- 1Facultad de Ciencias, Centro de Neurobiología y Fisiopatología Integrativa (CENFI), Instituto de Fisiología, Universidad de Valparaíso, Valparaíso, Chile
- 2Programa de Doctorado en Ciencias Mención Neurociencias, Universidad de Valparaíso, Valparaíso, Chile
- 3Programa de Doctorado en Ciencias e Ingeniería para la Salud, Universidad de Valparaíso, Valparaíso, Chile
- 4Escuela de Ciencias Agrícolas y Veterinarias, Universidad Viña del Mar, Viña del Mar, Chile
- 5Facultad de Ciencias, Grupo de NeuroGastroBioquímica, Instituto de Química, Pontificia Universidad Católica de Valparaíso, Valparaíso, Chile
- 6Facultad de Ciencias Biológicas, Departmento de Biología Celular y Molecular, Pontificia Universidad Católica de Chile, Santiago, Chile
Obesity is a pandemic associated with lifestyles changes. These include excess intake of obesogenic foods and decreased physical activity. Brain areas, like the lateral hypothalamus (LH), ventral tegmental area (VTA), and nucleus accumbens (NAcc) have been linked in both homeostatic and hedonic control of feeding in experimental models of diet-induced obesity. Interestingly, these control systems are regulated by the lateral septum (LS), a relay of γ-aminobutyric (GABA) acid neurons (GABAergic neurons) that inhibit the LH and GABAergic interneurons of the VTA. Furthermore, the LS has a diverse receptor population for neurotransmitters and neuropeptides such as dopamine, glutamate, GABA and corticotropin-releasing factor (CRF), among others. Particularly, CRF a key player in the stress response, has been related to the development of overweight and obesity. Moreover, evidence shows that LS neurons neurophysiologically regulate reward and stress, although there is little evidence of LS taking part in homeostatic and hedonic feeding. In this review, we discuss the evidence that supports the role of LS and CRF on feeding, and how alterations in this system contribute to weight gain obesity.
Introduction
Obesity is a global disease that has led the World Health Organization (WHO) to declare it a pandemic. Obesity is defined as an abnormal or excessive fat accumulation, which is detrimental to health, as there is an energy imbalance between calories consumed and calories expended. Quantitatively, the definition associated with the body mass index (BMI), which considers the height and weight of individuals, has defined the condition of obesity when the BMI is greater than 30 kg/m2 (World Health Organization [WHO], 2021). In addition, obesity has genetic and metabolic components, showing a higher prevalence in the offspring of obese parents, especially in developed societies (Cammarano et al., 2022). One of the most highly studied factors that lead to obesity is exposure to palatable hypercaloric diets, rich in lipids, carbohydrates and salt, and pro-obesogenic. In addition, low physical activity has led to an increase in life-threating sedentarism, favoring malnutrition, obesity development and metabolic diseases such as diabetes, dyslipidemia and hypertension (Wanderley and Ferreira, 2010; Barnes, 2011). At the pathological level, overweight (25 kg/m2 > BMI < 30 kg/m2), contribute at least 2.8 million deaths each year (World Health Organization [WHO], 2021), and negatively affect children and adolescents by increasing the risk of chronic non-communicable diseases (NCDs), such as coronary heart disease, metabolic syndrome, and diabetes in adulthood (Bibbins-Domingo et al., 2007). All this epidemiological data, plus the high availability of processed foods in developed countries, favoring a hedonic feeding behavior and neurophysiological signals that regulate satiation and hunger (Berthoud and Munzberg, 2011; Egecioglu et al., 2011; Lee and Dixon, 2017). In this context, understanding how obesogenic food intake affects brain areas associated with feeding control is a question that has not been fully elucidated.
Stress and related factors
Stress has been defined as a destabilizing factor of homeostasis, which leads organisms to generate adaptive responses, including the activation of autonomic nervous system and the hypothalamic-pituitary-adrenal (HPA) axis, affecting peripheral organs and brain areas such as the prefrontal cortex (PFC), amygdala, hippocampus and hypothalamus (McEwen and Akil, 2020). In this sense, several investigations have shown that stress causes adaptive plasticity in the brain, in which local neurotransmitters, and systemic hormones, interact to produce structural and functional changes in neural circuit (Patel et al., 2019). However, when demands related to chronic stress become constant, the body adapts to these new demands and these new changes can lead to pathophysiological conditions in other systems, such as depression (Van Praag, 2005; Patel et al., 2019), cardiovascular diseases (Steptoe and Kivimaki, 2012), eating disorders (e.g., bulimia and anorexia nervosa; Lo Sauro et al., 2008; Groesz et al., 2012) and obesity (Patterson and Abizaid, 2013).
Neurobiologically, the HPA axis integrates the physiological response to stress. A stressful stimulus, regardless in nature stimulates parvocellular neurons in the paraventricular nucleus of the hypothalamus (PVN; Smith and Vale, 2006). These neurons release corticotrophin releasing factor (CRF) and arginine vasopressin (AVP) on the pituitary stimulating specific receptors (GPCRs) expressed on corticotropic cells to synthesize and release adrenocorticotropic hormone (ACTH) into the systemic circulation. Then, ACTH activates melanocortin-2 receptor in the adrenal cortex, stimulating the steroidogenesis of glucocorticoids (Smith and Vale, 2006). Glucocorticoids are the main endocrine effectors of the HPA axis, and their levels are highly regulated not only by feedback inhibition at the pituitary and hypothalamus, but also at the hippocampus (Hipp), nucleus of the solitary tract, and pre-limbic cortex (Sapolsky et al., 1984; Blanchard et al., 1993; McEwen and Akil, 2020).
