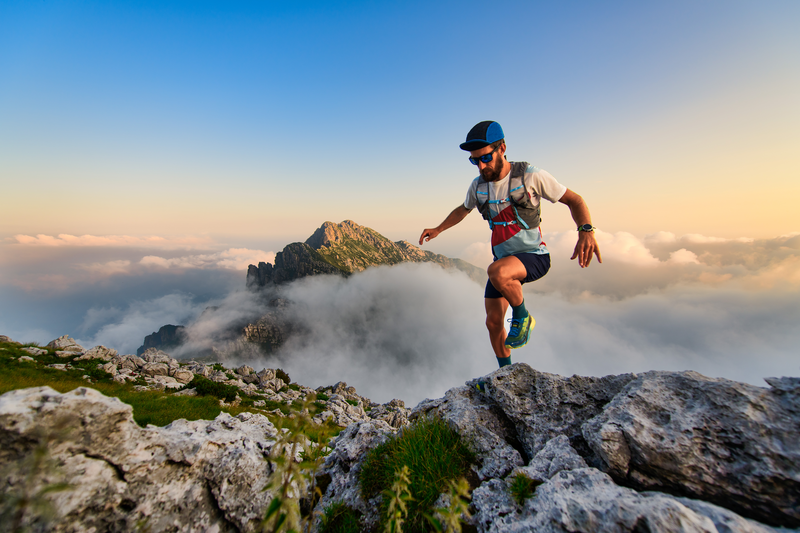
94% of researchers rate our articles as excellent or good
Learn more about the work of our research integrity team to safeguard the quality of each article we publish.
Find out more
MINI REVIEW article
Front. Mol. Neurosci. , 10 November 2022
Sec. Molecular Signalling and Pathways
Volume 15 - 2022 | https://doi.org/10.3389/fnmol.2022.1040699
This article is part of the Research Topic Sensory Changes Caused by Infectious, Inflammatory, and Metabolic Diseases: Focus on Neuroinflammatory Mechanisms. View all 4 articles
Finding new biomarkers and molecular targets to guide OA treatment remains a significant challenge. One of the most frequent forms of RNA methylation, N6-methyladenosine (m6A), can affect gene expression and RNA transcription, processing, translation, and metabolism. Osteoarthritis (OA) can cause disability and pain degenerative disease, reduce the quality of life of the elderly, and increase the social and economic burden. Changes in m6A levels are crucial in OA progress. In this review, the discussion will concentrate on the role that m6A plays in OA occurrence and progression. The m6A involved in the OA process mainly includes METTL3 and FTO. Current studies on m6A and OA primarily focus on four signaling pathways, namely, NF-κB, LNCRNAs, ATG7, and Bcl2. m6A participates in these signaling pathways and affects cellular inflammation, apoptosis, senescence, and autophagy, thus controlling the OA process. The modification of m6A affects so many signaling pathways. For the treatment of OA, it may represent a viable new therapeutic target.
Osteoarthritis (OA) is a chronic, degenerative joint disease that occurs mainly in older individuals. It is a primary cause of disability and social expense (Hunter and Bierma-Zeinstra, 2019). It predominantly affects joint cartilage, resulting in joint discomfort, edema, and stiffness (Jang et al., 2021). Around $81 billion in direct medical expenses are incurred annually in the US due to arthritis (Yelin et al., 2007). From 2013 to 2015, The US Centers for Disease Control and Prevention gathered statistics that show that the incidence of osteoarthritis in American adults was 21%, or about 54.4 million (Barbour et al., 2017), and it is expected that by 2040, the number of American adults with osteoarthritis will reach 78.4 million (Hootman et al., 2016). According to a new study, 23.9% of Chinese people suffer from OA (Li et al., 2020). With the aging population and rising obesity rates, the prevalence of OA is projected to increase progressively. No specific drugs exist for the treatment of OA. Physical therapy and non-steroidal anti-inflammatory medicines (NSAIDs) remain the primary options for treating clinical symptoms, and severe cases may require joint replacement.
Epigenetic studies focus on non-coding RNA regulation, histone modification, and DNA methylation modification. The selective transcriptional expression of genes is regulated by DNA methylation modification. Histone modification occurs after protein translation. The histones that make up nucleosomes can be modified by various compounds, such as phosphorylation, acetylation, and methylation. Non-coding RNA regulates post-transcriptional expression of genes (Skvortsova et al., 2018). RNA methylation, as one of the essential contents of epigenetic research, refers to the methylation modification that occurs in different positions of RNA molecules. The two most prevalent RNA post-transcriptional alterations in eukaryotes are 6-methyladenine (N6-methyladenine, m6A) and 5-methylcytosine (C5-methylcytidine, m5C) (Nombela et al., 2021). Specifically, m6A regulates methylation and demethylation, which are controlled by several enzymes, to control RNA splicing, output, translation, and degradation. It then affects a variety of physiological and pathological processes (Zhao et al., 2017).
Previous studies (Zhang et al., 2020; Li et al., 2021) have investigated the relationship between m6A and osteoarthritis, but their studies were limited to m6A mediating inflammatory factors and regulating OA through the NF-κB pathway. In this review, we summarized the role of m6A in the occurrence and development of OA to better comprehend the connection between the two from four signaling pathways, namely, NF-κB, LNCRNAs, ATG7, and Bcl2. The anticipation includes the possibility that m6A will someday be used as a therapeutic target for OA.
