- 1Department of Histology and Embryology, Medical College, Shaoxing University, Shaoxing, China
- 2School of Life Sciences, Shaoxing University, Shaoxing, China
- 3Institute of Neuroscience, Zhejiang University School of Medicine, Hangzhou, China
Spinal cord injury (SCI) is a high incident rate of central nervous system disease that usually causes paralysis below the injured level. The occurrence of chronic inflammation with the axonal regeneration difficulties are the underlying barriers for the recovery of SCI patients. Current studies have paid attention to controlling the instigative and developmental process of neuro-inflammation. Ethyl pyruvate, as a derivative of pyruvate, has strong anti-inflammatory and neuroprotective functions. Herein, we reviewed the recent studies of ethyl pyruvate and high mobility group box-1 (HMGB1). We think HMGB1 that is one of the main nuclear protein mediators to cause an inflammatory response. This protein induces astrocytic activation, and promotes glial scar formation. Interestingly, ethyl pyruvate has potent inhibitory effects on HMGB1 protein, as it inhibits chronic inflammatory response by modulating the HMGB1/TLR4/NF-κB signaling pathway. This paper discusses the potential mechanism of ethyl pyruvate in inhibiting chronic inflammation after SCI. Ethyl pyruvate can be a prospective therapeutic agent for SCI.
Introduction
Spinal cord injury (SCI) refers to spinal cord dysfunction or organic damage caused by direct or indirect external force affecting the spine (Fouad et al., 2021). The clinical manifestations of SCI are usually the motor and sensory dysfunctions of tissues and organs below the injury level such as dysfunctions of bladder and rectum (Leibinger et al., 2021). Severe SCI patients can present with the paraplegia (Bendella et al., 2019), respiratory disorders, and even cause the death. SCI mainly occurs in young adults, and is often caused by falling, traffic accidents, violence, and improper physical exercises. Statistics show that there are about 18,000 new SCI patients worldwide every year (Lv et al., 2021). The high cost of treatment coupled with the incomplete injury recovery, which contributes to the tremendous psychological, economic and social burden for patients and their families. In recent years, the high incidence and disability of SCI has become a major medical conundrum. SCI based on its physiological and pathological characteristics that is divided into the acute and chronic SCI. The physiopathological features are mainly temporary shock of the spinal cord in the initial stage of the acute SCI. A few minutes following the early inflammatory stage will trigger the biochemical disorders at the injured sites, cell microenvironment destruction, inflammatory response, together with detrimental effects to the vascular system after the acute SCI phase (Kumar et al., 2020). The chronic stage is characterized by the edema and glial scar formations at the injured sites from a few days to years after injury, and leads to the permanent autonomic dysregulation.
Chronic inflammation is the main obstacle in SCI treatment, as nerve cells are extremely active at this stage, especially astrocytes (Yoshizaki et al., 2021). Activated astrocytes not only proliferate rapidly, but also change cell morphologies, which become hypertrophic and conglutinate into pieces to form the scar-like glial cells (Okada et al., 2018). The scar prevents the expansion of the injured area, and blocks axonal repair (Cox et al., 2021). Therefore, at the chronic development stage of SCI patient, the activated astrocytes can form glial scar around the injured area to result in the injured area hollow and hinder axonal regeneration and repair (Edwards-Faret et al., 2021). Presently, SCI studies mainly focus on reducing chronic inflammatory response, preventing glial scar formation, and promoting axonal growth.
High-mobility group box 1 (HMGB1), as a DNA binding protein in the nucleus, is a structural cofactor for cells, and has an important regulatory function for transcription (Sun et al., 2017). HMGB1 is a key factor released during the apoptotic and necrotic processes, and plays a significant role in promoting local and systemic inflammatory response (Wang et al., 2020a). When cells are strongly stimulated or start necrosis, HMGB1 is secreted from the nucleus to the outside of cells, which activates inflammatory responses of glial cells, stimulates the cells to release neurotoxic factors, and aggravates the inflammatory responses (Shen et al., 2020). The high expression of HMGB1 can aggravate a patient’s condition in the injury and inflammation sites (Yang et al., 2018). Several studies show to curtail the inflammatory reaction, improve spinal cord edema and injury recovery by inhibiting HMGB1 activity (Sun et al., 2017, 2019a). HMGB1, as the upstream factor of secondary inflammatory reaction, can activate nuclear factor-kappa B (NF-κB) (Liang et al., 2020), mitogen-activated protein kinase (MAPK) (Xie et al., 2019), and other classical inflammatory pathways to trigger the activation and inflammation of glial cells after SCI (Ta Na et al., 2019). Furthermore, the high expression of HMGB1 promotes inflammatory reactions, and is closely related to the activation of several cell membrane receptors, such as toll-like receptor (TLR) 4, TLR2, and receptor of advanced glycation end product (RAGE) in the wake of SCI (Casula et al., 2011; Xia et al., 2019; Fan et al., 2020). Macrophages and injured neurons can release HMGB1 to activate microglia and astrocytes through the HMGB1/NF-κB signaling pathway, moreover, the reactive astrocytes and microglia further release HMGB1 to exacerbate the inflammatory response, apoptosis and oxidative stress in the damaged area (Liu et al., 2017; Sun et al., 2017; Wang et al., 2021; Du et al., 2022). However, the specific interaction between HMGB1 and its receptors is unclear, and needs further studies.
Ethyl pyruvate (EP), as a chemically stable derivative of pyruvate, has significant anti-inflammatory and neuroprotective effects (Lee et al., 2019a). Studies have shown that EP exerts the potent anti-inflammatory roles by inhibiting the expression of various inflammatory mediators and eliminating the release of oxidative stress factors (Dong et al., 2019b). In the EP-treated mice, the inflammatory signal of NF-κB was significantly inhibited (Liu et al., 2019a). Moreover, studies showed that EP could restore the axonal regeneration after SCI, and has an exceptional protective effect on nerve development, and is closely related to its inhibition of inflammatory response by downregulating the HMGB1 activity (Wang et al., 2009; Sun et al., 2017, 2019a). However, the specific physiological mechanism is still unclear. In addition, EP can significantly inhibit the astrocytic proliferation, and reduce the formation of glial scar (Djedović et al., 2017). The above evidences indicate that EP may act as a potential agent for SCI treatment.
Astrocytic activation and the high mobility group box-1/toll-like receptor4/nuclear factor-kappa B pathway in spinal cord injury
Astrocytic activation
The most important feature of the chronic stage is glial scar formation, which inhibits the regeneration and recovery of spinal cord (Leibinger et al., 2021). Glial scar is usually formed by the activated astrocytes. Many receptors on the surface of astrocytes receive various inflammatory factors. Hence, the destruction of the microenvironment can easily lead to the activation of astrocytes (Giovannoni and Quintana, 2020). Furthermore, cytokines, pathogen associated molecular patterns (PAMPs), damage-associated molecular patterns (DAMPs), and growth factors can cause astrocytic activation (Figure 1). These factors increase the expressions of vimentin, actin, chondroitin sulfate proteoglycans (CSPGs) and glial fibrillary acidic protein (GFAP), the activations of MAPK and NF-κB pathways, and STAT protein phosphorylation on astrocytes (Phuagkhaopong et al., 2017; Yuan et al., 2017; Han et al., 2018; Potokar et al., 2020). Obviously, reducing or clearing the expression of these factors can effectively inhibit the activations of astrocytes and inflammatory reactions, and improve the injury recovery. Currently, the glial scar of SCI can be directly removed by surgery, however, this poses certain risk to the patient. Thus, ameliorating this reactive process is a key factor.
