- 1Laboratory of Forensic and Biomedical Information, Chongqing Medical University, Chongqing, China
- 2Department of Gastroenterology, Second Affiliated Hospital of Chongqing Medical University, Chongqing, China
Ischemic stroke is one of the leading causes of global mortality and disability. It is a multi-factorial disease involving multiple factors, and gene dysregulation is considered as the major molecular mechanisms underlying disease progression. Angiogenesis can promote collateral circulation, which helps the restoration of blood supply in the ischemic area and reduces ischemic necrosis following ischemic injury. Aberrant expression of long non-coding RNAs (lncRNAs) in ischemic stroke is associated with various biological functions of endothelial cells and serves essential roles on the angiogenesis of ischemic stroke. The key roles of lncRNAs on angiogenesis suggest their potential as novel therapeutic targets for future diagnosis and treatment. This review elucidates the detailed regulatory functions of lncRNAs on angiogenesis following ischemic stroke through numerous mechanisms, such as interaction with target microRNAs, downstream signaling pathways and target molecules.
Introduction
Stroke is a type of acute cerebral vascular disease with high disability and mortality rate. It is the second leading cause of death and the third leading cause of disability worldwide (Mozaffarian et al., 2016; Feigin et al., 2017). The age-adjusted stroke mortality rate was 35/100,000, and there were 16.9 million cases of incident first stroke in 2010. Recently, the incidence of stroke increases rapidly and it is the leading cause of death in China, as over two million new cases were diagnosed in 2017 (Feigin et al., 2014; Wu S. et al., 2019). Ischemic stroke accounts for ~80–85% of all acute strokes, and its prevalence remarkably increases with advancing age (Randolph, 2016). Ischemic stroke is caused by cerebral artery occlusion, which reduces cerebral blood flow and causes neuronal cell death and brain dysfunction. The quality of life of patients can be severely affected by brain injury induced neurological deficits, leading to growing financial burden of health care.
It is well-established that early recanalization of blood vessels contributes to the restoration of blood supply in ischemic zone, and reduction of cerebral infarction area is the most effective treatment for acute ischemic brain injury. Angiogenesis following ischemic brain injury serves essential roles in clinical practice. Recent studies have indicated that enhancement of angiogenesis is a promising strategy for the treatment of ischemic stroke (Sun et al., 2020). Further understanding the detailed molecular mechanisms of post-ischemic stroke angiogenesis will facilitate the development of therapeutics.
Long non-coding RNAs (lncRNAs) are endogenous non-coding RNAs, which are >200 nucleotides in length but lack of open-reading frame. LncRNAs have been identified as key factors in regulating the expression and function of protein-coding genes through numerous mechanisms. LncRNAs with complex secondary and tertiary structure serve essential roles on epigenetics, transcription, and post-transcriptional modification via binding with target DNA, RNA or protein (Mousavi et al., 2013; Toiyama et al., 2014). LncRNAs are key regulators in biological processes such as cell proliferation, differentiation, apoptosis, autophagy, and angiogenesis, and they are involved in the pathogenesis of various types of diseases including tumors (Takahashi et al., 2020; Zhang Y. et al., 2020), cardiovascular disorders (Mao et al., 2019; Bian et al., 2020), and nervous system diseases (Liang et al., 2020). LncRNAs are considered as novel regulators in the pathogenesis of ischemic stroke. A recent study has suggested that lncRNAs are involved in the regulation of post-ischemic stroke gene expression (Yan Y. et al., 2020). Clinical research has also revealed that dysregulation of lncRNAs could be an essential mechanism in ischemic stroke (Huang J. et al., 2019). Therefore, lncRNAs could be novel therapeutic candidates in future targeted therapy.
However, the detailed roles and underlying mechanisms of lncRNAs on the pathogenesis of ischemic stroke remain largely unknown. Therefore, in order to explore putative therapeutic targets, this review highlights the importance of specific lncRNAs in angiogenesis following ischemic stroke.
Biological Functions of LncRNAs
LncRNAs regulate gene expression through affecting epigenetics, transcription and translation. They serve essential physiological and pathological roles and are involved in numerous signaling pathways during the development of various diseases. LncRNAs are also considered as potential biomarkers. A previous study has indicated that H19 can target ACP5 protein directly and is a putative risk factor of ischemic stroke (Huang Y. et al., 2019). According to the subcellular distribution of lncRNAs, they are localized within the nucleus or cytoplasm, and previous studies have revealed their regulatory functions on gene expression at both transcriptional and post-transcriptional levels.
LncRNAs are involved in the regulation at the transcriptional level through acting as chromatin remodelers and transcriptional coactivators. Nuclear lncRNAs can regulate the functions of chromosomes by recruiting chromatin modifying complexes to specific sites on chromosomes, which consequently affects histone methylation and deacetylation; alternatively, lncRNAs can bind to regulatory transcription factors and modulate the transcription of target genes (Zhang C. L. et al., 2017; Lei et al., 2019; Zhang L. et al., 2019). At post-transcriptional level, cytoplasmic lncRNAs can regulate the expression of target gene indirectly through the downstream miRNAs (Zhuang et al., 2019). LncRNAs can also affect pre-mRNA splicing, mRNA translation and localization. For instance, some lncRNAs contain the complementary binding sites of target miRNAs and function as competing endogenous RNA (ceRNA) through binding certain miRNA competitively, which results in the upregulation of target mRNA (Cao et al., 2020). In addition, due to the existence of lncRNAs base-pairing with pre-mRNAs, the splicing of pre-mRNAs could be prevented, which subsequently affects the transcription of target genes (Szcześniak and Makałowska, 2016). Furthermore, certain lncRNAs are involved in the pathophysiology of diseases through binding to mRNA directly, which influences the degradation and translation of mRNAs (Wu H. et al., 2019).
Regulatory Mechanisms of LncRNAs in Ischemic Stroke
LncRNAs are a group of non-coding RNAs involved in numerous biological processes and they are also considered as regulatory factors in ischemic stroke. In the human genome, ~40% of differentially expressed lncRNAs were detected in the brain and localized in specific cell types and subcellular structures in neural regions, which serve essential roles during brain injury (Derrien et al., 2012; Wang et al., 2017b). A recent study revealed significant alterations of lncRNAs in the brain after cerebral ischemia, suggesting the important physiological and pathological roles of lncRNAs on initiating endothelial responses to ischemic stimuli at transcriptional and translational level. Differentially expressed lncRNAs were detected in blood samples from patients with acute ischemic stroke, rat models of middle cerebral artery occlusion (MCAO), and oxygen/glucose deprivation (OGD) cells (Guo et al., 2018; Barangi et al., 2019; Zhu et al., 2019). The abovementioned lncRNAs are involved in various biological processes of ischemic stroke including cell proliferation, apoptosis (Zhang L. et al., 2020), oxidative stress, inflammation (Zhang and Zhang, 2020) and autophagy (Luo et al., 2020). Previous studies suggested that lncRNAs exert various regulatory functions after stroke. For example, they act as miRNA sponges competing with mRNA for miRNA binding. Moreover, lncRNAs could also regulate the expression of target genes directly through numerous signaling pathways.
Regulation of Neurogenesis
Previous studies have revealed that altered levels of lncRNAs were associated with neurogenesis after stroke. A recent study indicated that N1LR inhibited neuronal apoptosis after ischemic stroke through inactivating p53 (Wu et al., 2017). Similarly, MEG3 interacted with p53 to mediate neuronal death (Yan et al., 2016). Furthermore, knockdown of MEG3 could suppress neuronal death by targeting the miR-21/PDCD4 signaling pathway (Yan et al., 2017). MEG3 induced autophagy and neuronal cell death via the miR-378/GRB2 axis, which subsequently suppressed the activation of Akt/mTOR signaling (Luo et al., 2020).
A previous study also indicated that MALAT1 promoted neuronal cell death and suppressed autophagy through targeting miR-30a in ischemic stroke (Guo et al., 2017). In consistence with these findings, H19 induced neuronal death by activating autophagy in ischemic stroke (Wang et al., 2017a). In addition, GAS5 inhibited cell death and suppressed neuronal survival through targeting the miR-137/Notch1 signaling pathway (Chen et al., 2018). Furthermore, SNHG14 promoted neuronal apoptosis through microglia activation via regulating the miR-145-5p/PLA2G4A axis in cerebral infarction (Qi et al., 2017).