Stress and food intake
Stress is enough to initiate a highly catabolic physiological response, mobilizing stored energy, thus affecting anabolic processes such as feeding, thirst, and reproduction (Michel et al., 2003; Wingfield and Sapolsky, 2003). However, it is not known whether, the metabolic stress induced by obesogenic diets, nor the chronic stress response might contribute to initiate or reinforce hyperphagic behaviors in obese individuals, showing that CRF system is involved in the regulation of energy balance and the pathophysiology of obesity (Sominsky and Spencer, 2014). CRF was discovered in 1981 as part of the endocrine pathway that regulates ACTH and glucocorticoids (Vale et al., 1981). The CRF system includes CRF and urocortins (UCN; 1, 2, and 3) such as endogenous ligands, CRF receptors type 1 (CRF1) and type 2 (CRF2), and CRF binding protein (CRF-BP; for revision see Slater et al., 2016b). The affinity of CRF and UCN1 for the CRF1 and CRF2 are similar, while the UCN2 affinity for CRF2 is approximately 5,000 times greater than for CRF1. UCN2 and UCN3 are mainly considered selective agonist for CRF2 (Dautzenberg et al., 2019).
CRF receptors are widely expressed in other brain regions and peripheral organs, and therefore, CRF has extrahypothalamic effects, beyond HPA axis regulation (for revision see Deussing and Chen, 2018). For instance, it has been shown that systemic and central administration of CRF or UCN significantly decreases food intake, which could be reversed with the administration of a CRF2 antagonist, but not with the administration of a CRF1 antagonist (Smagin et al., 1998). In this context, administration of ASV-30, a selective CRF2 antagonist, to rats with diet-induced obesity has been shown to reduce the anorexigenic effects of CRF or UCN (Cullen et al., 2001). CRF and UCN are endogenous ligands of CRF receptors, so part of the decrease in food intake may be due to the associated activation of the HPA axis and elevation of plasma corticosteroids. On the other hand, it has been shown that UCN3, a selective agonist of CRF2, regulates feeding behaviors (for revision see Zhang et al., 2019). For example, both intracerebroventricular administration of UCN3 has been shown to reduce food intake in mice and rats (Pelleymounter et al., 2004; Chen et al., 2010). Interestingly, the hypothalamic expressions of UCN3 and CRF2, in lean rats, are high and low in ad libitum and fasted feeding condition, respectively (Poulin et al., 2012). However, the UCN3 and CRF2 expression levels are constant in obese rats (Poulin et al., 2012). Possibly this and other differences in CRF system could be participating in the maintenance of the obesity condition, especially under conditions of physiological stress such as chronic fasting.
In fact, in patients with anorexia nervosa, characterized by a significant decrease in food intake, the CRF and corticosteroids levels are elevated in plasma (Gross et al., 1994). CRF has been described as a potent anorexigenic agent and, therefore, increasing its bioavailability inhibits food intake. However, the end product of the HPA axis, corticosteroids, triggers orexigenic-type responses, as it influences eating behavior that might lead to excess malnutrition, and therefore obesity (Dallman et al., 2007). In addition, intraventricular injection of glucocorticoids in adrenalectomized (ADX) rats stimulates the CRF system, increasing caloric intake, especially carbohydrate consumption (Kumar and Leibowitz, 1988), while administration of corticosteroids has been shown to increase the intake of highly palatable foods (Bell et al., 2000). In Zucker rats (Duclos et al., 2005) and ob/ob mice, both genetic models of obesity, an increase in plasma levels of corticosteroids has been observed (Makimura et al., 2000; Duclos et al., 2005). While ADX in ob/ob mice normalized food intake (Makimura et al., 2000). In addition, the protracted exposure to a highly-palatable diet was associated with the development of stress-related behaviors, improving when the highly-palatable diet is changed back to chow diet (Sharma et al., 2013). However, the motivation toward palatable rewards was not normalized, associating with a decrease and increase in the expression of CRF1 and brain-derived neurotrophic factor (BDNF) in the nucleus accumbens (NAcc), respectively (Sharma et al., 2013). Interestingly, it has been shown that stress-induced CRF system modulate the activity of dopaminergic neurons, affecting the dopamine (DA) release (a neurotransmitter associated with pleasure) in limbic areas such as NAcc and lateral septum (LS; Sotomayor-Zarate et al., 2013; Sotomayor-Zarate et al., 2015; Slater et al., 2016a). In summary, the evidence suggests that the CRF system is related to energy homeostasis, and any alteration in this system will impact food intake behavior.
The lateral septum
The LS is a structure that is interconnected with brain areas involved to cognition, motivation, autonomic regulation, stress and feeding (Gray, 1977; Sheehan et al., 2004; Patel, 2022). It is part of a subcortical structure that delimits the midline of the brain called “septum” and is divided into two parts: the septum pellucidum and septum verum (Andy and Stephan, 1968). The septum verum is divided into medial (MS) and lateral (LS) parts, which differ in their connectivity and functions (Swanson and Cowan, 1979). The MS is cellularly heterogeneous, being composed mainly of excitatory glutamatergic and modulatory cholinergic neurons (Brashear et al., 1986) with bidirectional connections to the Hipp, a key structure involved in learning, memory, and mood regulation (Raisman, 1966; Takeuchi et al., 2021). The LS is constituted predominantly of GABAergic neurons and interneurons, mainly receiving glutamatergic input from the Hipp and projecting GABAergic output to the lateral hypothalamus (LH), between other brain connections (Risold and Swanson, 1997a,b).
Anatomically, LS is located in the subcortical forebrain, between the lateral ventricles, slightly dorsorostral to the nucleus accumbens (NAcc), dorsocaudal to the hypothalamus and ventral to corpus callosum (Swanson and Cowan, 1979). LS has been considered a neural relay station in the forebrain, related to the control of motivation, anger, stress and autonomic regulation (for revision see Sheehan et al., 2004; Figure 1). Also, LS integrates emotions, localization, cognitive information and motivation by rewarding stimuli (Rizzi-Wise and Wang, 2021). The pioneering work of Olds and Milner showed that LS is able to support self-stimulation suggesting its role on the reward system (Olds and Milner, 1954). In addition, Brady and Nauta (1953) suggest that the septum plays a prominent role in controlling stress-induced behavior. The role of LS in addiction has been poorly explored. Sotomayor et al. showed that systemic morphine administration increases LS DA extracellular levels through a decrease in GABA levels in ventral tegmental area (VTA; Sotomayor et al., 2005). In addition, LS GABAergic neurons activate the firing of VTA DA neurons, through the inhibition of VTA GABA interneurons (Luo et al., 2011; Vega-Quiroga et al., 2018; Figure 1). Finally, LS GABA neurons express receptors for several neurotransmitters and neuropeptides that modulate it neural activity, such as vasopressin (Garate-Perez et al., 2021), norepinephrine (NE; Scopinho et al., 2008), ghrelin (Terrill et al., 2018), glucagon-like peptide 1 (GLP-1; Terrill et al., 2016) and CRF (Sotomayor-Zarate et al., 2013), between others.