The glial scar blocks the axonal growth that is key to two aspects: (1) It hinders the connection of the spinal cord tract (Bradbury and Burnside, 2019); (2) it secretes molecules such as CSPGs and GFAP to inhibit the neuronal growth and plasticity. The upregulation of CSPG and GFAP expressions is an indication of astrocyte activation (Sun et al., 2020). Studies have shown that the regenerative ability of axons can be significantly restored by inhibiting CSPGs expression. For example, in a study by Tran et al. (2018) the failure of axonal regeneration upregulated CSPGs expression after SCI. However, the degradation of CSPGs could promote the axonal regeneration and recovery by hindering CSPGs to bind to PTPσ (Tran et al., 2018). Similarly, Xu et al. (2020) showed that Rg-1 could downregulate CSPGs expression after SCI, reduce the cavity area after injury, and promote hind limb recovery in mice. Therefore, lowering the expression level of CSPGs in glial scar could promote the axonal growth and enhance SCI recuperation. However, the downregulation of CSPGs required inhibiting the degree of astrocyte activation by reducing the expression of various pro-inflammatory factors and apoptotic factors within astrocytes.
The high mobility group box-1/toll-like receptor4/nuclear factor-kappa B signaling pathway
HMGB1, as a protein of the 215 amino acids, is located in a stable environment of the nucleus. In healthy cells, HMGB1 plays a non-histone role in the nucleus that is key to maintain the nucleosome stability and DNA transcription (Kim et al., 2021). HMGB1 can be secreted via two ways: One is actively secreted by the immune system as a warning signal during cell stress; the other is aggressively secreted by the necrotic cells (Manivannan et al., 2020). In general, the active and passive secretions of HMGB1s promote each other. In the wake of cells damaged, a large number of active HMGB1s are secreted from the nucleus to the cytoplasm and extracellular, and various HMGB1 responsive receptors in the cytoplasm or cell membrane surface are activated, and then promote a series of inflammation and apoptosis. These result in the secretions of more inflammatory factors, including the caspase family factors, TNF-α, IL-1β and IL-6 (Zheng et al., 2021). These cytokines induce cellular stress and trigger cells to release more HMGB1s. HMGB1s are secreted out of cells that can play a role in cytokines, and may induce the leukocytes to the injury tissues and exert the immune effects (Mu et al., 2019).
Human HMGB1 consists of three functional domains: A-box, B-box, and C-tail (Hazlett et al., 2021). The B-box domain participates in inflammation by binding to its receptors on the cell surface. The A-box competitively antagonizes the function of the B-box domain and weakens its inflammatory effect. The C-tail is involved in regulating the binding of HMGB1 to the DNA (Hazlett et al., 2021). The HMGB1 receptors include TLR2, TLR4, and RAGE (Xue et al., 2021). HMGB1s bind to corresponding receptors that produce a larger and more complex danger signals, and then activate a series of related inflammatory signaling pathways. TLRs are important receptors that bind the endogenous factors of cells (Arnaboldi et al., 2020), and induce the activation of the NF-κB signaling pathway. Moreover, the TLR4 is a key player in promoting inflammation in various diseases. The binding of HMGB1 and TLR4, TLR4 primarily recognizes myeloid discrimination protein 2 (MD-2) through the B-box domain of the HMGB1, and then triggers inflammation (Sun et al., 2019b). Notably, the combination of fluoroquinolone antibiotics in the hydrophobic region of MD-2 reduces the TLR4-MD-2 dimerization, while curtails the effectiveness of the TLR4 and I-κB kinase. The above events contribute to cell resistance to inflammation (Zusso et al., 2019). Usually, the extracellular inflammatory factors trigger the corresponding receptors on cell membrane to change its conformation and activate I-κB kinase. When cells are stimulated, TLRs recruit myeloid differentiation factor 88 (MyD88) that a key protein activates I-κB kinase. The inhibition of MyD88 expression blocks the NF-κB signaling pathway and reduces the risk of inflammatory response (Kiripolsky et al., 2020). The knockout of MyD88 gene of tumor cells inhibits their growth and migration by decreasing the activity of NF-κB (Zhu et al., 2020). Activated I-κB kinase further induces the phosphorylation or degradation of IκB-α to cause the dissociation and translocation of the p65/RelA pathway to the nucleus. If the P65/RelA enters into the nucleus and binds to the corresponding DNA, it will begin to active the inflammatory-related genes. It has a significant therapeutic effect on LPS-induced acute lung injury by using HMGB1 to impair the TLR4/MyD88/NF-κB pathway (Meng et al., 2018). Also, a significant downregulation of the HMGB1 activity can ameliorate neuropathic pain. In addition, the binding of the HMGB1 and TLR4 promotes the phosphorylations of the extracellular regulated protein kinases1/2 (ERK1/2) and dynamin-related protein 1 (Drp1), and the signals are translocated to the mitochondria and cause the mitochondrial rupture (Feng et al., 2021). RAGE, as an important receptor of HMGB1, has been widely studied. Noteworthy, HMGB1 can induce the ERK1/2 phosphorylation by binding to the RAGE, further triggering the activation of the Janus kinases 2/signal transducer and activator of transcription 3 (JAK2/STAT3) signaling pathway, which in turn causes the metabolic abnormalities and apoptosis (Zhang et al., 2019). Further, the combination of HMGB1 and RAGE can instigate the activation of JNK/NF-κB signaling pathway to promote the occurrence of inflammation (Wu et al., 2013). Following the combination of the extracellular HMGB1 and RAGE, a variety of extracellular cytokines are encapsulated and carried out to the lysosomes by intracellular endocytosis, and convey the detrimental information to cells (Andersson et al., 2018). Also, the HMGB1/RAGE/cathepsin B signaling pathway activates the nod-like receptor protein-3 (NLRP3) inflammasome, promotes the expressions of caspase family proteins, and triggers the inflammation and apoptosis (Jia et al., 2019).
In addition to the inflammation and apoptosis, the activity of HMGB1 is closely related to oxidative stress (Pauletti et al., 2019). Studies have demonstrated that the high secretion of HMGB1 is accompanied with the expression of reactive oxygen species (ROS) (Min et al., 2021; Zhou et al., 2021). The nuclear factor E2-related factor 2 (Nrf2) is an important protein in the antioxidant system, and its activation promotes the transcriptions and expressions of a variety of antioxidant and cytoprotective proteins in cells. In oxidative stress, the combination of HMGB1 and RAGE not only compromises Nrf2 translocation to the nucleus, but also reduces the activity and expression of Nrf2, and causes the imbalance of antioxidant system (Wang et al., 2020b; Arab et al., 2021; Figure 2).
