- 1Department of Biological Sciences, Tata Institute of Fundamental Research, Mumbai, India
- 2Medical Research Centre, Kasturba Health Society, Mumbai, India
- 3Department of Psychiatry and Behavioral Health, The Ohio State University College of Medicine, Columbus, OH, United States
- 4Department of Systems Pharmacology and Translational Therapeutics, Perelman School of Medicine, University of Pennsylvania, Philadelphia, PA, United States
Psychedelic compounds that target the 5-HT2A receptor are reported to evoke psychoplastogenic effects, including enhanced dendritic arborization and synaptogenesis. Transcriptional regulation of neuronal plasticity-associated genes is implicated in the cytoarchitectural effects of serotonergic psychedelics, however, the transcription factors that drive this regulation are poorly elucidated. Here, we addressed the contribution of the transcription factor cyclic adenosine monophosphate (cAMP)-response element binding protein (CREB) in the regulation of neuronal plasticity-associated genes by the hallucinogenic 5-HT2A receptor agonist, 2,5-dimethoxy-4-iodoamphetamine (DOI). In vitro studies with rat cortical neurons indicated that DOI enhances the phosphorylation of CREB (pCREB) through mitogen-activated protein (MAP) kinase and calcium/calmodulin dependent kinase II (CaMKII) pathways, with both cascades contributing to the DOI-evoked upregulation of Arc, Bdnf1, Cebpb, and Egr2 expression, whilst the upregulation of Egr1 and cFos mRNA involved the MAP kinase and CaMKII pathway respectively. We observed a robust DOI-evoked increase in the expression of several neuronal plasticity-associated genes in the rat neocortex in vivo. This DOI-evoked upregulation of neuronal plasticity-associated genes was completely blocked by the 5-HT2A receptor antagonist MDL100,907 in vitro and was also abrogated in the neocortex of 5-HT2A receptor deficient mice. Further, 5-HT2A receptor stimulation enhanced pCREB enrichment at putative cAMP response element (CRE) binding sites in the Arc, Bdnf1, Cebpb, cFos, but not Egr1 and Egr2, promoters in the rodent neocortex. The DOI-mediated transcriptional induction of Arc, cFos and Cebpb was significantly attenuated in the neocortex of CREB deficient/knockout (CREBαδ KO) mice. Collectively, these results indicate that the hallucinogenic 5-HT2A receptor agonist DOI leads to a rapid transcriptional upregulation of several neuronal plasticity-associated genes, with a subset of them exhibiting a CREB-dependent regulation. Our findings raise the intriguing possibility that similar to slow-acting classical antidepressants, rapid-action serotonergic psychedelics that target the 5-HT2A receptor may also recruit the transcription factor CREB to enhance the expression of neuronal plasticity-associated genes in the neocortex, which could in turn contribute to the rapid psychoplastogenic changes evoked by these compounds.
Introduction
There has been a renewal of interest in serotonergic psychedelics as potential rapid-acting antidepressants for the treatment of anxiety and depression (Nutt et al., 2020; Vollenweider and Preller, 2020; Banks et al., 2021; De Gregorio et al., 2021). Most serotonergic psychedelics target the serotonin2A (5-HT2A) receptor, and agonist action at the 5-HT2A receptor is implicated in the molecular, cytoarchitectural, hallucinogenic and mood-related behavioral effects of serotonergic psychedelics (Vollenweider et al., 1998; González-Maeso et al., 2003, 2007; Martin and Nichols, 2016; López-Giménez and González-Maeso, 2018; Preller et al., 2018; Madsen et al., 2019; Slocum et al., 2021). Diverse serotonergic psychedelics, as well as the hallucinogenic 5-HT2A receptor agonist, 2,5-dimethoxy-4-iodoamphetamine (DOI), can elicit both unique and distinctive molecular, behavioral and electrophysiological effects (Aghajanian and Marek, 1999; González-Maeso et al., 2003, 2007; Marek, 2017; Banerjee and Vaidya, 2020; Savino and Nichols, 2021). Common to all of these 5-HT2A receptor agonists is a regulation of neuronal structural plasticity in the neocortex; from increased dendritic complexity to enhanced synaptogenesis (Ly et al., 2018, 2020; Savalia et al., 2021; Shao et al., 2021; Vargas et al., 2021). The rapid transcriptional effects evoked via agonistic action at the 5-HT2A receptor, in particular the enhanced expression of neuronal plasticity-associated gene expression, are implicated in contributing to these psychoplastogenic effects (Nichols et al., 2003; Olson, 2018; Berthoux et al., 2019; Artin et al., 2021; de Vos et al., 2021; Jefsen et al., 2021).
5-HT2A receptors are G-protein coupled receptors that drive Gq-signaling to activate phospholipase C beta (PLCβ) -mediated cleavage of phosphatidylinositol bisphosphate (PIP2) into inositol triphosphate (IP3) and diacylglycerol (DAG) (Raymond et al., 2001; Sharp and Barnes, 2020). IP3 mobilizes release of calcium from intracellular endoplasmic reticulum stores, which activates calcium/calmodulin dependent kinase II (CaMKII), while DAG activates protein kinase C (PKC) that phosphorylates and activates mitogen-activated protein (MAP) kinase (MAPK) signaling (Banerjee and Vaidya, 2020). These kinases in turn are reported to enhance the phosphorylation of the transcription factor, cyclic adenosine monophosphate (cAMP)-response-element binding protein (CREB), that has been previously shown to regulate the expression of several neuronal plasticity-associated genes (Lonze and Ginty, 2002; Sakamoto et al., 2011; Belgacem and Borodinsky, 2017). CREB is also a central target for diverse classes of antidepressant treatments, contributes to the neurotrophic, neurogenic and behavioral effects of antidepressants (Nibuya et al., 1996; Duman and Nibuya, 1997; Thome et al., 2000; Chen et al., 2001; Nair and Vaidya, 2006), and is dysregulated in both animal models of depression (Carlezon et al., 2005; Duman et al., 2005; Krishnan and Nestler, 2008, 2010) and in major depressive disorder patients (Blendy et al., 1996; Koch et al., 2009). Here, we sought to address whether CREB plays an important role in the regulation of neuronal plasticity-associated gene expression within the neocortex that arise in response to treatment with the hallucinogenic 5-HT2A receptor agonist, DOI. Using both in vitro and in vivo approaches, as well as a CREB-deficient mouse line, we demonstrate that CREB contributes to the DOI-mediated regulation of a subset of neuronal plasticity-associated genes within the neocortex. This raises the intriguing possibility that similar to classical antidepressants, serotonergic psychedelics that target the 5-HT2A receptor may also recruit CREB-mediated transcriptional regulation of specific neuronal plasticity-associated genes in the neocortex, thus contributing to the effects on neuronal structural plasticity and mood-related behavior.
Materials and Methods
Animal Treatments
Male Sprague-Dawley rats (2–3 months), serotonin2A receptor knockout (5-HT2A–/–) mice (Weisstaub et al., 2006) and wild-type (WT) littermate controls (4–5 months) maintained on a 129S6/SvEv background were bred in the Tata Institute of Fundamental Research (TIFR) animal facility and CREB deficient/knockout (CREBαδ KO) mice were bred in the University of Pennsylvania animal facility and used for all experiments. For experiments using CREB deficient mice, the hypomorphic CREBαδ knockout mouse line that lacks the α and δ isoforms of CREB (CREBαδ KO) were used (Blendy et al., 1996). CREBαδ KO mice were generated as previously described (Walters and Blendy, 2001) and were maintained as F1 hybrids of 129SvEvTac:C57BL/6. For all experiments, CREBαδ KO mice and the WT controls were generated via crossing heterozygote CREBαδ 129SvEvTac with heterozygote CREBαδ C57BL/6 mice, allowing for a uniform genetic background in the experimental cohort. For the establishment of in vitro cortical cultures, rat embryos were derived at embryonic day 17.5 (E17.5) from timed pregnant Sprague-Dawley dams. All animal procedures using Sprague-Dawley rats and serotonin2A receptor knockout (5-HT2A–/–) mice were carried out in accordance with the Committee for Care and Supervision of Experimental Animals (CPCSEA) and approved by the TIFR Institutional Animal Ethics Committee. All experiments with the CREBαδ KO mouse line were carried out in accordance with the NIH guideline for the care and use of laboratory animals and were approved by the University of Pennsylvania Animal Care and Use Committee. Animals were group housed and maintained on a 12 h light–dark cycle (lights on at 7 am) with access to food and water ad libitum.
Sprague-Dawley rats, CREBαδ KO and litter-matched WT mice, received intraperitoneal injections of the 5-HT2A agonist, 2,5-dimethoxy-4-iodoamphetamine (DOI, 8 mg/kg, Sigma-Aldrich, United States) or vehicle (0.9% NaCl) (Vaidya et al., 1997). 5-HT2A–/– and litter-matched WT mice received intraperitoneal administration of DOI (2 mg/kg) or vehicle (0.9% saline) and animals were sacrificed 2 h post drug administration (Vaidya et al., 1997; Benekareddy et al., 2012).
To make comparisons with a rapid-action antidepressant treatment, we also subjected a cohort of Sprague Dawley rats to electroconvulsive seizure (ECS) treatment via ear-clip electrodes (ECS unit, UGO Basile, Comerio, Italy) (frequency: 100 pulses/s; pulse width: 0.9 ms; pulse duration: 0.5 s; current: 80 mA). ECS animals were subjected to a single ECS treatment while sham-treated control animals underwent the application and removal of ear-clip electrodes without electrical stimulation, and were sacrificed 2 h post treatment. Animals were decapitated immediately, neocortex was dissected and snap frozen in liquid nitrogen.
Cortical Neuron Cultures
Primary cortical neuron cultures were established from E17.5 rat embryos as described previously (Desouza et al., 2011; Fanibunda et al., 2019). Rat embryonic cortices were dissected and treated with trypsin-EDTA for 10 min, prior to dissociation in culture medium - Neurobasal medium supplemented with 2% B27 supplement, 0.5 mM L-glutamine, 5 U/ml penicillin and 5 U/ml streptomycin (Invitrogen, United States). Cells were plated on poly-D-lysine (Sigma-Aldrich, United States) coated 35 mm dishes at a density of 106 cells/dish. Following attachment and neurite extension in vitro for a period of 7 days, neurons were treated with DOI (10 μM) or vehicle (DMSO) for 2 h on day in vitro (DIV) 10. In experiments to delineate signaling events downstream of 5-HT2A receptor activation, neurons were treated with DOI (10 μM) for 2 h in the presence of the 5-HT2A receptor antagonist, MDL100,907 (10 μM) and specific signaling pathway inhibitors, namely the phospholipase C (PLC) inhibitor U73122 (5 μM), the mitogen activated protein kinase kinase (MAPKK) inhibitor U0126 (50 μM) and the CaM kinase II (CaMKII) inhibitor KN-62 (10 μM) (Tocris Bioscience, United Kingdom). The inhibitors were added to the cultures 30 min prior to DOI treatment, and were present throughout the duration of DOI exposure. Following treatments, cortical neurons were processed for immunofluorescence, RNA extraction for qPCR analysis, or western blot analysis.
Immunofluorescence
Immunofluorescence staining was performed as described previously (Fanibunda et al., 2019). In brief, cortical neurons were fixed in 4% paraformaldehyde, followed by blocking in 10% horse serum and incubated with primary antibodies, rabbit anti-pCREB (1:1000; Cell Signaling Technology, MA, United States) or goat anti-5-HT2A receptor (1:500, Santa Cruz Biotechnologies, United States) along with the pan-neuronal marker, mouse anti-MAP2 (1:1000, Sigma-Aldrich, United States) overnight at 4°C. This was followed by incubation with secondary antibodies, Alexa 488 conjugated anti-goat (1:500; Molecular probes, CA, United States) or Alexa 488 conjugated anti-rabbit (1:500; Molecular Probes, CA, United States) or biotinylated horse anti-mouse (1:500, Roche Applied Science, Switzerland) with subsequent incubation with streptavidin-conjugated Alexa 568 (1:500, Molecular Probes, CA, United States) for 2 h. Following secondary antibody incubation and serial washes, cortical neurons were mounted in Vectashield (Vector Laboratories, CA, United States), and images were captured on the Zeiss LSM5 Exciter laser scanning microscope.
