- 1Department of Otolaryngology-Head and Neck Surgery, Yijishan Hospital of Wannan Medical College, Wuhu, China
- 2State Key Laboratory of Medical Neurobiology and MOE Frontiers Center for Brain Science, ENT Institute and Department of Otorhinolaryngology, Eye and ENT Hospital, Fudan University, Shanghai, China
- 3NHC Key Laboratory of Hearing Medicine, Fudan University, Shanghai, China
- 4Nantong Laboratory of Development and Diseases, School of Life Sciences, Co-innovation Center of Neuroregeneration, Key Laboratory of Neuroregeneration of Jiangsu and MOE, Nantong University, Nantong, China
- 5Department of Forensic Medicine, Soochow University, Suzhou, China
Cingulin, a cytoplasmic element of tight junctions (TJs), is involved in maintenance of the integrity of epithelial and endothelial cells. However, the role of cingulin in the development of auditory organs remains unclear. Zebrafish is popular as a model organism for hearing research. Using the whole mount in situ hybridization (WISH) experiment, we detected the expression of cingulin b in the posterior lateral line system (PLLs) of zebrafish. We traced the early development progress of zebrafish PLLs from 36 hpf to 72 hpf, and found that inhibition of cingulin b by target morpholinos resulted in severe developmental obstruction, including decreased number of neuromasts, reduced proliferative cells in the primordium, and repressed hair cell differentiation in the neuromasts. To examine the potential mechanism of cingulin b in the development of zebrafish PLL neuromasts, we performed RNA-seq analysis to compare the differently expressed genes (DEGs) between cingulin b knockdown samples and the controls. The KEGG enrichment analysis revealed that MAPK signaling pathway and cellular senescence were the key pathways with most DEGs in cingulin b-MO morphants compared to the Control-MO embryos. Furthermore, quantitative RT-PCR analysis confirmed the findings by RNA-seq that the transcript levels of cell cycle negative regulators such as tp53 and cdkn1a, were remarkably upregulated after inhibition of cingulin b. Our results therefore indicated an important role of cingulin b in the development of auditory organs, and MAPK signaling pathway was inhibited while cellular senescence pathway was activated after downregulation of cingulin b. We bring forward new insights of cingulin by exploring its function in auditory system.
Introduction
Tight junctions (TJs), mainly composed of claudins, occludin, ZO proteins, cingulin and paracingulin, are widely localized at the apicolateral borders of cells, and play important roles in maintaining the integrity, permeability and polarity of cells (González-Mariscal et al., 2014; Citi, 2019). Cingulin is localized in the cytoplasmic region of TJs, comprised of a head, a rod and a tail domain (Cordenonsi et al., 1999). Cingulin connects to actin and microtubule cytoskeleton in the head domain, and interacts with Rho family GTPases in the coiled-coil rod region (Cordenonsi et al., 1999; D’atri et al., 2002; Ohnishi et al., 2004; Van Itallie et al., 2009; Yano et al., 2013). Cingulin is mainly involved in regulating the paracellular and blood-brain barrier, for example, edema is more severe in the specific cingulin knock-out mouse model compared to the controls (Hawkins and Davis, 2005; Zhuravleva et al., 2020). In addition, cingulin is found expressed in the organ of Corti, and its distribution is rearranged after high-intensity noise exposure (Raphael and Altschuler, 1991). In a kanamycin damaged guinea pig model, cingulin together with adherens junctions such as E-cadherin and beta-catenin are found reorganized in two distinct planes, and they would preserve the integrity of tissues during scar formation and hair cell degeneration, indicating a barrier function of cingulin in the organ of Corti (Leonova and Raphael, 1997). Cingulin is also expressed in key regions of mouse cochlea, such as spiral ligament, stria vascularis, spiral limbus, and tectorial membrane (Batissoco et al., 2018). However, the role of cingulin in the development of auditory system is unknown.
Zebrafish have a high genetic similarity with the genome of human, and many critical genes required for the development of eyes, ear, brain, heart and other organs are highly conserved between zebrafish and humans, which makes zebrafish an excellent model for studying the human disease (Dooley and Zon, 2000; Howe et al., 2013; Kalueff et al., 2014). Besides, the characteristics of short reproductive cycle, strong reproductive ability, and transparent embryos increase the popularity of zebrafish as an animal model compared to mice (Mandrekar and Thakur, 2009; He et al., 2017). The mature neuromast of zebrafish PLL is consisted of the central hair cells (HCs) and the surrounding supporting cells (SCs), which share many structural and functional similarities with the inner ear cochlea of mammals (Nicolson, 2005), making zebrafish lateral line system a significant model for studying hair cell development, survival and regeneration (Driever et al., 1994; Pyati et al., 2007; Brignull et al., 2009).
In this study, we chose zebrafish as the animal model to explore the potential role of cingulin in the development of lateral line system of zebrafish. In zebrafish, cingulin b is orthologous to human cingulin. We firstly designed anti-sense morpholinos to downregulate the expression of cingulin b, and the efficacy of cingulin b-MO was confirmed by ISH staining and qPCR analysis of cingulin b. We observed reduced number of neuromasts, decreased cell proliferation, and repressed HC differentiation in the PLL system of zebrafish after knocking down cingulin b compared to the control group. The RNA-seq analysis revealed that MAPK signaling pathway and cellular senescence genes were involved in the development of zebrafish PLL after inhibition of cingulin b. Our findings uncover a potential role of cingulin in the development of zebrafish mechanosensory organs.
Materials and Methods
Animal Operations
All zebrafish, including the wild type AB line and the transgenic Tg (cldnb: lynGFP) and Tg (brn3c: mGFP)s356t lines were bred in 28.5°C constant temperature incubator in embryo medium according to the standard formula (Kimmel et al., 1995). The stage of embryonic development was marked as hours- or days- after fertilization (hpf or dpf) (Kimmel et al., 1995). In order to avoid pigmentation, the embryos should be further immersed in 1-phenyl-2-thiourea (PTU) (Sigma-Aldrich) in the culture medium from 10 hpf (Tang et al., 2019). The operations on zebrafish were discussed and permitted by the Animal Conservation and Utilization Committee of Fudan University in Shanghai.
Morpholino Injection and mRNA Rescue Test
Cingulin b-MO, sequenced in 5’-TCCTGTCCGCAGAGAGGG AACTCAT-3’, was injected at a dose of 2 ng or 3 ng at one or two cell stage of embryos to reduce the expression of cingulin b. The other siblings were considered as controls by injection with a sequence of 5’-CCTCTTACCTCAGT TACAATTTATA-3’, namely control-MO (Control-MO). For the messenger RNA (mRNA) rescue experiment, a mixture of cingulin b-MO and cingulin b mRNA (Forward primer: 5’-AT GAGTTCCCTCTCTGCGGA-3’; Reverse primer: 5’-TCAACAG CTGGTGGTCTGAA-3’) was injected at the same stage with other groups.
Whole Mount in situ Hybridization in Zebrafish
WISH experiment was operated as previously disclosed (He et al., 2014; Thisse and Thisse, 2014). To examine the expression pattern of cingulin b in zebrafish, we collected embryos at various stages including 3.7, 14, and 48 hpf. To verify the efficacy of cingulin b-MO in the lateral line system of zebrafish, we collected embryos at 48 hpf. After a series of gradient solutions for dehydration, the collected embryos were stored in pure methanol (100% concentration) at −20°C. Before hybridization, the embryos would be gradient rehydrated first, and then digested with 20 μg/ml protease K. The probe was added and hybridized at 65°C constant temperature overnight. After thorough washes with the SSC-series at 65°C, the embryos were blocked in 2x BBR at room temperature for at least 1 h. Anti- digoxigenin (Dig)-AP Fab fragment (Roche) was added and incubated with specimens overnight at 4°C. Primers for synthesizing the objective genes were listed in Supplementary Table 1. Color reaction was implemented with BM purple AP substrate (Roche) in the dark at 37°C, and stopped with NTMT. The embryos after three times rinses were then re-fixed in 4% PFA and treated with different gradients of glycerol/PBS. The final specimens were stored in 100% glycerol and photographed by fluorescence stereoscopic microscope. All images were prepared by Photoshop and Illustrator software (2018, Adobe).
BrdU Labeled Cell Proliferation Analysis and Immunohistochemical Staining
Bromodeoxyuridine (BrdU) co-incubation was conducted to label the proliferative cells. The dechorionated embryos at 34 hpf were incubated in 10 mM BrdU (Sigma-Aldrich) for 2 hours to show the cell proliferation in the PLL primordium, while the dechorionated larvae at 2 dpf were incubated in 10 mM BrdU (Sigma-Aldrich) for 24 hours to examine the proliferative cells in PLL neuromasts of zebrafish. The corresponding embryos or larvae were collected, anesthetized in 0.02%MS-222 (Sigma-Aldrich), and fixed in 4% PFA at 4°C overnight. After washing with PBT-2 for 3 times, the collected embryos were soaked in 2 N HCl at 37°C for 30 min. After incubation with the primary anti-BrdU monoclonal antibody (1:200 dilution; Santa Cruz Biotechnology) for 1 h at 37°C following 4°C overnight, the samples were washed for several times and then incubated with the secondary Cy3 polyclonal antibody (1:300 dilution; Jackson) for 1 h at 37°C. DAPI (1:800 dilution; Invitrogen) was added and incubated with the embryos or larvae for 20 min at room temperature to label the nuclei. The fluorescence-labeled embryos were imaged by Leica confocal fluorescence microscope (TCS SP8; Leica). The images obtained were further rotated, cut, and adjusted in the brightness by Photoshop (2018, Adobe) and then the images were aligned and added with fonts or labels by Illustrator software (2018, Adobe).
