- Department of Medical Laboratory Sciences, Public Health, and Nutrition Science, College of Health Science, Tarleton State University, Fort Worth, TX, United States
Ischemic stroke is the fourth leading cause of adult disability in the US, and it is a huge social burden all over the world. However, the efficient treatment of ischemic stroke is not available. An apparent reason for failing to find or develop an intervention for ischemic stroke is contributed to the tight blood–brain barrier (BBB). The unique characteristics of exosomes that can traverse BBB have been highlighted among researchers investigating interventions for ischemic stroke conditions. Additionally, intermittent hypoxic training has been considered a potential intervention in the treatment or rehabilitation process of ischemic stroke patients. In this mini-review, we are going to review the possibility of applying exosomes produced by a subject who does intermittent hypoxic conditioning in a treatment program for ischemic stroke.
Introduction
In 2019, 77.19 million people worldwide had an ischemic stroke, which caused 11.6% of all deaths, making it the second leading cause of death and the top cause of chronic disability (World Health Organization, 2020; Pu et al., 2023). In the USA, it remained the fifth most common cause of death (Tsao et al., 2022). Stroke survivors often face lifelong disabilities that diminish their quality of life (Jiang et al., 2022; Gualerzi et al., 2021).
Cerebral ischemia causes reactive oxygen species accumulation, resulting in cerebral cell death and neuronal injury, with stroke pathophysiology being highly complex due to various endogenous and exogenous factors (Jung and Mallet, 2018; Mallet et al., 2018). The involvement of the immune response, excess inflammation, and programmed cell death in ischemic brain damage has been reported. BBB breakdown allows danger-associated molecular patterns (DAMP) and cytokines to leak into circulation, triggering systemic immunity, immunodepression, and severe infections. This can also cause cerebral edema due to vasodilation, increased BBB permeability, and cell swelling from ionic imbalance and inadequate metabolism (Gualerzi et al., 2021). Inflammation in the brain persists for years after stroke, which hinders long-term recovery and may contribute to the 5-year recurrence rate of 33% and mortality rate of approximately 70%.
Current stroke therapies, such as intravenous thrombolysis and endovascular thrombectomies, are effective but have a narrow therapeutic window. Delayed thrombolytic treatment can cause severe side effects, including potentially life-threatening hemorrhage (Ryou et al., 2013). Most of proposed alternative treatments for current limited options have failed clinical trials (Table 1) (Di Cesare et al., 2016; Elkind et al., 2020; Hill et al., 2020). Hence, additional therapeutic strategies are needed.
Therefore, understanding ischemic stroke pathogenesis is crucial for developing treatments. Efficiently delivering anti-inflammatory and protective agents to the brain without worsening BBB integrity could improve patient outcomes. This review explores the potential of exosomes obtained from an intervention in treatment and rehabilitation of stroke.
Methods
This review summarizes concepts and evaluates results from several research articles on related topics. It was conducted using the electronic literature databases PubMed, Academic Search Complete (EBSCO), and BioOne Complete. We narrowed down the academic and professional publishers available by searching the following keywords: exosomes, intermittent hypoxic training, ischemic stroke, and hypoxia. The search was performed by prioritizing the newest material to evaluate recent progress in this field, but there were no strict restrictions on dates if the content was relevant.
Exosomes
Exosomes are microvesicles (30–150 nm) in diameter, have a density of 1.08–1.19 g/mL, and can be found in most physiological fluids (Sidhom et al., 2020). Exosomes are secreted from diverse cells and are coated with a phospholipid bilayer derived from the membrane of the cell of origin. Upon being released into the extracellular medium, exosomes interact with the target cells located systemically or nearby and are involved in humoral intercellular communication. The unique characteristic is that exosomes contain cell-specific cargos of proteins, lipids, DNA, mRNA, and microRNA(miR). Hence, an intervention that can manipulate the biologically important cargo molecule carried by exosomes makes them a potential target for novel therapeutic manipulation and diagnostic biomarkers.
Exosomes originate by the endocytosis pathway in a cell of origin as vesicles known as multivesicular bodies (MVBs). The endosomal-sorting complex required for transport (ESCRT) family orchestrates early exosome’s molecular composition and formation. For example, ESCRT-0, ESCRT-I, and ESCRT-II regulate exosome cargo sorting. MVBs processed by lysosomes fuse with the plasma membrane to be released as mature exosomes. The secretion of exosomes is controlled by signal transduction factors, such as Rab GTPases and ESCRT-III, which undertake the deformation and fission of the membrane (Jiang et al., 2022; Cun et al., 2022). Upon being secreted, exosomes can be detected by many markers, including the tetraspanin family proteins (CD9, CD63, and CD81), heat shock proteins (Hsp), actin and flotillins, an endosomal sorting complex required for transport proteins (Alix and TSG101) and integrins (Jankovičová et al., 2020). However, the composition profile of exosomes is greatly influenced by the type and state of parental cells (Figure 1) (Ferreira et al., 2022).
