- 1Department of Sport Rehabilitation, Shanghai University of Sport, Shanghai, China
- 2Department of Luoyang Postgraduate Training, Henan University of Traditional Chinese Medicine, Luoyang, China
- 3Department of Rehabilitation Medicine, Shanghai Shangti Orthopaedic Hospital, Shanghai, China
- 4Shanghai Key Lab of Human Performance, Shanghai University of Sport, Shanghai, China
Structural and functional changes of the brain occur in many chronic pain conditions, including chronic low back pain (CLBP), and these brain abnormalities can be reversed by effective treatment. Research on the clinical applications of non-invasive brain neuromodulation (NIBS) techniques for chronic pain is increasing. Unfortunately, little is known about the effectiveness of NIBS on CLBP, which limits its application in clinical pain management. Therefore, we summarized the effectiveness and limitations of NIBS techniques on CLBP management and described the effects and mechanisms of NIBS approaches on CLBP in this review. Overall, NIBS may be effective for the treatment of CLBP. And the analgesic mechanisms of NIBS for CLBP may involve the regulation of pain signal pathway, synaptic plasticity, neuroprotective effect, neuroinflammation modulation, and variations in cerebral blood flow and metabolism. Current NIBS studies for CLBP have limitations, such as small sample size, relative low quality of evidence, and lack of mechanistic studies. Further studies on the effect of NIBS are needed, especially randomized controlled trials with high quality and large sample size.
Introduction
Low back pain, which refers to pain and discomfort in the lumbosacral region, is a highly prevalent condition with high burden worldwide (Balagué et al., 2012; Smith et al., 2022). The point prevalence and lifetime prevalence of low back pain are 7.83% (95% confidence interval [CI]: 7.04–8.64) and 84% (Balagué et al., 2012). Moreover, the prevalence of low back pain and years lived with disability caused by low back pain are expected to increase as a result of population growth and aging (Hartvigsen et al., 2018). In the US, the annual expenditures for low back pain exceed $100 billion (Katz, 2006), and the annual costs for each patient reached $8386 (Gore et al., 2012). Chronic low back pain (CLBP) is defined as low back pain with a course of over 12 weeks or 3 months (Andersson, 1999). Approximately two-thirds of low back pain cases will develop CLBP after the first episode (Morlion, 2013). Although CLBP has been recognized as a crucial global health and socioeconomic problem, its treatment has tremendous potential for improvement (Knezevic et al., 2021).
Alterations exist in the structure and function of several neural networks in patients with CLBP (Kregel et al., 2015; Ng et al., 2018). Brain imaging studies have confirmed the structural changes in gray matter in the thalamus, dorsolateral prefrontal cortex, temporal lobes, insula, and the primary somatosensory cortex in patients with CLBP (Giesecke et al., 2004; Vlaeyen et al., 2018). Moreover, patients with CLBP had increased activities in certain cortical and subcortical regions (such as the prefrontal cortex and cingulate cortex) and reduced activities in pain-relief areas. These neuroanatomical and functional abnormalities in the brain can be reversed by an effective treatment (Seminowicz et al., 2011). Therefore, directly targeting the brain region involved in pain processing may be an effective treatment for CLBP. Non-invasive brain neuromodulation (NIBS) was defined as any brain stimulation technique that directly modulates brain activity and the neural network involved in pain processing but does not require invasive methods (Jenkins and Tepper, 2011; Romanella et al., 2020). Repetitive transcranial magnetic stimulation (rTMS) and transcranial direct current stimulation (tDCS) are the two most commonly used forms of NIBS (O’Connell et al., 2018). Recently, NIBS has been used for the management of chronic pain, including neuropathic pain (Zhang et al., 2021) and chronic headache (Cheng et al., 2022). Many studies also explored the effect of NIBS on patients with CLBP and observed positive results, but the placebo effect should not be ignored (Ambriz-Tututi et al., 2016; Jiang et al., 2020). However, to our best knowledge, only one review summarized the effectiveness of NIBS for chronic non-specific low back pain, and no study summarized its mechanism (Patricio et al., 2021). Therefore, the aim of this review is to outline the effect of NIBS on patients with CLBP and summarize the possible mechanism of action.
Non-invasive brain neuromodulation for chronic low back pain
Repetitive transcranial magnetic stimulation for chronic low back pain
Repetitive transcranial magnetic stimulation, an FDA-approved NIBS technique, alters the excitability of the cerebral cortex by generating strong magnetic and electric fields through the stimulation coil applied over the scalp (Klomjai et al., 2015). Generally, rTMS can be divided into high-frequency rTMS (>1 Hz, HF-rTMS) and low-frequency rTMS (≤1 Hz, LF-rTMS) according to their frequencies (Pascual-Leone et al., 1999; Haraldsson et al., 2004; Guse et al., 2010).
Johnson et al. (2006) recruited 17 patients with CLBP and observed a decrease in brief pain ratings after a single session of HF-rTMS stimulation. They also found a remarkable decrease in the temperature for cold pain thresholds and a significant increase in the temperature for heat pain thresholds after a single session of HF-rTMS stimulation (Johnson et al., 2006; Table 1). And pain intensity was remarkably decreased in CLBP patients after repeated HF-rTMS session stimulation. Besides, rTMS had a greater analgesic effect without evident side effects compared with physical therapy (Ambriz-Tututi et al., 2016). Many lines of evidence suggested that the presence of attendant symptoms, such as depression and sleep disturbance, interferes with CLBP treatment and were associated with worse treatment outcomes (Sullivan et al., 1992; Nijs et al., 2018). Park et al. (2014) attempted to explore the effects of rTMS in treating depression and insomnia with CLBP and observed positive results. Therefore, rTMS maybe yield an optimal result in the concurrent treatment of these symptoms in patients suffering from CLBP and depression.
These investigations provided preliminary confirmation of the therapeutic effects of rTMS on CLBP. According to the updated guidelines on the therapeutic use of rTMS, level A (definite efficacy) evidence strongly suggested that rTMS is effective for managing neuropathic pain (Lefaucheur et al., 2020; Leung et al., 2020). However, more high-quality and large-scale randomized controlled trial (RCT) studies are needed to further support the benefit of rTMS for CLBP. Besides, no study has compared the effects of different NIBS techniques for CLBP. Whether the use of rTMS may offer more advantages than other NIBS techniques is unknown.
Transcranial direct current stimulation for chronic low back pain
Transcranial direct current stimulation modulates cortical excitability by passing positively or negatively charged currents (a weak current, 0.5–2 mA) using at least two surface electrodes on the scalp (Luedtke et al., 2012b; Chase et al., 2020). In many countries, tDCS can be used as an off-label treatment, and its official regulatory status is under development (Fregni et al., 2015). In the United States, no clinical indications have been approved for the use of tDCS, but tDCS is widely studied for the treatment of chronic pain, and CLBP is not an exception (Pacheco-Barrios et al., 2020).
Some studies used a single session to explore the therapeutic effect of tDCS on CLBP. A single, 20-min session of anodal tDCS stimulation at 2 mA targeting M1 remarkably improved the pain in the tDCS group compared with the sham group (Straudi et al., 2018; Jiang et al., 2020). Schabrun et al. (2014) observed that the analgesic effect of tDCS (a single session, 30 min, 1 mA) was maintained for at least 3 days. But Luedtke et al. (2012a) used a single, 15-min session of M1-tDCS stimulation in CLBP patients, they found that tDCS does not dramatically change the experimentally induced pain. Although they choose the stimulation paradigm most commonly used in most studies on experimentally induced pain in healthy subjects, this stimulation intensity and duration may not be sufficient for patients with chronic pain (Fregni et al., 2006; Soler et al., 2010). Only one meta-analysis summarized the effect of NIBS on CLBP and also found that a single session of NIBS remarkably reduced the pain intensity in patients with CLBP (Patricio et al., 2021). Therefore, these pieces of evidence showed that a single session of tDCS seem to be effective in CBLP treatment.