RNA-Sequencing Analysis
Before specimen collection, the zebrafish embryos at 48 hpf accepted depletion of chorion and the yolk sac. The total RNA was extracted with TRIzol reagent (Thermo Fisher Science) and reversely transcribed into cDNA using the first strand of transcriptional cDNA synthesis kit (Roche). An Illumina HiSeq X Ten platform was used for library sequencing. Raw reads were firstly filtered out the data in low-quality, and the remaining high quality raw data were used for downstream analyses. We used the Spliced Transcripts Alignment to a Reference (STAR) software as the reference genome library. Differential expression analysis was conducted with the DESeq (2012) R package, and p-value <0.05 indicated significant difference. R package was performed for KEGG pathway enrichment analysis of DEGs on the basis of hypergeometric distribution. KEGG pathway database were the reference for further functional and pathway enrichment analysis.
Quantitative Real-Time PCR
In order to fully quantity the mRNA level of target genes, a quantitative real-time PCR (qRT-PCR) system (LightCycler®480) was operated on 48 hpf larvae in the Control-MO group and the cingulin b-MO group, using the PrimeScript RT reagent Kit (RR047A, Takara Biomedical Technology) and the SYBR PreMix Ex Taq Kit (RR820A, Takara Biomedical Technology). ΔΔCt method was chosen for results analysis. The primer sequences used in the study were described in Supplementary Table 2. Each qPCR assay was repeated in triplicate, and GAPDH was used as the internal reference genes.
Statistical Analysis
All statistics were performed with GraphPad Prism software (version, 8.0c). Comparison between two groups was conducted with double-tailed Student t test, while comparisons among multiple groups were carried out by One-way ANOVA. Statistics were recorded as mean ± SEM (standard error of mean), and the difference was considered to be of significant difference with p-value less than 0.05.
Results
Expression of Cingulin b in Zebrafish
In order to detect whether cingulin b is expressed in zebrafish, we collected embryos at various stages and conducted WISH analysis for cingulin b staining. As shown in Figure 1, cingulin b was detected expressed in the oblong stage at 3.7 hpf (Figures 1A,B), the 10-somite stage at 14 hpf (Figure 1C), and the deposited PLL neuromasts at 48 hpf (Figure 1D), mainly in the central HC area (Figure 1E). To confirm the expression of cingulin b in the early development of zebrafish, we also conducted the sense control probe for cingulin b at 48 hpf, however, we didn’t detect any expression of cingulin b in the lateral line system of zebrafish compared to that using antisense mRNA probe for cingulin b (Supplementary Figure 1).
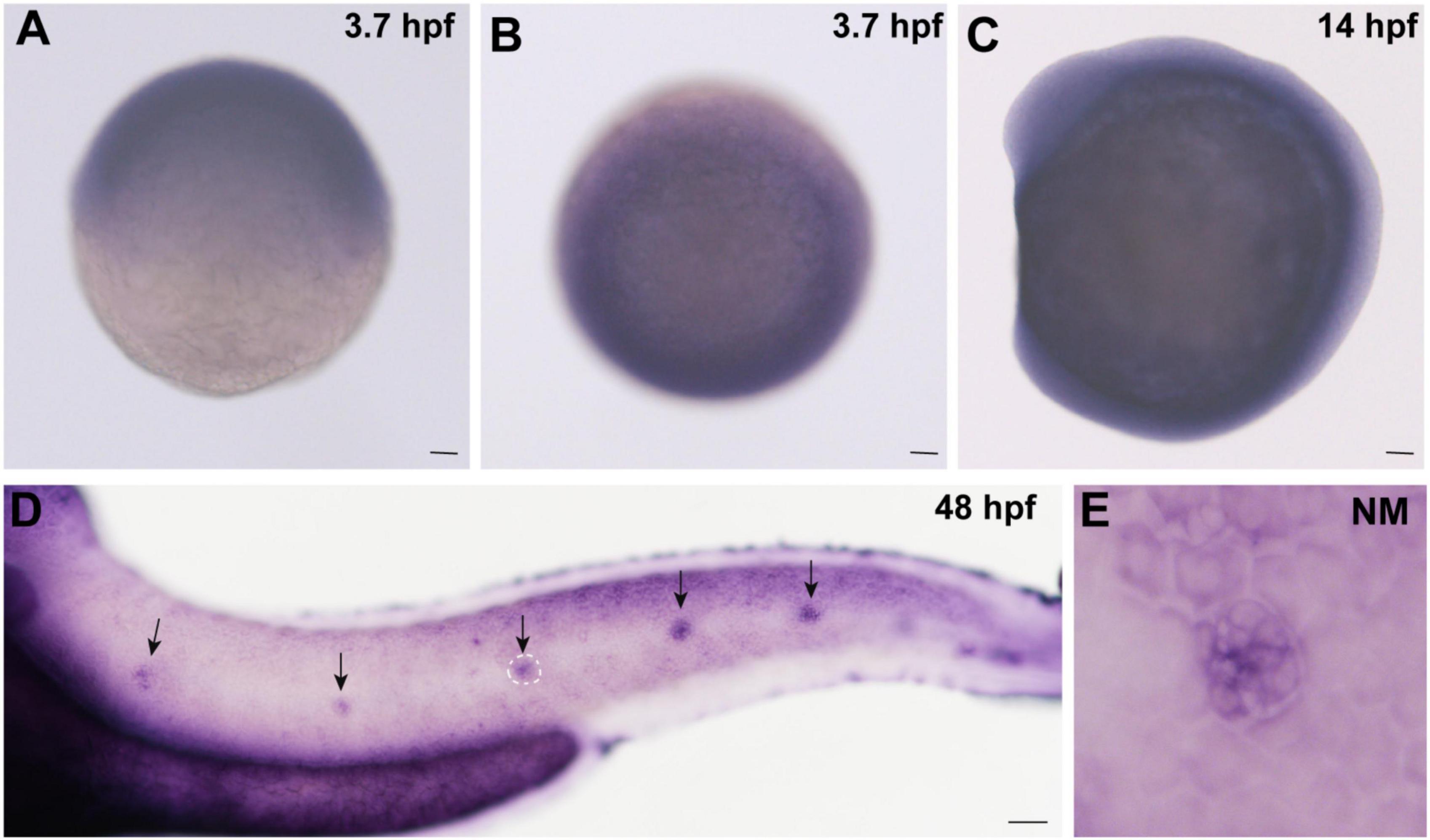
Figure 1. Expression of cingulin b is detected during the early development of zebrafish. (A,B) In situ hybridization staining of cingulin b at 3.7 hpf (n = 13) from the lateral view (A) and the top view (B). (C) Cingulin b is expressed in the whole somite at 14 hpf from the lateral view (n = 14). (D–E) The expression of cingulin b is focused on the neuromasts of the posterior lateral line system at 48 hpf (n = 11). Scale bars mark 50 μm in panel (A–D). The black arrows in D indicate neuromasts, and the white dotted lines labeled neuromast in D is magnified in panel (E).
Cingulin b Is Required for Normal Deposition of Neuromasts in Posterior Lateral Line System of Zebrafish
To explore the role of cingulin b in the development of zebrafish, we injected specific morpholino (MO) targeting cingulin b at one or two cell stage of embryos for knockdown of cingulin b. The control group was injected with Control-MO to eliminate the effect of injection operation. The efficacy of cingulin b-MO-injection was examined by in situ staining of cingulin b and qRT-PCR analysis, that we found significantly down-regulated expression of cingulin b in the PLL neuromasts in the cingulin b-MO morphants compared to the controls (Figures 2A–D), and the quantitative level of cingulin b was remarkably decreased after cingulin b-MO injection compared to the embryos injected with Control-MO (Figure 2E). We also examined the embryos as a whole in the Control-MO and cingulin b-MO groups, and we did not find any obvious malformation in the entire zebrafish after injection with cingulin b-MO (Supplementary Figure 2).