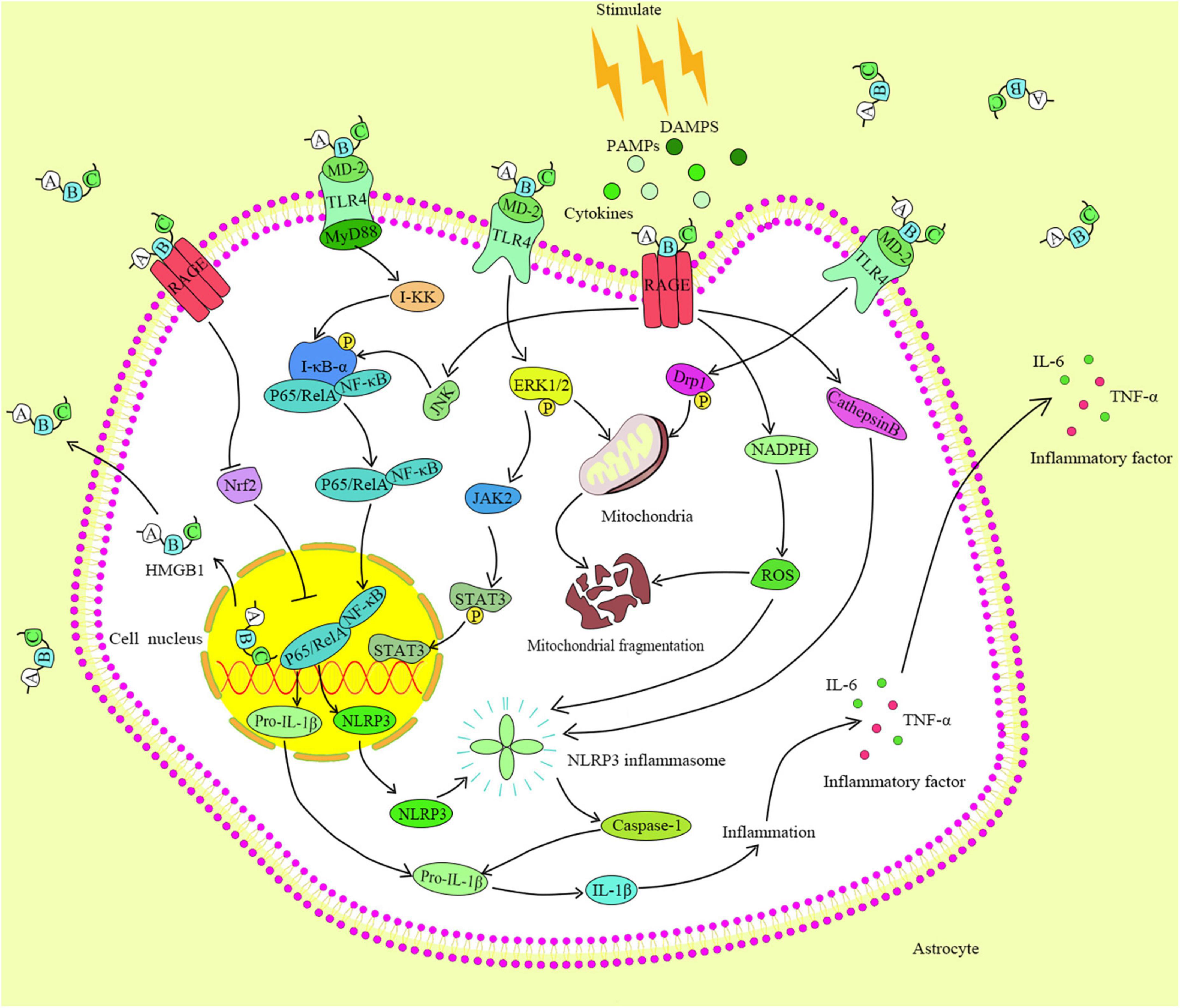
Figure 2. HMGB1 identification and its binding are by different receptors on the cell membrane. The pairing of TLR4 with HMGB1 activates the MyD88, which in turn activates the I-κB kinase and NF-κB pathway, and triggers the expressions of downstream factors.
The increased HMGB1 is related to astrocytic activation, which increases the expression of aquaporins on the surface of astrocytes and causes cell edema (Sun et al., 2017). Interestingly, when HMGB1 expression is increased, astrocytic functions are also intensified (Zhao et al., 2020). The extracellular HMGB1 activates astrocytes, which may promote the intracellular inflammatory signaling pathways by binding with the receptors on the membrane. However, the specific mechanism is presently unclear, and still needs further studies.
Ethyl pyruvate
The anti-inflammatory role of ethyl pyruvate
Pyruvate, as a neuroprotective agent, plays an important role in the glycolysis and tricarboxylic acid cycle. Pyruvate has long been studied as a scavenger of free radicals in vivo, as it can eliminate the ROS and H2O2, and inhibits the activity of endogenous cytokines to reduce oxidative stress response after cell injury (Guarino et al., 2019; Zhang et al., 2020, 2021). EP is an ester derivative of pyruvate, has better stability than pyruvate, and is an effective anti-inflammatory and anti-cancer agent that has been employed in several disease treatments (Table 1). EP can significantly reduce the expression of some cytokines (These cytokines are paramount in the occurrence and development of tumors.) to block inflammatory response. The anti-inflammatory effect of EP may be by inhibiting the activation of NLRP3 inflammasome and reducing the expressions of caspase-1 and IL-1β (Li et al., 2018). In sepsis-associated inflammation, EP inhibits the activity of NLRP3 inflammasome (Zhong et al., 2020).
The NF-κB signaling pathway after activation can promote the immune, inflammatory and stress responses of the cells and tissues. The phosphorylation of resting NF-κB by activating I-κB kinase that is the first step in NF-κB activation. Following an intricate intermediate reaction, the exposed p65 and p50 active the sites of NF-κB to enter the nucleus and participate in the transcription process of inflammatory factors (Colombo et al., 2018). EP has shown its potent repressive effect on p50/p65, thus being a potential inhibitor of NF-κB (Sharma et al., 2015). Johansson et al. (2008) showed EP could reduce the activities of I-κB kinase and p65 protein while regulating the stress response of neutrophils to the adhesion factors or cytokines. In addition, EP inhibited the activity of NF-κB before gene transcription. The propensity of EP inhibits the NF-κB signaling pathway that has compelled several scientists to employ it as a therapeutic agent for various inflammatory diseases and cancers. In addition, extensive researches on the neuroprotective role of EP has been conducted. For instance, the neuroinflammation and demyelination are most common pathological features in multiple sclerosis. EP significantly impaired the inflammatory response, enhanced the myelin sheath regeneration and recovery, and reduced the loss of oligodendrocytes to improve the behavioral ability of animals in a cuprizone-induced mouse model (He et al., 2019).
Ethyl pyruvate hinders the high mobility group box-1 signaling pathway
The regulatory mechanism of EP in terms of HMGB1 has been extensively studied. EP can inhibit HMGB1 secretion and alleviate inflammatory response. Ca2+ is involved in the release of HMGB1 (Zou et al., 2015). Interestingly, the extrication of HMGB1 is considerably impaired, which following EP enhance Ca2+ sequestration (Shin et al., 2015). Moreover, mesenchymal stem cells have been suggested as a probable treatment strategy for the treatment of systemic lupus erythematosus (Yuan et al., 2019). However, the conundrum regarding the aging and death of stem cell that can lead to the recurrence of the disease, which is yet to be unraveled. Not surprisingly, HMGB1 necessitates the ineffectiveness of stem cell function and promotes inflammatory response. In Ji et al. (2019) study corroborating the above statement, they also found EP to enhance the regulatory T-cells. This was concomitant with the reduced deterioration of bone marrow mesenchymal stem cells in MRL/lpr mice, as well as the significant inhibition of HMGB1 expression and TLR4/NF-κB signaling pathway. Also, EP improves cancer treatment by inhibiting the HMGB1 secretion. Nonetheless, EP treatment significantly inhibits the recognition of these two proteins, augments the death of tumor cells, inhibits the NF-κB/STAT3 pathway, and hinders the instigation of inflammatory response (Liu et al., 2019a). As another receptor of HMGB1, TLR4 can recognize and bind to the disulfide HMGB1. An intramolecular disulfide bond that exists between Cys23 and Cys45, and triggers an inflammatory response (Kwak et al., 2019). EP can significantly curtail the binding between TLR4 and HMGB1.