The effects of multiple sessions of tDCS on CLBP have been investigated, but the immediate changes after intervention are not remarkable (Alwardat et al., 2020; McPhee and Graven-Nielsen, 2021). The therapeutic effect of rTMS could be enhanced by multiple sessions of stimulation (Quesada et al., 2018). Similarly, the effect of tDCS may accumulate over time but may take a longer period to fully manifest (Bikson et al., 2018; Sampaio-Junior et al., 2018). Although Mariano et al. (2019) did not observe an immediate reduction in pain in the tDCS group after 10 sessions of tDCS, there was a remarkable decrease in disability, pain interference, and depression symptoms at 6 weeks of follow-up. Besides, the cognitive function of patients with CLBP appears to have a promotion at 3 days of follow-up after multiple tDCS intervention (O’Connell et al., 2013). This delayed effect also appears in the treatment of depression using rTMS or electroconvulsive therapy. In addition to the delayed effect, the negative results were associated with the less rigid inclusion criteria because of the complexity and heterogeneity of CLBP (Luedtke et al., 2015).
In addition to tDCS as monotherapy, some studies also explored the combined effect of tDCS and other interventions in the treatment of CLBP (Luedtke et al., 2015; Hazime et al., 2017). An increased treatment effect in postural stability, balance, and pain was found by adding tDCS to postural training in patients with CLBP (Jafarzadeh et al., 2019). tDCS remarkably improved the therapeutic effect of group exercises on pain and psychological well-being (particularly depression) in patients with CLBP (Straudi et al., 2018). In addition, a single session of combined tDCS and peripheral electrical stimulation (PES) could significantly increase the pressure pain thresholds and pain-free range of lumbar flexion and decrease the two-point discrimination threshold in CLBP patients compared with PES alone, and the effect was maintained for at least 3 days (Schabrun et al., 2014). Recently, Fregni et al. (2021) proposed that anodal tDCS should be moderately recommended (level B) in reducing chronic pain, such as neuropathic pain, fibromyalgia pain, and migraine pain. But for CLBP, the analgesic effect of tDCS appears to be inadequate. Given the pain relief after a single session of tDCS and the additional effect of tDCS in combination with other therapies on CLBP, the analgesic effect of tDCS for CLBP cannot be entirely denied based on the limited studies (Schabrun et al., 2014; Straudi et al., 2018; Jiang et al., 2020).
Transcranial alternating current stimulation for chronic low back pain
Transcranial alternating current stimulation (tACS) can regulate neural oscillation by applying an alternating current with a sinusoidal pattern to the scalp (Woods et al., 2016). Although it shares basic electrode montage and low-intensity features with tDCS, the functional interpretations of the two electrodes are different (Elyamany et al., 2021). Anodal and cathodal tDCS provide positive and negative currents, respectively. One electrode is an anode, and the other one is a cathode during the half cycle of the tACS oscillation cycle, and this pattern is reversed during the other half of the cycle (Antal and Herrmann, 2016). The use of tACS has a strong theoretical foundation and context for the treatment of chronic pain (Tan et al., 2019; Zhou et al., 2019a). The neural oscillation signals in the alpha and gamma bands during the pre-stimuli period negatively regulate the perception of nociceptive stimuli (Tu et al., 2016). Previous studies suggested a remarkable reduction in the experimental pain induced by pressure pain stimulator in healthy subjects after tACS at alpha frequency (Arendsen et al., 2018). tACS regulates pain intensity by altering neural oscillations (especially in alpha and gamma neural oscillation signals) in patients with chronic pain (Helfrich et al., 2014; Vossen et al., 2015). Although it is appealing, the application of tACS seems to be rare in pain management. Only one study has explored the analgesic effect of tACS on CLBP. Ahn et al. (2019) recruited 20 patients with CLBP and performed a crossover RCT. They observed a substantial pain reduction after a single session of 10 Hz tACS stimulation over the F3 and F4 (10–20 international coordinate system) for 40 min. Moreover, tACS stimulation induced an increase in the intensity of the alpha oscillation signal in the somatosensory area, which was closely associated with pain relief in patients with CLBP (Ahn et al., 2019).
Mechanisms of non-invasive brain neuromodulation for chronic low back pain
Regulation of pain-related signal pathway
Patients with CLBP exhibit changes in brain networks, including pain modulation network, attention network, and default mode network (Kregel et al., 2015; Ng et al., 2018; Vlaeyen et al., 2018). There was a significant difference in functional connectivity in the periaqueductal gray–centered pain modulation network between the patients with CLBP and healthy controls (Yu et al., 2014). This was compatible with the impairments of the descending pain modulation reported in patients with CLBP (Hemington and Coulombe, 2015; Yu et al., 2020). These pieces of evidence indicated the dysfunction in the pain modulatory system in patients with CLBP. Many studies have shown that rTMS and tDCS can modulate neural activity in brain structures associated with pain processing.
HF-rTMS increases cortical excitability in the stimulation site, whereas LF-rTMS stimulation decreases cortical excitability. rTMS can produce a local effect by stimulating neurons directly below the coil and induce distant effects through structural white matter connectivity (Valero-Cabré et al., 2017). Many studies have shown that rTMS can regulate neural activity in certain cortical and subcortical areas (e.g., thalamic and various remote structures) to achieve analgesic effects. In particular, rTMS directly stimulates the thalamus through the corticothalamic projection system to inhibit the transmission of injury information through the spinal thalamic pathway (Bestmann et al., 2004). And the bilateral analgesic effects induced by unilateral rTMS stimulation may be due to the activation of certain structures (e.g., periaqueductal gray) involved in the descending inhibitory controls (Pagano et al., 2012; Moisset et al., 2015).
Cathodal tDCS decreases cortical excitability, whereas anodal tDCS increases cortical excitability; the net effect depends on variations in the overall network balance (Truong and Bikson, 2018). In addition to the modulation of the activity in the stimulated region, tDCS can induce changes in structural and functional connections in unstimulated brain regions (Cummiford et al., 2016; Lin et al., 2017). A single session of M1-anodal tDCS can activate the activity of the left medial prefrontal cortex, right caudate, and pontine nuclei and inhibit the activity of the left precentral gyrus (Meeker et al., 2019). Moreover, pain reduction was related to the decreased functional connectivities of the ventral lateral thalamus to the posterior insula, primary motor cortex, and primary somatosensory cortices (Cummiford et al., 2016). These studies indicated that rTMS and tDCS can induce pain relief by regulating the activity of the primary nociceptive processing and inhibitory regions of the brain (Figure 1).