Early research found that m6A mainly occurs in the (G/A) (m6A) C sequence (Wang et al., 2014). Recent research has revealed that m6A predominantly appears in the termination codon and 3’UTR (Dominissini et al., 2012) and is translated in a cap-independent way near the 5′UTR (Karthiya and Khandelia, 2020). Thus, we can infer that m6A can affect gene expression, which in turn can affect RNA transcription, processing, translation, and metabolism. We emphasize the significance of this modification’s biological impact on the regulation of gene expression regulation (Roundtree et al., 2017), normal organismal development (Frye et al., 2018), and diseases (Hsu et al., 2017).
Mammalian cells undergo a dynamic and reversible m6A alteration mechanism (Zhou et al., 2020). Writers, erasers, and readers, which are the three essential components, can individually add, delete, or read m6A sites (Fu et al., 2014). The m6A modification procedure, which might potentially include reader involvement, can be launched by writers. Erasers, on the other hand, can promote the demethylation procedure. It has been discovered that these regulators are involved in RNA metabolic activities, such as selective cleavage, translation, stabilization, and miRNA treatment.
Methyltransferases, also known as writers, are a group of critical catalytic enzymes that can cause m6A methylation of bases on mRNA. The complex that connects m6A to mRNA is made up of METTL3, METTL14, METTL16, WTAP, VIRMA, ZC3H13, HAKAI, and RBM15/15B, among other subunits. The complex’s two primary components are METTL3 and METTL14, which control the majority of m6A modifications (Geula et al., 2015).
Methyltransferase-like protein 3 (METTL3) and METTL14 can be combined to form a METTL3/14 heterocomplex. Among these, METTL3 is a catalytically active subunit; METTL14 is necessary for substrate recognition and promotes the complex’s binding to RNA (Wang et al., 2016). The function of Wilms tumor 1-associating protein (WTAP) is to bind METTL3/14 and induce them to recruit and locate to the substrate, and WTAP is needed for METTL3/14 nuclear speckle localization and m6A modification (Ping et al., 2014). METTL16 can be used as a “writer” to catalyze the process of m6A modification in mRNA, especially nascent mRNA. In the cytoplasm, METTL16 promotes the assembly of 80s ribosomes by directly binding to eIF3a/b (eukaryotic initiation factor 3a/b) and rRNAs, thus improving protein translation efficiency and promoting tumor genesis and growth (Su et al., 2022). According to one study, the 3’UTR and stop codon regions of mRNA are preferentially methylated by the Vir like m6A methyltransferase associated (VIRMA) enzyme (Yue et al., 2018). The complex is promoted to become nuclear localized by the zinc finger CCCH-type containing 13 (ZC3H13), which also induces the transfer of the complex into the nucleus (Wen et al., 2018). RNA binding motif protein 15/15B (RBM15/15B) combines with the enrichment region of uracil to promote the methylation of some RNAs (Patil et al., 2016).
Demethylases are methyl group-removing enzymes that are also referred to as erasers. Fat mass and obesity-associated protein (FTO) or α-ketoglutarate-dependent dioxygenase alkB homolog 5 (ALKBH5) can reverse m6A methylation, and the two proteins are known as m6A erasers. Jia et al. (2011) confirmed for the first time in the world that FTO protein is a very important demethylase in DNA and RNA. FTO and ALKBH5 promote the demethylation of m6A in a Fe (II)- and -ketoglutaric acid-dependent manner. They initially oxidized m6A to produce N6-hydroxymethyladenosine (hm6A), which is subsequently transformed into N6-formyladenosine (f6A). The demethylation procedure was finished when f6A changed into adenosine (A) (Wang et al., 2020).
The core domain of FTO protein is comparable with the ALKB protein family, but the unique long loop at C-terminal is different from other proteins of the ALKB family. The ability of FTO proteins to demethylate the methylated single-stranded DNA or RNA is due to this specific domain (Gerken et al., 2007). FTO is relevant to human ponderal growth, obesity, and disease (Zhou et al., 2017). Once the transcription level of the FTO gene becomes abnormal, it will cause a variety of conditions, such as acute myeloid leukemia (Wei et al., 2018). ALKBH5 is the second m6A demethylase to be discovered (Zheng et al., 2013). Almost all tissues express ALKBH5, and it is essential for mouse spermatogenesis (Aik et al., 2014). However, ALKBH5 and FTO expression levels varied in various tissues. For instance, in mice, ALKHB5 is predominately expressed in the testes and female ovaries (Zheng et al., 2013). In contrast, FTO is predominately expressed in the brain, which may be related to various biochemical pathways in which they are involved. The target RNA is moved from the nucleus to the cytoplasm when ALKBH5 is knocked out in human cell lines (Zheng et al., 2013). Nearly all published studies about ALKBH5 function have identified similar molecular pathways. ALKBH5 modulates some transcripts of 3’UTR m6A, mediates hypoxia-inducible factor (HIF)-dependent breast CSC phenotype, and regulates glioblastoma growth and carcinogenesis via the ALKBH5-FOXM1 pathway, thereby indicating its essential role in tumorigenesis (Zhang et al., 2017).