Ethyl pyruvate attenuates astrocytic activation to improve spinal cord injury
In the wake of SCI, the excessive reaction of glial cells and inflammation cause the destruction of microenvironment at the injured sites. This complicates the neuronal recovery after injury. EP has been demonstrated potential anti-inflammatory and neuroprotective effects, is applied in the treatment of various diseases (Wagner et al., 2018; Liu et al., 2019b). In particular, EP treatment reduced the proliferation of over-activated astrocytes, prevented the neuroinflammation development, and promoted the axonal growth, along with the restoration of hind limb function in the SCI animal model (Sun et al., 2017). In both in vivo and in vitro studies, EP has been evidenced to significantly reduce the expression of neurotoxic and inflammatory factors (such as ROS, IL-1β, TNF-α, and HMGB1) produced by the impaired neurons (Birkenmeier et al., 2016; Dong et al., 2019a). These endogenous neurotoxic factors are conspicuous in the activation of astrocytes. Moreover, He et al. (2021) showed that the EP-treated astrocytes can upregulate the expressions of a variety of neurotrophic factors, including ciliary neurotrophic factor (CNTF) and brain-derived neurotrophic factor (BDNF). These neurotrophic factors are important conditions for axonal regeneration. Also, the extrication of HMGB1 by the damaged cells has previously been found to activate astrocytes (Hayakawa et al., 2010). Interestingly, EP indicates its neuroprotective effect on spinal cord neurons by impairing the cell apoptosis and HMGB1 release (Wang et al., 2009). Neuroinflammation is a key factor that induces astrocytic activation. The GFAP is often used as an important indicator of astrocytic activation. The activation of astrocytes appears to be related to several activations of inflammatory pathways. Lipopolysaccharide is usually used to induce the neuroinflammation in cells. Studies on lipopolysaccharide-induced the activation of astrocytes have revealed that the activities of the MAPK, STAT3, and NF-κB are enhanced, and targeted inhibition of these signaling pathways may significantly mitigate the activation of astrocytes and impair the secretion of various cytokines (Che et al., 2020). Olcum et al. (2021) explored the inhibitory process of EP on NLRP3 inflammasomes in the activated microglia, and found it to incapacitate cell activation induced by inflammasomes through facilitating the impaired activation of the HMGB1/NF-κB axis and inhibiting the secretion of multiple cytokines. In astrocytes, the stimulation of the HMGB1/NF-κB axis triggers cell activation, along with the significant upregulation of the GFAP expression (Zhao et al., 2020). These results suggest that EP might inhibit astrocyte activation through the HMGB1/NF-κB axis. EP promoted the axonal growth of spinal cord tract, induced the microglia regeneration and differentiation, and promoted the recovery of SCI in mice (He et al., 2019). The deletion of Rac1 gene or the induction of SOCS1 resulted in microglia activation, and EP treatment inhibited the activated microglia by regulating JAK/STAT pathway (Kim et al., 2008). Furthermore, JAK/STAT pathway is related to the activation of astrocytes (Lee et al., 2019b), and activation of the JAK/STAT pathway leads to the phosphorylation of STAT and translocates to the nucleus, where the associated inflammatory factors are transcribed. Moreover, in the activated astrocytes, the phosphorylation of the JAK/STAT signaling pathway positively correlates with the expression of GFAP (Wang et al., 2020c). In addition, some studies have shown the anti-inflammatory and neuroprotective effects of EP through regulating the MAPK/NF-κB pathway in the treatment of SCI (Genovese et al., 2009).
Conclusion
The complete treatment of SCI is a current persistent medical conundrum owing to chronic inflammation that complicates injury recovery, together with the formation of glial scar by astrocytes that hinders axonal regeneration of the spinal cord tract. Astrocytes are the most glial cells in the central nervous system, and are involved in neuroprotection. However, under unfavorable conditions, astrocytes proliferate through excessive activation, and cause harm to the body. Several studies have evidenced the adverse effects of activated astrocytes in SCI, and to inhibit this activation process is to attenuate cytokines. Additionally, extracellular HMGB1 stimulates astrocytes, triggers cell inflammation, and aggravates glial scars. The TLR4 on astrocyte membrane is the ligand that receives HMGB1. Following successful pairing, the HMGB1-TLR4 activates I-κB kinase that stimulates NF-κB and causes the downstream inflammatory response. EP, as a stable anti-inflammatory and neuroprotective agent, can effectively mitigate the proliferation and inflammatory response of astrocytes by inhibiting the HMGB1/TLR4/NF-κB pathway, and enhance the functional recovery of SCI patients. EP could be a potent ameliorative avenue to SCI. However, further studies need to unravel other possible mechanisms mediated by EP, along with the elucidation of probable side effects before being employed in the clinical setting.
Author contributions
XL designed the study. RF, LW, YZ, BB, and XL prepared the first draft of the manuscript and revised the manuscript. All authors approved the final manuscript.
Funding
This work was supported by the Natural Science Foundation of Zhejiang Province (no. LY19H170001).
Conflict of interest
The authors declare that the research was conducted in the absence of any commercial or financial relationships that could be construed as a potential conflict of interest.
Publisher’s note
All claims expressed in this article are solely those of the authors and do not necessarily represent those of their affiliated organizations, or those of the publisher, the editors and the reviewers. Any product that may be evaluated in this article, or claim that may be made by its manufacturer, is not guaranteed or endorsed by the publisher.
Abbreviations
BDNF, Brain-derived neurotrophic factor; CNTF, Ciliary neurotrophic factor; CSPGs, Chondroitin sulfate proteoglycans; DAMPs, Damage-associated molecular patterns; Drp1, Dynamin-related protein 1; EP, Ethyl pyruvate; ERK1/2, Extracellular regulated protein kinases1/2; GFAP, Glial fibrillary acidic protein; HMGB1, High mobility group box-1; JAK2, Janus kinases 2; JNK, c-JunN-terminal kinase; MAPK, Mitogen-activated protein kinase; MD-2, Myeloid discrimination protein 2; MyD88, Myeloid differentiation factor 88; NF- κ B, Nuclear factor-kappa B; NLRP3, Nod-like receptor protein-3; Nrf2, Nuclear factor E2-related factor 2; PAMPs, Pathogen associated molecular patterns; RAGE, Receptor of advanced glycation end product; ROS, Reactive oxygen species; SCI, Spinal cord injury; STAT3, Signal transducer and activator of transcription 3; TLR, Toll-like receptor.
