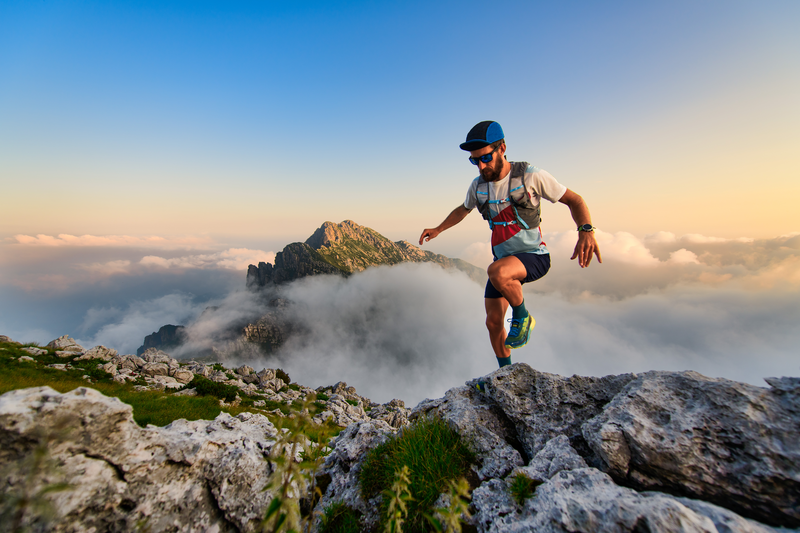
94% of researchers rate our articles as excellent or good
Learn more about the work of our research integrity team to safeguard the quality of each article we publish.
Find out more
MINI REVIEW article
Front. Mol. Neurosci. , 13 October 2022
Sec. Brain Disease Mechanisms
Volume 15 - 2022 | https://doi.org/10.3389/fnmol.2022.1043947
This article is part of the Research Topic Molecular Mechanisms and Pathways in Cerebellar Function View all 14 articles
Spinocerebellar ataxia (SCA) is an autosomal dominant neurodegenerative disease (ND) with a high mortality rate. Symptomatic treatment is the only clinically adopted treatment. However, it has poor effect and serious complications. Traditional diagnostic methods [such as magnetic resonance imaging (MRI)] have drawbacks. Presently, the superiority of RNA interference (RNAi) and extracellular vesicles (EVs) in improving SCA has attracted extensive attention. Both can serve as the potential biomarkers for the diagnosing and monitoring disease progression. Herein, we analyzed the basis and prospect of therapies for SCA. Meanwhile, we elaborated the development and application of miRNAs, siRNAs, shRNAs, and EVs in the diagnosis and treatment of SCA. We propose the combination of RNAi and EVs to avoid the adverse factors of their respective treatment and maximize the benefits of treatment through the technology of EVs loaded with RNA. Obviously, the combinational therapy of RNAi and EVs may more accurately diagnose and cure SCA.
Spinocerebellar ataxia (SCA) is a group of progressive neurodegenerative diseases (NDs) with autosomal dominant inheritance. They are divided into 48 subtypes according to the time sequence of discovery of the pathogenic gene loci. SCA has different clinical features, and ataxia is their core symptom (Chen H. Y. et al., 2021; Shin et al., 2021). The pathologically extended cytosine adenine guanine (CAG) repeat sequences encoded by poly-glutamine (polyQ) are their common pathogenesis, which leads to the misfolding and aggregation of translated protein and causes neuronal death (Shin et al., 2021). The prevalence of SCA is approximately 3/100,000 that varies in different subtypes and regions (Gómez et al., 2022). Currently, there are only several symptomatic treatments for SCA, which cannot prevent or delay the progression of SCA with severe complications. Patients usually die because brain stem lesions, pulmonary infection and respiratory failure within 10–30 years after diagnosis (Pérez Ortiz and Orr, 2018; Cendelin et al., 2022). Thyroid-stimulating hormone releasing hormone (TRH) can improve the symptoms (Shimizu et al., 2020). However, its complications such as headache and nausea occur in about 50% patients (Ghanekar et al., 2022). Aripiprazole improves dyskinesia but has significant side effects, such as anxiety, nausea, and dizziness (Jalles et al., 2022). The complex pathogenic mechanism and differences between subtypes, patients show the inadequacy of symptomatic treatment (Yap et al., 2022b).
Gene therapies, including RNA interference (RNAi), antisense oligonucleotides, and gene editing technology, are hoped to cure SCA by correcting the pathogenic genes (Vázquez-Mojena et al., 2021). RNAi is the most popular and effective way in preclinical trials mediated by microRNAs (miRNAs), small interfering RNAs (siRNAs), and short hair protein RNAs (shRNAs) (Costa, 2020). Repeat-targeting shRNAs specifically silence the expression of extended CAG repeats (Kotowska-Zimmer et al., 2020). Adeno-associated virus (AAV) loads with microRNA against ataxin (ATXN) 3 to restore the neuronal signaling and physiological functions in SCA3 mice (Nobre et al., 2022). Long-term and persistent RNAi reduces the cerebellar lesions and axonal demyelination in SCA3 mice by lowering the level of ATXN3 (Costa et al., 2020). However, RNAi has disadvantages such as carrier selection, poor stability in vivo, off-target effect and toxicity caused by excess effect (Vázquez-Mojena et al., 2021; Wang et al., 2022). Extracellular vesicles (EVs) as carriers have long plasma half-life, strong stability in vivo and can target the injury site (Sun et al., 2010; Huang et al., 2020). miRNA is an important component of EVs, which can treat SCA. Mesenchymal stem cell (MSC)-derived exosomes can alleviate the damage to Purkinje fibers and myelin sheath, motor function and neuroinflammation in SCA3 mice (You et al., 2020; Tao et al., 2021). The miRNA in EVs can be biomarkers of SCA3, revealing the subtypes and progression of SCA3 (Hou et al., 2019). Obviously, the combination of RNAi and EVs can avoid the adverse factors of RNAi or EVs treatment alone to maximize the benefits of treatment. The combinational therapy of RNAi and EVs is a brand new idea. It may more accurately diagnose and cure SCA, which still needs our in-depth research.
RNA interference is a process, which is mediated by double-stranded RNA (dsRNA) and leads to the silence of target genes (mRNA) containing complementary sequences and inhibition of related protein production (Kara et al., 2022). dsRNAs in the process include miRNAs, siRNAs and shRNAs (Costa, 2020). The pathogenesis of NDs remains unknown. However, their pathological features are the deposition of misfolded protein such as amyloid-β, alpha-synuclein, tau and prion proteins. RNAi can completely eliminate or alter the synthesis of target protein (Khan et al., 2022). RNAi can treat SCA by silencing the specific genes and assisting in the diagnosis and condition monitoring of SCA.
microRNAs are single-stranded non-coding RNAs, about 19–25 nucleotides in length. They are RNAi silencing trigger that binds to a specific region of 3′ untranslated region of messenger RNA, silences the target gene by altering RNA stability to inhibit the corresponding protein synthesis (Wei et al., 2021). miRNAs are divided into artificial synthesis miRNAs and natural synthesis miRNAs. Artificial miRNAs can target disease genes (Saad et al., 2021). They are important for brain development and homeostasis (Penning et al., 2021). The imbalance of miRNAs is closely related to the pathogenesis of NDs, which may be the biomarkers. The expressions of Hsa-miR-204-3p and hsa-miR-873-3p are significantly increased, and the expression of hsa-miR-6840-5p is decreased in the patients of progressive supranuclear palsy (Nonaka et al., 2022). The expressions of miR-132-5p, miR-138-5p, and miR-129-5p are reduced in the brain of Alzheimer’s disease (AD) patients (Dobricic et al., 2022). MIR-144 expression is significantly increased in Multiple Sclerosis (MS) patients (Roshani et al., 2021). miR-181 can be a potential biomarker for the prognosis of amyotrophic lateral sclerosis (ALS). Patients with low miR-181 content have a longer survival time (Magen et al., 2021).
microRNAs are important for the treatment of NDs. The increased serum miR-485-3p can be a diagnostic marker for AD. Knocking out miR-485-3p reduces neuro-inflammation and improves neuronal viability, which is conducive to protect neurons in AD patients (Yu et al., 2021). Injection of AAV encoding miRNA targets to the superoxide dismutase 1 and immunosuppressive agents to improve sensory dysfunction and delay disease progression in an ALS patient (Mueller et al., 2020). miRNA inhibits the effect of stress on visual transduction and reduces the retinal inflammation to treat the age-related macular degeneration (Chu-Tan et al., 2021).
microRNAs are potential in the diagnosis, treatment and monitoring of SCA. Three miRNAs interact with ATXN3-3′UTR (miR-9, mir-181a, and mir-494) that are overexpressed in SCA3 patients (Carmona et al., 2017). The injection of AAV expressing miR760 reduces ATXN1 levels in vivo and improves motor disharmony in the cerebellum of SCA1 mice (Nitschke et al., 2020). miR-32 and miR-181c target the 3′UTR of ATXN3 and inhibit the expression of ATXN3 to reduce the polyQ-mediated cytotoxicity (Krauss et al., 2019). AAV5-loaded miRNA ATXN3 specifically decreases the ATXN3 mRNA and protein in SCA3 mice and cell models (Martier et al., 2019). The lowest effective dose, the maximum tolerated dose and the toxicity threshold that can improve SCA1 to guide the clinical application of RNAi in the treatment of SCA1 (Keiser et al., 2016).
Small interfering RNAs are short dsRNAs of 20 to 25 base pairs produced by RNAse III family cutting long dsRNAs. They silence the post-transcription genes by interfering with the expression of specific genes through complementary nucleotides (Jiang et al., 2021; Akhilesh et al., 2022). In mammals, siRNAs only come from synthetic sources (Wang et al., 2021).
