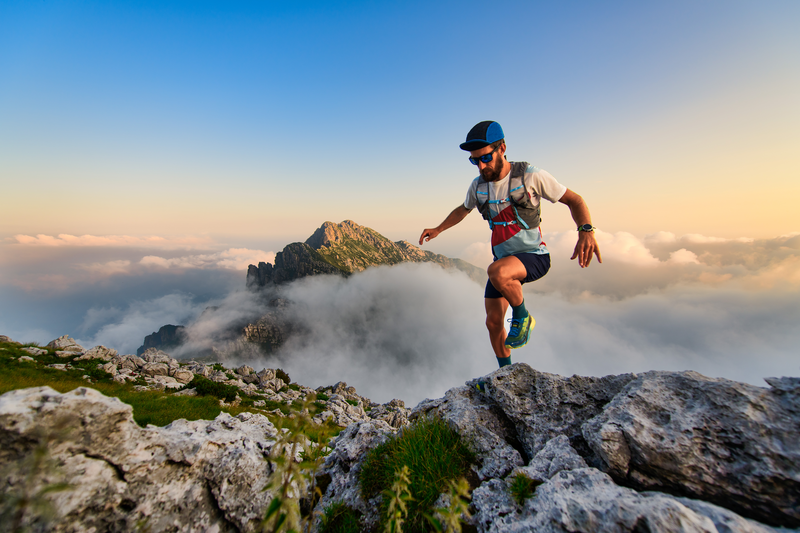
94% of researchers rate our articles as excellent or good
Learn more about the work of our research integrity team to safeguard the quality of each article we publish.
Find out more
EDITORIAL article
Front. Mol. Neurosci. , 11 February 2021
Sec. Methods and Model Organisms
Volume 14 - 2021 | https://doi.org/10.3389/fnmol.2021.636476
This article is part of the Research Topic Tropism, Mapping, Modeling, or Therapy Using Canine Adenovirus Type 2 (CAV-2) Vectors in the CNS View all 11 articles
Editorial on the Research Topic
Tropism, Mapping, Modeling, or Therapy Using Canine Adenovirus Type 2 (CAV-2) Vectors in the CNS
This Frontiers in Molecular Neuroscience Research Topic addresses tropism, mapping, modeling, and therapy using canine adenovirus (CAV-2) vectors in the CNS. Genetic modification of cells in the brain or spinal cord has undergone a revolution in the last decade, both in terms of furthering our understanding of brain structure/function and for therapeutic reasons. The current collection of viral vectors gives scientists a multifunctional kit to target neurons in many brain structures. Approximately 20 years ago, we demonstrated the capacity of CAV-2 vectors to preferentially infect neurons and their high retrograde transport capacity (Soudais et al., 2001). Richard Palmiter's lab was one of the first to take advantage of CAV-2 vector retrograde transport and addressed the physiological functions of the substantia nigra (Hnasko et al., 2005, 2006). The evolution of gene transfer vectors for the CNS has not abated. In this Research Topic, our goal was to provide a readily accessible collection of reviews and primary studies that epitomize the advantages and drawbacks of CAV-2 vectors in the mammalian CNS.
For some neuroscientists, the idiosyncrasies of each vector platform are confusing because the best choice will depend upon their unique question(s). The first question is likely “Which vector is best to infect neurons?” While adenoviral vectors can use numerous cell surface molecules to eventually infect cells (Arnberg, 2012), most of the >200 types have not been tested in the CNS. The uniqueness with CAV-2 is that for all intents and purposes it depends only on a cell adhesion molecule called “coxsackievirus and adenovirus receptor” (CAR) (Soudais et al., 2001, Salinas et al., 2009). Fortunately, CAR is highly conserved, and all mammals appear to express CAR on neurons in the brain parenchyma (Wehbi et al.). CAV-2 vectors have been used efficiently in rodents (Morceau et al.; Martel et al.; Cerpa et al.; Kakava-Georgiadou et al.), dogs (Cubizolle et al., 2014), and non-human primates (NHP) (di Caudo et al., reviewed by Lasbleiz et al.). Not only are CAV-2 vectors efficient but they also appear to drive highly selective expression, which is sometimes a concern with AAV-based conditional constructs with leakage of gene expression, prompting the need to either work out the optimal virus titration (Morceau et al.) or rely on CAV-2 vectors and transgenic lines (Cerpa et al.).
