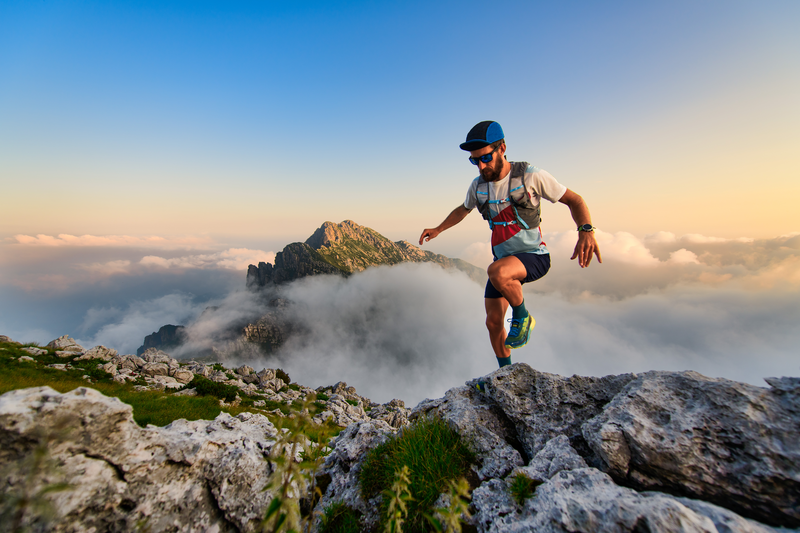
95% of researchers rate our articles as excellent or good
Learn more about the work of our research integrity team to safeguard the quality of each article we publish.
Find out more
ORIGINAL RESEARCH article
Front. Mol. Neurosci. , 27 October 2022
Sec. Pain Mechanisms and Modulators
Volume 15 - 2022 | https://doi.org/10.3389/fnmol.2022.1029278
This article is part of the Research Topic Molecular and Cellular Bases of Peripheral Neuropathies View all 9 articles
Sexual dimorphism is a powerful yet understudied factor that influences the timing and efficiency of gene regulation in axonal injury and repair processes in the peripheral nervous system. Here, we identified common and distinct biological processes in female and male degenerating (distal) nerve stumps based on a snapshot of transcriptional reprogramming 24 h after axotomy reflecting the onset of early phase Wallerian degeneration (WD). Females exhibited transcriptional downregulation of a larger number of genes than males. RhoGDI, ERBB, and ERK5 signaling pathways increased activity in both sexes. Males upregulated genes and canonical pathways that exhibited robust baseline expression in females in both axotomized and sham nerves, including signaling pathways controlled by neuregulin and nerve growth factors. Cholesterol biosynthesis, reelin signaling, and synaptogenesis signaling pathways were downregulated in females. Signaling by Rho Family GTPases, cAMP-mediated signaling, and sulfated glycosaminoglycan biosynthesis were downregulated in both sexes. Estrogens potentially influenced sex-dependent injury response due to distinct regulation of estrogen receptor expression. A crosstalk of cytokines and growth hormones could promote sexually dimorphic transcriptional responses. We highlighted prospective regulatory activities due to protein phosphorylation, extracellular proteolysis, sex chromosome-specific expression, major urinary proteins (MUPs), and genes involved in thyroid hormone metabolism. Combined with our earlier findings in the corresponding dorsal root ganglia (DRG) and regenerating (proximal) nerve stumps, sex-specific and universal early phase molecular triggers of WD enrich our knowledge of transcriptional regulation in peripheral nerve injury and repair.
Successful regeneration of a severed peripheral nerve relies on a well-coordinated multicellular distal degeneration process first described by Waller (1850). Significant insight into the cellular and molecular processes of Wallerian degeneration (WD) was obtained using rodent models of the peripheral nerve transection (Fawcett and Keynes, 1990; Stoll et al., 2002; Vargas and Barres, 2007). A high-efficiency acute-phase (within 24 h) peripheral WD process in the distal to transection segment, disconnected from neuronal soma, determines functional repair of the nerve (Gordon et al., 2003; McDonald et al., 2006; Wood et al., 2011; Zochodne, 2012). These prior acute-phase peripheral WD studies were done using rodents of one sex.
Sex-dependent response to peripheral nerve injury in rodents (Sorge et al., 2015; Chernov et al., 2020; Yu et al., 2020; Chernov and Shubayev, 2021, 2022) is thought to relate to sex differences in prevalence, incidence, mechanisms, or clinical presentation of peripheral neuropathies (Unruh, 1996; Greenspan et al., 2007; Fillingim et al., 2009; Mogil, 2012; Sorge and Totsch, 2017; Boerner et al., 2018). The transcriptional landscape of peripheral nerve in control human subjects and patients with radiculopathy assessed by RNA-sequencing (RNA-seq) analyses reveal sexual dimorphism in metabolic, neuroendocrine, immune regulatory, and sex chromosome-related programs both at baseline and in response to peripheral nerve damage (North et al., 2019; Ray et al., 2019; Stephens et al., 2019; Chernov et al., 2020; Mecklenburg et al., 2020; Paige et al., 2020; Tavares-Ferreira et al., 2020; Ahlström et al., 2021). Whether sexual dimorphism in acute-phase WD exists remains not understood.
During an acute-phase (within 24 h) peripheral WD, an immediate influx of extracellular calcium activates calpain- and ubiquitin-proteasome-dependent disintegration of the axonal cytoskeleton. A dominant cell type in peripheral nerve, denervated Schwann cells initiate axonal and myelin breakdown and phagocytosis. In concert with resident macrophages, endothelial cells, and fibroblasts, Schwann cells control a time-dependent and continuous stream of hematogenous immune cells, starting with neutrophils, mast cells, monocytes, and lymphocytes (Hirata and Kawabuchi, 2002; Bauer et al., 2007; Scholz and Woolf, 2007; Barrette et al., 2008; Jessen and Arthur-Farraj, 2019). In addition, Schwann cells engage vast molecular machinery required to support their robust phenotypic changes induced by injury, including de-differentiation, accompanied by myelin protein gene silencing and glial fibrillary acidic protein (GFAP) activation, followed by neuregulin-epidermal growth factor receptor (EGFR, also named ERBB) controlled mitosis, and c-Jun-regulated redifferentiation, axonal partnership and remyelination (Clemence et al., 1989; Triolo et al., 2006). Extensive molecular signatures reveal a dynamic interplay between immune, metabolic, kinase, neurotrophic, and other gene families jointly contributing to the functional activity of many cell types in a time-dependent manner. Thus, within 24 h post-axotomy, calcium- and zinc-dependent activation of extracellular matrix (ECM) remodeling by matrix metalloproteinase (MMP) family of 24 members, which control permeability of blood-nerve and perineurial barriers, Schwann cell signaling, as well as myelin and ion channel proteolysis (Shubayev and Myers, 2002; Myers et al., 2006; Shubayev et al., 2006; Chattopadhyay et al., 2007; Kobayashi et al., 2008; Chattopadhyay and Shubayev, 2009; Liu et al., 2010, 2012; Liu and Shubayev, 2011; Kim et al., 2012; Nishihara et al., 2015; Remacle et al., 2015).
Our comparative high-depth (over 5.0E + 07 paired-end reads per sample) RNA-seq and predictive bioinformatics analyses of the early response genome-wide transcriptional changes at 24 h after sciatic nerve axotomy in male and female mice indicated immediate and sex-dependent control of the transcriptional landscape in dorsal root ganglia (DRG) (Chernov and Shubayev, 2021), regenerating (proximal) nerve segment (Chernov and Shubayev, 2022). The present study of degenerating (distal) identified new sexually dimorphic protein-coding and non-coding (nc)RNAs and respective signaling pathways specific to peripheral nerve remodeling.
To assess sex differences in the early phase peripheral WD transcriptional (protein-coding mRNAs and ncRNA) response, at 24 h after complete sciatic nerve axotomy or sham operation, distal nerve stumps (Figure 1A) were collected in female and male mice (n = 6 mice/group). To obtain the optimal amount of total RNAs for whole-genome transcriptomics analysis by high-depth RNA-seq nerve stumps from two mice were pooled in all RNA samples (n = 3/groups, 2 mice/RNA sample). RNA-seq of the respective lumbar (L) 4/5 DRG and proximal sciatic nerve stumps from the same animal cohorts were reported earlier (Chernov and Shubayev, 2021, 2022).
