- 1Traditional Chinese Medicine Hospital Affiliated with Xinjiang Medical University, Ürümqi, China
- 2Postdoctoral Workstation of Traditional Chinese Medicine Hospital of Xinjiang Uygur Autonomous Region, Ürümqi, China
- 3Guizhou University of Traditional Chinese Medicine, Guiyang, China
- 4College of Traditional Chinese Medicine, Xinjiang Medical University, Ürümqi, China
- 5Xinjiang Urumqi Traditional Chinese Medicine Hospital, Ürümqi, China
Background: Insomnia is a clinical problem of significant public health importance; however, the underlying pathogenesis of this disorder is not comprehensively understood.
Methods: To identify potential treatment targets and unfold one of the gaps that were involved in insomnia pathological mechanisms, we employed a tandem mass tag-based (TMT) quantitative proteomics technology to detect differentially expressed proteins (DEPs) in serum from patients with insomnia and controls. DEPs were further analyzed by bioinformatics platforms. In addition, parallel reaction monitoring (PRM) was used to verify the TMT results.
Results: Patients with insomnia had poorer sleep quality compared with healthy controls. A total of 106 DEPs were identified among patients with insomnia and controls. They were mainly enriched in immune and inflammation-related biological functions and signaling pathways. Using the protein–protein interaction network, we screened the 10 most connected proteins as key DEPs. We predicted that four key DEPs were subject to targeted regulation by natural compounds of herbs. Eight key DEPs were validated using PRM in an additional 15 patients with insomnia and 15 controls, and the results also supported the experimental findings.
Conclusion: We identified aberrantly expressed proteins in insomnia that may be involved in the immune-inflammatory response. The 10 key DEPs screened may be potential targets for insomnia, especially FN1, EGF, HP, and IGF1. The results of this study will broaden our understanding of the pathological mechanisms of insomnia and provide more possibilities for pharmacotherapy.
Introduction
Insomnia is a public health problem and one of the most frequent sleep disorders in patients with other neurological disorders (Sutton, 2021). This disorder is characterized by difficulties in guaranteeing sleep quality, initiating or maintaining sleep, and severe distress and impairment of daytime functioning (Bollu and Kaur, 2019). The prevalence of insomnia disorder is approximately 10–20%, of which approximately 50% have a chronic course (Buysse, 2013). Patients with insomnia are often accompanied by higher levels of depression, anxiety, physical pain and discomfort, cognitive impairment, and cardiovascular disease (Javaheri and Redline, 2017; Riemann et al., 2020). These disorders impose a personal and economic burden that is difficult to quantify (Wickwire et al., 2020). However, the underlying pathogenesis of this disorder is not completely understood. Therefore, it is necessary to study the mechanism of insomnia to better understand its pathophysiology and produce effective therapeutic agents.
It is important to note that both pharmacological and non-pharmacological treatments have their advantages and disadvantages, and neither treatment is suitable for patients with all types of insomnia (Hassinger et al., 2020). Molecular factors of sleep–wake regulation include sleep-promoting chemicals, such as norepinephrine and histamine, γ-aminobutyric acid (GABA), melatonin, etc. (Levenson et al., 2015). Abnormal regulation of these chemicals is one of the possible mechanisms of insomnia. Studies have shown that insomnia can alter inflammatory processes (Li et al., 2020). Inflammatory factors in turn may increase the response to insomnia and contribute to the etiology of physical and psychiatric disorders (Ferrie et al., 2013; Dolsen et al., 2019). Interventions to improve sleep and reduce inflammation may have a positive impact on health in the general population.
Lots of studies have reported that many proteins changed after insomnia, which were wisely related to the complex and dynamic pathophysiology (Xu et al., 2020; Wang et al., 2021). Quantitative proteomics has a popular profiling approach in protein biomarker discovery and protein alterations so far (Jha and Trivedi, 2019). Currently, with the advent of quantitative proteomic technologies using an isobaric labeling strategy, it becomes possible to quantify several proteins in a single experiment for the comparative study of global protein regulation across various biological samples, and this method has been widely applied to elucidate disease mechanisms (Kibinge et al., 2020; Kohlschmidt et al., 2021).
Identification of novel pathogenesis and new traditional Chinese medicines is made more possible by proteomics. Herein, we used TMT-based quantitative proteomics to explore the potential mechanisms and therapeutic targets for insomnia. We identified disordered proteins associated with insomnia by comparing the proteomes of patients with insomnia and controls. Based on the bioinformatic analysis, we aimed to reveal the novel processes involved in insomnia.
Materials and methods
Participants and selection criteria
All study protocols were approved by the Ethics Committee of the First Affiliated Hospital of Xinjiang Medical University, Urumqi, China (Grant No. 20120220-133). Written informed consent was signed by eligible participants (or their legal guardians). All participants with 40 primary insomnia complaints and 4 well-sleeping controls were collected from the First Affiliated Hospital of Xinjiang Medical University and Traditional Chinese Medicine Hospital Affiliated with Xinjiang Medical University. Patients who met the inclusion criteria were included in the study. The diagnosis was provided by the same doctor.
Patients were recruited to meet the following inclusion criteria: (i) Meeting the Diagnostic and Statistical Manual of Mental Disorders (fifth edition) criteria for insomnia disorder (American Psychiatric Association [APA], 2013); (ii) age ≥20 and ≤50 years. The exclusion criteria were as follows: (i) Use of psychotropic substances or not discontinued for more than 2 weeks; (ii) Insomnia caused by drinking alcohol and taking central stimulant drugs; (iii) A history of major neurological, psychiatric, or other medical disorders; and (iv) Pregnancy or lactation. Additionally, healthy controls were recruited from healthy volunteers.
Pittsburgh Sleep Quality Index (PSQI) was calculated for the patients. Polysomnography (PSG) data were used to assess standard sleep architecture variables. Healthy controls self-reported that they were not having any symptoms of insomnia.
Blood sample collection and preparation
The subjects were fasted and watered for at least 12 h before drawing blood at 7–8 am. The blood samples were taken specifically for this study and coded to remain anonymous. A 3-ml blood serum sample was obtained from each enrolled subject. The blood samples were centrifuged at 2,000 × g for 15 min (4°C) and the serum was aliquoted. Then, the serum sample was divided into 0.5 ml aliquots and stored in the freezer (−80°C) until further analyses.
Serum samples from five patients with insomnia (G group) and five healthy controls (F group) randomly selected from all participants were analyzed by proteomics in the discovery phase. Furthermore, additionally, serum samples from 15 patients with insomnia and 15 healthy controls were randomly selected from all participants for parallel reaction monitoring (PRM) validation.