Small interfering RNAs participate in various biological mechanisms, including the proliferation, growth and differentiation of cells, as well as the arrangement of heterochromatin in nucleus (Mishra et al., 2022). SiRNA-mediated glycogen synthase kinase (GSK)3β silencing improves the cognitive function and relieves the progression of AD by eliminating the deposition of neurofibrils and amyloid plaques (Gupta et al., 2022). SiRNA restores the normal expression of schwann cells in Charcot-Marie-tooth disease type 1a mice, electrophysiological activity of motor nerves and muscular function. However, long-term treatment produces off-target and toxic side effects (Boutary et al., 2021). Anti-Huntington (HTT) siRNAs effectively reduce the expression of HTT gene and slow the disease’s progression in mice with Huntington’s disease (HD) (Sava et al., 2020).
CAG-siRNA specifically silences the alleles of estrogen receptor with CAG repeats in fibroblasts, inhibits the generation of polyQ protein in the central nervous system to treat the SCA, spinal cord and medullary atrophy, HD, dentatered-globus pallidus atrophy, etc., (Hirunagi et al., 2021). Intravenous siRNAs reduce the Axin-3 expression, alleviate the dyskinesia and improve the striatal and cerebellar lesions in SCA3 mice (Conceição et al., 2016).
Short hairpin RNA consists of two complementary 19–22 bp RNA sequences linked by a short loop consisting of 4–11 nt. It is similar to the hairpin in naturally synthesize miRNA, so it is called the short hairpin RNA (Moore et al., 2010). Although shRNAs are exogenous RNAs (Sheng et al., 2020), they can be assimilated into endogenous RNA pathways with greater efficiency than siRNAs (Gorabi et al., 2020).
Short hairpin RNAs inhibit the expressions of SNCA and endogenous α-synuclein to protect nerves and reduce dyskinesia in the substantia nigra of Parkinson’s disease (PD) rats (Nakamori et al., 2019). shRNAs convert the midbrain astrocytes into dopaminergic neurons to improve the motor dysfunction in PD (Qian et al., 2020). shRNAs target the glutamate N-methyl-d-aspartate receptor subunit (GluN) 3A inhibition to prevent the loss of dendritic spines and improve the locomotor performance in HD mice (Marco et al., 2018). shRNAs carry out spindle pole body component (SPC) 25 to pass through the blood-brain barrier (BBB), reduce the proliferation of microglia, and slow the disease progression in AD mice (Cui et al., 2021). For SCA3 and SCA7, shRNAs reduce the Axin-3 in mutant cells by 60%, and the expressions and aggregations of toxic mutant ataxin-7 proteins. The normal proteins are unchanged and stored in the non-aggregated diffuse cells (Scholefield et al., 2009; Kotowska-Zimmer et al., 2020). shRNAs restore the cerebellar morphology of SCA1 mice and improve the motor coordination of Purkinje cells (Xia et al., 2004).
The currently RNAi drugs only include N-acetylgalactosamine-siRNA conjugates (givosiran, inclisiran, and lumasiran), which targets to hepatocyte and siRNA drugs (vutrisiran and onpattro) that can treat the familial amyloid polyneuropathy (Kulkarni et al., 2021; Holm et al., 2022). Although RNAi shows the different ways of gene silencing in each ND, it seems to be a general treatment for them. However, it suffers from endosome escape, off-target effect, unclear targeting specificity, and short half-life (the half-life is only a few minutes due to rapid degradation by nucleases) (Aigner, 2019; Setten et al., 2019). Conventional RNAi vectors have shortcomings. Adenoviral vectors themselves can be toxic (Keiser et al., 2021). Liposomes have a short half-life due to the hydrolysis and oxidation of phospholipids and are vulnerable to temperature. Composite nanoparticle are cytotoxic which may destroy the genetic material of a body (Hernández-Soto and Chacón-Cerdas, 2021). The aforementioned disadvantages of RNAi make it difficult to treat SCA alone.
Extracellular vesicles have target specificity, long plasma half-life and wide distribution, and can cross the BBB. Their contents such as miRNAs can assist in the diagnosis and prognosis of NDs. Neurotrophic factors (NTFs), heat shock proteins, vascular endothelial growth factors and other factors can restore neuronal activity and maintain protein homeostasis.
Extracellular vesicles are nano-sized vesicles released by various cells and surrounded by phospholipid membranes (Ye et al., 2022). EVs contain abundant DNA, proteins, lipids, mRNA and small non-coding RNA, including miRNAs (account for half of the content of EVs), which are not affected by extracellular proteases and nucleases (Cuomo-Haymour et al., 2022; Zhu et al., 2022). According to the mechanism and diameter of the production, they are divided into exosomes (50–150 nm), microbubbles (100–1,000 nm) and apoptotic bodies (100–5,000 nm). Their functions are related to the originating cells (Mathieu et al., 2019).
As a novel carrier, EVs have high biocompatibility to avoid the adverse immune reactions, targeting specificity, low toxicity, long plasma half-life, source diversity, and can be easy to cross the BBB to overcome the shortcomings of conventional carriers and RNAi (Zhang et al., 2019; O’Brien et al., 2020; Herrmann et al., 2021).
Extracellular vesicles are important in the treatment of NDs (Table 1). Stem cell-derived EVs have neuroprotective and immunomodulatory effects (Niu et al., 2020; Garcia-Contreras and Thakor, 2021). EVs derive from MSCs and anti-inflammatory immune cells that can reduce the inflammatory damage and cell stress (Thome et al., 2022). The exosomes of human amniotic fluid MSCs can treat the inflammation-related neurological diseases by reducing inflammation caused by microglia and improving neurotoxicity (Zavatti et al., 2022). EV-mediated the delivery of DnaJ Homolog Subfamily B Member 6 (DNAJB6) molecular chaperone inhibits the aggregation of polyQ and HTT proteins to delay the onset of HD (Joshi et al., 2021). EVs derive from neural stem cells that target the functional substances to protect damaged neurons by resisting oxidation, apoptosis and inflammation (Lee et al., 2022). Most of the data on the treatment of EVs are concentrated in preclinical trials, but many clinical trials have proved the potential of EVs in diagnosis and monitoring.
Extracellular vesicles can assist in the diagnosis and monitoring of NDs (Table 2). The α-globin, β-globin, δ-globin, α-1-antichymotrypsin, beta-2-glycoprotein 1 and complement component C9 are increased, and apolipoprotein C-III is decreased in the neuron-derived exosomes of AD patients (Arioz et al., 2021; Soares Martins et al., 2022). The number of α-syn-carrying EVs in cerebrospinal fluid (CSF) and plasma of PD patients are significantly increased, which is positively correlated with the severity (Agliardi et al., 2021; Hong et al., 2021). Concentrations of syntaxin-1A (STX-1A) and vesicle associated membrane protein 2 (VAMP-2) are significantly reduced in PD (Agliardi et al., 2021). The concentration of toll-like receptor (TLR) 3 is decreased and TLR4 is increased in EVs from MS patients (Bhargava et al., 2019). The increased expressions of Junctional Adhesion Molecule A (JAM-A), TNF-R2, and Chitinase 1 lead to increase platelet activation, angiogenesis, motor neuron destruction and microglia activation in the EVs of ALS patients (Czubak-Prowizor et al., 2022; Sjoqvist and Otake, 2022). In the clinical trial of exenatide for PD, neuron-derived EVs, as biomarkers, have potential in examining the molecular mechanisms of patients’ motor function changes (Athauda et al., 2019).
Extracellular vesicles, as an important communication tool, can transmit the pathogenic gene, misfold, and aggregate proteins between cells, thus participating in the pathophysiology of NDs (Surgucheva et al., 2012). Other propagation mechanisms include soluble oligomers, synaptic connections, and nano tunnel tube (Takeuchi and Nagai, 2022; Figure 1). As EVs contain miRNAs, proteins and other components, their changes can reflect the condition of SCA (Cuomo-Haymour et al., 2022). There are few studies in the biomarkers of EVs in SCA compared to AD, PD, and ALS. Only one study of miRNA in the EVs is described below. None of the studies about protein biomarkers has demonstrated that they are in EVs. Both neurofilament medium (NFL) and myelin basic protein levels decrease in the cerebellar cortex of SCA3 patients, indicating axonal damage and myelin formation disorders (Paul et al., 2018). Increased levels of staufen 1 are associated with the abnormal metabolism of RNA due to ATXN2 mutations in the brain tissue of SCA2 patients (Yang et al., 2021). The traditional diagnostic methods for SCA include three aspects: (1) MRI can only show the pathological changes of extensive atrophy of the brain and thinning of the cortex, which lacks the functional changes corresponding to the pathological changes. Meanwhile, MRI is very expensive (Yap et al., 2022a). (2) The diagnosis of symptomatology is easily affected by subjectivity. (3) Genetic examination: The gene loci of mutations in different subtypes are different. For example, SCA1, SCA2, SCA3, SCA6, SCA7, SCA12, and SCA19/22 are, respectively, related to the CAG repeated amplification in ATXN1, ATXN2, ATXN3, CACNA1A, ATX7, PPP2R2B, and potassium voltage gated channel, Shal-related family, member 3 (KCND3) genes on different chromosomes (Li M. et al., 2022; Lian et al., 2022).
Figure 1. Transmission mechanism of polyQ protein. a. Error folding and aggregation of polyQ encoded protein. A. Extracellular vesicle pathway. B. Nano tunnel tube approach: the polyQ protein is exchanged by a tunnel-like membrane-derived structure that connects two cells. C. Soluble oligomer: due to its high solubility and small size, PolyQ protein may be able to escape from cells and diffuse to other cells. D. Synaptic connection: the polyQ protein is transmitted between neurons with high transmission efficiency.