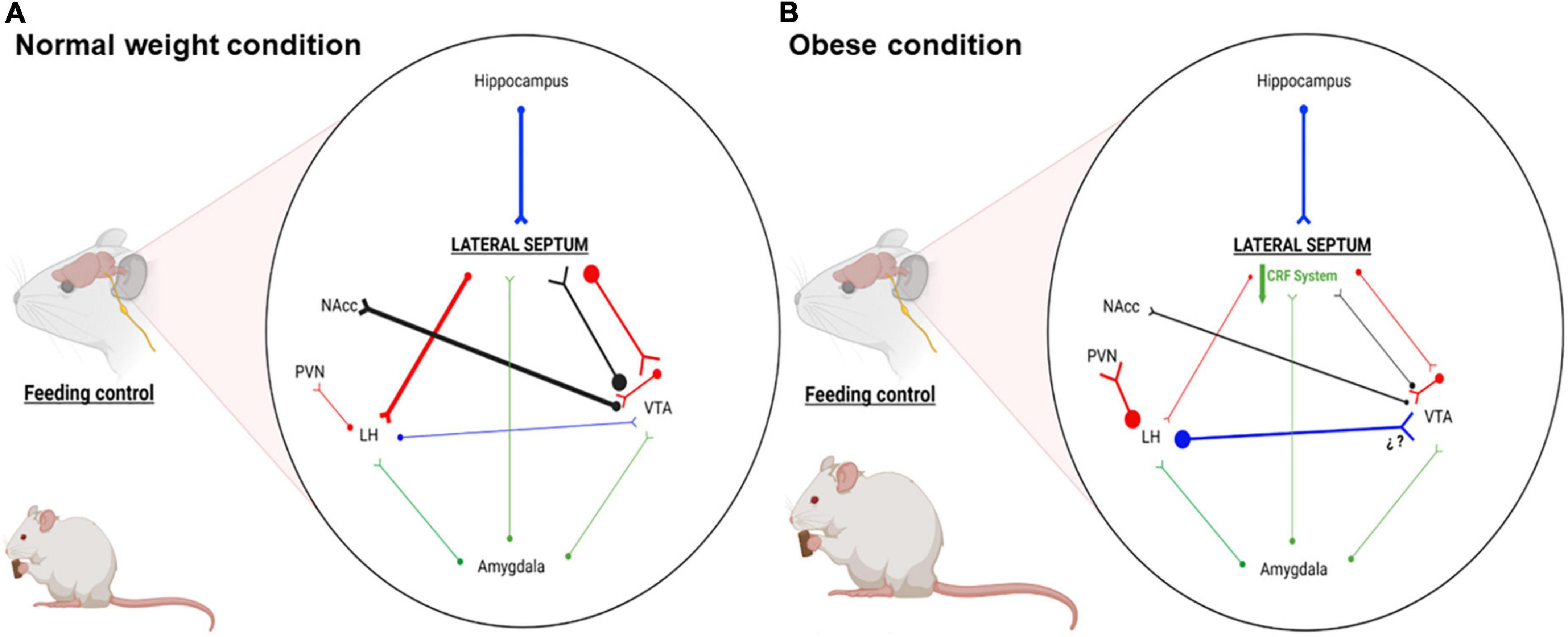
Figure 1. Schematic representation of the main LS connections associated with motivation, stress, emotional and feeding behaviors. (A) Representative connectivity in normal weight condition: LS GABAergic efferents regulate activity of LH and VTA neurons, impacting behaviors such as hunger and motivation. (B) Representative connectivity in obese condition: Our proposal shows that LS CRF system is downregulated, reducing the activity of LS GABAergic efferents. This reduced activity favors hyperactivation and hypoactivation of LH and VTA, leading to hyperphagia and less reward for foods. Blue, glutamatergic neurons; Red, GABAergic neurons; Black, dopaminergic neurons; Green, CRFergic neurons; NAcc, nucleus accumbens; LH, lateral hypothalamus; PVN, paraventricular nucleus; VTA, ventral tegmental area. This figure was created with BioRender.com under subscription and have a license of Biorender to use the figure in journal publications.
Lateral septum and feeding
Despite the inhibitory role of LS on LH (an orexinergic nucleus involved in homeostatic control of feeding), the role of LS in feeding has been poorly studied. Pankey et al. found opposing results on septal lesions and body weight. Lesion of the septal area in male rats produced weight loss, while in female rats induced weight gain (Pankey et al., 2008). On the other hand, the noradrenergic system in the LS is involved in feeding behavior. The LS microinjection of NE increases food intake and this effect is blocked when it is co-microinjected an α1-adrenergic receptor antagonist (Scopinho et al., 2008). LS is strongly activated by glutamatergic input from the cornus ammonis area 3 (CA3) of ventral hippocampus (vHipp), increasing LH GABA release from LS projection (for revision see Rizzi-Wise and Wang, 2021). It should be noted that LH activation triggers hunger behavior through intrahypothalamic GABAergic projections from LH to PVN, where anorexigenic neurons are inhibited (Boudaba et al., 1996). In this context, the LS activation would inhibit the activity of LH neurons and the associated behaviors. The importance of the Hipp-LS-LH pathway in the feeding control has been recently tested using Designer Receptors Exclusively Activated by Designer Drugs (DREADD)-based chemogenetic tools. One hand, the chemogenetic activation expressing hM3Dq in CA3-vHipp glutamatergic efferents to LS reduce food intake (Sweeney and Yang, 2015) and the chemogenetic activation (expressing hM3Dq) or inhibition (expressing hM4Di) of LS GABAergic neurons produce a reduction and increase in food intake, respectively (Sweeney and Yang, 2016). On the other hand, the chemogenetic activation (expressing hM3Dq) in LS neurotensin neurons that project to LH decreases food intake (Azevedo et al., 2020). Recently, it has been shown that the activation of LS neurotensin neurons that project to the nucleus tuberalis lateralis, a nucleus located posterior part of the hypothalamus, reduce the intake of palatable foods (rich in fat and sucrose) and sweet solutions (sucrose and Ensure) in ad libitum and fasted condition (Chen et al., 2022).