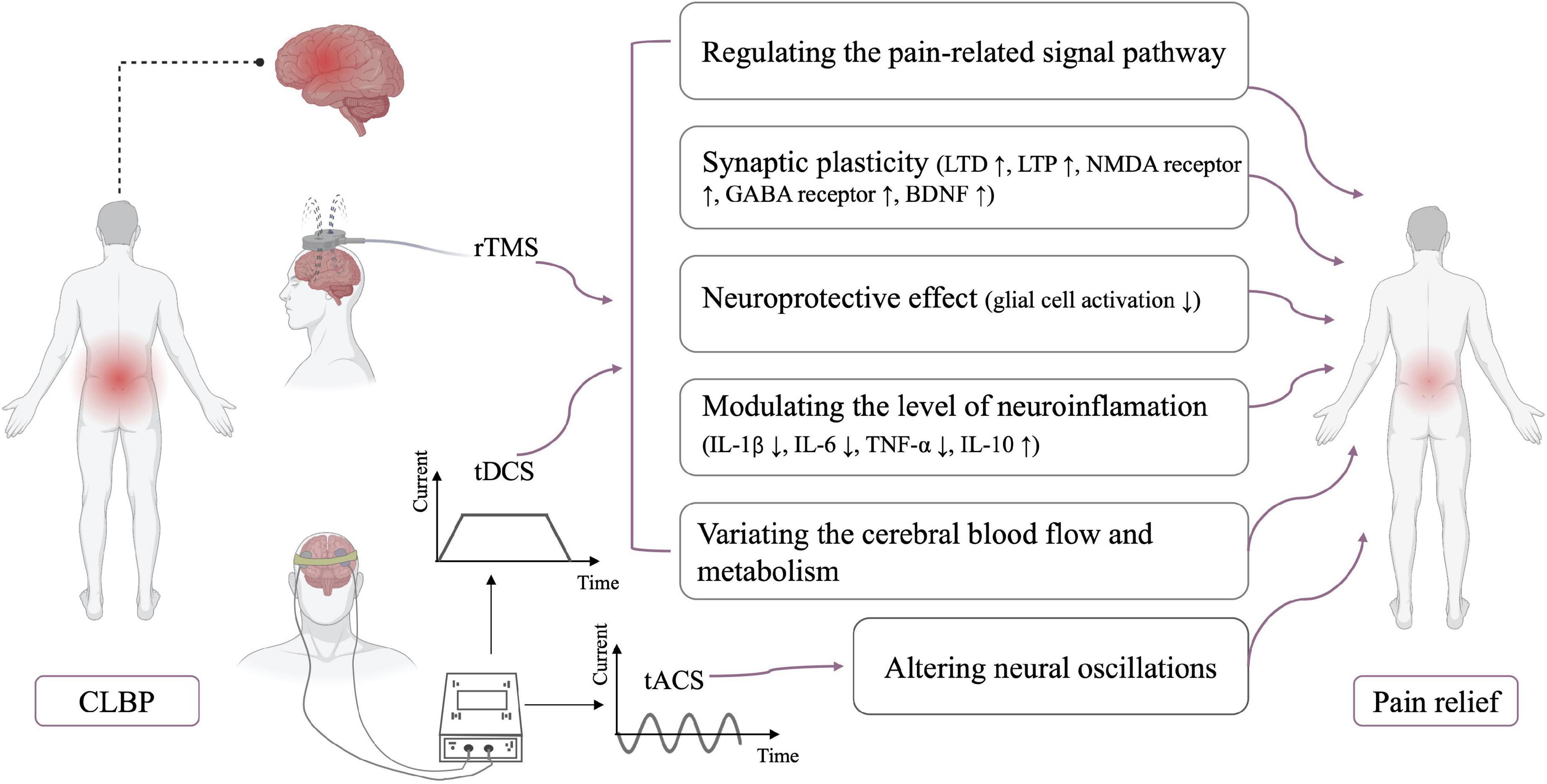
Figure 1. Neurophysiological mechanisms of NIBS. CLBP, chronic low back pain; LTD, long-term depression; LTP, long-term potentiation; NMDA receptor, N-methyl-D-aspartate receptor; GABA receptor, gamma-aminobutyric acid receptor; BDNF, brain-derived neurotrophic factor; IL, interleukin; TNF, tumor necrosis factor.
Synaptic plasticity
The subsequent effects of rTMS and tDCS beyond the stimulation period have been considered as the result of long-term synaptic plasticity (Hosomi et al., 2013; Agboada et al., 2020; Sudbrack-Oliveira et al., 2021). The mechanisms of synaptic plasticity are very complex, and many factors can induce long-term synaptic plasticity, especially the long-term potentiation (LTP) and long-term depression (LTD) phenomena (Connor and Wang, 2016). Similar to basic synaptic physiology, LTP enhances synaptic strength, whereas LTD results in the reduction of synaptic strength (Duffau, 2006). LTP is usually caused by high-frequency rTMS, whereas LTD is induced by low-frequency rTMS (Stanton and Sejnowski, 1989; Artola and Singer, 1993). rTMS and tDCS interact with a variety of neurotransmitters, such as the glutamatergic and GABAergic agents. N-methyl-D-aspartate (NMDA) receptor is among the major molecular channels controlling synaptic plasticity, and the after-effects of rTMS and tDCS are dependent on the NMDA receptor (Stagg and Nitsche, 2011; Muller et al., 2014). Furthermore, the analgesic effect of rTMS is attenuated by the use of glutamate antagonists (such as ketamine) prior to rTMS stimulation (Ciampi de Andrade et al., 2014). rTMS enhanced and reduced motor cortex excitability after using type A receptors for gamma-aminobutyric acid (GABA) receptor agonist and antagonist, respectively (Hsieh et al., 2012). The enhanced and prolonged effects of tDCS are also observed after the administration of GABA receptor agonist, lorazepam (Nitsche et al., 2004). rTMS/tDCS regulates NMDA expression and GABA release to induce LTP or LTD, leading to synaptic plasticity. In addition, brain-derived neurotrophic factor (BDNF) is believed to be an important driving force behind synaptic plasticity (Kowiański et al., 2018; Zhou et al., 2019b). Animal studies also showed that rTMS and tDCS could modulate BDNF expression to enhance synaptic plasticity (da Silva Moreira et al., 2016; Shang et al., 2016).
Neuroprotective effect
Glial activation is present in various chronic diseases, including CLBP (Grace, 2019; Albrecht et al., 2021). Loggia et al. (2015) found an increased level of glial cell activation marker (translocator protein) in the brains of patients with CLBP. Some studies confirmed that glial cell activation is the key factor in the development of chronic pain (Grace, 2019; Torrado-Carvajal et al., 2021). Activated glial cells (such as microglia and astrocytes) can produce various toxic substances, such as cytokines and nitric oxide, thereby aggravating apoptosis (Watkins et al., 2007). Suppression of microglia and astrocyte activation can reverse or reduce chronic pain (Raghavendra et al., 2003; Zheng et al., 2021). Some studies suggested that rTMS may reduce pain by inhibiting the activity and proliferation of microglia and astrocyte in the L4–L6 dorsal and ventral horns of the spinal cord (Kim et al., 2013; Yang et al., 2018). Besides, the activation level of astrocytes in the cerebrospinal fluid was significantly decreased after a single session of tDCS stimulation (Callai et al., 2022). In addition, tDCS and rTMS improved the B-cell lymphoma-2/Bcl2-associated X ratio and decreased apoptosis (Yoon et al., 2011; Zhang et al., 2020). The above studies suggested that rTMS and tDCS may induce a neuroprotective effect.