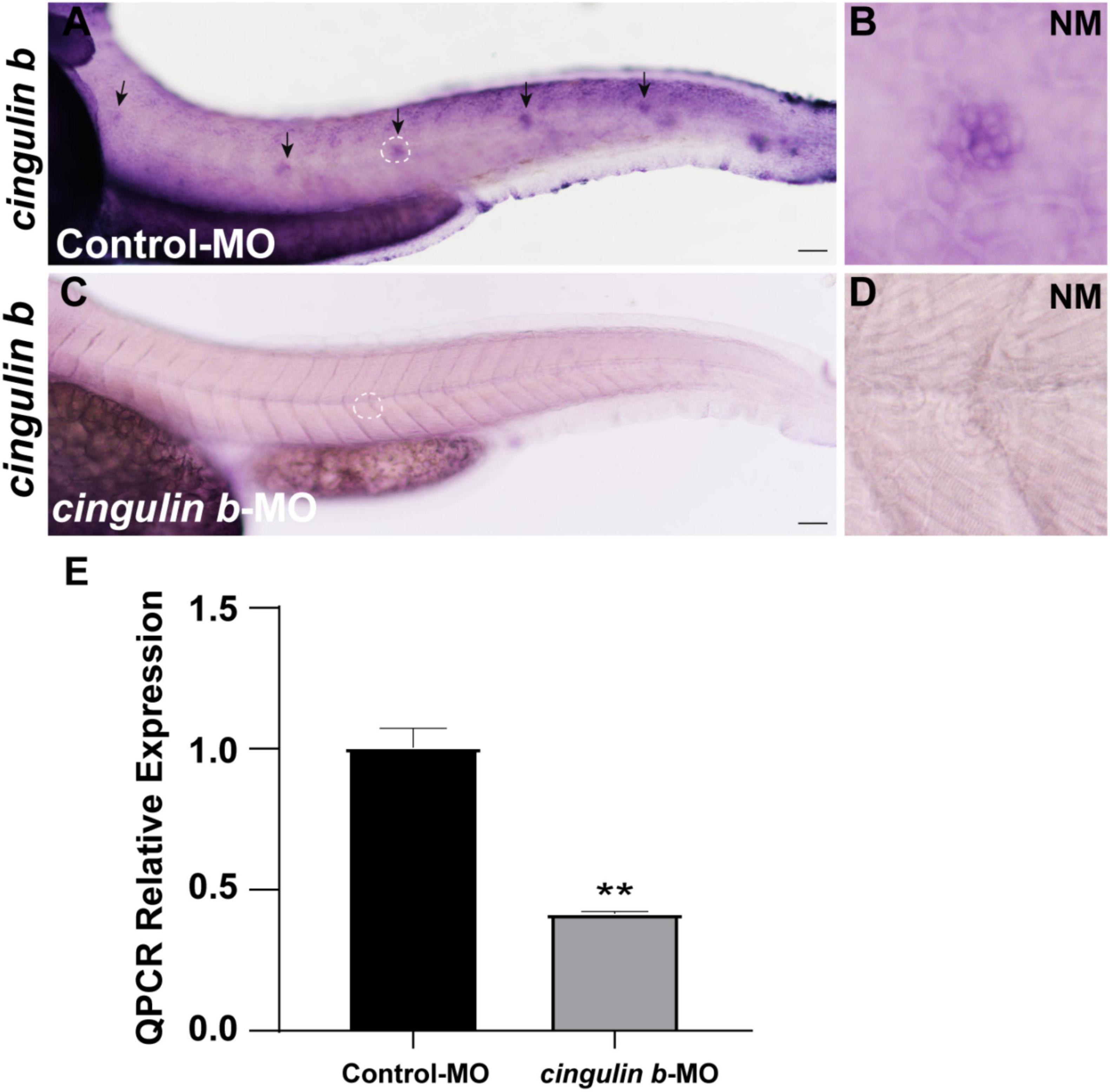
Figure 2. The efficacy of cingulin b-MO. (A–D) The expression of cingulin b is significantly reduced in the cingulin b-MO morphants (C), n = 8 compared to that in the Control-MO embryos (A) n = 5. (E) Quantitative analysis on the level of cingulin b between Control-MO and cingulin b-MO groups (n = 8 in each group). Data are shown in mean ± SEM, **p < 0.01. Scale bars in panel (A,C) mark 50 μm. The black arrows in panel (A) indicate the neuromasts, and the white dotted lines labeled neuromasts in panel (A,C) are magnified in panel (B,D), respectively.
The Tg (cldnb: lynGFP) zebrafish were used in this study to directly observe the morphology of neuromasts (Figure 3A). We counted the number of neuromasts at 48 hpf, a time point when the PLL primordium stops migration and finishes deposition (Kimmel et al., 1995; Nechiporuk and Raible, 2008). The average number of neuromasts in the trunk was notably decreased in the cingulin b-MO-injected morphants compared to that in the Control-MO-injected embryos (Figures 3A–C,F). The average number of neuromasts was even lower in 3 ng cingulin b-MO group than that in 2 ng cingulin b-MO group (Figures 3B–C,F), showing a dose-dependent manner, thus, we chose 3 ng dose for the following experiments. To avoid the non-specific effect of morpholino technology, we co-injected p53 with cingulin b-MO, and surprisingly the average number of neuromasts in the trunk in cingulin b-MO + p53 group was equivalent to that in cingulin b-MO-only group (Figures 3C–D,F). In addition, we also carried out rescue experiment, that combined injection with cingulin b mRNA and morpholino could partially restore the reduced number of neuromasts in the trunk (Figures 3E,F). The findings suggested that loss of cingulin b would affect the normal deposition of PLL neuromasts during the embryonic development of zebrafish. These findings were further validated by the expression of eya1, a marker for the neuromast in the lateral line of zebrafish (Kozlowski et al., 2005), that the number of neuromasts in the trunk was severely reduced in the cingulin b-MO morphants compared to that in the Control-MO embryos (Figures 3G,H). Taken together, our findings indicated that cingulin b was required in the lateral line system of zebrafish.
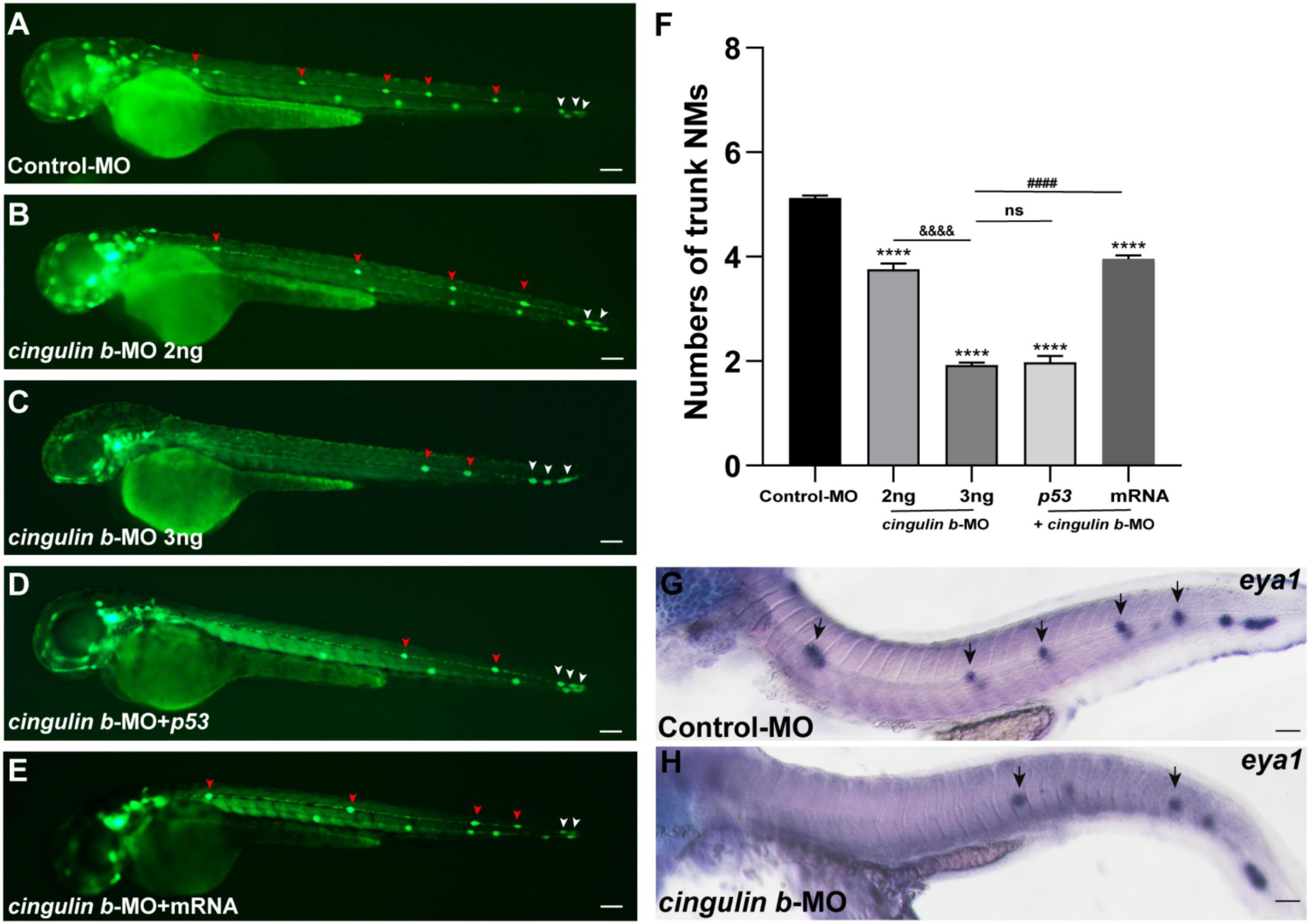
Figure 3. Inhibition of cingulin b affects the normal deposition of neuromasts in zebrafish. (A–E) In transgenic cldnb:lynGFP embryos, the neuromasts of PLL are labeled with green fluorescence. At 48 hpf, the deposition of neuromasts in zebrafish is shown in the control group (A), cingulin b knockdown group (B,C), cingulin b-MO + p53 group (D), and cingulin b-MO + cingulin b mRNA group (E), respectively. (F) The number of PLL neuromasts in controls (n = 235), cingulin b knockdown (2 ng or 3 ng) group (n = 86 and 264, respectively), cingulin b-MO (3 ng) + p53 group (n = 81), and cingulin b-MO (3 ng) + mRNA embryos (n = 180) at 48 hpf. The number of neuromasts decreased dose-dependently after knockdown of cingulin b (A–C,F). The decrease in the number of neuromasts is also confirmed when co-injecting with cingulin b-MO and p53 (C,D,F). Combined injection of cingulin b-MO and cingulin b mRNA can partially rescue the decrease in the number of neuromasts caused by cingulin b-MO (E,F). Red arrowheads mark the neuromasts in the trunk, and white arrowheads mark the terminal neuromasts of the PLL system (A–E). Scale bars represent 100 μm. Data are shown in mean ± SEM. *Stands by the comparison with the control group: ***p < 0.0001. #Stands by the comparison between cingulin b-MO group and cingulin b-MO + cingulin b mRNA group: ####p < 0.0001. &Stands by the comparison between 2 ng cingulin b-MO group and 3 ng cingulin b-MO group: &&&&p < 0.0001. ns means no significance. (G,H) The number of eya1 labeled neuromasts is markedly reduced after knocking down of cingulin b (G) n = 21 compared to the Control-MOs (H) n = 14. The black arrows in G and H indicate the neuromasts. Scale bars in panel (G,H) mark 50 μm.