Regard to neuropeptide systems that could regulate the activity of LS neurons, it has been shown that LS microinjection of GLP-1 decreases control food intake, while LS microinjections of antagonists of GLP-1 receptors only increases palatable food intake (Terrill et al., 2019). Recently, it has been shown that acute restraint stress increases the activity of LS neurons that express the GLP-1 receptor, decreasing food intake in male mice fed a control diet (Bales et al., 2022). However, in obese male mice the restraint stress does not increase the activity of LS GLP-1R neurons or produce hypophagia (Bales et al., 2022). Finally, the LS microinjection of μ-receptor agonists such as morphine and DAMGO reduces and increases the latency to eat and food intake, respectively (Calderwood et al., 2022).
The lateral septum - corticotropin releasing factor system on feeding
CRF1 mRNA expression has been found predominantly in the forebrain, olfactory regions, cerebellum, anterior pituitary corticotroph cells and in the PVN parvocellular zone (Bravo et al., 2011). On the other hand, CRF2 is expressed in the anterior hypothalamic area (AHA), ventromedial hypothalamus (VMH), nucleus tractus solitarius (NTS), dorsal raphe nucleus, and LS (Van Pett et al., 2000; Deussing and Chen, 2018). In this context, LS is a brain nucleus with high density of CRF, UCN1, and UCN3 containing nerves fibers from hypothalamic (PVN and supraoptic nuclei) and extra-hypothalamic areas (Edinger-Westphal), respectively (Joseph and Knigge, 1983; Swanson et al., 1983; Bittencourt et al., 1999).
At neurophysiological level, LS is considered as a brain relay station that plays an important role in regulate the limbic system, interpretation of several sensory inputs involved in stress, anxiety, motivation and homeostasis, between others (Calhoon and Tye, 2015). Furthermore, different types of stressors such as food deprivation, predator odor, subordination stress, exposure to aversive sounds, and chronic exposure to drugs of abuse, significantly activate the LS (Martin and Timofeeva, 2010; Mirrione et al., 2014). LS CRF receptors promote plasticity to exogenous stimuli such as chronic exposure to drugs of abuse. In this context, electrophysiological studies show that LS CRF2 activation decreases postsynaptic excitatory currents in rats chronically exposed to cocaine (Liu et al., 2004). In addition, in vivo optogenetic activation and inhibition of LS CRF2 evoked and suppressed anxiety-like behaviors, respectively (Anthony et al., 2014). On the other hand, chronic unpredictable stress (14 days) increases LS CRF2 mRNA (Malta et al., 2021).
Regard to food intake, LS UCN infusions produce a reduction in food intake, that it is prevented with the infusions of Astressin-2B, a potent and selective CRF2 antagonist (Bakshi et al., 2007). Acute stress exposure has been associated with anorexigenic and anhedonic responses to food (Foster et al., 2009), while chronic stress exposure increases or decreases eating behavior, generating under- and overeating phenotypes (Calvez and Timofeeva, 2016). However, chronic exposure to hypercaloric diets and stressors promote the development of overeating disorders in humans and binge eating behaviors in rodents (Mathes et al., 2009). Highly palatable foods have a positive valence that promotes a positive emotional state, decreasing negative emotions induced by stress and anxiety (Blasio et al., 2013). These effects trigger maladaptive behaviors to seek and eat palatable foods, promoting a positive feedback loop to alleviate chronic stress and favor overweight and obesity (Ulrich-Lai, 2016). In addition, the exposure to chronic stress stimulates the expression of relaxin-3, an orexinergic neuropeptide, that contribute to amplifying the palatable food intake (Calvez and Timofeeva, 2016; Calvez et al., 2017). In this sense, LS presents a high expression of CRF receptors, receives inputs from CRFergic neurons and it has an important role in regulating the neural activities in LH and VTA, which make it a good candidate to study feeding processes in stress conditions.
Summary and conclusion
The LS has been implicated in the regulation of motivation and feeding. This nucleus expresses several receptors for neurotransmitters and neuropeptides involved in feeding control, including CRF, neuropeptide Y, ghrelin, β-endorphin and GLP-1, between others. The main GABAergic projection of the LS goes to the LH, inhibiting it and reducing food intake. Furthermore, others LS GABAergic projections go to the VTA, increasing the firing of DA neurons. The septal connection shows us an integrative role of this nucleus on homeostatic and hedonic control feeding. However, the regulatory role of the LS on food intake has not been evaluated in obesity, so evaluating whether the lateral septum has a role in the pathophysiology of obesity or whether it can be considered a therapeutic target to treat this disease, are focuses of interest for our research group.
Author contributions
RO-B, JM, and RS-Z wrote the manuscript. JM-P, MF, KG, JB, and RS-Z revised and edited the manuscript. RS-Z obtained the funding. All authors approved the final version of the manuscript.
Funding
This research was funded by the National Agency of Research and Development (ANID-Chile) through FONDECYT Grant No. 120–0474 (to RS-Z). Partial supports were received from DIUV-CI Grant No. 01/2006 (to RS-Z) and PUENTE UVA 20993 (to MF). RO-B was recipient of graduate fellowship N° 21200338 “Beca de Doctorado Nacional” from ANID.
Acknowledgments
We thank the National Fund for Scientific and Technological Development (FONDECYT) from Chile and “Universidad de Valparaíso.”
Conflict of interest
The authors declare that the research was conducted in the absence of any commercial or financial relationships that could be construed as a potential conflict of interest.
Publisher’s note
All claims expressed in this article are solely those of the authors and do not necessarily represent those of their affiliated organizations, or those of the publisher, the editors and the reviewers. Any product that may be evaluated in this article, or claim that may be made by its manufacturer, is not guaranteed or endorsed by the publisher.