Modulation of neuroinflammation
Mounting evidence suggests that neuroinflammation is associated with CLBP (Torrado-Carvajal et al., 2021; Alshelh et al., 2022). Variations in neuroinflammation level were found in tDCS studies (Cioato et al., 2016; Zhang et al., 2020; Callai et al., 2022). Zhang et al. (2020) found a remarkable reduction in the levels of pro-inflammatory cytokines, such as IL-1β, IL-6, and TNF-α, and a substantial increase in the level of anti-inflammatory cytokines, such as IL-10, after tDCS stimulation. Animal studies also revealed an increase in IL-10 in the prefrontal cortex after rTMS stimulation. This variation in neuroinflammation level may be related to the partial reversal of mechanical ectopic pain and hyperalgesia by rTMS (Toledo et al., 2021). These pieces of evidence may be suggested that NIBS can modulate neuroinflammation.
Variations in cerebral blood flow and metabolism
Some studies reported that the analgesic effects of rTMS and tDCS may be correlated to variations in regional cerebral blood flow and metabolism. After M1-rTMS stimulation, cerebral blood flow was remarkably increased in the right anterior cingulate cortex and contralateral premotor area but substantially decreased in the right medial prefrontal cortex. Pain reduction was considerably associated with variations in the cerebral blood flow in the anterior cingulate cortex (Tamura et al., 2004). Similarly, the tDCS also induced changes in regional cerebral blood flow (Zheng et al., 2011). Additionally, compared with sham stimulation, active tDCS caused an increase in metabolism in the subgenual anterior cingulate cortex, insula, and medulla and a reduction in metabolism in the left dorsolateral prefrontal cortex in patients with chronic pain (Yoon et al., 2014).
Conclusion and future directions
This review described the effect of NIBS in patients with CLBP and discussed its possible mechanism of action. Overall, NIBS may be effective for CLBP management, and further studies on the effect of NIBS are needed, particularly RCTs with high quality and large sample size. Relatively few studies have examined the analgesic effect of rTMS on patients with CLBP and found promising results. The analgesic effect of tDCS appears to be suboptimal, but the effect of tDCS on CLBP cannot be entirely denied based on the limited studies. No study has compared the efficacy of different NIBS techniques in patients with CLBP. Further studies are warranted to fill this gap, and more different stimulus paradigms are needed to explore the optimal parameters of tDCS and rTMS. In addition, although the analgesic mechanism of NIBS is not understood, the mechanism of NIBS may involve the regulation of pain signal pathway, synaptic plasticity, neuroprotective effect, neuroinflammation modulation, and variations in cerebral blood flow and metabolism. The mechanisms of NIBS for CLBP can be investigated in the future by combining NIBS with imaging or electrophysiology.
Author contributions
T-TC and Y-HC drafted the manuscript and searched the literature to identify eligible trials. T-TC and S-HD extracted and analyzed the data. X-QW and P-JC conceived and revised this review. X-QW received the funding for this study. All authors read and approved the final manuscript.
Funding
The authors disclosed receipt of financial support from the following for the research, authorship and/or publication of this article: The scientific and technological research program of the Shanghai Science and Technology Committee (fund numbers: 19080503100 and 21S31902400), the Shanghai Key Lab of Human Performance (Shanghai University of Sport, fund number: 11DZ2261100), Shanghai Frontiers Science Research Base of Exercise and Metabolic Health, Talent Development Fund of Shanghai Municipal (fund number: 2021081), and Shanghai Clinical Research Center for Rehabilitation Medicine (fund number: 21MC1930200).
Acknowledgments
The authors thank all the participants and clinical researchers involved in the publications cited in this review and peer reviewers who contributed to the continuous improvement of this article.
Conflict of interest
The authors declare that the research was conducted in the absence of any commercial or financial relationships that could be construed as a potential conflict of interest.
Publisher’s note
All claims expressed in this article are solely those of the authors and do not necessarily represent those of their affiliated organizations, or those of the publisher, the editors and the reviewers. Any product that may be evaluated in this article, or claim that may be made by its manufacturer, is not guaranteed or endorsed by the publisher.
References
Agboada, D., Mosayebi-Samani, M., Kuo, M. F., and Nitsche, M. A. (2020). Induction of long-term potentiation-like plasticity in the primary motor cortex with repeated anodal transcranial direct current stimulation-Better effects with intensified protocols? Brain Stimul. 13, 987–997. doi: 10.1016/j.brs.2020.04.009
Ahn, S., Prim, J. H., Alexander, M. L., McCulloch, K. L., and Fröhlich, F. (2019). Identifying and engaging neuronal oscillations by transcranial alternating current stimulation in patients with chronic low back pain: A randomized, crossover, double-blind, sham-controlled pilot study. J. Pain 20, 277.e1–277.e11. doi: 10.1016/j.jpain.2018.09.004
Albrecht, D. S., Kim, M., Akeju, O., Torrado-Carvajal, A., Edwards, R. R., Zhang, Y., et al. (2021). The neuroinflammatory component of negative affect in patients with chronic pain. Mol. Psychiatry 26, 864–874. doi: 10.1038/s41380-019-0433-1
Alshelh, Z., Brusaferri, L., Saha, A., Morrissey, E., Knight, P., Kim, M., et al. (2022). Neuroimmune signatures in chronic low back pain subtypes. Brain 145, 1098–1110. doi: 10.1093/brain/awab336
Alwardat, M., Pisani, A., Etoom, M., Carpenedo, R., Chinè, E., Dauri, M., et al. (2020). Is transcranial direct current stimulation (tDCS) effective for chronic low back pain? A systematic review and meta-analysis. J. Neural Transm. 127, 1257–1270. doi: 10.1007/s00702-020-02223-w
Ambriz-Tututi, M., Alvarado-Reynoso, B., and Drucker-Colín, R. (2016). Analgesic effect of repetitive transcranial magnetic stimulation (rTMS) in patients with chronic low back pain. Bioelectromagnetics 37, 527–535. doi: 10.1002/bem.22001
Andersson, G. B. (1999). Epidemiological features of chronic low-back pain. Lancet 354, 581–585. doi: 10.1016/s0140-6736(99)01312-4
Antal, A., and Herrmann, C. S. (2016). Transcranial alternating current and random noise stimulation: Possible mechanisms. Neural Plast. 2016:3616807. doi: 10.1155/2016/3616807
