S., Gu, L. Y., Wang, L., et al. (2019). NLRP3 inflammasome inhibition attenuates cisplatin-induced renal fibrosis by decreasing oxidative stress and inflammation. Exp. Cell Res. 383, 111488. doi: 10.1016/j.yexcr.2019.07.001
Li, W., Chen, H., Xu, Z., Lv, Y., Zhao, Z., Zhang, J., et al. (2020a). Melodinhenine B attenuates NLRP3 expression in a cerebral ischemia/reperfusion-induced neuronal injury rat model. Folia Neuropathol. 58, 30–37. doi: 10.5114/fn.2020.94004
Li, Y., Wang, R., Xue, L., Yang, Y., and Zhi, F. (2020b). Astilbin protects against cerebral ischaemia/reperfusion injury by inhibiting cellular apoptosis and ROS-NLRP3 inflammasome axis activation. Int. Immunopharmacol. 84, 106571. doi: 10.1016/j.intimp.2020.106571
Liepinsh, E., Barbals, R., Dahl, E., Sharipo, A., Staub, E., and Otting, G. (2003). The death-domain fold of the ASC PYRIN domain, presenting a basis for PYRIN/PYRIN recognition. J. Mol. Biol. 332, 1155–1163. doi: 10.1016/j.jmb.2003.07.007
Liu, J., Zheng, J., Xu, Y., Cao, W., Wang, J., Wang, B., et al. (2021c). Enriched environment attenuates pyroptosis to improve functional recovery after cerebral ischemia/reperfusion injury. Front. Aging Neurosci. 13, 717644. doi: 10.3389/fnagi.2021.717644
Liu, L., Dong, Y., Ye, M., Jin, S., Yang, J. B., Joosse, M. E., et al. (2017). The pathogenic role of NLRP3 inflammasome activation in inflammatory bowel diseases of both mice and humans. J. Crohns. Colitis. 11, 737–750. doi: 10.1093/ecco-jcc/jjw219
Liu, Q. P., Zhang, F. J., Zhang, X., Cheng, R., Ma, J. X., Yi, J. L., et al. (2018). Fenofibrate ameliorates diabetic retinopathy by modulating Nrf2 signaling and NLRP3 inflammasome activation. Mol. Cell Biochem. 445, 105–115. doi: 10.1007/s11010-017-3256-x
Liu, Q. Y., Zhang, D. Y., Hu, D. Y., Zhou, X. M., and Zhou, Y. (2018). The role of mitochondria in NLRP3 inflammasome activation. Mol. Immunol. 103, 115–124. doi: 10.1016/j.molimm.2018.09.010
Liu, T., Wang, X., Guo, F., Sun, X., Yuan, K., Wang, Q., et al. (2021a). Lysophosphatidylcholine induces apoptosis and inflammatory damage in brain microvascular endothelial cells via GPR4-mediated NLRP3 inflammasome activation. Toxicol. In Vitro 77, 105227. doi: 10.1016/j.tiv.2021.105227
Liu, X., Zhang, M., Liu, H., Zhu, R., He, H., Zhou, Y., et al. (2021b). Bone marrow mesenchymal stem cell-derived exosomes attenuate cerebral ischemia-reperfusion injury-induced neuroinflammation and pyroptosis by modulating microglia M1/M2 phenotypes. Exp. Neurol. 341, 113700. doi: 10.1016/j.expneurol.2021.113700
Liu, Z. N., Zhao, H. Y., Liu, W., Li, T. G., Wang, Y., and Zhao, M. (2015). NLRP3 inflammasome activation is essential for paraquat-induced acute lung injury. Inflammation 38, 433–444. doi: 10.1007/s10753-014-0048-2
Lo, E. H., Moskowitz, M. A., and Jacobs, T. P. (2005). Exciting, radical, suicidal: how brain cells die after stroke. Stroke 36, 189–192. doi: 10.1161/01.STR.0000153069.96296.fd
Lu, D., Hu, M., Zhang, B., Lin, Y., Zhu, Q., Men, X., et al. (2021). Temporal and spatial dynamics of inflammasome activation after ischemic stroke. Front. Neurol. 12, 621555. doi: 10.3389/fneur.2021.621555