Figure 1. Global transcriptional profiling in distal nerve 24 h post-axotomy identified sexually dimorphic changes. (A) A schematic of the sciatic nerve axotomy followed by ipsilateral distal stump tissue analysis. RNA-seq of the respective lumbar (L) 4/5 DRG and proximal sciatic nerve stumps from the same animal cohorts were reported earlier (Chernov and Shubayev, 2021, 2022). (B) MA plots display log2FC and normalized counts calculated using the default DESeq2 method. The left and right panels show up- and downregulated genes in females (red dots) and males (blue dots), respectively. Colored dots indicate DEGs with Padj < 0.1. Blue lines correspond to log2FC thresholds above 1 or below –1. (C) Principal component analysis of female (red) and male (blue) groups of sham (rectangles) and axotomy (circles) samples (n = 3 samples/group). Red and blue arrows indicate genes with the most influence on variance in females and males, respectively.
Genes with greater than 10 transcript counts across all samples were used for normalization and identification of differentially expressed genes (DEGs) in axotomy samples relative to respective shams by DESeq2 using Wald’s test (Love et al., 2014). The standard significance criteria Padj < 0.1 and log2FC > 1 were used to identify significant DEGs (Figure 1B) in this report and our prior comparative analyses of regenerating peripheral nerves (Chernov and Shubayev, 2022) and DRG (Chernov and Shubayev, 2021). Baseline gene expression [log2(counts)], log2FC, and significance scores of 1,017 DEGs identified by DESeq2 were used for predictive system analysis (Supplementary Table 1). Comparative analysis of RNA-seq and protein immunoblotting data of select genes was conducted in DRG of the same animal cohort, paired-end library preparation, and RNA-seq. A high level of correlation between mRNA and protein levels was demonstrated in post-axotomy and sham samples (Chernov and Shubayev, 2021).
The principal component (PC) analysis (Figure 1C) identified sex-specific variance-driving DEGs related to ECM homeostasis, nerve regrowth, axonal guidance, and cytokine signaling among the most significant contributors to injury-related transcriptional changes in distal nerve stumps. We predicted that 80.6% (PC1) and 8.9% (PC2) of DEGs could contribute to variance based on axotomy and sex differences. Specifically, sonic hedgehog (Shh), cardiotrophin-like cytokine factor 1 (Clcf1), ECM-localized corticotropin-releasing factor urocortin-2 (Ucn2), and glia-derived neurotrophic factor (Gdnf), leucine-rich repeat-containing protein 15 (Lrrc15), and major urinary proteins Mup18/Mup22 genes were the most significant drivers of sexually dimorphic transcriptional variance. Heatmap histograms ranked by absolute expression in three biological replicates (Figure 2A) and volcano plots (Figure 2B) display DEG’s ranked by statistical significance (Padj) and log2FC ranking.
Figure 2. Nerve axotomy induced distinct sexually dimorphic regulation of DEGs in female and male mice. (A) Hierarchical clustering plot of 100 most significant upregulated DEGs [log2FC > 1, Padj < 0.1, n = 6 mice/group, 2 mice/sample (pooled), 3 sample/group]. Heatmap colors correspond to log2(counts): blue, yellow, and red correspond to low, medium, and high gene expression, respectively. DEGs were clustered based on the Euclidean distance. (B) Significant DEGs in female (left panel) and male (right panel) mice. Volcano scatter plots show -log10Padj and log2FC. Red and green colors indicate up- and down-regulated DEGs, respectively. Thresholds (log2FC > 1 or log2FC < –1) and –log10Padj < 0.1 are indicated by yellow dashed lines. Selected DEG symbols are shown.
In both sexes, we identified high expression levels of genes related to signaling pathways specific to nerve injury response. Some of these pathways exhibited additional activation or inhibition at 24 h post-axotomy as outlined below. RhoGDI, ERBB, ERK5 Signaling pathways, and Epithelial/Mesenchymal Transition by Growth Factors Signaling pathway showed significant activation in both sexes (Figure 3). Compared to males, females demonstrated distinct, albeit mild, upregulation of the Osteoarthritis Signaling, Protein Kinase A Signaling, and Wnt/β-catenin Signaling pathways. Males activated signaling pathways related to Neuregulin Signaling consistent with upregulation of Ereg, Areg, and Hbegf genes (Figure 4). In addition, the activity of HMGB1 Signaling and IL-17 Signaling pathways additionally increased in males due to stronger upregulation of IL-6, LIF, Fos, Fosl1, and related genes. GDNF Family Ligand Receptor Interactions, CSDE1 Signaling, Mouse Embryonic Stem Cell Pluripotency, and Aryl Hydrocarbon Receptor Signaling demonstrated a male-specific up-regulation. Cardiac Hypertrophy Signaling and Wound Healing pathways were activated in males but inhibited in females.
Figure 3. Canonical signaling pathways demonstrate distinct regulation patterns in females and males. Canonical pathways were predicted in IPA and ranked by z-scores. Positive (red) and negative (green) z-scores indicate pathway up-regulation or down-regulation, respectively, according to the color scale. Asterisks indicate significance *, z < –2.0 or z > 2.0; **, z < –3.0, or z > 3.0. The gray color indicates that the directionality of pathway regulation was not determined. Purple (females) and blue (males) horizontal bars correspond to –log10(P) ≥ 1.3 calculated in IPA using Fisher exact test.
Figure 4. Axotomy affected major nerve injury-related canonical pathways. Histograms show DEG specific to canonical pathways highlighted in Figure 3. Heatmaps display normalized log2(counts) in sample groups (FS, female sham; MS, male sham; FA, female axotomy; MA, male axotomy) and log2FC in female (F) and male (M); [n = 6 mice/group, 2 mice/sample (pooled), 3 sample/group] of respective DEGs. Log2FC significance was determined in DESeq2 using the Wald test. DEGs were sorted by log2FC.
Baseline expression levels of DEGs related to Axonal Guidance Signaling in both sexes due to a robust expression of endothelin-converting enzyme Ecel1, Shh, Ngfr, Glis1, and other related genes (Figure 4). Cholesterol Biosynthesis, Reelin Signaling in Neurons, Synaptogenesis Signaling Pathway, and GP6 Signaling pathways were downregulated in females but were not significantly altered in males. Signaling by Rho Family GTPases, cAMP-mediated signaling, and sulfated glycosaminoglycan biosynthesis were downregulated in both sexes. The iodothyronine deiodinase genes Dio2 and Dio2, which regulate thyroid hormone conversion, increased in both sexes, yet the increase was higher in males. It is important to note that many DEGs related to pathways activated in males exhibited robust expression in females in both axotomized and sham nerves (Figure 4). Thus, male-specific post-injury DEG regulation balanced the activity of respective pathways to levels observed in females. The most significant sex-specific DEGs were further highlighted on heatmap diagrams grouped by gene relevance to peripheral nerve injury processes according to IPA predictions and GO terms.
The cascade of predicted upstream transcriptional regulators (small endogenous molecules, proteins, including transcription factors, and RNAs) could explain the observed gene expression changes. According to IPA predictions, growth factors (including Egf, Fgf2, Vegf, Hgf, and others) could contribute to transcriptional regulation in both sexes (Figure 5A). In addition, IPA predicted that steroid hormone β-estradiol could regulate downstream transcription distinctly in females in males. In females, among 144 DEGs targeted by β-estradiol, 44 DEGs were upregulated, and 68 DEGs were downregulated. In males, among 77 genes 64 were upregulated more than twofold but only 12 DEGs were downregulated (Padj < 0.1) (Figure 5B and Supplementary Table 2). The Esr1 gene, encoding one of two main types of nuclear estrogen receptors ERα that transmit β-estradiol signaling in the nucleus (Rettberg et al., 2014), maintained moderate levels of expression in both sexes. Remarkably, the Esr2 gene encoding ERβ demonstrated a 10-fold decrease in females (Figure 6). In males, ERβ expression decreased fivefold, yet a moderate expression level was maintained. We proposed that estrogen receptor signaling could distinctly regulate early phase WD responses in nerves of both sexes, which is modulated, in part, via estrogen receptors’ sexually-dimorphic expression.