Western Blot Analysis
Rat cortical neurons were lysed in Laemmli sample buffer (2% SDS, 10% glycerol, 60 mM Tris-Cl, 0.01% bromophenol blue) and proteins were resolved via sodium dodecyl sulfate polyacrylamide gel electrophoresis (SDS-PAGE), followed by transfer onto polyvinylidene fluoride (PVDF, GE Healthcare, United Kingdom) membranes. Blots were blocked in 5% fat-free milk in 0.05% Tris Buffered Saline-Tween 20 (TBS-T) and incubated in primary antibodies in 0.05% TBS-T, overnight at 4°C. Primary antibodies included rabbit anti-pCREB (1:500; Cell Signaling Technology, MA, United States) and rabbit anti-CREB (1:1000, Cell Signaling Technology). Blots were washed 3–5 times and incubated with a 1:5000 dilution of horseradish peroxidase-conjugated goat anti-rabbit antibody (GE Healthcare, United Kingdom) for 1 h. Protein-antibody complexes were detected on X-ray films following addition of Enhanced Chemiluminescence (ECL) substrate (GE Healthcare, United Kingdom). The relative density of the pCREB and CREB bands was quantitated using ImageJ software (NIH, United States), and was represented as a pCREB/CREB ratio.
Quantitative PCR Analysis
RNA was isolated using Tri Reagent (Sigma-Aldrich, United States), according to the manufacturer’s protocols. Two μg of RNA per sample was reverse transcribed using a complementary DNA (cDNA) synthesis kit (QuantiTect reverse transcription kit, Qiagen, Germany). Quantitative PCR (qPCR) was performed in a Mastercycler® ep realplex real-time PCR system (Eppendorf, Germany). cDNA was amplified using a SYBR Green kit (Applied Biosystems, CA, United States), with primers for the genes of interest (Supplementary Table 1). Hypoxanthine phosphoribosyl transferase (Hprt) was used as an endogenous housekeeping gene control for normalization. The relative expression levels between control and treated samples were computed by the comparative Ct method, as described previously (Schmittgen and Livak, 2008). Data are represented as fold change ± SEM as compared to control.
Chromatin Immunoprecipitation
Chromatin immunoprecipitation (ChIP) was carried out as described previously (Desouza et al., 2011). Briefly, bilateral frontal neocortices were dissected and fixed to crosslink the DNA with the bound proteins. The tissue was placed in a pre-chilled Dounce homogenizer, sonicated and immunoprecipitated using a phosphorylated CREB (pCREB) antibody (1 μg; Cell Signaling Technology, MA, United States). Following reverse crosslinking and chromatin precipitation, qPCR analysis was performed for upstream sequences of the Arc, BdnfI, Cebpb, cFos, Egr1, and Egr2 promoters that contained putative CRE sites as predicted using AliBaba 2.11. For each sample the results were normalized to the input chromatin from the same sample. (Primer sequences used in ChIP experiments: Supplementary Table 2).
Dimethoxy-4-Iodoamphetamine-Mediated Head Twitch Behavior
Administration of DOI results in a stereotypical head twitch behavior, characterized by rapid radial movements of the head (Canal and Morgan, 2012; Halberstadt and Geyer, 2013). This behavior was videotaped in the home cage for a total duration of 20 min, commencing 20 min following administration of the DOI or vehicle (saline) treatment. The total number of head twitches in this time window were counted by an experimenter blind to the experimental treatment groups.
In situ Hybridization
CREBαδ KO and litter-matched WT mice were administered DOI and vehicle (Veh) resulting in four groups: WT + Veh, WT + DOI, CREBαδ KO + Veh, CREBαδ KO + DOI. Mice were anesthetized with sodium thiopentone and transcardially perfused with 4% paraformaldehyde (PFA). The brains were postfixed in 4% PFA overnight and cryoprotected in 30% sucrose in 4% PFA prior to being shipped to TIFR, India. Coronal sections of 30 μM thickness were cut on the freezing microtome (Leica Biosystems, Germany), fixed, blocked and acetylated. The floating sections were incubated for 20 h at 60°C in a hybridization buffer (50% formamide, 1xSSC, 25xDenhardt’s solution, 40 mM dithiothreitol, 150 μg/ml yeast tRNA, 10% dextran sulfate, 400 μg/ml salmon sperm DNA) containing 35S-UTP labeled antisense riboprobes for Arc mRNA at a concentration of 1 × 106 cpm/300 μl. Antisense riboprobes to Arc mRNA were generated from a transcription-competent plasmid kindly provided by Dr. Oswald Steward (University of California, Irvine, CA, United States). Following hybridization, all sections were washed in ribonuclease A (20 mg/ml; USB corporation, United States), followed by stringent washes in decreasing concentrations of SSC, mounted on slides, air dried and exposed to Hyper film β-max (GE Healthcare, United States) for 7 days. Levels of Arc mRNA were quantified using Scion Image (Scion, United States) and calibrated using 14C standards to correct for non-linearity. Equivalent areas of the somatosensory and prefrontal cortex were outlined and optical density measurements were determined (3–4 sections/animal).
Statistical Analysis
Results were subjected to statistical analysis using Student’s unpaired t-test for experiments with two groups (GraphPad InStat) and one-way ANOVA (GraphPad, Prism 8) for experiments using signaling pathway inhibitors, followed by Tukey’s post hoc test for group comparisons. For four group experiments statistical analysis was performed using two-way ANOVA (GraphPad, Prism 8). Tukey’s post hoc test for group comparisons was applied only when there was a significant two-way ANOVA interaction observed between the two variables of DOI treatment and 5-HT2A–/– KO genotype or DOI treatment and CREBαδ KO genotype. Statistical significance was determined at p < 0.05.
Results
Acute Treatment With the 5-HT2A Receptor Agonist, Dimethoxy-4-Iodoamphetamine, Regulates Neuronal Plasticity-Associated Gene Expression via the Mitogen-Activated Protein Kinase and CaMKII Signaling Pathways and Enhances Phosphorylated cAMP Response Element Binding Protein Expression in vitro
The 5-HT2A receptor agonist DOI, is a potent hallucinogen that is known to evoke an increase in the expression of several neuronal plasticity-associated genes (González-Maeso et al., 2003). Following ligand binding, the Gq-coupled 5-HT2A receptor can differentially recruit multiple signaling pathways to bring about distinct signaling responses. We sought to address the contribution of the 5-HT2A receptor, the Gq-coupled phospholipase C (PLC) signaling pathway, the MAP kinase and CaM Kinase II (CaMKII) signaling pathways and the transcription factor CREB to the DOI-evoked induction of neuronal plasticity-associated gene expression. We stimulated primary rat cortical neurons in vitro with DOI, in the presence or absence of the 5-HT2A receptor antagonist, MDL100,907; PLC inhibitor, U73122; CaMKII inhibitor, KN-62 or the MAPKK inhibitor, U0126 (Figure 1A). We observed an increase in transcript expression of Arc, Bdnf1, Cebpb, cFos, Egr1, and Egr2 mRNA levels following DOI treatment, which was inhibited by the 5-HT2A receptor antagonist MDL100,907, as well as the PLC signaling pathway inhibitor, U73122 (Figure 1B) {one-way ANOVA: Arc: [F(3,12) = 11.23, p = 0.0008]; Bdnf1: [F(3,12) = 90.59, p < 0.0001]; Cebpb: [F(3,12) = 14.78, p = 0.0002], cFos: [F(3,12) = 135.6, p < 0.0001], Egr1: [F(3,12) = 26.17, p < 0.0001], Egr2: [F(3,12) = 28.45, p < 0.0001]}. These observations indicate that the 5-HT2A receptor mediates the DOI-evoked induction in neuronal plasticity-associated genes, via recruiting the Gq-coupled PLC pathway. We next sought to delineate the contribution of the downstream MAP kinase and CaM Kinase II (CaMKII) signaling pathways to the DOI-evoked upregulation of neuronal plasticity-associated genes. We observed an upregulation of Arc, Bdnf1, Cebpb, and Egr2 mRNA levels following DOI treatment, which was inhibited by both the CaMKII inhibitor KN-62 and the MAPKK inhibitor U0126 (Figure 1C) {one-way ANOVA: Arc: [F(3,9) = 7.551, p = 0.008]; Bdnf1: [F(3,11) = 6.436, p = 0.009]; Cebpb: [F(3,8) = 5.293, p = 0.027], Egr2: [F(3,10) = 7.685, p = 0.006]}. In contrast, the DOI-mediated upregulation of cFos mRNA levels was prevented by only the CaMKII inhibitor KN-62, while the increase in the Egr1 transcript levels was abrogated exclusively by the MAPKK inhibitor U0126 (Figure 1C) {one-way ANOVA: cFos: [F(3,11) = 5.563, p = 0.014], Egr1: [F(3,11) = 14.02, p = 0.0004]}. This indicates a differential involvement of the MAP kinase and the CaMKII pathway in the 5-HT2A receptor-mediated transcriptional regulation of specific neuronal plasticity-associated genes (Figure 1D).
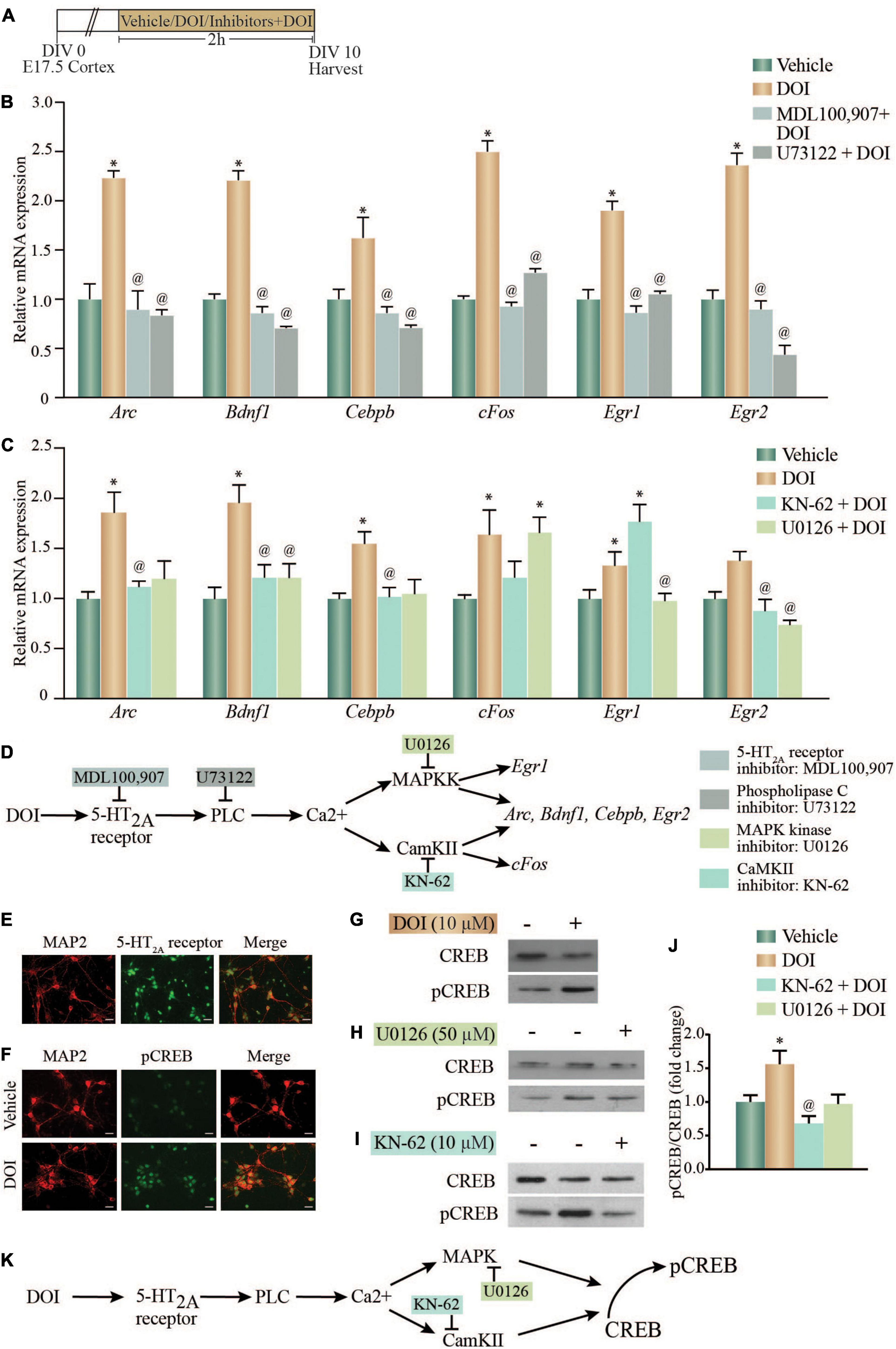
Figure 1. Acute treatment with the 5-HT2A receptor agonist, DOI regulates neuronal plasticity-associated gene expression via the MAP kinase and CaMKII signaling pathways and enhances phosphorylated CREB (pCREB) expression in vitro. (A) Shown is a schematic of the treatment paradigm for cortical neurons derived from E17.5 rat embryos, allowed to differentiate till day in vitro (DIV) 10, following which neurons were treated with vehicle (DMSO) or the 5-HT2A receptor agonist, DOI (10 μM), in the presence or absence of CaMKII and MAP kinase signaling pathway inhibitors (CaMKII inhibitor: KN-62; MAPKK inhibitor: U0126). (B) Shown is the relative mRNA expression for plasticity-associated genes following DOI treatment in the presence or absence of the 5-HT2A receptor antagonist, MDL100,907 or PLC inhibitor, U73122, represented as fold change of vehicle ± SEM. (Representative results from n = 4 wells per treatment group/N = 2, *p < 0.05 as compared to vehicle, @p < 0.05 as compared to DOI, one-way ANOVA, Tukey’s post hoc test). (C) Shown is the relative mRNA expression for plasticity-associated genes following DOI treatment in the presence or absence of MAP kinase and CaMKII signaling pathway inhibitors, represented as fold change of vehicle ± SEM. (Representative results from n = 3–5 wells per treatment group/N = 3, *p < 0.05 as compared to vehicle, @p < 0.05 as compared to DOI, one-way ANOVA, Tukey’s post hoc test). (D) Shown is a schematic summarizing the putative signaling pathways that may contribute to DOI-induced gene expression. The CaMKII inhibitor, KN-62 and the MAPKK inhibitor, U0126 inhibit the CaMKII and MAP kinase signaling pathways respectively. The DOI-mediated upregulation of Arc, Bdnf1, Cebpb, and Egr2 mRNA levels was blocked by both the MAPKK and CaMKII inhibitors, whereas the increase in cFos mRNA was blocked by the CaMKII, not the MAPKK, inhibitor and the upregulation of Egr1 mRNA was blocked by the MAPKK, not the CaMKII, inhibitor. (E) Shown are representative immunofluorescence images of rat cortical neurons in vitro with double staining for the neuronal marker MAP2 (red) and 5-HT2A receptor (green). Scale bar: 30 μm. Magnification: 20X. (F) Shown are representative immunofluorescence images of rat cortical neurons with double staining for pCREB (green) and the neuronal marker MAP2 (red) – upper panel: Vehicle; lower panel: DOI. Scale bar: 30 μm. Magnification: 20X. (G–J) Shown are representative immunoblots for pCREB and CREB protein levels in rat cortical neurons treated with DOI (G) or with DOI in the presence or absence of the MAPKK inhibitor U0126 (H) or the CaMKII inhibitor KN-62 (I). (J) Quantitative densitometric analysis of pCREB/CREB levels in rat cortical neurons treated with DOI in the presence or absence of U0126 or KN-62. Results are expressed as fold change of vehicle ± SEM. (Representative results from n = 3–5 wells per treatment group/N = 3, *p < 0.05 as compared to vehicle, @p < 0.05 as compared to DOI, one-way ANOVA, Tukey’s post hoc test). (K) Shown is a schematic depicting the putative pathway via which pCREB levels are enhanced following DOI administration, indicative of a role for the MAP kinase and CaMKII signaling pathways.