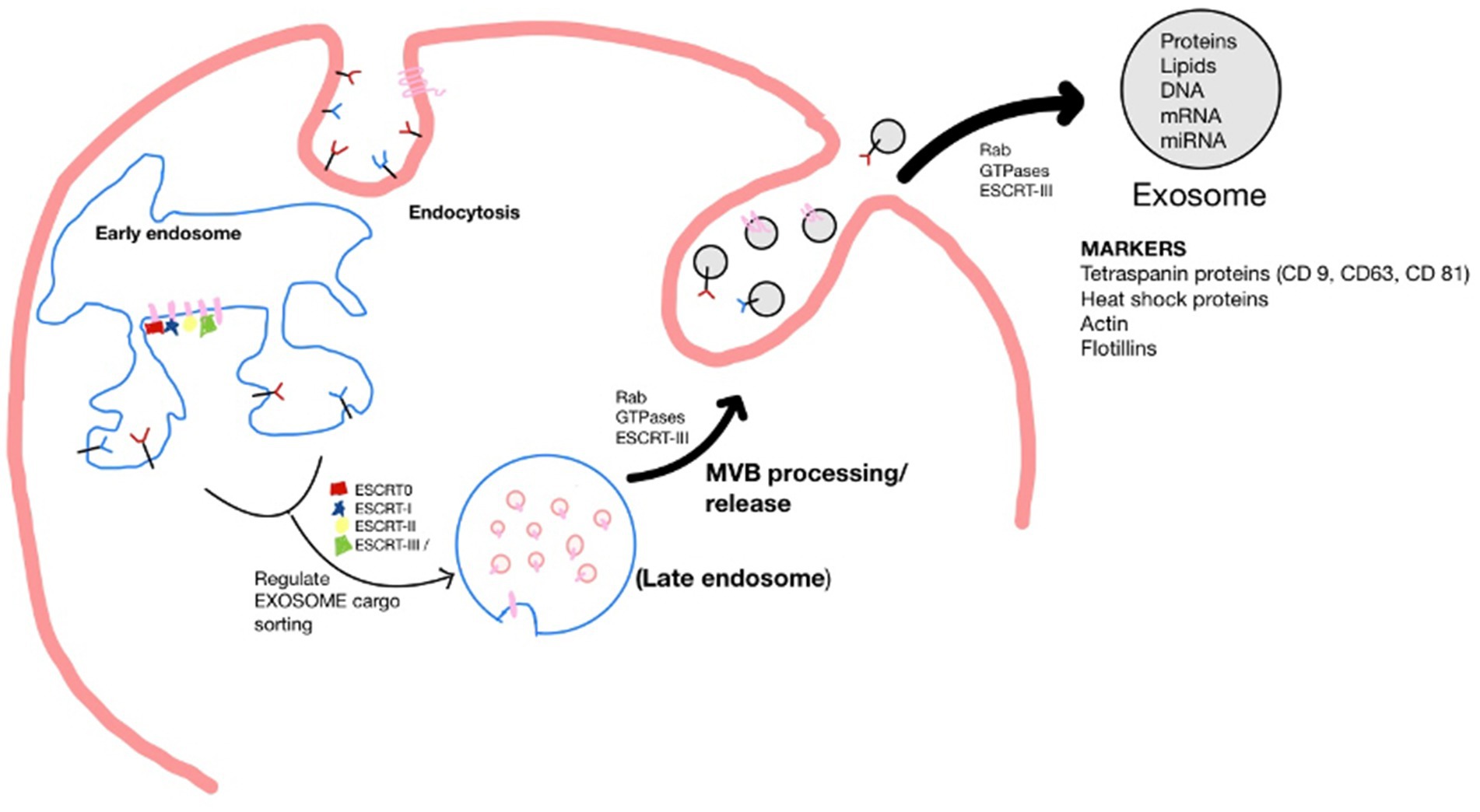
Figure 1. Schematic of the biogenesis of the exosome. Where, Endosomal-sorting complex required for transport (ESCRT); Multivesicular body (MVB).
Biocompatibility of exosomes
Exosomes are unique in representing the molecular signature of their particular cell-of-origin and can cross most anatomical barriers, including BBB, due to their biocompatibility (Ferreira et al., 2022). Therefore, exosomes can be exploited to reach hard-to-reach organs and to aid delivery to specific target sites (Rech et al., 2022). Exosomes can be retrieved and administered by minimally invasive procedures. Multiple techniques have been used for exosome isolation, including differential centrifugation (Kamerkar et al., 2017), ultrafiltration (Klymiuk et al., 2019), and size exclusion chromatography (Stranska et al., 2018).
Through surface engineering strategies, targets of exosomes and their circulation time can be modified accordingly, which can be achieved by sonication (Pham et al., 2021; Zhu et al., 2022), electroporation (Jia et al., 2018; Haney et al., 2020), and incubation (Belhadj et al., 2020). Furthermore, isolated exosomes can be loaded with therapeutic cargo, including small molecular-weight drugs, nucleic acids, proteins, and viral vectors (Ferreira et al., 2022). The most straightforward technique to enrich them with modifying agents is incubation (Gong et al., 2019). This reasoning explains the surge in research aimed at improving therapy outcomes and focusing on precision medicine through exosome therapy (Gualerzi et al., 2021).
Clinical application of exosomes
Exosomes’ diverse applications span diagnostic procedures, where they serve as carriers of biomarkers essential for disease detection and monitoring. Stem cell-derived exosomes are particularly valuable in facilitating tissue repair and regeneration. Emerging data suggests exosomes manifest several favorable features in their utilization as endogenous drug delivery in many therapies due to their low immunogenicity, innate stability, and high delivery efficiency. For example, engineered exosomes were exploited to simultaneously deliver an anticancer drug 5-FU and miR-21 inhibitor oligonucleotide (miR-21i) to Her2-expressing cancer cells. Tumor-bearing mice indicated a significant anti-tumor effect by inducing cell cycle arrest, reducing tumor proliferation, and increasing apoptosis (Liang et al., 2020).
Furthermore, they have been studied to aid therapy effects in a wide variety of other prevalent diseases worldwide, including different cancers such as colon and non-small cell lung cancer (NSCLC), myocardial infarction, kidney failure, and wound healing (Liang et al., 2020; Hu et al., 2023; Zhu et al., 2018; Kim et al., 2021; Shi et al., 2021). Exosomes have also been manipulated to target the brain specifically by surface modifications. The utilization of iron oxide nanoparticles (IONP) on the surfaces of exosomes not only enhances cell targeting but upregulates the expressions of angiogenic factors (Ang-1, FGF2, and VEGF) and anti-inflammation factors (TGF-β1 and TGF-β3) (Kim et al., 2020).
Exosomes are favorable in treating central nervous system (CNS) disorders because of their unique properties, as described earlier, and facilitate intercellular communication in the intracranial environment (Rech et al., 2022). Exosomes mediate therapeutic effects by transferring their cargos, thus modulating various pathways; all these types of cargo can result in multiple cerebroprotective properties in ischemic conditions, including angiogenesis, neurogenesis, anti-apoptosis, and inflammation (Jiang et al., 2022). Therefore, utilizing these exosomes to treat pathological conditions of the CNS is being focused.