Arendsen, L. J., Hugh-Jones, S., and Lloyd, D. M. (2018). Transcranial alternating current stimulation at alpha frequency reduces pain when the intensity of pain is uncertain. J. Pain 19, 807–818. doi: 10.1016/j.jpain.2018.02.014
Artola, A., and Singer, W. (1993). Long-term depression of excitatory synaptic transmission and its relationship to long-term potentiation. Trends Neurosci. 16, 480–487. doi: 10.1016/0166-2236(93)90081-v
Balagué, F., Mannion, A. F., Pellisé, F., and Cedraschi, C. (2012). Non-specific low back pain. Lancet 379, 482–491. doi: 10.1016/s0140-6736(11)60610-7
Bestmann, S., Baudewig, J., Siebner, H. R., Rothwell, J. C., and Frahm, J. (2004). Functional MRI of the immediate impact of transcranial magnetic stimulation on cortical and subcortical motor circuits. Eur. J. Neurosci. 19, 1950–1962. doi: 10.1111/j.1460-9568.2004.03277.x
Bikson, M., Brunoni, A. R., Charvet, L. E., Clark, V. P., Cohen, L. G., Deng, Z. D., et al. (2018). Rigor and reproducibility in research with transcranial electrical stimulation: An NIMH-sponsored workshop. Brain Stimul. 11, 465–480. doi: 10.1016/j.brs.2017.12.008
Callai, E., Zin, L., Catarina, L. S., Ponzoni, D., Gonçalves, C., Vizuete, A., et al. (2022). Evaluation of the immediate effects of a single transcranial direct current stimulation session on astrocyte activation, inflammatory response, and pain threshold in naïve rats. Behav. Brain Res. 428:113880. doi: 10.1016/j.bbr.2022.113880
Chase, H. W., Boudewyn, M. A., Carter, C. S., and Phillips, M. L. (2020). Transcranial direct current stimulation: A roadmap for research, from mechanism of action to clinical implementation. Mol. Psychiatry 25, 397–407. doi: 10.1038/s41380-019-0499-9
Cheng, Y. C., Zeng, B. Y., Hung, C. M., Su, K. P., Wu, Y. C., Tu, Y. K., et al. (2022). Effectiveness and acceptability of noninvasive brain and nerve stimulation techniques for migraine prophylaxis: A network meta-analysis of randomized controlled trials. J. Headache Pain 23:28. doi: 10.1186/s10194-022-01401-3
Ciampi de Andrade, D., Mhalla, A., Adam, F., Texeira, M. J., and Bouhassira, D. (2014). Repetitive transcranial magnetic stimulation induced analgesia depends on N-methyl-D-aspartate glutamate receptors. Pain 155, 598–605. doi: 10.1016/j.pain.2013.12.022
Cioato, S. G., Medeiros, L. F., Marques Filho, P. R., Vercelino, R., de Souza, A., Scarabelot, V. L., et al. (2016). Long-lasting effect of transcranial direct current stimulation in the reversal of hyperalgesia and cytokine alterations induced by the neuropathic pain model. Brain Stimul. 9, 209–217. doi: 10.1016/j.brs.2015.12.001
Connor, S. A., and Wang, Y. T. (2016). A place at the table: LTD as a mediator of memory genesis. Neuroscientist 22, 359–371. doi: 10.1177/1073858415588498
Cummiford, C. M., Nascimento, T. D., Foerster, B. R., Clauw, D. J., Zubieta, J. K., Harris, R. E., et al. (2016). Changes in resting state functional connectivity after repetitive transcranial direct current stimulation applied to motor cortex in fibromyalgia patients. Arthritis Res. Ther. 18:40. doi: 10.1186/s13075-016-0934-0
da Silva Moreira, S. F., Medeiros, L. F., de Souza, A., de Oliveira, C., Scarabelot, V. L., Fregni, F., et al. (2016). Transcranial direct current stimulation (tDCS) neuromodulatory effects on mechanical hyperalgesia and cortical BDNF levels in ovariectomized rats. Life Sci. 145, 233–239. doi: 10.1016/j.lfs.2015.10.011
Duffau, H. (2006). Brain plasticity: From pathophysiological mechanisms to therapeutic applications. J. Clin. Neurosci. 13, 885–897. doi: 10.1016/j.jocn.2005.11.045
Elyamany, O., Leicht, G., Herrmann, C. S., and Mulert, C. (2021). Transcranial alternating current stimulation (tACS): From basic mechanisms towards first applications in psychiatry. Eur. Arch. Psychiatry Clin. Neurosci. 271, 135–156. doi: 10.1007/s00406-020-01209-9
Fregni, F., Boggio, P. S., Lima, M. C., Ferreira, M. J., Wagner, T., Rigonatti, S. P., et al. (2006). A sham-controlled, phase II trial of transcranial direct current stimulation for the treatment of central pain in traumatic spinal cord injury. Pain 122, 197–209. doi: 10.1016/j.pain.2006.02.023
Fregni, F., El-Hagrassy, M. M., Pacheco-Barrios, K., Carvalho, S., Leite, J., Simis, M., et al. (2021). Evidence-based guidelines and secondary meta-analysis for the use of transcranial direct current stimulation in neurological and psychiatric disorders. Int. J. Neuropsychopharmacol. 24, 256–313. doi: 10.1093/ijnp/pyaa051
Fregni, F., Nitsche, M. A., Loo, C. K., Brunoni, A. R., Marangolo, P., Leite, J., et al. (2015). Regulatory considerations for the clinical and research use of transcranial direct current stimulation (tDCS): Review and recommendations from an expert panel. Clin. Res. Regul. Aff. 32, 22–35. doi: 10.3109/10601333.2015.980944
Giesecke, T., Gracely, R. H., Grant, M. A., Nachemson, A., Petzke, F., Williams, D. A., et al. (2004). Evidence of augmented central pain processing in idiopathic chronic low back pain. Arthritis Rheum. 50, 613–623. doi: 10.1002/art.20063
Gore, M., Sadosky, A., Stacey, B. R., Tai, K. S., and Leslie, D. (2012). The burden of chronic low back pain: Clinical comorbidities, treatment patterns, and health care costs in usual care settings. Spine 37, E668–E677. doi: 10.1097/BRS.0b013e318241e5de
Grace, P. M. (2019). A backbone for reverse-translation: Evidence for neuroinflammation in patients with low back pain. Brain Behav. Immun. 75, 8–9. doi: 10.1016/j.bbi.2018.11.001
Guse, B., Falkai, P., and Wobrock, T. (2010). Cognitive effects of high-frequency repetitive transcranial magnetic stimulation: A systematic review. J. Neural Transm. 117, 105–122. doi: 10.1007/s00702-009-0333-7
Haraldsson, H. M., Ferrarelli, F., Kalin, N. H., and Tononi, G. (2004). Transcranial magnetic stimulation in the investigation and treatment of schizophrenia: A review. Schizophr. Res. 71, 1–16. doi: 10.1016/j.schres.2003.10.006
Hartvigsen, J., Hancock, M. J., Kongsted, A., Louw, Q., Ferreira, M. L., Genevay, S., et al. (2018). What low back pain is and why we need to pay attention. Lancet 391, 2356–2367. doi: 10.1016/S0140-6736(18)30480-X
Hazime, F. A., Baptista, A. F., de Freitas, D. G., Monteiro, R. L., Maretto, R. L., Hasue, R. H., et al. (2017). Treating low back pain with combined cerebral and peripheral electrical stimulation: A randomized, double-blind, factorial clinical trial. Eur. J. Pain 21, 1132–1143. doi: 10.1002/ejp.1037