References
Andy, O. J., and Stephan, H. (1968). The septum in the human brain. J. Comp. Neurol. 133, 383–410. doi: 10.1002/cne.901330308
Anthony, T. E., Dee, N., Bernard, A., Lerchner, W., Heintz, N., and Anderson, D. J. (2014). Control of stress-induced persistent anxiety by an extra-amygdala septohypothalamic circuit. Cell 156, 522–536. doi: 10.1016/j.cell.2013.12.040
Azevedo, E. P., Tan, B., Pomeranz, L. E., Ivan, V., Fetcho, R., Schneeberger, M., et al. (2020). A limbic circuit selectively links active escape to food suppression. eLife 9:e58894. doi: 10.7554/eLife.58894
Bakshi, V. P., Newman, S. M., Smith-Roe, S., Jochman, K. A., and Kalin, N. H. (2007). Stimulation of lateral septum CRF2 receptors promotes anorexia and stress-like behaviors: Functional homology to CRF1 receptors in basolateral amygdala. J. Neurosci. 27, 10568–10577. doi: 10.1523/JNEUROSCI.3044-06.2007
Bales, M. B., Centanni, S. W., Luchsinger, J. R., Fathi, P., Biddinger, J. E., Le, T. D. V., et al. (2022). High Fat Diet Blunts Stress-Induced Hypophagia and Activation of Glp1r Dorsal Lateral Septum Neurons in Male but not in Female Mice. Mol. Metab. 64:101571. doi: 10.1016/j.molmet.2022.101571
Barnes, A. S. (2011). The epidemic of obesity and diabetes: Trends and treatments. Tex. Heart Inst. J. 38, 142–144.
Bell, M. E., Bhatnagar, S., Liang, J., Soriano, L., Nagy, T. R., and Dallman, M. F. (2000). Voluntary sucrose ingestion, like corticosterone replacement, prevents the metabolic deficits of adrenalectomy. J. Neuroendocrinol. 12, 461–470. doi: 10.1046/j.1365-2826.2000.00488.x
Berthoud, H. R., and Munzberg, H. (2011). The lateral hypothalamus as integrator of metabolic and environmental needs: From electrical self-stimulation to opto-genetics. Physiol. Behav. 104, 29–39. doi: 10.1016/j.physbeh.2011.04.051
Bibbins-Domingo, K., Coxson, P., Pletcher, M. J., Lightwood, J., and Goldman, L. (2007). Adolescent overweight and future adult coronary heart disease. N. Engl. J. Med. 357, 2371–2379. doi: 10.1056/NEJMsa073166
Bittencourt, J. C., Vaughan, J., Arias, C., Rissman, R. A., Vale, W. W., and Sawchenko, P. E. (1999). Urocortin expression in rat brain: Evidence against a pervasive relationship of urocortin-containing projections with targets bearing type 2 CRF receptors. J. Comp. Neurol. 415, 285–312. doi: 10.1002/(SICI)1096-9861(19991220)415:3<285::AID-CNE1>3.0.CO;2-0
Blanchard, D. C., Sakai, R. R., Mcewen, B., Weiss, S. M., and Blanchard, R. J. (1993). Subordination stress: Behavioral, brain, and neuroendocrine correlates. Behav. Brain. Res. 58, 113–121. doi: 10.1016/0166-4328(93)90096-9
Blasio, A., Iemolo, A., Sabino, V., Petrosino, S., Steardo, L., Rice, K. C., et al. (2013). Rimonabant precipitates anxiety in rats withdrawn from palatable food: Role of the central amygdala. Neuropsychopharmacology 38, 2498–2507. doi: 10.1038/npp.2013.153
Boudaba, C., Szabo, K., and Tasker, J. G. (1996). Physiological mapping of local inhibitory inputs to the hypothalamic paraventricular nucleus. J. Neurosci. 16, 7151–7160. doi: 10.1523/JNEUROSCI.16-22-07151.1996
Brady, J. V., and Nauta, W. J. (1953). Subcortical mechanisms in emotional behavior: Affective changes following septal forebrain lesions in the albino rat. J. Comp. Physiol. Psychol. 46, 339–346. doi: 10.1037/h0059531
Brashear, H. R., Zaborszky, L., and Heimer, L. (1986). Distribution of GABAergic and cholinergic neurons in the rat diagonal band. Neuroscience 17, 439–451. doi: 10.1016/0306-4522(86)90258-7
Bravo, J. A., Dinan, T. G., and Cryan, J. F. (2011). Alterations in the central CRF system of two different rat models of comorbid depression and functional gastrointestinal disorders. Int. J. Neuropsychopharmacol. 14, 666–683. doi: 10.1017/S1461145710000994
Calderwood, M. T., Tseng, A., Gabriella, I., and Stanley, B. G. (2022). Feeding behavior elicited by mu opioid and GABA receptor activation in the lateral septum. Pharmacol. Biochem. Behav. 217:173395. doi: 10.1016/j.pbb.2022.173395
Calhoon, G. G., and Tye, K. M. (2015). Resolving the neural circuits of anxiety. Nat. Neurosci. 18, 1394–1404. doi: 10.1038/nn.4101
Calvez, J., and Timofeeva, E. (2016). Behavioral and hormonal responses to stress in binge-like eating prone female rats. Physiol. Behav. 157, 28–38. doi: 10.1016/j.physbeh.2016.01.029
Calvez, J., De Avila, C., and Timofeeva, E. (2017). Sex-specific effects of relaxin-3 on food intake and body weight gain. Br. J. Pharmacol. 174, 1049–1060. doi: 10.1111/bph.13530
Cammarano, A., Marella, G. L., Tavone, A. M., Scipione, C., Marsella, L. T., Potenza, S., et al. (2022). Obesity as a social phenomenon: A narrative review. Epidemiol. Prev. 46, 168–172.