The cargo potential of exosomes has been explored specifically with microRNAs (miR) to develop an efficient delivery system (Gong et al., 2019). MicroRNAs have critical roles in regulating gene expression and hold great promise for enhancing the efficiency of ischemic stroke treatment, given their ability to regulate multiple neuroprotective-targeted genes and related pathways (Tian et al., 2021). A study focused on the master hypoxia-induced miR-210, which promotes angiogenesis mediated by the vascular endothelial growth factor (VEGF) signaling pathway. Mice were subjected to 60 min of middle cerebral artery occlusion and 24 h of reperfusion (MCAO/R) to mimic an ischemic stroke, and, after 24 h of reperfusion, engineered exosomes carrying miR-210 were intravenously administered. After 14 days post-treatment, angiogenesis was improved, and there was a significant increase in animal survival (Zhang et al., 2019).
Furthermore, exosome-based intervention also improves neurogenesis by enhancing the differentiation of stem cells. The exosome enriched with MiR-17-92 cluster harvested from MSCs significantly improved neurological function and enhancements of oligodendrogenesis, neurogenesis, and neurite remodeling. It is suggested that this is due to targeting PTEN to activate the PI3K/Akt/mTOR, which leads to cell proliferation (Xin et al., 2017). Enkephalin delivery via exosomes released from MSCs is successful in preventing apoptosis. Enkephalin is an endogenous opioid peptide that decreases the expression of p53, caspase 3, and NO, contributing to apoptosis. At the same time, it increased neuronal density and accelerated neurological recovery after stroke was induced in rat models (Liu et al., 2019). Exosomal miR-137 was upregulated and participated in the partial neuroprotective effect by the Notch1 pathway (Zhang D. et al., 2021). Exosomes loaded with curcumin, known as antioxidant and anti-inflammatory, have strongly suppressed the lesion’s inflammatory response and cellular apoptosis (Tian et al., 2018; Hewlings and Kalman, 2017). Furthermore, Liu et al. reported that exosomes alleviated neuronal damage caused via pyroptosis via the inhibition of NLRP3 inflammasome-mediated inflammation; pyroptosis is a highly inflammatory mode of regulated cell death and contributes to the pathophysiology of ischemia–reperfusion (I/R) injury (Liu et al., 2021).
Exosomes released from microglial cells contribute to neuroprotection after stroke by anti-apoptotic and anti-inflammatory effects. Microglia, a resident cerebral phagocyte, is responsible for clearing metabolic wastes to maintain CNS homeostasis and promote removing or recovering brain cells injured by ischemia. Noxious conditions polarize the microglia to M1 (classical) or M2 (alternative) phenotypes. M1 microglia mediate pro-inflammatory responses, and M2 microglia play critical roles in healing and repairing damaged cells. M2 microglia-derived exosomes attenuated ischemic brain injury and promoted neuronal survival via exosomal miR-124 and its downstream target USP14, both antiinflammation regulators (Song et al., 2019). Furthermore, exosomes derived from M2 microglia attenuated neuronal apoptosis, decreased infarct volume, and reduced behavioral deficits in ischemic mice. Exosomes enriched with zinc finger E-box binding homeobox 2 protein (zeb2) and Axin2 have also enhanced neurogenesis. Zeb2 is a transcriptional regulator and is associated with the transforming growth factor β (TGF-β) signaling pathway, which is essential during early neurodevelopment. Axin 2 is related to TGF-β pathway, related to endothelial cell angiogenesis and Ent/ β-catenin pathway. These results show that the treated exosomes induced functional recovery after stroke by stimulating endogenous neurogenesis in MCAO rats, and the mechanism is believed to be related to the SOX10, Wnt/β-catenin, and endothelin-3/EDNRB pathways (Wei et al., 2022) (Table 2).
Clinical applications of intermittent hypoxic training in stroke
Hypoxic stresses can elicit a series of physiological responses in our system and are involved in many physiological and pathological processes (Yang et al., 2023). Stroke recovery is associated with restorative events that increase angiogenesis and neurogenesis levels and reduce inflammation.
Intermittent hypoxia (IH) at approximately 10% oxygen level has been documented to improve ischemic injury in cerebrovascular diseases and associated risk factors (Yuan et al., 2022; Jackman et al., 2014). For example, short-term IHT has been shown to promote gliogenesis in middle cerebral artery occlusion rat models (Roshan et al., 2023). IHT has also been shown to promote the recovery of motor function of cerebral ischemia by regulating mitochondrial function (Su et al., 2022). It has been reported that Repetitive hypoxic preconditioning has been shown to upregulate the chemokine CXCL12, which contributes to the endogenous, anti-inflammatory phenotype before stroke onset (Selvaraj et al., 2017).
Based on favorable reports, IHT could be used as an intervention for ischemic stroke therapy. Recently, we reported that nIHT modulates microglial polarization, specifically increasing the rate of M2 phenotype (Tantingco and Ryou, 2020). Microglial phenotype should be considered when developing interventions for ischemic stroke patients due to its anti-inflammatory and neuroprotective properties. Overall, evidence suggests nIHT may be an innovative, non-invasive way to augment damage and promote recovery associated with neurovascular injury.
Challenges regarding IHT have been documented. Despite being noninvasive and nonpharmacological, it requires intense investigation for safe application to patients in a clinical setting. The brain’s metabolism is highly active and very sensitive to changes in oxygen levels, especially in several groups of individuals. Elderly patients who are more at risk for IS are also susceptible to hypoxia due to preexisting heart or lung conditions.
Therefore, the intensity and timing are essential and may vary significantly between patient groups. Since this is also a systemic and multi-targeted intervention, off-target effects and potential damage to other organs must be explored (Yuan et al., 2022).
Clinical applications of pre-conditioned exosomes
It is well documented that hypoxic conditions affect exosome contents, thereby regulating various functions that may promote or augment pathophysiology. Exosomes pre-conditioned with hypoxia in vivo have recently been explored in one study where they determined that the expression of exosomal metabolites and proteomics varied significantly compared to controls (Fan et al., 2023). Similarly, these results have been reinforced in vitro by next-generation sequencing, where exosomal-miRNAs expression profile and function were differentially expressed between hypoxic preconditioned and control groups (Zhang et al., 2018). Specific cell lineages and the duration and severity they are exposed to hypoxia exhibit divergent exosome changes (Senda et al., 2023). Nevertheless, the exact mechanisms underlying the hypoxia regulation on exosome production, cargo composition, and function still need to be understood entirely.