Knockdown of Cingulin b Inhibits Cell Proliferation and Hair Cell Differentiation in the Lateral Line System of Zebrafish
During the development of zebrafish lateral line, the collective cells migrate and form rosette-like structure in the trailing region (Aman and Piotrowski, 2008). The deposition of neuromasts occurs after assembly of the last rosette (Nechiporuk and Raible, 2008). Here, we found that cell proliferation in the primordium was destroyed during the embryonic development of zebrafish by BrdU staining after knocking down the gene expression of cingulin b (Figures 4A–F). BrdU index was defined as the number of BrdU-positive cells divided by the number of total cells labeled by DAPI in this article, which was used to evaluate cell proliferation ability. In 36 dpf, the BrdU index in the primordium of cingulin b-MO morphants decreased significantly, that the BrdU index was 10.04% ± 0.02 (n = 17) compared to 39.74% ± 0.02 in the Control-MO group (Figure 4G).
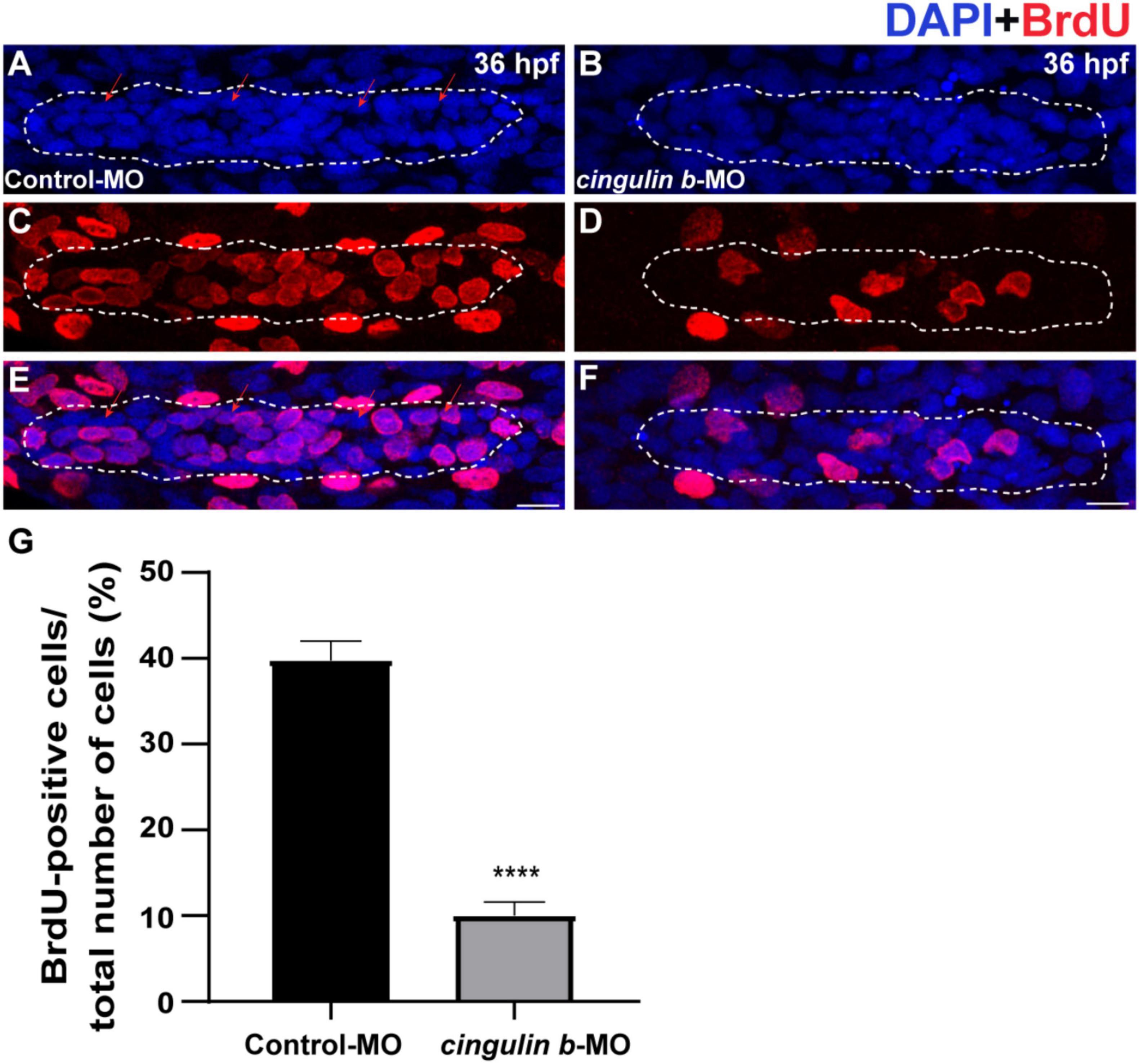
Figure 4. The proliferative cells in the PLL primordium are severely decreased while downregulation of cingulin b. (A–F) Representative images of BrdU positive proliferating cells and DAPI labeled nuclei in the controls (A,C,E) and cingulin b-deficient embryos (B,D,F) at 36 hpf. Red arrows indicate the rosette-shaped cell clusters in the primordium (A). Scale bars mark the 10 μm scale. (G) The quantitative analysis of BrdU index in control (n = 16) and cingulin b-MO embryos (n = 18). Data are shown in mean ± SEM. ****p < 0.0001.
To investigate the sustained effect of cingulin b in zebrafish embryonic development, we stained the proliferative cells with BrdU (Figures 5B,F) and collected embryos at 3 dpf. The Tg (brn3c: mGFP)s356t zebrafish were used here because of the HCs in neuromasts were labeled with GFP (Figures 5C,G). The total cells in neuromast were labeled with DAPI (Figures 5A,E). The merged images were shown in Figures 5D,H. The number of neuromast HCs in the trunk in cingulin b-MO experimental group decreased significantly compared to that of Control-MO group (Figure 5I). The BrdU index was also decreased severely in the cingulin b-MO group compared to that in the Control-MO group (Figure 5J). We also performed ISH staining of atohla, a maeker of HC, and found the expression of atoh1a was significantly decressed after knocking down of cigulin b compared to the control group (Figure 5K). Altogether, the data showed that knocking down cingulin b inhibited cell proliferation during primordia migration and neuromasts deposition in the early development process of zebrafish PLL system.
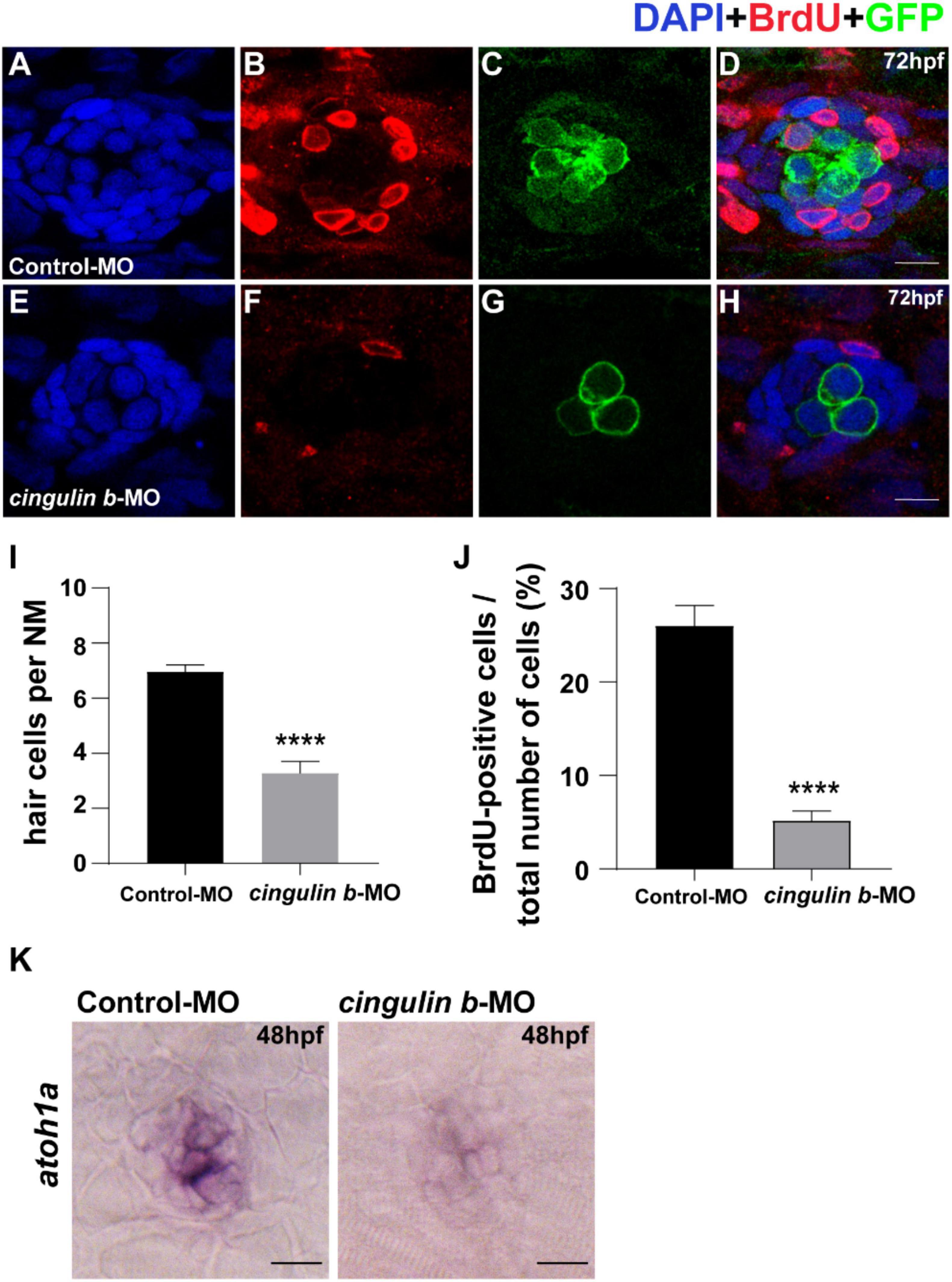
Figure 5. Knockdown of cingulin b reduces the number of HCs and cell proliferation in the neuromasts of zebrafish at 72 hpf. (A–H) The immunochemical staining of PLL neuromasts in the Control-MO group (n = 19) and cingulin b-MO group (n = 15). DAPI (green) labels nuclei (A,E) and BrdU (red) labels proliferative cells in the neuromast (B,F). In transgenic Tg (brn3c: mGFP)s356t lines, the membrane of HCs in PLL neuromasts are labeled with green fluorescence (GFP) (C,G). (I) The average number of hair cells per neuromast is significantly reduced after inhibition of cingulin b. (J) BrdU index in the neuromasts is also severely downregulated in the cingulin b-MO injected embryos compared to the controls. Scale bars mark 10 μm (A–H). Data are shown in mean ± SEM, and ****p < 0.0001. (K) The differentiation of HCs indicated by atoh1 ISH staining is inhibited after injection with cingulin b-MO. Scale bars in panel (K) mark 30 μm.