Luo, J., Chen, J., Yang, C., Tan, J., Zhao, J., Jiang, N., et al. (2021). 6-Gingerol protects against cerebral ischemia/reperfusion injury by inhibiting NLRP3 inflammasome and apoptosis via TRPV1/FAF1 complex dissociation-mediated autophagy. Int. Immunopharmacol. 100, 108146. doi: 10.1016/j.intimp.2021.108146
Ma, Z. Z., Sun, H. S., Lv, J. C., Guo, L., and Yang, Q. R. (2018). Expression and clinical significance of the NEK7-NLRP3 inflammasome signaling pathway in patients with systemic lupus erythematosus. J Inflamm. 15, 16. doi: 10.1186/s12950-018-0192-9
Mariathasan, S., Weiss, D. S., Newton, K., McBride, J., O'Rourke, K., Roose-Girma, M., et al. (2006). Cryopyrin activates the inflammasome in response to toxins and ATP. Nature 440, 228–232. doi: 10.1038/nature04515
Martinon, F., Burns, K., and Tschopp, J. (2002). The inflammasome: a molecular platform triggering activation of inflammatory caspases and processing of proIL-beta. Mol. Cell. 10, 417–426. doi: 10.1016/S1097-2765(02)00599-3
Matzinger, P. (2002). The danger model: a renewed sense of self. Science 296, 301–305. doi: 10.1126/science.1071059
McKenzie, B. A., Mamik, M. K., Saito, L. B., Boghozian, R., Monaco, M. C., Major, E. O., et al. (2018). Caspase-1 inhibition prevents glial inflammasome activation and pyroptosis in models of multiple sclerosis. Proc. Nat. Acad. Sci. U.S.A. 115, E6065. doi: 10.1073/pnas.1722041115
Menu, P., and Vince, J. E. (2011). The NLRP3 inflammasome in health and disease: the good, the bad and the ugly. Clin. Exp. Immunol. 166, 1–15. doi: 10.1111/j.1365-2249.2011.04440.x
Minutoli, L., Puzzolo, D., Rinaldi, M., Irrera, N., Marini, H., Arcoraci, V., et al. (2016). ROS-Mediated NLRP3 inflammasome activation in brain, heart, kidney, and testis ischemia/reperfusion injury. Oxid. Med. Cell. Longev. 2016, 2183026. doi: 10.1155/2016/2183026
Mo, Z.-T., Zheng, J., and Liao, Y.-L. (2021). Icariin inhibits the expression of IL-1β, IL-6 and TNF-α induced by OGD/R through the IRE1/XBP1s pathway in microglia. Pharm Biol. 59, 1473–1479. doi: 10.1080/13880209.2021.1991959
Mridha, A. R., Wree, A., Robertson, A. A. B., Yeh, M. M., Johnson, C. D., Van Rooyen, D. M., et al. (2017). NLRP3 inflammasome blockade reduces liver inflammation and fibrosis in experimental NASH in mice. J. Hepatol. 66, 1037–1046. doi: 10.1016/j.jhep.2017.01.022
Nomura, J., So, A., Tamura, M., and Busso, N. (2015). Intracellular ATP decrease mediates NLRP3 inflammasome activation upon nigericin and crystal stimulation. J. Immunol. 195, 5718–5724. doi: 10.4049/jimmunol.1402512
Pan, R., Xie, Y., Fang, W., Liu, Y., and Zhang, Y. (2022a). USP20 mitigates ischemic stroke in mice by suppressing neuroinflammation and neuron death via regulating PTEN signal. Int. Immunopharmacol. 103, 107840. doi: 10.1016/j.intimp.2021.107840
Pan, X., Fan, J., Peng, F., Xiao, L., and Yang, Z. (2022b). SET domain containing 7 promotes oxygen-glucose deprivation/reoxygenation-induced PC12 cell inflammation and oxidative stress by regulating Keap1/Nrf2/ARE and NF-κB pathways. Bioengineered 13, 7253–7261. doi: 10.1080/21655979.2022.2045830
Park, J. H., Kim, D. W., Shin, M. J., Park, J., Han, K. H., Lee, K. W., et al. (2020). Tat-indoleamine 2,3-dioxygenase 1 elicits neuroprotective effects on ischemic injury. BMB Rep. 53, 582–587. doi: 10.5483/BMBRep.2020.53.11.114