Tandem mass tag-based quantitative proteomics
Pooled serum samples were generated by combining the same volume of individual serum samples in each group. The high-abundance proteins in the serum of each group were removed using the Pierce™ Top 12 Abundant Protein Depletion Spin Columns Kit (Thermo Fisher, Waltham, MA, United States). Finally, Protein concentration was done using a BCA kit (Sigma, St. Louis, MO, United States) according to the manufacturer’s instructions. For each sample, 50 μg of serum proteins was reduced using 5 mM dithiothreitol (Thermo Fisher, United States), and alkylated using 11 mM iodoacetamide (Sigma, United States). The proteins were then diluted with 50 mM Tris-HCl (pH 8.0) to reduce urea concentration and digested with trypsin. The resulting peptide was desalted by Strata X C18 SPE column (Phenomenex) and vacuum-dried. The peptide was reconstituted and processed according to the manufacturer’s protocol (Thermo Fisher, Waltham, MA, United States) for the TMT kit.
The mixed peptides were fractionated into fractions by high pH reverse-phase HPLC using Thermo Betasil C18 column (5 μm particles, 10 mm ID, 250 mm length). Then, the TMT-labeled tryptic peptides were reconstituted with 0.1% formic acid (solvent A) and loaded onto a homemade reversed-phase analytical column (15 cm length, 75 μm i.d.). The peptides were separated by UPLC and implanted into the NSI ion source for ionization, and then, analyzed by tandem mass spectrometry (MS/MS) in Q Exactive™ Plus (Thermo). The electrospray voltage was set to 2 kV. The scanning range of the primary-level MS was 350–1,800 m/z, and the scanning resolution is 70,000. The scanning range of the second-level MS was 100 m/z with a resolution of 17,500.
Proteomics data analysis
The resulting MS/MS data were processed using MaxQuant (v.1.5.2.8). The retrieval parameters were set as: the database was human_swissprot_9606; enzyme mode was set to Trypsin/P; maximum missed cleavage number was 2; the mass tolerance for precursor ions was set as 20 ppm in the first search and 5 ppm in the main search; the mass tolerance for fragment ions was set as 0.02 Da; the signal threshold was set as 100,00 ions/s; maximum injection time set to 200 ms; false discovery rate (FDR) was adjusted to <1%. Protein quantification data with p < 0.05, ratio-fold change > 1.2 (upregulation) or <0.83 (downregulation) were considered as significant differentially expressed proteins (DEPs). Data in this study was submitted to the PRIDE database with an internal ID of PXD026610.
Enrichment analysis
To annotate the potential functions of DEPs, the GO and KEGG pathway enrichment analysis was performed using the clusterProfiler R package (Yu et al., 2012). A p < 0.05 was considered statistically significant. Gene set variation analysis (GSVA) was performed using the GSVA R package to evaluate the activation of the KEGG pathway. In addition, the hallmark was obtained using a gene set enrichment analysis (GSEA) software.
Protein–protein interaction network
All DEPs were searched against the STRING database version 11.01 for protein–protein interaction (PPI) networks. The PPI network was visualized by the Gephi software. To identify the connectivity degree of proteins in the PPI network, the top ten proteins with the largest degree were considered as the key DEPs. The interaction network of key proteins was displayed with the Cytoscape software.
The Traditional Chinese Medicine Systems Pharmacology (TCMSP) database (Ru et al., 2014) was used to obtain natural compounds of herbal medicines. According to the criteria suggested by the TCMSP, we predicted natural compounds for key DEPs.
Parallel reaction monitoring-mass spectrometry analysis
Targeted proteomics analysis, the PRM, was used to validate key DEPs screened by TMT analysis. PRM analysis defined the signature peptides of the target protein according to the TMT data. A unique peptide included for analysis in a minimum number of subjects was identified. More than two unique peptides per protein were used for quantification using fragment ion peak areas of peptides. The serum samples were prepared, reduced, alkylated, and digested with trypsin following the protocol for TMT analysis. The obtained peptide mixtures were separated and analyzed with nano-UPLC (EASY-nLC 1000) and MS/MS in Q Exactive™ Plus (Thermo). The resulting MS data were processed using the Skyline software (v.3.6). Peptide parameters are as follows: enzyme was set as Trypsin [KR/P]; Max missed cleavage was 0. The peptide length was set at 7–25; the alkylation of cysteine was set as a fixed modification. The parameters of transition were set as follows: precursor charges were set to 2, 3; ion charges were set as 1; ion types were set as b, y. The fragment ions were set from ion 3 to the last ion, and the ion match tolerance was set as 0.02 Da.
Statistics
Statistical analysis was analyzed by the SPSS software (version 23.0). Results were compared by independent t-test or chi-square analyses. All data were expressed as mean ± SD, p < 0.05 was considered statistically significant.
Results
Baseline characteristics
A flowchart of the study is shown in Figure 1. Participants in this study included primary insomnia complaints (n = 40) and well-sleeping controls (n = 40). The demographic and baseline data of registered subjects are shown in Table 1. Participants tended to be average-aged of 41.85 ± 5.27 in the insomnia group and average-aged of 41.06 ± 7.64 in the control group. Likewise, there were no significant differences between the insomnia and controls on sex and body mass index. In terms of sleep disorders, patients with insomnia had significantly worse sleep quality than healthy controls.