Figure 5. Upstream regulators of the injury response in distal nerve segments. (A) Activation (positive z, red color) and inhibition (negative z, green color) z-scores indicate IPA-predicted regulator effects to upregulate or downregulate respective DEGs. Circle diameters correspond to the p–values [as –log10(P) according to a significance scale] that measure the statistical significance of the overlap between the dataset DEGs and all genes regulated by a given regulator calculated using Fisher’s exact test in IPA. (B) Upregulation of DEGs in females (left panels) and males (right panels) (log2FC > 1, Padj < 0.1). Yellow circles correspond to select upstream regulators (beta-estradiol, IL-6, IL-1b, and IL-17) predicted by IPA. All predicted upstream regulators are included in Supplementary Table 2.
Figure 6. Regulated gene families. Heatmaps on panels display normalized log2(counts) in sample groups (FS, female sham; MS, male sham; FA, female axotomy; MA, male axotomy) and log2FC in female (F) and male (M); [n = 6 mice/group, 2 mice/sample (pooled), 3 sample/group] of respective DEGs. Log2FC significance was determine in DESeq2 using Wald test: *p ≤ 0.05; **p ≤ 0.005; ***p ≤ 0.0005. DEGs were sorted by log2FC.
Gene ontology (GO) analysis (Ashburner et al., 2000) identified a relatively small number of regulated DEGs generally attributed to the innate immunity system (GO term 0045087) (Figure 6). Likewise, IPA did not detect significant changes of immune-related canonical pathways (Figure 3). Consistent with time-lapse microarrays in axotomized male rats (Yu et al., 2016), robust baseline expression of pro-inflammatory interleukins IL-1b and IL-6 was observed in both sexes in axotomized and sham mice, albeit post-axotomy activation of IL-6 was stronger in males. Upstream regulator analysis in IPA predicted that interleukins IL-6 and IL-1B could promote upregulation of a larger number of DEGs in males, including important growth and transcription factors (Figure 5B and Supplementary Table 2).
Both females and males attenuated expression levels of kinases, including protein kinases (Figure 6). Males predominantly increased Sphk1 encoding sphingosine kinase 1 that catalyzes the phosphorylation of sphingosine, a lipid mediator with intra- and extracellular functions, including neuroinflammation. The following kinases showed a moderate mRNA increase. The protein kinase adapter gene involved in ubiquitin-dependent protein degradation Trib1 (Satoh et al., 2013), Plk2 (a serine/threonine-protein kinase involved in synaptic plasticity), cyclin-dependent kinase 18 (Cdk18), cGMP-dependent protein kinase 2 (Prkg2), paralemmin A kinase anchor protein (Pakap), and serine/threonine-protein kinase Nek6 important for mitotic cell cycle progression. Females, but not males, exhibited significant downregulation of multiple kinases and kinase-associated protein genes, including Cdkn1c, Plk3, Mapk3k13, Camk2n1, and Camk2b.
In both sexes, MMPs gelatinase A (Mmp2), stromelysin-1 (Mmp3), neutrophil collagenase (Mmp8), gelatinase A (Mmp9), Mmp19, Mmp23, membrane-type MMPs (MT-MMPs) MT1-MMP (Mmp14), MT2-MMP (Mmp15), MT3-MMP (Mmp16), and MT4-MMP (Mmp17) genes exhibited high expression levels in both distal nerve stumps and sham at 24 h (Figure 6). Stromelysin-2 (Mmp10) exhibited a low expression level in both sexes. Several MMPs colocalized in the genomic locus on chromosome 9, Mmp12, Mmp13, and Mmp27, exhibited mild activation in females and a decrease in males. Many ADAM/ADAM-TS genes were expressed at high levels irrespective of sex (Supplementary Table 1). Timp1, Timp2, and Timp3 but not Timp4 expressed at high levels. Timp1 and Timp3 showed a mild increase in both sexes in axotomized nerves. MMP, ADAM/ADAM-TS, and TIMPs gene expression patterns were remarkably consistent in axotomized distal rat nerve stumps (Chernov et al., 2015), suggesting universal proteolytic activities.
Both sexes reduced levels of many myelin-associated genes, including myelin basic protein (MBP), periaxin (Prx), myelin protein zero (Mpz), and myelin-associated glycoprotein (Mag). GFAP maintained high expression in females and was significantly increased in males to a matching mRNA level (Figure 6).
Males, but not females, significantly upregulated Neuregulin Signaling implicated in growth factor-dependent repair and maintenance of the nervous system (Fricker and Bennett, 2011) (Figure 4). Rho GDP-dissociation inhibitor (RhoGDI) encoded by the ARHGDIA gene, p75 neurotrophin receptor (p75NTR, also named NGFR) demonstrated a twofold increase in both sexes. In males, we observed a 4–8-fold increase of the epiregulin (Ereg) and amphiregulin (Areg) autocrine growth factors, ligands of EGF receptor, known to stimulate Schwann cells in axotomized PNS (Rosenbaum et al., 1997; Meier et al., 1999; Jessen and Mirsky, 2021). The proheparin-binding EGF-like growth factor Hbegf associated with macrophage-mediated cellular proliferation exhibited a fourfold increase in males. In addition, probetacellulin (Btc) EGFR ligand showed eightfold upregulation in both sexes.
The GDNF Family Ligand Receptor Interactions signaling is regulated by a spectrum of polypeptide growth factors, including the GDNF (Gordon, 2009). Regulation of Mouse Embryonic Stem Cell Pluripotency was male-dominant potentially due to the increase of LIF extrinsic pluripotency factor accompanied by the induction of the intrinsic transcription factors Sox2 and c-Myc.
As reported in the DRGs (Chernov and Shubayev, 2021) and proximal nerve stumps (Chernov and Shubayev, 2022) of the same animal cohort genomic localization of DEGs on a sex-chromosome could determine sexually dimorphic expression. Because females can up- or down-regulate the dosage of X-linked genes by female-exclusive X-chromosome inactivation epigenetic mechanism, the expression of X-linked genes could exhibit significant sexual dimorphism. In the distal stump, females upregulated two X-linked genes for the gap-junction β-1 protein (Gjb1) and the fibronectin type III domain-containing protein 3C1 gene (Fndc3c1). Gjb1 was also activated in males (Figure 7A). In addition, females reduced the expression of over a dozen genes, including Gm7598, Cltrn, Prrg3, Tmem28, Zcchc18, Col4a5, Fgf16, Tnmd, Cnga2, Itm2a, and Xlr3a that did not significantly change in males. Several Y-linked genes in males were strongly expressed, including lysine (K)-specific demethylase 5D (Kdm5d), eukaryotic translation initiation factor Eif2s3y, DEAD box helicase Ddx3y, and ncRNAs (Gm21860 and Gm47283) in both shams and axotomized nerves.
Figure 7. Regulated X-linked and ncRNA DEGs exhibited sexual dimorphism. (A) Regulated sex chromosome-linked DEGs. Heatmaps display normalized log2(counts) in sample groups (FS, female sham; MS, male sham; FA, female axotomy; MA, male axotomy) and log2FC in female (F) and male (M); [n = 6 mice/group, 2 mice/sample (pooled), 3 sample/group] of respective DEGs. Heatmap colors correspond to the respective scales. Log2FC significance was determine in DESeq2 using Wald test: *p ≤ 0.05; **p ≤ 0.005; ***p ≤ 0.0005. DEGs were sorted by log2FC. Significant ncRNA DEGs in female (B) and male (C) mice. Volcano scatter plots show –log10Padj and log2FC. Red and green colors indicate up- and downregulated ncRNAs, respectively. Thresholds (log2FC > 1 or log2FC < –1) and –log10Padj < 0.1 are shown by dotted lines. Selected DEGs are labeled by gene symbols.