References
Andersson, U., Yang, H., and Harris, H. (2018). High-mobility group box 1 protein (HMGB1) operates as an alarmin outside as well as inside cells. Semin. Immunol. 38, 40–48. doi: 10.1016/j.smim.2018.02.011
Arab, H. H., Al-Shorbagy, M. Y., and Saad, M. A. (2021). Activation of autophagy and suppression of apoptosis by dapagliflozin attenuates experimental inflammatory bowel disease in rats: Targeting AMPK/mTOR, HMGB1/RAGE and Nrf2/HO-1 pathways. Chem. Biol. Interact. 335:109368. doi: 10.1016/j.cbi.2021.109368
Arnaboldi, F., Sommariva, M., Opizzi, E., Rasile, M., Camelliti, S., Busnelli, M., et al. (2020). Expression of Toll-like receptors 4 and 7 in murine peripheral nervous system development. Ann. Anat. 231:151526. doi: 10.1016/j.aanat.2020.151526
Bendella, H., Rink, S., Wöhler, A., Feiser, J., Wilden, A., Grosheva, M., et al. (2019). Anatomic conditions for bypass surgery between rostral (T7-T9) and caudal (L2, L4, S1) ventral roots to treat paralysis after spinal cord injury. Ann. Anat. 222, 139–145. doi: 10.1016/j.aanat.2018.12.008
Birkenmeier, G., Hemdan, N. Y., Kurz, S., Bigl, M., Pieroh, P., Debebe, T., et al. (2016). Ethyl pyruvate combats human leukemia cells but spares normal blood cells. PLoS One 11:e0161571. doi: 10.1371/journal.pone.0161571
Bradbury, E. J., and Burnside, E. R. (2019). Moving beyond the glial scar for spinal cord repair. Nat. Commun. 10:3879. doi: 10.1038/s41467-019-11707-7
Casula, M., Iyer, A. M., Spliet, W. G., Anink, J. J., Steentjes, K., Sta, M., et al. (2011). Toll-like receptor signaling in amyotrophic lateral sclerosis spinal cord tissue. Neuroscience 179, 233–243. doi: 10.1016/j.neuroscience.2011.02.001
Chakhtoura, M., Chain, R. W., Sato, P. Y., Qiu, C. C., Lee, M. H., Meissler, J. J., et al. (2019). Ethyl pyruvate modulates murine dendritic cell activation and survival through their immunometabolism. Front. Immunol. 10:30. doi: 10.3389/fimmu.2019.00030
Chavali, V. D., Agarwal, M., Vyas, V. K., and Saxena, B. (2020). Neuroprotective effects of ethyl pyruvate against aluminum chloride-induced Alzheimer’s disease in rats via inhibiting toll-like receptor 4. J. Mol. Neurosci. 70, 836–850. doi: 10.1007/s12031-020-01489-9
Che, D. N., Cho, B. O., Kim, J. S., Shin, J. Y., Kang, H. J., and Jang, S. I. (2020). Luteolin and apigenin attenuate LPS-induced astrocyte activation and cytokine production by targeting MAPK, STAT3, and NF-κB signaling pathways. Inflammation 43, 1716–1728. doi: 10.1007/s10753-020-01245-6
Colombo, J., Jardim-Perassi, B. V., Ferreira, J. P. S., Braga, C. Z., Sonehara, N. M., Júnior, R. P., et al. (2018). Melatonin differentially modulates NF-êB expression in breast and liver cancer cells. Anticancer Agents Med. Chem. 18, 1688–1694. doi: 10.2174/1871520618666180131112304
Cox, A., Capone, M., Matzelle, D., Vertegel, A., Bredikhin, M., Varma, A., et al. (2021). Nanoparticle-based estrogen delivery to spinal cord injury site reduces local parenchymal destruction and improves functional recovery. J. Neurotrauma 38, 342–352. doi: 10.1089/neu.2020.7047
Dieteren, S., Franz, N., Köhler, K., Nowak, A., Ehnert, S., Surov, A., et al. (2020). Ethyl pyruvate reduces systemic leukocyte activation via caspase-1 and NF-κB after blunt chest trauma and haemorrhagic shock. Front. Med. 7:562904. doi: 10.3389/fmed.2020.562904
Djedović, N., Stanisavljevic, S., Jevtić, B., Momčilović, M., Lavrnja, I., and Miljković, D. (2017). Anti-encephalitogenic effects of ethyl pyruvate are reflected in the central nervous system and the gut. Biomed. Pharmacother. 96, 78–85. doi: 10.1016/j.biopha.2017.09.110
Dong, N., Xu, X., Xue, C., Wang, C., Li, X., Bi, C., et al. (2019a). Ethyl pyruvate inhibits LPS induced IPEC-J2 inflammation and apoptosis through p38 and ERK1/2 pathways. Cell Cycle 18, 2614–2628. doi: 10.1080/15384101.2019.1653106
Dong, N., Xu, X., Xue, C., Wang, C., Li, X., Shan, A., et al. (2019b). Ethyl pyruvate protects against Salmonella intestinal infection in mice through down-regulation of pro-inflammatory factors and inhibition of TLR4/MAPK pathway. Int. Immunopharmacol. 71, 155–163. doi: 10.1016/j.intimp.2019.03.019
Du, Y., Xu, C. L., Yu, J., Liu, K., Lin, S. D., Hu, T. T., et al. (2022). HMGB1 in the mPFC governs comorbid anxiety in neuropathic pain. J. Headache Pain 23:102. doi: 10.1186/s10194-022-01475-z
Edwards-Faret, G., González-Pinto, K., Cebrián-Silla, A., Peñailillo, J., García-Verdugo, J. M., and Larraín, J. (2021). Cellular response to spinal cord injury in regenerative and non-regenerative stages in Xenopus laevis. Neural Dev. 16:2. doi: 10.1186/s13064-021-00152-2
Fan, H., Tang, H. B., Chen, Z., Wang, H. Q., Zhang, L., Jiang, Y., et al. (2020). Inhibiting HMGB1-RAGE axis prevents pro-inflammatory macrophages/microglia polarization and affords neuroprotection after spinal cord injury. J. Neuroinflammation 17:295. doi: 10.1186/s12974-020-01973-4
Feng, W., Wang, J., Yan, X., Zhang, Q., Chai, L., Wang, Q., et al. (2021). /Drp1-dependent mitochondrial fission contributes to HMGB1-induced autophagy in pulmonary arterial hypertension. Cell Prolif. 54:e13048. doi: 10.1111/cpr.13048
Fouad, K., Popovich, P. G., Kopp, M. A., and Schwab, J. M. (2021). The neuroanatomical-functional paradox in spinal cord injury. Nat. Rev. Neurol. 17, 53–62. doi: 10.1038/s41582-020-00436-x
Genovese, T., Esposito, E., Mazzon, E., Di Paola, R., Meli, R., Caminiti, R., et al. (2009). Beneficial effects of ethyl pyruvate in a mouse model of spinal cord injury. Shock 32, 217–227. doi: 10.1097/SHK.0b013e31818d4073
Giovannoni, F., and Quintana, F. J. (2020). The role of astrocytes in CNS inflammation. Trends Immunol. 41, 805–819. doi: 10.1016/j.it.2020.07.007
Guarino, V. A., Oldham, W. M., Loscalzo, J., and Zhang, Y. Y. (2019). Reaction rate of pyruvate and hydrogen peroxide: assessing antioxidant capacity of pyruvate under biological conditions. Sci. Rep. 9:19568. doi: 10.1038/s41598-019-55951-9
Haga, H., Matsuo, K., Yabuki, Y., Zhang, C., Han, F., and Fukunaga, K. (2019). Enhancement of ATP production ameliorates motor and cognitive impairments in a mouse model of MPTP-induced Parkinson’s disease. Neurochem. Int. 129:104492. doi: 10.1016/j.neuint.2019.104492
Han, C. L., Ge, M., Liu, Y. P., Zhao, X. M., Wang, K. L., Chen, N., et al. (2018). LncRNA H19 contributes to hippocampal glial cell activation via JAK/STAT signaling in a rat model of temporal lobe epilepsy. J. Neuroinflammation 15:103. doi: 10.1186/s12974-018-1139-z