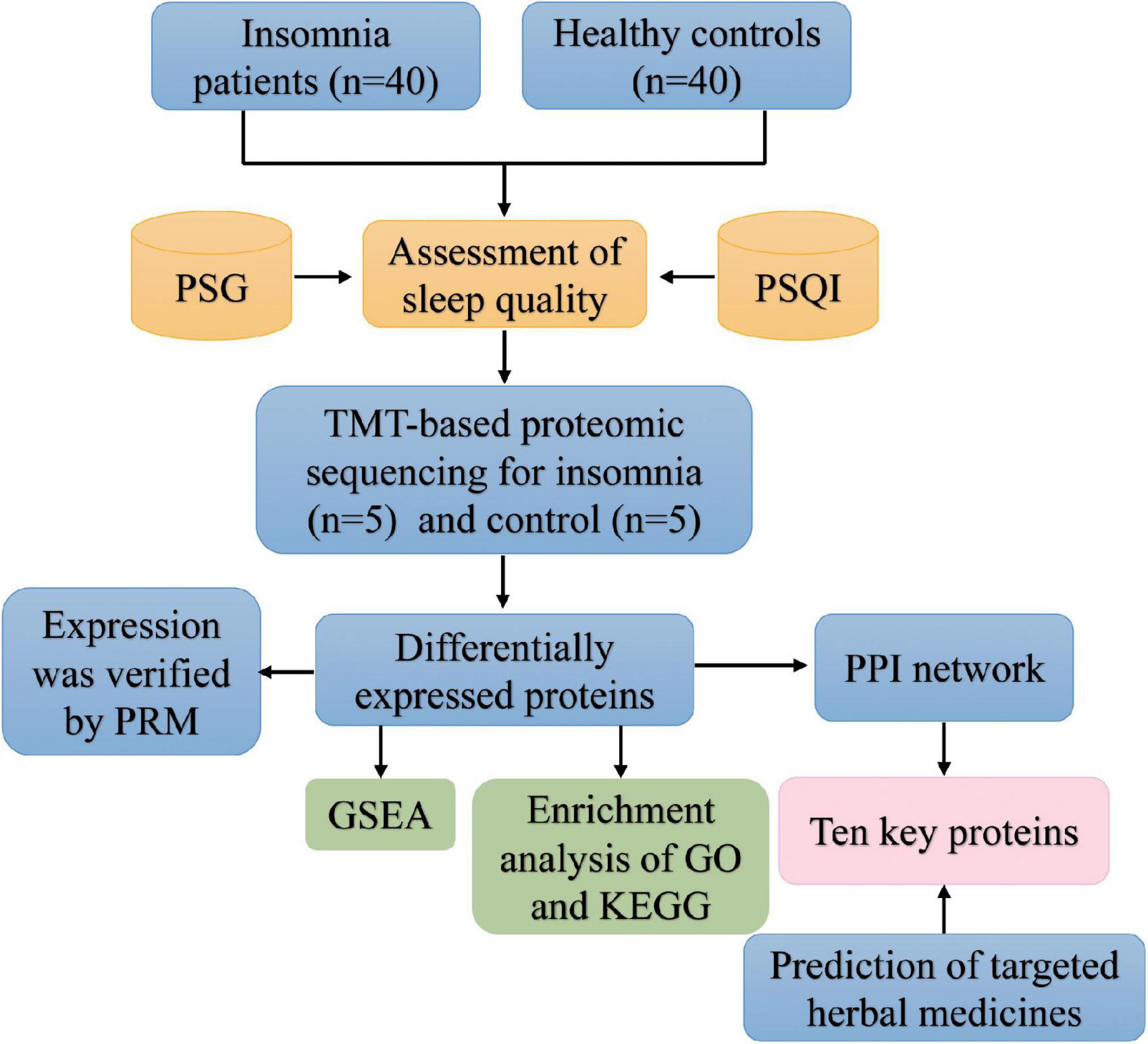
Figure 1. The flowchart of this study. GO, Gene Ontology; GSEA, gene set enrichment analysis; KEGG, Kyoto Encyclopedia of Genes and Genomes; PPI, protein–protein interaction; PRM, parallel reaction monitoring; PSG, polysomnography; PSQI, Pittsburgh Sleep Quality Index.
Tandem mass tag analysis of differentially expressed proteins
We then performed TMT quantitative proteomics using five primary insomnia complaints and five controls (Table 2). To identify aberrantly expressed proteins in insomnia, we performed a differential analysis of expressed proteins between insomnia and control groups. First, 276,800 secondary spectra were obtained by TMT quantitative proteomics, and the number of available spectra was 16,913. A total of 5,218 peptide segments were identified by spectrogram analysis, in which the specific peptide segment was 5,020. We identified 836 proteins, 741 of which were quantifiable. A total of 106 DEPs were screened out of quantifiable proteins by the criteria described above (Figure 2A and Supplementary Table 1). We found that 52 proteins were upregulated and 54 proteins were downregulated in the insomnia group compared with controls (Figure 2B).
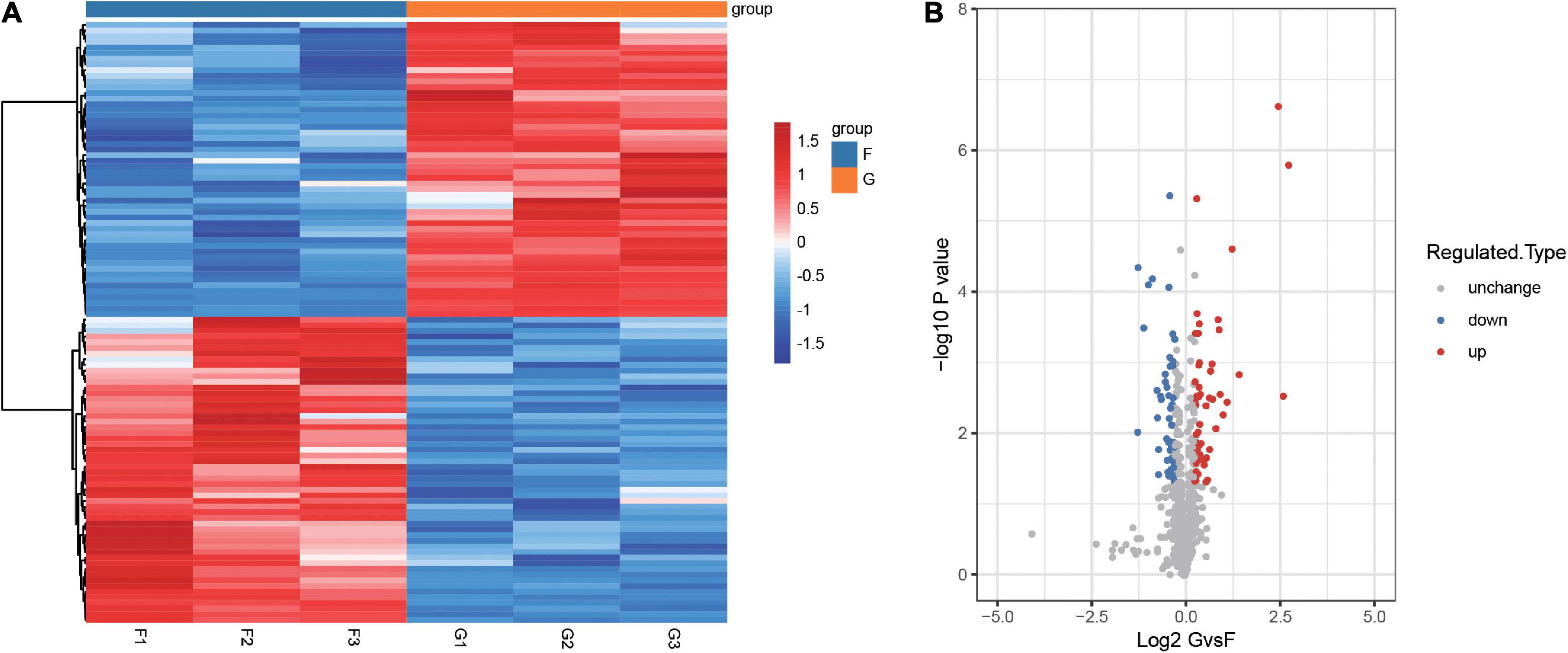
Figure 2. Identification of differentially expressed proteins between insomnia patients and controls. (A) Heatmap clustered with unsupervised for differentially expressed proteins between patients with insomnia and controls. F1-3 and G1-3 refer to technical replicates. (B) Volcano plot of differentially expressed proteins between patients with insomnia and controls. Red is upregulated proteins and blue is downregulated. G, insomnia group; F, control group.