To perform specific biological functions, a reader, which is also referred to as a particular RNA binding protein-methylated reading protein, is necessary for m6A-modified mRNA. By using an RNA pull-down assay, several reading proteins were discovered. These consist of YTH domain-containing proteins, nuclear heterogeneous ribosomal proteins (hnRNP), insulin-like growth factor 2 mRNA-binding proteins (IGF2BPs), and eukaryotic initiation factor (eIF) (He et al., 2019). These reading proteins have several roles, such as binding selectively to the m6A methylation region, diminishing homologous binding to RNA-binding proteins, and changing the secondary structure of RNA to affect protein-RNA interactions. A couple of proteins with YTH domains are the YTH domain-containing protein 1–2 (YTHDC1-2) and YTH N6-methyladenosine RNA binding protein 1–3 (YTHDF1-3). The cytoplasm is where YTHDF1-3 primarily identifies m6A-modified mRNA, whereas the nucleus is where YTHDC1-2 mostly functions. YTHDF1 promotes mRNA translation and protein synthesis by affecting the translation mechanism (Wang et al., 2015). By reducing its stability, YTHDF2 can regulate the rate of degradation of its target m6A transcript (Li et al., 2018). As a cofactor, target transcripts are translated and degraded more quickly in cells when YTHDF3 interacts with YTHDF1 and YTHDF2 (Shi et al., 2017). Proteins that contain YTH domains include YTHDC1-2. YTHDC1, which is located in the nucleus, has several functions, such as attracting some splicing factors to modulate mRNA splicing (Kasowitz et al., 2018), boosting mRNA output (Roundtree et al., 2017), and accelerating the decay of some transcripts (Shima et al., 2017). YTHDC2 modulates mRNA stability, translation (Mao et al., 2019), and spermatogenesis (Hsu et al., 2017). HnRNPC, hnRNPG, and hnRNPA2B1 are hnRNPs members, which can control how target transcripts are processed and spliced and also bind to some structures of RNA, which are mediated by m6A. The m6A reconstructs the local RNA structure and regulates the interaction between RNA- proteins. This phenomenon is called the m6A switch (Liu et al., 2015). IGF2BPs enhance RNA expression by promoting the stability of RNA (Muller et al., 2019).
Previously, OA was considered to be essentially a disease of mechanical damage, in which chronic loading and impaired joint biomechanics lead to the destruction of joint cartilage and inflammation, followed by stiffness, swelling, and reduced mobility. Studies suggested that OA is a more complex process regulated by inflammation and metabolic factors. At the cellular and molecular levels, OA is characterized by a transition from a healthy homeostasis state to a catabolic state (Chen et al., 2017). Articular cartilage is made up of chondrocytes, fibers, and stroma. Chondrocyte, the only type of cell found in cartilage, is responsible for generating and maintaining the cartilage matrix, which is mainly composed of type II collagen (coll-II) and proteoglycans (Glyn-Jones et al., 2015). In addition, chondrocytes are encapsulated in a rich extracellular matrix (ECM) and lack vascular, nerve, and lymphatic tissue (He et al., 2020). Changes in these components affect the dynamic balance of cartilage. At the molecular level, one of the significant causes of cartilage degeneration is the imbalance in ECM synthesis and breakdown in articular chondrocytes. Inflammatory mediators contribute to the degradation of cartilage ECM. These pro-inflammatory cytokines’ aberrant expression results in altered chondrocyte phenotypes. Interleukin-1 (IL)-1β and tumor necrosis factor-alpha (TNF-α) are two examples of conventionally pro-inflammatory cytokines that are elevated in the OA animal model. By activating the nuclear factor-κB (NF-κB) signaling pathway, they stimulate cellular inflammatory responses; they lead to extracellular matrix degradation by upregulation of matrix metalloproteinases (MMPs) (Choi et al., 2019). With age, the immune and surveillance functions are significantly weakened, and the aging cells cannot be effectively phagocytosed or cleared in time, resulting in accumulation (He and Sharpless, 2017; Mas-Bargues et al., 2021). Some inflammatory factors, such as IL-6 and IL-8, can accelerate cell aging in the way of autocrine, leading to cell apoptosis, thus accelerating the progression of OA (Soto-Gamez and Demaria, 2017). LncRNAs are functionally implicated throughout the whole life cycle of chondrocytes and in relation to OA progression. Over 20 different lncRNAs regulate OA pathogenesis by controlling the ECM degradation, chondrocyte activity, immunological response, and angiogenesis (Sun et al., 2019). The upregulation of MMP-13 and ADAMTS5 in OA is correlated with the expression of some lncRNAs, such as H19 (H19 imprinted maternally expressed transcript), and CTD-2574D22.4 (Xing et al., 2014).
m6A modifications are linked to OA. However, its biochemical mechanism and functional features are ambiguous. Thus, we summarize the existing evidence regarding m6A’s pleiotropic role in OA (Table 1).
NF-κB has been recognized as a typical proinflammatory signaling pathway for more than 30 years based on its role in the expression of pro-inflammatory cytokines and other pro-inflammatory genes. This pathway regulates immune-related pathways and affects cell survival, differentiation, and proliferation (Hayden and Ghosh, 2008). Liu et al. (2019) indicated a functional way of METTL3 in the process and underlying mechanisms of OA. METTL3 is expressed differently in various cells and tissues under varied experimental conditions, but METTL3 affects the development of OA by modulating the NF-κB signaling and ECM synthesis in chondrocytes. To simulate the inflammatory state in vitro, the inflammatory response of ATDC5 cells was stimulated by interfering with IL-1β. According to the findings, METTL3 expression was elevated in a dose-dependent manner. Inflammatory cytokines IL and TNF-α were also measured in terms of mRNA levels. Interfering with METTL3 silencing considerably decreased the expression of these molecules. By silencing the expression of METTL3, phosphorylated-p65 (p-p65), and p-κBα, NF-κB was inactivated. Silencing METTL3 with shRNA reduced the inflammatory cytokine levels and inhibited NF-κB signaling in chondrocytes, which delayed OA development. Moreover, METTL3 silencing decreased the expression of MMP13, and increased the expression of Aggrecan, thereby inhibiting ECM degradation. However, Sang et al. (2021) showed different results when they treated SW1353 cells with IL-1β. They found a decrease in METTL3 expression. Besides increasing the expression of P-65 and phosphorylated extracellular signal-regulated kinase (p-ERK), overexpression of METTL3 can also activate the NF-B signaling pathway and lower the levels of inflammatory cytokines. At the levels of mRNA and protein expression, MMP13, tissue inhibitors of metalloprotease (TIMP)-1, and TIMP-2 were decreased by METTL3 overexpression, but elevated levels of MMP1 and MMP3 increased ECM degradation. The different experimental results may be due to the different cell models selected. ATDC5 and SW1353 cells cannot wholly simulate the primary articular chondrocytes. Moreover, because collecting articular cartilage from people without OA is unethical, Sang et al. (2021) collected articular cartilage from patients undergoing hip replacement surgery for femoral neck fracture as normal control, but whether it can reflect the expression of METTL3 in normal human should be reconsidered. Liu et al. (2019) did not verify the expression of clinical OA, which may not reflect the real expression of OA patients.