Park, S., Juliana, C., Hong, S., Datta, P., Hwang, I., Fernandes-Alnemri, T., et al. (2013). The mitochondrial antiviral protein MAVS associates with NLRP3 and regulates its inflammasome activity. J. Immunol. 191, 4358–4366. doi: 10.4049/jimmunol.1301170
Peng, J., Wang, H., Gong, Z., Li, X., He, L., Shen, Q., et al. (2020). Idebenone attenuates cerebral inflammatory injury in ischemia and reperfusion via dampening NLRP3 inflammasome activity. Mol. Immunol. 123, 74–87. doi: 10.1016/j.molimm.2020.04.013
Pétrilli, V., Papin, S., Dostert, C., Mayor, A., Martinon, F., and Tschopp, J. (2007). Activation of the NALP3 inflammasome is triggered by low intracellular potassium concentration. Cell Death Differ. 14, 1583–1589. doi: 10.1038/sj.cdd.4402195
Qiu, Z., Lei, S., Zhao, B., Wu, Y., Su, W., Liu, M., et al. (2017). NLRP3 inflammasome activation-mediated pyroptosis aggravates myocardial ischemia/reperfusion injury in diabetic rats. Oxid. Med. Cell. Longev. 2017, 9743280. doi: 10.1155/2017/9743280
Qu, X.-Y., Zhang, Y.-M., Tao, L.-N., Gao, H., Zhai, J.-H., Sun, J.-M., et al. (2019). XingNaoJing injections protect against cerebral ischemia/reperfusion injury and alleviate blood-brain barrier disruption in rats, through an underlying mechanism of NLRP3 inflammasomes suppression. Chin. J. Nat. Med. 17, 498–505. doi: 10.1016/S1875-5364(Menu and Vince, 2011)30071-8
Ramos-Cabrer, P., Campos, F., Sobrino, T., and Castillo, J. (2011). Targeting the ischemic penumbra. Stroke. 42 (1 Suppl), S7–11. doi: 10.1161/STROKEAHA.110.596684
Ren, J.-X., Li, C., Yan, X.-L., Qu, Y., Yang, Y., and Guo, Z.-N. (2021). Crosstalk between oxidative stress and ferroptosis/oxytosis in ischemic stroke: possible targets and molecular mechanisms. Oxid. Med. Cell. Longev. 2021, 6643382. doi: 10.1155/2021/6643382
Rupalla, K., Allegrini, P. R., Sauer, D., and Wiessner, C. (1998). Time course of microglia activation and apoptosis in various brain regions after permanent focal cerebral ischemia in mice. Acta Neuropathol. 96, 172–178. doi: 10.1007/s004010050878
Sapkota, A., and Choi, J. W. (2021). Oleanolic acid provides neuroprotection against ischemic stroke through the inhibition of microglial activation and NLRP3 inflammasome activation. Biomol. Ther. 30, 55–63. doi: 10.4062/biomolther.2021.154
Schilling, J. D. (2016). Dousing fire with gasoline: interplay between lysosome damage and the NLRP3 inflammasome. Focus on “NLRP3 inflammasome signaling is activated by low-level lysosome disruption but inhibited by extensive lysosome disruption: roles for K+ efflux and Ca2+ influx”. Am. J. Physiol. Cell Physiol. 311, C81–C2. doi: 10.1152/ajpcell.00174.2016
Shao, B. Z., Xu, Z. Q., Han, B. Z., Su, D. F., and Liu, C. (2015). NLRP3 inflammasome and its inhibitors: a review. Front. Pharmacol. 6, 262. doi: 10.3389/fphar.2015.00262
She, Y., Shao, L., Zhang, Y., Hao, Y., Cai, Y., Cheng, Z., et al. (2019). Neuroprotective effect of glycosides in Buyang Huanwu Decoction on pyroptosis following cerebral ischemia-reperfusion injury in rats. J. Ethnopharmacol. 242, 112051. doi: 10.1016/j.jep.2019.112051