Hayakawa, K., Arai, K., and Lo, E. H. (2010). Role of ERK map kinase and CRM1 in IL-1beta-stimulated release of HMGB1 from cortical astrocytes. Glia 58, 1007–1015. doi: 10.1002/glia.20982
Hazlett, L. D., McClellan, S., Somayajulu, M., and Bessert, D. (2021). Targeting inflammation driven by HMGB1 in bacterial keratitis-a review. Pathogens 10:1235. doi: 10.3390/pathogens10101235
He, Y., An, J., Yin, J. J., Miao, Q., Sui, R. X., Han, Q. X., et al. (2021). Ethyl pyruvate-derived transdifferentiation of astrocytes to oligodendrogenesis in cuprizone-induced demyelinating model. Neurotherapeutics 18, 488–502. doi: 10.1007/s13311-020-00947-x
He, Y., An, J., Yin, J. J., Sui, R. X., Miao, Q., Ding, Z. B., et al. (2019). Ethyl pyruvate enhances spontaneous remyelination by targeting microglia phagocytosis. Int. Immunopharmacol. 77:105929. doi: 10.1016/j.intimp.2019.105929
Huang, B., Lv, D. J., Wang, C., Shu, F. P., Gong, Z. C., Xie, T., et al. (2018). Suppressed epithelial-mesenchymal transition and cancer stem cell properties mediate the anti-cancer effects of ethyl pyruvate via regulation of the AKT/nuclear factor-κB pathway in prostate cancer cells. Oncol. Lett. 16, 2271–2278. doi: 10.3892/ol.2018.8958
Huang, Q., Fu, Y., Zhang, S., Zhang, Y., Chen, S., and Zhang, Z. (2020). Ethyl pyruvate inhibits glioblastoma cells migration and invasion through modulation of NF-κB and ERK-mediated EMT. PeerJ 8:e9559. doi: 10.7717/peerj.9559
Ji, J., Fu, T., Dong, C., Zhu, W., Yang, J., Kong, X., et al. (2019). Targeting HMGB1 by ethyl pyruvate ameliorates systemic lupus erythematosus and reverses the senescent phenotype of bone marrow-mesenchymal stem cells. Aging 11, 4338–4353. doi: 10.18632/aging.102052
Jia, C., Zhang, J., Chen, H., Zhuge, Y., Chen, H., Qian, F., et al. (2019). Endothelial cell pyroptosis plays an important role in Kawasaki disease via HMGB1/RAGE/cathespin B signaling pathway and NLRP3 inflammasome activation. Cell Death Dis. 10:778. doi: 10.1038/s41419-019-2021-3
Johansson, A. S., Johansson-Haque, K., Okret, S., and Palmblad, J. (2008). Ethyl pyruvate modulates acute inflammatory reactions in human endothelial cells in relation to the NF-kappaB pathway. Br. J. Pharmacol. 154, 1318–1326. doi: 10.1038/bjp.2008.201
Jun, J. H., Song, J. W., Shin, E. J., Kwak, Y. L., Choi, N., and Shim, J. K. (2018). Ethyl pyruvate is renoprotective against ischemia-reperfusion injury under hyperglycemia. J. Thorac. Cardiovasc. Surg. 155, 1650–1658. doi: 10.1016/j.jtcvs.2017.10.069
Kim, H. C., Oh, H., You, J. S., and Chung, Y. E. (2021). Glycyrrhizin ameliorating sterile inflammation induced by low-dose radiation exposure. Sci. Rep. 11:18356. doi: 10.1038/s41598-021-97800-8
Kim, H. S., Cho, I. H., Kim, J. E., Shin, Y. J., Jeon, J. H., Kim, Y., et al. (2008). Ethyl pyruvate has an anti-inflammatory effect by inhibiting ROS-dependent STAT signaling in activated microglia. Free Radic. Biol. Med. 45, 950–963. doi: 10.1016/j.freeradbiomed.2008.06.009
Kiripolsky, J., Romano, R. A., Kasperek, E. M., Yu, G., and Kramer, J. M. (2020). Activation of Myd88-dependent TLRs mediates local and systemic inflammation in a mouse model of primary sjögren’s syndrome. Front. Immunol. 10:2963. doi: 10.3389/fimmu.2019.02963
Koprivica, I., Vujičić, M., Gajić, D., Saksida, T., and Stojanović, I. (2019). Ethyl pyruvate stimulates regulatory T cells and ameliorates type 1 diabetes development in mice. Front. Immunol. 9:3130. doi: 10.3389/fimmu.2018.03130
Kumar, H., Lim, C. S., Choi, H., Joshi, H. P., Kim, K. T., Kim, Y. H., et al. (2020). Elevated TRPV4 levels contribute to endothelial damage and scarring in experimental spinal cord injury. J. Neurosci. 40, 1943–1955. doi: 10.1523/JNEUROSCI.2035-19.2020
Kwak, M. S., Kim, H. S., Lkhamsuren, K., Kim, Y. H., Han, M. G., Shin, J. M., et al. (2019). Peroxiredoxin-mediated disulfide bond formation is required for nucleocytoplasmic translocation and secretion of HMGB1 in response to inflammatory stimuli. Redox Biol. 24:101203. doi: 10.1016/j.redox.2019.101203
Lee, H. C., Md Yusof, HH, Leong, M. P., Zainal, Abidin S, Seth, E. A., Hewitt, C. A., et al. (2019a). Gene and protein expression profiles of JAK-STAT signalling pathway in the developing brain of the Ts1Cje down syndrome mouse model. Int. J. Neurosci. 129, 871–881. doi: 10.1080/00207454.2019.1580280
Lee, H. K., Park, J. Y., Lee, H., Kim, I. D., Kim, S. W., Yoon, S. H., et al. (2019b). Anti-inflammatory and neuroprotective effects of DIPOPA (N,N-Diisopropyl-2-Oxopropanamide), an ethyl pyruvate bioisoster, in the postischemic brain. Neurotherapeutics 16, 523–537. doi: 10.1007/s13311-019-00711-w
Leibinger, M., Zeitler, C., Gobrecht, P., Andreadaki, A., Gisselmann, G., and Fischer, D. (2021). Transneuronal delivery of hyper-interleukin-6 enables functional recovery after severe spinal cord injury in mice. Nat. Commun. 12:391. doi: 10.1038/s41467-020-20112-4
Li, S., Liang, F., Kwan, K., Tang, Y., Wang, X., Tang, Y., et al. (2018). Identification of ethyl pyruvate as a NLRP3 inflammasome inhibitor that preserves mitochondrial integrity. Mol. Med. 24:8. doi: 10.1186/s10020-018-0006-9
Liang, W. J., Yang, H. W., Liu, H. N., Qian, W., and Chen, X. L. (2020). HMGB1 upregulates NF-kB by inhibiting IKB-α and associates with diabetic retinopathy. Life Sci. 241:117146. doi: 10.1016/j.lfs.2019.117146
Liu, F., Wang, Z., Qiu, Y., Wei, M., Li, C., Xie, Y., et al. (2017). Suppression of MyD88-dependent signaling alleviates neuropathic pain induced by peripheral nerve injury in the rat. J. Neuroinflammation 14:70. doi: 10.1186/s12974-017-0822-9
Liu, Q., Huo, Y., Zheng, H., Zhao, J., Jia, L., and Wang, P. (2019a). Ethyl pyruvate suppresses the growth, invasion and migration and induces the apoptosis of non small cell lung cancer cells via the HMGB1/RAGE axis and the NF κB/STAT3 pathway. Oncol. Rep. 42, 817–825. doi: 10.3892/or.2019.7176
Liu, Y. Y., Chen, N. H., Chang, C. H., Lin, S. W., Kao, K. C., Hu, H. C., et al. (2019b). Ethyl pyruvate attenuates ventilation-induced diaphragm dysfunction through high-mobility group box-1 in a murine endotoxaemia model. J. Cell Mol. Med. 23, 5679–5691. doi: 10.1111/jcmm.14478
Lv, B., Zhang, X., Yuan, J., Chen, Y., Ding, H., Cao, X., et al. (2021). Biomaterial-supported MSC transplantation enhances cell-cell communication for spinal cord injury. Stem Cell Res. Ther. 12:36. doi: 10.1186/s13287-020-02090-y