Extracellular vesicles can transmit NTFs, heat shock proteins, and others to promote the damaged neurons and maintain the stability and activity of proteins (Backe et al., 2022; Harrell et al., 2022; Van den Broek et al., 2022). The proteins encoded by polyQ are the key to the pathogenesis of SCA, and Heat shock protein (HSP) 70 can promote the synthesis of correctly folded proteins and the degradation of misfolded proteins, prevent the aggregation of abnormal proteins and disaggregate the aggregation of abnormal proteins (Johnson et al., 2020; Serlidaki et al., 2020). MSCs-derived exosomes reduce the loss of purkinje cells and cerebellar myelin sheaths, alleviate neuroinflammation and improve the locomotor function in SCA3 mice [19] (You et al., 2020). MSC-conditioned medium (simulates EVs) significantly reduces the motor disharmony and neuronal degeneration in SCA1 mice, due to the inclusion of hepatocyte growth factor, fibroblast growth factor-2, insulin-like growth factor-1 and vascular endothelial growth factor (VEGF) (Suto et al., 2016). Studies have shown that VEGF can reduce ataxia and dendritic defects and even reverse cerebellar lesions in SCA1 (Hu et al., 2019).
Extracellular vesicles also face some challenges, which have been nowly solved. Endogenous EVs competing for binding sites and the clearance of macrophages can be solved by specifically modifying the surface of EVs (Zhang et al., 2022). EVs from the different sources have the different effects. Novel micro-nano technology tool can sensitively separate the different subsets of EVs (Chiang and Chen, 2019; Wang et al., 2020). Ultra-centrifugation, ultrafiltration, precipitation and size exclusion chromatography improve the separation and purification of EVs (Li M. et al., 2020). Membrane extrusion technology combine the surface composition of EVs with lipid materials to achieve mass production of EVs (Jhan et al., 2020).
Extracellular vesicles not only can treat SCA, but also can be carriers of RNAi, which can make up for the deficiencies of RNAi. Treating SCA with the combination of EVs and RNAi is a promising direction. The miRNAs and proteins contained in EVs will undergo corresponding significant changes, making them biomarkers to assist the diagnosis and monitoring of SCA.
Several cells can self-secrete miRNAs through paracrine and transport them to the receptor cells, and then change the expression of target genes, such as macrophages, microglia, oligodendrocytes, astrocytes, schwann cells and so on (Loch-Neckel et al., 2022; Zhu et al., 2022). Astrocyte-derived exosomes carrying miR-17-5p reduce oxidative stress, inflammatory, cerebral infarction and neurological disorders in hypoxic-ischemic brain injured rats (Du et al., 2021). Microglia secretes the exosomes containing miR-190b to inhibit neuronal apoptosis by targeting autophagy-related protein 7 to regulate autophagy (Pei et al., 2020). M2 microglia secretes the exosomes containing miR-124 to target ubiquitin-specific protease 14 to promote neuronal survival and mitigate ischemic brain injury (Song et al., 2019). Fibroblasts secrete the exosomes containing miR-673-5p to stimulate myelin gene expression by activating the tuberous sclerosis complex 2/mTOR complex 1/sterol-regulatory element binding protein 2 axis in Schwann cells and peripheral myelin formation (Zhao et al., 2022b). It is infeasible to implant them directly to patients. On the one hand, they may contain pathogenic substances. The transmission of astrocyte-derived EVs carrying casein kinase 1 increases the formation of Aβ Aand promotes inflammatory response by targeting the related neurons, which is the pathogenesis of AD (Li Z. et al., 2020). EVs can spread α-synuclein in the brain and accelerate the progress of PD [126] (Longoni et al., 2019). On the other hand, as there are 48 subtypes of SCA having the different genetic changes which require the different types of RNAi (Chen H. Y. et al., 2021; Shin et al., 2021). The composition and function of EVs are related to its secretory cells and their environments. The MSC-derived EVs in an inflammatory environment reduce inflammatory response more than the normal MSC-derived EVs (Mathieu et al., 2019; Liu Y. et al., 2022). Hypoxia-treated exosomes improve the inflammatory responses and synaptic functions by increasing the production of miR-21 to improve the cognitive and memory disorders (Cui et al., 2018). Therefore, we should select the metrocyte that can alleviate nerve damage and movement disorder, and appropriately stimulate the metrocyte to maximize the therapeutic value of EVs derived from it.
The desired RNAi can be loaded into the isolated and purified EVs. Electroporation is the most common and optimal technology. Each EV is probably loaded with 3,000 miRNA molecules (Batrakova and Kim, 2015; Pomatto et al., 2022). Incubation is a simple method while maintaining the integrity and stability of the EVs. It is achieved by incubating EVs with miRNA in Roswell Park Memorial Institute (RPMI) medium at 37°C for 1 h (Tapparo et al., 2021). Ultrasound damages the integrity of EVs membranes, in which drugs, protein and nanoparticles are loaded, and then membranes are recovered by incubation (Li Y. J. et al., 2020). Under the cross substitution of heat shock and freezing, CaCl2 mediates the loading of miRNA into the exosomes without losing activity and with high efficiency (Rezaei et al., 2021). Although the method of encapsulating RNAi in EVs has not been tested in SCA, many studies have proved the feasibility in NDs, autoimmune diseases, tumors, cardiovascular diseases, etc. The EVs-derived from human neural stem cells attenuate the neuroinflammation and ROS-induced apoptosis, and promote the survival of dopaminergic neurons in PD, which may be related to the specific miRNA-embedded in EVs (Lee et al., 2022). Increasing the paracrine miR-146a of cortical astrocytes eliminate their phenotypic abnormalities through pre-miR-146a or dipeptidyl vinyl sulfone and play a neuro-glial repair role in ALS (Barbosa et al., 2021). Exosomes-loaded with miRNA-22 improve AD by inhibiting the pyroptosis and neuroinflammation (Zhai et al., 2021). The miRNA-encapsulated in exosomes control the target genes (nuclear factor kappa-B (NF-κB), signal transducer and activator of transcription (STAT) 1, and phosphatidylinositol 3 kinase/protein kinase B (PI3K/Akt), etc.) in the regulatory signaling pathway in immune response to treat the autoimmune diseases (Matsuzaka and Yashiro, 2022). Exosomes-loaded with miR-145-5p reduce the growth of pancreatic ductal adenocarcinoma (PDAC) by inhibiting the proliferation and invasion of PDAC cells (Ding et al., 2019). Exosomes carrying mir-6785-5P inhibit the angiogenesis and metastasis by inhibiting the inhibin subunit beta A in gastric cancer (Chen Z. et al., 2021). Cardiac progenitor cell-derived exosomes containing miR-146, miR-210, and miR-132 reduce the infarct size and improve the cardiac functions in infarcted hearts (Cervio et al., 2015; Figure 2).
Figure 2. Mechanism of the binding of EVs and miRNA in the treatment of SCA3. (A) Appropriately stimulate the specific metrocyte (such as hypoxia treatment) to maximize the therapeutic value of EVs derived from it. (B) Load the desired miRNA into the isolated and purified EVs through electroporation, incubation or ultrasound, etc. (C) EVs with specific miRNA acts on corresponding miRNA sites (there are 1,137 known sites presently) in the 3’UTR of mutant ATXN3 mRNA. (D) The mutated ATXN3 mRNA that binds to the miRNA in the 3’UTR region is degraded or its translation is inhibited. (E) Misfolding and aggregation of the mutated ATXN3 protein occur. Transcription factors are adsorbed by protein aggregates, interfering with the expression and biogenesis of normal miRNA. Eventually the neurons degenerate.
The onset and progression are vary significantly among SCA patients. Biomarkers can sensitively monitor the SCA progression that is needed (Borgonio-Cuadra et al., 2019). Conventional diagnostic methods for SCA have shortcomings and cannot accurately monitor the disease progression. Existing measurements of protein biomarkers such as NfL in CSF and blood fail to locate the site of injury (Yap et al., 2022a). Ataxin-3 produced by polyQ amplification in plasma is highly stable only for 1 year (Hübener-Schmid et al., 2021). The protein biomarkers in EVs may specifically concentrate at the injured site and remain stable for longer time unaffected by proteolytic enzymes.
The miRNA in exosomes directly reflect the physiological conditions of brain, which are highly expressed in brain and body fluids, such as serum, plasma and CSF (Mori et al., 2019). Furthermore, a DNA-assembled advanced plasmonic architecture-based plasmonic biosensor is sensitive and accurate enough to detect miRNA in exosomes for clinical applications (Song et al., 2022). The miRNAs in the SCA patients undergo the specific changes with low stability. Loading miRNA in EVs may protect them from nuclease-rich environments (Borgonio-Cuadra et al., 2019). The miR-7014 in EVs of SCA3 patients is downregulated in plasma and upregulated in CSF (Hou et al., 2019). Although there is only one case of RNAi in EVs as SCA biomarkers, its feasibility has been confirmed in cancer, autoimmune diseases, kidney diseases, and NDs. miR-320a and miR-4433b-5p in EVs can differentiate breast cancer from the control population (Carvalho et al., 2022). Blood-derived EVs show that the level of miRNA-146a is reduced in the systemic lupus erythematosus patients, as oppose to urine-derived EVs (Zhou et al., 2022). Renal function is negatively correlated with the levels of miRNA-12136 and miRNA-483-5p (Sun et al., 2022). Expression of miR-16-5p, -331-3p, -409-3p, and -454-3p increase 1.5-fold in the CSF-derived EVs in AD patients (Sandau et al., 2022). The combination of miR-125b and -361 in exosomes can distinguish AD patients from healthy control with an accuracy rate of 99.52% (Song et al., 2022). MS patients show the upregulation of miR-21-5p and the downregulation of miR-6735-3p, miR-6833-5p, and miR-510-3p in the EVs (Cuomo-Haymour et al., 2022). Hsa-miR-374a-5, -374b-5p,-199a-3p, -28-5p, -22-5p, and -151a-5p are potential biomarkers for the diagnosis of PD at different stages (He et al., 2021). Therefore, RNAi in EVs can assist in the diagnosis and monitoring of NDs.
There are still some limitations in the research of RNAi biomarkers in EVs. Most studies on biomarkers are conducted in SCA3 because it is the most common hypotype. Most RNAi studies have focused on miRNA because it accounts for half of the EVs content. Since SCA is a rare disease, the sample size of cases is insufficient, leading to the possibility of biased results. EVs and RNAi are affected by many factors in vitro and in vivo, so currently they can only serve as reference indicators for the diagnosis and monitoring of SCA.