In the distal nerve stump, 172 ncRNA DEGs were detected, including 153 in females, and 51 in males (Figures 7B,C). Reduced expression of opposite strand ncRNA genes occurred in females and included Hoxb3os, H3f3aos, Prdm16os, Rapgef3os2, Tspan32os, Tbx3os1, Pard3bos3, Slc36a1os, Igf1os, and Sap30bpos. Runx2os1, and Junos genes exhibited upregulation in females. Slc36a1os and Igf1os genes were reduced in males.
Interactive network predictions in IPA suggested that networks of cytokines and growth factors, including neuron-specific growth factors, act via their specific receptors to positively regulate neuron growth and proliferation of cells of supporting tissues. The upregulation of these networks was higher in males as compared to females (Figure 8A). Cytokines localized in the extracellular milieu could interact with plasma membrane-associated EGFR, ERBB, and HRAS GTPase and mediation of cytoplasmic and nuclear factors. This regulatory network leads to the activation of axonal guidance, motor neuron outgrowth, mitogenic activity, lipids synthesis, and other pathways promoting axon regrowth. In addition, female-specific networks predicted signaling processes and metabolic changes that could cause a deficit in the myelin maintenance system, leading to pathologic states, such as dysmyelination (Figure 8B).
Figure 8. Crosstalk of cytokines and growth factors could regulate reprogramming in WD. (A) Predicted regulation of male-specific processes based on RNA-seq analysis. (B) Predicted female-specific regulatory network. Networks represent comprehensive synopsis that summarizes the most significant findings in the canonical pathway, upstream regulators, and disease and biological function categories of analysis. Diagrams were constructed in IPA using Graphical Summary function to visualize related biological activities.
Sciatic nerve axotomy triggers a strong sex-specific early phase transcriptional response in regenerating (proximal) nerve stump (Chernov and Shubayev, 2022) and corresponding DRGs (Chernov and Shubayev, 2021). The present study documents sexual dimorphism in early phase WD response in the distal sciatic nerve segment of the same animal cohort.
WD is a prerequisite to a successful peripheral nerve repair post-injury (Gerdts et al., 2016) orchestrated by denervated Schwann cells. Due to their own remarkable plasticity, Schwann cells de-differentiate immediately after injury to acquire an injury-specific phenotype that drives a sequence of reparative processes in both neurons and immune cells (Arthur-Farraj et al., 2012; Jessen et al., 2015). Through a coordinated transcriptional reprogramming, Schwann cells expedite axonal and myelin proteolytic fragmentation, phagocytosis, and secretion of cytokines and chemokines to foster the immune cell recruitment (Tofaris et al., 2002; Chernov et al., 2015; Jessen et al., 2015).
Interleukins IL6 and IL1b could play a significant role to promote transcriptional reprogramming of a large number of genes in WD nerves, especially in males. Except for the moderately upregulated IL-17b, the panel-wide activation of the IL-17 family cytokines was not observed in either sex, consistent with the well-established notion that recruitment of IL-17-expressing immune [T helper (Th)17] cells starts days post-injury (Kim and Moalem-Taylor, 2011). It is important to note, that the upregulation of many cytokine genes in males led to the equalized absolute expression of respective mRNAs to matching levels in both sexes.
Estrogens, including β-estradiol, and targeted genes showed sex-dependent WD response. Both Esr1 and Esr2 are expressed in nerves of both sexes and the corresponding DRGs (Chernov and Shubayev, 2022). Injury induced a 10- and 5-fold decrease in Esr2 in female and male nerves, respectively. Under acute pathological conditions, estrogens regulate the neuronal (Hucho et al., 2006) and immune functions (Straub, 2007). In the corresponding DRGs, Esr1 signaling mediates the female-specific pain hypersensitivity (Khomula et al., 2017; Chernov et al., 2020). In the nervous system, rapid attenuation of estrogens in DRG can be complemented by a neuron-specific β-estradiol biosynthesis (Evrard and Balthazart, 2004). In addition to estrogens of gonadal origin (Rettberg et al., 2014) the downstream signaling is likely influenced by the naturally higher β-estradiol levels in females. We concluded that estrogen signaling is a potent regulator of early phase WD response in nerves of both sexes, which is modulated, in part, via estrogen receptors’ sexually-dimorphic expression.
Sex specificity in Schwann cell signaling, the dominant cell type in the damaged nerve within 24 h post-injury, manifested in myelin protein and growth factor expression patterns. Thus, the GDNF family of neurotrophic factors known as survival factors for neurons, bind to respective receptors, including upregulated NGFR, which could trigger the phosphorylation of RET tyrosine kinase receptor (Durbec et al., 1996) that is implicated in the regulation of PI3k/Akt and Plcγ/Ip3r dependent Ca2+ signaling during neurogenesis (Fukuda et al., 2002; Lundgren et al., 2012) and, sometimes, hyperalgesia (Bogen et al., 2008). In addition, GDNF/Gfra1 could interact with neural cell adhesion molecules such as NCAM to induce an axonal expansion (Nielsen et al., 2009). GDNF family of ligands can act in a synergetic manner with other growth factors, including transforming growth factor-β (Tgf-β) and sonic hedgehog (Shh) (reviewed in Jessen and Mirsky, 2021). GDNF signaling can stimulate the migration of neuronal precursors and Schwann cells (Iwase et al., 2005) to the site of the injury in both sexes. Action by the neurotrophin growth factors, their receptors, and RhoGDI could inhibit the Rho signaling pathway in both sexes and stimulate the neurite outgrowth (Yamashita and Tohyama, 2003; Dovas and Couchman, 2005).
Proteinases and their intrinsic inhibitors are indispensable for remodeling ECM after nerve damage, establishing a permissive environment for axonal regrowth, promoting regeneration and remyelination (Shubayev and Strongin, 2018; Pellegatta and Taveggia, 2019). MMP and disintegrin ADAM/ADAM-TS protease families control cytokine and growth factor receptors and their ligands in peripheral nerves, including IGF and neuregulin/ERBB after the sciatic nerve axotomy (English et al., 2000; Chattopadhyay and Shubayev, 2009; Liu et al., 2010). MMPs in the orthologous human chromosome 11q22.3 region could carry distinct histone modification marks (Chernov et al., 2010), epigenetic control of this set of metzincins could be involved. Consistent with our previous report (Chernov et al., 2015), of the ECM-remodeling metzincins, demonstrate regulated expression in injured nerves. Protease expression levels were high in intact and axotomized nerves. Processes of post-translational protease activation and binding to intrinsic proteinase inhibitors control potentially cytotoxic proteolytic activities. Aberrant cleavage of mediators of nociceptive signaling during neurogenesis could cause neuropathic pain (Remacle et al., 2015). TIMP family members are important regulators of extracellular proteolysis in normal and damaged nerves (Kim et al., 2012; Liu et al., 2015; Nishihara et al., 2015; Chernov and Shubayev, 2021). Timp1 and Timp3 demonstrated high levels of expression and further increased post-axotomy in both sexes.
Females reduced the expression of over a dozen of sex chromosome-related genes, including Gm7598, Cltrn, Prrg3, Tmem28, Zcchc18, Col4a5, Fgf16, Tnmd, Cnga2, Itm2a, and Xlr3a that did not significantly change in males. As reported in the DRGs (Chernov and Shubayev, 2021) and proximal nerve stumps (Chernov and Shubayev, 2022), at least in part, the sexual dimorphism may be attributed to differences in baseline expression of sex chromosome-linked genes rather than due to post-axotomy regulation.
Sexually dimorphic regulation of lipocalins of the major urinary protein (MUP) family was observed in our study. The male-prevalent activity of MUPs has been attributed to regulating metabolic rate, toxin removal, and survival (Penn et al., 2022). In addition, MUPs carry on chemical communication and pheromone signaling functions (Beynon and Hurst, 2003) in response to a physical injury.
The thyroid hormones (T4 and T3) play an essential role in peripheral nerve regeneration producing a lasting and stimulatory action on axotomized neurons and Schwann cells (Barakat-Walter, 1999). Limited data exist on their role in the development of demyelinating diseases (reviewed in Zorrilla Veloz et al., 2022). Disorders of thyroid metabolism are possible causes of inflammation and autoimmune reactions. Selenocysteine-containing Dio2 and Dio3 deiodinases are primarily responsible for T3/T4 enzymatic activation and inactivation, respectively. At 24 h post-axotomy, the upregulation of both enzymes was stronger in males. Thyroid hormone metabolic enzymes exhibited sexually dimorphic expression at the site of nerve axotomy. Their role in nerve injury response requires a focused investigation.