The second question is likely to be— “Which vector has the capacity to harbor my expression cassette of interest?” E1/E3-deleted CAV-2 vectors have a cloning capacity >7 kbp, while helper-dependent CAV-2 vectors (HD-CAV2) have a cloning capacity up to 36 kbp (del Rio et al.). This characteristic sets CAV-2 vectors apart from others (except HSV vectors, which have a theoretical capacity of 150 kb). By exploiting this characteristic, di Caudo et al. and Mestre-Francés et al. (2019) created vectors harboring a leucine-rich repeat kinase 2 (LRRK2) cassette containing a point mutation (G2019S) to produce genetic NHP models of Parkinson's disease (reviewed by Lasbleiz et al.).
The third issue typically concerns the type of neuron that needs to be targeted. CAR is present mainly but not exclusively in the axons (Wehbi et al.) of different neuronal populations (reviewed by Lavoie and Liu), allowing one to target different types of neurons in different areas of the CNS in rodents and the peripheral nervous system in primates (Bohlen et al.). If one combines the presence of CAR and a specific promoter, transgene expression can be targeted to cholinergic interneurons (Martel et al.) or neurons in the locus coeruleus (Hirschberg et al., 2017; Xiang et al., 2019; Hayat et al., 2020). Furthermore, Lavoie and Liu have compiled a list of possible neuronal subtypes (glutamatergic, dopaminergic, catecholaminergic, serotoninergic, oxytonic, and GABAergic) that have been effectively transduced by CAV-2 vectors and they also point out that the lack of infection of some projecting neuron types is possible.
The ability of the neuronal pre-synapse to endocytose CAV-2 particles and confuse them for cargo destined for transport to the soma (retrograde transport) has provided neuroscientists with one of the first efficient vectors to address neuronal circuitry. How the 100 billion neurons, via the trillions of synapses, in a human brain interact, and how a given subset influences behavior may never be understood. However, this daunting task has not stopped many from trying to use less complex systems to create a rough blueprint as a starting point. In addition to circuitry, the advent of opto- and chemogenetics now allows one to address the function of selected neuronal populations (Cerpa et al.).
Finally, CAV-2 vectors (both E1/E3-deleted and HD) are also capable of long-term expression (del Rio et al.; Hirschberg et al., 2017). A study of the transcriptional pathways affected by HD-CAV2 demonstrated that there was a modest modulation of genes involved in the immune response, intracellular trafficking, and transcriptional regulation (reviewed by del Rio et al.).
In summary, the future of neuron-targeted gene transfer has many options—and in particular, CAV-2 vectors have many favorable characteristics.
IGD-R, MW, MA, and EK wrote the manuscript, edited, and approved of the manuscript.
Work in Andrews lab is funded by the Biotechnology and Biological Sciences Research Council and the International Foundation for Research in Paraplegia. Work in the Kremer lab was support by the LabEx EpiGenMed, an Investissements d'avenir program, Université de Montpellier, the Agence Nationale de la Recherche (ANR), ERA-Net (E-Rare) Joint Transnational Call, the Fondation pour la Recherche Médicale/Fondation Alzheimer's, and l'Institut de Génétique Moléculaire de Montpellier. The funders had no role in study design, data collection and analysis, decision to publish, or preparation of the manuscript.
The authors declare that the research was conducted in the absence of any commercial or financial relationships that could be construed as a potential conflict of interest.
We thank all the authors that contributed to this Research Topic.