Regulation of Inflammation
Proinflammatory cytokines were released after ischemic brain injury, consequently activating NF-κB pathway that is involved in inflammatory responses. Nespas inhibited proinflammatory cytokine production and suppressed NF-κB signaling after ischemic stroke through TAK1 activation (Deng Y. et al., 2019). Gm4419 promoted neuroinflammation by inducing IκB phosphorylation and NF-κB signaling activation after ischemic stroke, and the levels of tumor necrosis factor-α (TNF-α), interleukin-1β (IL-1β) and IL-6 were increased (Wen et al., 2017). A previous study also indicated that H19 promoted neuroinflammation by M1 microglial polarization, which lead to increased production of TNF-α and IL-1β in ischemic stroke (Wang et al., 2017b).
Regulation of Angiogenesis
Previous studies have suggested that lncRNAs serve essential roles on the regulation of endothelial cell survival, vascular integrity and angiogenesis in stroke. In this review, the mechanisms underlying lncRNA-modulated angiogenesis are summerized.
Recent studies have revealed altered expression of lncRNAs in ischemic stroke (He et al., 2018; Ruan et al., 2020). Recently, lncRNA-mediated gene regulation has been intensively investigated. LncRNAs can regulate gene expression in ischemic stroke through affecting mRNA stability and epigenetic modifications. They function as decoys, scaffolds and enhancer RNAs of corresponding targets to regulate their functions. Furthermore, lncRNAs can interact with DNA and RNA by modulating pre-mRNA splicing or acting as miRNA sponge, resulting in impaired miRNA expression and functions. Numerous lncRNAs are associated with angiogenesis after ischemic stroke through affecting the transcription and translation (Figure 1, Table 1).
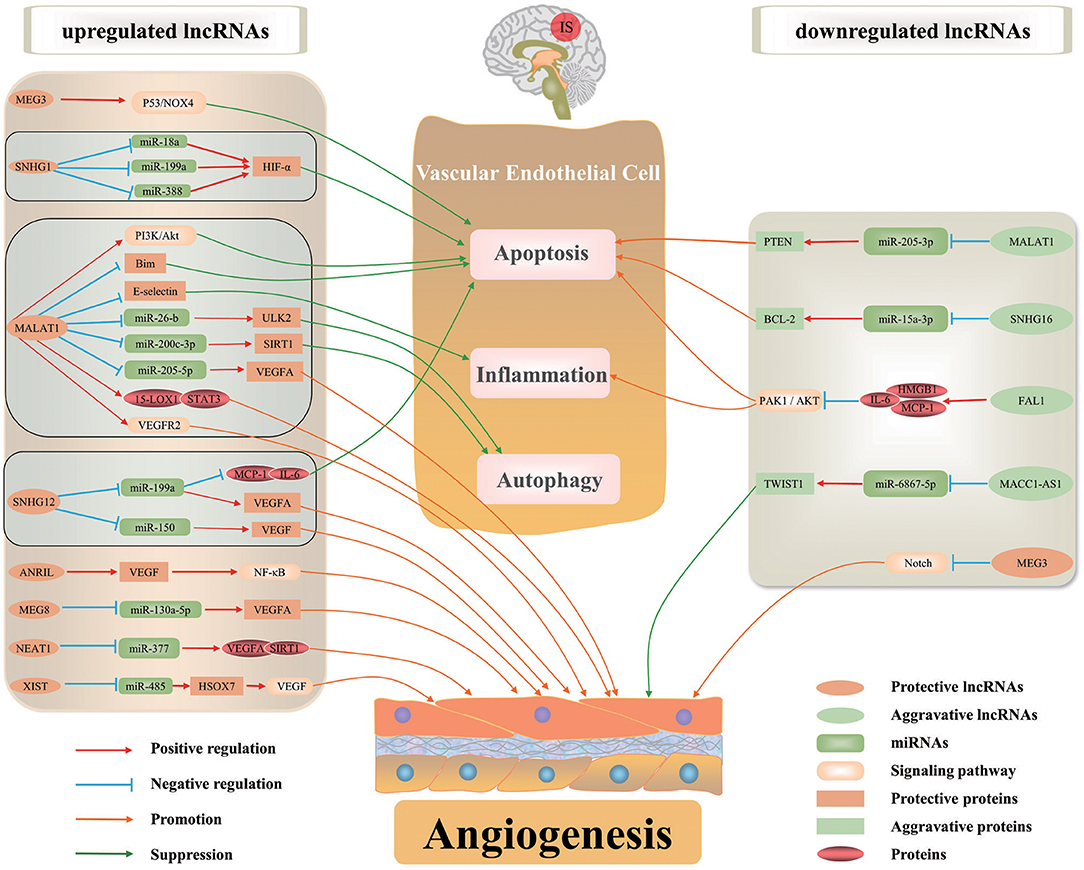
Figure 1. The regulatory mechanisms of lncRNAs in ischemic stroke-induced angiogenesis. Some lncRNAs serve essential roles on angiogenesis after ischemic stroke by regulating inflammation, autophagy and apoptosis of BMECs, or directly regulating angiogenesis-associated proteins/signaling pathways to maintain vascular function and integrity. Certain lncRNA may regulate the expression of multiple target genes in various pathways involved in angiogenesis.
Brain Microvascular Endothelial Cells (BMECs)
BMECs are major structural and functional elements of brain microvasculature, which serve key roles on maintaining the integrity of the blood-brain barrier (BBB) and brain homeostasis by responding to the changes in cellular microenvironment dynamically. Ischemic stroke could cause structural and functional injuries to cerebrovascular endothelial cells, consequently increasing cerebrovascular permeability and damaging the BBB, which can result in neuroinflammation and neuronal damage. In addition, ischemic stroke results in BMECs injury and death. During cerebral ischemia, BMECs are stimulated by inflammatory mediators, which activates proinflammatory responses.
Angiogenesis after ischemic stroke promotes collateral circulation and restores blood supply in the ischemic area, subsequently reducing ischemic necrosis caused by ischemic injury and reversing the nerve damage (Li L. et al., 2017). LncRNAs were abundantly expressed in vascular endothelial cells and involved in endothelial biology and physiological functions.
Zhang et al. (2016) evaluated the expression levels of lncRNAs in primary BMECs after OGD using RNA-sequencing (RNA-seq) and differentially expressed lncRNAs were detected, including 147 up-regulated and 70 down-regulated lncRNAs. Among them, MALAT1 and SNHG12 are the most up-regulated lncRNAs. Highly conserved binding sites of transcription factors on the promoter region of lncRNAs reveals potential transcriptional regulation of lncRNAs, which could be associated with angiogenesis and serve essential roles on the response to ischemic stimuli; however, the detailed functions of lncRNAs in cerebral vascular endothelial biology is unclear.
A previous study indicated that cerebral vascular damage was aggravated in MALAT1 knock-out (KO) mice, where larger cerebral infarct volume and more severe neurological deficits were detected in response to ischemic insults (Zhang X. et al., 2017). Activation of MALAT1 was able to protect cerebral microvascular endothelial cells from ischemic injury (Yang et al., 2018). Additionally, SNHG12 was significantly up-regulated in microvascular endothelial cells under OGD/R conditions. Overexpression of SNHG12 inhibited brain microvascular endothelial cell death and inflammatory response, but promoted angiogenesis following OGD/R. Furthermore, SNHG12 suppressed brain microvascular endothelial cell injury by targeting miR-199a (Long et al., 2018). In addition, MACC1-AS1 was down-regulated in hypoxia-induced human brain microvascular endothelial cells (HBMECs). Overexpressed MACC1-AS1 reduced cell apoptosis and oxidative stress, while promoting cell proliferation, migration, and angiogenesis; meanwhile, cell permeability was decreased, which can affect cell barrier function. Moreover, MACC1-AS1 exert protective function of anti-apoptosis, pro-angiogenesis and anti-HBMECs injury via targeting the miR-6867-5p/TWIST1 signaling (Yan G. et al., 2020). Overexpression of FAL1 could reverse OGD/R-induced injury and cell death in brain microvascular endothelial cells through ameliorating oxidative stress, suppressing the secretion of pro-inflammatory cytokines and activating the PAK1/AKT signaling pathway (Gao M. et al., 2020).