Mitogen-Activated Protein Kinase and Cellular Senescence Signaling Pathway Are Significantly Affected After Inhibition of Cingulin b
To explore the potential mechanism of cingulin b in regulating the development of zebrafish PLL system, we conducted RNA sequencing analysis to compare the difference between the control group and the cingulin b-MO mutants. KEGG analysis figured out the 13 top enriched pathways, of which MAPK signaling pathway and cellular senescence were the most two significant pathways evaluated by p value and gene counts (Figure 6). The key KEGG pathways, namely MAPK signaling pathway and cellular senescence pathway were listed in Figure 7. Also, the location of DEGs in cingulin b-MO siblings and overlapping genes of enriched pathways were revealed.
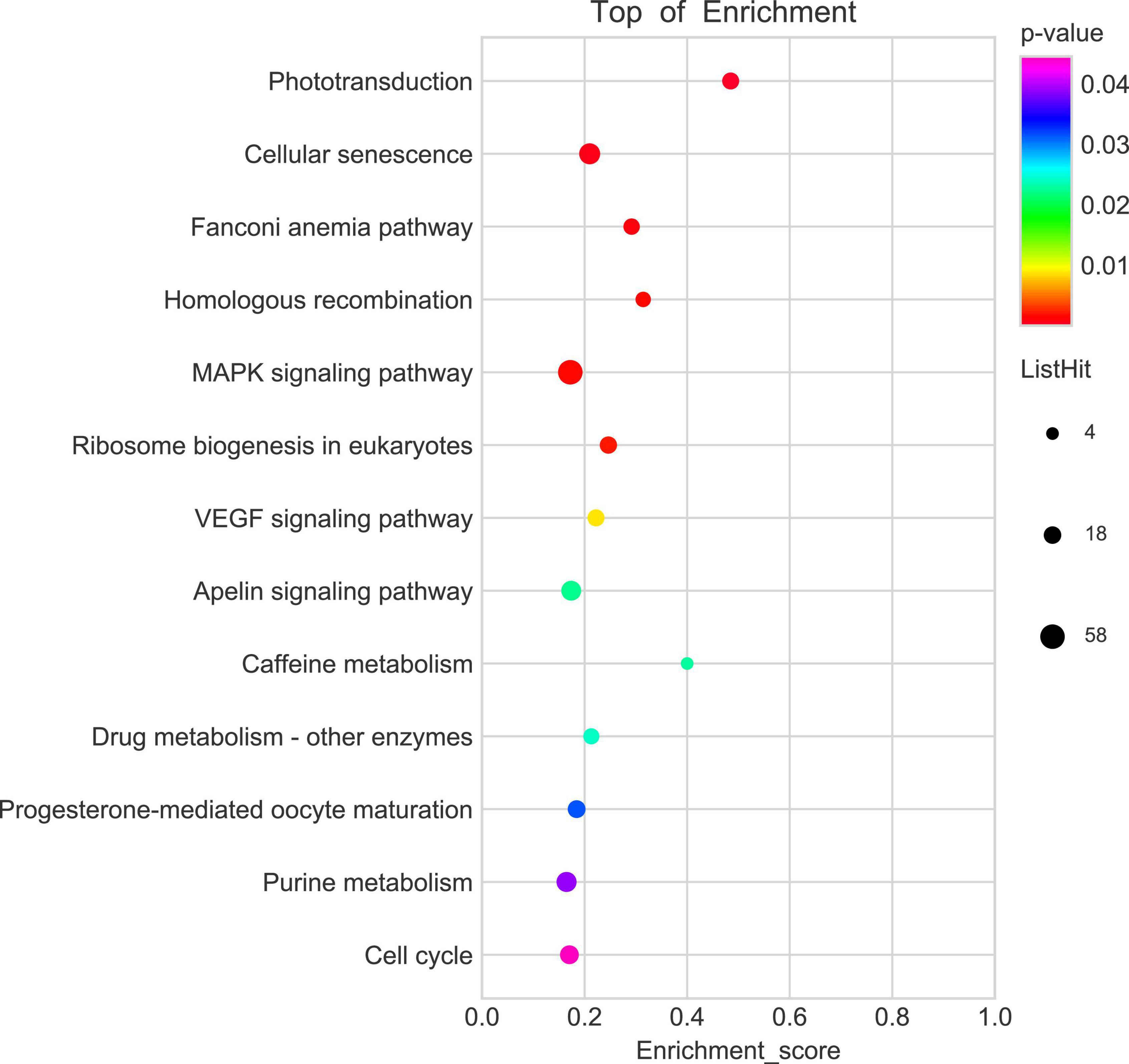
Figure 6. KEGG enrichment analysis screens out top 13 pathways which are highly differentiated expressed between controls and cingulin b-MO mutants. The analysis is conducted from three independent experiments in different groups (n = 30 embryos in each group), and p value <0.05 is considered as remarkable difference.
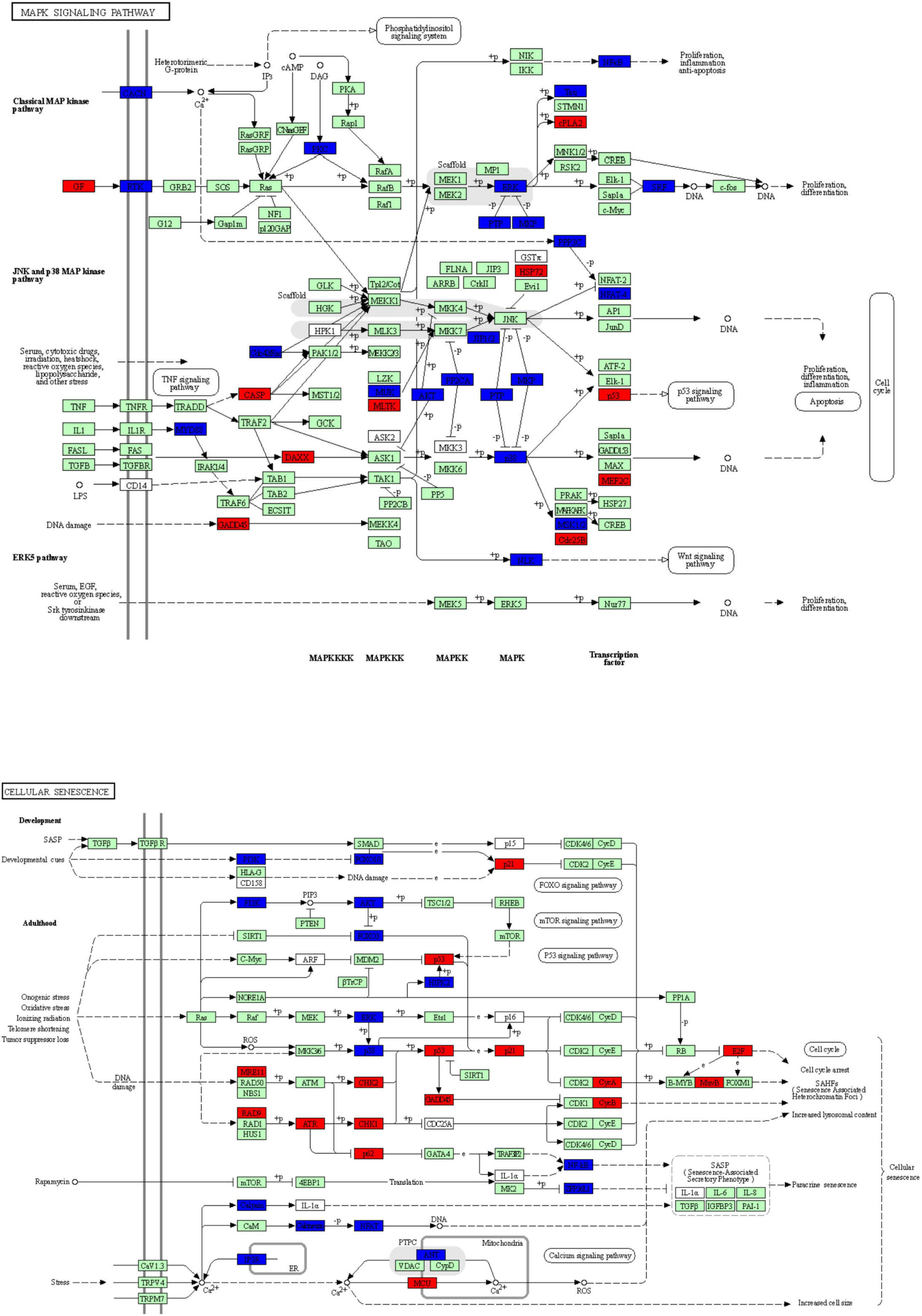
Figure 7. The key KEGG pathways: MAPK signaling pathway and cellular senescence signaling pathway. The red nodes represent upregulated DEGs in cingulin b-MO mutants, the green marked node represents downregulated DEGs in cingulin b-MO mutants, and the blue marked node represents overlapping targets between Control-MO and cingulin b-MO embryos. The analysis is conducted from three independent experiments in different groups, and each group has 30 embryos.