At present, symptomatic treatment is the only clinical treatment for SCA that has many side effects and limited efficacy. Gene therapy is the most popular potential treatment for SCA, and RNAi is the most effective among it. Many studies have shown that EVs and RNAi work well in SCA cells, animal models, and patients. In particular, the combination of them can make up for their deficiencies. The therapeutic effect, target specificity, stability and plasma half-life of EVs containing RNAi secreted by cells or EVs loaded with specific RNAi are significantly improved. They also have potential in the diagnosis and condition monitoring of SCA. Changes of RNAi in patients’ EVs may reflect disease progression. However, there are few studies on the combination treatment, diagnosis and monitoring for SCA. In addition, most of them are animal and cell experiments, which do not eliminate the influence of sample size, gender, age, and race of the experimental subjects. Most studies have focused on SCA3 and miRNA while ignoring other subtypes of SCA and RNAi. Therefore, the combination of RNAi and EVs is a new therapeutic way for SCA, further in-depth clinical studies on large-scale multiple subtypes of RNAi and EVs are needed.
XL and YD designed the study. YD, YZ, and XL prepared the first draft of the manuscript and revised the manuscript. All authors agreed to publish this article and approved the final manuscript.
The authors declare that the research was conducted in the absence of any commercial or financial relationships that could be construed as a potential conflict of interest.
All claims expressed in this article are solely those of the authors and do not necessarily represent those of their affiliated organizations, or those of the publisher, the editors and the reviewers. Any product that may be evaluated in this article, or claim that may be made by its manufacturer, is not guaranteed or endorsed by the publisher.
Agliardi, C., Meloni, M., Guerini, F. R., Zanzottera, M., Bolognesi, E., Baglio, F., et al. (2021). Oligomeric α-Syn and SNARE complex proteins in peripheral extracellular vesicles of neural origin are biomarkers for Parkinson’s disease. Neurobiol. Dis. 148:105185. doi: 10.1016/j.nbd.2020.105185
Aigner, A. (2019). Perspectives, issues and solutions in RNAi therapy: The expected and the less expected. Nanomedicine (Lond) 14, 2777–2782. doi: 10.2217/nnm-2019-0321
Akhilesh, Uniyal, A., Gadepalli, A., Tiwari, V., Allani, M., Chouhan, D., et al. (2022). Unlocking the potential of TRPV1 based siRNA therapeutics for the treatment of chemotherapy-induced neuropathic pain. Life Sci. 288:120187. doi: 10.1016/j.lfs.2021.120187
Arioz, B. I., Tufekci, K. U., Olcum, M., Durur, D. Y., Akarlar, B. A., Ozlu, N., et al. (2021). Proteome profiling of neuron-derived exosomes in Alzheimer’s disease reveals hemoglobin as a potential biomarker. Neurosci. Lett. 755:135914. doi: 10.1016/j.neulet.2021.135914
Athauda, D., Gulyani, S., Karnati, H. K., Li, Y., Tweedie, D., Mustapic, M., et al. (2019). Utility of neuronal-derived exosomes to examine molecular mechanisms that affect motor function in patients with Parkinson disease: A secondary analysis of the Exenatide-PD trial. JAMA Neurol. 76, 420–429. doi: 10.1001/jamaneurol.2018.4304
Backe, S. J., Sager, R. A., Regan, B. R., Sit, J., Major, L. A., Bratslavsky, G., et al. (2022). A specialized Hsp90 co-chaperone network regulates steroid hormone receptor response to ligand. Cell Rep. 40:111039. doi: 10.1016/j.celrep.2022.111039
Barbosa, M., Gomes, C., Sequeira, C., Gonçalves-Ribeiro, J., Pina, C. C., Carvalho, L. A., et al. (2021). Recovery of depleted miR-146a in ALS cortical astrocytes reverts cell aberrancies and prevents paracrine pathogenicity on microglia and motor neurons. Front. Cell Dev. Biol. 9:634355. doi: 10.3389/fcell.2021.634355
Batrakova, E. V., and Kim, M. S. (2015). Using exosomes, naturally-equipped nanocarriers, for drug delivery. J. Control Release 219, 396–405. doi: 10.1016/j.jconrel.2015.07.030
Bhargava, P., Nogueras-Ortiz, C., Chawla, S., Bæk, R., Jørgensen, M. M., and Kapogiannis, D. (2019). Altered levels of toll-like receptors in circulating extracellular vesicles in multiple sclerosis. Cells 8:1058. doi: 10.3390/cells8091058
Blommer, J., Pitcher, T., Mustapic, M., Eren, E., Yao, P. J., Vreones, M. P., et al. (2022). Extracellular vesicle biomarkers for cognitive impairment in Parkinson’s disease. Brain awac258. doi: 10.1093/brain/awac258
Bonafede, R., Brandi, J., Manfredi, M., Scambi, I., Schiaffino, L., Merigo, F., et al. (2019). The anti-apoptotic effect of ASC-Exosomes in an in vitro ALS model and their proteomic analysis. Cells 8:1087. doi: 10.3390/cells8091087
Bonafede, R., Turano, E., Scambi, I., Busato, A., Bontempi, P., Virla, F., et al. (2020). Exosomes ameliorate the disease progression in SOD1(G93A) murine model underlining their potential therapeutic use in human ALS. Int. J. Mol. Sci. 21:3651. doi: 10.3390/ijms21103651
Borgonio-Cuadra, V. M., Valdez-Vargas, C., Romero-Córdoba, S., Hidalgo-Miranda, A., Tapia-Guerrero, Y., Cerecedo-Zapata, C. M., et al. (2019). Wide profiling of circulating MicroRNAs in spinocerebellar ataxia type 7. Mol. Neurobiol. 56, 6106–6120. doi: 10.1007/s12035-019-1480-y
Boutary, S., Caillaud, M., El Madani, M., Vallat, J. M., Loisel-Duwattez, J., Rouyer, A., et al. (2021). Squalenoyl siRNA PMP22 nanoparticles are effective in treating mouse models of Charcot-Marie-Tooth disease type 1 A. Commun. Biol. 4:317. doi: 10.1038/s42003-021-01839-2
Carmona, V., Cunha-Santos, J., Onofre, I., Simões, A. T., Vijayakumar, U., Davidson, B. L., et al. (2017). Unravelling endogenous MicroRNA system dysfunction as a new pathophysiological mechanism in machado-joseph disease. Mol. Ther. 25, 1038–1055. doi: 10.1016/j.ymthe.2017.01.021
Carvalho, T. M., Brasil, G. O., Jucoski, T. S., Adamoski, D., de Lima, R. S., Spautz, C. C., et al. (2022). MicroRNAs miR-142-5p, miR-150-5p, miR-320a-3p, and miR-4433b-5p in serum and tissue: Potential biomarkers in sporadic breast cancer. Front. Genet. 13:865472. doi: 10.3389/fgene.2022.865472
Cendelin, J., Cvetanovic, M., Gandelman, M., Hirai, H., Orr, H. T., Pulst, S. M., et al. (2022). Consensus paper: Strengths and weaknesses of animal models of spinocerebellar ataxias and their clinical implications. Cerebellum 21, 452–481. doi: 10.1007/s12311-021-01311-1
Cervio, E., Barile, L., Moccetti, T., and Vassalli, G. (2015). Exosomes for intramyocardial intercellular communication. Stem Cells Int. 2015:482171. doi: 10.1155/2015/482171
Chen, H. Y., Hsu, C. L., Lin, H. Y., Lin, Y. F., Tsai, S. F., Ho, Y. J., et al. (2021). Clinical and functional characterization of a novel STUB1 frameshift mutation in autosomal dominant spinocerebellar ataxia type 48 (SCA48). J. Biomed. Sci. 28:65. doi: 10.1186/s12929-021-00763-1
Chen, W., Zheng, P., Hong, T., Wang, Y., Liu, N., He, B., et al. (2020). Astrocytes-derived exosomes induce neuronal recovery after traumatic brain injury via delivering gap junction alpha 1-20 k. J. Tissue Eng. Regen. Med. 14, 412–423. doi: 10.1002/term.3002
Chen, Z., Xie, Y., Chen, W., Li, T., Chen, X., and Liu, B. (2021). microRNA-6785-5p-loaded human umbilical cord mesenchymal stem cells-derived exosomes suppress angiogenesis and metastasis in gastric cancer via INHBA. Life Sci. 284:119222. doi: 10.1016/j.lfs.2021.119222
Chiang, C. Y., and Chen, C. (2019). Toward characterizing extracellular vesicles at a single-particle level. J. Biomed. Sci. 26:9. doi: 10.1186/s12929-019-0502-4
Chung, C. C., Chan, L., Chen, J. H., Bamodu, O. A., and Hong, C. T. (2020). Neurofilament light chain level in plasma extracellular vesicles and Parkinson’s disease. Ther. Adv. Neurol. Disord. 13:1756286420975917. doi: 10.1177/1756286420975917
Chu-Tan, J. A., Cioanca, A. V., Feng, Z. P., Wooff, Y., Schumann, U., Aggio-Bruce, R., et al. (2021). Functional microRNA targetome undergoes degeneration-induced shift in the retina. Mol Neurodegener. 16:60. doi: 10.1186/s13024-021-00478-9
Conceição, M., Mendonça, L., Nóbrega, C., Gomes, C., Costa, P., Hirai, H., et al. (2016). Intravenous administration of brain-targeted stable nucleic acid lipid particles alleviates Machado-Joseph disease neurological phenotype. Biomaterials 82, 124–137. doi: 10.1016/j.biomaterials.2015.12.021