BMECs and Inflammation
Accumulating evidence revealed the importance of inflammation in the pathogenesis of stroke, which involves the networking of numerous inflammatory cells and cytokines. Multiple inflammatory cells and cytokines participate in various stages of ischemic stroke. Aberrantly expressed lncRNAs in ischemic stroke are associated with the production of inflammatory factors and activation of various signaling pathways. Cerebral endothelial cells are stimulated by inflammatory mediators produced by neuronal cells in ischemic brains, which triggers proinflammatory activation. Activated cerebral endothelium could release inflammatory factors such as IL-1β, IL-6, MCP-1 and induce the activation of NF-κB pathway, consequently leading to cerebral ischemic injury (Shekhar et al., 2018). Moreover, OGD/R could aggravate inflammation by enhancing the production of proinflammatory cytokines. Additionally, lncRNAs could regulate cerebrovascular function in ischemic stroke through targeting apoptotic factors.
MALAT1 could bind to IL-6, E-selectin and MCP-1, which prevent OGD/R-induced inflammatory response in ischemic stroke by reducing the production of proinflammatory cytokines (Zhang X. et al., 2017; Yang et al., 2018). For instance, SNHG12 was up-regulated under OGD/R conditions in microvascular endothelial cells of mouse brain, which decreased the mRNA and protein levels of proinflammatory cytokines E-selectin, MCP-1 and IL-6, subsequently inhibiting the inflammatory response. Furthermore, SNHG12 exerts anti-inflammatory function in brain microvasculature cells after OGD/R by reducing ischemic cerebral vascular injury and parenchymal damage (Long et al., 2018). In addition, FAL1 was significantly down-regulated in OGD/R-stimulated HBMECs, and overexpression of FAL1 ameliorated OGD/R-induced oxidative stress by suppressing the production of IL-6, MCP-1 and HMGB-1. FAL1 might serve essential roles on regulating OGD/R-induced inflammation in HBMECs (Gao M. et al., 2020). LncRNAs were involved in the inflammatory response in BMECs, which might affect brain endothelial cell damage through modulating the release of inflammatory factors in BMECs.
BMECs and Autophagy
Autophagy is a lysosome-dependent homeostatic process. As an endogenous defense mechanism, it might also inhibit cell death. It has been well-established that lncRNAs are involved in the pathogenesis of ischemic stroke through regulating cell autophagy, which protects BMECs from ischemic injury. Autophagy can be activated by OGD/R as a defense response to protect BMECs against OGD-induced injury. Furthermore, lncRNAs are novel regulators involved in autophagy, and they can affect autophagy through downstream miRNAs; however, the underlying molecular mechanisms remain unclear.
Li Z. et al. (2017) revealed that MALAT1 was up-regulated in BMECs following OGD/R, which activated autophagy and promoted cell survival through down-regulating miR-26b and up-regulating ULK2. Wang S. et al. (2019) suggested that MALAT1 stimulated autophagy activation in BMECs via the miR-200c-3p/SIRT1 signaling, subsequently down-regulating p62 and inhibiting cell death. At present, the molecular mechanisms of autophagy in ischemic stroke are still unclear. Therefore, the detailed mechanisms of lncRNA-modulated regulatory functions on autophagy in BMECs under OGD/R conditions require further investigation.
BMECs and Apoptosis
Dysfunction in the blood-brain barrier caused by the apoptosis of microvascular endothelial cells is one of the key pathological features of ischemic stroke, which contributes to secondary damage and poor prognosis in patients. LncRNAs might be involved in the apoptosis of BMECs through targeting apoptotic factors or various signaling pathways associated with the development of stroke. A recent study indicated that OGD/R down-regulated FAL1 and further reduced the phosphorylation of PAK1 and AKT, consequently promoting OGD/R-induced cell death. FAL1 could serve essential roles on regulating OGD/R-induced endothelial damage in HBMECs (Gao M. et al., 2020). Xin and Jiang (2017) revealed that the levels of MALAT1 in cells under OGD-R conditions were significantly increased, but down-regulation of MALAT1 was detected as oxygen levels increased. Additionally, MALAT1 suppressed OGD/R-induced apoptosis and the activity of caspase-3 in HBMECs by activating the PI3K/Akt pathway. These findings suggested that MALAT1 could protect HBMECs against OGD/R-induced endothelial damage. Furthermore, a previous study indicated that the expression of MALAT1 was down-regulated in OGD-induced apoptosis and negatively correlated with the expression of miR-205-3p. Subsequently, down-regulation of MALAT1 induced a reduction in PTEN levels, which further promoted OGD-induced apoptosis (Gao and Wang, 2020).
However, a recent study also revealed OGD-induced up-regulation of MALAT1 and down-regulation of miR-126 in HBMECs. In addition, knockdown of MALAT1 activated the PI3K/Akt pathway, increased the expression of phosphorylated PI3K/Akt and inhibited cell apoptosis. Moreover, MALAT1 down-regulated the expression of miR-126, which subsequently suppressed the proliferation and enhanced the apoptosis through inactivating the PI3K/AKT pathway. Therefore, up-regulation of MALAT1 could promote the development of ischemic stroke (Zhang L. et al., 2020). Another study indicated that MALAT1 could induce cell apoptosis. MALAT1 activated p53 signaling through targeting MDM2, consequently promoting the progression of ischemic stroke (Zhang T. et al., 2018). Furthermore, a previous study suggested that the expression of SNHG1 was remarkably increased in BMECs under OGD conditions and reversely correlated with the expression of miR-18a. Additionally, SNHG1 acted as miR-18a sponge and regulated the expression of its endogenous target HIF-1α, which inhibited cell apoptosis and promoted the survival of BMECs via the HIF-1α/VEGF signaling (Zhang L. et al., 2018). Another study also revealed that the levels of SNHG1 and miR-338 were up-regulated in OGD-induced BMECs. Knockdown of SNHG1 reduced the expression of HIF-1α/VEGF-A and promote the apoptosis of BMECs through targeting miR-338. As a result, OGD-induced injury in BMECs was aggravated. Therefore, SNHG1 exerted protective function against OGD-induced damage in BMECs via sponging miR-338 and up-regulate the HIF-1α/VEGF-A signaling (Yang and Zi, 2019). Additionally, the expression of SNHG12 was significantly increased in BMECs under OGD conditions, which inhibited cell death through targeting miR-199a (Long et al., 2018). Furthermore, SNHG16 suppressed OGD-induced apoptosis in HBMECs via the miR-15a-5p/Bcl-2 axis (Teng et al., 2020). In addition, up-regulation of Rmst inhibited cell proliferation/migration and promoted the apoptosis of OGD-induced cells through targeting miR-150 (Qiao et al., 2020). Moreover, MIAT was overexpressed in OGD-treated BMECs, and it could affect the injury of BMECs after cerebral ischemia through targeting the miR-204-5p/HMGB1 signaling (Deng W. et al., 2020). Taken all together, previous studies revealed the involvement of numerous lncRNAs in the apoptosis of BMECs after ischemic stroke.
BMECs and Angiogenesis
The key steps of angiogenesis include the proliferation and migration of blood vascular endothelial cells. The Notch and VEGF signaling pathways are involved in angiogenesis, which could regulate cell differentiation and migration, contributing to the formation of blood vessels. Ischemic stroke promoted the proliferation and migration of BMECs, subsequently activating angiogenesis. Angiogenesis is a complex process that involves the regulation of numerous genes. LncRNAs are novel key regulators associated with endothelial dysfunction after ischemic stroke. Thus, lncRNAs could be involved in angiogenesis of endothelial cells by maintaining vascular function and integrity through various pathways.
Michalik et al. (2014) revealed that silenced MALAT1 could suppress the proliferation of vascular endothelial cells, suggesting that MALAT1 was able to promote endothelial cell growth and might serve essential roles on protecting cerebral microvasculature against ischemic brain injury. Furthermore, lncRNAs regulated the expression of proximal protein, which are major angiogenic molecules, subsequently affecting the process of angiogenesis. In addition, MALAT1 could enhance angiogenesis by binding to VEGFR2 (Zhang X. et al., 2018). Wang C. et al. (2019) also indicated that OGD/R increased the expression levels of MALAT1, 15-LOX1, VEGF and pSTAT3. Knockdown of MALAT1 inhibited the proliferation and migration of BMECs, suggesting that MALAT1 could promote angiogenesis through activating the 15-LOX1/STAT3 axis.