Manivannan, S., Harari, B., Muzaffar, M., Elalfy, O., Hettipathirannahelage, S., James, Z., et al. (2020). Glycyrrhizin blocks the detrimental effects of HMGB1 on cortical neurogenesis after traumatic neuronal injury. Brain Sci. 10:760. doi: 10.3390/brainsci10100760
Mao, L., Sun, L., Sun, J., Sun, B., Gao, Y., and Shi, H. (2021). Ethyl pyruvate improves white matter remodeling in rats after traumatic brain injury. CNS Neurosci. Ther. 27, 113–122. doi: 10.1111/cns.13534
Meng, L., Li, L., Lu, S., Li, K., Su, Z., Wang, Y., et al. (2018). The protective effect of dexmedetomidine on LPS-induced acute lung injury through the HMGB1-mediated TLR4/NF-κB and PI3K/Akt/mTOR pathways. Mol. Immunol. 94, 7–17. doi: 10.1016/j.molimm.2017.12.008
Min, H. J., Park, J. S., Kim, K. S., Park, S. Y., Choi, H., Seo, J. H., et al. (2021). Th2 cytokines-DUOX2-ROS-HMGB1 translocation axis is important in the pathogenesis of allergic rhinitis. Clin. Sci. 135, 483–494. doi: 10.1042/CS20201212
Mu, S. W., Dang, Y., Fan, Y. C., Zhang, H., Zhang, J. H., Wang, W., et al. (2019). Effect of HMGB1 and RAGE on brain injury and the protective mechanism of glycyrrhizin in intracranial-sinus occlusion followed by mechanical thrombectomy recanalization. Int. J. Mol. Med. 44, 813–822. doi: 10.3892/ijmm.2019.4248
Okada, S., Hara, M., Kobayakawa, K., Matsumoto, Y., and Nakashima, Y. (2018). Astrocyte reactivity and astrogliosis after spinal cord injury. Neurosci. Res. 126, 39–43. doi: 10.1016/j.neures.2017.10.004
Olcum, M., Tufekci, K. U., Durur, D. Y., Tastan, B., Gokbayrak, I. N., Genc, K., et al. (2021). Ethyl pyruvate attenuates microglial NLRP3 inflammasome activation via inhibition of HMGB1/NF-κB/miR-223 signaling. Antioxidants 10:745. doi: 10.3390/antiox10050745
Pauletti, A., Terrone, G., Shekh-Ahmad, T., Salamone, A., Ravizza, T., Rizzi, M., et al. (2019). Targeting oxidative stress improves disease outcomes in a rat model of acquired epilepsy. Brain 142:e39. doi: 10.1093/brain/awz130
Phuagkhaopong, S., Ospondpant, D., Kasemsuk, T., Sibmooh, N., Soodvilai, S., Power, C., et al. (2017). Cadmium-induced IL-6 and IL-8 expression and release from astrocytes are mediated by MAPK and NF-κB pathways. Neurotoxicology 60, 82–91. doi: 10.1016/j.neuro.2017.03.001
Potokar, M., Morita, M., Wiche, G., and Jorgačevski, J. (2020). The diversity of intermediate filaments in astrocytes. Cells 9:1604. doi: 10.3390/cells9071604
Qiu, X., Cheng, X., Zhang, J., Yuan, C., Zhao, M., and Yang, X. (2020). Ethyl pyruvate confers protection against endotoxemia and sepsis by inhibiting caspase-11-dependent cell pyroptosis. Int. Immunopharmacol. 78:106016. doi: 10.1016/j.intimp.2019.106016
Sharma, V., Jordan, J. J., Ciribilli, Y., Resnick, M. A., Bisio, A., and Inga, A. (2015). Quantitative analysis of NF-κB transactivation specificity using a yeast-based functional assay. PLoS One. 10:e0130170. doi: 10.1371/journal.pone.0130170
Shen, C. H., Ma, Z. Y., Li, J. H., Li, R. D., Tao, Y. F., Zhang, Q. B., et al. (2020). Glycyrrhizin improves inflammation and apoptosis via suppressing HMGB1 and PI3K/mTOR pathway in lipopolysaccharide-induced acute liver injury. Eur. Rev. Med. Pharmacol. Sci. 24, 7122–7130. doi: 10.26355/eurrev_202006_21706
Shin, J. H., Kim, I. D., Kim, S. W., Lee, H. K., Jin, Y., Park, J. H., et al. (2015). Ethyl pyruvate inhibits HMGB1 phosphorylation and release by chelating calcium. Mol. Med. 20, 649–657. doi: 10.2119/molmed.2014.00039
Sun, J., Zhang, J., Li, K., Zheng, Q., Song, J., Liang, Z., et al. (2020). Photobiomodulation therapy inhibit the activation and secretory of astrocytes by altering macrophage polarization. Cell Mol. Neurobiol. 40, 141–152. doi: 10.1007/s10571-019-00728-x
Sun, L., Li, M., Ma, X., Feng, H., Song, J., Lv, C., et al. (2017). Inhibition of HMGB1 reduces rat spinal cord astrocytic swelling and AQP4 expression after oxygen-glucose deprivation and reoxygenation via TLR4 and NF-κB signaling in an IL-6-dependent manner. J. Neuroinflammation 14:231. doi: 10.1186/s12974-017-1008-1
Sun, L., Li, M., Ma, X., Zhang, L., Song, J., Lv, C., et al. (2019a). Inhibiting high mobility group box-1 reduces early spinal cord edema and attenuates astrocyte activation and aquaporin-4 expression after spinal cord injury in rats. J. Neurotrauma 36, 421–435. doi: 10.1089/neu.2018.5642
Sun, S., He, M., VanPatten, S., and Al-Abed, Y. (2019b). Mechanistic insights into high mobility group box-1 (HMGb1)-induced Toll-like receptor 4 (TLR4) dimer formation. J. Biomol. Struct. Dyn. 37, 3721–3730. doi: 10.1080/07391102.2018.1526712
Sun, X., Zhu, S., Dong, X., Strand-Amundsen, R. J., Tonnessen, T. I., and Yang, R. (2021). Ethyl pyruvate supplemented in drinking water ameliorates experimental nonalcoholic steatohepatitis. Biomed. Pharmacother. 137:111392. doi: 10.1016/j.biopha.2021.111392
Ta Na, H. S., Nuo, M., Meng, Q. T., and Xia, Z. Y. (2019). The pathway of Let-7a-1/2-3p and HMGB1 mediated dexmedetomidine inhibiting microglia activation in spinal cord ischemia-reperfusion injury mice. J. Mol. Neurosci. 69, 106–114. doi: 10.1007/s12031-019-01338-4
Tran, A. P., Sundar, S., Yu, M., Lang, B. T., and Silver, J. (2018). Modulation of receptor protein tyrosine phosphatase sigma increases chondroitin sulfate proteoglycan degradation through cathepsin B secretion to enhance axon outgrowth. J. Neurosci. 38, 5399–5414. doi: 10.1523/JNEUROSCI.3214-17.2018
Wagner, N., Dieteren, S., Franz, N., Köhler, K., Mörs, K., Nicin, L., et al. (2018). Ethyl pyruvate ameliorates hepatic injury following blunt chest trauma and hemorrhagic shock by reducing local inflammation, NF-kappaB activation and HMGB1 release. PLoS One 13:e0192171. doi: 10.1371/journal.pone.0192171
Wang, H., Yao, G., Li, L., Ma, Z., Chen, J., and Chen, W. (2020a). LncRNA-UCA1 inhibits the astrocyte activation in the temporal lobe epilepsy via regulating the JAK/STAT signaling pathway. J. Cell Biochem. 121, 4261–4270. doi: 10.1002/jcb.29634
Wang, H., Zhou, X. M., Wu, L. Y., Liu, G. J., Xu, W. D., Zhang, X. S., et al. (2020b). Aucubin alleviates oxidative stress and inflammation via Nrf2-mediated signaling activity in experimental traumatic brain injury. J. Neuroinflammation 17:188. doi: 10.1186/s12974-020-01863-9
Wang, Y., Shen, S., Li, Z., Li, W., and Weng, X. (2020c). MIR-140-5p affects chondrocyte proliferation, apoptosis, and inflammation by targeting HMGB1 in osteoarthritis. Inflamm. Res. 69, 63–73. doi: 10.1007/s00011-019-01294-0
Wang, L., Botchway, B. O. A., and Liu, X. (2021). The repression of the HMGB1-TLR4-NF-κB signaling pathway by safflower yellow may improve spinal cord injury. Front. Neurosci. 15:803885. doi: 10.3389/fnins.2021.803885