Arnberg, N. (2012). Adenovirus receptors: implications for targeting of viral vectors. Trends Pharmacol. Sci. 33, 442–448. doi: 10.1016/j.tips.2012.04.005
Cubizolle, A., Serratrice, N., Skander, N., Colle, M. A., Ibanes, S., Gennetier, A., et al. (2014). Corrective GUSB transfer to the canine mucopolysaccharidosis VII brain. Mol. Ther. 22, 762–773. doi: 10.1038/mt.2013.283
Hayat, H., Regev, N., Matosevich, N., Sales, A., Paredes-Rodriguez, E., Krom, A. J., et al. (2020). Locus coeruleus norepinephrine activity mediates sensory-evoked awakenings from sleep. Sci. Adv. 6:eaaz4232. doi: 10.1126/sciadv.aaz4232
Hirschberg, S., Li, Y., Randall, A., Kremer, E. J., and Pickering, A. E. (2017). Functional dichotomy in spinal- vs prefrontal-projecting locus coeruleus modules splits descending noradrenergic analgesia from ascending aversion and anxiety in rats. Elife. 6:e29808. doi: 10.7554/eLife.29808
Hnasko, T. S., Perez, F. A., Scouras, A. D., Stoll, E. A., Gale, S. D., Luquet, S., et al. (2006). Cre recombinase-mediated restoration of nigrostriatal dopamine in dopamine-deficient mice reverses hypophagia and bradykinesia. Proc. Natl. Acad. Sci. U.S.A. 103, 8858–8863. doi: 10.1073/pnas.0603081103
Hnasko, T. S., Sotak, B. N., and Palmiter, R. D. (2005). Morphine reward in dopamine-deficient mice. Nature 438, 854–857. doi: 10.1038/nature04172
Mestre-Francés, N., Serratrice, N., Gennetier, A., Devau, G., Cobo, S., Trouche, S., et al. (2019). Exogenous LRRK2G2019S induces parkinsonian-like pathology in a nonhuman primate. JCI Insight 3:98202. doi: 10.1172/jci.insight.98202
Salinas, S., Bilsland, L. G., Henaff, D., Weston, A. E., Keriel, A., Schiavo, G., et al. (2009). CAR-associated vesicular transport of an adenovirus in motor neuron axons. PLoS Pathog. 5:e1000442. doi: 10.1371/journal.ppat.1000442
Soudais, C., Laplace-Builhe, C., Kissa, K., and Kremer, E. J. (2001). Preferential transduction of neurons by canine adenovirus vectors and their efficient retrograde transport in vivo. FASEB J. 15, 2283–2285. doi: 10.1096/fj.01-0321fje
Keywords: CAV-2, neural circuit tracing, gene transfer, Parkinson's disease, coxsackievirus adenovirus receptor, retrograde transport, DREADDs, optogenetics
Citation: Dopeso-Reyes IG, Wolff M, Andrews MR and Kremer EJ (2021) Editorial: Tropism, Mapping, Modeling, or Therapy Using Canine Adenovirus Type 2 (CAV-2) Vectors in the CNS. Front. Mol. Neurosci. 14:636476. doi: 10.3389/fnmol.2021.636476
Received: 01 December 2020; Accepted: 05 January 2021;
Published: 11 February 2021.
Edited by:
Ildikó Rácz, University Hospital Bonn, GermanyReviewed by:
Mitsuhiro Hashimoto, Fukushima Medical University, JapanCopyright © 2021 Dopeso-Reyes, Wolff, Andrews and Kremer. This is an open-access article distributed under the terms of the Creative Commons Attribution License (CC BY). The use, distribution or reproduction in other forums is permitted, provided the original author(s) and the copyright owner(s) are credited and that the original publication in this journal is cited, in accordance with accepted academic practice. No use, distribution or reproduction is permitted which does not comply with these terms.
*Correspondence: Iria Gonzalez Dopeso-Reyes, aXJpYS5nb256YWxlei1kb3Blc28tcmV5ZXNAaWdtbS5jbnJzLmZy; Mathieu Wolff, bWF0aGlldS53b2xmZkB1LWJvcmRlYXV4LmZy; Melissa R. Andrews, bS5yLmFuZHJld3NAc290b24uYWMudWs=; Eric J. Kremer, ZXJpYy5rcmVtZXJAaWdtbS5jbnJzLmZy
Disclaimer: All claims expressed in this article are solely those of the authors and do not necessarily represent those of their affiliated organizations, or those of the publisher, the editors and the reviewers. Any product that may be evaluated in this article or claim that may be made by its manufacturer is not guaranteed or endorsed by the publisher.
Research integrity at Frontiers
Learn more about the work of our research integrity team to safeguard the quality of each article we publish.