Biological functions of differentially expressed proteins
To further explore the potential mechanism in patients with insomnia, we performed an enrichment analysis of DEPs. In the results of GO enrichment, the biological process (BP), cellular component (CC), and molecular function (MF) were included (Figure 3A). The DEPs are mainly located in platelet degranulation, neutrophil-mediated immunity, and extracellular matrix organization of BP. For the CC, DEPs were mainly enriched in secretory granule lumen, platelet alpha granule, and endoplasmic reticulum lumen. As for MF, DEPs were mainly involved in serine-type endopeptidase activity, endopeptidase activity, and collagen binding.
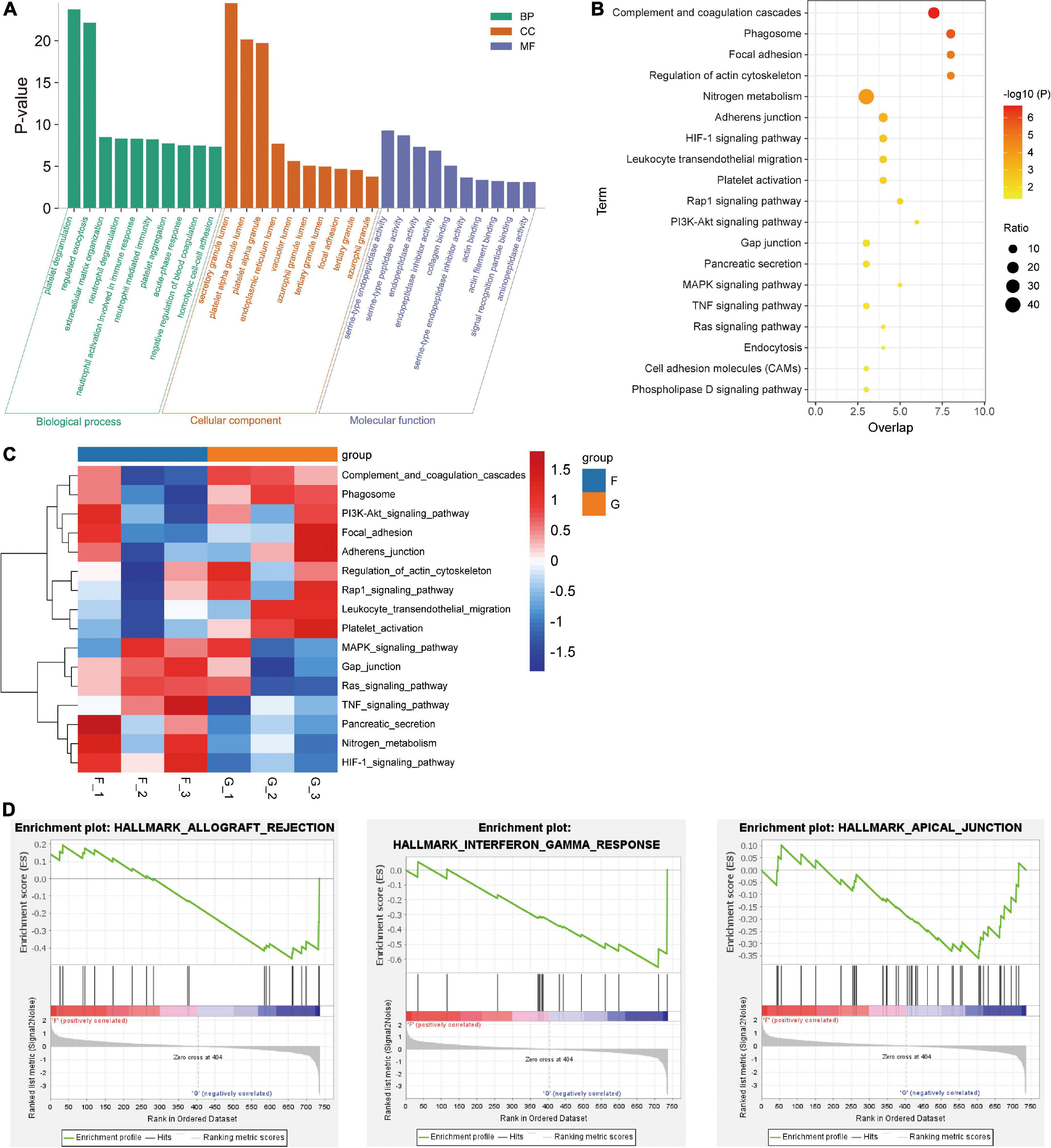
Figure 3. Identification of GO and signaling pathways enriched by differentially expressed proteins. (A) Top 10 terms of BP, CC, and MF enriched in insomnia patients. (B) KEGG pathways of DEPs significantly enriched. (C) Heatmap clustered with unsupervised for the activated or inhibited KEGG pathways were calculated by GSVA. (D) The significant hallmark terms positively corrected with patients with insomnia. G, insomnia group; F, control group.
Meanwhile, 19 KEGG pathways were identified based on DEPs (Figure 3B), which mainly included complement and coagulation cascades, HIF-1 signaling pathway, and focal adhesion. We then identified the expression of these signaling pathways in different samples by GSVA (Figure 3C). Complement and coagulation cascades and focal adhesion were activated in patients with insomnia, and the HIF-1 signaling pathway was inhibited. In addition, GSEA was used to gain the hallmark (Figure 3D). We found that interferon-gamma response, allograft rejection, and apical junction were markedly enriched in the insomnia group.
Protein–protein interaction analysis
STRING database was used to determine the relationship between DEPs. Of the 106 identified DEPs, 83 DEPs were interconnected (Figure 4A). The network exhibited a complex relationship among proteins. In addition, the Cytoscape software was served for PPI analysis of DEPs, the top 10 proteins with the largest connection degree (FN1, APP, HP, MMP9, EGF, FGG, FGB, AHSG, FGA, and IGF1) in the network were identified as key DEPs (Figure 4B). Key DEPs may have a huge impact on insomnia. Notably, we utilized the TCMSP database to identify potentially targeted drugs for key DEPs. In the results, we found FN1, EGF, HP, and IGF1 may be targeted by many natural compounds in herbal medicines (Figure 4C).