Costa, M. D. C. (2020). Recent therapeutic prospects for Machado-Joseph disease. Curr. Opin. Neurol. 33, 519–526. doi: 10.1097/WCO.0000000000000832
Costa, M. D. C., Radzwion, M., McLoughlin, H. S., Ashraf, N. S., Fischer, S., Shakkottai, V. G., et al. (2020). In vivo molecular signatures of cerebellar pathology in spinocerebellar ataxia type 3. Mov. Disord. 35, 1774–1786. doi: 10.1002/mds.28140
Cui, F., Xu, Z., Lv, Y., and Hu, J. (2021). Role of spindle pole body component 25 in neurodegeneration. Ann. Transl. Med. 9:1432. doi: 10.21037/atm-21-4064
Cui, G. H., Wu, J., Mou, F. F., Xie, W. H., Wang, F. B., Wang, Q. L., et al. (2018). Exosomes derived from hypoxia-preconditioned mesenchymal stromal cells ameliorate cognitive decline by rescuing synaptic dysfunction and regulating inflammatory responses in APP/PS1 mice. FASEB J. 32, 654–668. doi: 10.1096/fj.201700600R
Cuomo-Haymour, N., Bergamini, G., Russo, G., Kulic, L., Knuesel, I., Martin, R., et al. (2022). Differential expression of serum extracellular vesicle miRNAs in multiple sclerosis: Disease-Stage specificity and relevance to pathophysiology. Int. J. Mol. Sci. 23:1664. doi: 10.3390/ijms23031664
Czubak-Prowizor, K., Babinska, A., and Swiatkowska, M. (2022). The F11 Receptor (F11R)/Junctional Adhesion Molecule-A (JAM-A) (F11R/JAM-A) in cancer progression. Mol. Cell Biochem. 477, 79–98. doi: 10.1007/s11010-021-04259-2
Ding, Y., Cao, F., Sun, H., Wang, Y., Liu, S., Wu, Y., et al. (2019). Exosomes derived from human umbilical cord mesenchymal stromal cells deliver exogenous miR-145-5p to inhibit pancreatic ductal adenocarcinoma progression. Cancer Lett. 442, 351–361. doi: 10.1016/j.canlet.2018.10.039
Dobricic, V., Schilling, M., Schulz, J., Zhu, L. S., Zhou, C. W., Fuß, J., et al. (2022). Differential microRNA expression analyses across two brain regions in Alzheimer’s disease. Transl. Psychiatry 12:352. doi: 10.1038/s41398-022-02108-4
Du, L., Jiang, Y., and Sun, Y. (2021). Astrocyte-derived exosomes carry microRNA-17-5p to protect neonatal rats from hypoxic-ischemic brain damage via inhibiting BNIP-2 expression. Neurotoxicology 83, 28–39. doi: 10.1016/j.neuro.2020.12.006
Garcia-Contreras, M., and Thakor, A. S. (2021). Human adipose tissue-derived mesenchymal stem cells and their extracellular vesicles modulate lipopolysaccharide activated human microglia. Cell Death Discov. 7:98. doi: 10.1038/s41420-021-00471-7
Ghanekar, S. D., Kuo, S. H., Staffetti, J. S., and Zesiewicz, T. A. (2022). Current and emerging treatment modalities for spinocerebellar ataxias. Expert Rev. Neurother. 22, 101–114. doi: 10.1080/14737175.2022.2029703
Gómez, R., Tapia-Guerrero, Y. S., Cisneros, B., Orozco, L., Cerecedo-Zapata, C., Mendoza-Caamal, E., et al. (2022). Genetic distribution of five spinocerebellar ataxia microsatellite loci in mexican native american populations and its impact on contemporary mestizo populations. Genes (Basel) 13:157. doi: 10.3390/genes13010157
Gorabi, A. M., Kiaie, N., Aslani, S., Jamialahmadi, T., Johnston, T. P., and Sahebkar, A. (2020). Prospects for the potential of RNA interference in the treatment of autoimmune diseases: Small interfering RNAs in the spotlight. J Autoimmun. 114:102529. doi: 10.1016/j.jaut.2020.102529
Gupta, S., Singh, V., Ganesh, S., Singhal, N. K., and Sandhir, R. (2022). siRNA Mediated GSK3β knockdown targets insulin signaling pathway and rescues Alzheimer’s disease pathology: Evidence from in vitro and in vivo studies. ACS Appl. Mater. Interfaces 14, 69–93. doi: 10.1021/acsami.1c15305
Harrell, C. R., Volarevic, V., Djonov, V., and Volarevic, A. (2022). Therapeutic potential of exosomes derived from adipose tissue-sourced mesenchymal stem cells in the treatment of neural and retinal diseases. Int. J. Mol. Sci. 23:4487. doi: 10.3390/ijms23094487
He, S., Huang, L., Shao, C., Nie, T., Xia, L., Cui, B., et al. (2021). Several miRNAs derived from serum extracellular vesicles are potential biomarkers for early diagnosis and progression of Parkinson’s disease. Transl. Neurodegener. 10:25. doi: 10.1186/s40035-021-00249-y
Hernández-Soto, A., and Chacón-Cerdas, R. (2021). RNAi crop protection advances. Int. J. Mol. Sci. 22:12148. doi: 10.3390/ijms222212148
Herrmann, I. K., Wood, M. J. A., and Fuhrmann, G. (2021). Extracellular vesicles as a next-generation drug delivery platform. Nat. Nanotechnol. 16, 748–759. doi: 10.1038/s41565-021-00931-2
Hirunagi, T., Sahashi, K., Tachikawa, K., Leu, A. I., Nguyen, M., Mukthavaram, R., et al. (2021). Selective suppression of polyglutamine-expanded protein by lipid nanoparticle-delivered siRNA targeting CAG expansions in the mouse CNS. Mol. Ther. Nucleic Acids 24, 1–10. doi: 10.1016/j.omtn.2021.02.007
Holm, A., Hansen, S. N., Klitgaard, H., and Kauppinen, S. (2022). Clinical advances of RNA therapeutics for treatment of neurological and neuromuscular diseases. RNA Biol. 19, 594–608. doi: 10.1080/15476286.2022.2066334
Hong, Z., Tian, C., Stewart, T., Aro, P., Soltys, D., Bercow, M., et al. (2021). Development of a sensitive diagnostic assay for parkinson disease quantifying α-synuclein-containing extracellular vesicles. Neurology 96, e2332–e2345. doi: 10.1212/WNL.0000000000011853
Hou, X., Gong, X., Zhang, L., Li, T., Yuan, H., Xie, Y., et al. (2019). Identification of a potential exosomal biomarker in spinocerebellar ataxia Type 3/Machado-Joseph disease. Epigenomics 11, 1037–1056. doi: 10.2217/epi-2019-0081
Hu, Y. S., Do, J., Edamakanti, C. R., Kini, A. R., Martina, M., Stupp, S. I., et al. (2019). Self-assembling vascular endothelial growth factor nanoparticles improve function in spinocerebellar ataxia type 1. Brain 142, 312–321. doi: 10.1093/brain/awy328
Huang, Y., Li, R., Ye, S., Lin, S., Yin, G., and Xie, Q. (2020). Recent advances in the use of exosomes in Sjögren’s syndrome. Front. Immunol. 11:1509. doi: 10.3389/fimmu.2020.01509
Hübener-Schmid, J., Kuhlbrodt, K., Peladan, J., Faber, J., Santana, M. M., Hengel, H., et al. (2021). Polyglutamine-Expanded Ataxin-3: A target engagement marker for spinocerebellar ataxia Type 3 in peripheral blood. Mov. Disord. 36, 2675–2681. doi: 10.1002/mds.28749
Jalles, A., Vieira, C., Pereira-Sousa, J., Vilasboas-Campos, D., Mota, A. F., Vasconcelos, S., et al. (2022). Aripiprazole offsets mutant ATXN3-Induced motor dysfunction by targeting dopamine D2 and serotonin 1A and 2A receptors in C. elegans. Biomedicines 10:370. doi: 10.3390/biomedicines10020370
Jhan, Y. Y., Prasca-Chamorro, D., Palou Zuniga, G., Moore, D. M., Arun Kumar, S., Gaharwar, A. K., et al. (2020). Engineered extracellular vesicles with synthetic lipids via membrane fusion to establish efficient gene delivery. Int. J. Pharm. 573:118802. doi: 10.1016/j.ijpharm.2019.118802
Jiang, Q., Yue, S., Yu, K., Tian, T., Zhang, J., Chu, H., et al. (2021). Endogenous microRNA triggered enzyme-free DNA logic self-assembly for amplified bioimaging and enhanced gene therapy via in situ generation of siRNAs. J. Nanobiotechnol. 19:288. doi: 10.1186/s12951-021-01040-x
Johnson, S. L., Ranxhi, B., Libohova, K., Tsou, W. L., and Todi, S. V. (2020). Ubiquitin-interacting motifs of ataxin-3 regulate its polyglutamine toxicity through Hsc70-4-dependent aggregation. Elife 9:e60742. doi: 10.7554/eLife.60742
Joshi, B. S., Youssef, S. A., Bron, R., de Bruin, A., Kampinga, H. H., and Zuhorn, I. S. (2021). DNAJB6b-enriched small extracellular vesicles decrease polyglutamine aggregation in in vitro and in vivo models of Huntington disease. iScience 24:103282. doi: 10.1016/j.isci.2021.103282
Kara, G., Calin, G. A., and Ozpolat, B. (2022). RNAi-based therapeutics and tumor targeted delivery in cancer. Adv. Drug Deliv. Rev. 182:114113. doi: 10.1016/j.addr.2022.114113
Kasai, T., Kojima, Y., Ohmichi, T., Tatebe, H., Tsuji, Y., Noto, Y. I., et al. (2019). Combined use of CSF NfL and CSF TDP-43 improves diagnostic performance in ALS. Ann. Clin. Transl. Neurol. 6, 2489–2502. doi: 10.1002/acn3.50943
Keiser, M. S., Monteys, A. M., Corbau, R., Gonzalez-Alegre, P., and Davidson, B. L. (2016). RNAi prevents and reverses phenotypes induced by mutant human ataxin-1. Ann. Neurol. 80, 754–765. doi: 10.1002/ana.24789