Furthermore, lncRNAs might be involved in angiogenesis through numerous signaling pathways. A previous study indicated that HOTTIP promoted the growth of BMECs via activating the Wnt/β-catenin axis (Liao et al., 2018). Zhang B. et al. (2017) also suggested that the expression of ANRIL was significantly up-regulated in the infarcted tissues of MCAO rat model. In addition, overexpression of ANRIL enhanced the expression of VEGF and promoted the angiogenesis by activating the NF-κB signaling.
LncRNAs could also function as ceRNAs of miRNAs to regulate the expression of target mRNAs, further affecting the process of angiogenesis. For instance, MEG3 is a key regulator in the proliferation and angiogenesis of BMECs by acting as miR-9 sponge (He et al., 2017). Moreover, MEG8 was up-regulated in OGD-treated BMECs. Silenced MEG8 inhibited the viability, migration and angiogenesis of BMECs via targeting miR-130a-5p. These findings suggested that MEG8 could protect BMECs from OGD-induced injury by promoting the angiogenesis following ischemic stroke through the miR-130a-5p/VEGFA axis (Sui et al., 2020). In addition, the expression of NEAT1 was elevated in BMECs under OGD conditions, which promoted the angiogenesis in BMECs by targeting the miR-377/SIRT1/VEGFA axis (Zhou et al., 2019). Both SNGH1 and SNGH12 were remarkably up-regulated in BMECs under OGD/R conditions, which promoted the survival and migration of BMECs. SNGH1 and SNGH12 exert their functions through targeting miR-199a and up-regulating their downstream molecules VEGFA and FGFb (Long et al., 2018; Wang Z. et al., 2018). Similarly, another study has suggested that up-regulation of SNHG12 could facilitate the restoration of neurological function in the infarct boundary zone of MCAO mice via the miR-150/VEGF signaling. Infarct volume was reduced, and cell growth/angiogenesis was enhanced by up-regulated SNHG12, suggesting that SNHG12 could promote angiogenesis following ischemic stroke (Zhao et al., 2018). Furthermore, XIST induced cell proliferation, migration and angiogenesis in BMECs via the miR-485/SOX7 axis (Hu et al., 2019). Another study also revealed that MALAT1 expression was increased in OGD/R-induced HBMECs. Up-regulated MALAT1 promoted cell proliferation and served protective roles on angiogenesis by targeting the miR-205-5p/VEGFA signaling in HBMECs under OGD/R conditions (Gao C. et al., 2020).
In summary, these findings indicated that lncRNAs including MALAT1, HOTTIP, ANRIL, MEG3, MEG8, NEAT1, SNHG1, SNHG12 and XIST were involved in post-ischemic stroke angiogenesis. Abovementioned lncRNAs are key regulators in angiogenesis by acting as ceRNA or targeting downstream molecules/pathways directly.
LncRNAs as Novel Diagnostic and Prognostics Biomarkers for Ischemic Stroke
Recently, numerous lncRNAs have been identified using genome-wide analyses, and they are involved in various physiological and pathological processes in ischemic stroke through the regulation at both transcriptional and post-transcriptional levels. Previous findings revealed alteration of lncRNAs expression profiles in peripheral blood samples from patients with ischemic stroke, suggesting that lncRNAs could be novel diagnostic and prognostic biomarkers for this disease. A total of 206 lncRNAs were differentially expressed in the specimens from ischemic stroke patients, including 70 up-regulated and 128 down-regulated lncRNAs (Deng et al., 2018). Xu et al. (2020) revealed differential expression of 1,096 lncRNAs in the exosomes isolated from blood samples of patients with ischemic stroke, including 307 up-regulated and 789 down-regulated lncRNAs. Moreover, lnc-CRKL-2, lnc-NTRK3-4, RPS6KA2-AS1 and lnc-CALM1-7 could be potential biomarkers for the early diagnosis of ischemic stroke. Furthermore, Guo et al. (2018) suggested that 1,250 lncRNAs were differentially expressed in the peripheral blood samples following ischemic stroke. Additionally, ENST00000568297, ENST00000568243 and NR_046084 were identified as novel diagnostic biomarkers for ischemic stroke.
Moreover, the levels of lncRNAs might be associated with the severity of stroke. A previous study was performed on the plasma samples from patients with ischemic stroke, and up-regulated levels of lncRNAs including H19, MIAT, ANRIL, MEG3 and NEAT1 were detected. Up-regulation of abovementioned lncRNAs were positively correlated with the National Institutes of Health Stroke Scale (NIHSS) scores and infarct volume, which could affect the progression of ischemic stroke. Furthermore, the levels of H19 and NEAT1 were associated with the production of inflammatory factors including TNF-α, IL-6, IL-8, and IL-22. In addition, the expression levels of MIAT, ANRIL and NEAT1 were related to the up-regulation of high-sensitivity C-reactive protein. Abovementioned lncRNAs were considered as novel biomarker for the diagnosis of ischemic stroke (Wang et al., 2017b; Zhu et al., 2018; Zhang K. et al., 2019). In contrast, previous findings also revealed that ZFAS1 was significantly down-regulated in patients with large-artery atherosclerosis (LAA) stroke compared to non-LAA stroke group (Wang J. et al., 2019).
Some lncRNAs are associated with the prognosis of patients with ischemic stroke. A previous study indicated that the expression of MIAT was correlated with the therapeutic outcome. MIAT could be an unfavorable indicator of functional recovery, as reduced MIAT levels were detected in patients with favorable outcome, and vice versa. More importantly, the risk of death was higher in high MIAT expression group compared with the patients with low MIAT expression, in whom better prognosis and survival rate were observed (Zhu et al., 2018). In consistence with these findings, Wang et al. (2020) also suggested that MEG3 expression was associated with poor prognosis in patients with ischemic stroke. The risk of death was increased in high MEG3 expression group compared with the control. Furthermore, the expression levels of NEAT1 in patients with recurrence were elevated compared with non-recurrence group. In addition, patients with high NEAT1 expression exhibited shorter RFS compared with low NEAT1 expression group (Li et al., 2020). These studies revealed the expression profiles of lncRNAs in patients with cerebral ischemia, suggesting that lncRNAs could be potential diagnostic and prognostic biomarkers for ischemic stroke.
Previous studies have indicated that microvessel density in the peri-infarct region was increased in patients with ischemic stroke. Angiogenesis was able to promote the recruitment of blood flow and metabolic nutrients into the infarct regions, subsequently facilitating functional recovery. Enhancement of angiogenesis is a promising therapeutic strategy for the treatment of ischemic stroke (Oshikawa et al., 2017; Zhang J. et al., 2019).
At present, the most effective treatment for ischemic stroke is vascular recanalization including interventional treatment and intravenous thrombolysis, which could restore the cerebral blood flow and reduce the infarction size. Until now, recombinant tissue plasminogen activator (rtPA) is the only FDA-approved drug for the treatment of ischemic stroke. However, the use of tPA is limited by its narrow therapeutic window and side effects including high risk of secondary bleeding. Patients with proximal occlusion in large arteries of the brain could be subjected to endovascular interventions. So far, no effective treatment is available for patients whose blood vessels cannot be recanalized at early stage. Therefore, new therapeutic strategies should be developed for patients with ischemic stroke, and enhancement of angiogenesis is a major target in the treatment of this disease.