We predicted regulatory changes in female and male mice to constitute an immediate pro-regenerative response to nerve injury relative to shams. It is conceivable that, at least partially, sex differences in gene transcription could be matched over a more extended time course of nerve injury response. It is important to note that protein-level expression profiles cannot be directly interpreted using RNA-seq data. Single-cell RNA-seq, spatial transcriptomics analysis could provide additional functional information and a precise understanding of sexually dimorphic mechanisms.
Reagents and resources are listed in Supplementary Table 3.
Female and male C57BL6/J mice (6–8 weeks old, Jackson Labs) were randomly assigned to axotomy (n = 6/group) and sham (n = 6/group). The mice were housed in a temperature-controlled room (∼22°C), on a 12 h light/dark cycle, and had free access to food and water. All procedures were conducted between 8.00 and 12.00 daytime. Under isoflurane anesthesia, the left sciatic nerve was exposed at the mid-thigh level. The entire width of the nerve was axotomized using sterile microsurgery scissors. In a sham surgery animal cohort, nerves were exposed using surgical scissors without transection. The muscle was then sutured, and the skin stapled. At 24 h after surgery, distal stumps of the axotomized sciatic nerve were collected for RNA isolation.
All surgical and tissue harvesting instruments were sterilized and repeatedly treated with RNase Away reagent followed by a rinse in RNase-free water. Tissues were submerged in 500 μl RNAlater stabilization solution, placed at 4°C overnight, then transferred for storage at −20°C. All sample groups were processed in parallel to minimize batch effects.
DRG tissues from 2 animals were pooled to obtain at least 500 ng of total RNAs. Tissues were transferred in Trizol solution and disrupted by mechanical homogenization. Total RNAs were purified using RNeasy RNA purification reagents. RNA concentrations and quality were determined using NanoDrop absorbance ratios at 260/280 nm and 260/230 nm. RNA integrity was determined using the Agilent Bioanalyzer Nano RNA chip. Five hundred nanogram of total RNA samples with RIN ≥ 7.0 were used for RNA-seq.
mRNA libraries were generated following the TruSeq Stranded mRNA library preparation protocol (Illumina). In brief, the Poly-A enriched mRNAs were purified using poly-T oligo coupled magnetic beads, followed by mRNA fragmentation, first and second strands synthesis, cleaning on AMPure XP beads, and 3′-adenylation. Ligation of TruSeq dual-index adapters was used for barcoding. The quality of RNA-seq libraries was validated using qPCR. Libraries were sized on Agilent Bioanalyzer DNA high sensitivity chip and normalized. RNA-seq was performed using the paired-end 100 cycle program on the NovaSeq 6000 system at the Genomics High Throughput Facility (University of California Irvine). Base calls were recorded and converted to FASTQ files containing sequencing reads and the corresponding quality scores using Illumina software. Sequencing was conducted until we acquired at least 50 million paired-end reads per sample.
Data analysis steps are summarized in Supplementary Figure 1. FASTQ files were processed using Ubuntu server 20.04 LTS (64-bit ARM). FASTQ files were filtered to remove low-quality bases, TruSeq dual-index adapter sequences, and unpaired reads using Trimmomatic (Bolger et al., 2014). Transcript-level quantification was performed using Salmon (Patro et al., 2017) in quasi-mapping mode using version M27 of the Gencode mouse genome. To correct systematic biases commonly present in RNA-seq data, both -seqBias and -gcBias features were used. Transcript- to gene-level conversion was done using Tximeta (Love et al., 2020). RNA-seq quantification data quality was assessed using MultiQC (Ewels et al., 2016).
Gene count matrices were imported into the DESeq2 package (Love et al., 2014). Outliers were identified by Cook’s distance method and excluded. Dataset’s normalization was conducted using trimmed M-values (TMM). Log2FC we calculated using the Wald test. The adjusted (shrunken) log2FC values were calculated using the adaptive t-prior apeglm method (Love et al., 2014). Significant DEGs defined by Padj < 0.1 and used in downstream analyses. Batch effects were controlled using removeBatchEffect (Ritchie et al., 2015) and RUVseq (Risso et al., 2014) functions. DEGs were visualized using PCAtools, ComplexHeatmap, and EnhancedVolcano R packages.
Biological interpretation of the regulated signaling pathways in female and male animals was conducted by the Ingenuity Pathway Analysis (IPA) as described previously (Chernov and Shubayev, 2021, 2022) based on causal network approaches (Krämer et al., 2014). Cut-off filtering was applied (Padj < 0.1, |Log2FC| > 1) for IPA. Signaling pathway regulation directionality and upstream regulator analysis were based on statistical z-scores calculated in IPA using default parameters. Ontology terms were retrieved from the GO public database (Ashburner et al., 2000).
The original contributions presented in this study are publicly available. This data can be found here: www.ncbi.nlm.nih.gov/geo/ (GSE182751 and GSE182709).
The animal study was reviewed and approved by the Institutional Animal Care and Use Committee at the VA San Diego Healthcare System.
AC: conceptualization, methodology, software, formal analysis, investigation, data curation, writing—original draft, review and editing, and visualization. VS: conceptualization, resources, writing—review and editing, project administration, and funding acquisition. Both authors contributed to the article and approved the submitted version.
This research was supported by the National Institutes of Health (NIH) RO1 DE022757 (to VS) and the Department of Veterans Affairs Merit Review Award 5I01BX000638 (to VS). The content was solely the authors’ responsibility and does not necessarily represent the official views of the funding agencies.
We thank Jennifer Dolkas and Mila Angert for their expert technical assistance.
The authors declare that the research was conducted in the absence of any commercial or financial relationships that could be construed as a potential conflict of interest.
All claims expressed in this article are solely those of the authors and do not necessarily represent those of their affiliated organizations, or those of the publisher, the editors and the reviewers. Any product that may be evaluated in this article, or claim that may be made by its manufacturer, is not guaranteed or endorsed by the publisher.
The Supplementary Material for this article can be found online at: https://www.frontiersin.org/articles/10.3389/fnmol.2022.1029278/full#supplementary-material
DEG, differentially expressed gene; DRG, dorsal root ganglia; ECM, extracellular matrix; ERBB/EGFR, epidermal growth factor receptor; ERK5/MAPK7, mitogen-activated protein kinase 7; ESR/ER, estrogen receptor; FC, fold change; IL, interleukin; IPA, Ingenuity Pathway Analysis; MMP, matrix metalloproteinase; ncRNA, non-coding RNAs; Padj, adjusted P-value; PNS, peripheral nervous system; RhoGDI, Rho GDP-dissociation inhibitor; RNA-seq, RNA sequencing; WD, Wallerian degeneration.