An increasing number of studies indicated that m6A and long non-coding RNA (lncRNA) play a role in OA disease. However, the relationship between m6A and lncRNA’s biological role and clinical implications remain unclear. Yang et al. (2021) identified differential expression between normal and osteoarthritic cartilage by microarray analysis. AC008, Aquaporin 1 (AQP1) and ANK human gene (ANKH) are highly expressed in human OA. The high levels of AC008 and AQP1/ANKH expression can reduce the vitality of articular cartilage and promote chondrocyte apoptosis. AC008 promoted the expression of MMP13 and a disintegrin and metalloproteinase with thrombospondin motifs (ADAMTS)-5 and inhibited the expression of collagen type II alpha 1 (COL2A1) and Aggrecan, thus promoting ECM degradation. Through bioinformatics analysis, RNA immunoprecipitation (RIP), and luciferase analysis, it was observed that mir-328-3p might be targeting AQP1/ANKH, and AC008 plays the role of ceRNA through the sponge mir-328-3p. Thus, AC008 indirectly inhibits the effect of mir-328-3p on AQP1/ANKH. In addition, high expression of FTO in primary chondrocytes can down-regulate AC008 transcription, leading to instability of AC008, thus delaying the progression of OA. However, the low expression of FTO in OA leads to the up-regulation of AC008 transcription, thus accelerating the progression of arthritis. Ren et al. (2022) confirmed that LINC00680 was increased in OA tissue and isolated primary chondrocytes stimulated by 1 l-1β. METTL3 binds to the m6A site of LINC00680 and upregulates its expression. In 1 l-1β-induced chondrocytes, LINC00680 knockdown facilitates cell proliferation and inhibits ECM degradation. In terms of mechanism, LINC00680 can inhibit the transcription and expression of COL2A1 while stimulating the transcription and expression of MMP13, thus aggravating the degradation process of ECM, and accelerating the development of arthritis. Moreover, SIRT1 and IGF2BP2 are enzymes related to chondrocyte apoptosis. When IGF2BP2 interacts with LINC00680, which is triggered by METTL3 at the m6A modification site, Sirt1 mRNA becomes more stable. A suggestion for the future treatment of OA is provided by the interplay between m6A and lncRNA.
Autophagy Related Protein 7 (ATG7) is an autophagy effector enzyme that regulates the cell cycle and apoptosis in senescent cells (Collier et al., 2021). Senescence of synovial and cartilage can be triggered by aged fibroblast-like synovial cells (FLSs), which can also cause cartilage dysfunction. To study how METTL3 affects the control of autophagy, Chen et al. (2022) collected synovial tissues from healthy individuals and OA patients. The outcomes demonstrated that m6A modification by METTL3 could weaken ATG7 RNA’s stability, reduce the expression of ATG7, induce the generation and elevation of senescence-associated secretory phenotype (SASP), promote the aging of the synovial tissue, and accelerate the progression of OA. METTL3 was silenced by siRNA on the destabilization of the medial meniscus (DMM) surgery mice. The autophagy flux of OA-FLS increased. SASP decreased. METTL3 silencing inhibited cell senescence. Moreover, GATA4, a new aging regulator, was confirmed to be significantly upregulated in FLSs both in vitro and in vivo. GATA4 gene knockout inhibited 1 l-1β secretion and significantly inhibited SASP expression.
BCL2 is a pro-survival protein that participates in the regulation of apoptosis and plays an important role (Willimott and Wagner, 2010). He et al. (2022) proved that METTL3 decreased in both in vivo mouse models of temporomandibular joint (TMJ) OA and in vitro inflammation. They identified Bcl2 mRNA as a downstream target of METTL3 using m6A-RNA immunoprecipitation (MeRIP). By siRNA, targeting Bcl2 silenced it, and the level of pro-apoptotic factors, such as bax and cleaved-caspase3, increased. However, the levels of anti-apoptotic factors, such as P62 and SRY-Box Transcription Factor 9 (SOX9), decreased. The role of METTL3 overexpression in controlling chondrocyte apoptosis and autophagy was reversed by silencing Bcl2. When YTHDF1 was silenced, Bcl2 mRNA’s stability was drastically decreased, because Bcl2 mRNA was regulated by YTHDF1 in an m6A-dependent way. METTL3 enhances the stability of Bcl2 through YTHDF1, thereby providing new insight into the treatment of TMJ OA.
The m6A is so comprehensively distributed across tissues, compared with other tissues, the m6A methyl group in brain tissue is highly specific (Liu et al., 2020). The role of m6A in the central nervous system, hematopoietic system and reproductive system has been widely discussed, especially in cancer progression (Jiang et al., 2021). Because the effect of m6A is so widespread, it is uncertain whether the compound that will eventually be developed to bind to the m6A will affect m6A in other tissues of patients with OA and there is a lack of studies on the safety and efficacy of m6A.