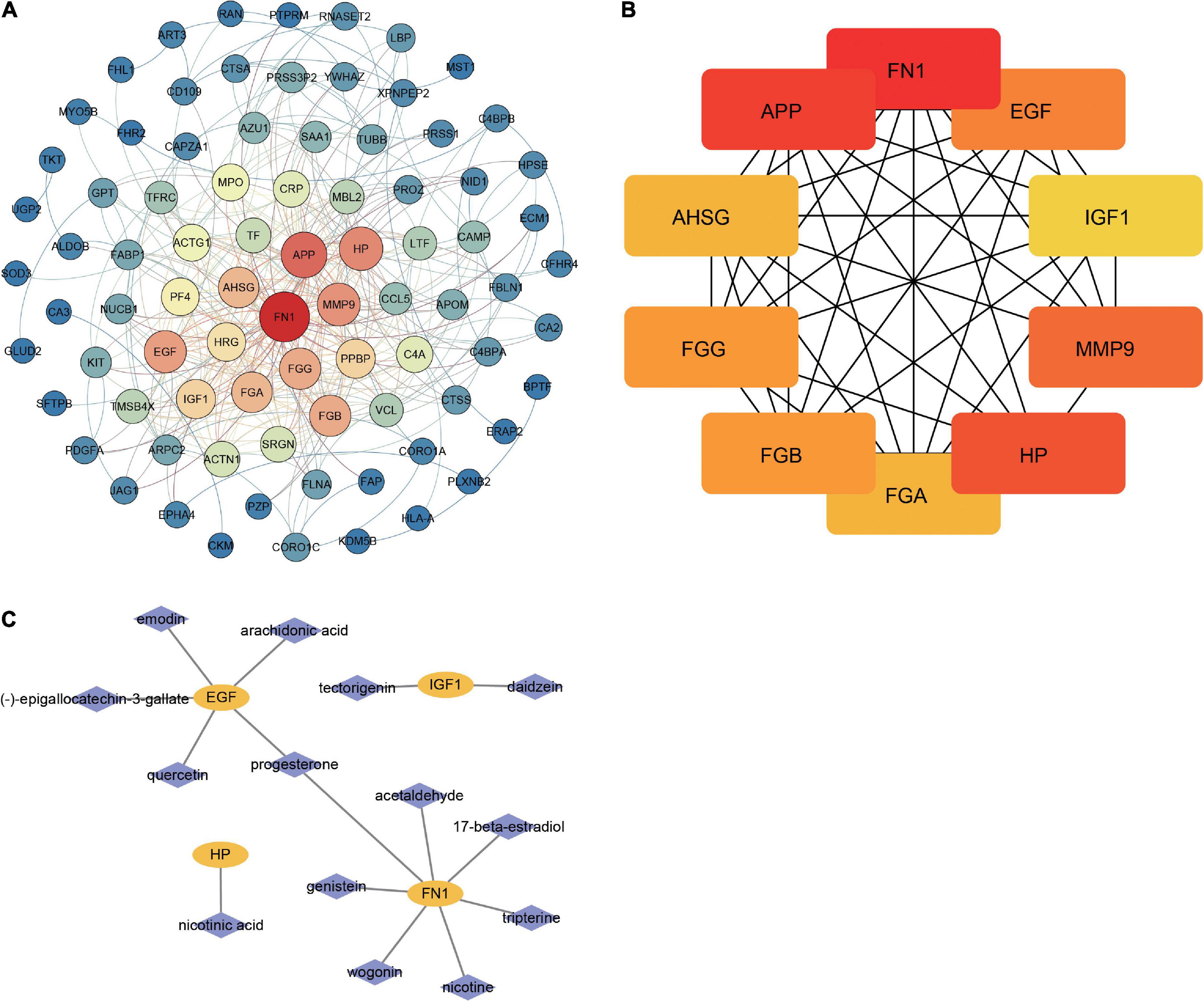
Figure 4. Identification of key proteins involved in insomnia. (A) The PPI network of DEPs. Nodes are colored from blue to red, indicating increased connectivity of proteins. (B) Top 10 proteins with the highest connectivity in the network. (C) Prediction network for natural compounds of herbal medicines targeting key DEPs.
Validation of differentially expressed proteins
For TMT quantitative proteomics, we selected 15 patients with insomnia and 15 controls (Table 3). In the results, HP, FGA, FGG, FGB, and MMP9 were upregulated in patients with insomnia, and FN1, APP, EGF, AHSG, and IGF1 were downregulated compared with controls (Figure 5). Importantly, through PRM analysis for 106 DEPs, we quantified 34 DEPs (Table 4). Among them, the differential expression for eight key DEPs was validated. Among them, HP, MMP9, FGA, FGB, and FGG were validated as upregulated, and APP, AHSG, and IGF1 were downregulated in patients with insomnia.
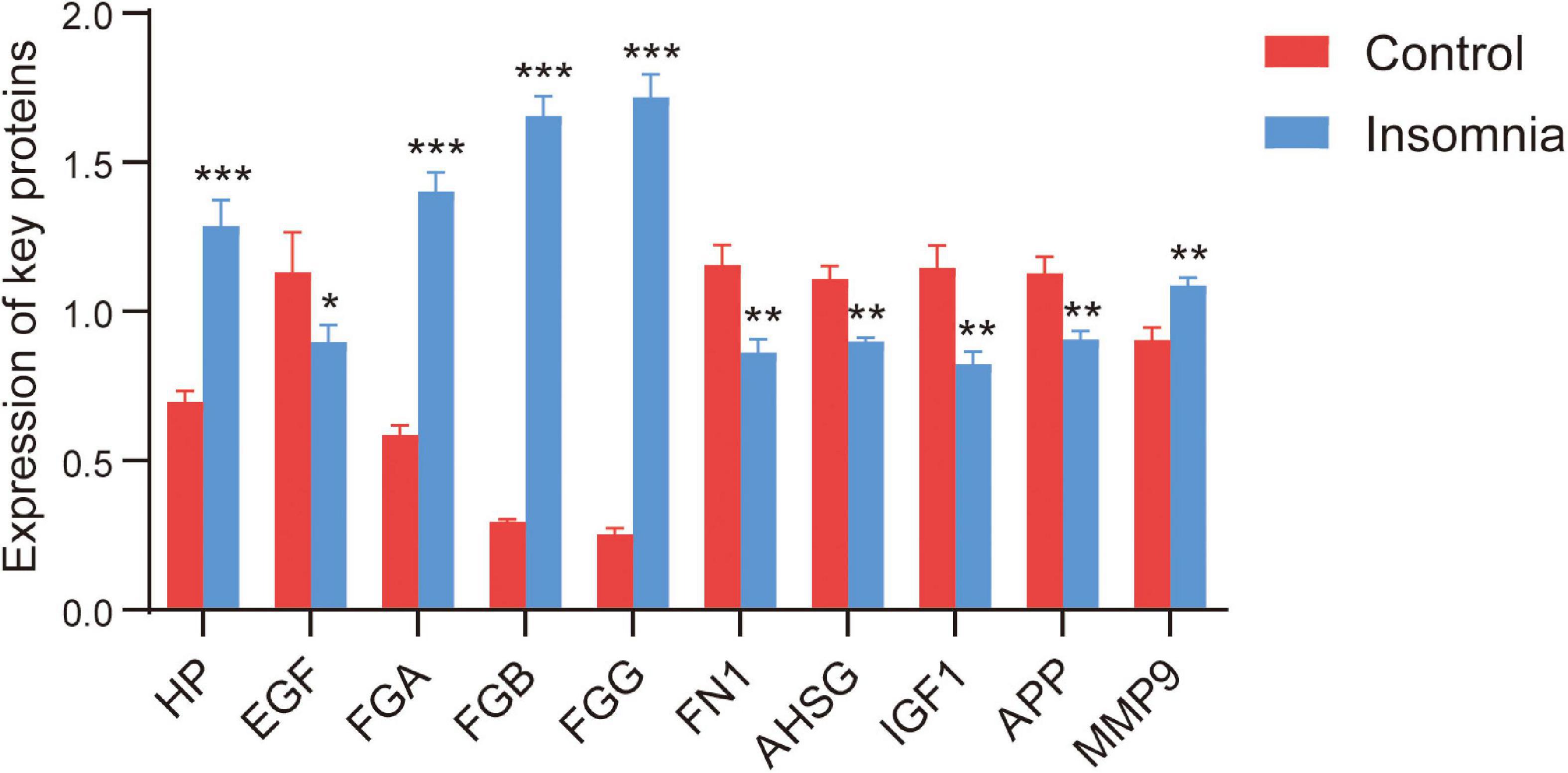
Figure 5. The expression of key DEPs. The expression of key DEPs in insomnia patients and controls in TMT-based proteomics. *p < 0.05, **p < 0.01, ***p < 0.001.