We next sought to address the possible role of the transcription factor CREB in mediating the signaling events evoked by DOI, downstream of the 5-HT2A receptor. The DOI regulated genes, Arc, Bdnf1, Cebpb, cFos, Egr1, and Egr2 were found to contain putative cAMP response element (CRE) (TGACG/CGTCA/TGACGTCA) sites in the upstream promoter regions, suggestive of the possibility of a CREB-dependent transcriptional regulation. We first confirmed 5-HT2A receptor expression in cortical neurons, by immunofluorescence staining (Figure 1E). Immunostaining with the phosphorylated CREB (pCREB) antibody demonstrated an increase in pCREB immunofluorescence intensity in DOI-treated rat cortical neurons as compared to vehicle-treated control neurons (Figure 1F). Immunoblotting to detect pCREB levels, also demonstrated a robust increase in pCREB/CREB levels in DOI-treated cortical neurons (Figure 1G), further corroborating that DOI treatment enhances pCREB levels. Further, the MAPKK inhibitor (Figure 1H) and the CaMKII inhibitor (Figure 1I), both prevented the DOI-evoked increase in pCREB/CREB levels, demonstrating a role for the MAP kinase pathway and CaMKII pathway in mediating the DOI-evoked increase in pCREB levels (Figure 1J) [pCREB/CREB: F(3,17) = 5.561, p = 0.008]. This data collectively suggests that DOI, a hallucinogenic ligand of the Gq-coupled 5-HT2A receptor, recruits the PLC pathway driving MAP kinase and CaMKII signaling to enhance the phosphorylation of the transcription factor CREB in rat cortical neurons (Figure 1K).
Dimethoxy-4-Iodoamphetamine-Mediated Regulation of Plasticity-Associated Gene Expression in the Neocortex Is Altered in 5-HT2A–/– Receptor Deficient Mice
Given that the hallucinogen DOI, in addition to exhibiting agonist action at the 5-HT2A receptor, also binds to the 5-HT2C receptor with lower affinity (Titeler et al., 1988), we further sought to evaluate the contribution of the 5-HT2A receptor to the DOI-mediated induction of neuronal plasticity-associated genes in 5-HT2A–/– receptor deficient mice (Figure 2A). We confirmed that 5-HT2A–/– mice exhibit both a robust reduction in cortical 5-HT2A, but not in 5-HT2C, mRNA expression (Figure 2B), accompanied by a significant decline in a stereotypical head twitch response (HTR) behavior evoked by the 5-HT2A receptor agonist DOI (Canal and Morgan, 2012; Halberstadt and Geyer, 2013). Two-way ANOVA analysis for HTR events (Figure 2C) indicated a significant DOI by 5-HT2A–/– genotype interaction [F(1,29) = 113.4, p < 0.0001], as well as significant main effects of DOI [F(1,29) = 189.1, p < 0.0001] and 5-HT2A–/– genotype [F(1,29) = 107.6, p < 0.0001].
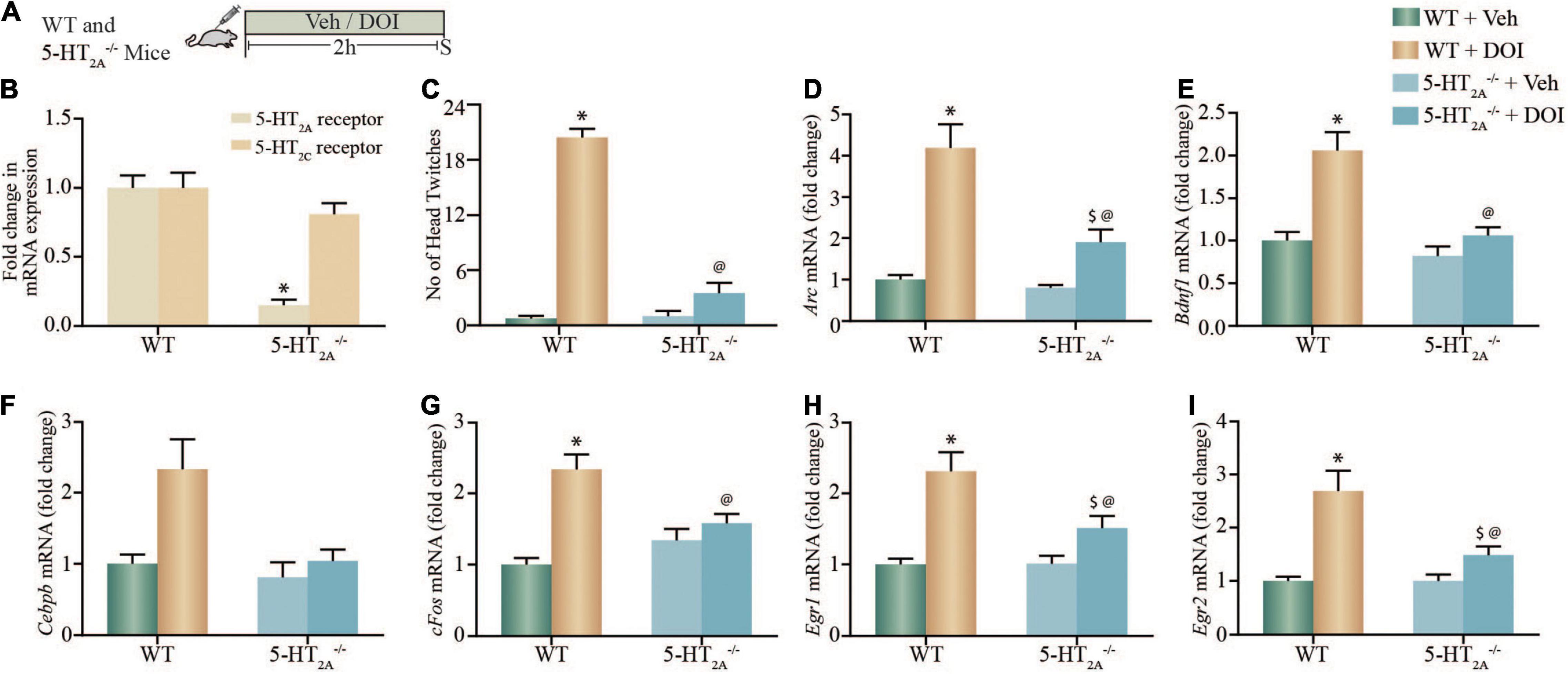
Figure 2. DOI-mediated regulation of plasticity-associated gene expression in cortical brain regions is altered in serotonin2A receptor knockout (5-HT2A–/–) mice. (A) Shown is a schematic of the acute treatment paradigm for wild type (WT) and 5-HT2A receptor deficient (5-HT2A–/–) mice, with vehicle (saline) or DOI (2 mg/kg) followed by sacrifice 2 h after treatment. (B) The bar graph depicts quantitative qPCR analysis for 5-HT2A and 5-HT2C receptor mRNA in the neocortex of WT and 5-HT2A–/– mice represented as fold change of WT ± SEM. (n = 12 animals per treatment group, *p < 0.05 as compared to WT, unpaired Students t-test). (C) The bar graph depicts the quantitation of head-twitch responses evoked in response to acute treatment with the 5-HT2A receptor agonist, DOI or vehicle in both WT and 5-HT2A–/– mice (n = 7–9 animals per treatment group, *p < 0.05 as compared to WT mice, @p < 0.05 as compared to WT + DOI mice, two-way ANOVA, Tukey’s post hoc test). (D–I) Bar graphs depict quantitation of qPCR analysis for mRNA expression of Arc (D), Bdnf1 (E), Cebpb (F), cFos (G), Egr1 (H), and Egr2 (I), following acute DOI or vehicle treatment to WT and 5-HT2A–/– mice. (n = 12 animals per group, *p < 0.05 as compared to WT mice, $p < 0.05 as compared to 5-HT2A–/– mice, @p < 0.05 as compared to WT + DOI mice, two-way ANOVA, Tukey’s post hoc test).
We next performed qPCR analysis to assess whether the DOI-evoked upregulation of specific neuronal plasticity-associated genes Arc, Bdnf1, Cebpb, cFos, Egr1, and Egr2 mRNA expression observed in vitro, was altered in the neocortex of WT and 5-HT2A–/– mice following acute DOI administration (Figures 2D–I). We noted significant two-way ANOVA interactions of DOI by 5-HT2A–/– genotype for the neocortical mRNA expression of Arc [F(1,44) = 4.920, p = 0.0318], Bdnf1 [F(1,44) = 4.45, p = 0.04], cFos [F(1,44) = 12.59, p = 0.0009], Egr1 [F(1,44) = 4.257, p = 0.045] and Egr2 [F(1,44) = 1118, p < 0.0001], but not for Cebpb mRNA expression [F(1,44) = 2.49, p = 0.12]. We also noted significant main effects of DOI for Arc [F(1,44) = 81.38, p < 0.0001], Bdnf1 [F(1,44) = 20.37, p < 0.0001], Cebpb [F(1,44) = 8.518, p = 0.0055], cFos [F(1,44) = 26.72, p < 0.0001], Egr1 [F(1,44) = 34.58, p < 0.0001], and Egr2 [F(1,44) = 1392, p < 0.0001] mRNA expression in the neocortex. Significant main effects of 5-HT2A–/– genotype were observed for neocortical mRNA levels of Arc [F(1,44) = 15.79, p = 0.0003], Bdnf1 [F(1,44) = 15.77, p = 0.0003], Cebpb [F(1,44) = 7.399, p = 0.009], and Egr2 [F(1,44) = 1122, p < 0.0001], but not for cFos and Egr1 mRNA expression. Post hoc Tukey comparison analysis revealed that the DOI-evoked upregulation of Bdnf1 and cFos expression noted in the neocortex of WT mice was completely abrogated in 5-HT2A–/– receptor deficient mice, and the DOI-evoked increase in Arc, Egr1, and Egr2 neocortical mRNA levels noted in WT mice was significantly attenuated in the 5-HT2A–/– receptor deficient genotype. Collectively, studies with pharmacological blockade of the 5-HT2A receptor in vitro, and using 5-HT2A–/– mice in vivo, indicate the critical contribution of the 5-HT2A receptor to the DOI-mediated regulation of a subset of neuronal plasticity-associated genes in the neocortex.