Exosomes that have been pre-conditioned with hypoxia are the current focus of research. Investigating changes in exosomes and their role in hypoxic injury and adaptation may help in preventing secondary damage caused by hypoxia. It has been widely reported that these conditioned exosomes obtained after hypoxia may have therapeutic potential in various diseases, including ischemic-related injury. For example, exosomes extracted from splenic ischemic preconditioning models exerted a protective effect to attenuate renal I/R injury by reducing apoptosis and increasing TNF-α and interleukin-1β (Liu and Shen, 2023). In addition, repeated remote ischemic conditioning is cardioprotective in rat models after myocardial infarction (Luo et al., 2023; Yamaguchi et al., 2015).
Furthermore, novel studies have investigated the effects of preconditioned exosomes on the central nervous system. Exosomes secreted from MSCs under ischemia carry an increased concentration of miRNA-21, which may be responsible for mediating synaptic dysfunction and regulating inflammatory responses in APP/PS1 mice. Rescuing cognitive decline is an attractive approach to developing a potential treatment for Alzheimer’s disease (Cui et al., 2018).
Neuronal death is a leading cause of the pathophysiology following spinal-cord injury (SCI). Therapies that target revascularization and inflammation are vital components when exploring treatment options for spinal cord repair. Exosomes derived from hypoxia preconditioned human umbilical vein endothelial cells have demonstrated angiogenic function by cell-to-cell communication with mesenchymal stem cells (MSCs) in spinal cord repair. Stimulated MSCs showed significant tube formation within 2 h and promoted nerve tissue repair following spinal cord transection in rats through their proangiogenic and anti-inflammatory effects (Li et al., 2022) Additionally, exosomes derived from hypoxia-conditioned adipose tissue-derived stromal cells significantly reduced neuronal apoptosis after OGD in rat SCI models; miR-499a-5p expression in these exosomes was believed to regulate the JNK3/c-jun-apoptotic signaling pathway by targeting JNK3 (Liang et al., 2022). Another study noted miR-216a-5p shuttled by pre-conditioned exosomes helps repair traumatic spinal cord injury by shifting microglial M1/M2 polarization (Liu et al., 2020). These results combined all support pre-conditioned exosome therapy to reduce neuronal damage after spinal cord injury and may pose an effective treatment strategy.
Clinical applications of pre-conditioned exosomes in stroke
Pre-conditioned exosomes derived from human microglial cells, astrocytes, adipose-derived stromal cells, and neural and mesenchymal stem cells have therapeutic effects on neuronal injury in vitro (Zhang D. et al., 2021; Zhang L. et al., 2021; Xin et al., 2023; Yang H. et al., 2022; Ye et al., 2022; Guitart et al., 2016).
Cerebral ischemia induces inflammation due to high levels of cytokines and chemokines at the early stage of the disease. Extracellular vesicles (EV) from hypoxia-preconditioned microglia promote tissue regeneration and neurological recovery in stroke mice via the TGF-β/Smad2/3 pathway. This is due to the M2 phenotype shift post-conditioning, which yields enrichment of the TGF-β1 protein inside EVs harvested from microglia. These EVs cross the BBB to further increase the numbers of resident anti-inflammatory M2 microglia within the ischemic brain. M2 microglia alter the cellular microenvironment following cerebral damage by clearing cell debris and releasing immunomodulatory factors.
Furthermore, TGF-β1 activates the Smad2/3 signaling pathway, ultimately contributing to cellular protection and increased neurological recovery both in vitro and in vivo (Zhang D. et al., 2021; Zhang L. et al., 2021). In addition, another study confirmed that preconditioned EVs from microglia augmented M2 polarization and repressed M1 microglia polarization even further than non-conditioned EVs. They report a decreased periinfarct AQP4 depolarization, brain edema, astrogliosis, and inflammation in stroke mice (Xin et al., 2023).
Furthermore, previous investigations have shown therapeutic effects of using hypoxic pre-treated adipose-derived stromal cell exosomes in cerebral ischemic injury. The mechanism involves improved cognitive function after cerebral infarction via delivery of circ-Rps5; circ-Rps5-mediated SIRT7 suppresses LPS-induced inflammation and apoptosis through the NF-κB signaling pathway. Neuronal damage was attenuated, and the hippocampus’s microglia shifted from M1 to M2 phenotype (Yang H. et al., 2022; Yang C. et al., 2022).
Vascular remodeling is essential for a better prognosis after a stroke. Infarct-preconditioned exosomes of umbilical cord mesenchymal stem cells promoted neurological recovery after stroke in rats by minimizing apoptosis and enhancing migration and vascular endothelial remodeling (Ye et al., 2022). Another study suggested that hypoxia enhanced the angiogenic potential of DPSC-derived exosomes via the transfer of lysyl oxidase-like 2 (LOXL2) (Li et al., 2023).
Hypoxic pre-conditioned exosomes isolated from neural stem cells SCs (NSCs-Ex) have shown neuroprotective characteristics. NSCs-Ex prevents cerebral injury by transferring miR-150-3p, which promotes neuron proliferation and suppresses neuronal apoptosis by inhibiting the CASP2 signaling pathway in vitro (Luo et al., 2022). These results have been reconfirmed in another study in vivo preconditioning on NSCs exosomes further exerted therapeutic effects on both survival and behavioral outcomes in ischemic stroke mice. Overall, hypoxic preconditioning NSCs can produce effective nano agents and may represent a promising strategy for clinical neurorestorative therapy (Jiang et al., 2023).
Exosomes derived from astrocytes under oxygen/glucose deprivation conditions were said to increase prion protein (PrP) levels. PrP has a protective role for neural cells, specifically against ischemic injury (Onodera et al., 2014).
Future studies could, therefore, investigate the neuroprotective adaptations of exosomes after repetitive low-dose nIH exposures and how this may be used to advantage in an innovative new strategy for stroke patient rehabilitation.