In this review, we emphasize the function of m6A and its regulatory mechanism in the genesis of OA (Figure 1). METTL3 plays a role in OA progression by mediating NF-κB signaling way and ECM synthesis in chondrocytes (Liu et al., 2019; Sang et al., 2021). METTL3 connects to the LINC00680 m6A site and promotes its expression. LINC00680 knockdown increases cell proliferation while inhibiting ECM breakdown (Ren et al., 2022). Furthermore, inhibiting METTL3 decreases cell senescence (Chen et al., 2022). The role of METTL3 overexpression in controlling chondrocyte apoptosis and autophagy was reversed when Bcl2 was silenced (He et al., 2022). High FTO expression in primary chondrocytes can inhibit AC008 transcription, causing AC008 instability and slowing the onset of OA (Yang et al., 2021). m6A regulates the inflammatory response, senescence, apoptosis, and autophagy by mediating these four pathways, delaying inevitable cartilage destruction, and thus plays a disease-modifying role in controlling the OA process.
Figure 1. The role of m6A regulators in ATDC5, FLSs, SWB53, and Chondrocytes. ADAMTS-5, a disintegrin and metalloproteinase with thrombospondin motifs-5; ATG7, autophagy related protein 7; COL2A1, collagen type II alpha 1; Coll II, type II collagen; ECM, extracellular matrix; FLSs, fibroblast like synoviocytes; FTO, fat mass and obesity-associated protein; IL, interleukin; LC3B-II, microtubule-associated protein 1 light chain 3B-II; MMP, matrix metalloproteinase NF-κB, nuclear factor-κB; p-p65, phosphorylated-p65; TIMP, tissue inhibitors of metalloprotease; TNF-α, tumor necrosis factor alpha; YTHDF, YTH N6-methyladenosine RNA binding protein.
In summary, for OA treatment, m6A offers a new approach. However, more studies are needed to comprehend the mechanisms and the correlation between m6A modification and OA, and on the safety and efficacy. Then, the m6A might represent a viable new treatment target for OA.
X-QW contributed to conceptualization, project administration, writing—review and editing, and funding acquisition. Y-LZ contributed to conceptualization, writing—review, and editing. HL contributed to writing—original draft preparation. All authors contributed to the article and approved the submitted version.
The authors disclosed receipt of financial support from the following for the research, authorship and/or publication of this article: the National Natural Science Foundation of China (81871844), Shanghai Key Lab of Human Performance (Shanghai University of Sport, fund number: 11DZ2261100), Shanghai Frontiers Science Research Base of Exercise and Metabolic Health, Talent Development Fund of Shanghai Municipal (fund number: 2021081), Shanghai Clinical Research Center for Rehabilitation Medicine (fund number: 21MC1930200), and the Scientific and Technological Research Program of the Shanghai Science and Technology Committee (fund number: 21S31902400).
The authors declare that the research was conducted in the absence of any commercial or financial relationships that could be construed as a potential conflict of interest.
All claims expressed in this article are solely those of the authors and do not necessarily represent those of their affiliated organizations, or those of the publisher, the editors and the reviewers. Any product that may be evaluated in this article, or claim that may be made by its manufacturer, is not guaranteed or endorsed by the publisher.
Aik, W., Scotti, J. S., Choi, H., Gong, L., Demetriades, M., Schofield, C. J., et al. (2014). Structure of human RNA N6-methyladenine demethylase ALKBH5 provides insights into its mechanisms of nucleic acid recognition and demethylation. Nucleic Acids Res. 42, 4741–4754. doi: 10.1093/nar/gku085
Barbour, K. E., Helmick, C. G., Boring, M., and Brady, T. J. (2017). Vital signs: prevalence of doctor-diagnosed arthritis and arthritis- attributable activity limitation – United States, 2013–2015. MMWR Morb. Mortal. Wkly Rep. 66, 246–253. doi: 10.15585/mmwr.mm6609e1
Chen, X., Gong, W., Shao, X., Shi, T., Zhang, L., Dong, J., et al. (2022). METTL3-mediated m(6)a modification of ATG7 regulates autophagy-GATA4 axis to promote cellular senescence and osteoarthritis progression. Ann. Rheum. Dis. 81, 87–99. doi: 10.1136/annrheumdis-2021-221091
Chen, D., Shen, J., Zhao, W., Wang, T., Han, L., Hamilton, J. L., et al. (2017). Osteoarthritis: toward a comprehensive understanding of pathological mechanism. Bone Res. 5:16044. doi: 10.1038/boneres.2016.44