Heatmap analysis of DEGs of MAPK pathway and cellular senescence was screened in Figure 8 for Control-MO group vs. cingulin b-MO experimental group, respectively. RT-PCR analysis for some genes from MAPK and cellular senescence signaling pathways was conducted to verify our findings in RNA-sequencing data. The primer sequences were as listed in Supplementary Table 2. As shown in Figure 9, a total 9 genes in MAPK signaling pathways, 4 genes in cellular senescence pathway, and 9 genes overlapped in the two signaling pathways were examined. The mRNA levels of mapk1, mapk3, akt2, akt3b, atf7b, ppp3cca, and ppp3r1a were significantly decreased after knockdown of cingulin b, while the expression levels of tp53, mef2ca, mapk12b, and gadd45aa were significantly increased in cingulin b-MO group. The results of qRT-PCR were in consistency with those found in KEGG analysis, indicating that MAPK signaling was inhibited whereas cellular senescence was activated by repression of cingulin b.
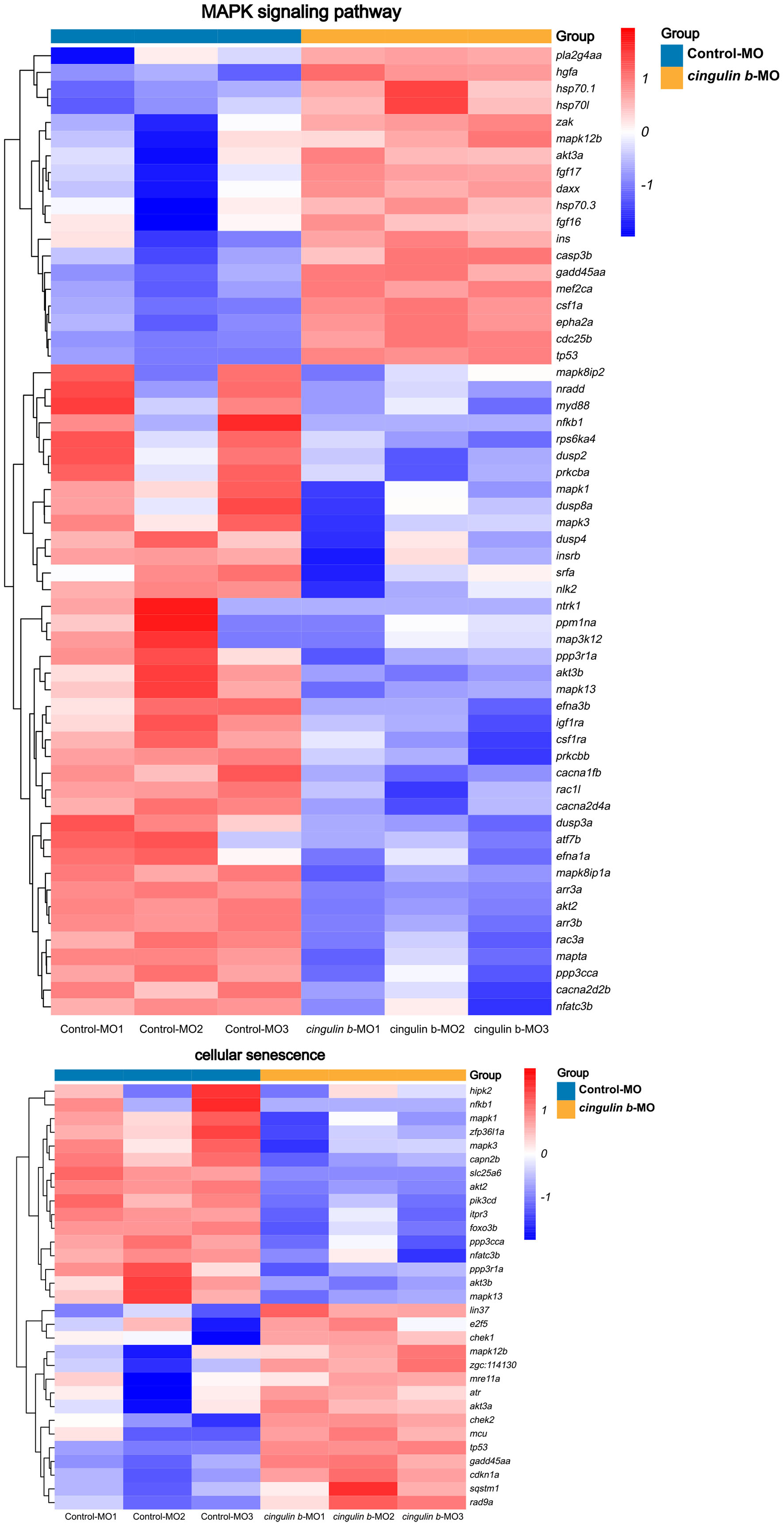
Figure 8. Heatmap analysis of MAPK signaling pathway and cellular senescence signaling pathway in comparison between Control-MO embryos and cingulin b-MO morphants. The red indicates upregulated DEGs and the blue indicates downregulated DEGs. The analysis is conducted from three independent experiments in different groups, and each group has 30 embryos.
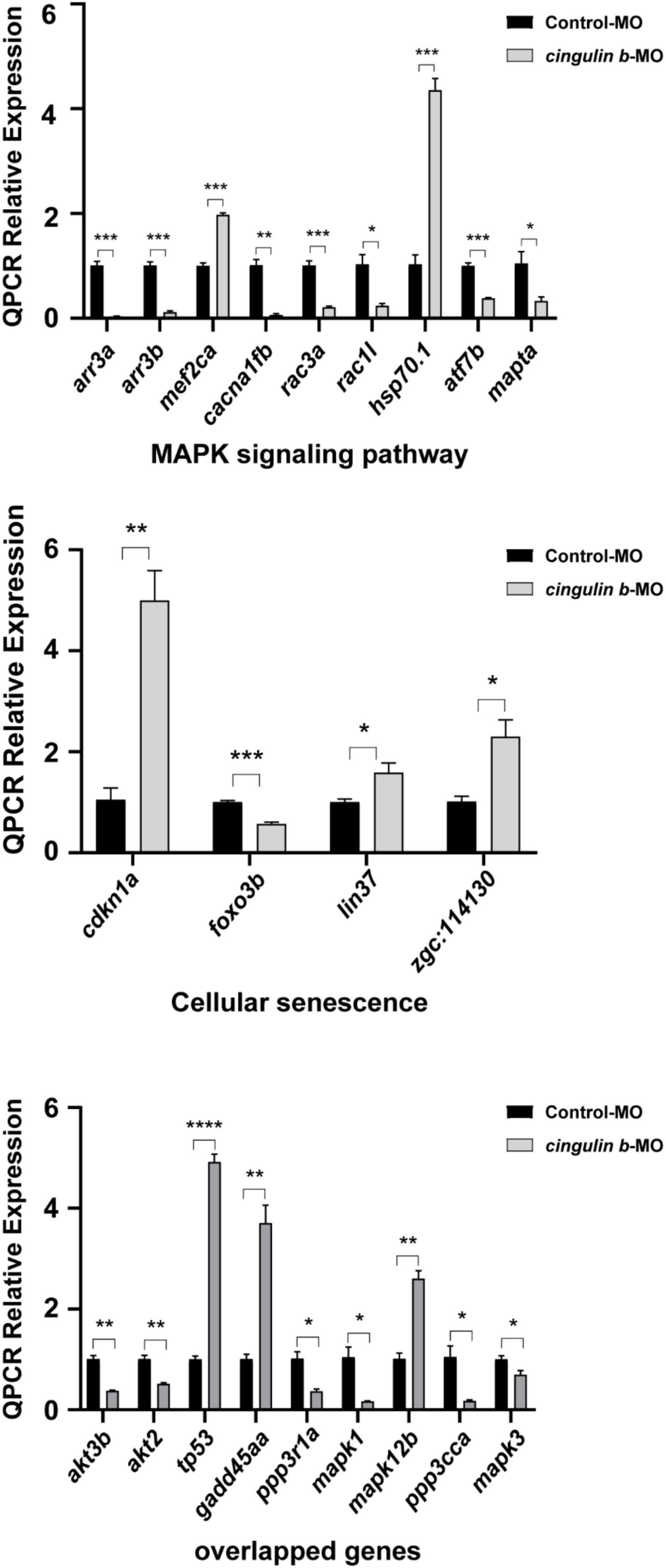
Figure 9. The relative mRNA levels of the indicated genes from MAPK and cellular senescence signaling pathways were normalized to the GAPDH level as determined by qRT-PCR. The results are recorded as mean ± SEM from three independent experiments (n = 8 embryos in each group). *p < 0.05, **p < 0.01, ***p < 0.001, and ****p < 0.0001.