Chen, P., Vaughan, J., Donaldson, C., Vale, W., and Li, C. (2010). Injection of Urocortin 3 into the ventromedial hypothalamus modulates feeding, blood glucose levels, and hypothalamic POMC gene expression but not the HPA axis. Am. J. Physiol. Endocrinol. Metab. 298:E337–E345. doi: 10.1152/ajpendo.00402.2009
Chen, Z., Chen, G., Zhong, J., Jiang, S., Lai, S., Xu, H., et al. (2022). A circuit from lateral septum neurotensin neurons to tuberal nucleus controls hedonic feeding. Mol. Psychiatry [Epub ahead of print]. doi: 10.1038/s41380-022-01742-0
Cullen, M. J., Ling, N., Foster, A. C., and Pelleymounter, M. A. (2001). Urocortin, corticotropin releasing factor-2 receptors and energy balance. Endocrinology 142, 992–999. doi: 10.1210/endo.142.3.7989
Dallman, M. F., Akana, S. F., Pecoraro, N. C., Warne, J. P., La Fleur, S. E., and Foster, M. T. (2007). Glucocorticoids, the etiology of obesity and the metabolic syndrome. Curr. Alzheimer Res. 4, 199–204. doi: 10.2174/156720507780362236
Dautzenberg, F. M., Grigoriadis, D. E., Hauger, R. L., Risbrough, V. B., Steckler, T., Vale, W. W., et al. (2019). Corticotropin-releasing factor receptors (version 2019.4) in the IUPHAR/BPS Guide to Pharmacology Database. IUPHAR/BPS Guide Pharmacol. 2019*. doi: 10.2218/gtopdb/F19/2019.4
Deussing, J. M., and Chen, A. (2018). The Corticotropin-Releasing Factor Family: Physiology of the Stress Response. Physiol. Rev. 98, 2225–2286. doi: 10.1152/physrev.00042.2017
Duclos, M., Timofeeva, E., Michel, C., and Richard, D. (2005). Corticosterone-dependent metabolic and neuroendocrine abnormalities in obese Zucker rats in relation to feeding. Am. J. Physiol. Endocrinol. Metab. 288:E254–E266. doi: 10.1152/ajpendo.00087.2004
Egecioglu, E., Skibicka, K. P., Hansson, C., Alvarez-Crespo, M., Friberg, P. A., Jerlhag, E., et al. (2011). Hedonic and incentive signals for body weight control. Rev. Endocr. Metab. Disord. 12, 141–151. doi: 10.1007/s11154-011-9166-4
Foster, M. T., Warne, J. P., Ginsberg, A. B., Horneman, H. F., Pecoraro, N. C., Akana, S. F., et al. (2009). Palatable foods, stress, and energy stores sculpt corticotropin-releasing factor, adrenocorticotropin, and corticosterone concentrations after restraint. Endocrinology 150, 2325–2333. doi: 10.1210/en.2008-1426
Garate-Perez, M. F., Mendez, A., Bahamondes, C., Sanhueza, C., Guzman, F., Reyes-Parada, M., et al. (2021). Vasopressin in the lateral septum decreases conditioned place preference to amphetamine and nucleus accumbens dopamine release. Addict. Biol. 26:e12851. doi: 10.1111/adb.12851
Gray, J. A. (1977). Functions of the septo-hippocampal system. Objectives of the meeting. Ciba Found Symp.* 3–4. doi: 10.1002/9780470720394.ch2
Groesz, L. M., Mccoy, S., Carl, J., Saslow, L., Stewart, J., Adler, N., et al. (2012). What is eating you? Stress and the drive to eat. Appetite 58, 717–721. doi: 10.1016/j.appet.2011.11.028
Gross, M. J., Kahn, J. P., Laxenaire, M., Nicolas, J. P., and Burlet, C. (1994). [Corticotropin-releasing factor and anorexia nervosa: Reactions of the hypothalamus-pituitary-adrenal axis to neurotropic stress]. Ann. Endocrinol. 55, 221–228.
Joseph, S. A., and Knigge, K. M. (1983). Corticotropin releasing factor: Immunocytochemical localization in rat brain. Neurosci. Lett. 35, 135–141. doi: 10.1016/0304-3940(83)90540-2
Kumar, B. A., and Leibowitz, S. F. (1988). Impact of acute corticosterone administration on feeding and macronutrient self-selection patterns. Am. J. Physiol. 254, R222–R228. doi: 10.1152/ajpregu.1988.254.2.R222
Lee, P. C., and Dixon, J. B. (2017). Food for Thought: Reward Mechanisms and Hedonic Overeating in Obesity. Curr. Obes. Rep. 6, 353–361. doi: 10.1007/s13679-017-0280-9
Liu, J., Yu, B., Neugebauer, V., Grigoriadis, D. E., Rivier, J., Vale, W. W., et al. (2004). Corticotropin-releasing factor and Urocortin I modulate excitatory glutamatergic synaptic transmission. J. Neurosci. 24, 4020–4029. doi: 10.1523/JNEUROSCI.5531-03.2004
Lo Sauro, C., Ravaldi, C., Cabras, P. L., Faravelli, C., and Ricca, V. (2008). Stress, hypothalamic-pituitary-adrenal axis and eating disorders. Neuropsychobiology 57, 95–115. doi: 10.1159/000138912
Luo, A. H., Tahsili-Fahadan, P., Wise, R. A., Lupica, C. R., and Aston-Jones, G. (2011). Linking context with reward: A functional circuit from hippocampal CA3 to ventral tegmental area. Science 333, 353–357. doi: 10.1126/science.1204622
Makimura, H., Mizuno, T. M., Roberts, J., Silverstein, J., Beasley, J., and Mobbs, C. V. (2000). Adrenalectomy reverses obese phenotype and restores hypothalamic melanocortin tone in leptin-deficient ob/ob mice. Diabetes 49, 1917–1923. doi: 10.2337/diabetes.49.11.1917
Malta, M. B., Martins, J., Novaes, L. S., Dos Santos, N. B., Sita, L., Camarini, R., et al. (2021). Norepinephrine and Glucocorticoids Modulate Chronic Unpredictable Stress-Induced Increase in the Type 2 CRF and Glucocorticoid Receptors in Brain Structures Related to the HPA Axis Activation. Mol. Neurobiol. 58, 4871–4885. doi: 10.1007/s12035-021-02470-2
Martin, J., and Timofeeva, E. (2010). Intermittent access to sucrose increases sucrose-licking activity and attenuates restraint stress-induced activation of the lateral septum. Am. J. Physiol. Regul. Integr. Comp. Physiol. 298, R1383–R1398. doi: 10.1152/ajpregu.00371.2009