Choi, M. C., Jo, J., Park, J., Kang, H. K., and Park, Y. (2019). NF-kappaB signaling pathways in osteoarthritic cartilage destruction. Cells 8:734. doi: 10.3390/cells8070734
Collier, J. J., Suomi, F., Olahova, M., McWilliams, T. G., and Taylor, R. W. (2021). Emerging roles of ATG7 in human health and disease. EMBO Mol. Med. 13:e14824. doi: 10.15252/emmm.202114824
Dominissini, D., Moshitch-Moshkovitz, S., Schwartz, S., Salmon-Divon, M., Ungar, L., Osenberg, S., et al. (2012). Topology of the human and mouse m6A RNA methylomes revealed by m6A-seq. Nature 485, 201–206. doi: 10.1038/nature11112
Frye, M., Harada, B. T., Behm, M., and He, C. (2018). RNA modifications modulate gene expression during development. Science 361, 1346–1349. doi: 10.1126/science.aau1646
Fu, Y., Dominissini, D., Rechavi, G., and He, C. (2014). Gene expression regulation mediated through reversible m6A RNA methylation. Nat. Rev. Genet. 15, 293–306. doi: 10.1038/nrg3724
Gerken, T., Girard, C. A., Tung, Y. C., Webby, C. J., Saudek, V., Hewitson, K. S., et al. (2007). The obesity-associated FTO gene encodes a 2-oxoglutarate-dependent nucleic acid demethylase. Science 318, 1469–1472. doi: 10.1126/science.1151710
Geula, S., Moshitch-Moshkovitz, S., Dominissini, D., Mansour, A. A., Kol, N., Salmon-Divon, M., et al. (2015). Stem cells. m6A mRNA methylation facilitates resolution of naive pluripotency toward differentiation. Science 347, 1002–1006. doi: 10.1126/science.1261417
Glyn-Jones, S., Palmer, A. J., Agricola, R., Price, A. J., Vincent, T. L., Weinans, H., et al. (2015). Osteoarthritis. Lancet. 386, 376–387. doi: 10.1016/S0140-6736(14)60802-3
Hayden, M. S., and Ghosh, S. (2008). Shared principles in NF-kappaB signaling. Cells 132, 344–362. doi: 10.1016/j.cell.2008.01.020
He, Y., Li, Z., Alexander, P. G., Ocasio-Nieves, B. D., Yocum, L., Lin, H., et al. (2020). Pathogenesis of osteoarthritis: risk factors, regulatory pathways in chondrocytes, and experimental models. Biology 9:194. doi: 10.3390/biology9080194
He, L., Li, H., Wu, A., Peng, Y., Shu, G., and Yin, G. (2019). Functions of N6-methyladenosine and its role in cancer. Mol. Cancer 18:176. doi: 10.1186/s12943-019-1109-9
He, S., and Sharpless, N. E. (2017). Senescence in health and disease. Cells 169, 1000–1011. doi: 10.1016/j.cell.2017.05.015
He, Y., Wang, W., Xu, X., Yang, B., Yu, X., Wu, Y., et al. (2022). Mettl3 inhibits the apoptosis and autophagy of chondrocytes in inflammation through mediating Bcl2 stability via Ythdf1-mediated m(6)a modification. Bone 154:116182. doi: 10.1016/j.bone.2021.116182
Hootman, J. M., Helmick, C. G., Barbour, K. E., Theis, K. A., and Boring, M. A. (2016). Updated projected prevalence of self-reported doctor-diagnosed arthritis and arthritis-attributable activity limitation among US adults, 2015–2040. Arthritis Rheumatol. 68, 1582–1587. doi: 10.1002/art.39692
Hsu, P. J., Shi, H., and He, C. (2017). Epitranscriptomic influences on development and disease. Genome Biol. 18:197. doi: 10.1186/s13059-017-1336-6
Hsu, P. J., Zhu, Y., Ma, H., Guo, Y., Shi, X., Liu, Y., et al. (2017). Ythdc2 is an N(6)-methyladenosine binding protein that regulates mammalian spermatogenesis. Cell Res. 27, 1115–1127. doi: 10.1038/cr.2017.99
Hunter, D. J., and Bierma-Zeinstra, S. (2019). Osteoarthritis. Lancet 393, 1745–1759. doi: 10.1016/S0140-6736(19)30417-9
Jang, S., Lee, K., and Ju, J. H. (2021). Recent updates of diagnosis, pathophysiology, and treatment on osteoarthritis of the knee. Int. J. Mol. Sci. 22:2619. doi: 10.3390/ijms22052619
Jia, G., Fu, Y., Zhao, X., Dai, Q., Zheng, G., Yang, Y., et al. (2011). N6-methyladenosine in nuclear RNA is a major substrate of the obesity- associated FTO. Nat. Chem. Biol. 7, 885–887. doi: 10.1038/nchembio.687
Jiang, X., Liu, B., Nie, Z., Duan, L., Xiong, Q., Jin, Z., et al. (2021). The role of m6A modification in the biological functions and diseases. Signal Transduct. Target. Ther. 6:74. doi: 10.1038/s41392-020-00450-x
Karthiya, R., and Khandelia, P. (2020). m6A RNA methylation: ramifications for gene expression and human health. Mol. Biotechnol. 62, 467–484. doi: 10.1007/s12033-020-00269-5
Kasowitz, S. D., Ma, J., Anderson, S. J., Leu, N. A., Xu, Y., Gregory, B. D., et al. (2018). Nuclear m6A reader YTHDC1 regulates alternative polyadenylation and splicing during mouse oocyte development. PLoS Genet. 14:e1007412. doi: 10.1371/journal.pgen.1007412
Li, Z., Liu, Q., Zhao, C., Gao, X., Han, W., Stefanik, J. J., et al. (2020). High prevalence of patellofemoral osteoarthritis in China: a multi-center population-based osteoarthritis study. Clin. Rheumatol. 39, 3615–3623. doi: 10.1007/s10067-020-05110-7