RNA therapeutic strategies have been developed to intervene the angiogenesis after ischemic stroke. Therapeutic strategies by manipulation of lncRNAs have been evaluated in the treatment of cancer and ulcerative colitis (Velagapudi et al., 2017; Vautrin et al., 2019). RNA therapeutics mainly base on the inhibition or overexpression of certain RNAs. RNA knockdown technique was used to examine the functions of target RNAs. As lncRNAs are located in the nucleus and/or cytoplasm, antisense therapy and RNA interference (RNAi) are commonly used to induce the degradation of lncRNAs (Lennox and Behlke, 2016, 2020). MEG3 was upregulated in cerebral infarction area, and MEG3 knockdown vector was transfected into the striatum of ischemia/reperfusion rat, which reduced infarct size and promoted angiogenesis (Liu et al., 2017). Furthermore, treatment with nano polymer wrapped MEG3 shRNA conjugated with OX26 antibody was able to reduce the ischemic lesion volumes and enhance the angiogenesis in cerebral infarction area (Shen et al., 2018). MALAT1 was upregulated in mouse BMECs after OGD. Antisense LNA GapmeR was used to knockdown MALAT1, which increased cell death, aggravated inflammatory reaction and reduced vascular growth in BMECs (Michalik et al., 2014; Zhang X. et al., 2017). Chemical modifications of lncRNAs could also improve the therapeutic efficacy (Zhang H. et al., 2019). The effects of adeno associated virus (AAV), CRISPR/Cas9, gapmer antisense oligonucleotides (ASOs) remain to be further elucidated.
However, the translational value of most lncRNAs remains unclear. Firstly, in previous studies on the regulatory roles of lncRNAs in ischemic stroke, sample sizes are relatively small, and further large-scale cohort studies are required to confirm the existing findings. LncRNAs are not necessarily conserved in different species. Most studies on lncRNAs were performed on animals, but the effects of putative therapeutics on patients with ischemic stroke should be considered. Secondly, the detailed regulatory functions of lncRNAs should be identified. Better understanding of the mechanisms could improve drug development to reduce off-target effects. Furthermore, the complex nature of brain structure and function resulted in various regulatory mechanisms of lncRNAs in different types of brain cells involved in ischemic stroke, a better understanding of lncRNAs in specific cell types under normal and pathophysiological conditions could improve the specificity of lncRNA-targeted therapies. In addition, multiple lncRNAs are simultaneously involved in the regulation of angiogenesis after ischemic stroke, combinations of lncRNAs with different pathological targets could improve the therapeutic outcome of cerebral ischemia. The accuracy and stability of RNA-based therapeutics should be considered in clinical practice.
Conclusion
Emerging evidence has suggested that lncRNAs serve essential roles in the pathogenesis of ischemic stroke. LncRNAs could regulate the biological bahaviors of BMVECs through their target genes and serve essential roles on cell apoptosis, autophagy, inflammation, and angiogenesis. Furthermore, lncRNAs were closely associated with the prognosis of patients with ischemic stroke, and they could facilitate functional recovery by activating angiogenesis. In addition, lncRNAs are involved in angiogenesis by acting as miRNAs sponges or targeting the downstream signaling pathways/proteins directly. Therefore, lncRNAs might be potential diagnostic and prognostic biomarkers for ischemic stroke. However, only a few lncRNAs involved in angiogenesis have been studied, including MEG3, MEG8, MALAT1, SNHG1, SNHG12, SNHG16, ANRIL, FAL1, XIST, MACC1-AS1, NEAT1, Rmst, and MIAT. Therefore, further investigation is required to elucidate the detailed functions of lncRNAs in ischemic stroke. A better understanding of the underlying mechanisms could provide novel insights on the development of therapeutic targets and diagnostic biomarkers for patients with ischemic stroke.
Author Contributions
LG and SL wrote the manuscript. YX and SD edited the manuscript. All authors contributed to the article and approved the submitted version.
Conflict of Interest
The authors declare that the research was conducted in the absence of any commercial or financial relationships that could be construed as a potential conflict of interest.
References
Barangi, S., Hayes, A. W., Reiter, R., and Karimi, G. (2019). The therapeutic role of long non-coding RNAs in human diseases: a focus on the recent insights into autophagy. Pharmacol. Res. 142, 22–29. doi: 10.1016/j.phrs.2019.02.010
Bian, W., Jing, X., Yang, Z., Shi, Z., Chen, R., Xu, A., et al. (2020). Downregulation of LncRNA NORAD promotes Ox-LDL-induced vascular endothelial cell injury and atherosclerosis. Aging 12, 6385–6400. doi: 10.18632/aging.103034
Cao, D., Liu, M., Duan, R., Tao, Y., Zhou, J., Fang, W., et al. (2020). The lncRNA Malat1 functions as a ceRNA to contribute to berberine-mediated inhibition of HMGB1 by sponging miR-181c-5p in poststroke inflammation. Acta Pharmacol. Sin. 41, 22–33. doi: 10.1038/s41401-019-0284-y
Chen, F., Zhang, L., Wang, E., Zhang, C., and Li, X. (2018). LncRNA GAS5 regulates ischemic stroke as a competing endogenous RNA for miR-137 to regulate the Notch1 signaling pathway. Biochem. Bioph. Res. Co. 496, 184–190. doi: 10.1016/j.bbrc.2018.01.022
Deng, Q., Li, S., Wang, H., Sun, H., Zuo, L., Gu, Z., et al. (2018). Differential long noncoding RNA expressions in peripheral blood mononuclear cells for detection of acute ischemic stroke. Clin. Sci. 132, 1597–1614. doi: 10.1042/CS20180411
Deng, W., Fan, C., Shen, R., Wu, Y., Du, R., and Teng, J. (2020). Long noncoding MIAT acting as a ceRNA to sponge microRNA-204-5p to participate in cerebral microvascular endothelial cell injury after cerebral ischemia through regulating HMGB1. J. Cell. Physiol. 235, 4571–4586. doi: 10.1002/jcp.29334
Deng, Y., Chen, D., Wang, L., Gao, F., Jin, B., Lv, H., et al. (2019). Silencing of long noncoding RNA nespas aggravates microglial cell death and neuroinflammation in ischemic stroke. Stroke 50, 1850–1858. doi: 10.1161/STROKEAHA.118.023376
Derrien, T., Johnson, R., Bussotti, G., Tanzer, A., Djebali, S., Tilgner, H., et al. (2012). The GENCODE v7 catalog of human long noncoding RNAs: analysis of their gene structure, evolution, and expression. Genome Res. 22, 1775–1789. doi: 10.1101/gr.132159.111
Feigin, V. L., Forouzanfar, M. H., Krishnamurthi, R., Mensah, G. A., Connor, M., Bennett, D. A., et al. (2014). Global and regional burden of stroke during 1990-2010: findings from the global burden of disease study 2010. Lancet 383, 245–254. doi: 10.1016/S0140-6736(13)61953-4
Feigin, V. L., Norrving, B., and Mensah, G. A. (2017). Global burden of stroke. Circ. Res. 120, 439–448. doi: 10.1161/CIRCRESAHA.116.308413
Gao, C., Zhang, C., Yang, H., and Hao, Y. (2020). MALAT1 protected the angiogenesis function of human brain microvascular endothelial Cells (HBMECs) under oxygen glucose deprivation/re-oxygenation (OGD/R) challenge by interacting with miR-205-5p/VEGFA pathway. Neuroscience 435, 135–145. doi: 10.1016/j.neuroscience.2020.03.027
Gao, M., Fu, J., and Wang, Y. (2020). The lncRNA FAL1 protects against hypoxia-reoxygenation- induced brain endothelial damages through regulating PAK1. J. Bioenerg. Biomembr. 52, 17–25. doi: 10.1007/s10863-019-09819-2
Gao, Q., and Wang, Y. (2020). Long noncoding RNA MALAT1 regulates apoptosis in ischemic stroke by sponging miR-205-3p and modulating PTEN expression. Am. J. Transl. Res. 12, 2738–2748.