Keiser, M. S., Ranum, P. T., Yrigollen, C. M., Carrell, E. M., Smith, G. R., Muehlmatt, A. L., et al. (2021). Toxicity after AAV delivery of RNAi expression constructs into nonhuman primate brain. Nat. Med. 27, 1982–1989. doi: 10.1038/s41591-021-01522-3
Khan, I., Preeti, K., Fernandes, V., Khatri, D. K., and Singh, S. B. (2022). Role of MicroRNAs, aptamers in neuroinflammation and neurodegenerative disorders. Cell Mol. Neurobiol. 42, 2075–2095. doi: 10.1007/s10571-021-01093-4
Kotowska-Zimmer, A., Ostrovska, Y., and Olejniczak, M. (2020). Universal RNAi triggers for the specific inhibition of mutant huntingtin, Atrophin-1, Ataxin-3, and Ataxin-7 expression. Mol. Ther. Nucleic Acids 19, 562–571. doi: 10.1016/j.omtn.2019.12.012
Krauss, S., Nalavade, R., Weber, S., Carter, K., and Evert, B. O. (2019). Upregulation of miR-25 and miR-181 family members correlates with reduced expression of ATXN3 in lymphocytes from SCA3 patients. Microrna 8, 76–85. doi: 10.2174/2211536607666180821162403
Kulkarni, J. A., Witzigmann, D., Thomson, S. B., Chen, S., Leavitt, B. R., Cullis, P. R., et al. (2021). The current landscape of nucleic acid therapeutics. Nat. Nanotechnol. 16, 630–643. doi: 10.1038/s41565-021-00898-0
Lee, E. J., Choi, Y., Lee, H. J., Hwang, D. W., and Lee, D. S. (2022). Human neural stem cell-derived extracellular vesicles protect against Parkinson’s disease pathologies. J. Nanobiotechnol. 20:198. doi: 10.1186/s12951-022-01356-2
Lee, M., Im, W., and Kim, M. (2021). Exosomes as a potential messenger unit during heterochronic parabiosis for amelioration of Huntington’s disease. Neurobiol. Dis. 155:105374. doi: 10.1016/j.nbd.2021.105374
Li, M., Liu, F., Hao, X., Fan, Y., Li, J., Hu, Z., et al. (2022). Rare KCND3 Loss-of-Function mutation associated with the SCA19/22. Front. Mol. Neurosci. 15:919199. doi: 10.3389/fnmol.2022.919199
Li, M., Zhou, H., Yang, C., Wu, Y., Zhou, X., Liu, H., et al. (2020). Bacterial outer membrane vesicles as a platform for biomedical applications: An update. J. Control Release 323, 253–268. doi: 10.1016/j.jconrel.2020.04.031
Li, T. R., Yao, Y. X., Jiang, X. Y., Dong, Q. Y., Yu, X. F., Wang, T., et al. (2022). β-Amyloid in blood neuronal-derived extracellular vesicles is elevated in cognitively normal adults at risk of Alzheimer’s disease and predicts cerebral amyloidosis. Alzheimers Res. Ther. 14:66. doi: 10.1186/s13195-022-01010-x
Li, Y. J., Wu, J. Y., Wang, J. M., Hu, X. B., Cai, J. X., and Xiang, D. X. (2020). Gemcitabine loaded autologous exosomes for effective and safe chemotherapy of pancreatic cancer. Acta Biomater. 101, 519–530. doi: 10.1016/j.actbio.2019.10.022
Li, Z., Moniruzzaman, M., Dastgheyb, R. M., Yoo, S. W., Wang, M., Hao, H., et al. (2020). Astrocytes deliver CK1 to neurons via extracellular vesicles in response to inflammation promoting the translation and amyloidogenic processing of APP. J. Extracell. Vesicles 10:e12035. doi: 10.1002/jev2.12035
Lian, M., Limwongse, C., Yoon, C. S., Lee, C. G., Law, H. Y., and Chong, S. S. (2022). Single-Tube screen for rapid detection of repeat expansions in seven common spinocerebellar ataxias. Clin. Chem. 68, 794–802. doi: 10.1093/clinchem/hvac011
Lin, Z., Gu, Y., Zhou, R., Wang, M., Guo, Y., Chen, Y., et al. (2020). Serum exosomal proteins F9 and TSP-1 as potential diagnostic biomarkers for newly diagnosed epilepsy. Front. Neurosci. 14:737. doi: 10.3389/fnins.2020.00737
Liu, S., Fan, M., Xu, J. X., Yang, L. J., Qi, C. C., Xia, Q. R., et al. (2022). Exosomes derived from bone-marrow mesenchymal stem cells alleviate cognitive decline in AD-like mice by improving BDNF-related neuropathology. J. Neuroinflammation 19:35. doi: 10.1186/s12974-022-02393-2
Liu, Y., Zhang, Z., Wang, B., Dong, Y., Zhao, C., Zhao, Y., et al. (2022). Inflammation-Stimulated MSC-Derived small extracellular vesicle miR-27b-3p regulates macrophages by targeting CSF-1 to promote temporomandibular joint condylar regeneration. Small 18:e2107354. doi: 10.1002/smll.202107354
Loch-Neckel, G., Matos, A. T., Vaz, A. R., and Brites, D. (2022). Challenges in the development of drug delivery systems based on small extracellular vesicles for therapy of brain diseases. Front. Pharmacol. 13:839790. doi: 10.3389/fphar.2022.839790
Long, Q., Upadhya, D., Hattiangady, B., Kim, D. K., An, S. Y., Shuai, B., et al. (2017). Intranasal MSC-derived A1-exosomes ease inflammation, and prevent abnormal neurogenesis and memory dysfunction after status epilepticus. Proc. Natl. Acad. Sci. U.S.A. 114, E3536–E3545. doi: 10.1073/pnas.1703920114
Longoni, B., Fasciani, I., Kolachalam, S., Pietrantoni, I., Marampon, F., Petragnano, F., et al. (2019). Neurotoxic and neuroprotective role of exosomes in Parkinson’s disease. Curr. Pharm. Des. 25, 4510–4522. doi: 10.2174/1381612825666191113103537
Magen, I., Yacovzada, N. S., Yanowski, E., Coenen-Stass, A., Grosskreutz, J., Lu, C. H., et al. (2021). Circulating miR-181 is a prognostic biomarker for amyotrophic lateral sclerosis. Nat. Neurosci. 24, 1534–1541. doi: 10.1038/s41593-021-00936-z
Marco, S., Murillo, A., and Pérez-Otaño, I. (2018). RNAi-Based GluN3A silencing prevents and reverses disease phenotypes induced by mutant huntingtin. Mol. Ther. 26, 1965–1972. doi: 10.1016/j.ymthe.2018.05.013
Martier, R., Sogorb-Gonzalez, M., Stricker-Shaver, J., Hübener-Schmid, J., Keskin, S., Klima, J., et al. (2019). Development of an AAV-Based MicroRNA gene therapy to treat Machado-Joseph disease. Mol. Ther. Methods Clin. Dev. 15, 343–358. doi: 10.1016/j.omtm.2019.10.008
Mathieu, M., Martin-Jaular, L., Lavieu, G., and Théry, C. (2019). Specificities of secretion and uptake of exosomes and other extracellular vesicles for cell-to-cell communication. Nat. Cell Biol. 21, 9–17. doi: 10.1038/s41556-018-0250-9
Matsuzaka, Y., and Yashiro, R. (2022). Immune modulation using extracellular vesicles encapsulated with MicroRNAs as novel drug delivery systems. Int. J. Mol. Sci. 23:5658. doi: 10.3390/ijms23105658
Mishra, N., Ashique, S., Garg, A., Rai, V. K., Dua, K., Goyal, A., et al. (2022). Role of siRNA-based nanocarriers for the treatment of neurodegenerative diseases. Drug Discov. Today 27, 1431–1440. doi: 10.1016/j.drudis.2022.01.003
Moore, C. B., Guthrie, E. H., Huang, M. T., and Taxman, D. J. (2010). Short hairpin RNA (shRNA): Design, delivery, and assessment of gene knockdown. Methods Mol. Biol. 629, 141–158. doi: 10.1007/978-1-60761-657-3_10
Mori, M. A., Ludwig, R. G., Garcia-Martin, R., Brandão, B. B., and Kahn, C. R. (2019). Extracellular miRNAs: From biomarkers to mediators of physiology and disease. Cell Metab. 30, 656–673. doi: 10.1016/j.cmet.2019.07.011
Mueller, C., Berry, J. D., McKenna-Yasek, D. M., Gernoux, G., Owegi, M. A., Pothier, L. M., et al. (2020). SOD1 suppression with adeno-associated virus and MicroRNA in familial ALS. N. Engl. J. Med. 383, 151–158. doi: 10.1056/NEJMoa2005056
Muraoka, S., Lin, W., Takamatsu-Yukawa, K., Hu, J., Ikezu, S., DeTure, M. A., et al. (2021b). Enrichment of phosphorylated tau (Thr181) and functionally interacting molecules in chronic traumatic encephalopathy brain-derived extracellular vesicles. Aging Dis. 12, 1376–1388. doi: 10.14336/AD.2020.1007
Muraoka, S., DeLeo, A. M., Yang, Z., Tatebe, H., Yukawa-Takamatsu, K., Ikezu, S., et al. (2021a). Proteomic profiling of extracellular vesicles separated from plasma of former national football league players at risk for chronic traumatic encephalopathy. Aging Dis. 12, 1363–1375. doi: 10.14336/AD.2020.0908
Nakamori, M., Junn, E., Mochizuki, H., and Mouradian, M. M. (2019). Nucleic Acid-Based therapeutics for Parkinson’s disease. Neurotherapeutics 16, 287–298. doi: 10.1007/s13311-019-00714-7
Nitschke, L., Tewari, A., Coffin, S. L., Xhako, E., Pang, K., Gennarino, V. A., et al. (2020). miR760 regulates ATXN1 levels via interaction with its 5’ untranslated region. Genes Dev. 34, 1147–1160. doi: 10.1101/gad.339317.120
Niu, Y., Wang, X., Li, M., and Niu, B. (2020). Exosomes from human umbilical cord Mesenchymal stem cells attenuates stress-induced hippocampal dysfunctions. Metab. Brain Dis. 35, 1329–1340. doi: 10.1007/s11011-019-00514-0