Li, H., Xiao, W., He, Y., Wen, Z., Cheng, S., Zhang, Y., et al. (2021). Novel insights into the multifaceted functions of RNA n(6)-methyladenosine modification in degenerative musculoskeletal diseases. Front. Cell Dev. Biol. 9:766020. doi: 10.3389/fcell.2021.766020
Li, M., Zhao, X., Wang, W., Shi, H., Pan, Q., Lu, Z., et al. (2018). Ythdf2-mediated m(6)a mRNA clearance modulates neural development in mice. Genome Biol. 19:69. doi: 10.1186/s13059-018-1436-y
Liu, N., Dai, Q., Zheng, G., He, C., Parisien, M., and Pan, T. (2015). N(6)-methyladenosine-dependent RNA structural switches regulate RNA- protein interactions. Nature 518, 560–564. doi: 10.1038/nature14234
Liu, J., Li, K., Cai, J., Zhang, M., Zhang, X., Xiong, X., et al. (2020). Landscape and regulation of m(6)a and m(6)am methylome across human and mouse tissues. Mol. Cell 77, 426–440.e6. doi: 10.1016/j.molcel.2019.09.032
Liu, Q., Li, M., Jiang, L., Jiang, R., and Fu, B. (2019). METTL3 promotes experimental osteoarthritis development by regulating inflammatory response and apoptosis in chondrocyte. Biochem. Biophys. Res. Commun. 516, 22–27. doi: 10.1016/j.bbrc.2019.05.168
Mao, Y., Dong, L., Liu, X. M., Guo, J., Ma, H., Shen, B., et al. (2019). M(6)a in mRNA coding regions promotes translation via the RNA helicase- containing YTHDC2. Nat. Commun. 10:5332. doi: 10.1038/s41467-019-13317-9
Mas-Bargues, C., Borras, C., and Vina, J. (2021). Bcl-xL as a modulator of senescence and aging. Int. J. Mol. Sci. 22:1527. doi: 10.3390/ijms22041527
Muller, S., Glass, M., Singh, A. K., Haase, J., Bley, N., Fuchs, T., et al. (2019). IGF2BP1 promotes SRF-dependent transcription in cancer in a m6A- and miRNA-dependent manner. Nucleic Acids Res. 47, 375–390. doi: 10.1093/nar/gky1012
Nombela, P., Miguel-López, B., and Blanco, S. (2021). The role of m(6)a, m(5)C and Ψ RNA modifications in cancer: novel therapeutic opportunities. Mol. Cancer 20:18. doi: 10.1186/s12943-020-01263-w
Patil, D. P., Chen, C. K., Pickering, B. F., Chow, A., Jackson, C., Guttman, M., et al. (2016). M(6)a RNA methylation promotes XIST-mediated transcriptional repression. Nature 537, 369–373. doi: 10.1038/nature19342
Ping, X. L., Sun, B. F., Wang, L., Xiao, W., Yang, X., Wang, W. J., et al. (2014). Mammalian WTAP is a regulatory subunit of the RNA N6-methyladenosine methyltransferase. Cell Res. 24, 177–189. doi: 10.1038/cr.2014.3
Ren, J., Li, Y., Wuermanbieke, S., Hu, S., and Huang, G. (2022). N(6)-methyladenosine (m(6)a) methyltransferase METTL3-mediated LINC00680 accelerates osteoarthritis through m(6)a/SIRT1 manner. Cell Death Discov. 8:240. doi: 10.1038/s41420-022-00890-0
Roundtree, I. A., Evans, M. E., Pan, T., and He, C. (2017). Dynamic RNA modifications in gene expression regulation. Cells 169, 1187–1200. doi: 10.1016/j.cell.2017.05.045
Roundtree, I. A., Luo, G. Z., Zhang, Z., Wang, X., Zhou, T., Cui, Y., et al. (2017). YTHDC1 mediates nuclear export of N(6)-methyladenosine methylated mRNAs. elife 6.
Sang, W., Xue, S., Jiang, Y., Lu, H., Zhu, L., Wang, C., et al. (2021). METTL3 involves the progression of osteoarthritis probably by affecting ECM degradation and regulating the inflammatory response. Life Sci. 278:119528. doi: 10.1016/j.lfs.2021.119528
Shi, H., Wang, X., Lu, Z., Zhao, B. S., Ma, H., Hsu, P. J., et al. (2017). YTHDF3 facilitates translation and decay of N(6)-methyladenosine-modified RNA. Cell Res. 27, 315–328. doi: 10.1038/cr.2017.15
Shima, H., Matsumoto, M., Ishigami, Y., Ebina, M., Muto, A., Sato, Y., et al. (2017). S-Adenosylmethionine synthesis is regulated by selective N(6)-adenosine methylation and mRNA degradation involving METTL16 and YTHDC1. Cell Rep. 21, 3354–3363. doi: 10.1016/j.celrep.2017.11.092
Skvortsova, K., Iovino, N., and Bogdanovic, O. (2018). Functions and mechanisms of epigenetic inheritance in animals. Nat. Rev. Mol. Cell Biol. 19, 774–790. doi: 10.1038/s41580-018-0074-2
Soto-Gamez, A., and Demaria, M. (2017). Therapeutic interventions for aging: the case of cellular senescence. Drug Discov. Today 22, 786–795. doi: 10.1016/j.drudis.2017.01.004
Su, R., Dong, L., Li, Y., Gao, M., He, P. C., Liu, W., et al. (2022). METTL16 exerts an m(6)A-independent function to facilitate translation and tumorigenesis. Nat. Cell Biol. 24, 205–216. doi: 10.1038/s41556-021-00835-2
Sun, H., Peng, G., Ning, X., Wang, J., Yang, H., and Deng, J. (2019). Emerging roles of long noncoding RNA in chondrogenesis, osteogenesis, and osteoarthritis. Am. J. Transl. Res. 11, 16–30.