Acute Treatment With Dimethoxy-4-Iodoamphetamine Enhances Both the Expression of Putative cAMP Response Element-Containing Plasticity-Associated Genes and the Enrichment of Phosphorylation of cAMP Response Element Binding Protein Within the Promoter Regions of Specific Plasticity-Associated Genes in the Neocortex of Adult Rats
Given that we observed that the 5-HT2A receptor agonist, DOI, induced a robust upregulation of neuronal plasticity-associated genes in rat cortical neurons in culture, as well as in WT but not 5-HT2A–/– mice, we next examined whether DOI administration to Sprague-Dawley male rats could evoke similar alterations in neuronal plasticity-associated genes in the neocortex. We systemically administered DOI (8 mg/kg) and evaluated gene expression in the neocortex at a 2 h time-point post treatment (Figure 3A). DOI administration evoked a robust increase in the gene expression of Arc, Atf3, Atf4, Bdnf1, Cebpb, Cebpd, Egr1, Egr2, Egr3, Egr4, cFos, JunB, and Nfkbia in the rat neocortex (Figure 3B).
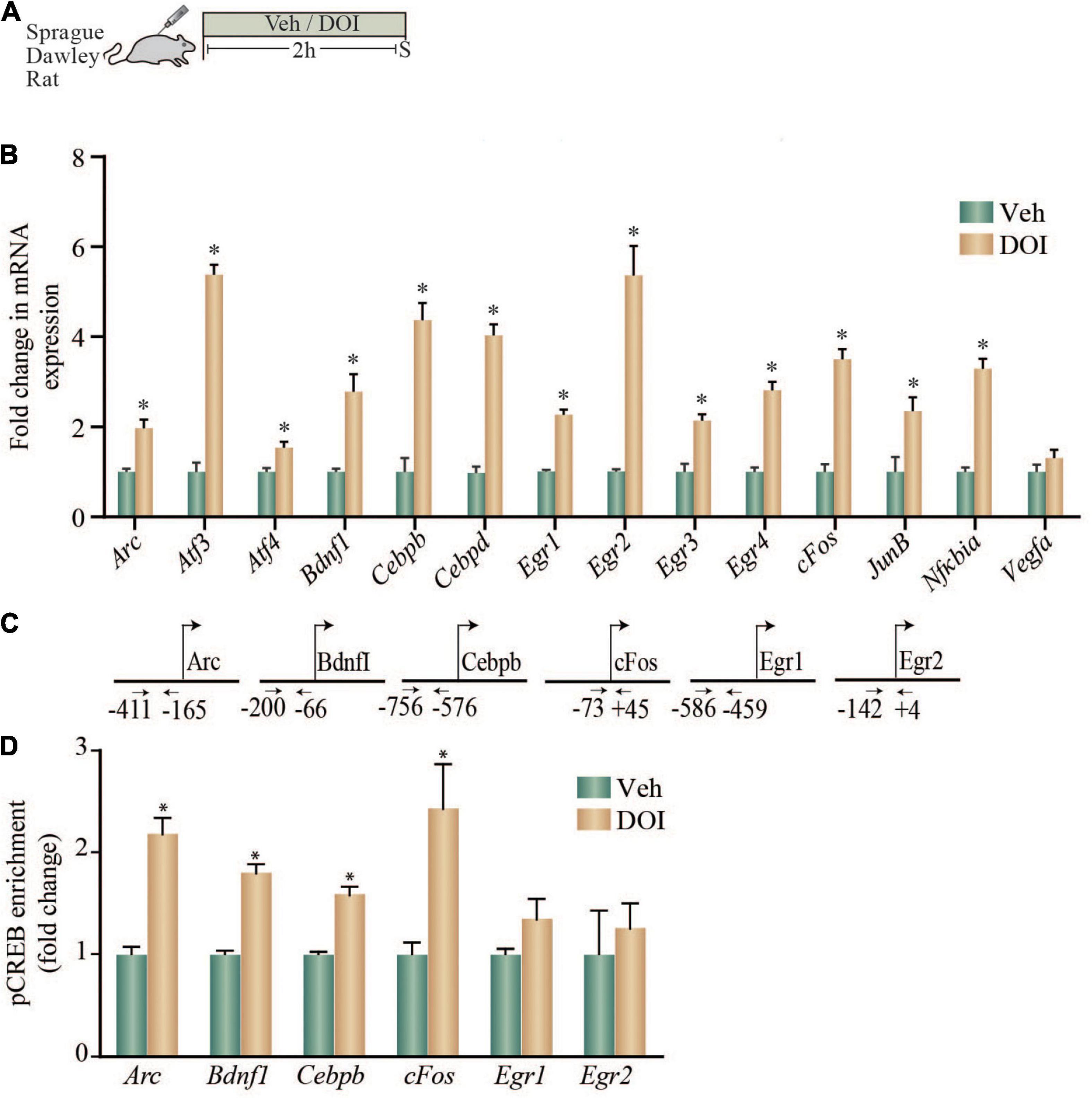
Figure 3. Acute treatment with DOI enhances both the expression of putative CRE-containing plasticity-associated genes and the enrichment of pCREB within the promoter regions of specific plasticity-associated genes in the neocortex of adult rats. (A) Shown is a schematic of the treatment paradigm wherein adult Sprague-Dawley rats were injected with vehicle or DOI (8 mg/kg) and were sacrificed 2 h following treatment. (B) The bar graph indicates the fold change in mRNA expression of specific plasticity-associated genes in the neocortex of vehicle and DOI-treated rats represented as fold change of vehicle ± SEM (n = 4–6 per treatment group, *p < 0.05 as compared to vehicle, unpaired Students t-test). (C) Shown are the chromatin immunoprecipitation (ChIP) PCR amplicons with primer locations spanning putative CRE sequences in the upstream gene regulatory sequences for Arc, Bdnf1, Cebpb, cFos, Egr1, and Egr2. (D) Shown is a bar graph for pCREB enrichment at the Arc, Bdnf1, Cebpb, cFos, Egr1, and Egr2 promoters based on ChIP analysis performed on tissue derived from the neocortex of vehicle and DOI treated adult rats. Results are expressed as the fold change of vehicle ± SEM. (n = 7–10 animals per treatment group, *p < 0.05 as compared to vehicle, unpaired Students t-test).
We next examined if a subset of the genes upregulated by DOI treatment, that are known to contain putative CRE binding sites in their promoter regions based on in silico analysis, also exhibit enrichment for pCREB within their promoters (Figure 3C). Enhanced expression of Arc, Bdnf1, Cebpb, and cFos in the neocortex of DOI-treated animals was accompanied by a significant enrichment of pCREB at the promoters of Arc, Bdnf1, Cebpb, and cFos (Figure 3D). In contrast, the enhanced gene expression of Egr1 and Egr2 was not associated with any significant change in pCREB enrichment at putative CRE sites within their promoter regions (Figure 3D). Taken together, these results indicate that in vivo administration of the 5-HT2A receptor agonist DOI, evokes a robust upregulation of several neuronal plasticity-associated genes in the neocortex, a subset of which exhibit significant pCREB enrichment in their promoter regions.
To draw a comparison of the nature and magnitude of regulation of this subset of neuronal plasticity-associated genes in the neocortex by the serotonergic hallucinogen, DOI, with that evoked by a rapid-action antidepressant treatment, electroconvulsive seizure (ECS) treatment, neocortices derived from acute ECS or sham treated rats were subjected to qPCR analysis (Supplementary Figure 1). We noted a robust increase in Arc, Bdnf1, Cebpb, cFos, and Egr2 but not Egr1 mRNA levels following acute ECS treatment (Supplementary Figure 1), with the nature and scale of upregulation comparable to that following DOI treatment.
Dimethoxy-4-Iodoamphetamine-Mediated Regulation of Plasticity-Associated Gene Expression in Cortical Brain Regions Is Perturbed in CREBαδ Knockout (CREBαδ KO) Mice
Given the evidence both in vitro and in vivo, that the 5-HT2A receptor agonist, DOI induces (1) a robust increase in pCREB levels in rat cortical neurons, (2) a significant enrichment of pCREB at the promoters of specific plasticity-associated genes that are robustly enhanced by DOI treatment, we next sought to address the contribution of CREB to the regulation of these transcripts. We used hypomorphic CREBαδ KO mice (Blendy et al., 1996) that are reported to have a greater than 90% reduction in CREB binding activity to consensus CRE target sites (Walters and Blendy, 2001). We treated WT and CREBαδ KO mice with vehicle or DOI (8 mg/kg), and assessed transcript expression of specific neuronal plasticity-associated genes at a 2 h time-point post treatment (Figure 4A). To rule out the possibility that CREB may indirectly regulate 5-HT2A or 5-HT2C receptors, we first assessed whether the baseline expression of these receptors was altered in CREBαδ KO mice as compared to their WT controls, and observed no change in 5-HT2A or 5-HT2C receptor mRNA levels within the neocortex (Figure 4B). Further to assess whether CREB deficient mice exhibit any change in 5-HT2A receptor evoked behavioral responses, we evaluated the HTR behavior evoked by DOI. Behavioral analysis to quantify the number of HTR events in WT and CREBαδ KO mice, indicated that the number of HTR responses evoked by DOI were unaltered in CREBαδ KO mice (Figure 4C). While we noted no significant two-way ANOVA interaction of DOI and CREBαδ KO genotype, we observed a significant main effect of DOI [F(1,12) = 58.64, p < 0.0001]. This indicates that the loss of CREBαδ isoforms does not alter either the expression of the 5-HT2A receptor, or the behavioral HTRs evoked by the 5-HT2A receptor agonist DOI, that are critically dependent on the cortical 5-HT2A receptor.