Ahlström, F. H. G., Mätlik, K., Viisanen, H., Blomqvist, K. J., Liu, X., Lilius, T. O., et al. (2021). Spared nerve injury causes sexually dimorphic mechanical allodynia and differential gene expression in spinal cords and dorsal root ganglia in rats. Mol. Neurobiol. 58, 5396–5419. doi: 10.1007/s12035-021-02447-1
Arthur-Farraj, P. J., Latouche, M., Wilton, D. K., Quintes, S., Chabrol, E., Banerjee, A., et al. (2012). C-Jun reprograms Schwann cells of injured nerves to generate a repair cell essential for regeneration. Neuron 75, 633–647. doi: 10.1016/j.neuron.2012.06.021
Ashburner, M., Ball, C. A., Blake, J. A., Botstein, D., Butler, H., Cherry, J. M., et al. (2000). Gene ontology: tool for the unification of biology. The gene ontology consortium. Nat. Genet. 25, 25–29. doi: 10.1038/75556
Barakat-Walter, I. (1999). Role of thyroid hormones and their receptors in peripheral nerve regeneration. J. Neurobiol. 40, 541–559. doi: 10.1002/(sici)1097-4695(19990915)40:4<541::aid-neu10<3.0.co;2-q
Barrette, B., Hébert, M. A., Filali, M., Lafortune, K., Vallières, N., Gowing, G., et al. (2008). Requirement of myeloid cells for axon regeneration. J. Neurosci. 28, 9363–9376. doi: 10.1523/jneurosci.1447-08.2008
Bauer, S., Kerr, B. J., and Patterson, P. H. (2007). The neuropoietic cytokine family in development, plasticity, disease and injury. Nat. Rev. Neurosci. 8, 221–232. doi: 10.1038/nrn2054
Beynon, R. J., and Hurst, J. L. (2003). Multiple roles of major urinary proteins in the house mouse, Mus domesticus. Biochem. Soc. Trans. 31(Pt 1), 142–146. doi: 10.1042/bst0310142
Boerner, K. E., Chambers, C. T., Gahagan, J., Keogh, E., Fillingim, R. B., and Mogil, J. S. (2018). Conceptual complexity of gender and its relevance to pain. Pain 159, 2137–2141. doi: 10.1097/j.pain.0000000000001275
Bogen, O., Joseph, E. K., Chen, X., and Levine, J. D. (2008). GDNF hyperalgesia is mediated by PLCgamma, MAPK/ERK, PI3K, CDK5 and Src family kinase signaling and dependent on the IB4-binding protein versican. Eur. J. Neurosci. 28, 12–19. doi: 10.1111/j.1460-9568.2008.06308.x
Bolger, A. M., Lohse, M., and Usadel, B. (2014). Trimmomatic: a flexible trimmer for Illumina sequence data. Bioinformatics 30, 2114–2120. doi: 10.1093/bioinformatics/btu170
Chattopadhyay, S., and Shubayev, V. I. (2009). MMP-9 controls Schwann cell proliferation and phenotypic remodeling via IGF-1 and ErbB receptor-mediated activation of MEK/ERK pathway. Glia 57, 1316–1325. doi: 10.1002/glia.20851
Chattopadhyay, S., Myers, R. R., Janes, J., and Shubayev, V. (2007). Cytokine regulation of MMP-9 in peripheral glia: implications for pathological processes and pain in injured nerve. Brain Behav. Immun. 21, 561–568. doi: 10.1016/j.bbi.2006.10.015
Chernov, A. V., and Shubayev, V. I. (2021). Sexual dimorphism of early transcriptional reprogramming in dorsal root ganglia after peripheral nerve injury. Front. Mol. Neurosci. 14:779024. doi: 10.3389/fnmol.2021.779024
Chernov, A. V., and Shubayev, V. I. (2022). Sexually dimorphic transcriptional programs of early-phase response in regenerating peripheral nerves. Front. Mol. Neurosci. 15:958568. doi: 10.3389/fnmol.2022.958568
Chernov, A. V., Baranovskaya, S., Golubkov, V. S., Wakeman, D. R., Snyder, E. Y., Williams, R., et al. (2010). Microarray-based transcriptional and epigenetic profiling of matrix metalloproteinases, collagens, and related genes in cancer. J. Biol. Chem. 285, 19647–19659. doi: 10.1074/jbc.M109.088153
Chernov, A. V., Dolkas, J., Hoang, K., Angert, M., Srikrishna, G., Vogl, T., et al. (2015). The calcium-binding proteins S100A8 and S100A9 initiate the early inflammatory program in injured peripheral nerves. J. Biol. Chem. 290, 11771–11784. doi: 10.1074/jbc.M114.622316
Chernov, A. V., Hullugundi, S. K., Eddinger, K. A., Dolkas, J., Remacle, A. G., Angert, M., et al. (2020). A myelin basic protein fragment induces sexually dimorphic transcriptome signatures of neuropathic pain in mice. J. Biol. Chem. 295, 10807–10821. doi: 10.1074/jbc.RA120.013696
Clemence, A., Mirsky, R., and Jessen, K. R. (1989). Non-myelin-forming Schwann cells proliferate rapidly during Wallerian degeneration in the rat sciatic nerve. J. Neurocytol. 18, 185–192. doi: 10.1007/BF01206661
Dovas, A., and Couchman, J. R. (2005). RhoGDI: multiple functions in the regulation of Rho family GTPase activities. Biochem. J. 390(Pt 1), 1–9. doi: 10.1042/bj20050104
Durbec, P., Marcos-Gutierrez, C. V., Kilkenny, C., Grigoriou, M., Wartiowaara, K., Suvanto, P., et al. (1996). GDNF signalling through the Ret receptor tyrosine kinase. Nature 381, 789–793. doi: 10.1038/381789a0
English, W. R., Puente, X. S., Freije, J. M., Knauper, V., Amour, A., Merryweather, A., et al. (2000). Membrane type 4 matrix metalloproteinase (MMP17) has tumor necrosis factor-alpha convertase activity but does not activate pro-MMP2. J. Biol. Chem. 275, 14046–14055. doi: 10.1074/jbc.275.19.14046
Evrard, H. C., and Balthazart, J. (2004). Rapid regulation of pain by estrogens synthesized in spinal dorsal horn neurons. J. Neurosci. 24, 7225–7229. doi: 10.1523/jneurosci.1638-04.2004
Ewels, P., Magnusson, M., Lundin, S., and Käller, M. (2016). MultiQC: summarize analysis results for multiple tools and samples in a single report. Bioinformatics 32, 3047–3048. doi: 10.1093/bioinformatics/btw354
Fawcett, J. W., and Keynes, R. J. (1990). Peripheral nerve regeneration. Annu. Rev. Neurosci. 13, 43–60.
Fillingim, R. B., King, C. D., Ribeiro-Dasilva, M. C., Rahim-Williams, B., and Riley, J. L. III (2009). Sex, gender, and pain: a review of recent clinical and experimental findings. J. Pain 10, 447–485. doi: 10.1016/j.jpain.2008.12.001
Fricker, F. R., and Bennett, D. L. (2011). The role of neuregulin-1 in the response to nerve injury. Future Neurol. 6, 809–822. doi: 10.2217/fnl.11.45
Fukuda, T., Kiuchi, K., and Takahashi, M. (2002). Novel mechanism of regulation of rac activity and lamellipodia formation by RET tyrosine kinase. J. Biol. Chem. 277, 19114–19121. doi: 10.1074/jbc.M200643200
Gerdts, J., Summers, D. W., Milbrandt, J., and DiAntonio, A. (2016). Axon self-destruction: new links among SARM1, MAPKs, and NAD+ metabolism. Neuron 89, 449–460. doi: 10.1016/j.neuron.2015.12.023
Gordon, T. (2009). The role of neurotrophic factors in nerve regeneration. Neurosurg. Focus 26:E3. doi: 10.3171/foc.2009.26.2.E3
Gordon, T., Sulaiman, O., and Boyd, J. G. (2003). Experimental strategies to promote functional recovery after peripheral nerve injuries. J. Peripher. Nerv. Syst. 8, 236–250.