Wang, P., Doxtader, K. A., and Nam, Y. (2016). Structural basis for cooperative function of Mettl3 and Mettl14 methyltransferases. Mol. Cell 63, 306–317. doi: 10.1016/j.molcel.2016.05.041
Wang, T., Kong, S., Tao, M., and Ju, S. (2020). The potential role of RNA N6-methyladenosine in cancer progression. Mol. Cancer 19:88. doi: 10.1186/s12943-020-01204-7
Wang, X., Lu, Z., Gomez, A., Hon, G. C., Yue, Y., Han, D., et al. (2014). N6-methyladenosine-dependent regulation of messenger RNA stability. Nature 505, 117–120. doi: 10.1038/nature12730
Wang, X., Zhao, B. S., Roundtree, I. A., Lu, Z., Han, D., Ma, H., et al. (2015). N(6)-methyladenosine modulates messenger RNA translation efficiency. Cells 161, 1388–1399. doi: 10.1016/j.cell.2015.05.014
Wei, J., Liu, F., Lu, Z., Fei, Q., Ai, Y., He, P. C., et al. (2018). Differential m(6)a, m(6)am, and m(1)a demethylation mediated by FTO in the cell nucleus and cytoplasm. Mol. Cell 71, 973–85.e5. doi: 10.1016/j.molcel.2018.08.011
Wen, J., Lv, R., Ma, H., Shen, H., He, C., Wang, J., et al. (2018). Zc3h13 regulates nuclear RNA m(6)a methylation and mouse embryonic stem cell self-renewal. Mol. Cell 69, 1028–38.e6. doi: 10.1016/j.molcel.2018.02.015
Willimott, S., and Wagner, S. D. (2010). Post-transcriptional and post-translational regulation of Bcl2. Biochem. Soc. Trans. 38, 1571–1575. doi: 10.1042/BST0381571
Xing, D., Liang, J. Q., Li, Y., Lu, J., Jia, H. B., Xu, L. Y., et al. (2014). Identification of long noncoding RNA associated with osteoarthritis in humans. Orthop. Surg. 6, 288–293. doi: 10.1111/os.12147
Yang, J., Zhang, M., Yang, D., Ma, Y., Tang, Y., Xing, M., et al. (2021). M(6)A-mediated upregulation of AC008 promotes osteoarthritis progression through the miR-328-3pAQP1/ANKH axis. Exp. Mol. Med. 53, 1723–1734. doi: 10.1038/s12276-021-00696-7
Yelin, E., Murphy, L., Cisternas, M. G., Foreman, A. J., Pasta, D. J., and Helmick, C. G. (2007). Medical care expenditures and earnings losses among persons with arthritis and other rheumatic conditions in 2003, and comparisons with 1997. Arthritis Rheum. 56, 1397–1407. doi: 10.1002/art.22565
Yue, Y., Liu, J., Cui, X., Cao, J., Luo, G., Zhang, Z., et al. (2018). VIRMA mediates preferential m(6)a mRNA methylation in 3'UTR and near stop codon and associates with alternative polyadenylation. Cell Discov. 4:10. doi: 10.1038/s41421-018-0019-0
Zhang, W., He, L., Liu, Z., Ren, X., Qi, L., Wan, L., et al. (2020). Multifaceted functions and novel insight into the regulatory role of RNA N(6)-methyladenosine modification in musculoskeletal disorders. Front. Cell Dev. Biol. 8:870. doi: 10.3389/fcell.2020.00870
Zhang, S., Zhao, B. S., Zhou, A., Lin, K., Zheng, S., Lu, Z., et al. (2017). M(6)a demethylase ALKBH5 maintains tumorigenicity of glioblastoma stem- like cells by sustaining FOXM1 expression and cell proliferation program. Cancer Cell 31, 591–606.e6. doi: 10.1016/j.ccell.2017.02.013
Zhao, B. S., Roundtree, I. A., and He, C. (2017). Post-transcriptional gene regulation by mRNA modifications. Nat. Rev. Mol. Cell Biol. 18, 31–42. doi: 10.1038/nrm.2016.132
Zheng, G., Dahl, J. A., Niu, Y., Fedorcsak, P., Huang, C. M., Li, C. J., et al. (2013). ALKBH5 is a mammalian RNA demethylase that impacts RNA metabolism and mouse fertility. Mol. Cell 49, 18–29. doi: 10.1016/j.molcel.2012.10.015
Zhou, Y., Hambly, B. D., and McLachlan, C. S. (2017). FTO associations with obesity and telomere length. J. Biomed. Sci. 24:65. doi: 10.1186/s12929-017-0372-6
Keywords: N6-methyladenosine, osteoarthritis, inflammation, apoptosis, senescence, autophagy, LncRNAs
Citation: Liu H, Zheng Y-L and Wang X-Q (2022) The emerging roles of N6-methyladenosine in osteoarthritis. Front. Mol. Neurosci. 15:1040699. doi: 10.3389/fnmol.2022.1040699
Received: 09 September 2022; Accepted: 19 October 2022;
Published: 10 November 2022.
Edited by:
Nara L. M. Quintão, Universidade do Vale do Itajaí, BrazilReviewed by:
Nick Andrews, Salk Institute for Biological Studies, United StatesCopyright © 2022 Liu, Zheng and Wang. This is an open-access article distributed under the terms of the Creative Commons Attribution License (CC BY). The use, distribution or reproduction in other forums is permitted, provided the original author(s) and the copyright owner(s) are credited and that the original publication in this journal is cited, in accordance with accepted academic practice. No use, distribution or reproduction is permitted which does not comply with these terms.
*Correspondence: Xue-Qiang Wang, d2FuZ3h1ZXFpYW5nQHN1cy5lZHUuY24=
†These authors have contributed equally to this work and share first authorship
Disclaimer: All claims expressed in this article are solely those of the authors and do not necessarily represent those of their affiliated organizations, or those of the publisher, the editors and the reviewers. Any product that may be evaluated in this article or claim that may be made by its manufacturer is not guaranteed or endorsed by the publisher.
Research integrity at Frontiers
Learn more about the work of our research integrity team to safeguard the quality of each article we publish.