Helfrich, R. F., Schneider, T. R., Rach, S., Trautmann-Lengsfeld, S. A., Engel, A. K., and Herrmann, C. S. (2014). Entrainment of brain oscillations by transcranial alternating current stimulation. Curr. Biol. 24, 333–339. doi: 10.1016/j.cub.2013.12.041
Hemington, K. S., and Coulombe, M. A. (2015). The periaqueductal gray and descending pain modulation: Why should we study them and what role do they play in chronic pain? J. Neurophysiol. 114, 2080–2083. doi: 10.1152/jn.00998.2014
Hosomi, K., Kishima, H., Oshino, S., Hirata, M., Tani, N., Maruo, T., et al. (2013). Cortical excitability changes after high-frequency repetitive transcranial magnetic stimulation for central poststroke pain. Pain 154, 1352–1357. doi: 10.1016/j.pain.2013.04.017
Hsieh, T. H., Dhamne, S. C., Chen, J. J., Pascual-Leone, A., Jensen, F. E., and Rotenberg, A. (2012). A new measure of cortical inhibition by mechanomyography and paired-pulse transcranial magnetic stimulation in unanesthetized rats. J. Neurophysiol. 107, 966–972. doi: 10.1152/jn.00690.2011
Jafarzadeh, A., Ehsani, F., Yosephi, M. H., Zoghi, M., and Jaberzadeh, S. (2019). Concurrent postural training and M1 anodal transcranial direct current stimulation improve postural impairment in patients with chronic low back pain. J. Clin. Neurosci. 68, 224–234. doi: 10.1016/j.jocn.2019.07.017
Jenkins, B., and Tepper, S. J. (2011). Neurostimulation for primary headache disorders, part 1: Pathophysiology and anatomy, history of neuromodulation in headache treatment, and review of peripheral neuromodulation in primary headaches. Headache 51, 1254–1266. doi: 10.1111/j.1526-4610.2011.01966.x
Jiang, N., Wei, J., Li, G., Wei, B., Zhu, F. F., and Hu, Y. (2020). Effect of dry-electrode-based transcranial direct current stimulation on chronic low back pain and low back muscle activities: A double-blind sham-controlled study. Restor. Neurol Neurosci. 38, 41–54. doi: 10.3233/rnn-190922
Johnson, S., Summers, J., and Pridmore, S. (2006). Changes to somatosensory detection and pain thresholds following high frequency repetitive TMS of the motor cortex in individuals suffering from chronic pain. Pain 123, 187–192. doi: 10.1016/j.pain.2006.02.030
Katz, J. N. (2006). Lumbar disc disorders and low-back pain: Socioeconomic factors and consequences. J. Bone Joint Surg. Am. 88(Suppl. 2) 21–24. doi: 10.2106/jbjs.E.01273
Kim, J. Y., Choi, G. S., Cho, Y. W., Cho, H., Hwang, S. J., and Ahn, S. H. (2013). Attenuation of spinal cord injury-induced astroglial and microglial activation by repetitive transcranial magnetic stimulation in rats. J. Korean Med. Sci. 28, 295–299. doi: 10.3346/jkms.2013.28.2.295
Klomjai, W., Katz, R., and Lackmy-Vallée, A. (2015). Basic principles of transcranial magnetic stimulation (TMS) and repetitive TMS (rTMS). Ann. Phys. Rehabil. Med. 58, 208–213. doi: 10.1016/j.rehab.2015.05.005
Knezevic, N. N., Candido, K. D., Vlaeyen, J. W. S., Van Zundert, J., and Cohen, S. P. (2021). Low back pain. Lancet 398, 78–92. doi: 10.1016/s0140-6736(21)00733-9
Kowiański, P., Lietzau, G., Czuba, E., Waśkow, M., Steliga, A., and Moryś, J. (2018). BDNF: A key factor with multipotent impact on brain signaling and synaptic plasticity. Cell Mol. Neurobiol. 38, 579–593. doi: 10.1007/s10571-017-0510-4
Kregel, J., Meeus, M., Malfliet, A., Dolphens, M., Danneels, L., Nijs, J., et al. (2015). Structural and functional brain abnormalities in chronic low back pain: A systematic review. Semin. Arthritis Rheum. 45, 229–237. doi: 10.1016/j.semarthrit.2015.05.002
Lefaucheur, J. P., Aleman, A., Baeken, C., Benninger, D. H., Brunelin, J., Di Lazzaro, V., et al. (2020). Evidence-based guidelines on the therapeutic use of repetitive transcranial magnetic stimulation (rTMS): An update (2014-2018). Clin. Neurophysiol. 131, 474–528. doi: 10.1016/j.clinph.2019.11.002
Leung, A., Shirvalkar, P., Chen, R., Kuluva, J., Vaninetti, M., Bermudes, R., et al. (2020). Transcranial magnetic stimulation for pain, headache, and comorbid depression: INS-NANS expert consensus panel review and recommendation. Neuromodulation 23, 267–290. doi: 10.1111/ner.13094
Lin, R. L., Douaud, G., Filippini, N., Okell, T. W., Stagg, C. J., and Tracey, I. (2017). Structural connectivity variances underlie functional and behavioral changes during pain relief induced by neuromodulation. Sci. Rep. 7:41603. doi: 10.1038/srep41603
Loggia, M. L., Chonde, D. B., Akeju, O., Arabasz, G., Catana, C., Edwards, R. R., et al. (2015). Evidence for brain glial activation in chronic pain patients. Brain 138, 604–615. doi: 10.1093/brain/awu377
Luedtke, K., May, A., and Jürgens, T. P. (2012a). No effect of a single session of transcranial direct current stimulation on experimentally induced pain in patients with chronic low back pain–an exploratory study. PLoS One 7:e48857. doi: 10.1371/journal.pone.0048857
Luedtke, K., Rushton, A., Wright, C., Geiss, B., Juergens, T. P., and May, A. (2012b). Transcranial direct current stimulation for the reduction of clinical and experimentally induced pain: A systematic review and meta-analysis. Clin. J. Pain 28, 452–461. doi: 10.1097/AJP.0b013e31823853e3
Luedtke, K., Rushton, A., Wright, C., Jürgens, T., Polzer, A., Mueller, G., et al. (2015). Effectiveness of transcranial direct current stimulation preceding cognitive behavioural management for chronic low back pain: Sham controlled double blinded randomised controlled trial. BMJ 350:h1640. doi: 10.1136/bmj.h1640
Mariano, T. Y., Burgess, F. W., Bowker, M., Kirschner, J., Van’t Wout-Frank, M., Jones, R. N., et al. (2019). Transcranial direct current stimulation for affective symptoms and functioning in chronic low back pain: A pilot double-blinded, randomized, placebo-controlled trial. Pain Med. 20, 1166–1177. doi: 10.1093/pm/pny188
McPhee, M. E., and Graven-Nielsen, T. (2021). Medial prefrontal high-definition transcranial direct current stimulation to improve pain modulation in chronic low back pain: A pilot randomized double-blinded placebo-controlled crossover trial. J. Pain 22, 952–967. doi: 10.1016/j.jpain.2021.02.012
Meeker, T. J., Keaser, M. L., Khan, S. A., Gullapalli, R. P., Seminowicz, D. A., and Greenspan, J. D. (2019). Non-invasive motor cortex neuromodulation reduces secondary hyperalgesia and enhances activation of the descending pain modulatory network. Front. Neurosci. 13:467. doi: 10.3389/fnins.2019.00467
Moisset, X., Goudeau, S., Poindessous-Jazat, F., Baudic, S., Clavelou, P., and Bouhassira, D. (2015). Prolonged continuous theta-burst stimulation is more analgesic than ‘classical’ high frequency repetitive transcranial magnetic stimulation. Brain stimul. 8, 135–141. doi: 10.1016/j.brs.2014.10.006