Guo, D., Ma, J., Yan, L., Li, T., Li, Z., Han, X., et al. (2017). Down-regulation of lncrna MALAT1 attenuates neuronal cell death through suppressing Beclin1-dependent autophagy by regulating Mir-30a in cerebral ischemic stroke. Cell. Physiol. Biochem. 43, 182–194. doi: 10.1159/000480337
Guo, X., Yang, J., Liang, B., Shen, T., Yan, Y., Huang, S., et al. (2018). Identification of novel LncRNA biomarkers and construction of LncRNA-related networks in Han Chinese patients with ischemic stroke. Cell. Physiol. Biochem. 50, 2157–2175. doi: 10.1159/000495058
He, C., Yang, W., Yang, J., Ding, J., Li, S., Wu, H., et al. (2017). Long noncoding RNAMEG3 negatively regulates proliferation and angiogenesis in vascular endothelial cells. DNA Cell Biol. 36, 475–481. doi: 10.1089/dna.2017.3682
He, W., Wei, D., Cai, D., Chen, S., Li, S., and Chen, W. (2018). Altered long non-coding RNA transcriptomic profiles in ischemic stroke. Hum. Gene Ther. 29, 719–732. doi: 10.1089/hum.2017.064
Hu, C., Bai, X., Liu, C., and Hu, Z. (2019). Long noncoding RNA XIST participates hypoxia-induced angiogenesis in human brain microvascular endothelial cells through regulating miR-485/SOX7 axis. Am. J. Transl. Res. 11, 6487–6497. doi: 10.1111/micc.12601
Huang, J., Yang, J., Li, J., Chen, Z., Guo, X., Huang, S., et al. (2019). Association of long noncoding RNA H19 polymorphisms with the susceptibility and clinical features of ischemic stroke in southern Chinese Han population. Metab. Brain Dis. 34, 1011–1021. doi: 10.1007/s11011-019-00417-0
Huang, Y., Wang, L., Mao, Y., and Nan, G. (2019). Long noncoding RNA-H19 contributes to atherosclerosis and induces ischemic stroke via the upregulation of acid phosphatase 5. Front. Neurol. 10:32. doi: 10.3389/fneur.2019.00032
Lei, J. J., Li, H. Q., Mo, Z. H., Liu, K. J., Zhu, L. J., Li, C. Y., et al. (2019). Long noncoding RNA CDKN2B-AS1 interacts with transcription factor BCL11A to regulate progression of cerebral infarction through mediating MAP4K1 transcription. FASEB J. 33, 7037–7048. doi: 10.1096/fj.201802252R
Lennox, K. A., and Behlke, M. A. (2016). Cellular localization of long non-coding RNAs affects silencing by RNAi more than by antisense oligonucleotides. Nucleic Acids Res. 44, 863–877. doi: 10.1093/nar/gkv1206
Lennox, K. A., and Behlke, M. A. (2020). Tips for successful lncRNA knockdown using gapmers. Methods Mol. Biol 2176, 121–140. doi: 10.1007/978-1-0716-0771-8_9
Li, L., Wang, M., Mei, Z., Cao, W., Yang, Y., Wang, Y., et al. (2017). lncRNAs HIF1A-AS2 facilitates the up-regulation of HIF-1α by sponging to miR-153-3p, whereby promoting angiogenesis in HUVECs in hypoxia. Biomed. Pharmacother. 96, 165–172. doi: 10.1016/j.biopha.2017.09.113
Li, P., Duan, S., and Fu, A. (2020). Long noncoding RNA NEAT1 correlates with higher disease risk, worse disease condition, decreased miR-124 and miR-125a and predicts poor recurrence-free survival of acute ischemic stroke. J. Clin. Lab. Anal. 34:e23056. doi: 10.1002/jcla.23056
Li, Z., Li, J., and Tang, N. (2017). Long noncoding RNA Malat1 is a potent autophagy inducer protecting brain microvascular endothelial cells against oxygen-glucose deprivation/reoxygenation-induced injury by sponging miR-26b and upregulating ULK2 expression. Neuroscience 354, 1–10. doi: 10.1016/j.neuroscience.2017.04.017
Liang, J., Wang, Q., Li, J. Q., Guo, T., and Yu, D. (2020). Long non-coding RNA MEG3 promotes cerebral ischemia-reperfusion injury through increasing pyroptosis by targeting miR-485/AIM2 axis. Exp. Neurol. 325:113139. doi: 10.1016/j.expneurol.2019.113139
Liao, B., Chen, R., Lin, F., Mai, A., Chen, J., Li, H., et al. (2018). Long noncoding RNA HOTTIP promotes endothelial cell proliferation and migration via activation of the Wnt/β-catenin pathway. J. Cell. Biochem. 119, 2797–2805. doi: 10.1002/jcb.26448
Liu, J., Li, Q., Zhang, K., Hu, B., Niu, X., Zhou, S., et al. (2017). Downregulation of the long non-coding RNA Meg3 promotes angiogenesis after ischemic brain injury by activating notch signaling. Mol. Neurobiol. 54, 8179–8190. doi: 10.1007/s12035-016-0270-z
Long, F., Su, Q., Zhou, J., Wang, D., Li, P., Zeng, C., et al. (2018). LncRNA SNHG12 ameliorates brain microvascular endothelial cell injury by targeting miR-199a. Neural Regen. Res. 13, 1919. doi: 10.4103/1673-5374.238717
Luo, H. C., Yi, T. Z., Huang, F. G., Wei, Y., Luo, X. P., and Luo, Q. S. (2020). Role of long noncoding RNA MEG3/miR-378/GRB2 axis in neuronal autophagy and neurological functional impairment in ischemic stroke. J. Biol. Chem. 295, 14125–14139. doi: 10.1074/jbc.RA119.010946
Mao, Q., Liang, X. L., Zhang, C. L., Pang, Y. H., and Lu, Y. X. (2019). LncRNA KLF3-AS1 in human mesenchymal stem cell-derived exosomes ameliorates pyroptosis of cardiomyocytes and myocardial infarction through miR-138-5p/Sirt1 axis. Stem Cell Res. Ther. 10, 393. doi: 10.1186/s13287-019-1522-4
Michalik, K. M., You, X., Manavski, Y., Doddaballapur, A., Zörnig, M., Braun, T., et al. (2014). Long noncoding RNA MALAT1 regulates endothelial cell function and vessel growth. Circ. Res. 114, 1389–1397. doi: 10.1161/CIRCRESAHA.114.303265
Mousavi, K., Zare, H., Dell Orso, S., Grontved, L., Gutierrez-Cruz, G., Derfoul, A., et al. (2013). eRNAs promote transcription by establishing chromatin accessibility at defined genomic loci. Mol. Cell 51, 606–617. doi: 10.1016/j.molcel.2013.07.022
Mozaffarian, D., Benjamin, E. J., Go, A. S., Arnett, D. K., Blaha, M. J., Cushman, M., et al. (2016). Heart disease and stroke statistics-2016 update: a report from the american heart association. Circulation 133, e38–e360. doi: 10.1161/CIR.0000000000000350
Oshikawa, M., Okada, K., Kaneko, N., Sawamoto, K., and Ajioka, I. (2017). Affinity-immobilization of VEGF on laminin porous sponge enhances angiogenesis in the ischemic brain. Adv. Healthc. Mater. 6:1700183. doi: 10.1002/adhm.201700183
Qi, X., Shao, M., Sun, H., Shen, Y., Meng, D., and Huo, W. (2017). Long non-coding RNA SNHG14 promotes microglia activation by regulating miR-145-5p/PLA2G4A in cerebral infarction. Neuroscience 348, 98–106. doi: 10.1016/j.neuroscience.2017.02.002
Qiao, P., Yan, H., and Wang, J. (2020). EGb761 Protects brain microvascular endothelial cells against oxygen–glucose deprivation-induced injury through lncRNA Rmst/miR-150 axis. Neurochem. Res. 45, 2398–2408. doi: 10.1007/s11064-020-03099-8
Randolph, S. A. (2016). Ischemic stroke. Workplace Health Saf. 64:444. doi: 10.1177/2165079916665400
Ruan, W., Li, J., Xu, Y., Wang, Y., Zhao, F., Yang, X., et al. (2019). MALAT1 up-regulator polydatin protects brain microvascular integrity and ameliorates stroke through C/EBPbeta/MALAT1/CREB/PGC-1alpha/PPARgamma pathway. Cell. Mol. Neurobiol. 39, 265–286. doi: 10.1007/s10571-018-00646-4
Ruan, W., Wu, J., Su, J., Jiang, Y., Pang, T., and Li, J. (2020). Altered lncRNAs transcriptomic profiles in atherosclerosis-induced ischemic stroke. Cell. Mol. Neurobiol. doi: 10.1007/s10571-020-00918-y. [Epub ahead of print].