Shi, J., Zhao, Y., Wang, K., Shi, X., Wang, Y., Huang, H., et al. (2015). Cleavage of GSDMD by inflammatory caspases determines pyroptotic cell death. Nature 526, 660–665. doi: 10.1038/nature15514
Shi, M., Chen, J., Liu, T., Dai, W., Zhou, Z., Chen, L., et al. (2022). Protective effects of remimazolam on cerebral ischemia/reperfusion injury in rats by inhibiting of NLRP3 inflammasome-dependent pyroptosis. Drug Des. Devel. Ther. 16, 413–423. doi: 10.2147/DDDT.S344240
Shimada, K., Crother, T. R., Karlin, J., Dagvadorj, J., Chiba, N., Chen, S., et al. (2012). Oxidized mitochondrial DNA activates the NLRP3 inflammasome during apoptosis. Immunity 36, 401–414. doi: 10.1016/j.immuni.2012.01.009
Sho, T., and Xu, J. X. (2019). Role and mechanism of ROS scavengers in alleviating NLRP3-mediated inflammation. Biotechnol. Appl. Biochem. 66, 4–13. doi: 10.1002/bab.1700
Sommer, C. J. (2017). Ischemic stroke: experimental models and reality. Acta Neuropathol. 133, 245–261. doi: 10.1007/s00401-017-1667-0
Srinivasula, S. M., Poyet, J.-L., Razmara, M., Datta, P., Zhang, Z., and Alnemri, E. S. (2002). The PYRIN-CARD protein ASC is an activating adaptor for caspase-1. J. Biol. Chem. 277, 21119–21122. doi: 10.1074/jbc.C200179200
Subramanian, N., Natarajan, K., Clatworthy, M. R., Wang, Z., and Germain, R. N. (2013). The adaptor MAVS promotes NLRP3 mitochondrial localization and inflammasome activation. Cell. 153, 348–361. doi: 10.1016/j.cell.2013.02.054
Sun, R., Peng, M., Xu, P., Huang, F., Xie, Y., Li, J., et al. (2020). Low-density lipoprotein receptor (LDLR) regulates NLRP3-mediated neuronal pyroptosis following cerebral ischemia/reperfusion injury. J. Neuroinflammation. 17, 330. doi: 10.1186/s12974-020-01988-x
Swanson, K. V., Deng, M., and Ting, J. P. Y. (2019). The NLRP3 inflammasome: molecular activation and regulation to therapeutics. Nat. Rev. Immunol. 19, 477–489. doi: 10.1038/s41577-019-0165-0
Tang, T. T., Lv, L. L., Pan, M. M., Wen, Y., Wang, B., Li, Z. L., et al. (2018). Hydroxychloroquine attenuates renal ischemia/reperfusion injury by inhibiting cathepsin mediated NLRP3 inflammasome activation. Cell Death Dis. 9, 351. doi: 10.1038/s41419-018-0378-3
Tapia-Abellán, A., Angosto-Bazarra, D., Martínez-Banaclocha, H., de Torre-Minguela, C., Cerón-Carrasco, J. P., Pérez-Sánchez, H., et al. (2019). MCC950 closes the active conformation of NLRP3 to an inactive state. Nat. Chem. Biol. 15, 560–564. doi: 10.1038/s41589-019-0278-6
Tschopp, J., and Schroder, K. (2010). NLRP3 inflammasome activation: the convergence of multiple signalling pathways on ROS production? Nat. Rev. Immunol. 10, 210–215. doi: 10.1038/nri2725
Tuo, Q.-Z., Zhang, S.-T., and Lei, P. (2022). Mechanisms of neuronal cell death in ischemic stroke and their therapeutic implications. Med. Res. Rev. 42, 259–305. doi: 10.1002/med.21817
Vande Walle, L., and Lamkanfi, M. (2016). Pyroptosis. Curr. Biol. 26, R568–R72. doi: 10.1016/j.cub.2016.02.019
Varin, A., and Gordon, S. (2009). Alternative activation of macrophages: immune function and cellular biology. Immunobiology 214, 630–641. doi: 10.1016/j.imbio.2008.11.009
Voet, S., Srinivasan, S., Lamkanfi, M., and van Loo, G. (2019). Inflammasomes in neuroinflammatory and neurodegenerative diseases. EMBO Mol. Med. 11, e10248. doi: 10.15252/emmm.201810248