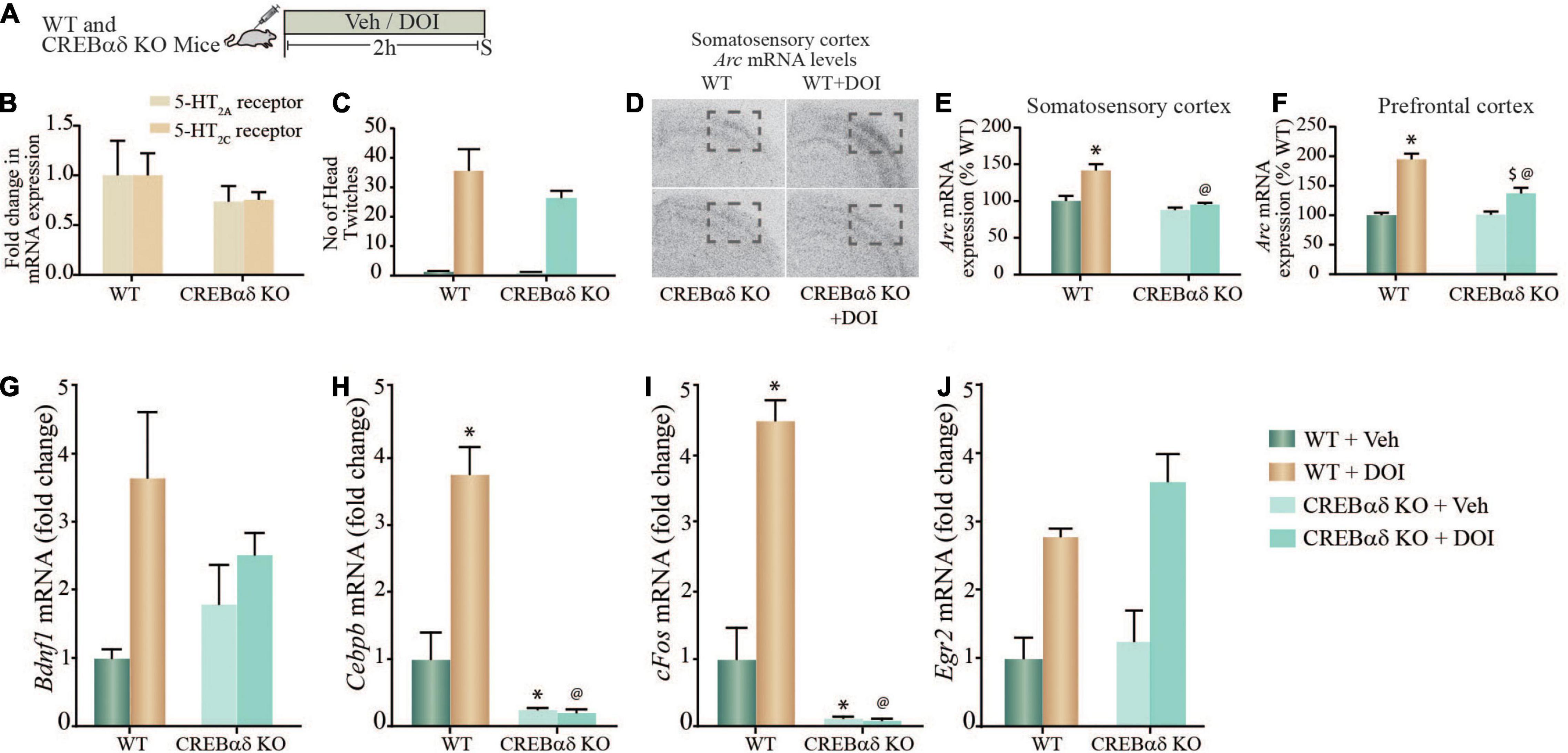
Figure 4. DOI-mediated regulation of plasticity-associated gene expression in cortical brain regions is perturbed in CREBαδ knockout (CREBαδ KO) mice. (A) Shown is a schematic of the acute treatment paradigm for wild type (WT) and CREBαδ KO mice, with vehicle (saline) or DOI (8 mg/kg) followed by sacrifice 2 h after treatment. (B) The bar graph depicts quantitative qPCR analysis for 5-HT2A and 5-HT2C receptors in the neocortex of WT and CREBαδ KO mice represented as fold change of WT ± SEM. (n = 3–4 animals per treatment group). (C) The bar graph depicts the quantitation of head-twitch responses evoked in response to acute treatment with the 5-HT2A receptor agonist, DOI or vehicle in both WT and CREBαδ KO mice (n = 4 animals per treatment group). (D) Shown are representative autoradiographs for Arc mRNA expression in the neocortex from WT, WT + DOI, CREBαδ KO, and CREBαδ KO + DOI mice, with the outline inset indicating the somatosensory region. Shown are bar graphs for the quantitative densitometric analysis of levels of Arc mRNA expression in the somatosensory (E) and prefrontal (F) cortex following DOI or vehicle treatment to WT and CREBαδ KO mice. Results are represented as percentage of WT and are mean ± SEM (n = 3–4 animals per group, *p < 0.05 as compared to WT + Veh mice, $p < 0.05 as compared to CREBαδ KO + Veh mice, @p < 0.05 as compared to WT + DOI mice, two-way ANOVA, Tukey’s post hoc test). (G–J) Bar graphs depict quantitation of qPCR analysis for mRNA expression of Bdnf1 (G), Cebpb (H), cFos (I), and Egr2 (J), following acute DOI or vehicle treatment to WT and CREBαδ KO mice. (n = 3–4 animals per group, *p < 0.05 as compared to WT mice, $p < 0.05 as compared to CREBαδ KO mice, @p < 0.05 as compared to WT + DOI mice, two-way ANOVA, Tukey’s post hoc test).
We next performed in situ hybridization and qPCR analysis to address whether the regulation of specific neuronal plasticity-associated genes that exhibit pCREB enrichment at their promoters following DOI treatment, were altered in the neocortex of CREBαδ KO mice. Radioactive in situ hybridization analysis indicated that the DOI-evoked robust induction of Arc mRNA levels both in the somatosensory cortex and in the prefrontal cortex was significantly attenuated in CREBαδ KO mice (Figure 4D). Two-way ANOVA analysis for Arc mRNA levels in the somatosensory cortex (Figure 4E) indicated a significant DOI by CREBαδ KO genotype interaction [F(1,12) = 8.39, p = 0.013], as well as significant main effects of DOI [F(1,12) = 16.81, p = 0.002] and CREBαδ KO genotype [F(1,12) = 24.39, p = 0.0003]. The robust DOI-evoked upregulation of Arc mRNA in the somatosensory cortex of WT control mice was completely lost in the CREBαδ KO mice. Two-way ANOVA analysis for Arc mRNA levels in the prefrontal cortex (Figure 4F) indicated a significant DOI by CREBαδ KO genotype interaction [F(1,11) = 15.73, p = 0.002], as well as significant main effects of DOI [F(1,11) = 78.83, p = 0.0001] and CREBαδ KO genotype [F(1,11) = 14.75, p = 0.003]. The robust DOI-evoked upregulation of Arc mRNA in the prefrontal cortex of WT control mice was significantly attenuated in the CREBαδ KO mice. No change was observed in the basal expression of Arc mRNA in either the somatosensory or prefrontal of CREBαδ KO mice, which did not differ from vehicle-treated WT controls.
qPCR analysis was carried out to assess whether the DOI-evoked upregulation of Bdnf1, Cebpb, cFos, and Egr2 mRNA expression was altered in the neocortex of CREBαδ KO mice following acute DOI administration (Figures 4G–J). Two-way ANOVA analysis for Bdnf1 mRNA levels in the neocortex indicated no significant DOI by CREBαδ KO genotype interaction (Figure 4G), however we did observe a significant main effect of DOI [F(1,10) = 10.19, p = 0.01] and no main effect of CREBαδ KO genotype. Two-way ANOVA analysis for Cebpb mRNA levels in the neocortex (Figure 4H) indicated a significant DOI by CREBαδ KO genotype interaction [F(1,12) = 6.413, p = 0.026], as well as a trend toward a main effect of DOI [F(1,12) = 3.388, p = 0.09] and a significant main effect of CREBαδ KO genotype [F(1,12) = 50.49, p = 0.0001]. Tukey’s post hoc group comparisons indicated that the CREBαδ KO mice exhibited a significant baseline decrease (p = 0.03) in Cebpb mRNA levels in the neocortex. Further, while DOI-evoked a robust and significant upregulation of Cebpb mRNA levels in WT animals (p = 0.04), this was completely lost in the CREBαδ KO mice. Cebpb mRNA levels in the DOI-treated WT cohort differed significantly from the DOI-treated CREBαδ KO mice (p < 0.0001). Two-way ANOVA analysis for cFos mRNA levels in the neocortex (Figure 4I) indicated a significant DOI by CREBαδ KO genotype interaction [F(1,12) = 8.966, p = 0.011], as well as a trend toward a main effect of DOI [F(1,12) = 4.493, p = 0.056] and a significant main effect of CREBαδ KO genotype [F(1,12) = 102.5, p < 0.0001]. Tukey’s post hoc group comparisons indicated that the CREBαδ KO mice exhibited a significant baseline reduction (p = 0.001) in expression of cFos mRNA in the neocortex, and while DOI-evoked a robust and significant upregulation of cFos mRNA levels in WT animals (p = 0.02) this was completely lost in the CREBαδ KO mice. Neocortical c-Fos mRNA levels differed significantly between DOI-treated WT mice and the DOI-treated CREBαδ KO cohort (p < 0.0001). In contrast, we observed no significant DOI by CREBαδ KO genotype interaction for Egr2 mRNA levels within the neocortex (Figure 4J). Further, while we did note a significant main effect of DOI for Egr2 expression [F(1,11) = 10.19, p = 0.003] we observed no main effect of CREBαδ KO genotype. Collectively, these observations indicate that CREB contributes to the acute DOI-evoked upregulation of Arc, Cebpb, and cFos mRNA within the neocortex, but not to the DOI-induced neocortical increase in Bdnf1 and Egr2 mRNA expression. Furthermore, baseline expression of Cebpb and cFos mRNA, but not Arc mRNA is also significantly attenuated in the CREBαδ KO mice. These results demonstrate that the transcription factor CREB contributes to the regulation of a subset of neuronal plasticity-associated genes that are regulated by the hallucinogenic 5-HT2A receptor agonist, DOI in the neocortex.
Discussion
Here, we show that DOI, a hallucinogenic ligand of the Gq-coupled 5-HT2A receptor, recruits the nuclear transcription factor CREB to influence the expression of a specific subset of neuronal plasticity-associated genes in the rodent neocortex. DOI mediated stimulation of the 5-HT2A receptor in rat cortical neurons recruits the PLC, MAP kinase and CaMKII signaling pathways to rapidly increase the phosphorylation of CREB. 5-HT2A receptor stimulation by DOI, results in the upregulation of Arc, Bdnf1, Cebpb, and Egr2 mRNA in cortical neurons, with a role for both the MAP kinase and CaMKII signaling pathways. We also noted that while the regulation of Egr1 is dependent on the MAP kinase pathway, the regulation of cFos expression by DOI is dependent on the CaMKII pathway. Further, we show recruitment of pCREB at CRE binding sites within the promoters of a subset of DOI-regulated target genes (Arc, Bdnf1, Cebpb, cFos) in the neocortex. In contrast, there are also specific DOI-regulated plasticity-associated genes (Egr1, Egr2) wherein we did not observe pCREB enrichment at putative CRE sites in their promoters. These findings collectively indicate that 5-HT2A receptor stimulation enhances pCREB enrichment at the promoters of a subset of DOI regulated target genes, suggestive of an upregulation of neuronal plasticity-associated genes in both a CREB-dependent and independent manner. This is further supported by evidence that the DOI-evoked transcriptional upregulation of specific neuronal plasticity-associated genes (Arc, cFos, and Cebpb) is attenuated in the neocortex of CREBαδ KO mice (Figure 5).
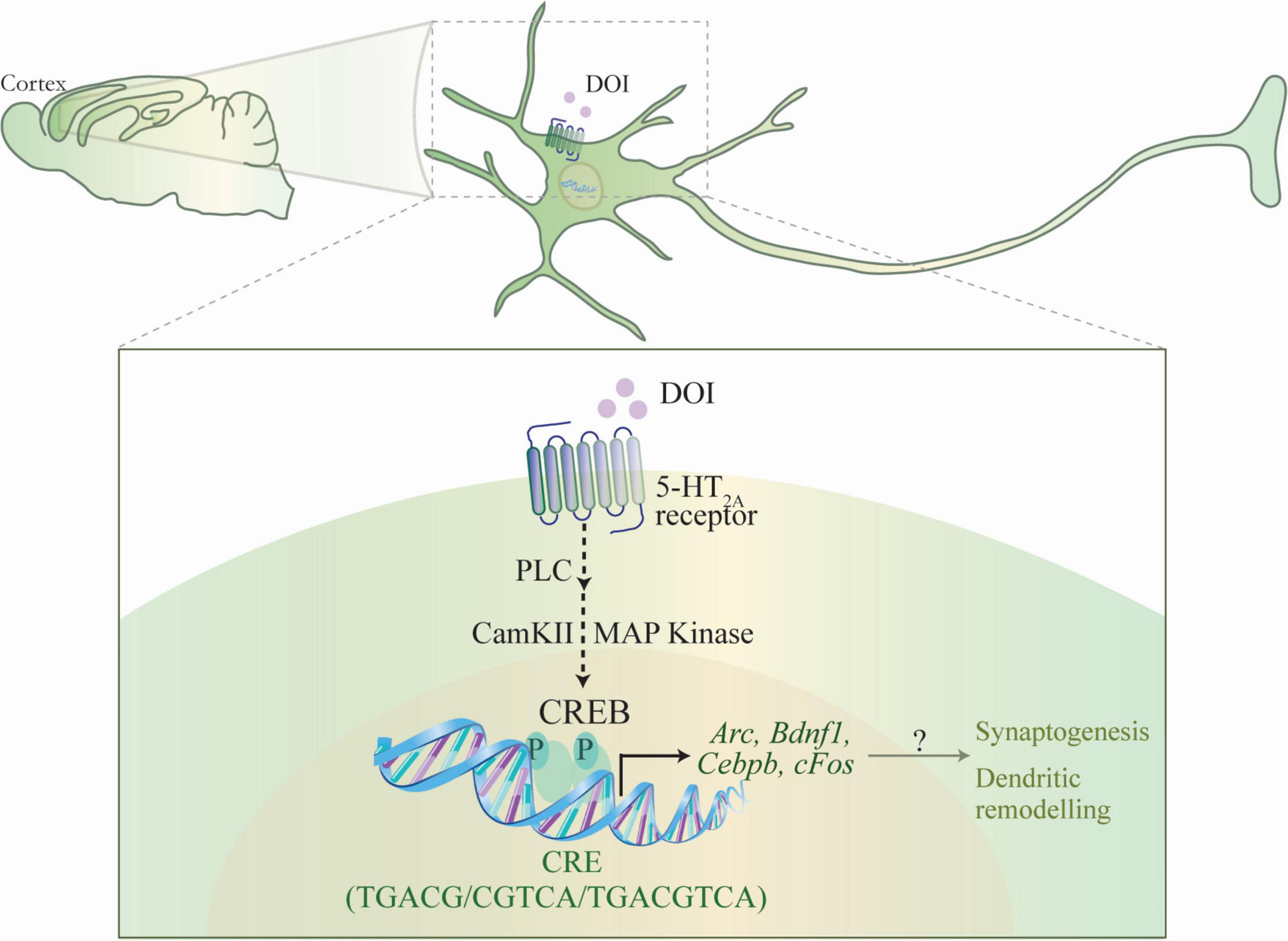
Figure 5. Schematic depicting the putative mechanism for the CREB-dependent regulation of neuronal plasticity-associated gene expression by the hallucinogenic 5-HT2A receptor agonist DOI. DOI, a hallucinogenic agonist of the 5-HT2A receptor is known to evoke a specific transcriptome signature within the neocortex, including the upregulation of the expression of several plasticity-associated genes. The schematic indicates a putative mechanism through which DOI-mediated stimulation of the Gq-coupled 5-HT2A receptor results in the recruitment of the phospholipase C (PLC), MAP kinase and CaMKII signaling pathways, which would further result in the phosphorylation of the transcription factor CREB, thus facilitating the CREB-dependent transcription of plasticity-associated genes, Arc, Bdnf1, Cebpb, cFos. This raises the intriguing possibility that CREB-dependent regulation of gene expression could contribute to the effects of the hallucinogenic 5-HT2A receptor agonist DOI, on neuronal plasticity, synaptogenesis and cell survival.