Morlion, B. (2013). Chronic low back pain: Pharmacological, interventional and surgical strategies. Nat. Rev. Neurol 9, 462–473. doi: 10.1038/nrneurol.2013.130
Muller, P. A., Dhamne, S. C., Vahabzadeh-Hagh, A. M., Pascual-Leone, A., Jensen, F. E., and Rotenberg, A. (2014). Suppression of motor cortical excitability in anesthetized rats by low frequency repetitive transcranial magnetic stimulation. PLoS One 9:e91065. doi: 10.1371/journal.pone.0091065
Ng, S. K., Urquhart, D. M., Fitzgerald, P. B., Cicuttini, F. M., Hussain, S. M., and Fitzgibbon, B. M. (2018). The relationship between structural and functional brain changes and altered emotion and cognition in chronic low back pain brain changes: A systematic review of MRI and fMRI studies. Clin. J. Pain 34, 237–261. doi: 10.1097/ajp.0000000000000534
Nijs, J., Mairesse, O., Neu, D., Leysen, L., Danneels, L., Cagnie, B., et al. (2018). Sleep disturbances in chronic pain: Neurobiology, assessment, and treatment in physical therapist practice. Phys. Ther. 98, 325–335. doi: 10.1093/ptj/pzy020
Nitsche, M. A., Liebetanz, D., Schlitterlau, A., Henschke, U., Fricke, K., Frommann, K., et al. (2004). GABAergic modulation of DC stimulation-induced motor cortex excitability shifts in humans. Eur. J. Neurosci. 19, 2720–2726. doi: 10.1111/j.0953-816X.2004.03398.x
O’Connell, N. E., Cossar, J., Marston, L., Wand, B. M., Bunce, D., De Souza, L. H., et al. (2013). Transcranial direct current stimulation of the motor cortex in the treatment of chronic nonspecific low back pain: A randomized, double-blind exploratory study. Clin. J. Pain 29, 26–34. doi: 10.1097/AJP.0b013e318247ec09
O’Connell, N. E., Marston, L., Spencer, S., DeSouza, L. H., and Wand, B. M. (2018). Non-invasive brain stimulation techniques for chronic pain. Cochrane Database Syst. Rev. 4:CD008208. doi: 10.1002/14651858.CD008208.pub5
Pacheco-Barrios, K., Cardenas-Rojas, A., Thibaut, A., Costa, B., Ferreira, I., Caumo, W., et al. (2020). Methods and strategies of tDCS for the treatment of pain: Current status and future directions. Exp. Rev. Med. Devices 17, 879–898. doi: 10.1080/17434440.2020.1816168
Pagano, R. L., Fonoff, E. T., Dale, C. S., Ballester, G., Teixeira, M. J., and Britto, L. (2012). Motor cortex stimulation inhibits thalamic sensory neurons and enhances activity of PAG neurons: Possible pathways for antinociception. Pain 153, 2359–2369. doi: 10.1016/j.pain.2012.08.002
Park, E. J., Lee, S. J., Koh, D. Y., and Han, Y. M. (2014). Repetitive transcranial magnetic stimulation to treat depression and insomnia with chronic low back pain. Korean J. Pain 27, 285–289. doi: 10.3344/kjp.2014.27.3.285
Pascual-Leone, A., Tarazona, F., Keenan, J., Tormos, J. M., Hamilton, R., and Catala, M. D. (1999). Transcranial magnetic stimulation and neuroplasticity. Neuropsychologia 37, 207–217. doi: 10.1016/s0028-3932(98)00095-5
Patricio, P., Roy, J. S., Rohel, A., Gariépy, C., Émond, C., Hamel, É, et al. (2021). The effect of noninvasive brain stimulation to reduce nonspecific low back pain: A systematic review and meta-analysis. Clin. J. Pain 37, 475–485. doi: 10.1097/ajp.0000000000000934
Quesada, C., Pommier, B., Fauchon, C., Bradley, C., Créac’h, C., Vassal, F., et al. (2018). Robot-guided neuronavigated repetitive transcranial magnetic stimulation (rTMS) in central neuropathic pain. Arch. Phys. Med. Rehabil. 99, 2203.e–2215.e. doi: 10.1016/j.apmr.2018.04.013
Raghavendra, V., Tanga, F., and DeLeo, J. A. (2003). Inhibition of microglial activation attenuates the development but not existing hypersensitivity in a rat model of neuropathy. J. Pharmacol. Exp. Ther. 306, 624–630. doi: 10.1124/jpet.103.052407
Romanella, S. M., Sprugnoli, G., Ruffini, G., Seyedmadani, K., Rossi, S., and Santarnecchi, E. (2020). Noninvasive brain stimulation & space exploration: Opportunities and challenges. Neurosci. Biobehav. Rev. 119, 294–319. doi: 10.1016/j.neubiorev.2020.09.005
Sampaio-Junior, B., Tortella, G., Borrione, L., Moffa, A. H., Machado-Vieira, R., Cretaz, E., et al. (2018). Efficacy and safety of transcranial direct current stimulation as an add-on treatment for bipolar depression: A randomized clinical trial. JAMA. Psychiatry 75, 158–166. doi: 10.1001/jamapsychiatry.2017.4040
Schabrun, S. M., Jones, E., Elgueta Cancino, E. L., and Hodges, P. W. (2014). Targeting chronic recurrent low back pain from the top-down and the bottom-up: A combined transcranial direct current stimulation and peripheral electrical stimulation intervention. Brain Stimul. 7, 451–459. doi: 10.1016/j.brs.2014.01.058
Seminowicz, D. A., Wideman, T. H., Naso, L., Hatami-Khoroushahi, Z., Fallatah, S., Ware, M. A., et al. (2011). Effective treatment of chronic low back pain in humans reverses abnormal brain anatomy and function. J. Neurosci. 31, 7540–7550. doi: 10.1523/jneurosci.5280-10.2011
Shang, Y., Wang, X., Shang, X., Zhang, H., Liu, Z., Yin, T., et al. (2016). Repetitive transcranial magnetic stimulation effectively facilitates spatial cognition and synaptic plasticity associated with increasing the levels of BDNF and synaptic proteins in Wistar rats. Neurobiol. Learn. Mem. 134, 369–378. doi: 10.1016/j.nlm.2016.08.016
Smith, J. A., Stabbert, H., Bagwell, J. J., Teng, H. L., Wade, V., and Lee, S. P. (2022). Do people with low back pain walk differently? A systematic review and meta-analysis. J. Sport Health Sci. 11, 450–465. doi: 10.1016/j.jshs.2022.02.001
Soler, M. D., Kumru, H., Pelayo, R., Vidal, J., Tormos, J. M., Fregni, F., et al. (2010). Effectiveness of transcranial direct current stimulation and visual illusion on neuropathic pain in spinal cord injury. Brain 133, 2565–2577. doi: 10.1093/brain/awq184
Stagg, C. J., and Nitsche, M. A. (2011). Physiological basis of transcranial direct current stimulation. Neuroscientist 17, 37–53. doi: 10.1177/1073858410386614
Stanton, P. K., and Sejnowski, T. J. (1989). Associative long-term depression in the hippocampus induced by hebbian covariance. Nature 339, 215–218. doi: 10.1038/339215a0
Straudi, S., Buja, S., Baroni, A., Pavarelli, C., Pranovi, G., Fregni, F., et al. (2018). The effects of transcranial direct current stimulation (tDCS) combined with group exercise treatment in subjects with chronic low back pain: A pilot randomized control trial. Clin. Rehabil. 32, 1348–1356. doi: 10.1177/0269215518777881
Sudbrack-Oliveira, P., Razza, L. B., and Brunoni, A. R. (2021). Non-invasive cortical stimulation: Transcranial direct current stimulation (tDCS). Int. Rev. Neurobiol. 159, 1–22. doi: 10.1016/bs.irn.2021.01.001