Wan, Z. F., Fan, Y., Liu, X. J., Xue, J. H., Han, Z. H., Zhu, C. Z., et al. (2019). NLRP3 inflammasome promotes diabetes-induced endothelial inflammation and atherosclerosis. Diabetes Metab. Syndr. Obes. 12, 1931–1942. doi: 10.2147/DMSO.S222053
Wang, B., Lyu, Z., Chan, Y., Li, Q., Zhang, L., Liu, K., et al. (2021b). Tongxinluo Exerts inhibitory effects on pyroptosis and amyloid-β peptide accumulation after cerebral ischemia/reperfusion in rats. Evid. Based Complement Alternat. Med. 2021, 5788602. doi: 10.1155/2021/5788602
Wang, H.-Y., Zhou, H.-F., He, Y., Yu, L., Li, C., Yang, J.-H., et al. (2021a). Protective effect of naoxintong capsule () combined with guhong injection () on rat brain microvascular endothelial cells during cerebral ischemia-reperfusion injury. Chin. J. Integr. Med. 27, 744–751. doi: 10.1007/s11655-020-3215-3
Wang, J., Guo, M., Ma, R., Wu, M., and Zhang, Y. (2020b). Tetrandrine alleviates cerebral ischemia/reperfusion injury by suppressing NLRP3 inflammasome activation via Sirt-1. PeerJ. 8, e9042. doi: 10.7717/peerj.9042
Wang, K., Sun, Z., Ru, J., Wang, S., Huang, L., Ruan, L., et al. (2020a). Ablation of gsdmd improves outcome of ischemic stroke through blocking canonical and non-canonical inflammasomes dependent pyroptosis in microglia. Front. Neurol. 11, 577927. doi: 10.3389/fneur.2020.577927
Wang, N., Nie, H., Zhang, Y., Han, H., Wang, S., Liu, W., et al. (2021c). Dexmedetomidine exerts cerebral protective effects against cerebral ischemic injury by promoting the polarization of M2 microglia via the Nrf2/HO-1/NLRP3 pathway. Inflammat. Res. 71, 93–106. doi: 10.1007/s00011-021-01515-5
Wang, Q., Wang, F., Li, X., Yang, Q., Li, X., Xu, N., et al. (2012). Electroacupuncture pretreatment attenuates cerebral ischemic injury through α7 nicotinic acetylcholine receptor-mediated inhibition of high-mobility group box 1 release in rats. J. Neuroinflammation 9, 24. doi: 10.1186/1742-2094-9-24
Wang, Q.-S., Luo, X.-Y., Fu, H., Luo, Q., Wang, M.-Q., and Zou, D.-Y. (2020). MiR-139 protects against oxygen-glucose deprivation/reoxygenation (OGD/R)-induced nerve injury through targeting c-jun to inhibit NLRP3 inflammasome activation. J. Stroke Cerebrovasc. Dis. 29, 105037. doi: 10.1016/j.jstrokecerebrovasdis.2020.105037
Wang, Q. Y., Ou, Y. J., Hu, G. M., Wen, C., Yue, S. S., Chen, C., et al. (2020). Naringenin attenuates non-alcoholic fatty liver disease by down-regulating the NLRP3/NF-κB pathway in mice. Br. J. Pharmacol. 177, 1806–1821. doi: 10.1111/bph.14938
Wang, R., Li, L., and Wang, B. (2022). Poncirin ameliorates oxygen glucose deprivation/reperfusion injury in cortical neurons via inhibiting NOX4-mediated NLRP3 inflammasome activation. Int. Immunopharmacol. 102, 107210. doi: 10.1016/j.intimp.2020.107210
Wang, S. H., Yao, Q. H., Wan, Y., Wang, J. Q., Huang, C. G., Li, D., et al. (2020). Adiponectin reduces brain injury after intracerebral hemorrhage by reducing NLRP3 inflammasome expression. Int. J. Neurosci. 130, 301–308. doi: 10.1080/00207454.2019.1679810
Wang, W., Jiang, B., Sun, H., Ru, X., Sun, D., Wang, L., et al. (2017). Prevalence, incidence, and mortality of stroke in china: results from a nationwide population-based survey of 480 687 adults. Circulation 135, 759–771. doi: 10.1161/CIRCULATIONAHA.116.025250