Discussion
Cingulin is found interacting with connexin-26, a GJB2 encoding gene pivotal in hearing (Kelsell et al., 1997; Najmabadi et al., 2002), through the protein-protein interaction analysis (Batissoco et al., 2018). Besides, cingulin and connexin-26 are also found co-immuno-precipitated in the mouse organ of Cotri and stria vascularis (Batissoco et al., 2018). However, the role of cingulin in the cochlear development has not been identified. Previous studies have demonstrated that the PLL system of zebrafish is a good animal model for the research of mechanosensory organ development for the reason that the HCs in PLL neuromasts share similar structure and function with the mammalian inner ear HCs (He et al., 2017; Tang et al., 2019; Tang et al., 2021). In this study, we detected obvious expression of cingulin b in the PLL neuromasts of zebrafish. However, the number of PLL neuromasts was significantly decreased after knockdown of cingulin b by antisense MO injection, and we also found severe repression of cell proliferation and hair cell differentiation in the PLL primordium and neuromasts. Additionally, the RNA sequence analysis revealed that MAPK signaling was downregulated while cellular senescence signaling was upregulated in the cingulin b-MO embryos compared to the Control-MO injection embryos. Furthermore, we also confirmed the findings by heatmap differential analysis through qRT-PCR experiment. Our findings demonstrated that cingulin b was required for the normal development of zebrafish posterior lateral line by regulating the MAPK and cellular senescence signaling pathways.
Mitogen-activated protein kinase (MAPK) has been reported to be related to the formation of primordium in the posterior lateral line system of zebrafish (Harding and Nechiporuk, 2012). MAPK signaling pathway has three subfamilies, namely classical ERK pathway, Jun N-terminal kinase (JNK) pathway, and p38 pathway (Zhang and Liu, 2002). Activation of ERK1/2 enhances cell proliferation (Lavoie et al., 2020), induces the expression of Cyclin D1 (Chen et al., 2020), and regulates the G1/S progression of cell cycle (Jirmanova et al., 2002). JNK and p38 pathways are often activated by stresses from environment or toxic agents, and usually exert antagonistic effects on cell proliferation and cell survival (Wagner and Nebreda, 2009). As previously reported, p38 is considered as a negative regulator of cell cycle procession through downregulating cyclins and upregulating inhibitors of cyclin-dependent kinase (CDKIs) (Thornton and Rincon, 2009). In our previous study, we find that JNK inhibitor SP600125 suppresses the development of zebrafish lateral line by induction of p21 and p53 (Cai et al., 2016), which links the JNK pathway with tumor suppressor p53. Another study also demonstrates that JNK is the negative modulation of p53 (Das et al., 2007). In the present study, mapk1 and mapk3 were significantly downregulated while tp53 and gadd45aa were remarkably upregulated after knockdown of cingulin b in comparison with the Control-MO-injected controls, which were in consistency with the previous reporters.
Cellular senescence is a permanent cell cycle arrest after different damages, such as aging, oncogenes, oxidative agents, chemotherapeutic drugs, or epigenetic modulators (Hernandez-Segura et al., 2018). Senescent cells have variable phenotypes but share some common hallmarks in the mechanism, of which CDKIs are widely involved in the progression of cellular senescence, and the main components driving cell cycle arrest in senescence are cdkn1a (p21), cdkn2a (p16), and cdkn2b (p15) (Hernandez-Segura et al., 2018). Cellular senescence is found relevant to the development and tissue regeneration of zebrafish (Da Silva-Alvarez et al., 2020a,b). In this study, we observed strong elevation in the expression of tp53, cdkn1a, and gadd45aa in the morphants injected with cingulin b-MO compared to the control embryos, suggesting the activation of cellular senescence after inhibition of cingulin b.
Conclusion
We demonstrate that cingulin b is required in the development of zebrafish lateral line system, and MAPK signaling pathway and cellular senescence are regulated by morpholino knockdown of cingulin b. To our knowledge, it’s the first time that the function of cingulin b is explored in the mechanosensory organs of zebrafish, but further studies are needed to detect direct evidence between auditory organ development and cingulin, the proteins of tight junctions.
Data Availability Statement
The datasets presented in this study can be found in online repositories. The names of the repository/repositories and accession number(s) can be found below: https://www.ncbi.nlm.nih.gov/search/all/?term=PRJNA802059.
Ethics Statement
The animal study was reviewed and approved by the Animal Conservation and Utilization Committee of Fudan University in Shanghai.
Author Contributions
YH, DL, and SL: conceptualization, methodology, writing—review and editing, and project administration. YL, DT, ZZ, XW, NZ, RY, CeW, HX, JM, and CuW: methodology and formal analysis. YL, DT, and ZZ: validation, investigation, and formal analysis. All authors read and approved the final manuscript.
Funding
This work was supported by grants from the National Natural Science Foundation of China (Nos. 82071045, 81870728, and 81800912) and Shanghai Rising-Star Program (19QA1401800).
Conflict of Interest
The authors declare that the research was conducted in the absence of any commercial or financial relationships that could be construed as a potential conflict of interest.
The reviewer ZS declared a shared affiliation with several of the authors DT and ZZ to the handling editor at the time of the review.
Publisher’s Note
All claims expressed in this article are solely those of the authors and do not necessarily represent those of their affiliated organizations, or those of the publisher, the editors and the reviewers. Any product that may be evaluated in this article, or claim that may be made by its manufacturer, is not guaranteed or endorsed by the publisher.
Supplementary Material
The Supplementary Material for this article can be found online at: https://www.frontiersin.org/articles/10.3389/fnmol.2022.844668/full#supplementary-material
Supplementary Figure 1 | The representative S (sense control) and AS (antisense mRNA probe) images of cingulin b in the PLL of zebrafish at 48 hpf. Black arrowheads indicate neuromasts. Scale bars represent 50 μm, n = 10 in S group, and n = 7 in AS group.
Supplementary Figure 2 | The representative images of embryos as a whole as followed in the Control-MO and cingulin b-MO groups.
Supplementary Table 1 | Primers for the synthesis of objective genes in WISH experiment.
Supplementary Table 2 | Primers for Real-Time PCR experiment.
Abbreviations
DEGs, differently expressed genes; HCs, hair cells; hpf, hours post-fertilization; JNK, Jun N-terminal kinase; MAPK, Mitogen-activated protein kinase; PLL, posterior lateral line; qRT-PCR, quantitative real-time PCR; TJ, tight junctions; WISH, whole mount in situ hybridization; GFP, green fluorescent protein.
References
Aman, A., and Piotrowski, T. (2008). Wnt/beta-catenin and Fgf signaling control collective cell migration by restricting chemokine receptor expression. Dev. Cell 15, 749–761. doi: 10.1016/j.devcel.2008.10.002
Batissoco, A. C., Salazar-Silva, R., Oiticica, J., Bento, R. F., Mingroni-Netto, R. C., and Haddad, L. A. (2018). A Cell Junctional Protein Network Associated with Connexin-26. Int. J. Mol. Sci. 2018:19. doi: 10.3390/ijms19092535
Brignull, H. R., Raible, D. W., and Stone, J. S. (2009). Feathers and fins: non-mammalian models for hair cell regeneration. Brain Res. 1277, 12–23. doi: 10.1016/j.brainres.2009.02.028
Cai, C., Lin, J., Sun, S., and He, Y. (2016). JNK Inhibition Inhibits Lateral Line Neuromast Hair Cell Development. Front. Cell Neurosci. 10:19. doi: 10.3389/fncel.2016.00019
Chen, C. A., Chang, J. M., Yang, Y. L., Chang, E. E., and Chen, H. C. (2020). Macrophage migration inhibitory factor regulates integrin-β1 and cyclin D1 expression via ERK pathway in podocytes. Biomed. Pharmacother. 124:109892. doi: 10.1016/j.biopha.2020.109892
Citi, S. (2019). The mechanobiology of tight junctions. Biophys. Rev. 11, 783–793. doi: 10.1007/s12551-019-00582-7
Cordenonsi, M., D’atri, F., Hammar, E., Parry, D. A., Kendrick-Jones, J., Shore, D., et al. (1999). Cingulin contains globular and coiled-coil domains and interacts with ZO-1, ZO-2, ZO-3, and myosin. J. Cell Biol. 147, 1569–1582. doi: 10.1083/jcb.147.7.1569
Da Silva-Alvarez, S., Guerra-Varela, J., Sobrido-Camean, D., Quelle, A., Barreiro-Iglesias, A., Sanchez, L., et al. (2020a). Cell senescence contributes to tissue regeneration in zebrafish. Aging Cell 19:e13052. doi: 10.1111/acel.13052
Da Silva-Alvarez, S., Guerra-Varela, J., Sobrido-Camean, D., Quelle, A., Barreiro-Iglesias, A., Sanchez, L., et al. (2020b). Developmentally-programmed cellular senescence is conserved and widespread in zebrafish. Aging 12, 17895–17901. doi: 10.18632/aging.103968
Das, M., Jiang, F., Sluss, H. K., Zhang, C., Shokat, K. M., Flavell, R. A., et al. (2007). Suppression of p53-dependent senescence by the JNK signal transduction pathway. Proc. Natl. Acad. Sci. U S A 104, 15759–15764. doi: 10.1073/pnas.0707782104
D’atri, F., Nadalutti, F., and Citi, S. (2002). Evidence for a functional interaction between cingulin and ZO-1 in cultured cells. J. Biol. Chem. 277, 27757–27764. doi: 10.1074/jbc.M203717200
Dooley, K., and Zon, L. I. (2000). Zebrafish: a model system for the study of human disease. Curr. Opin. Genet. Dev. 10, 252–256. doi: 10.1016/s0959-437x(00)00074-5
Driever, W., Stemple, D., Schier, A., and Solnica-Krezel, L. (1994). Zebrafish: genetic tools for studying vertebrate development. Trends Genet. 10, 152–159. doi: 10.1016/0168-9525(94)90091-4
González-Mariscal, L., Domínguez-Calderón, A., Raya-Sandino, A., Ortega-Olvera, J. M., Vargas-Sierra, O., and Martínez-Revollar, G. (2014). Tight junctions and the regulation of gene expression. Semin. Cell Dev. Biol. 36, 213–223.