Shekhar, S., Cunningham, M. W., Pabbidi, M. R., Wang, S., Booz, G. W., and Fan, F. (2018). Targeting vascular inflammation in ischemic stroke: recent developments on novel immunomodulatory approaches. Eur. J. Pharmacol. 833, 531–544. doi: 10.1016/j.ejphar.2018.06.028
Shen, J., Zhao, Z., Shang, W., Liu, C., Zhang, B., Xu, Z., et al. (2018). Fabrication of a nano polymer wrapping Meg3 ShRNA plasmid for the treatment of cerebral infarction. Artif. Cells Nanomed. Biotechnol. 46, 894–903. doi: 10.1080/21691401.2018.1471483
Sui, S., Sun, L., Zhang, W., Li, J., Han, J., Zheng, J., et al. (2020). LncRNA MEG8 attenuates cerebral ischemia after ischemic stroke through targeting miR-130a-5p/VEGFA signaling. Cell. Mol. Neurobiol. doi: 10.1007/s10571-020-00904-4. [Epub ahead of print].
Sun, P., Zhang, K., Hassan, S. H., Zhang, X., Tang, X., Pu, H., et al. (2020). Endothelium-targeted deletion of microRNA-15a/16-1 promotes poststroke angiogenesis and improves long-term neurological recovery. Circ. Res. 126, 1040–1057. doi: 10.1161/CIRCRESAHA.119.315886
Szcześniak, M. W., and Makałowska, I. (2016). lncRNA-RNA interactions across the human transcriptome. PLoS ONE 11:e0150353. doi: 10.1371/journal.pone.0150353
Takahashi, K., Ota, Y., Kogure, T., Suzuki, Y., Iwamoto, H., Yamakita, K., et al. (2020). Circulating extracellular vesicle-encapsulated HULC is a potential biomarker for human pancreatic cancer. Cancer Sci 111, 98–111. doi: 10.1111/cas.14232
Teng, H., Li, M., Qian, L., Yang, H., and Pang, M. (2020). Long noncoding RNA SNHG16 inhibits the oxygenglucose deprivation and reoxygenationinduced apoptosis in human brain microvascular endothelial cells by regulating miR15a5p/bcl2. Mol. Med. Rep. 22, 2685–2694. doi: 10.3892/mmr.2020.11385
Toiyama, Y., Okugawa, Y., and Goel, A. (2014). DNA methylation and microRNA biomarkers for noninvasive detection of gastric and colorectal cancer. Biochem. Bioph. Res. Co. 455, 43–57. doi: 10.1016/j.bbrc.2014.08.001
Vautrin, A., Manchon, L., Garcel, A., Campos, N., Lapasset, L., Laaref, A. M., et al. (2019). Both anti-inflammatory and antiviral properties of novel drug candidate ABX464 are mediated by modulation of RNA splicing. Sci. Rep. 9:792. doi: 10.1038/s41598-018-37813-y
Velagapudi, S. P., Luo, Y., Tran, T., Haniff, H. S., Nakai, Y., Fallahi, M., et al. (2017). Defining RNA–Small molecule affinity landscapes enables design of a small molecule inhibitor of an oncogenic noncoding RNA. ACS Central Sci. 3, 205–216. doi: 10.1021/acscentsci.7b00009
Wang, C., Qu, Y., Suo, R., and Zhu, Y. (2019). Long non-coding RNA MALAT1 regulates angiogenesis following oxygen-glucose deprivation/reoxygenation. J. Cell. Mol. Med. 23, 2970–2983. doi: 10.1111/jcmm.14204
Wang, G., Wu, Y., and Zhu, Y. (2018). Mechanism of MALAT1 preventing apoptosis of vascular endothelial cells induced by oxygen–glucose deficiency and reoxidation. Artif. Cell Nanomed. Biotechnol. 46, 798–805. doi: 10.1080/21691401.2018.1436065
Wang, J., Cao, B., Han, D., Sun, M., and Feng, J. (2017a). Long non-coding RNA h19 induces cerebral ischemia reperfusion injury via activation of autophagy. Aging Dis. 8:71. doi: 10.14336/AD.2016.0530
Wang, J., Ruan, J., Zhu, M., Yang, J., Du, S., Xu, P., et al. (2019). Predictive value of long noncoding RNA ZFAS1 in patients with ischemic stroke. Clin. Exp. Hypertens. 41, 615–621. doi: 10.1080/10641963.2018.1529774
Wang, J., Zhao, H., Fan, Z., Li, G., Ma, Q., Tao, Z., et al. (2017b). Long noncoding RNA H19 promotes neuroinflammation in ischemic stroke by driving histone deacetylase 1–dependent M1 microglial polarization. Stroke 48, 2211–2221. doi: 10.1161/STROKEAHA.117.017387
Wang, M., Chen, W., Geng, Y., Xu, C., Tao, X., and Zhang, Y. (2020). Long non-coding RNA MEG3 promotes apoptosis of vascular cells and is associated with poor prognosis in ischemic stroke. J. Atheroscler. Thromb. 27, 718–726. doi: 10.5551/jat.50674
Wang, S., Han, X., Mao, Z., Xin, Y., Maharjan, S., and Zhang, B. (2019). MALAT1 lncRNA induces autophagy and protects brain microvascular endothelial cells against oxygen–glucose deprivation by binding to miR-200c-3p and upregulating SIRT1 expression. Neuroscience 397, 116–126. doi: 10.1016/j.neuroscience.2018.11.024
Wang, Z., Wang, R., Wang, K., and Liu, X. (2018). Upregulated long noncoding RNA Snhg1 promotes the angiogenesis of brain microvascular endothelial cells after oxygen–glucose deprivation treatment by targeting miR-199a. Can. J. Physiol. Pharm. 96, 909–915. doi: 10.1139/cjpp-2018-0107
Wen, Y., Yu, Y., and Fu, X. (2017). LncRNA Gm4419 contributes to OGD/R injury of cerebral microglial cells via IκB phosphorylation and NF-κB activation. Biochem. Bioph. Res. Co. 487, 923–929. doi: 10.1016/j.bbrc.2017.05.005
Wu, H., He, Y., Chen, H., Liu, Y., Wei, B., Chen, G., et al. (2019). LncRNA THOR increases osteosarcoma cell stemness and migration by enhancing SOX9 mRNA stability. FEBS Open Bio. 9, 781–790. doi: 10.1002/2211-5463.12620
Wu, S., Wu, B., Liu, M., Chen, Z., Wang, W., Anderson, C. S., et al. (2019). Stroke in China: advances and challenges in epidemiology, prevention, and management. Lancet Neurol. 18, 394–405. doi: 10.1016/S1474-4422(18)30500-3
Wu, Z., Wu, P., Zuo, X., Yu, N., Qin, Y., Xu, Q., et al. (2017). LncRNA-N1LR enhances neuroprotection against ischemic stroke probably by inhibiting p53 phosphorylation. Mol. Neurobiol. 54, 7670–7685. doi: 10.1007/s12035-016-0246-z
Xin, J., and Jiang, Y. (2017). Long noncoding RNA MALAT1 inhibits apoptosis induced by oxygen-glucose deprivation and reoxygenation in human brain microvascular endothelial cells. Exp. Ther. Med. 13, 1225–1234. doi: 10.3892/etm.2017.4095
Xu, X., Zhuang, C., and Chen, L. (2020). Exosomal long non-coding RNA expression from serum of patients with acute minor stroke. Neuropsychiatr. Dis. Treat 16, 153–160. doi: 10.2147/NDT.S230332
Yan, G., Zhao, H., and Hong, X. (2020). LncRNA MACC1-AS1 attenuates microvascular endothelial cell injury and promotes angiogenesis under hypoxic conditions via modulating miR-6867-5p/TWIST1 in human brain microvascular endothelial cells. Annals of Translational Medicine 8, 876–876. doi: 10.21037/atm-20-4915
Yan, H., Rao, J., Yuan, J., Gao, L., Huang, W., Zhao, L., et al. (2017). Long non-coding RNA MEG3 functions as a competing endogenous RNA to regulate ischemic neuronal death by targeting miR-21/PDCD4 signaling pathway. Cell Death Dis. 8, 3211. doi: 10.1038/s41419-017-0047-y