Wang, X. Q., Jiang, W., Yan, Y. Q., Gong, T., Han, J. H., Tian, Z. G., et al. (2014). RNA viruses promote activation of the NLRP3 inflammasome through a RIP1-RIP3-DRP1 signaling pathway. Nat. Immunol. 15, 1126–1133. doi: 10.1038/ni.3015
Wang, Y., Guan, X., Gao, C.-L., Ruan, W., Zhao, S., Kai, G., et al. (2021d). Medioresinol as a novel PGC-1α activator prevents pyroptosis of endothelial cells in ischemic stroke through PPARα-GOT1 axis. Pharmacol Res. 169, 105640. doi: 10.1016/j.phrs.2021.105640
Wang, Y., Huang, H., He, W., Zhang, S., Liu, M., and Wu, S. (2021e). Association between serum NLRP3 and malignant brain edema in patients with acute ischemic stroke. BMC Neurol. 21, 341. doi: 10.1186/s12883-021-02369-4
Wang, Z. L., Xu, G., Gao, Y., Zhan, X. Y., Qin, N., Fu, S. B., et al. (2019). Cardamonin from a medicinal herb protects against LPS-induced septic shock by suppressing NLRP3 inflammasome. Acta Pharm. Sin. B. 9, 734–744. doi: 10.1016/j.apsb.2019.02.003
Warpsinski, G., Smith, M. J., Srivastava, S., Keeley, T. P., Siow, R. C. M., Fraser, P. A., et al. (2020). Nrf2-regulated redox signaling in brain endothelial cells adapted to physiological oxygen levels: Consequences for sulforaphane mediated protection against hypoxia-reoxygenation. Redox Biol. 37, 101708. doi: 10.1016/j.redox.2020.101708
Wree, A., Eguchi, A., McGeough, M. D., Pena, C. A., Johnson, C. D., Canbay, A., et al. (2014). NLRP3 inflammasome activation results in hepatocyte pyroptosis, liver inflammation, and fibrosis in mice. Hepatology 59, 898–910. doi: 10.1002/hep.26592
Wu, D., Chen, Y., Sun, Y., Gao, Q., Li, H., Yang, Z., et al. (2020). Target of MCC950 in inhibition of NLRP3 inflammasome activation: a literature review. Inflammation 43, 17–23. doi: 10.1007/s10753-019-01098-8
Xiong, X., Xu, L., Wei, L., White, R. E., Ouyang, Y.-B., and Giffard, R. G. (2015). IL-4 is required for sex differences in vulnerability to focal ischemia in mice. Stroke 46, 2271–2276. doi: 10.1161/STROKEAHA.115.008897
Xu, J., Wang, A., Meng, X., Yalkun, G., Xu, A., Gao, Z., et al. (2021a). Edaravone dexborneol versus edaravone alone for the treatment of acute ischemic stroke: a phase III, randomized, double-blind, comparative trial. Stroke 52, 772–780. doi: 10.1161/STROKEAHA.120.031197
Xu, L. L., Shen, L. Y., Yu, X. L., Li, P., Wang, Q., and Li, C. Q. (2020). Effects of irisin on osteoblast apoptosis and osteoporosis in postmenopausal osteoporosis rats through upregulating Nrf2 and inhibiting NLRP3 inflammasome. Exp. Ther. Med. 19, 1084–1090. doi: 10.3892/etm.2019.8313
Xu, Y., Gao, X., Wang, L., Yang, M., and Xie, R. (2021b). Bakuchiol ameliorates cerebral ischemia-reperfusion injury by modulating NLRP3 inflammasome and Nrf2 signaling. Respir. Physiol. Neurobiol. 292, 103707. doi: 10.1016/j.resp.2021.103707
Xue, M., Li, T., Wang, Y., Chang, Y. P., Cheng, Y., Lu, Y. H., et al. (2019). Empagliflozin prevents cardiomyopathy via sGC-cGMP-PKG pathway in type 2 diabetes mice. Clin. Sci. 133, 1705–1720. doi: 10.1042/CS20190585