Conclusion
The brain, which consumes the most oxygen of any organ, is highly sensitive to damage that may be induced by hypoxia, which is closely related to stroke and other brain diseases. However, nIHT has shown promising effects to mitigate harmful effects and enhance protective responses to protect the brain from ischemic injury. As an important intercellular player in neurovascular communication, the exosome mediates cerebral injury by transferring protein and RNA cargoes.
In conclusion, novel evidence suggests exosomes that have undergone hypoxic preconditioning have neuroprotective properties, but the therapeutic effects of intermittent hypoxia have not yet been explored. Published journals investigating exosomes that have been pre-conditioned with IHT are very limited. Nevertheless, exploring the potential benefits of combining IHT and exosome therapy would be of great value, which has been noted separately as successful. In contrast, several areas allow improvement of current strategies for disease treatment using exosomes. Current isolation and purification techniques are time-consuming and have low yield rates (Stranska et al., 2018). Challenges associated with the rapid biological clearance rate of exosomes post-local administration must be addressed to maximize therapeutic efficacy while minimizing unwanted effects.
Furthermore, IHT can elicit different exosome responses depending on their cell lineage and the hypoxic exposure’s duration, frequency, and intensity.
Overall, the protective mechanisms underlying intermittent hypoxic conditioning and utilizing exosomes are complex and diverse and have yet to be fully explored. Despite this, current evidence suggests this may hold great potential in stroke rehabilitation in the future.
Author contributions
M-GR: Writing – review & editing, Visualization, Validation, Supervision, Resources. SB: Writing – original draft.
Funding
The author(s) declare financial support was received for the research, authorship, and/or publication of this article. This study was supported by the Tarleton State University College of Graduate Studies provided Research assistantship to SB.
Conflict of interest
The authors declare that the research was conducted in the absence of any commercial or financial relationships that could be construed as a potential conflict of interest.
The author(s) declared that they were an editorial board member of Frontiers, at the time of submission. This had no impact on the peer review process and the final decision.
Publisher’s note
All claims expressed in this article are solely those of the authors and do not necessarily represent those of their affiliated organizations, or those of the publisher, the editors and the reviewers. Any product that may be evaluated in this article, or claim that may be made by its manufacturer, is not guaranteed or endorsed by the publisher.
References
Belhadj, Z., He, B., Deng, H., Song, S., Zhang, H., Wang, X., et al. (2020). A combined "eat me/don't eat me" strategy based on extracellular vesicles for anticancer nanomedicine. J. Extracell. Vesicles 9:1806444. doi: 10.1080/20013078.2020.1806444
ClinicalTrials.gov . (2016). A study evaluating the safety and efficacy of PF-03049423 in subjects with ischemic stroke. Available at: https://classic.clinicaltrials.gov/ct2/show/NCT01208233
Cui, G. H., Wu, J., Mou, F. F., Xie, W. H., Wang, F. B., Wang, Q. L., et al. (2018). Exosomes derived from hypoxia-preconditioned mesenchymal stromal cells ameliorate cognitive decline by rescuing synaptic dysfunction and regulating inflammatory responses in APP/PS1 mice. FASEB J. 32, 654–668. doi: 10.1096/fj.201700600R
Cun, Y., Jin, Y., Wu, D., Zhou, L., Zhang, C., Zhang, S., et al. (2022). Exosome in crosstalk between inflammation and angiogenesis: a potential therapeutic strategy for stroke. Mediators Inflamm. 2022:7006281. doi: 10.1155/2022/7006281
Di Cesare, F., Mancuso, J., Woodward, P., Bednar, M. M., and Loudon, P. T.A9541004 Stroke Study Group (2016). Phosphodiesterase-5 inhibitor PF-03049423 effect on stroke recovery: a double-blind, placebo-controlled randomized clinical trial. J. Stroke Cerebrovasc. Dis. 25, 642–649. doi: 10.1016/j.jstrokecerebrovasdis.2015.11.026
Elkind, M. S. V., Veltkamp, R., Montaner, J., Johnston, S. C., Singhal, A. B., Becker, K., et al. (2020). Natalizumab in acute ischemic stroke (ACTION II): a randomized, placebo-controlled trial. Neurology 95, e1091–e1104. doi: 10.1212/WNL.0000000000010038
Fan, F., Du, Y., Chen, L., Chen, Y., Zhong, Z., Li, P., et al. (2023). Metabolomic and proteomic identification of serum exosome for hypoxic preconditioning participants. Oxidative Med. Cell. Longev. 2023:5509913. doi: 10.1155/2023/5509913
Ferreira, D., Moreira, J. N., and Rodrigues, L. R. (2022). New advances in exosome-based targeted drug delivery systems. Crit. Rev. Oncol. Hematol. 172:103628. doi: 10.1016/j.critrevonc.2022.103628
Gong, C., Tian, J., Wang, Z., Gao, Y., Wu, X., Ding, X., et al. (2019). Functional exosome-mediated co-delivery of doxorubicin and hydrophobically modified microRNA 159 for triple-negative breast cancer therapy. J. Nanobiotechnology 17:93. doi: 10.1186/s12951-019-0526-7