Discussion
Although evidence-based approaches to the assessment and treatment of insomnia have been developed, all are based on clinical consensus (Kalmbach et al., 2019). Further progress depends on a better understanding of the etiology and pathophysiology of mental health problems. Despite recent advances in the understanding of the nature, etiology, and pathophysiology of insomnia, there is no generally accepted model (Levenson et al., 2015). This study combined relevant biological information to identify important proteins in patients with insomnia and tried to reveal herbal medicines for targeted therapy.
The total sleep time of the patients with insomnia who participated in this study was short, while insomnia time and awakening times were significantly higher than those of the control group, which was consistent with the study of Johann et al. (2017). Interestingly, patients with insomnia had no significant difference between rapid eye movement sleep (REM) and controls, whereas non-rapid eye movement sleep (NREM) was significantly lower than controls. Most insomnia research has focused on quantitative electroencephalogram (EEGs) obtained during NREM sleep, rather than REM sleep, and differences in the control of insomnia have largely focused on EEG activity in the NREM during the night (Buysse et al., 2008).
Based on the proteomic quantification results, we identified a large number of DEPs. The enrichment results of DEPs identified biological functions and signaling pathways related to insomnia. It has been shown that insufficient sleep has a great impact on our immune system (Irwin and Opp, 2017). Christoffersson et al. (2014) found that a decreased sleep also decreased reactive oxygen species production by neutrophils. However, the existing studies on the relationship between insomnia and neutrophils are still less, and the specific effect mechanisms between the two need to be further investigated. Platelet degranulation is often associated with the innate immune response and may be involved in the regulation of physiological sleep (Besedovsky et al., 2019). Extracellular matrix (ECM) organization has been reported to be involved in the occurrence and development of insomnia (Xu et al., 2020). MMP9 (matrix metallopeptidase 9) is involved in the proteolytic modification of ECM proteins, which affects neuronal plasticity and the synaptic properties of neurons (Hayashi et al., 2013). In addition, melatonin has also a regulatory effect on the MMP9 pathway and ECM remodeling (Jensen et al., 2021). These results further confirmed a link between the immune-inflammatory response and insomnia.
In the KEGG pathway results, complement and coagulation cascades, and focal adhesion were activated by DEPs in patients with insomnia, and the HIF-1 signaling pathway was inhibited. Complement and coagulation play a role in the pathophysiology of REM sleep behavior disorder (Mondello et al., 2018). Complement and coagulation cascades were shown to stimulate the production of circadian regulatory hormones (Budkowska et al., 2018). Proteins are regulated by the coagulation cascade can disturb the homeostasis of synapses, affecting the pathophysiology of the central nervous system (CNS) (De Luca et al., 2017). Targeting the Ros-HIF-1 axis may enable the treatment of obstructive sleep apnea-related cardiovascular complications (Belaidi et al., 2016). In addition, the study showed that nurses with short sleep duration and poor sleep quality had elevated interferon gamma levels (Bjorvatn et al., 2020). Excessive secretion of interferon-gamma in the periphery and the brain triggers inflammatory cascades involved in aging and aging-related medical and psychiatric disorders (Oxenkrug, 2011).
Additively, this study also explored the interaction network between DEPs, and in turn screened out key DEPs (FN1, APP, HP, MMP9, EGF, FGG, FGB, AHSG, FGA, and IGF1) that may have important effects on insomnia. Importantly, upregulated HP, MMP9, FGA, FGB, FGG, and downregulated APP, AHSG, and IGF1 were validated by PRM analysis. HP (haptoglobin) is an inflammatory marker whose expression levels are significantly increased in patients with insomnia (Maes et al., 1993; Mominoki et al., 2005). MMP9 is an inflammatory protein that proved to be a potential target of action in the treatment of insomnia (Lee et al., 2012). High expression of fibrinogen is associated with more sleep problems (von Kanel et al., 2018). APP (amyloid-beta precursor protein) mutations severely affect sleep status in Alzheimer’s disease model mice (Huitron-Resendiz et al., 2005). Acute sleep deprivation elevates amyloid β-protein (Aβ) levels in mouse interstitial fluid and human cerebrospinal fluid (CSF) (Shokri-Kojori et al., 2018). In cardiovascular disease, the levels of AHSG (alpha 2-HS glycoprotein) are significantly lower than in controls (Zheng et al., 2014). However, since direct reports of its involvement in insomnia have not been found, it may be a new marker for insomnia. Reduced levels of IGF1 (insulin-like growth factor 1) were found to be associated with poor sleep quality, excessive daytime sleepiness, and sleep apnea (Reddy et al., 2014). Our results further emphasize abnormalities of these proteins in the process of insomnia.
The pharmacotherapy of insomnia has been changing over the past few decades, and traditional Chinese medicine (TCM) has been widely used for the treatment of insomnia due to its advantages, such as high efficacy, no drug resistance, and low toxicity (Singh and Zhao, 2017; Yue et al., 2019). For drug prediction of key DEPs, FN1, EGF, HP, and IGF1 were found to be potentially target-regulated by natural compounds. Therefore, they may be potential herbal therapeutic targets for patients with insomnia. Genistein contained in soja (Glycine max) and red clover (Trifolium pratense L.) was beneficial in antioxidant, anti-inflammatory, cardiovascular, and anxiolytic-like effects (Sharifi-Rad et al., 2021). Recent studies have shown that genistein can improve brain function through the blood–brain barrier, antagonize Aβ protein toxicity, and has neuroprotective effects (Duan et al., 2021). The 17-beta-estradiol is reported to moderately reduce insomnia symptoms and improve subjective sleep quality (Ensrud et al., 2015). Cohrs et al. (2014) found that sleep quality and sleep duration are impaired in nicotine-dependent inhalers. Acetaldehyde, a central nervous system depressant, has sedative, as well as sleep–wake cycle disturbances (Plescia et al., 2021). Wogonin inhibits inflammatory responses and, in turn, exerts neuroprotective effects (Piao et al., 2004). Arachidonic acid is a precursor for the production of prostaglandin PGD2, which is essential for sleep quality (Sejbuk et al., 2022). Emodin has various pharmacological properties, including anti-inflammatory, antioxidant, and neuroprotective effects (Li et al., 2021). Quercetin is one of the most potent antioxidants of plant origin, and its neuroprotective effects have been reported in several in vitro studies (Khan et al., 2019). This may provide new or improved therapeutic strategies in clinical practice.