Mathes, W. F., Brownley, K. A., Mo, X., and Bulik, C. M. (2009). The biology of binge eating. Appetite 52, 545–553. doi: 10.1016/j.appet.2009.03.005
McEwen, B. S., and Akil, H. (2020). Revisiting the Stress Concept: Implications for Affective Disorders. J. Neurosci. 40, 12–21. doi: 10.1523/JNEUROSCI.0733-19.2019
Michel, C., Levin, B. E., and Dunn-Meynell, A. A. (2003). Stress facilitates body weight gain in genetically predisposed rats on medium-fat diet. Am. J. Physiol. Regul. Integr. Comp. Physiol. 285, R791–R799. doi: 10.1152/ajpregu.00072.2003
Mirrione, M. M., Schulz, D., Lapidus, K. A., Zhang, S., Goodman, W., and Henn, F. A. (2014). Increased metabolic activity in the septum and habenula during stress is linked to subsequent expression of learned helplessness behavior. Front. Hum. Neurosci. 8:29. doi: 10.3389/fnhum.2014.00029
Olds, J., and Milner, P. (1954). Positive reinforcement produced by electrical stimulation of septal area and other regions of rat brain. J. Comp. Physiol. Psychol. 47, 419–427. doi: 10.1037/h0058775
Pankey, E. A., Shurley, M. R., and King, B. M. (2008). A re-examination of septal lesion-induced weight gain in female rats. Physiol. Behav. 93, 8–12. doi: 10.1016/j.physbeh.2007.07.019
Patel, D., Kas, M. J., Chattarji, S., and Buwalda, B. (2019). Rodent models of social stress and neuronal plasticity: Relevance to depressive-like disorders. Behav. Brain Res. 369:111900. doi: 10.1016/j.bbr.2019.111900
Patel, H. (2022). The role of the lateral septum in neuropsychiatric disease. J. Neurosci. Res. 100, 1422–1437. doi: 10.1002/jnr.25052
Patterson, Z. R., and Abizaid, A. (2013). Stress induced obesity: Lessons from rodent models of stress. Front. Neurosci. 7:130. doi: 10.3389/fnins.2013.00130
Pelleymounter, M. A., Joppa, M., Ling, N., and Foster, A. C. (2004). Behavioral and neuroendocrine effects of the selective CRF2 receptor agonists urocortin II and urocortin III. Peptides 25, 659–666. doi: 10.1016/j.peptides.2004.01.008
Poulin, A. M., Lenglos, C., Mitra, A., and Timofeeva, E. (2012). Hypothalamic expression of urocortin 3 and the type 2 corticotropin-releasing factor receptor is regulated according to feeding state in lean but not obese Zucker rats. Neuropharmacology 63, 147–153. doi: 10.1016/j.neuropharm.2011.12.023
Risold, P. Y., and Swanson, L. W. (1997b). Connections of the rat lateral septal complex. Brain Res. Brain Res. Rev. 24, 115–195. doi: 10.1016/S0165-0173(97)00009-X
Risold, P. Y., and Swanson, L. W. (1997a). Chemoarchitecture of the rat lateral septal nucleus. Brain Res. Brain Res. Rev. 24, 91–113. doi: 10.1016/S0165-0173(97)00008-8
Rizzi-Wise, C. A., and Wang, D. V. (2021). Putting Together Pieces of the Lateral Septum: Multifaceted Functions and Its Neural Pathways. eNeuro 8:ENEURO.315–ENEURO.321. doi: 10.1523/ENEURO.0315-21.2021
Sapolsky, R. M., Krey, L. C., and Mcewen, B. S. (1984). Glucocorticoid-sensitive hippocampal neurons are involved in terminating the adrenocortical stress response. Proc. Natl. Acad. Sci. U.S.A. 81, 6174–6177. doi: 10.1073/pnas.81.19.6174
Scopinho, A. A., Resstel, L. B., and Correa, F. M. (2008). alpha(1)-Adrenoceptors in the lateral septal area modulate food intake behaviour in rats. Br. J. Pharmacol. 155, 752–756. doi: 10.1038/bjp.2008.295
Sharma, S., Fernandes, M. F., and Fulton, S. (2013). Adaptations in brain reward circuitry underlie palatable food cravings and anxiety induced by high-fat diet withdrawal. Int. J. Obes. 37, 1183–1191. doi: 10.1038/ijo.2012.197
Sheehan, T. P., Chambers, R. A., and Russell, D. S. (2004). Regulation of affect by the lateral septum: Implications for neuropsychiatry. Brain Res. Brain Res. Rev. 46, 71–117. doi: 10.1016/j.brainresrev.2004.04.009
Slater, P. G., Yarur, H. E., and Gysling, K. (2016b). Corticotropin-Releasing Factor Receptors and Their Interacting Proteins: Functional Consequences. Mol. Pharmacol. 90, 627–632. doi: 10.1124/mol.116.104927
Slater, P. G., Noches, V., and Gysling, K. (2016a). Corticotropin-releasing factor type-2 receptor and corticotropin-releasing factor-binding protein coexist in rat ventral tegmental area nerve terminals originated in the lateral hypothalamic area. Eur. J. Neurosci. 43, 220–229. doi: 10.1111/ejn.13113
Smagin, G. N., Howell, L. A., Ryan, D. H., De Souza, E. B., and Harris, R. B. (1998). The role of CRF2 receptors in corticotropin-releasing factor- and urocortin-induced anorexia. Neuroreport 9, 1601–1606. doi: 10.1097/00001756-199805110-00063
Smith, S. M., and Vale, W. W. (2006). The role of the hypothalamic-pituitary-adrenal axis in neuroendocrine responses to stress. Dialogues Clin. Neurosci. 8, 383–395. doi: 10.31887/DCNS.2006.8.4/ssmith
Sominsky, L., and Spencer, S. J. (2014). Eating behavior and stress: A pathway to obesity. Front. Psychol. 5:434. doi: 10.3389/fpsyg.2014.00434
Sotomayor, R., Forray, M. I., and Gysling, K. (2005). Acute morphine administration increases extracellular DA levels in the rat lateral septum by decreasing the GABAergic inhibitory tone in the ventral tegmental area. J. Neurosci. Res. 81, 132–139. doi: 10.1002/jnr.20537