Yang, Z., Zhao, T.-Z., Zou, Y.-J., Zhang, J. H., and Feng, H. (2014). Hypoxia Induces autophagic cell death through hypoxia-inducible factor 1α in microglia. PLoS ONE 9, e96509. doi: 10.1371/journal.pone.0096509
Yao, S., Li, L. J., Sun, X., Hua, J., Zhang, K. Q., Hao, L., et al. (2019). FTY720 inhibits MPP-Induced microglial activation by affecting NLRP3 Inflammasome activation. J. Neuroimmune Pharmacol. 14, 478–492. doi: 10.1007/s11481-019-09843-4
Ye, Y., Jin, T., Zhang, X., Zeng, Z., Ye, B., Wang, J., et al. (2019). Meisoindigo protects against focal cerebral ischemia-reperfusion injury by inhibiting NLRP3 inflammasome activation and regulating microglia/macrophage polarization via TLR4/NF-κB signaling pathway. Front. Cell. Neurosci. 13, 553. doi: 10.3389/fncel.2019.00553
Yin, M., Chen, W.-P., Yin, X.-P., Tu, J.-L., Hu, N., and Li, Z.-Y. (2021). LncRNA TUG1 demethylated by TET2 promotes NLRP3 expression, contributes to cerebral ischemia/reperfusion inflammatory injury. ASN Neuro. 13, 17590914211003247. doi: 10.1177/17590914211003247
Yu, C., He, Q., Zheng, J., Li, L. Y., Hou, Y. H., and Song, F. Z. (2017). Sulforaphane improves outcomes and slows cerebral ischemic/reperfusion injury via inhibition of NLRP3 inflammasome activation in rats. Int. Immunopharmacol. 45, 74–78. doi: 10.1016/j.intimp.2017.01.034
Yu, J.j, Nagasu, H., Murakami, T., Hoang, H., Broderick, L., Hoffman, H. M., et al. (2014). Inflammasome activation leads to Caspase-1-dependent mitochondrial damage and block of mitophagy. Proc. Natl. Acad. Sci. U.S.A. 111, 15514–15519. doi: 10.1073/pnas.1414859111
Yu, J. W., and Lee, M. S. (2016). Mitochondria and the NLRP3 inflammasome: physiological and pathological relevance. Arch. Pharm. Res. 39, 1503–1518. doi: 10.1007/s12272-016-0827-4
Zeng, Q., Zhou, Y., Liang, D., He, H., Liu, X., Zhu, R., et al. (2020). Exosomes secreted from bone marrow mesenchymal stem cells attenuate oxygen-glucose deprivation/reoxygenation-induced pyroptosis in PC12 cells by promoting AMPK-dependent autophagic flux. Front. Cell. Neurosci. 14, 182. doi: 10.3389/fncel.2020.00182
Zhang, D., Qian, J., Zhang, P., Li, H., Shen, H., Li, X., et al. (2019). Gasdermin D serves as a key executioner of pyroptosis in experimental cerebral ischemia and reperfusion model both in vivo and in vitro. J. Neurosci. Res. 97, 645–660. doi: 10.1002/jnr.24385
Zhang, H.-S., Ouyang, B., Ji, X.-Y., and Liu, M.-F. (2021). Gastrodin alleviates cerebral ischaemia/reperfusion injury by inhibiting pyroptosis by regulating the lncRNA NEAT1/miR-22-3p axis. Neurochem. Res. 46, 1747–1758. doi: 10.1007/s11064-021-03285-2
Zhang, L. P., Li, H. Z., Zang, Y. W., and Wang, F. (2019). NLRP3 inflammasome inactivation driven by miR-223-3p reduces tumor growth and increases anticancer immunity in breast cancer. Mol. Med. Rep. 19, 2180–2188. doi: 10.3892/mmr.2019.9889
Zhang, W.-Y., Guo, Y.-J., Han, W.-X., Yang, M.-Q., Wen, L.-P., Wang, K.-Y., et al. (2019). Curcumin relieves depressive-like behaviors via inhibition of the NLRP3 inflammasome and kynurenine pathway in rats suffering from chronic unpredictable mild stress. Int. Immunopharmacol. 67, 138–144. doi: 10.1016/j.intimp.2018.12.012