This study also has some limitations. Limited by the number of experimental samples, not many DEPs were identified, although our filter condition was set at p < 0.05. The systemic findings reported here with CNS changes were important, while some of the altered serum proteins may reflect changes occurring in the brain, this is likely not the case for all proteins. In addition, the identified protein changes may be a cause or a consequence of insomnia; this need to be further explored in in vivo and in vitro experiments. In a follow-up study, we will focus on the expression changes of 10 key DEPs in patients with different degrees of insomnia in a large sample size.
Conclusion
In this study, we applied TMT quantitative proteomic and bioinformatic analysis to explore possible mechanisms of patients with insomnia. After a series of detection, we discovered 106 DEPs associated with complement and coagulation cascades and HIF-1 signaling pathway. Then, we screened 10 key DEPs and validated them by PRM. This study has the potential to add to the current knowledge of pathophysiology of insomnia.
Data availability statement
The datasets presented in this study can be found in online repositories. The name of the repository and accession number can be found below: ProteomeXchange Consortium, http://www.proteomexchange.org/, PXD026610.
Ethics statement
Written informed consent was not obtained from the individual(s) for the publication of any potentially identifiable images or data included in this article.
Author contributions
TL designed this work, collected samples, and drafted the manuscript. GW and XL performed data analysis and pathway analysis. ZL and XR interpreted data and conducted experiments. DY and WZ performed the statistical analyses. All authors contributed to manuscript development and had approved the final and submitted draft of the manuscript.
Funding
This work was supported by the China Postdoctoral Science Foundation (Grant No. 2020M673545); the Postdoctoral program of Affiliated Hospital of traditional Chinese medicine of Xinjiang Medical University (Grant No. zyy2019bsh2); the National Natural Science Foundation of China (Grant Nos. 81260526, 81560762, and 81960837); the Key disciplines of TCM theories in the 12th Five Year Plan of State Administration of traditional Chinese medicine; and the emerging key specialty of TCM strategy in Xinjiang Uygur Autonomous Region during the 13th five-year plan.
Conflict of interest
The authors declare that the research was conducted in the absence of any commercial or financial relationships that could be construed as a potential conflict of interest.
Publisher’s note
All claims expressed in this article are solely those of the authors and do not necessarily represent those of their affiliated organizations, or those of the publisher, the editors and the reviewers. Any product that may be evaluated in this article, or claim that may be made by its manufacturer, is not guaranteed or endorsed by the publisher.
Acknowledgments
We thank all volunteers who participated in the study.
Supplementary material
The Supplementary Material for this article can be found online at: https://www.frontiersin.org/articles/10.3389/fnint.2022.936955/full#supplementary-material
Supplementary Table 1 | The differentially expressed proteins between patients with insomnia and controls.
Footnotes
References
American Psychiatric Association [APA] (2013). Diagnostic and Statistical Manual of Mental Disorders (DSM-5§). Virginia: American Psychiatric Pub. doi: 10.1176/appi.books.9780890425596
Belaidi, E., Morand, J., Gras, E., Pepin, J. L., and Godin-Ribuot, D. (2016). Targeting the ROS-HIF-1-endothelin axis as a therapeutic approach for the treatment of obstructive sleep apnea-related cardiovascular complications. Pharmacol. Ther. 168, 1–11. doi: 10.1016/j.pharmthera.2016.07.010
Besedovsky, L., Lange, T., and Haack, M. (2019). The Sleep-Immune Crosstalk in Health and Disease. Physiol. Rev. 99, 1325–1380. doi: 10.1152/physrev.00010.2018
Bjorvatn, B., Axelsson, J., Pallesen, S., Waage, S., Vedaa, O., Blytt, K. M., et al. (2020). The Association Between Shift Work and Immunological Biomarkers in Nurses. Front. Public Health 8:415. doi: 10.3389/fpubh.2020.00415
Budkowska, M., Ostrycharz, E., Wojtowicz, A., Marcinowska, Z., Wozniak, J., Ratajczak, M. Z., et al. (2018). A Circadian Rhythm in both Complement Cascade (ComC) Activation and Sphingosine-1-Phosphate (S1P) Levels in Human Peripheral Blood Supports a Role for the ComC-S1P Axis in Circadian Changes in the Number of Stem Cells Circulating in Peripheral Blood. Stem Cell Rev. Rep. 14, 677–685. doi: 10.1007/s12015-018-9836-7
Buysse, D. J., Germain, A., Hall, M. L., Moul, D. E., Nofzinger, E. A., Begley, A., et al. (2008). EEG spectral analysis in primary insomnia: NREM period effects and sex differences. Sleep 31, 1673–1682. doi: 10.1093/sleep/31.12.1673
Christoffersson, G., Vagesjo, E., Pettersson, U. S., Massena, S., Nilsson, E. K., Broman, J. E., et al. (2014). Acute sleep deprivation in healthy young men: impact on population diversity and function of circulating neutrophils. Brain Behav. Immun. 41, 162–172. doi: 10.1016/j.bbi.2014.05.010
Cohrs, S., Rodenbeck, A., Riemann, D., Szagun, B., Jaehne, A., Brinkmeyer, J., et al. (2014). Impaired sleep quality and sleep duration in smokers-results from the German Multicenter Study on Nicotine Dependence. Addict. Biol. 19, 486–496. doi: 10.1111/j.1369-1600.2012.00487.x
De Luca, C., Virtuoso, A., Maggio, N., and Papa, M. (2017). Neuro-Coagulopathy: blood Coagulation Factors in Central Nervous System Diseases. Int. J. Mol. Sci. 18:2128. doi: 10.3390/ijms18102128