Sotomayor-Zarate, R., Abarca, J., Araya, K. A., Renard, G. M., Andres, M. E., and Gysling, K. (2015). Exposure to repeated immobilization stress inhibits cocaine-induced increase in dopamine extracellular levels in the rat ventral tegmental area. Pharmacol. Res. 101, 116–123. doi: 10.1016/j.phrs.2015.08.015
Sotomayor-Zarate, R., Renard, G. M., Araya, K. A., Carreno, P., Fuentealba, J. A., Andres, M. E., et al. (2013). Long-term loss of dopamine release mediated by CRF-1 receptors in the rat lateral septum after repeated cocaine administration. Behav. Brain Res. 250, 206–210. doi: 10.1016/j.bbr.2013.05.012
Steptoe, A., and Kivimaki, M. (2012). Stress and cardiovascular disease. Nat. Rev. Cardiol. 9, 360–370. doi: 10.1038/nrcardio.2012.45
Swanson, L. W., and Cowan, W. M. (1979). The connections of the septal region in the rat. J. Comp. Neurol. 186, 621–655. doi: 10.1002/cne.901860408
Swanson, L. W., Sawchenko, P. E., Rivier, J., and Vale, W. W. (1983). Organization of ovine corticotropin-releasing factor immunoreactive cells and fibers in the rat brain: An immunohistochemical study. Neuroendocrinology 36, 165–186. doi: 10.1159/000123454
Sweeney, P., and Yang, Y. (2015). An excitatory ventral hippocampus to lateral septum circuit that suppresses feeding. Nat. Commun. 6:10188. doi: 10.1038/ncomms10188
Sweeney, P., and Yang, Y. (2016). An Inhibitory Septum to Lateral Hypothalamus Circuit That Suppresses Feeding. J. Neurosci. 36, 11185–11195. doi: 10.1523/JNEUROSCI.2042-16.2016
Takeuchi, Y., Nagy, A. J., Barcsai, L., Li, Q., Ohsawa, M., Mizuseki, K., et al. (2021). The Medial Septum as a Potential Target for Treating Brain Disorders Associated With Oscillopathies. Front. Neural Circuits 15:701080. doi: 10.3389/fncir.2021.701080
Terrill, S. J., Holt, M. K., Maske, C. B., Abrams, N., Reimann, F., Trapp, S., et al. (2019). Endogenous GLP-1 in lateral septum promotes satiety and suppresses motivation for food in mice. Physiol. Behav. 206, 191–199. doi: 10.1016/j.physbeh.2019.04.008
Terrill, S. J., Jackson, C. M., Greene, H. E., Lilly, N., Maske, C. B., Vallejo, S., et al. (2016). Role of lateral septum glucagon-like peptide 1 receptors in food intake. Am. J. Physiol. Regul. Integr. Comp. Physiol. 311, R124–R132. doi: 10.1152/ajpregu.00460.2015
Terrill, S. J., Wall, K. D., Medina, N. D., Maske, C. B., and Williams, D. L. (2018). Lateral septum growth hormone secretagogue receptor affects food intake and motivation for sucrose reinforcement. Am. J. Physiol. Regul. Integr. Comp. Physiol. 315, R76–R83. doi: 10.1152/ajpregu.00339.2017
Ulrich-Lai, Y. M. (2016). Self-medication with sucrose. Curr. Opin. Behav. Sci. 9, 78–83. doi: 10.1016/j.cobeha.2016.02.015
Vale, W., Spiess, J., Rivier, C., and Rivier, J. (1981). Characterization of a 41-residue ovine hypothalamic peptide that stimulates secretion of corticotropin and beta-endorphin. Science 213, 1394–1397. doi: 10.1126/science.6267699
Van Pett, K., Viau, V., Bittencourt, J. C., Chan, R. K., Li, H. Y., Arias, C., et al. (2000). Distribution of mRNAs encoding CRF receptors in brain and pituitary of rat and mouse. J. Comp. Neurol. 428, 191–212. doi: 10.1002/1096-9861(20001211)428:2<191::AID-CNE1>3.0.CO;2-U
Van Praag, H. M. (2005). Can stress cause depression? World J. Biol. Psychiatry 6, 5–22. doi: 10.1080/15622970510030018
Vega-Quiroga, I., Yarur, H. E., and Gysling, K. (2018). Lateral septum stimulation disinhibits dopaminergic neurons in the antero-ventral region of the ventral tegmental area: Role of GABA-A alpha 1 receptors. Neuropharmacology 128, 76–85. doi: 10.1016/j.neuropharm.2017.09.034
Wanderley, E. N., and Ferreira, V. A. (2010). [Obesity: A plural perspective]. Cien Saude Colet 15, 185–194. doi: 10.1590/S1413-81232010000100024
Wingfield, J. C., and Sapolsky, R. M. (2003). Reproduction and resistance to stress: When and how. J. Neuroendocrinol. 15, 711–724. doi: 10.1046/j.1365-2826.2003.01033.x
Keywords: obesity, feeding control, lateral septum (LS), CRF system, addiction
Citation: Olivares-Barraza R, Marcos JL, Martínez-Pinto J, Fuenzalida M, Bravo JA, Gysling K and Sotomayor-Zárate R (2022) Corticotropin-releasing factor system in the lateral septum: Implications in the pathophysiology of obesity. Front. Mol. Neurosci. 15:1020903. doi: 10.3389/fnmol.2022.1020903
Received: 16 August 2022; Accepted: 05 September 2022;
Published: 20 September 2022.
Edited by:
Braulio Alfredo Muñoz Ramirez, Indiana University Bloomington, United StatesReviewed by:
Denis Richard, Laval University, CanadaCopyright © 2022 Olivares-Barraza, Marcos, Martínez-Pinto, Fuenzalida, Bravo, Gysling and Sotomayor-Zárate. This is an open-access article distributed under the terms of the Creative Commons Attribution License (CC BY). The use, distribution or reproduction in other forums is permitted, provided the original author(s) and the copyright owner(s) are credited and that the original publication in this journal is cited, in accordance with accepted academic practice. No use, distribution or reproduction is permitted which does not comply with these terms.
*Correspondence: Ramón Sotomayor-Zárate, ramon.sotomayor@uv.cl
†These authors have contributed equally to this work and share first authorship