Harding, M. J., and Nechiporuk, A. V. (2012). Fgfr-Ras-MAPK signaling is required for apical constriction via apical positioning of Rho-associated kinase during mechanosensory organ formation. Development 139, 3130–3135. doi: 10.1242/dev.082271
Hawkins, B. T., and Davis, T. P. (2005). The blood-brain barrier/neurovascular unit in health and disease. Pharmacol. Rev. 57, 173–185. doi: 10.1124/pr.57.2.4
He, Y., Bao, B., and Li, H. (2017). Using zebrafish as a model to study the role of epigenetics in hearing loss. Expert Opin. Drug Discov. 12, 967–975. doi: 10.1080/17460441.2017.1340270
He, Y., Wu, J., Mei, H., Yu, H., Sun, S., Shou, J., et al. (2014). Histone deacetylase activity is required for embryonic posterior lateral line development. Cell Prolif 47, 91–104. doi: 10.1111/cpr.12081
Hernandez-Segura, A., Nehme, J., and Demaria, M. (2018). Hallmarks of Cellular Senescence. Trends Cell Biol. 28, 436–453. doi: 10.1016/j.tcb.2018.02.001
Howe, K., Clark, M. D., Torroja, C. F., Torrance, J., Berthelot, C., Muffato, M., et al. (2013). The zebrafish reference genome sequence and its relationship to the human genome. Nature 496, 498–503. doi: 10.1038/nature12111
Jirmanova, L., Afanassieff, M., Gobert-Gosse, S., Markossian, S., and Savatier, P. (2002). Differential contributions of ERK and PI3-kinase to the regulation of cyclin D1 expression and to the control of the G1/S transition in mouse embryonic stem cells. Oncogene 21, 5515–5528. doi: 10.1038/sj.onc.1205728
Kalueff, A. V., Stewart, A. M., and Gerlai, R. (2014). Zebrafish as an emerging model for studying complex brain disorders. Trends Pharmacol. Sci. 35, 63–75. doi: 10.1016/j.tips.2013.12.002
Kelsell, D. P., Dunlop, J., Stevens, H. P., Lench, N. J., Liang, J. N., Parry, G., et al. (1997). Connexin 26 mutations in hereditary non-syndromic sensorineural deafness. Nature 387, 80–83. doi: 10.1038/387080a0
Kimmel, C. B., Ballard, W. W., Kimmel, S. R., Ullmann, B., and Schilling, T. F. (1995). Stages of embryonic development of the zebrafish. Dev. Dyn. 203, 253–310. doi: 10.1002/aja.1002030302
Kozlowski, D. J., Whitfield, T. T., Hukriede, N. A., Lam, W. K., and Weinberg, E. S. (2005). The zebrafish dog-eared mutation disrupts eya1, a gene required for cell survival and differentiation in the inner ear and lateral line. Dev. Biol. 277, 27–41. doi: 10.1016/j.ydbio.2004.08.033
Lavoie, H., Gagnon, J., and Therrien, M. (2020). ERK signalling: a master regulator of cell behaviour, life and fate. Nat. Rev. Mol. Cell Biol. 21, 607–632. doi: 10.1038/s41580-020-0255-7
Leonova, E. V., and Raphael, Y. (1997). Organization of cell junctions and cytoskeleton in the reticular lamina in normal and ototoxically damaged organ of Corti. Hear Res. 113, 14–28. doi: 10.1016/s0378-5955(97)00130-5
Mandrekar, N., and Thakur, N. L. (2009). Significance of the zebrafish model in the discovery of bioactive molecules from nature. Biotechnol. Lett. 31, 171–179. doi: 10.1007/s10529-008-9868-1
Najmabadi, H., Cucci, R. A., Sahebjam, S., Kouchakian, N., Farhadi, M., Kahrizi, K., et al. (2002). GJB2 mutations in Iranians with autosomal recessive non-syndromic sensorineural hearing loss. Hum. Mutat. 19:572. doi: 10.1002/humu.9033
Nechiporuk, A., and Raible, D. W. (2008). FGF-dependent mechanosensory organ patterning in zebrafish. Science 320, 1774–1777. doi: 10.1126/science.1156547
Nicolson, T. (2005). The genetics of hearing and balance in zebrafish. Annu. Rev. Genet. 39, 9–22. doi: 10.1146/annurev.genet.39.073003.105049
Ohnishi, H., Nakahara, T., Furuse, K., Sasaki, H., Tsukita, S., and Furuse, M. (2004). JACOP, a novel plaque protein localizing at the apical junctional complex with sequence similarity to cingulin. J. Biol. Chem. 279, 46014–46022. doi: 10.1074/jbc.M402616200
Pyati, U. J., Look, A. T., and Hammerschmidt, M. (2007). Zebrafish as a powerful vertebrate model system for in vivo studies of cell death. Semin. Cancer Biol. 17, 154–165. doi: 10.1016/j.semcancer.2006.11.007
Raphael, Y., and Altschuler, R. A. (1991). Reorganization of cytoskeletal and junctional proteins during cochlear hair cell degeneration. Cell Motil. Cytoskeleton. 18, 215–227. doi: 10.1002/cm.970180307
Tang, D., He, Y., Li, W., and Li, H. (2019). Wnt/β-catenin interacts with the FGF pathway to promote proliferation and regenerative cell proliferation in the zebrafish lateral line neuromast. Exp. Mol. Med. 51, 1–16. doi: 10.1038/s12276-019-0247-x
Tang, D., Lu, Y., Zuo, N., Yan, R., Wu, C., Wu, L., et al. (2021). The H3K27 demethylase controls the lateral line embryogenesis of zebrafish. Cell Biol. Toxicol. 2021:9669. doi: 10.1007/s10565-021-09669-y
Thisse, B., and Thisse, C. (2014). In situ hybridization on whole-mount zebrafish embryos and young larvae. Methods Mol. Biol. 1211, 53–67. doi: 10.1007/978-1-4939-1459-3_5
Thornton, T. M., and Rincon, M. (2009). Non-classical p38 map kinase functions: cell cycle checkpoints and survival. Int. J. Biol. Sci. 5, 44–51. doi: 10.7150/ijbs.5.44
Van Itallie, C. M., Fanning, A. S., Bridges, A., and Anderson, J. M. (2009). ZO-1 stabilizes the tight junction solute barrier through coupling to the perijunctional cytoskeleton. Mol. Biol. Cell 20, 3930–3940. doi: 10.1091/mbc.e09-04-0320
Wagner, E. F., and Nebreda, A. R. (2009). Signal integration by JNK and p38 MAPK pathways in cancer development. Nat. Rev. Cancer 9, 537–549. doi: 10.1038/nrc2694
Yano, T., Matsui, T., Tamura, A., Uji, M., and Tsukita, S. (2013). The association of microtubules with tight junctions is promoted by cingulin phosphorylation by AMPK. J. Cell Biol. 203, 605–614. doi: 10.1083/jcb.201304194
Zhang, W., and Liu, H. T. (2002). MAPK signal pathways in the regulation of cell proliferation in mammalian cells. Cell Res. 12, 9–18. doi: 10.1038/sj.cr.7290105
Keywords: cingulin b, zebrafish, development, MAPK signaling pathway, cellular senescence
Citation: Lu Y, Tang D, Zheng Z, Wang X, Zuo N, Yan R, Wu C, Ma J, Wang C, Xu H, He Y, Liu D and Liu S (2022) Cingulin b Is Required for Zebrafish Lateral Line Development Through Regulation of Mitogen-Activated Protein Kinase and Cellular Senescence Signaling Pathways. Front. Mol. Neurosci. 15:844668. doi: 10.3389/fnmol.2022.844668
Received: 28 December 2021; Accepted: 11 March 2022;
Published: 05 May 2022.
Edited by:
Pranav Mathur, Otonomy Inc., United StatesReviewed by:
Allison B. Coffin, Washington State University, United StatesZhiyong Shao, Fudan University, China
Phillip Uribe, Otonomy Inc., United States
Copyright © 2022 Lu, Tang, Zheng, Wang, Zuo, Yan, Wu, Ma, Wang, Xu, He, Liu and Liu. This is an open-access article distributed under the terms of the Creative Commons Attribution License (CC BY). The use, distribution or reproduction in other forums is permitted, provided the original author(s) and the copyright owner(s) are credited and that the original publication in this journal is cited, in accordance with accepted academic practice. No use, distribution or reproduction is permitted which does not comply with these terms.
*Correspondence: Yingzi He, eWluZ3ppaGUwOTYxMUAxMjYuY29t; Dong Liu, bGl1ZG9uZ3RvbUBnbWFpbC5jb20=, dG9tQG50dS5lZHUuY24=; Shaofeng Liu, bGl1c2ZfY25AMTYzLmNvbQ==
†These authors have contributed equally to this work