Sullivan, M. J. L., Reesor, K., Mikail, S., and Fisher, R. (1992). The treatment of depression in chronic low back pain: Review and recommendations. Pain 50, 5–13. doi: 10.1016/0304-3959(92)90107-m
Tamura, Y., Okabe, S., Ohnishi, T., Saito, D. N., Arai, N., Mochio, S., et al. (2004). Effects of 1-Hz repetitive transcranial magnetic stimulation on acute pain induced by capsaicin. Pain 107, 107–115. doi: 10.1016/j.pain.2003.10.011
Tan, L. L., Oswald, M. J., Heinl, C., Retana Romero, O. A., Kaushalya, S. K., Monyer, H., et al. (2019). Gamma oscillations in somatosensory cortex recruit prefrontal and descending serotonergic pathways in aversion and nociception. Nat. Commun. 10:983. doi: 10.1038/s41467-019-08873-z
Toledo, R. S., Stein, D. J., Sanches, P. R. S., da Silva, L. S., Medeiros, H. R., Fregni, F., et al. (2021). rTMS induces analgesia and modulates neuroinflammation and neuroplasticity in neuropathic pain model rats. Brain Res. 1762:147427. doi: 10.1016/j.brainres.2021.147427
Torrado-Carvajal, A., Toschi, N., Albrecht, D. S., Chang, K., Akeju, O., Kim, M., et al. (2021). Thalamic neuroinflammation as a reproducible and discriminating signature for chronic low back pain. Pain 162, 1241–1249. doi: 10.1097/j.pain.0000000000002108
Truong, D. Q., and Bikson, M. (2018). Physics of transcranial direct current stimulation devices and their history. J. ECT 34, 137–143. doi: 10.1097/yct.0000000000000531
Tu, Y., Zhang, Z., Tan, A., Peng, W., Hung, Y. S., Moayedi, M., et al. (2016). Alpha and gamma oscillation amplitudes synergistically predict the perception of forthcoming nociceptive stimuli. Hum. Brain Mapp. 37, 501–514. doi: 10.1002/hbm.23048
Valero-Cabré, A., Amengual, J. L., Stengel, C., Pascual-Leone, A., and Coubard, O. A. (2017). Transcranial magnetic stimulation in basic and clinical neuroscience: A comprehensive review of fundamental principles and novel insights. Neurosci. Biobehav. Rev. 83, 381–404. doi: 10.1016/j.neubiorev.2017.10.006
Vlaeyen, J. W. S., Maher, C. G., Wiech, K., Van Zundert, J., Meloto, C. B., Diatchenko, L., et al. (2018). Low back pain. Nat. Rev. Dis. Primers 4:52. doi: 10.1038/s41572-018-0052-1
Vossen, A., Gross, J., and Thut, G. (2015). Alpha power increase after transcranial alternating current stimulation at alpha frequency (α-tACS) reflects plastic changes rather than entrainment. Brain Stimul. 8, 499–508. doi: 10.1016/j.brs.2014.12.004
Watkins, L. R., Hutchinson, M. R., Ledeboer, A., Wieseler-Frank, J., Milligan, E. D., and Maier, S. F. (2007). Norman cousins lecture. Glia as the “bad guys”: Implications for improving clinical pain control and the clinical utility of opioids.. Brain Behav. Immun. 21, 131–146. doi: 10.1016/j.bbi.2006.10.011
Woods, A. J., Antal, A., Bikson, M., Boggio, P. S., Brunoni, A. R., Celnik, P., et al. (2016). A technical guide to tDCS, and related non-invasive brain stimulation tools. Clin. Neurophysiol. 127, 1031–1048. doi: 10.1016/j.clinph.2015.11.012
Yang, L., Wang, S. H., Hu, Y., Sui, Y. F., Peng, T., and Guo, T. C. (2018). Effects of repetitive transcranial magnetic stimulation on astrocytes proliferation and nNOS expression in neuropathic pain rats. Curr. Med. Sci. 38, 482–490. doi: 10.1007/s11596-018-1904-3
Yoon, E. J., Kim, Y. K., Kim, H. R., Kim, S. E., Lee, Y., and Shin, H. I. (2014). Transcranial direct current stimulation to lessen neuropathic pain after spinal cord injury: A mechanistic PET study. Neurorehabil. Neural Repair 28, 250–259. doi: 10.1177/1545968313507632
Yoon, K. J., Lee, Y. T., and Han, T. R. (2011). Mechanism of functional recovery after repetitive transcranial magnetic stimulation (rTMS) in the subacute cerebral ischemic rat model: Neural plasticity or anti-apoptosis? Exp. Brain Res. 214, 549–556. doi: 10.1007/s00221-011-2853-2
Yu, R., Gollub, R. L., Spaeth, R., Napadow, V., Wasan, A., and Kong, J. (2014). Disrupted functional connectivity of the periaqueductal gray in chronic low back pain. Neuroimage Clin. 6, 100–108. doi: 10.1016/j.nicl.2014.08.019
Yu, S., Ortiz, A., Gollub, R. L., Wilson, G., Gerber, J., Park, J., et al. (2020). Acupuncture treatment modulates the connectivity of key regions of the descending pain modulation and reward systems in patients with chronic low back pain. J. Clin. Med. 9:1719. doi: 10.3390/jcm9061719
Zhang, K. L., Yuan, H., Wu, F. F., Pu, X. Y., Liu, B. Z., Li, Z., et al. (2021). Analgesic effect of noninvasive brain stimulation for neuropathic pain patients: A systematic review. Pain Ther. 10, 315–332. doi: 10.1007/s40122-021-00252-1
Zhang, K. Y., Rui, G., Zhang, J. P., Guo, L., An, G. Z., Lin, J. J., et al. (2020). Cathodal tDCS exerts neuroprotective effect in rat brain after acute ischemic stroke. BMC Neurosci. 21:21. doi: 10.1186/s12868-020-00570-8
Zheng, X., Alsop, D. C., and Schlaug, G. (2011). Effects of transcranial direct current stimulation (tDCS) on human regional cerebral blood flow. Neuroimage 58, 26–33. doi: 10.1016/j.neuroimage.2011.06.018
Zheng, Y., Jia, C., Jiang, X., Chen, J., Chen, X. L., Ying, X., et al. (2021). Electroacupuncture effects on the P2X4R pathway in microglia regulating the excitability of neurons in the substantia gelatinosa region of rats with spinal nerve ligation. Mol. Med. Rep. 23:175. doi: 10.3892/mmr.2020.11814
Zhou, H., Zhang, Q., Martinez, E., Dale, J., Robinson, E., Huang, D., et al. (2019a). A novel neuromodulation strategy to enhance the prefrontal control to treat pain. Mol. Pain 15:1744806919845739. doi: 10.1177/1744806919845739
Keywords: pain, mechanisms, brain neuromodulation, rTMS, tDCS, low back pain
Citation: Chang T-T, Chang Y-H, Du S-H, Chen P-J and Wang X-Q (2022) Non-invasive brain neuromodulation techniques for chronic low back pain. Front. Mol. Neurosci. 15:1032617. doi: 10.3389/fnmol.2022.1032617
Received: 31 August 2022; Accepted: 03 October 2022;
Published: 19 October 2022.
Edited by:
Ma Ke, Shanghai Jiao Tong University, ChinaReviewed by:
Kai Yu, Carnegie Mellon University, United StatesCopyright © 2022 Chang, Chang, Du, Chen and Wang. This is an open-access article distributed under the terms of the Creative Commons Attribution License (CC BY). The use, distribution or reproduction in other forums is permitted, provided the original author(s) and the copyright owner(s) are credited and that the original publication in this journal is cited, in accordance with accepted academic practice. No use, distribution or reproduction is permitted which does not comply with these terms.
*Correspondence: Pei-Jie Chen, Y2hlbnBlaWppZUBzdXMuZWR1LmNu; Xue-Qiang Wang, d2FuZ3h1ZXFpYW5nQHN1cy5lZHUuY24=