Zhang, Y., Wu, Z., Huang, Z., Liu, Y., Chen, X., Zhao, X., et al. (2022). GSK-3β inhibition elicits a neuroprotection by restoring lysosomal dysfunction in neurons via facilitation of TFEB nuclear translocation after ischemic stroke. Brain Res. 1778, 147768. doi: 10.1016/j.brainres.2021.147768
Zhao, B., Fei, Y., Zhu, J., Yin, Q., Fang, W., and Li, Y. (2021). PAF receptor inhibition attenuates neuronal pyroptosis in cerebral ischemia/reperfusion injury. Mol. Neurobiol. 58, 6520–6539. doi: 10.1007/s12035-021-02537-0
Zhao, J., Piao, X., Wu, Y., Liang, S., Han, F., Liang, Q., et al. (2020). Cepharanthine attenuates cerebral ischemia/reperfusion injury by reducing NLRP3 inflammasome-induced inflammation and oxidative stress via inhibiting 12/15-LOX signaling. Biomed. Pharmacother. 127, 110151. doi: 10.1016/j.biopha.2020.110151
Zheng, F., Xing, S., Gong, Z., and Xing, Q. (2013). NLRP3 inflammasomes show high expression in aorta of patients with atherosclerosis. Heart Lung Circ. 22, 746–750. doi: 10.1016/j.hlc.2013.01.012
Zheng, M., Williams, E. P., Malireddi, R. K. S., Karki, R., Banoth, B., Burton, A., et al. (2020). Impaired NLRP3 inflammasome activation/pyroptosis leads to robust inflammatory cell death via caspase-8/RIPK3 during coronavirus infection. J. Biol. Chem. 295, 14040–14052. doi: 10.1074/jbc.RA120.015036
Zhong, X. Z., Yang, Y.m, Sun, X., and Dong, X. P. (2017). Methods for monitoring Ca2+ and ion channels in the lysosome. Cell Calcium. 64, 20–28. doi: 10.1016/j.ceca.2016.12.001
Zhong, Z., Liang, S., Sanchez-Lopez, E., He, F., Shalapour, S., Lin, X.-J., et al. (2018). New mitochondrial DNA synthesis enables NLRP3 inflammasome activation. Nature 560, 198–203. doi: 10.1038/s41586-018-0372-z
Zhong, Z. Y., Umemura, A., Sanchez-Lopez, E., Liang, S., Shalapour, S., Wong, J., et al. (2016). NF-κB restricts inflammasome activation via elimination of damaged mitochondria. Cell 164, 896–910. doi: 10.1016/j.cell.2015.12.057
Keywords: NLRP3 inflammasome activation, ischemic stroke, pyroptosis, cerebral I/R injury, mitochondrion
Citation: Wang L, Ren W, Wu Q, Liu T, Wei Y, Ding J, Zhou C, Xu H and Yang S (2022) NLRP3 Inflammasome Activation: A Therapeutic Target for Cerebral Ischemia–Reperfusion Injury. Front. Mol. Neurosci. 15:847440. doi: 10.3389/fnmol.2022.847440
Received: 02 January 2022; Accepted: 06 April 2022;
Published: 06 May 2022.
Edited by:
Jingqi Yan, Cleveland State University, United StatesReviewed by:
Markus Spurlock, University of Miami, United StatesXiuting Liu, Washington University in St. Louis, United States
Shi Yu, Institut Pasteur of Shanghai (CAS), China
Copyright © 2022 Wang, Ren, Wu, Liu, Wei, Ding, Zhou, Xu and Yang. This is an open-access article distributed under the terms of the Creative Commons Attribution License (CC BY). The use, distribution or reproduction in other forums is permitted, provided the original author(s) and the copyright owner(s) are credited and that the original publication in this journal is cited, in accordance with accepted academic practice. No use, distribution or reproduction is permitted which does not comply with these terms.
*Correspondence: Sijin Yang, ysjimn@sina.com; Houping Xu, xuhoupingphd@163.com
†These authors have contributed equally to this work and share first authorship