Greenspan, J. D., Craft, R. M., LeResche, L., Arendt-Nielsen, L., Berkley, K. J., Fillingim, R. B., et al. (2007). Studying sex and gender differences in pain and analgesia: a consensus report. Pain 132(Suppl. 1), S26–S45. doi: 10.1016/j.pain.2007.10.014
Hirata, K., and Kawabuchi, M. (2002). Myelin phagocytosis by macrophages and nonmacrophages during Wallerian degeneration. Microsc. Res. Tech. 57, 541–547. doi: 10.1002/jemt.10108
Hucho, T. B., Dina, O. A., Kuhn, J., and Levine, J. D. (2006). Estrogen controls PKCepsilon-dependent mechanical hyperalgesia through direct action on nociceptive neurons. Eur. J. Neurosci. 24, 527–534. doi: 10.1111/j.1460-9568.2006.04913.x
Iwase, T., Jung, C. G., Bae, H., Zhang, M., and Soliven, B. (2005). Glial cell line-derived neurotrophic factor-induced signaling in Schwann cells. J. Neurochem. 94, 1488–1499. doi: 10.1111/j.1471-4159.2005.03290.x
Jessen, K. R., and Arthur-Farraj, P. (2019). Repair Schwann cell update: adaptive reprogramming, EMT, and stemness in regenerating nerves. Glia 67, 421–437. doi: 10.1002/glia.23532
Jessen, K. R., and Mirsky, R. (2021). The role of c-Jun and autocrine signaling loops in the control of repair Schwann cells and regeneration. Front. Cell Neurosci. 15:820216. doi: 10.3389/fncel.2021.820216
Jessen, K. R., Mirsky, R., and Lloyd, A. C. (2015). Schwann cells: development and role in nerve repair. Cold Spring Harb. Perspect. Biol. 7:a020487. doi: 10.1101/cshperspect.a020487
Khomula, E. V., Ferrari, L. F., Araldi, D., and Levine, J. D. (2017). Sexual dimorphism in a reciprocal interaction of ryanodine and IP3 receptors in the induction of hyperalgesic priming. J. Neurosci. 37, 2032–2044. doi: 10.1523/JNEUROSCI.2911-16.2017
Kim, C. F., and Moalem-Taylor, G. (2011). Detailed characterization of neuro-immune responses following neuropathic injury in mice. Brain Res. 1405, 95–108. doi: 10.1016/j.brainres.2011.06.022
Kim, Y., Remacle, A. G., Chernov, A. V., Liu, H., Shubayev, I., Lai, C., et al. (2012). The MMP-9/TIMP-1 axis controls the status of differentiation and function of myelin-forming Schwann cells in nerve regeneration. PLoS One 7:e33664. doi: 10.1371/journal.pone.0033664
Kobayashi, H., Chattopadhyay, S., Kato, K., Dolkas, J., Kikuchi, S., Myers, R. R., et al. (2008). MMPs initiate Schwann cell-mediated MBP degradation and mechanical nociception after nerve damage. Mol. Cell. Neurosci. 39, 619–627. doi: 10.1016/j.mcn.2008.08.008
Krämer, A., Green, J., Pollard, J. Jr., and Tugendreich, S. (2014). Causal analysis approaches in ingenuity pathway analysis. Bioinformatics 30, 523–530. doi: 10.1093/bioinformatics/btt703
Liu, H., and Shubayev, V. I. (2011). Matrix metalloproteinase-9 controls proliferation of NG2+ progenitor cells immediately after spinal cord injury. Exp. Neurol. 231, 236–246. doi: 10.1016/j.expneurol.2011.06.015
Liu, H., Angert, M., Nishihara, T., Shubayev, I., Dolkas, J., and Shubayev, V. I. (2015). Spinal glia division contributes to conditioning lesion–induced axon regeneration into the injured spinal cord. J. Neuropathol. Exp. Neurol. 74, 500–511. doi: 10.1097/nen.0000000000000192
Liu, H., Kim, Y., Chattopadhyay, S., Shubayev, I., Dolkas, J., and Shubayev, V. I. (2010). Matrix metalloproteinase inhibition enhances the rate of nerve regeneration in vivo by promoting dedifferentiation and mitosis of supporting schwann cells. J. Neuropathol. Exp. Neurol. 69, 386–395. doi: 10.1097/NEN.0b013e3181d68d12
Liu, H., Shiryaev, S. A., Chernov, A. V., Kim, Y., Shubayev, I., Remacle, A. G., et al. (2012). Immunodominant fragments of myelin basic protein initiate T cell-dependent pain. J. Neuroinflamm. 9:119. doi: 10.1186/1742-2094-9-119
Love, M. I., Huber, W., and Anders, S. (2014). Moderated estimation of fold change and dispersion for RNA-seq data with DESeq2. Genome Biol. 15:550. doi: 10.1186/s13059-014-0550-8
Love, M. I., Soneson, C., Hickey, P. F., Johnson, L. K., Pierce, N. T., Shepherd, L., et al. (2020). Tximeta: reference sequence checksums for provenance identification in RNA-seq. PLoS Comput. Biol. 16:e1007664. doi: 10.1371/journal.pcbi.1007664
Lundgren, T. K., Nakahata, K., Fritz, N., Rebellato, P., Zhang, S., and Uhlén, P. (2012). RET PLCγ phosphotyrosine binding domain regulates Ca2+ signaling and neocortical neuronal migration. PLoS One 7:e31258. doi: 10.1371/journal.pone.0031258
McDonald, D., Cheng, C., Chen, Y., and Zochodne, D. (2006). Early events of peripheral nerve regeneration. Neuron Glia Biol. 2, 139–147.
Mecklenburg, J., Zou, Y., Wangzhou, A., Garcia, D., Lai, Z., Tumanov, A. V., et al. (2020). Transcriptomic sex differences in sensory neuronal populations of mice. Sci. Rep. 10:15278. doi: 10.1038/s41598-020-72285-z
Meier, C., Parmantier, E., Brennan, A., Mirsky, R., and Jessen, K. R. (1999). Developing Schwann cells acquire the ability to survive without axons by establishing an autocrine circuit involving insulin-like growth factor, neurotrophin-3, and platelet-derived growth factor-BB. J. Neurosci. 19, 3847–3859. doi: 10.1523/JNEUROSCI.19-10-03847.1999
Mogil, J. S. (2012). Sex differences in pain and pain inhibition: multiple explanations of a controversial phenomenon. Nat. Rev. Neurosci. 13, 859–866. doi: 10.1038/nrn3360
Myers, R. R., Campana, W. M., and Shubayev, V. I. (2006). The role of neuroinflammation in neuropathic pain: mechanisms and therapeutic targets. Drug Discov. Today 11, 8–20.
Nielsen, J., Gotfryd, K., Li, S., Kulahin, N., Soroka, V., Rasmussen, K. K., et al. (2009). Role of glial cell line-derived neurotrophic factor (GDNF)-neural cell adhesion molecule (NCAM) interactions in induction of neurite outgrowth and identification of a binding site for NCAM in the heel region of GDNF. J. Neurosci. 29, 11360–11376. doi: 10.1523/JNEUROSCI.3239-09.2009
Nishihara, T., Remacle, A. G., Angert, M., Shubayev, I., Shiryaev, S. A., Liu, H., et al. (2015). Matrix metalloproteinase-14 both sheds cell surface neuronal glial antigen 2 (NG2) proteoglycan on macrophages and governs the response to peripheral nerve injury. J. Biol. Chem. 290, 3693–3707. doi: 10.1074/jbc.M114.603431
North, R. Y., Li, Y., Ray, P., Rhines, L. D., Tatsui, C. E., Rao, G., et al. (2019). Electrophysiological and transcriptomic correlates of neuropathic pain in human dorsal root ganglion neurons. Brain 142, 1215–1226. doi: 10.1093/brain/awz063
Paige, C., Barba-Escobedo, P. A., Mecklenburg, J., Patil, M., Goffin, V., Grattan, D. R., et al. (2020). Neuroendocrine mechanisms governing sex differences in hyperalgesic priming involve prolactin receptor sensory neuron signaling. J. Neurosci. 40, 7080–7090. doi: 10.1523/jneurosci.1499-20.2020
Patro, R., Duggal, G., Love, M. I., Irizarry, R. A., and Kingsford, C. (2017). Salmon provides fast and bias-aware quantification of transcript expression. Nat. Methods 14, 417–419. doi: 10.1038/nmeth.4197
Pellegatta, M., and Taveggia, C. (2019). The complex work of proteases and secretases in wallerian degeneration: beyond Neuregulin-1. Front. Cell. Neurosci. 13:93. doi: 10.3389/fncel.2019.00093
Penn, D. J., Zala, S. M., and Luzynski, K. C. (2022). Regulation of sexually dimorphic expression of major urinary proteins. Front. Physiol. 13:73. doi: 10.3389/fphys.2022.822073