Wang, Q., Ding, Q., Zhou, Y., Gou, X., Hou, L., Chen, S., et al. (2009). Ethyl pyruvate attenuates spinal cord ischemic injury with a wide therapeutic window through inhibiting high-mobility group box 1 release in rabbits. Anesthesiology 110, 1279–1286. doi: 10.1097/ALN.0b013e3181a160d6
Wu, X., Mi, Y., Yang, H., Hu, A., Zhang, Q., and Shang, C. (2013). The activation of HMGB1 as a progression factor on inflammation response in normal human bronchial epithelial cells through RAGE/JNK/NF-κB pathway. Mol. Cell Biochem. 380, 249–257. doi: 10.1007/s11010-013-1680-0
Xia, Y. Y., Xue, M., Wang, Y., Huang, Z. H., and Huang, C. (2019). Electroacupuncture alleviates spared nerve injury-induced neuropathic pain and modulates HMGB1/NF-κB signaling pathway in the spinal cord. J. Pain Res. 12, 2851–2863. doi: 10.2147/JPR.S220201
Xie, W., Zhu, T., Dong, X., Nan, F., Meng, X., Zhou, P., et al. (2019). HMGB1-triggered inflammation inhibition of notoginseng leaf triterpenes against cerebral ischemia and reperfusion injury via MAPK and NF-κB signaling pathways. Biomolecules 9:512. doi: 10.3390/biom9100512
Xu, L., Tang, Y. Y., Ben, X. L., Cheng, M. H., Guo, W. X., Liu, Y., et al. (2020). Ginsenoside Rg1-induced activation of astrocytes promotes functional recovery via the PI3K/Akt signaling pathway following spinal cord injury. Life Sci. 252:117642. doi: 10.1016/j.lfs.2020.117642
Xue, J., Suarez, J. S., Minaai, M., Li, S., Gaudino, G., Pass, H. I., et al. (2021). HMGB1 as a therapeutic target in disease. J. Cell Physiol. 236, 3406–3419. doi: 10.1002/jcp.30125
Yang, Y., Han, C., Guo, L., and Guan, Q. (2018). High expression of the HMGB1-TLR4 axis and its downstream signaling factors in patients with Parkinson’s disease and the relationship of pathological staging. Brain Behav. 8:e00948. doi: 10.1002/brb3.948
Yoshizaki, S., Tamaru, T., Hara, M., Kijima, K., Tanaka, M., Konno, D. J., et al. (2021). Microglial inflammation after chronic spinal cord injury is enhanced by reactive astrocytes via the fibronectin/β1 integrin pathway. J. Neuroinflammation 18:12. doi: 10.1186/s12974-020-02059-x
Yuan, J., Liu, W., Zhu, H., Chen, Y., Zhang, X., Li, L., et al. (2017). Curcumin inhibits glial scar formation by suppressing astrocyte-induced inflammation and fibrosis in vitro and in vivo. Brain Res. 1655, 90–103. doi: 10.1016/j.brainres.2016.11.002
Yuan, X., Qin, X., Wang, D., Zhang, Z., Tang, X., Gao, X., et al. (2019). Mesenchymal stem cell therapy induces FLT3L and CD1c+ dendritic cells in systemic lupus erythematosus patients. Nat. Commun. 10:2498. doi: 10.1038/s41467-019-10491-8
Zhang, H., Yan, K., Sui, L., Li, P., Du, Y., Hu, J., et al. (2021). Low-level pyruvate inhibits early embryonic development and maternal mRNA clearance in mice. Theriogenology 166, 104–111. doi: 10.1016/j.theriogenology.2021.02.022
Zhang, J. J., Shen, H. Q., Deng, J. T., Jiang, L. L., Zhang, Q. Y., Xiong, Y., et al. (2020). Effect of peritoneal dialysis solution with different pyruvate concentrations on intestinal injury. Exp. Biol. Med. 245, 644–653. doi: 10.1177/1535370220909332
Zhang, L., Yang, X., Jiang, G., Yu, Y., Wu, J., Su, Y., et al. (2019). HMGB1 enhances mechanical stress-induced cardiomyocyte hypertrophy in vitro via the RAGE/ERK1/2 signaling pathway. Int. J. Mol. Med. 44, 885–892. doi: 10.3892/ijmm.2019.4276
Zhao, E., Bai, L., Li, S., Li, L., Dou, Z., Huang, Y., et al. (2020). Dexmedetomidine alleviates CCI-induced neuropathic pain via inhibiting HMGB1-mediated astrocyte activation and the TLR4/NF-κB signaling pathway in rats. Neurotox. Res. 38, 723–732. doi: 10.1007/s12640-020-00245-6
Zhao, J., Cheng, J., Li, C., Xu, M., Ma, C., Qin, L., et al. (2018). Ethyl pyruvate attenuates CaCl2-induced tubular epithelial cell injury by inhibiting autophagy and inflammatory responses. Kidney Blood Press Res. 43, 1585–1595. doi: 10.1159/000494445
Zheng, B., Yang, Y., Li, J., Li, J., Zuo, S., Chu, X., et al. (2021). Magnesium isoglycyrrhizinate alleviates arsenic trioxide-induced cardiotoxicity: Contribution of Nrf2 and TLR4/NF-κB signaling pathway. Drug Des. Devel. Ther. 15, 543–556. doi: 10.2147/DDDT.S296405
Zhong, X., Xie, L., Yang, X., Liang, F., Yang, Y., Tong, J., et al. (2020). Ethyl pyruvate protects against sepsis-associated encephalopathy through inhibiting the NLRP3 inflammasome. Mol. Med. 26:55. doi: 10.1186/s10020-020-00181-3
Zhou, Q., Tu, T., Tai, S., Tang, L., Yang, H., and Zhu, Z. (2021). Endothelial specific deletion of HMGB1 increases blood pressure and retards ischemia recovery through eNOS and ROS pathway in mice. Redox Biol. 41:101890. doi: 10.1016/j.redox.2021.101890
Zhu, G., Cheng, Z., Huang, Y., Zheng, W., Yang, S., Lin, C., et al. (2020). MyD88 mediates colorectal cancer cell proliferation, migration and invasion via NF−κB/AP-1 signaling pathway. Int. J. Mol. Med. 45, 131–140. doi: 10.3892/ijmm.2019.4390
Zou, M., Dong, H., Meng, X., Cai, C., Li, C., Cai, S., et al. (2015). Store-operated Ca2+ entry plays a role in HMGB1-induced vascular endothelial cell hyperpermeability. PLoS One 10:e0123432. doi: 10.1371/journal.pone.0123432
Keywords: spinal cord injury, ethyl pyruvate, high mobility group box-1 (HMGB1), toll-like receptors (TLRs), nuclear factor-kappa B (NF-κB), astrocytic activation
Citation: Fan R, Wang L, Botchway BOA, Zhang Y and Liu X (2022) Protective role of ethyl pyruvate in spinal cord injury by inhibiting the high mobility group box-1/toll-like receptor4/nuclear factor-kappa B signaling pathway. Front. Mol. Neurosci. 15:1013033. doi: 10.3389/fnmol.2022.1013033
Received: 06 August 2022; Accepted: 29 August 2022;
Published: 16 September 2022.
Edited by:
Nara L. M. Quintão, Universidade do Vale do Itajaí, BrazilReviewed by:
Tingting Sun, Zaozhuang University, ChinaCopyright © 2022 Fan, Wang, Botchway, Zhang and Liu. This is an open-access article distributed under the terms of the Creative Commons Attribution License (CC BY). The use, distribution or reproduction in other forums is permitted, provided the original author(s) and the copyright owner(s) are credited and that the original publication in this journal is cited, in accordance with accepted academic practice. No use, distribution or reproduction is permitted which does not comply with these terms.
*Correspondence: Xuehong Liu, bGl1eHVlaDY1ODhAMTI2LmNvbQ==; orcid.org/0000-0003-4325-6762
†These authors have contributed equally to this work