Prior literature indicates that ligands at the 5-HT2A receptor can differentially recruit unique transcriptome fingerprints in the neocortex (González-Maeso et al., 2003), with the hallucinogenic compounds DOI and LSD enhancing the expression of specific transcripts, including Egr1 and Egr2 (González-Maeso et al., 2003, 2007). It is interesting that the nature and magnitude of regulation of specific neuronal plasticity-associated genes is comparable to that noted with rapid-action antidepressant treatments such as ECS. Despite the knowledge that hallucinogenic agonists of the 5-HT2A receptor, such as DOI, induce the expression of multiple neuronal plasticity-associated genes, the contribution of specific signaling pathways and transcription factors to the 5-HT2A receptor regulated cortical gene expression remains poorly elucidated. DOI-mediated 5-HT2A receptor stimulation recruits the phospholipase C (PLC)-PKC-MAP kinase cascade and the PLC-CaMKII pathways that are known to also target the transcription factor CREB (Banerjee and Vaidya, 2020; Sharp and Barnes, 2020). The beta-arrestin-receptor complex also acts as a scaffold to activate the Raf-MEK-MAP kinase cascade (Schmid et al., 2008; McCorvy and Roth, 2015). Following 5-HT2A receptor activation by DOI, we observe that both the MAP kinase and CaMKII pathways, contribute to the DOI-evoked transcriptional increase of Arc, Bdnf1, Cebpb, and Egr2 expression in rat cortical neurons, whilst the CaMKII pathway and the MAP kinase pathway contribute to the DOI-mediated regulation of cFos and Egr1 expression respectively. This highlights the recruitment of distinct signaling pathways by the 5-HT2A receptor agonist, DOI (Banerjee and Vaidya, 2020), several of which converge on the transcription factor CREB (Shaywitz and Greenberg, 1999), placing it as a potential key ‘hub’ regulator of the transcriptional changes that arise in response to DOI treatment. Interestingly, many of the genes we find to be robustly enhanced in expression following DOI treatment in cortical neurons Arc, Bdnf1, Cebpb, cFos, Egr1, and Egr2, are reported to contain putative CRE sites (TGACG/CGTCA/TGACGTCA) (Sheng et al., 1990; Niehof et al., 1997; Ahn et al., 1998; Walters and Blendy, 2001; Fukuchi et al., 2005; Bilbao et al., 2014; Duclot and Kabbaj, 2017; Ly et al., 2020). Our in vitro studies clearly indicate a robust induction in pCREB levels at the Ser133 site, with phosphorylation via the MAP kinase and CaMKII pathways likely contributing to this induction. Our experiments motivate further characterization of the target sites of CREB phosphorylation (Kornhauser et al., 2002), as certain phosphorylation signatures like Ser142 (Sun et al., 1994) can also serve to evoke an inhibitory effect on CREB mediated transcription.
A previous microarray study from our lab has reported several genes to be upregulated by DOI in the rodent neocortex (Benekareddy et al., 2010). We performed in silico analysis on the published array results, and noted that several of the genes upregulated by DOI have CRE elements in their upstream regulatory promoter regions, suggestive of a putative role for CREB in the regulation of multiple DOI-evoked transcripts. DOI-evoked a robust increase in the transcript expression of several CRE-containing genes, namely Arc, Atf3, Atf4, Bdnf1, Cebpb, Cebpd, Egr1, Egr2, Egr3, Egr4, cFos, JunB, and Nfkbia in the rat neocortex. Interestingly, we observed pCREB enrichment at the promoters of specific DOI-regulated genes. The expression of Arc, Bdnf1, Cebpb, and cFos genes but not Egr1 and Egr2 expression was accompanied by a significant enrichment of pCREB at their promoters in the neocortex of DOI-treated animals. In this regard, it is interesting to note that the genes, Egr1 and Egr2 which are part of the reported DOI-evoked hallucinogenic fingerprint (González-Maeso et al., 2003) do not appear to show pCREB enrichment at their promoters. While we have restricted our analysis of pCREB recruitment to CRE sites close to the promoter region, we cannot preclude the possibility of CREB binding at remote CRE sites either in distal enhancer regions, or within introns as we have not scanned pCREB enrichment at these loci. It is also important to keep in mind that the differential regulation of target genes by CREB may further be influenced by CRE sequence composition, location of the CRE and distance from the transcription start site (Mayr and Montminy, 2001; Altarejos and Montminy, 2011; Davis et al., 2020). Thus, the presence of a CRE site does not necessarily predict the recruitment of pCREB, and other transcription factors besides CREB are also likely to be recruited by DOI and by neuronal activity-dependent mechanisms, to evoke transcriptional increase of target genes. Our findings provide impetus for a broader genome-wide analysis of pCREB enrichment to get a sense of the span of pCREB-mediated regulation of transcription by DOI, and an understanding of recruitment of pCREB at both canonical and non-canonical CREs.
It is also of interest to note that the target genes regulated by DOI are known to exhibit substantial signaling crosstalk, and could also exert further feedback effects on CREB-dependent transcriptional regulation (Wu et al., 2001; Deisseroth and Tsien, 2002; Wiegert and Bading, 2011; Belgacem and Borodinsky, 2017). Bdnf, a DOI-regulated target gene, also contributes to the transcriptional regulation of Arc mRNA expression (Bramham et al., 2008; Benekareddy et al., 2012), and could further via regulation of the MAP kinase cascade impinge on pCREB mediated gene regulation (Finkbeiner et al., 1997). This raises the intriguing possibility that reciprocal interactions between BDNF and CREB could serve to amplify the regulation of several neuronal plasticity-associated genes (Finkbeiner et al., 1997; Shieh and Ghosh, 1999; Nair and Vaidya, 2006). These include the transcriptional regulation of Arc and c-Fos which are reported to play a significant role in coupling experience-dependent transcriptional regulation to synaptic plasticity (Duman et al., 2005; Bramham et al., 2008; Flavell and Greenberg, 2008; Shepherd and Bear, 2011; Minatohara et al., 2015). Fos may also function at enhancer elements to coordinate global activity-dependent gene transcription (Malik et al., 2014; Joo et al., 2016). Further, following DOI-evoked stimulation of the 5-HT2A receptor, membrane depolarization and enhanced firing could also recruit additional activity-dependent mechanisms that drive this transcriptional program. This then suggests that stimulation of the 5-HT2A receptor, sets into play a coordinated transcriptional program that involves crosstalk of diverse signaling cascades and transcription factors that drive the expression of several neuronal plasticity-associated genes (Arc, Bdnf1, cFos), with CREB being amongst the key hub transcriptional factors that contributes to an important component of the DOI-evoked gene regulation pattern.
We have capitalized on the use of the CREBαδ KO mouse line, which is deficient for the α and δ CREB isoforms leading to a robust reduction in CREB binding to consensus CRE target sites (Walters and Blendy, 2001), to evaluate the contribution of CREB to the effects of DOI on neuronal plasticity-associated gene expression. It is important to note that the CREBαδ KO mice do not exhibit alterations in either the baseline expression of the 5-HT2A receptor, or the stereotypical HTR behavior evoked by the 5-HT2A receptor agonist DOI, that is known to be dependent on the cortical 5-HT2A receptor. This would suggest that alterations in DOI-evoked gene expression in CREBαδ KO mice are unlikely to arise due to a change at the level of 5-HT2A receptor expression and coupling given that the CREBαδ KO mice exhibit a robust DOI-evoked HTR response no different from WT controls and show no change in 5-HT2A receptor expression. Interestingly, the DOI-evoked induction in cortical Arc mRNA expression was lost in CREBαδ KO mice. Similar to this observation, the DOI-evoked increase in Cebpb and cFos mRNA levels were also abolished in the neocortex of CREBαδ KO mice. Further, in CREB deficient mice the baseline expression of Cebpb and cFos, but not Arc mRNA was also reduced, thus supporting a role for CREB in regulation of basal expression of Cebpb and cFos. The DOI-evoked induction in Egr2 mRNA was not altered in the neocortex of CREBαδ KO mice, which is consistent with the evidence that pCREB was not found to be enriched at the Egr2 promoter following DOI treatment. The DOI-evoked increase in Bdnf1 transcript variant levels was also not significantly attenuated in CREBαδ KO mice, and further we did not observe a change in basal Bdnf1 transcript expression either. We have focused on Bdnf1 which has been reported to contain CRE elements at its promoter, and exhibit CREB-mediated regulation in other contexts (Tabuchi et al., 2002; Esvald et al., 2020). BdnfIII and BdnfIV promoters are also known to contain CRE elements, as is the Bdnf coding exon, and it will be important to systematically address the contribution of CREB to the DOI-mediated regulation of multiple Bdnf transcript variants (Tao et al., 1998; Esvald et al., 2020). Indeed, prior reports clearly indicate several conditions in which CREB-dependent regulation of Bdnf gene expression contributes to neuroplasticity, in particular in the context of neuronal activity-dependent transcriptional coupling that drives structural and synaptic plasticity (Shieh and Ghosh, 1999; Vogt et al., 2014; Yan et al., 2016; Rafa-Zabłocka et al., 2018). While the CREB deficient mice provide a valuable tool to address the contribution of CREB to the DOI-mediated regulation of plasticity-associated gene expression, they come with a caveat of a constitutive, developmental onset loss-of-function of CREB which could in turn disrupt several key signaling pathways. In addition, we have not extensively profiled the consequence of loss of CREBαδ subunits on the composition of the transcription factors that now occupy CRE sites in the absence of CREBαδ, which could itself substantially influence CRE-mediated transcriptional outcomes. Further experiments are warranted to systematically evaluate the contribution of CREB to the effects of DOI on neuronal plasticity-associated genes using approaches that allow for a more targeted strategy of adult onset, neuronal circuit-specific loss of function of CREB.
Our findings highlight a key role for CREB in contributing to the DOI-mediated regulation of specific neuronal plasticity-associated genes in the neocortex. This raises the intriguing possibility that similar to both slow-onset and rapid action antidepressants that recruit CREB to drive transcriptional changes in neurotrophic and plasticity-associated genes (Nibuya et al., 1996; Duman and Nibuya, 1997; Thome et al., 2000; Chen et al., 2001), serotonergic psychedelics that target the 5-HT2A receptor may also recruit CREB to drive a plasticity-associated transcriptional program. These observations encourage further investigation into the role of CREB in regulating the transcription of plasticity-associated genes evoked by hallucinogenic 5-HT2A receptor agonists, thus creating a conducive milieu for the psychoplastogenic actions of serotonergic psychedelics on dendritic plasticity and synaptogenesis in the neocortex.
Data Availability Statement
The raw data supporting the conclusions of this article will be made available by the authors, without undue reservation.
Ethics Statement
The animal study was reviewed and approved by the University of Pennsylvania Animal Care and Use Committee. All animal procedures using Sprague-Dawley rats and serotonin2A receptor knockout (5-HT2A–/–) mice were carried out in accordance with the Committee for Care and Supervision of Experimental Animals (CPCSEA) and approved by the TIFR Institutional Animal Ethics Committee. All experiments with the CREBαδ KO mouse line were carried out in accordance with the NIH guideline for the care and use of laboratory animals.
Author Contributions
LD, MB, SF, FM, BJ, UG, and TG performed the experiments and analyzed the data. JB and VV designed the experiments and analyzed the data. SF and VV wrote the manuscript. All authors contributed to the article and approved the submitted version.
Funding
We acknowledge funding from the Tata Institute of Fundamental Research and Department of Atomic Energy, Mumbai (Grant reference number: RTI4003) and the Department of Biotechnology, Government of India (Grant BT/COE/34/SP17426/2016).