Ray, P. R., Khan, J., Wangzhou, A., Tavares-Ferreira, D., Akopian, A. N., Dussor, G., et al. (2019). Transcriptome analysis of the human tibial nerve identifies sexually dimorphic expression of genes involved in pain, inflammation, and neuro-immunity. Front. Mol. Neurosci. 12:37. doi: 10.3389/fnmol.2019.00037
Remacle, A. G., Kumar, S., Motamedchaboki, K., Cieplak, P., Hullugundi, S., Dolkas, J., et al. (2015). Matrix metalloproteinase (MMP) proteolysis of the extracellular loop of voltage-gated sodium channels and potential alterations in pain signaling. J. Biol. Chem. 290, 22939–22944. doi: 10.1074/jbc.C115.671107
Rettberg, J. R., Yao, J., and Brinton, R. D. (2014). Estrogen: a master regulator of bioenergetic systems in the brain and body. Front. Neuroendocrinol. 35:8–30. doi: 10.1016/j.yfrne.2013.08.001
Risso, D., Ngai, J., Speed, T. P., and Dudoit, S. (2014). Normalization of RNA-seq data using factor analysis of control genes or samples. Nat. Biotechnol. 32, 896–902. doi: 10.1038/nbt.2931
Ritchie, M. E., Phipson, B., Wu, D., Hu, Y., Law, C. W., Shi, W., et al. (2015). Limma powers differential expression analyses for RNA-sequencing and microarray studies. Nucleic Acids Res. 43:e47. doi: 10.1093/nar/gkv007
Rosenbaum, C., Karyala, S., Marchionni, M. A., Kim, H. A., Krasnoselsky, A. L., Happel, B., et al. (1997). Schwann cells express NDF and SMDF/n-ARIA mRNAs, secrete neuregulin, and show constitutive activation of erbB3 receptors: evidence for a neuregulin autocrine loop. Exp. Neurol. 148, 604–615. doi: 10.1006/exnr.1997.6696
Satoh, T., Kidoya, H., Naito, H., Yamamoto, M., Takemura, N., Nakagawa, K., et al. (2013). Critical role of Trib1 in differentiation of tissue-resident M2-like macrophages. Nature 495, 524–528. doi: 10.1038/nature11930
Scholz, J., and Woolf, C. J. (2007). The neuropathic pain triad: neurons, immune cells and glia. Nat. Neurosci. 10, 1361–1368. doi: 10.1038/nn1992
Shubayev, V. I., and Myers, R. R. (2002). Endoneurial remodeling by TNFalpha- and TNFalpha-releasing proteases. A spatial and temporal co-localization study in painful neuropathy. J. Peripher. Nerv. Syst. 7, 28–36. doi: 10.1046/j.1529-8027.2002.02003.x
Shubayev, V. I., and Strongin, A. Y. (2018). Tissue inhibitors of metalloproteases strike a nerve. Neural Regen. Res. 13, 1890–1892. doi: 10.4103/1673-5374.239437
Shubayev, V. I., Angert, M., Dolkas, J., Campana, W. M., Palenscar, K., and Myers, R. R. (2006). TNFalpha-induced MMP-9 promotes macrophage recruitment into injured peripheral nerve. Mol. Cell. Neurosci. 31, 407–415. doi: 10.1016/j.mcn.2005.10.011
Sorge, R. E., and Totsch, S. K. (2017). Sex differences in pain. J. Neurosci. Res. 95, 1271–1281. doi: 10.1002/jnr.23841
Sorge, R. E., Mapplebeck, J. C., Rosen, S., Beggs, S., Taves, S., Alexander, J. K., et al. (2015). Different immune cells mediate mechanical pain hypersensitivity in male and female mice. Nat. Neurosci. 18, 1081–1083. doi: 10.1038/nn.4053
Stephens, K. E., Zhou, W., Ji, Z., Chen, Z., He, S., Ji, H., et al. (2019). Sex differences in gene regulation in the dorsal root ganglion after nerve injury. BMC Genomics 20:147. doi: 10.1186/s12864-019-5512-9
Stoll, G., Jander, S., and Myers, R. R. (2002). Degeneration and regeneration of the peripheral nervous system: from Augustus Waller’s observations to neuroinflammation. J. Peripher. Nerv. Syst. 7, 13–27. doi: 10.1046/j.1529-8027.2002.02002.x
Straub, R. H. (2007). The complex role of estrogens in inflammation. Endocr. Rev. 28, 521–574. doi: 10.1210/er.2007-0001
Tavares-Ferreira, D., Ray, P. R., Sankaranarayanan, I., Mejia, G. L., Wangzhou, A., Shiers, S., et al. (2020). Sex differences in nociceptor translatomes contribute to divergent prostaglandin signaling in male and female mice. bioRxiv [Preprirnt]. doi: 10.1101/2020.07.31.231753
Tofaris, G. K., Patterson, P. H., Jessen, K. R., and Mirsky, R. (2002). Denervated Schwann cells attract macrophages by secretion of leukemia inhibitory factor (LIF) and monocyte chemoattractant protein-1 in a process regulated by interleukin-6 and LIF. J. Neurosci. 22, 6696–6703. doi: 10.1523/jneurosci.22-15-06696.2002
Triolo, D., Dina, G., Lorenzetti, I., Malaguti, M., Morana, P., Del Carro, U., et al. (2006). Loss of glial fibrillary acidic protein (GFAP) impairs Schwann cell proliferation and delays nerve regeneration after damage. J. Cell. Sci. 119(Pt 19), 3981–3993. doi: 10.1242/jcs.03168
Vargas, M. E., and Barres, B. A. (2007). Why is wallerian degeneration in the CNS so slow? Annu. Rev. Neurosci. 30, 153–179. doi: 10.1146/annurev.neuro.30.051606.094354
Waller, A. (1850). Experiments on the section of the glossopharyngeal and hypoglossal nerves of the frog and observations of the alterations produced thereby in the structure of their primitive fibers. Philos. Trans. R. Soc. Lond. B Biol. Sci. 140, 423–429.
Wood, M. D., Kemp, S. W., Weber, C., Borschel, G. H., and Gordon, T. (2011). Outcome measures of peripheral nerve regeneration. Ann. Anat. 193, 321–333. doi: 10.1016/j.aanat.2011.04.008
Yamashita, T., and Tohyama, M. (2003). The p75 receptor acts as a displacement factor that releases Rho from Rho-GDI. Nat. Neurosci. 6, 461–467. doi: 10.1038/nn1045
Yu, J., Gu, X., and Yi, S. (2016). Ingenuity pathway analysis of gene expression profiles in distal nerve stump following nerve injury: insights into wallerian degeneration. Front. Cell. Neurosci. 10:274. doi: 10.3389/fncel.2016.00274
Yu, X., Liu, H., Hamel, K. A., Morvan, M. G., Yu, S., Leff, J., et al. (2020). Dorsal root ganglion macrophages contribute to both the initiation and persistence of neuropathic pain. Nat. Commun. 11:264. doi: 10.1038/s41467-019-13839-2
Zochodne, D. W. (2012). The challenges and beauty of peripheral nerve regrowth. J. Peripher. Nerv. Syst. 17, 1–18. doi: 10.1111/j.1529-8027.2012.00378.x
Keywords: Mus musculus, sexual dimorphism, peripheral nerve injury, axotomy, RNA-seq, Wallerian degeneration
Citation: Chernov AV and Shubayev VI (2022) Sexual dimorphism of early transcriptional reprogramming in degenerating peripheral nerves. Front. Mol. Neurosci. 15:1029278. doi: 10.3389/fnmol.2022.1029278
Received: 27 August 2022; Accepted: 06 October 2022;
Published: 27 October 2022.
Edited by:
Lingxiao Deng, Indiana University, United StatesReviewed by:
Pradipta R. Ray, The University of Texas at Dallas, United StatesCopyright © 2022 Chernov and Shubayev. This is an open-access article distributed under the terms of the Creative Commons Attribution License (CC BY). The use, distribution or reproduction in other forums is permitted, provided the original author(s) and the copyright owner(s) are credited and that the original publication in this journal is cited, in accordance with accepted academic practice. No use, distribution or reproduction is permitted which does not comply with these terms.
*Correspondence: Andrei V. Chernov, YWNoZXJub3ZAaGVhbHRoLnVjc2QuZWR1
Disclaimer: All claims expressed in this article are solely those of the authors and do not necessarily represent those of their affiliated organizations, or those of the publisher, the editors and the reviewers. Any product that may be evaluated in this article or claim that may be made by its manufacturer is not guaranteed or endorsed by the publisher.
Research integrity at Frontiers
Learn more about the work of our research integrity team to safeguard the quality of each article we publish.