Nobre, R. J., Lobo, D. D., Henriques, C., Duarte, S. P., Lopes, S. M., Silva, A. C., et al. (2022). miRNA-Mediated Knockdown of ATXN3 alleviates molecular disease hallmarks in a mouse model for spinocerebellar ataxia type 3. Nucleic Acid Ther. 32, 194–205. doi: 10.1089/nat.2021.0020
Nonaka, W., Takata, T., Iwama, H., Komatsubara, S., Kobara, H., Kamada, M., et al. (2022). A cerebrospinal fluid microRNA analysis: Progressive supranuclear palsy. Mol. Med. Rep. 25:88. doi: 10.3892/mmr.2022.12604
O’Brien, K., Breyne, K., Ughetto, S., Laurent, L. C., and Breakefield, X. O. (2020). RNA delivery by extracellular vesicles in mammalian cells and its applications. Nat. Rev. Mol. Cell Biol. 21, 585–606. doi: 10.1038/s41580-020-0251-y
Paul, S., Dansithong, W., Figueroa, K. P., Scoles, D. R., and Pulst, S. M. (2018). Staufen1 links RNA stress granules and autophagy in a model of neurodegeneration. Nat. Commun. 9:3648. doi: 10.1038/s41467-018-06041-3
Pei, X., Li, Y., Zhu, L., and Zhou, Z. (2020). Astrocyte-derived exosomes transfer miR-190b to inhibit oxygen and glucose deprivation-induced autophagy and neuronal apoptosis. Cell Cycle 19, 906–917. doi: 10.1080/15384101.2020.1731649
Penning, A., Tosoni, G., Abiega, O., Bielefeld, P., Gasperini, C., De Pietri Tonelli, D., et al. (2021). Adult neural stem cell regulation by small non-coding RNAs: Physiological significance and pathological implications. Front. Cell Neurosci. 15:781434. doi: 10.3389/fncel.2021.781434
Pérez Ortiz, J. M., and Orr, H. T. (2018). Spinocerebellar ataxia type 1: Molecular mechanisms of neurodegeneration and preclinical studies. Adv. Exp. Med. Biol. 1049, 135–145. doi: 10.1007/978-3-319-71779-1_6
Pomatto, M. A. C., Negro, F., and Camussi, G. (2022). Optimized protocol for plasma-derived extracellular vesicles loading with synthetic miRNA mimic using electroporation. Methods Mol. Biol. 2504, 219–230. doi: 10.1007/978-1-0716-2341-1_16
Qian, H., Kang, X., Hu, J., Zhang, D., Liang, Z., Meng, F., et al. (2020). Reversing a model of Parkinson’s disease with in situ converted nigral neurons. Nature 582, 550–556. doi: 10.1038/s41586-020-2388-4
Rezaei, R., Baghaei, K., Amani, D., Piccin, A., Hashemi, S. M., Asadzadeh Aghdaei, H., et al. (2021). Exosome-mediated delivery of functionally active miRNA-375-3p mimic regulate epithelial mesenchymal transition (EMT) of colon cancer cells. Life Sci. 269:119035. doi: 10.1016/j.lfs.2021.119035
Roshani, F., Delavar Kasmaee, H., Falahati, K., Arabzade, G., Sohan Forooshan Moghadam, A., and Sanati, M. H. (2021). Analysis of Micro-RNA-144 expression profile in patients with multiple sclerosis in comparison with healthy individuals. Rep. Biochem. Mol. Biol. 10, 396–401. doi: 10.52547/rbmb.10.3.396
Rübsamen, N., Pape, S., Konigorski, S., Zapf, A., Rücker, G., and Karch, A. (2022). Diagnostic accuracy of cerebrospinal fluid biomarkers for the differential diagnosis of sporadic Creutzfeldt-Jakob disease: A (network) meta-analysis. Eur. J. Neurol. 29, 1366–1376. doi: 10.1111/ene.15258
Saad, N. Y., Al-Kharsan, M., Garwick-Coppens, S. E., Chermahini, G. A., Harper, M. A., Palo, A., et al. (2021). Human miRNA miR-675 inhibits DUX4 expression and may be exploited as a potential treatment for facioscapulohumeral muscular dystrophy. Nat. Commun. 12:7128. doi: 10.1038/s41467-021-27430-1
Sanchez, I. I., Nguyen, T. B., England, W. E., Lim, R. G., Vu, A. Q., Miramontes, R., et al. (2021). Huntington’s disease mice and human brain tissue exhibit increased G3BP1 granules and TDP43 mislocalization. J. Clin. Invest. 131:e140723. doi: 10.1172/JCI140723
Sandau, U. S., McFarland, T. J., Smith, S. J., Galasko, D. R., Quinn, J. F., and Saugstad, J. A. (2022). Differential effects of APOE genotype on MicroRNA cargo of cerebrospinal fluid extracellular vesicles in females with Alzheimer’s disease compared to males. Front. Cell Dev. Biol. 10:864022. doi: 10.3389/fcell.2022.864022
Sava, V., Fihurka, O., Khvorova, A., and Sanchez-Ramos, J. (2020). Enriched chitosan nanoparticles loaded with siRNA are effective in lowering Huntington’s disease gene expression following intranasal administration. Nanomedicine 24:102119. doi: 10.1016/j.nano.2019.102119
Scholefield, J., Greenberg, L. J., Weinberg, M. S., Arbuthnot, P. B., Abdelgany, A., and Wood, M. J. (2009). Design of RNAi hairpins for mutation-specific silencing of ataxin-7 and correction of a SCA7 phenotype. PLoS One 4:e7232. doi: 10.1371/journal.pone.0007232
Serlidaki, D., van Waarde, M. A. W. H., Rohland, L., Wentink, A. S., Dekker, S. L., Kamphuis, M. J., et al. (2020). Functional diversity between HSP70 paralogs caused by variable interactions with specific co-chaperones. J. Biol. Chem. 295, 7301–7316. doi: 10.1074/jbc.RA119.012449
Setten, R. L., Rossi, J. J., and Han, S. P. (2019). The current state and future directions of RNAi-based therapeutics. Nat. Rev. Drug Discov. 18, 421–446. doi: 10.1038/s41573-019-0017-4
Sheng, P., Flood, K. A., and Xie, M. (2020). Short hairpin RNAs for strand-specific small interfering RNA production. Front. Bioeng. Biotechnol. 8:940. doi: 10.3389/fbioe.2020.00940
Shimizu, T., Tsutsumi, R., Shimizu, K., Tominaga, N., Nagai, M., Ugawa, Y., et al. (2020). Differential effects of thyrotropin releasing hormone (TRH) on motor execution and motor adaptation process in patients with spinocerebellar degeneration. J. Neurol. Sci. 415:116927. doi: 10.1016/j.jns.2020.116927
Shin, H. R., Moon, J., Lee, W. J., Lee, H. S., Kim, E. Y., Shin, S., et al. (2021). Serum neurofilament light chain as a severity marker for spinocerebellar ataxia. Sci. Rep. 11:13517. doi: 10.1038/s41598-021-92855-z
Sjoqvist, S., and Otake, K. (2022). A pilot study using proximity extension assay of cerebrospinal fluid and its extracellular vesicles identifies novel amyotrophic lateral sclerosis biomarker candidates. Biochem. Biophys. Res. Commun. 613, 166–173. doi: 10.1016/j.bbrc.2022.04.127
Soares Martins, T., Marçalo, R., da Cruz, E., Silva, C. B., Trindade, D., Catita, J., et al. (2022). Novel exosome biomarker candidates for Alzheimer’s disease unravelled through mass spectrometry analysis. Mol. Neurobiol. 59, 2838–2854 doi: 10.1007/s12035-022-02762-1
Song, S., Lee, J. U., Jeon, M. J., Kim, S., and Sim, S. J. (2022). Detection of multiplex exosomal miRNAs for clinically accurate diagnosis of Alzheimer’s disease using label-free plasmonic biosensor based on DNA-Assembled advanced plasmonic architecture. Biosens. Bioelectron. 199:113864. doi: 10.1016/j.bios.2021.113864
Song, Y., Li, Z., He, T., Qu, M., Jiang, L., Li, W., et al. (2019). M2 microglia-derived exosomes protect the mouse brain from ischemia-reperfusion injury via exosomal miR-124. Theranostics 9, 2910–2923. doi: 10.7150/thno.30879
Sun, D., Zhuang, X., Xiang, X., Liu, Y., Zhang, S., Liu, C., et al. (2010). A novel nanoparticle drug delivery system: The anti-inflammatory activity of curcumin is enhanced when encapsulated in exosomes. Mol. Ther. 18, 1606–1614. doi: 10.1038/mt.2010.105
Sun, I. O., Bae, Y. U., Lee, H., Kim, H., Jeon, J. S., Noh, H., et al. (2022). Circulating miRNAs in extracellular vesicles related to treatment response in patients with idiopathic membranous nephropathy. J. Transl. Med. 20:224. doi: 10.1186/s12967-022-03430-7
Sun, T., Ding, Z. X., Luo, X., Liu, Q. S., and Cheng, Y. (2020). Blood exosomes have neuroprotective effects in a mouse model of Parkinson’s disease. Oxid. Med. Cell. Longev. 2020:3807476. doi: 10.1155/2020/3807476
Surgucheva, I., Sharov, V. S., and Surguchov, A. (2012). γ-Synuclein: Seeding of α-synuclein aggregation and transmission between cells. Biochemistry 51, 4743–4754. doi: 10.1021/bi300478w
Suto, N., Mieda, T., Iizuka, A., Nakamura, K., and Hirai, H. (2016). Morphological and functional attenuation of degeneration of peripheral neurons by mesenchymal stem cell-conditioned medium in spinocerebellar ataxia type 1-Knock-in Mice. CNS Neurosci. Ther. 22, 670–676. doi: 10.1111/cns.12560
Takeuchi, T., and Nagai, Y. (2022). Emerging roles of extracellular vesicles in polyglutamine diseases: Mutant protein transmission, therapeutic potential, and diagnostics. Neurochem. Int. 157:105357. doi: 10.1016/j.neuint.2022.105357
Tao, L., Xu, C., Shen, W., Tan, J., Li, L., Fan, M., et al. (2021). HIPK3 inhibition by exosomal hsa-miR-101-3p is related to metabolic reprogramming in colorectal cancer. Front. Oncol. 11:758336. doi: 10.3389/fonc.2021.758336