Conflict of Interest
The authors declare that the research was conducted in the absence of any commercial or financial relationships that could be construed as a potential conflict of interest.
Publisher’s Note
All claims expressed in this article are solely those of the authors and do not necessarily represent those of their affiliated organizations, or those of the publisher, the editors and the reviewers. Any product that may be evaluated in this article, or claim that may be made by its manufacturer, is not guaranteed or endorsed by the publisher.
Acknowledgments
We would like to thank Noelia Weisstaub, University of Buenos Aires and Jay Gingrich, Columbia University for the gift of the serotonin2A receptor deficient mouse line. We would also like to thank Shital Suryavanshi and the animal house staff at the Tata Institute of Fundamental Research (TIFR), Mumbai for technical assistance.
Supplementary Material
The Supplementary Material for this article can be found online at: https://www.frontiersin.org/articles/10.3389/fnmol.2021.790213/full#supplementary-material
Footnotes
References
Aghajanian, G. K., and Marek, G. J. (1999). Serotonin and hallucinogens. Neuropsychopharmacology 21, 16S–23S. doi: 10.1016/S0893-133X(98)00135-3
Ahn, S., Olive, M., Aggarwal, S., Krylov, D., Ginty, D. D., and Vinson, C. (1998). A dominant-negative inhibitor of CREB reveals that it is a general mediator of stimulus-dependent transcription of c-fos. Mol. Cell. Biol. 18, 967–977. doi: 10.1128/MCB.18.2.967
Altarejos, J. Y., and Montminy, M. (2011). CREB and the CRTC co-activators: sensors for hormonal and metabolic signals. Nat. Rev. Mol. Cell Biol. 12, 141–151. doi: 10.1038/nrm3072
Artin, H., Zisook, S., and Ramanathan, D. (2021). How do serotonergic psychedelics treat depression: the potential role of neuroplasticity. World J. Psychiatry 11, 201–214. doi: 10.5498/wjp.v11.i6.201
Banerjee, A. A., and Vaidya, V. A. (2020). Differential signaling signatures evoked by DOI versus lisuride stimulation of the 5-HT2A receptor. Biochem. Biophys. Res. Commun. 531, 609–614. doi: 10.1016/j.bbrc.2020.08.022
Banks, M. I., Zahid, Z., Jones, N. T., Sultan, Z. W., and Wenthur, C. J. (2021). Catalysts for change: the cellular neurobiology of psychedelics. Mol. Biol. Cell 32, 1135–1144. doi: 10.1091/MBC.E20-05-0340
Belgacem, Y. H., and Borodinsky, L. N. (2017). CREB at the crossroads of activity-dependent regulation of nervous system development and function. Adv. Exp. Med. Biol. 1015, 19–39.
Benekareddy, M., Goodfellow, N. M., Lambe, E. K., and Vaidya, V. A. (2010). Enhanced function of prefrontal serotonin 5-HT2 receptors in a rat model of psychiatric vulnerability. J. Neurosci. 30, 12138–12150. doi: 10.1523/JNEUROSCI.3245-10.2010
Benekareddy, M., Nair, A. R., Dias, B. G., Suri, D., Autry, A. E., Monteggia, L. M., et al. (2012). Induction of the plasticity-Associated immediate early gene Arc by stress and hallucinogens: role of brain-derived neurotrophic factor. Int. J. Neuropsychopharmacol. 16, 405–415. doi: 10.1017/S1461145712000168
Berthoux, C., Barre, A., Bockaert, J., Marin, P., and Bécamel, C. (2019). Sustained activation of postsynaptic 5-HT 2A receptors gates plasticity at prefrontal cortex synapses. Cereb. Cortex 29, 1659–1669. doi: 10.1093/cercor/bhy064
Bilbao, A., Rieker, C., Cannella, N., Parlato, R., Golda, S., Piechota, M., et al. (2014). CREB activity in dopamine D1 receptor expressing neurons regulates cocaine-induced behavioral effects. Front. Behav. Neurosci. 8:212. doi: 10.3389/fnbeh.2014.00212
Blendy, J. A., Kaestner, K. H., Schmid, W., Gass, P., and Schutz, G. (1996). Targeting of the CREB gene leads to up-regulation of a novel CREB mRNA isoform. EMBO J. 15, 1098–1106.
Bramham, C. R., Worley, P. F., Moore, M. J., and Guzowski, J. F. (2008). The immediate early gene Arc/Arg3.1: regulation, mechanisms, and function. J. Neurosci. 28, 11760–11767. doi: 10.1523/JNEUROSCI.3864-08.2008
Canal, C. E., and Morgan, D. (2012). Head-twitch response in rodents induced by the hallucinogen 2,5-dimethoxy-4-iodoamphetamine: a comprehensive history, a re-evaluation of mechanisms, and its utility as a model. Drug Test. Anal. 4, 556–576. doi: 10.1002/dta.1333
Carlezon, W. A., Duman, R. S., and Nestler, E. J. (2005). The many faces of CREB. Trends Neurosci. 28, 436–445. doi: 10.1016/j.tins.2005.06.005
Chen, A. C. H., Shirayama, Y., Shin, K. H., Neve, R. L., and Duman, R. S. (2001). Expression of the cAMP response element binding protein (CREB) in hippocampus produces an antidepressant effect. Biol. Psychiatry 49, 753–762. doi: 10.1016/S0006-3223(00)01114-8
Davis, J. E., Insigne, K. D., Jones, E. M., Hastings, Q. A., Boldridge, W. C., and Kosuri, S. (2020). Dissection of c-AMP response element architecture by using genomic and episomal massively parallel reporter assays. Cell Syst. 11, 75.e7–85.e7. doi: 10.1016/j.cels.2020.05.011
De Gregorio, D., Aguilar-Valles, A., Preller, K. H., Heifets, B. D., Hibicke, M., Mitchell, J., et al. (2021). Hallucinogens in mental health: preclinical and clinical Studies on LSD, Psilocybin, MDMA, and ketamine. J. Neurosci. 41, 891–900. doi: 10.1523/JNEUROSCI.1659-20.2020
de Vos, C. M. H., Mason, N. L., and Kuypers, K. P. C. (2021). Psychedelics and neuroplasticity: a systematic review unraveling the biological underpinnings of psychedelics. Front. Psychiatry 12:724606. doi: 10.3389/fpsyt.2021.724606
Deisseroth, K., and Tsien, R. W. (2002). Dynamic multiphosphorylation passwords for activity-dependent gene expression. Neuron 34, 179–182. doi: 10.1016/s0896-6273(02)00664-5
Desouza, L. A., Sathanoori, M., Kapoor, R., Rajadhyaksha, N., Gonzalez, L. E., Kottmann, A. H., et al. (2011). Thyroid hormone regulates the expression of the sonic hedgehog signaling pathway in the embryonic and adult Mammalian brain. Endocrinology 152, 1989–2000. doi: 10.1210/en.2010-1396
Duclot, F., and Kabbaj, M. (2017). The role of early growth response 1 (EGR1) in brain plasticity and neuropsychiatric disorders. Front. Behav. Neurosci. 11:35. doi: 10.3389/fnbeh.2017.00035
Duman, R. S., Adams, D. H., and Simen, B. B. (2005). “Transcription factors as modulators of stress responsivity,” in Handbook of Stress and the Brain, eds T. Steckler, N. H. Kalin, and J. M. H. M. Reul (Amsterdam: Elsevier), 679–698.
Duman, R. S., and Nibuya, M. V. V. (1997). “A Role for CREB in Antidepressant Action,” in Antidepressants: New Pharmacological Strategies, ed. P. Skolnick (Totowa, NJ: Humana Press Inc.), 173–194.
Esvald, E. E., Tuvikene, J., Sirp, A., Patil, S., Bramham, C. R., and Timmusk, T. (2020). CREB family transcription factors are major mediators of BDNF transcriptional autoregulation in cortical neurons. J. Neurosci. 40, 1405–1426. doi: 10.1523/JNEUROSCI.0367-19.2019
Fanibunda, S. E., Deb, S., Maniyadath, B., Tiwari, P., Ghai, U., Gupta, S., et al. (2019). Serotonin regulates mitochondrial biogenesis and function in rodent cortical neurons via the 5-HT2A receptor and SIRT1–PGC-1α axis. Proc. Natl. Acad. Sci. U.S.A. 166, 11028–11037. doi: 10.1073/pnas.1821332116
Finkbeiner, S., Tavazoie, S. F., Maloratsky, A., Jacobs, K. M., Harris, K. M., and Greenberg, M. E. (1997). CREB: a major mediator of neuronal neurotrophin responses. Neuron 19, 1031–1047. doi: 10.1016/s0896-6273(00)80395-5
Flavell, S. W., and Greenberg, M. E. (2008). Signaling mechanisms linking neuronal activity to gene expression and plasticity of the nervous system. Annu. Rev. Neurosci. 31, 563–590. doi: 10.1146/annurev.neuro.31.060407.125631
Fukuchi, M., Tabuchi, A., and Tsuda, M. (2005). Transcriptional regulation of neuronal genes and its effect on neural functions: cumulative mRNA expression of PACAP and BDNF genes controlled by calcium and cAMP signals in neurons. J. Pharmacol. Sci. 98, 212–218. doi: 10.1254/jphs.fmj05001x4
González-Maeso, J., Weisstaub, N. V., Zhou, M., Chan, P., Ivic, L., Ang, R., et al. (2007). Hallucinogens recruit specific cortical 5-HT2A receptor-mediated signaling pathways to affect behavior. Neuron 53, 439–452. doi: 10.1016/j.neuron.2007.01.008
González-Maeso, J., Yuen, T., Ebersole, B. J., Wurmbach, E., Lira, A., Zhou, M., et al. (2003). Transcriptome fingerprints distinguish hallucinogenic and nonhallucinogenic 5-hydroxytryptamine 2A receptor agonist effects in mouse somatosensory cortex. J. Neurosci. 23, 8836–8843. doi: 10.1523/jneurosci.23-26-08836.2003
Halberstadt, A. L., and Geyer, M. A. (2013). Characterization of the head-twitch response induced by hallucinogens in mice: detection of the behavior based on the dynamics of head movement. Psychopharmacology 227, 727–739. doi: 10.1007/s00213-013-3006-z
Jefsen, O. H., Elfving, B., Wegener, G., and Müller, H. K. (2021). Transcriptional regulation in the rat prefrontal cortex and hippocampus after a single administration of psilocybin. J. Psychopharmacol. 35, 483–493. doi: 10.1177/0269881120959614
Joo, J.-Y., Schaukowitch, K., Farbiak, L., Kilaru, G., and Kim, T.-K. (2016). Stimulus-specific combinatorial functionality of neuronal c-fos enhancers. Nat. Neurosci. 19, 75–83. doi: 10.1038/nn.4170
Koch, J. M., Hinze-Selch, D., Stingele, K., Huchzermeier, C., Göder, R., Seeck-Hirschner, M., et al. (2009). Changes in CREB Phosphorylation and BDNF plasma levels during Psychotherapy of depression. Psychother. Psychosom. 78, 187–192. doi: 10.1159/000209350
Kornhauser, J. M., Cowan, C. W., Shaywitz, A. J., Dolmetsch, R. E., Griffith, E. C., Hu, L. S., et al. (2002). CREB transcriptional activity in neurons is regulated by multiple, calcium-specific phosphorylation events. Neuron 34, 221–233. doi: 10.1016/s0896-6273(02)00655-4
Krishnan, V., and Nestler, E. J. (2008). The molecular neurobiology of depression. Nature 455, 894–902. doi: 10.1038/nature07455
Krishnan, V., and Nestler, E. J. (2010). Linking molecules to mood: new insight into the biology of depression. Am. J. Psychiatry 167, 1305–1320. doi: 10.1176/appi.ajp.2009.10030434
Lonze, B. E., and Ginty, D. D. (2002). Function and regulation of CREB family transcription factors in the nervous system. Neuron 35, 605–623. doi: 10.1016/S0896-6273(02)00828-0
López-Giménez, J. F., and González-Maeso, J. (2018). Hallucinogens and serotonin 5-HT2A receptor-mediated signaling pathways. Curr. Top. Behav. Neurosci. 36, 45–73. doi: 10.1007/7854_2017_478
Ly, C., Greb, A. C., Cameron, L. P., Wong, J. M., Barragan, E. V., Wilson, P. C., et al. (2018). Psychedelics promote structural and functional neural plasticity. Cell Rep. 23, 3170–3182. doi: 10.1016/j.celrep.2018.05.022
Ly, C., Greb, A. C., Vargas, M. V., Duim, W. C., Grodzki, A. C. G., Lein, P. J., et al. (2020). Transient stimulation with psychoplastogens is sufficient to initiate neuronal growth. ACS Pharmacol. Transl. Sci. 4, 452–460. doi: 10.1021/acsptsci.0c00065