Gualerzi, A., Picciolini, S., Rodà, F., and Bedoni, M. (2021). Extracellular vesicles in regeneration and rehabilitation recovery after stroke. Biology (Basel) 10:843. doi: 10.3390/biology10090843
Guitart, K., Loers, G., Buck, F., Bork, U., Schachner, M., and Kleene, R. (2016). Improvement of neuronal cell survival by astrocyte-derived exosomes under hypoxic and ischemic conditions depends on prion protein. Glia 64, 896–910. doi: 10.1002/glia.22963
Haney, M. J., Zhao, Y., Jin, Y. S., Li, S. M., Bago, J. R., Klyachko, N. L., et al. (2020). Macrophage-derived extracellular vesicles as drug delivery Systems for Triple Negative Breast Cancer (TNBC) therapy. J. Neuroimmune Pharmacol. 15, 487–500. doi: 10.1007/s11481-019-09884-9
Hewlings, S. J., and Kalman, D. S. (2017). Curcumin: a review of its effects on human health. Foods 6:92. doi: 10.3390/foods6100092
Hill, M. D., Goyal, M., Menon, B. K., Nogueira, R. G., McTaggart, R., Demchuk, A. M., et al. (2020). Efficacy and safety of nerinetide for the treatment of acute ischaemic stroke (ESCAPE-NA1): a multicentre, double-blind, randomised controlled trial. Lancet 395, 878–887. doi: 10.1016/S0140-6736(20)30258-0
Hu, N., Cai, Z., Jiang, X., Wang, C., Tang, T., Xu, T., et al. (2023). Hypoxia-pretreated ADSC-derived exosome-embedded hydrogels promote angiogenesis and accelerate diabetic wound healing. Acta Biomater. 157, 175–186. doi: 10.1016/j.actbio.2022.11.057
Jackman, K. A., Zhou, P., Faraco, G., Peixoto, P. M., Coleman, C., Voss, H. U., et al. (2014). Dichotomous effects of chronic intermittent hypoxia on focal cerebral ischemic injury. Stroke 45, 1460–1467. doi: 10.1161/STROKEAHA.114.004816
Jankovičová, J., Sečová, P., Michalková, K., and Antalíková, J. (2020). Tetraspanins, more than markers of extracellular vesicles in reproduction. Int. J. Mol. Sci. 21:7568. doi: 10.3390/ijms21207568
Jia, G., Han, Y., An, Y., Ding, Y., He, C., Wang, X., et al. (2018). NRP-1 targeted and cargo-loaded exosomes facilitate simultaneous imaging and therapy of glioma in vitro and in vivo. Biomaterials 178, 302–316. doi: 10.1016/j.biomaterials.2018.06.029
Jiang, L., Chen, W., Ye, J., and Wang, Y. (2022). Potential role of exosomes in ischemic stroke treatment. Biomol. Ther. 12:115. doi: 10.3390/biom12010115
Jiang, X.-C., Wu, H. H., Zhang, T., Dong, Y. F., Li, Y. S., Huang, T., et al. (2023). Biological nano agent produced by hypoxic preconditioning stem cell for stroke treatment. Nano Res. 16, 7413–7421. doi: 10.1007/s12274-023-5470-z
Jung, M. E., and Mallet, R. T. (2018). Intermittent hypoxia training: powerful, non-invasive cerebroprotection against ethanol withdrawal excitotoxicity. Respir. Physiol. Neurobiol. 256, 67–78. doi: 10.1016/j.resp.2017.08.007
Kamerkar, S., LeBleu, V., Sugimoto, H., Yang, S., Ruivo, C. F., Melo, S. A., et al. (2017). Exosomes facilitate therapeutic targeting of oncogenic KRAS in pancreatic cancer. Nature 546, 498–503. doi: 10.1038/nature22341
Kim, H. Y., Kim, T., Kang, L., Kim, Y. J., Kang, M., Kim, J., et al. (2020). Mesenchymal stem cell-derived magnetic extracellular nanovesicles for targeting and treatment of ischemic stroke. Biomaterials 243:119942. doi: 10.1016/j.biomaterials.2020.119942
Kim, S., Lee, S. A., Yoon, H., Kim, M. Y., Yoo, J. K., Ahn, S. H., et al. (2021). Exosome-based delivery of super-repressor IkappaBalpha ameliorates kidney ischemia-reperfusion injury. Kidney Int. 100, 570–584. doi: 10.1016/j.kint.2021.04.039
Klymiuk, M. C., Balz, N., Elashry, M. I., Heimann, M., Wenisch, S., and Arnhold, S. (2019). Exosomes isolation and identification from equine mesenchymal stem cells. BMC Vet. Res. 15:42. doi: 10.1186/s12917-019-1789-9
Li, B., Liang, A., Zhou, Y., Huang, Y., Liao, C., Zhang, X., et al. (2023). Hypoxia preconditioned DPSC-derived exosomes regulate angiogenesis via transferring LOXL2. Exp. Cell Res. 425:113543. doi: 10.1016/j.yexcr.2023.113543
Li, L., Mu, J., Zhang, Y., Zhang, C., Ma, T., Chen, L., et al. (2022). Stimulation by exosomes from hypoxia preconditioned human umbilical vein endothelial cells facilitates mesenchymal stem cells Angiogenic function for spinal cord repair. ACS Nano 16, 10811–10823. doi: 10.1021/acsnano.2c02898
Liang, Y., Wu, J. H., Zhu, J. H., and Yang, H. (2022). Exosomes secreted by hypoxia-pre-conditioned adipose-derived mesenchymal stem cells reduce neuronal apoptosis in rats with spinal cord injury. J. Neurotrauma 39, 701–714. doi: 10.1089/neu.2021.0290
Liang, G., Zhu, Y., Ali, D. J., Tian, T., Xu, H., Si, K., et al. (2020). Engineered exosomes for targeted co-delivery of miR-21 inhibitor and chemotherapeutics to reverse drug resistance in colon cancer. J. Nanobiotechnology 18:10. doi: 10.1186/s12951-019-0563-2
Liu, Y., Fu, N., Su, J., Wang, X., and Li, X. (2019). Rapid Enkephalin delivery using exosomes to promote neurons recovery in ischemic stroke by inhibiting neuronal p53/Caspase-3. Biomed. Res. Int. 2019:4273290. doi: 10.1155/2019/4273290
Liu, W., Rong, Y., Wang, J., Zhou, Z., Ge, X., Ji, C., et al. (2020). Exosome-shuttled miR-216a-5p from hypoxic preconditioned mesenchymal stem cells repair traumatic spinal cord injury by shifting microglial M1/M2 polarization. J. Neuroinflammation 17:47. doi: 10.1186/s12974-020-1726-7