Tapparo, M., Pomatto, M. A. C., Deregibus, M. C., Papadimitriou, E., Cavallari, C., D’Antico, S., et al. (2021). Serum derived extracellular vesicles mediated delivery of synthetic miRNAs in human endothelial cells. Front. Mol. Biosci. 8:636587. doi: 10.3389/fmolb.2021.636587
Thome, A. D., Thonhoff, J. R., Zhao, W., Faridar, A., Wang, J., Beers, D. R., et al. (2022). Extracellular vesicles derived from ex vivo expanded regulatory T cells modulate in vitro and in vivo inflammation. Front. Immunol. 13:875825. doi: 10.3389/fimmu.2022.875825
Tian, C., Stewart, T., Hong, Z., Guo, Z., Aro, P., Soltys, D., et al. (2022). Alzheimer’s disease neuroimaging initiative. Blood extracellular vesicles carrying synaptic function- and brain-related proteins as potential biomarkers for Alzheimer’s disease. Alzheimers Dement. doi: 10.1002/alz.12723
Van den Broek, B., Wuyts, C., Sisto, A., Pintelon, I., Timmermans, J. P., Somers, V., et al. (2022). Oligodendroglia-derived extracellular vesicles activate autophagy via LC3B/BAG3 to protect against oxidative stress with an enhanced effect for HSPB8 enriched vesicles. Cell Commun. Signal. 20:58. doi: 10.1186/s12964-022-00863-x
Vázquez-Mojena, Y., León-Arcia, K., González-Zaldivar, Y., Rodríguez-Labrada, R., and Velázquez-Pérez, L. (2021). Gene therapy for polyglutamine spinocerebellar ataxias: Advances, challenges, and perspectives. Mov. Disord. 36, 2731–2744. doi: 10.1002/mds.28819
Wang, P., Zhou, Y., and Richards, A. M. (2021). Effective tools for RNA-derived therapeutics: siRNA interference or miRNA mimicry. Theranostics 11, 8771–8796. doi: 10.7150/thno.62642
Wang, S., Khan, A., Huang, R., Ye, S., Di, K., Xiong, T., et al. (2020). Recent advances in single extracellular vesicle detection methods. Biosens. Bioelectron. 154:112056. doi: 10.1016/j.bios.2020.112056
Wang, W., Zhou, P., Wang, X., Chen, F., Christensen, E., Thompson, J., et al. (2022). Efficient and precise processing of the optimized primary artificial MicroRNA in a Huntingtin-Lowering Adeno-Associated viral gene therapy in vitro and in mice and nonhuman primates. Hum. Gene Ther. 33, 37–60. doi: 10.1089/hum.2021.221
Wei, X. C., Xia, Y. R., Zhou, P., Xue, X., Ding, S., Liu, L. J., et al. (2021). Hepatitis B core antigen modulates exosomal miR-135a to target vesicle-associated membrane protein 2 promoting chemoresistance in hepatocellular carcinoma. World J. Gastroenterol. 27, 8302–8322. doi: 10.3748/wjg.v27.i48.8302
Williams, A. M., Bhatti, U. F., Brown, J. F., Biesterveld, B. E., Kathawate, R. G., Graham, N. J., et al. (2020). Early single-dose treatment with exosomes provides neuroprotection and improves blood-brain barrier integrity in swine model of traumatic brain injury and hemorrhagic shock. J. Trauma Acute Care Surg. 88, 207–218. doi: 10.1097/TA.0000000000002563
Xia, H., Mao, Q., Eliason, S. L., Harper, S. Q., Martins, I. H., Orr, H. T., et al. (2004). RNAi suppresses polyglutamine-induced neurodegeneration in a model of spinocerebellar ataxia. Nat. Med. 10, 816–820. doi: 10.1038/nm1076
Yang, L., Shao, Y. R., Li, X. Y., Ma, Y., Dong, Y., and Wu, Z. Y. (2021). Association of the level of neurofilament light with disease severity in patients with spinocerebellar ataxia type 2. Neurology 97, e2404–e2413. doi: 10.1212/WNL.0000000000012945
Yap, K. H., Azmin, S., Che Hamzah, J., Ahmad, N., van de Warrenburg, B., and Mohamed Ibrahim, N. (2022b). Pharmacological and non-pharmacological management of spinocerebellar ataxia: A systematic review. J. Neurol. 269, 2315–2337. doi: 10.1007/s00415-021-10874-2
Yap, K. H., Abdul Manan, H., Yahya, N., Azmin, S., Mohamed Mukari, S. A., and Mohamed Ibrahim, N. (2022a). Magnetic resonance imaging and its clinical correlation in spinocerebellar ataxia type 3: A systematic review. Front. Neurosci. 16:859651. doi: 10.3389/fnins.2022.859651
Ye, C., Zheng, F., Xu, T., Wu, N., Tong, Y., Xiong, X. Q., et al. (2022). Norepinephrine acting on adventitial fibroblasts stimulates vascular smooth muscle cell proliferation via promoting small extracellular vesicle release. Theranostics 12, 4718–4733. doi: 10.7150/thno.70974
You, H. J., Fang, S. B., Wu, T. T., Zhang, H., Feng, Y. K., Li, X. J., et al. (2020). Mesenchymal stem cell-derived exosomes improve motor function and attenuate neuropathology in a mouse model of Machado-Joseph disease. Stem Cell Res. Ther. 11:222. doi: 10.1186/s13287-020-01727-2
Yu, L., Li, H., Liu, W., Zhang, L., Tian, Q., Li, H., et al. (2021). MiR-485-3p serves as a biomarker and therapeutic target of Alzheimer’s disease via regulating neuronal cell viability and neuroinflammation by targeting AKT3. Mol. Genet. Genomic Med. 9:e1548. doi: 10.1002/mgg3.1548
Zavatti, M., Gatti, M., Beretti, F., Palumbo, C., and Maraldi, T. (2022). Exosomes derived from human amniotic fluid mesenchymal stem cells preserve microglia and neuron cells from Aβ. Int. J. Mol. Sci. 23:4967. doi: 10.3390/ijms23094967
Zhai, L., Shen, H., Sheng, Y., and Guan, Q. A. D. M. S. C. (2021). Exo-MicroRNA-22 improve neurological function and neuroinflammation in mice with Alzheimer’s disease. J. Cell Mol. Med. 25, 7513–7523. doi: 10.1111/jcmm.16787
Zhang, Q., Higginbotham, J. N., Jeppesen, D. K., Yang, Y. P., Li, W., McKinley, E. T., et al. (2019). Transfer of functional cargo in exomeres. Cell Rep. 27, 940.e–954.e. doi: 10.1016/j.celrep.2019.01.009
Zhang, Y., Liu, Q., Zhang, X., Huang, H., Tang, S., Chai, Y., et al. (2022). Recent advances in exosome-mediated nucleic acid delivery for cancer therapy. J. Nanobiotechnol. 20:279. doi: 10.1186/s12951-022-01472-z
Zhao, Y., Haney, M. J., Fallon, J. K., Rodriguez, M., Swain, C. J., Arzt, C. J., et al. (2022a). Using extracellular vesicles released by GDNF-Transfected macrophages for therapy of parkinson disease. Cells 11:1933. doi: 10.3390/cells11121933
Zhao, Y., Liang, Y., Xu, Z., Liu, J., Liu, X., Ma, J., et al. (2022b). Exosomal miR-673-5p from fibroblasts promotes schwann cell-mediated peripheral neuron myelination by targeting the TSC2/mTORC1/SREBP2 axis. J. Biol. Chem. 298:101718. doi: 10.1016/j.jbc.2022.101718
Zhou, Z. R., Chen, X., Lv, J., Li, D. W., Yang, C. D., Liu, H. L., et al. (2022). A plasmonic nanoparticle-embedded polydopamine substrate for fluorescence detection of extracellular vesicle biomarkers in serum and urine from patients with systemic lupus erythematosus. Talanta 247:123620. doi: 10.1016/j.talanta.2022.123620
Keywords: extracellular vesicle, RNA interference, miRNA, siRNA, shRNA, spinocerebellar ataxia, neurodegenerative disease, combinational treatment
Citation: Ding Y, Zhang Y and Liu X (2022) Combinational treatments of RNA interference and extracellular vesicles in the spinocerebellar ataxia. Front. Mol. Neurosci. 15:1043947. doi: 10.3389/fnmol.2022.1043947
Received: 14 September 2022; Accepted: 21 September 2022;
Published: 13 October 2022.
Edited by:
Andrei Surguchov, University of Kansas Medical Center, United StatesReviewed by:
Irina G. Sourgoutcheva, University of Kansas Medical Center, United StatesCopyright © 2022 Ding, Zhang and Liu. This is an open-access article distributed under the terms of the Creative Commons Attribution License (CC BY). The use, distribution or reproduction in other forums is permitted, provided the original author(s) and the copyright owner(s) are credited and that the original publication in this journal is cited, in accordance with accepted academic practice. No use, distribution or reproduction is permitted which does not comply with these terms.
*Correspondence: Xuehong Liu, bGl1eHVlaDY1ODhAMTI2LmNvbQ==
†These authors have contributed equally to this work
Disclaimer: All claims expressed in this article are solely those of the authors and do not necessarily represent those of their affiliated organizations, or those of the publisher, the editors and the reviewers. Any product that may be evaluated in this article or claim that may be made by its manufacturer is not guaranteed or endorsed by the publisher.
Research integrity at Frontiers
Learn more about the work of our research integrity team to safeguard the quality of each article we publish.