Madsen, M. K., Fisher, P. M., Burmester, D., Dyssegaard, A., Stenbæk, D. S., Kristiansen, S., et al. (2019). Psychedelic effects of psilocybin correlate with serotonin 2A receptor occupancy and plasma psilocin levels. Neuropsychopharmacology 44, 1328–1334. doi: 10.1038/S41386-019-0324-9
Malik, A. N., Vierbuchen, T., Hemberg, M., Rubin, A. A., Ling, E., Couch, C. H., et al. (2014). Genome-wide identification and characterization of functional neuronal activity-dependent enhancers. Nat. Neurosci. 17, 1330–1339. doi: 10.1038/nn.3808
Marek, G. J. (2017). “Interactions of hallucinogens with the glutamatergic system: permissive network effects mediated through cortical layer V pyramidal neurons. Curr. Top. Behav. Neurosci. 36, 107–135. doi: 10.1007/7854_2017_480
Martin, D. A., and Nichols, C. D. (2016). Psychedelics recruit multiple cellular types and produce complex transcriptional responses within the brain. EBioMedicine 11, 262–277. doi: 10.1016/j.ebiom.2016.08.049
Mayr, B., and Montminy, M. (2001). Transcriptional regulation by the phosphorylation-dependent factor creb. Nat. Rev. Mol. Cell Biol. 2, 599–609. doi: 10.1038/35085068
McCorvy, J. D., and Roth, B. L. (2015). Structure and function of serotonin G protein-coupled receptors. Pharmacol. Ther. 150, 129–142. doi: 10.1016/j.pharmthera.2015.01.009
Minatohara, K., Akiyoshi, M., and Okuno, H. (2015). Role of immediate-early genes in synaptic plasticity and neuronal ensembles underlying the memory trace. Front. Mol. Neurosci. 8:78. doi: 10.3389/fnmol.2015.00078
Nair, A., and Vaidya, V. A. (2006). Cyclic AMP response element binding protein and brain-derived neurotrophic factor: molecules that modulate our mood? J. Biosci. 31, 423–434. doi: 10.1007/BF02704114
Nibuya, M., Nestler, E. J., and Duman, R. S. (1996). Chronic antidepressant administration increases the expression of cAMP response element binding protein (CREB) in rat hippocampus. J. Neurosci. 16, 2365–2372. doi: 10.1523/jneurosci.16-07-02365.1996
Nichols, C. D., Garcia, E. E., and Sanders-Bush, E. (2003). Dynamic changes in prefrontal cortex gene expression following lysergic acid diethylamide administration. Brain Res. Mol. Brain Res. 111, 182–188. doi: 10.1016/s0169-328x(03)00029-9
Niehof, M., Manns, M. P., and Trautwein, C. (1997). CREB controls LAP/C/EBP beta transcription. Mol. Cell. Biol. 17, 3600–3613. doi: 10.1128/MCB.17.7.3600
Nutt, D., Erritzoe, D., and Carhart-Harris, R. (2020). Psychedelic Psychiatry’s brave new world. Cell 181, 24–28. doi: 10.1016/j.cell.2020.03.020
Olson, D. E. (2018). Psychoplastogens: a promising class of plasticity-promoting neurotherapeutics. J. Exp. Neurosci. 12:1179069518800508. doi: 10.1177/1179069518800508
Preller, K. H., Burt, J. B., Ji, J. L., Schleifer, C. H., Adkinson, B. D., Stämpfli, P., et al. (2018). Changes in global and thalamic brain connectivity in LSD-induced altered states of consciousness are attributable to the 5-HT2A receptor. eLife 7:e35082. doi: 10.7554/eLife.35082
Rafa-Zabłocka, K., Kreiner, G., Bagińska, M., and Nalepa, I. (2018). Selective depletion of CREB in serotonergic neurons affects the upregulation of brain-derived neurotrophic factor evoked by chronic fluoxetine treatment. Front. Neurosci. 12:637. doi: 10.3389/fnins.2018.00637
Raymond, J. R., Mukhin, Y. V., Gelasco, A., Turner, J., Collinsworth, G., Gettys, T. W., et al. (2001). Multiplicity of mechanisms of serotonin receptor signal transduction. Pharmacol. Ther. 92, 179–212. doi: 10.1016/s0163-7258(01)00169-3
Sakamoto, K., Karelina, K., and Obrietan, K. (2011). CREB: a multifaceted regulator of neuronal plasticity and protection. J. Neurochem. 116, 1–9. doi: 10.1111/j.1471-4159.2010.07080.x
Savalia, N. K., Shao, L. X., and Kwan, A. C. (2021). A dendrite-focused framework for understanding the actions of ketamine and psychedelics. Trends Neurosci. 44, 260–275. doi: 10.1016/j.tins.2020.11.008
Savino, A., and Nichols, C. D. (2021). Lysergic acid diethylamide induces increased signalling entropy in rats’ prefrontal cortex. J. Neurochem. [Epub ahead of print]. doi: 10.1111/jnc.15534
Schmid, C. L., Raehal, K. M., and Bohn, L. M. (2008). Agonist-directed signaling of the serotonin 2A receptor depends on beta-arrestin-2 interactions in vivo. Proc. Natl. Acad. Sci. U.S.A. 105, 1079–1084. doi: 10.1073/pnas.0708862105
Schmittgen, T. D., and Livak, K. J. (2008). Analyzing real-time PCR data by the comparative C(T) method. Nat. Protoc. 3, 1101–1108. doi: 10.1038/nprot.2008.73
Shao, L. X., Liao, C., Gregg, I., Davoudian, P. A., Savalia, N. K., Delagarza, K., et al. (2021). Psilocybin induces rapid and persistent growth of dendritic spines in frontal cortex in vivo. Neuron 109, 2535.e4–2544.e4. doi: 10.1016/j.neuron.2021.06.008
Sharp, T., and Barnes, N. M. (2020). Central 5-HT receptors and their function; present and future. Neuropharmacology 177:108155. doi: 10.1016/j.neuropharm.2020.108155
Shaywitz, A. J., and Greenberg, M. E. (1999). CREB: a stimulus-induced transcription factor activated by a diverse array of extracellular signals. Annu. Rev. Biochem. 68, 821–861. doi: 10.1146/annurev.biochem.68.1.821
Sheng, M., McFadden, G., and Greenberg, M. E. (1990). Membrane depolarization and calcium induce c-fos transcription via phosphorylation of transcription factor CREB. Neuron 4, 571–582. doi: 10.1016/0896-6273(90)90115-v
Shepherd, J. D., and Bear, M. F. (2011). New views of Arc, a master regulator of synaptic plasticity. Nat. Neurosci. 14, 279–284. doi: 10.1038/nn.2708
Shieh, P. B., and Ghosh, A. (1999). Molecular mechanisms underlying activity-dependent regulation of BDNF expression. J. Neurobiol. 41, 127–134.
Slocum, S. T., DiBerto, J. F., and Roth, B. L. (2021). Molecular insights into psychedelic drug action. J. Neurochem. [Epub ahead of print]. doi: 10.1111/jnc.15540
Sun, P., Enslen, H., Myung, P. S., and Maurer, R. A. (1994). Differential activation of CREB by Ca2+/calmodulin-dependent protein kinases type II and type IV involves phosphorylation of a site that negatively regulates activity. Genes Dev. 8, 2527–2539. doi: 10.1101/gad.8.21.2527
Tabuchi, A., Sakaya, H., Kisukeda, T., Fushiki, H., and Tsuda, M. (2002). Involvement of an upstream stimulatory factor as well as cAMP-responsive element-binding protein in the activation of brain-derived neurotrophic factor gene promoter I. J. Biol. Chem. 277, 35920–35931. doi: 10.1074/jbc.M204784200
Tao, X., Finkbeiner, S., Arnold, D. B., Shaywitz, A. J., and Greenberg, M. E. (1998). Ca2+ influx regulates BDNF transcription by a CREB family transcription factor-dependent mechanism. Neuron 20, 709–726. doi: 10.1016/S0896-6273(00)81010-7
Thome, J., Sakai, N., Shin, K. H., Steffen, C., Zhang, Y. J., Impey, S., et al. (2000). cAMP response element-mediated gene transcription is upregulated by chronic antidepressant treatment. J. Neurosci. 20, 4030–4036. doi: 10.1523/jneurosci.20-11-04030.2000
Titeler, M., Lyon, R. A., and Glennon, R. A. (1988). Radioligand binding evidence implicates the brain 5-HT2 receptor as a site of action for LSD and phenylisopropylamine hallucinogens. Psychopharmacology 94, 213–216. doi: 10.1007/BF00176847
Vaidya, V. A., Marek, G. J., Aghajanian, G. K., and Duman, R. S. (1997). 5-HT2A receptor-mediated regulation of brain-derived neurotrophic factor mRNA in the hippocampus and the neocortex. J. Neurosci. 17, 2785–2795.
Vargas, M. V., Meyer, R., Avanes, A. A., Rus, M., and Olson, D. E. (2021). Psychedelics and other psychoplastogens for treating mental illness. Front. Psychiatry 12:727117. doi: 10.3389/fpsyt.2021.727117
Vogt, M. A., Inta, D., Luoni, A., Elkin, H., Pfeiffer, N., Riva, M. A., et al. (2014). Inducible forebrain-specific ablation of the transcription factor Creb during adulthood induces anxiety but no spatial/contextual learning deficits. Front. Behav. Neurosci. 8:407. doi: 10.3389/FNBEH.2014.00407
Vollenweider, F. X., and Preller, K. H. (2020). Psychedelic drugs: neurobiology and potential for treatment of psychiatric disorders. Nat. Rev. Neurosci. 21, 611–624. doi: 10.1038/S41583-020-0367-2
Vollenweider, F. X., Vollenweider-Scherpenhuyzen, M. F. I., Bäbler, A., Vogel, H., and Hell, D. (1998). Psilocybin induces schizophrenia-like psychosis in humans via a serotonin-2 agonist action. Neuroreport 9, 3897–3902. doi: 10.1097/00001756-199812010-00024
Walters, C. L., and Blendy, J. A. (2001). Different requirements for cAMP response element binding protein in positive and negative reinforcing properties of drugs of abuse. J. Neurosci. 21, 9438–9444.
Weisstaub, N. V., Zhou, M., Lira, A., Lambe, E., González-Maeso, J., Hornung, J.-P., et al. (2006). Cortical 5-HT2A receptor signaling modulates anxiety-like behaviors in mice. Science 313, 536–540. doi: 10.1126/science.1123432
Wiegert, J. S., and Bading, H. (2011). Activity-dependent calcium signaling and ERK-MAP kinases in neurons: a link to structural plasticity of the nucleus and gene transcription regulation. Cell Calcium 49, 296–305. doi: 10.1016/j.ceca.2010.11.009
Wu, G. Y., Deisseroth, K., and Tsien, R. W. (2001). Activity-dependent CREB phosphorylation: convergence of a fast, sensitive calmodulin kinase pathway and a slow, less sensitive mitogen-activated protein kinase pathway. Proc. Natl. Acad. Sci. U.S.A. 98, 2808–2813. doi: 10.1073/pnas.051634198
Keywords: 5-HT2A receptor, cAMP response element binding protein, immediate early gene, BDNF, Arc, serotonergic psychedelic, cortical neuron, CREB deficient mice
Citation: Desouza LA, Benekareddy M, Fanibunda SE, Mohammad F, Janakiraman B, Ghai U, Gur T, Blendy JA and Vaidya VA (2021) The Hallucinogenic Serotonin2A Receptor Agonist, 2,5-Dimethoxy-4-Iodoamphetamine, Promotes cAMP Response Element Binding Protein-Dependent Gene Expression of Specific Plasticity-Associated Genes in the Rodent Neocortex. Front. Mol. Neurosci. 14:790213. doi: 10.3389/fnmol.2021.790213
Received: 06 October 2021; Accepted: 26 November 2021;
Published: 24 December 2021.
Edited by:
Jason D. Shepherd, The University of Utah, United StatesReviewed by:
Hiroyuki Okuno, Kagoshima University, JapanAmy Lasek, University of Illinois Chicago, United States
Copyright © 2021 Desouza, Benekareddy, Fanibunda, Mohammad, Janakiraman, Ghai, Gur, Blendy and Vaidya. This is an open-access article distributed under the terms of the Creative Commons Attribution License (CC BY). The use, distribution or reproduction in other forums is permitted, provided the original author(s) and the copyright owner(s) are credited and that the original publication in this journal is cited, in accordance with accepted academic practice. No use, distribution or reproduction is permitted which does not comply with these terms.
*Correspondence: Vidita A. Vaidya, dnZhaWR5YUB0aWZyLnJlcy5pbg==
†These authors have contributed equally to this work and share first authorship