Liu, H., and Shen, Y. (2023). Renal ischemia-reperfusion injury attenuated by exosomes extracted from splenic ischemic preconditioning models. Transplantation 107, e90–e97. doi: 10.1097/TP.0000000000004514
Liu, X., Zhang, M., Liu, H., Zhu, R., He, H., Zhou, Y., et al. (2021). Bone marrow mesenchymal stem cell-derived exosomes attenuate cerebral ischemia-reperfusion injury-induced neuroinflammation and pyroptosis by modulating microglia M1/M2 phenotypes. Exp. Neurol. 341:113700. doi: 10.1016/j.expneurol.2021.113700
Luo, Z., Hu, X., Wu, C., Chan, J., Liu, Z., Guo, C., et al. (2023). Plasma exosomes generated by ischaemic preconditioning are cardioprotective in a rat heart failure model. Br. J. Anaesth. 130, 29–38. doi: 10.1016/j.bja.2022.08.040
Luo, H., Ye, G., Liu, Y., Huang, D., Luo, Q., Chen, W., et al. (2022). miR-150-3p enhances neuroprotective effects of neural stem cell exosomes after hypoxic-ischemic brain injury by targeting CASP2. Neurosci. Lett. 779:136635. doi: 10.1016/j.neulet.2022.136635
Mallet, R. T., Manukhina, E. B., Ruelas, S. S., Caffrey, J. L., and Downey, H. F. (2018). Cardioprotection by intermittent hypoxia conditioning: evidence, mechanisms, and therapeutic potential. Am. J. Physiol. Heart Circ. Physiol. 315, H216–H232. doi: 10.1152/ajpheart.00060.2018
Onodera, T., Sakudo, A., Tsubone, H., and Itohara, S. (2014). Review of studies that have used knockout mice to assess normal function of prion protein under immunological or pathophysiological stress. Microbiol. Immunol. 58, 361–374. doi: 10.1111/1348-0421.12162
Pham, T. C., Jayasinghe, M. K., Pham, T. T., Yang, Y., Wei, L., Usman, W. M., et al. (2021). Covalent conjugation of extracellular vesicles with peptides and nanobodies for targeted therapeutic delivery. J. Extracell. Vesicles 10:e12057. doi: 10.1002/jev2.12057
Pu, L., Wang, L., Zhang, R., Zhao, T., Jiang, Y., and Han, L. (2023). Projected global trends in ischemic stroke incidence, deaths and disability-adjusted life years from 2020 to 2030. Stroke 54, 1330–1339. doi: 10.1161/STROKEAHA.122.040073
Rech, J., Gałka, S., and Bednarek, I. (2022). Origin and composition of exosomes as crucial factors in designing drug delivery systems. Appl. Sci. 12:12259. doi: 10.3390/app122312259
Roshan, S., Gunaseelan, D., Jayachandran, S. K., Kandasamy, M., and Anusuyadevi, M. (2023). Short-term intermittent hypoxia therapy promotes gliogenesis in a rat model of middle cerebral artery occlusion (MCAO) stroke. bioRxiv 2023.06.26.546509. doi: 10.1101/2023.06.26.546509
Ryou, M. G., Choudhury, G. R., Winters, A., Xie, L., Mallet, R. T., and Yang, S. H. (2013). Pyruvate minimizes rtPA toxicity from in vitro oxygen-glucose deprivation and reoxygenation. Brain Res. 1530, 66–75. doi: 10.1016/j.brainres.2013.07.029
Selvaraj, U. M., Ortega, S. B., Hu, R., Gilchrist, R., Kong, X., Partin, A., et al. (2017). Preconditioning-induced CXCL12 upregulation minimizes leukocyte infiltration after stroke in ischemia-tolerant mice. J. Cereb. Blood Flow Metab. 37, 801–813. doi: 10.1177/0271678X16639327
Senda, A., Kojima, M., Watanabe, A., Kobayashi, T., Morishita, K., Aiboshi, J., et al. (2023). Profiles of lipid, protein and microRNA expression in exosomes derived from intestinal epithelial cells after ischemia-reperfusion injury in a cellular hypoxia model. PLoS One 18:e0283702. doi: 10.1371/journal.pone.0283702
Shi, A., Li, J., Qiu, X., Sabbah, M., Boroumand, S., Huang, T. C., et al. (2021). TGF-beta loaded exosome enhances ischemic wound healing in vitro and in vivo. Theranostics 11, 6616–6631. doi: 10.7150/thno.57701
Sidhom, K., Obi, P. O., and Saleem, A. (2020). A review of exosomal isolation methods: is size exclusion chromatography the best option? Int. J. Mol. Sci. 21:6466. doi: 10.3390/ijms21186466
Song, Y., Li, Z., He, T., Qu, M., Jiang, L., Li, W., et al. (2019). M2 microglia-derived exosomes protect the mouse brain from ischemia-reperfusion injury via exosomal miR-124. Theranostics 9, 2910–2923. doi: 10.7150/thno.30879
Stranska, R., Gysbrechts, L., Wouters, J., Vermeersch, P., Bloch, K., Dierickx, D., et al. (2018). Comparison of membrane affinity-based method with size-exclusion chromatography for isolation of exosome-like vesicles from human plasma. J. Transl. Med. 16:1. doi: 10.1186/s12967-017-1374-6
Su, Y., Ke, C., Li, C., Huang, C., and Wan, C. (2022). Intermittent hypoxia promotes the recovery of motor function in rats with cerebral ischemia by regulating mitochondrial function. Exp. Biol. Med. (Maywood) 247, 1364–1378. doi: 10.1177/15353702221098962
Tantingco, G., and Ryou, M. G. (2020). Normobaric intermittent hypoxic training regulates microglia phenotype and enhances phagocytic activity. Exp. Biol. Med. (Maywood) 245, 740–747. doi: 10.1177/1535370220919361
Tian, T., Cao, L., He, C., Ye, Q., Liang, R., You, W., et al. (2021). Targeted delivery of neural progenitor cell-derived extracellular vesicles for anti-inflammation after cerebral ischemia. Theranostics 11, 6507–6521. doi: 10.7150/thno.56367
Tian, T., Zhang, H. X., He, C. P., Fan, S., Zhu, Y. L., Qi, C., et al. (2018). Surface functionalized exosomes as targeted drug delivery vehicles for cerebral ischemia therapy. Biomaterials 150, 137–149. doi: 10.1016/j.biomaterials.2017.10.012