Yan, H., Yuan, J., Gao, L., Rao, J., and Hu, J. (2016). Long noncoding RNA MEG3 activation of p53 mediates ischemic neuronal death in stroke. Neuroscience 337, 191–199. doi: 10.1016/j.neuroscience.2016.09.017
Yan, Y., Chen, L., Zhou, J., and Xie, L. (2020). SNHG12 inhibits oxygenglucose deprivationinduced neuronal apoptosis via the miR181a5p/NEGR1 axis. Mol. Med. Rep. 22, 3886–3894. doi: 10.3892/mmr.2020.11459
Yang, H., Xi, X., Zhao, B., Su, Z., and Wang, Z. (2018). KLF4 protects brain microvascular endothelial cells from ischemic stroke induced apoptosis by transcriptionally activating MALAT1. Biochem. Bioph. Res. Co. 495, 2376–2382. doi: 10.1016/j.bbrc.2017.11.205
Yang, X., and Zi, X. (2019). LncRNA SNHG1 alleviates OGD induced injury in BMEC via miR-338/HIF-1α axis. Brain Res. 1714, 174–181. doi: 10.1016/j.brainres.2018.11.003
Zhan, R., Xu, K., Pan, J., Xu, Q., Xu, S., and Shen, J. (2017). Long noncoding RNA MEG3 mediated angiogenesis after cerebral infarction through regulating p53/NOX4 axis. Biochem. Bioph. Res. Co. 490, 700–706. doi: 10.1016/j.bbrc.2017.06.104
Zhang, B., Wang, D., Ji, T., Shi, L., and Yu, J. (2017). Overexpression of lncRNA ANRIL up-regulates VEGF expression and promotes angiogenesis of diabetes mellitus combined with cerebral infarction by activating NF-κB signaling pathway in a rat model. Oncotarget 8, 17347–17359. doi: 10.18632/oncotarget.14468
Zhang, C. L., Zhu, K. P., and Ma, X. L. (2017). Antisense lncRNA FOXC2-AS1 promotes doxorubicin resistance in osteosarcoma by increasing the expression of FOXC2. Cancer Lett. 396, 66–75. doi: 10.1016/j.canlet.2017.03.018
Zhang, H., Wu, J., Wu, J., Fan, Q., Zhou, J., Wu, J., et al. (2019). Exosome-mediated targeted delivery of miR-210 for angiogenic therapy after cerebral ischemia in mice. J. Nanobiotechnol. 17, 29–29. doi: 10.1186/s12951-019-0461-7
Zhang, J., Liu, M., Huang, M., Chen, M., Zhang, D., Luo, L., et al. (2019). Ginsenoside F1 promotes angiogenesis by activating the IGF-1/IGF1R pathway. Pharmacol. Res. 144, 292–305. doi: 10.1016/j.phrs.2019.04.021
Zhang, J., Yuan, L., Zhang, X., Hamblin, M. H., Zhu, T., Meng, F., et al. (2016). Altered long non-coding RNA transcriptomic profiles in brain microvascular endothelium after cerebral ischemia. Exp. Neurol. 277, 162–170. doi: 10.1016/j.expneurol.2015.12.014
Zhang, K., Qi, M., Yang, Y., Xu, P., Zhua, Y., and Zhang, J. (2019). Circulating lncRNA ANRIL in the serum of patients with ischemic stroke. Clin. Lab. 65, 1459–1465. doi: 10.7754/Clin.Lab.2019.190143
Zhang, L., Luo, X., Chen, F., Yuan, W., Xiao, X., Zhang, X., et al. (2018). LncRNA SNHG1 regulates cerebrovascular pathologies as a competing endogenous RNA through HIF-1α/VEGF signaling in ischemic stroke. J. Cell. Biochem. 119, 5460–5472. doi: 10.1002/jcb.26705
Zhang, L., Niu, H., Ma, J., Yuan, B. Y., Chen, Y. H., Zhuang, Y., et al. (2019). The molecular mechanism of LncRNA34a-mediated regulation of bone metastasis in hepatocellular carcinoma. Mol. Cancer 18:120. doi: 10.1186/s12943-019-1044-9
Zhang, L., Yang, H., Li, W., and Liu, Y. (2020). LncRNA MALAT1 Promotes OGD-induced apoptosis of brain microvascular endothelial cells by sponging miR-126 to repress PI3K/Akt signaling pathway. Neurochem. Res. 45, 2091–2099. doi: 10.1007/s11064-020-03071-6
Zhang, T., Wang, H., Li, Q., Fu, J., Huang, J., and Zhao, Y. (2018). MALAT1 activates the P53 signaling pathway by regulating MDM2 to promote ischemic stroke. Cell. Physiol. Biochem. 50, 2216–2228. doi: 10.1159/000495083
Zhang, X., Tang, X., Hamblin, M., and Yin, K. (2018). Long non-coding RNA Malat1 regulates angiogenesis in hindlimb ischemia. Int. J. Mol. Sci. 19:1723. doi: 10.3390/ijms19061723
Zhang, X., Tang, X., Liu, K., Hamblin, M. H., and Yin, K. (2017). Long noncoding RNA Malat1 regulates cerebrovascular pathologies in ischemic stroke. J. Neurosci. 37, 1797–1806. doi: 10.1523/JNEUROSCI.3389-16.2017
Zhang, Y., Yuan, Y., Zhang, Y., Cheng, L., Zhou, X., and Chen, K. (2020). SNHG7 accelerates cell migration and invasion through regulating miR-34a-Snail-EMT axis in gastric cancer. Cell Cycle 19, 142–152. doi: 10.1080/15384101.2019.1699753
Zhang, Y., and Zhang, Y. (2020). lncRNA ZFAS1 improves neuronal injury and inhibits inflammation, oxidative stress, and apoptosis by sponging miR-582 and upregulating NOS3 expression in cerebral ischemia/reperfusion injury. Inflammation 43, 1337–1350. doi: 10.1007/s10753-020-01212-1
Zhao, M., Wang, J., Xi, X., Tan, N., and Zhang, L. (2018). SNHG12 promotes angiogenesis following ischemic stroke via regulating miR-150/VEGF pathway. Neuroscience 390, 231–240. doi: 10.1016/j.neuroscience.2018.08.029
Zhou, Z., Zheng, L., Ren, X., Li, A., and Zhou, W. (2019). LncRNA NEAT1 facilitates survival and angiogenesis in oxygen-glucose deprivation (OGD)-induced brain microvascular endothelial cells (BMECs) via targeting miR-377 and upregulating SIRT1, VEGFA, and BCL-XL. Brain Res. 1707, 90–98. doi: 10.1016/j.brainres.2018.10.031
Zhu, M., Li, N., Luo, P., Jing, W., Wen, X., Liang, C., et al. (2018). Peripheral blood leukocyte expression of lncRNA MIAT and its diagnostic and prognostic value in ischemic stroke. J. Stroke Cerebrovasc. Dis. 27, 326–337. doi: 10.1016/j.jstrokecerebrovasdis.2017.09.009
Zhu, W., Tian, L., Yue, X., Liu, J., Fu, Y., and Yan, Y. (2019). LncRNA expression profiling of ischemic stroke during the transition from the acute to subacute stage. Front. Neurol. 10:36. doi: 10.3389/fneur.2019.00036
Keywords: long non-coding RNAs, ischemic stroke, angiogenesis, endothelial cells, therapeutic targets
Citation: Gan L, Liao S, Xing Y and Deng S (2021) The Regulatory Functions of lncRNAs on Angiogenesis Following Ischemic Stroke. Front. Mol. Neurosci. 13:613976. doi: 10.3389/fnmol.2020.613976
Received: 04 October 2020; Accepted: 28 December 2020;
Published: 04 February 2021.
Edited by:
Shilei Hao, Chongqing University, ChinaReviewed by:
Kai K. Kummer, Innsbruck Medical University, AustriaMatthew B. Veldman, Medical College of Wisconsin, United States
Copyright © 2021 Gan, Liao, Xing and Deng. This is an open-access article distributed under the terms of the Creative Commons Attribution License (CC BY). The use, distribution or reproduction in other forums is permitted, provided the original author(s) and the copyright owner(s) are credited and that the original publication in this journal is cited, in accordance with accepted academic practice. No use, distribution or reproduction is permitted which does not comply with these terms.
*Correspondence: Shixiong Deng, ZGVuZ3NoaXhpb25nMTk2M0AxNjMuY29t