Tsao, C. W., Aday, A. W., Almarzooq, Z. I., Alonso, A., Beaton, A. Z., Bittencourt, M. S., et al. (2022). Heart disease and stroke Statistics-2022 update: a report from the American Heart Association. Circulation 145, e153–e639. doi: 10.1161/CIR.0000000000001052
Wei, R., Zhang, L., Hu, W., Shang, X., He, Y., and Zhang, W. (2022). Zeb2/Axin2-enriched BMSC-derived exosomes promote post-stroke functional recovery by enhancing neurogenesis and neural plasticity. J. Mol. Neurosci. 72, 69–81. doi: 10.1007/s12031-021-01887-7
World Health Organization (2020). The top 10 causes of death : World Health Organization. Available at: https://www.who.int/news-room/fact-sheets/detail/the-top-10-causes-of-death
Xin, H., Katakowski, M., Wang, F., Qian, J. Y., Liu, X. S., Ali, M. M., et al. (2017). MicroRNA cluster miR-17-92 cluster in exosomes enhance neuroplasticity and functional recovery after stroke in rats. Stroke 48, 747–753. doi: 10.1161/STROKEAHA.116.015204
Xin, W., Pan, Y., Wei, W., Tatenhorst, L., Graf, I., Popa-Wagner, A., et al. (2023). Preconditioned extracellular vesicles from hypoxic microglia reduce poststroke AQP4 depolarization, disturbed cerebrospinal fluid flow, astrogliosis, and neuroinflammation. Theranostics 13, 4197–4216. doi: 10.7150/thno.84059
Yamaguchi, T., Izumi, Y., Nakamura, Y., Yamazaki, T., Shiota, M., Sano, S., et al. (2015). Repeated remote ischemic conditioning attenuates left ventricular remodeling via exosome-mediated intercellular communication on chronic heart failure after myocardial infarction. Int. J. Cardiol. 178, 239–246. doi: 10.1016/j.ijcard.2014.10.144
Yang, R., Li, Z., Xu, J., Luo, J., Qu, Z., Chen, X., et al. (2023). Role of hypoxic exosomes and the mechanisms of exosome release in the CNS under hypoxic conditions. Front. Neurol. 14:1198546. doi: 10.3389/fneur.2023.1198546
Yang, H., Tu, Z., Yang, D., Hu, M., Zhou, L., Li, Q., et al. (2022). Exosomes from hypoxic pre-treated ADSCs attenuate acute ischemic stroke-induced brain injury via delivery of circ-Rps5 and promote M2 microglia/macrophage polarization. Neurosci. Lett. 769:136389. doi: 10.1016/j.neulet.2021.136389
Yang, C., Yuan, F., Shao, W., Yao, L., Jin, S., and Han, F. (2022). Protective role of exosomes derived from regulatory T cells against inflammation and apoptosis of BV-2 microglia under oxygen-glucose deprivation/reperfusion challenge. Genet. Mol. Biol. 45:e20220119. doi: 10.1590/1678-4685-GMB-2022-0119
Ye, Y. C., Chang, Z. H., Wang, P., Wang, Y. W., Liang, J., Chen, C., et al. (2022). Infarct-preconditioning exosomes of umbilical cord mesenchymal stem cells promoted vascular remodeling and neurological recovery after stroke in rats. Stem Cell Res Ther 13:378. doi: 10.1186/s13287-022-03083-9
Yuan, H., Liu, J., Gu, Y., Ji, X., and Nan, G. (2022). Intermittent hypoxia conditioning as a potential prevention and treatment strategy for ischemic stroke: current evidence and future directions. Front. Neurosci. 16:1067411. doi: 10.3389/fnins.2022.1067411
Zhang, D., Cai, G., Liu, K., Zhuang, Z., Jia, K., Pei, S., et al. (2021). Microglia exosomal miRNA-137 attenuates ischemic brain injury through targeting Notch1. Aging (Albany NY) 13, 4079–4095. doi: 10.18632/aging.202373
Zhang, G., Chen, L., Guo, X., Wang, H., Chen, W., Wu, G., et al. (2018). Comparative analysis of microRNA expression profiles of exosomes derived from Normal and hypoxic preconditioning human neural stem cells by next generation sequencing. J. Biomed. Nanotechnol. 14, 1075–1089. doi: 10.1166/jbn.2018.2567
Zhang, L., Wei, W., Ai, X., Kilic, E., Hermann, D. M., Venkataramani, V., et al. (2021). Extracellular vesicles from hypoxia-preconditioned microglia promote angiogenesis and repress apoptosis in stroke mice via the TGF-beta/Smad2/3 pathway. Cell Death Dis. 12:1068. doi: 10.1038/s41419-021-04363-7
Zhang, H., Wu, J., Wu, J., Fan, Q., Zhou, J., Wu, J., et al. (2019). Exosome-mediated targeted delivery of miR-210 for angiogenic therapy after cerebral ischemia in mice. J. Nanobiotechnology 17:29. doi: 10.1186/s12951-019-0461-7
Zhu, S., Huang, H., Liu, D., Wen, S., Shen, L., and Lin, Q. (2022). Augmented cellular uptake and homologous targeting of exosome-based drug loaded IOL for posterior capsular opacification prevention and biosafety improvement. Bioact. Mater. 15, 469–481. doi: 10.1016/j.bioactmat.2022.02.019
Keywords: exosome, stroke, hypoxia, intermittent hypoxic training (IHT), microglia, Neuroprotection
Citation: Ryou M-G and Burton S (2024) Intermittent hypoxic training – derived exosomes in stroke rehabilitation. Front. Integr. Neurosci. 18:1475234. doi: 10.3389/fnint.2024.1475234
Edited by:
Vinay V. Parikh, Temple University, United StatesReviewed by:
Sandhya Bansal, St. Joseph’s Hospital and Medical Center, United StatesCopyright © 2024 Ryou and Burton. This is an open-access article distributed under the terms of the Creative Commons Attribution License (CC BY). The use, distribution or reproduction in other forums is permitted, provided the original author(s) and the copyright owner(s) are credited and that the original publication in this journal is cited, in accordance with accepted academic practice. No use, distribution or reproduction is permitted which does not comply with these terms.
*Correspondence: Myoung-Gwi Ryou, cnlvdUB0YXJsZXRvbi5lZHU=