Dolsen, M. R., Crosswell, A. D., and Prather, A. A. (2019). Links Between Stress, Sleep, and Inflammation: are there Sex Differences?. Curr. Psychiatry Rep. 21:8. doi: 10.1007/s11920-019-0993-4
Duan, X., Li, Y., Xu, F., and Ding, H. (2021). Study on the neuroprotective effects of Genistein on Alzheimer’s disease. Brain Behav. 11:e02100. doi: 10.1002/brb3.2100
Ensrud, K. E., Guthrie, K. A., Hohensee, C., Caan, B., Carpenter, J. S., Freeman, E. W., et al. (2015). Effects of estradiol and venlafaxine on insomnia symptoms and sleep quality in women with hot flashes. Sleep 38, 97–108. doi: 10.5665/sleep.4332
Ferrie, J. E., Kivimaki, M., Akbaraly, T. N., Singh-Manoux, A., Miller, M. A., Gimeno, D., et al. (2013). Associations between change in sleep duration and inflammation: findings on C-reactive protein and interleukin 6 in the Whitehall II Study. Am. J. Epidemiol. 178, 956–961. doi: 10.1093/aje/kwt072
Hassinger, A. B., Bletnisky, N., Dudekula, R., and El-Solh, A. A. (2020). Selecting a pharmacotherapy regimen for patients with chronic insomnia. Exp. Opin. Pharmacother. 21, 1035–1043. doi: 10.1080/14656566.2020.1743265
Hayashi, Y., Koyanagi, S., Kusunose, N., Okada, R., Wu, Z., Tozaki-Saitoh, H., et al. (2013). The intrinsic microglial molecular clock controls synaptic strength via the circadian expression of cathepsin S. Sci. Rep. 3:2744. doi: 10.1038/srep02744
Huitron-Resendiz, S., Sanchez-Alavez, M., and Criado, J. R. (2005). Sleep-wake states in transgenic mouse models overexpressing the human beta-amyloid precursor protein. Am. J. Alzheimers Dis. Other Demen. 20, 87–90. doi: 10.1177/153331750502000204
Irwin, M. R., and Opp, M. R. (2017). Sleep Health: reciprocal Regulation of Sleep and Innate Immunity. Neuropsychopharmacology 42, 129–155. doi: 10.1038/npp.2016.148
Javaheri, S., and Redline, S. (2017). Insomnia and Risk of Cardiovascular Disease. Chest 152, 435–444. doi: 10.1016/j.chest.2017.01.026
Jensen, L. D., Oliva, D., Andersson, B. A., and Lewin, F. (2021). A multidisciplinary perspective on the complex interactions between sleep, circadian, and metabolic disruption in cancer patients. Cancer Metastasis Rev. 40, 1055–1071. doi: 10.1007/s10555-021-10010-6
Jha, M. K., and Trivedi, M. H. (2019). Pharmacogenomics and Biomarkers of Depression. Handb. Exp. Pharmacol. 250, 101–113. doi: 10.1007/164_2018_171
Johann, A. F., Hertenstein, E., Kyle, S. D., Baglioni, C., Feige, B., Nissen, C., et al. (2017). Insomnia with objective short sleep duration is associated with longer duration of insomnia in the Freiburg Insomnia Cohort compared to insomnia with normal sleep duration, but not with hypertension. PLoS One 12:e0180339. doi: 10.1371/journal.pone.0180339
Kalmbach, D. A., Cheng, P., Arnedt, J. T., Cuamatzi-Castelan, A., Atkinson, R. L., Fellman-Couture, C., et al. (2019). Improving Daytime Functioning, Work Performance, and Quality of Life in Postmenopausal Women With Insomnia: comparing Cognitive Behavioral Therapy for Insomnia, Sleep Restriction Therapy, and Sleep Hygiene Education. J. Clin. Sleep Med. 15, 999–1010. doi: 10.5664/jcsm.7882
Khan, H., Ullah, H., Aschner, M., Cheang, W. S., and Akkol, E. K. (2019). Neuroprotective Effects of Quercetin in Alzheimer’s Disease. Biomolecules 10:59. doi: 10.3390/biom10010059
Kibinge, N. K., Relton, C. L., Gaunt, T. R., and Richardson, T. G. (2020). Characterizing the Causal Pathway for Genetic Variants Associated with Neurological Phenotypes Using Human Brain-Derived Proteome Data. Am. J. Hum. Genet. 106, 885–892. doi: 10.1016/j.ajhg.2020.04.007
Kohlschmidt, N., Elbracht, M., Czech, A., Hausler, M., Phan, V., Topf, A., et al. (2021). Molecular pathophysiology of human MICU1 deficiency. Neuropathol. Appl. Neurobiol. 47, 840–855. doi: 10.1111/nan.12694
Lee, T. H., Jung, C. H., and Lee, D. H. (2012). Neuroprotective effects of Schisandrin B against transient focal cerebral ischemia in Sprague-Dawley rats. Food Chem. Toxicol. 50, 4239–4245. doi: 10.1016/j.fct.2012.08.047
Levenson, J. C., Kay, D. B., and Buysse, D. J. (2015). The pathophysiology of insomnia. Chest 147, 1179–1192. doi: 10.1378/chest.14-1617
Li, Y., Zhang, B., Zhou, Y., Wang, D., Liu, X., Li, L., et al. (2020). Gut Microbiota Changes and Their Relationship with Inflammation in Patients with Acute and Chronic Insomnia. Nat. Sci. Sleep 12, 895–905. doi: 10.2147/NSS.S271927
Li, Z., Bi, H., Jiang, H., Song, J., Meng, Q., Zhang, Y., et al. (2021). Neuroprotective effect of emodin against Alzheimer’s disease via Nrf2 signaling in U251 cells and APP/PS1 mice. Mol. Med. Rep. 23:108. doi: 10.3892/mmr.2020.11747
Maes, M., Meltzer, H. Y., Scharpe, S., Cooreman, W., Uyttenbroeck, W., Suy, E., et al. (1993). Psychomotor retardation, anorexia, weight loss, sleep disturbances, and loss of energy: psychopathological correlates of hyperhaptoglobinemia during major depression. Psychiatry Res. 47, 229–241. doi: 10.1016/0165-1781(93)90081-q
Mominoki, K., Morimatsu, M., Karjalainen, M., Hohtola, E., Hissa, R., and Saito, M. (2005). Elevated plasma concentrations of haptoglobin in European brown bears during hibernation. Comp. Biochem. Physiol. A Mol. Integr. Physiol. 142, 472–477. doi: 10.1016/j.cbpa.2005.09.017
Mondello, S., Kobeissy, F., Mechref, Y., Zhao, J., Talih, F. R., Cosentino, F., et al. (2018). Novel biomarker signatures for idiopathic REM sleep behavior disorder: a proteomic and system biology approach. Neurology 91, e1710–e1715. doi: 10.1212/WNL.0000000000006439
Oxenkrug, G. F. (2011). Interferon-gamma-inducible kynurenines/pteridines inflammation cascade: implications for aging and aging-associated psychiatric and medical disorders. J. Neural Transm. 118, 75–85. doi: 10.1007/s00702-010-0475-7
Piao, H. Z., Jin, S. A., Chun, H. S., Lee, J. C., and Kim, W. K. (2004). Neuroprotective effect of wogonin: potential roles of inflammatory cytokines. Arch. Pharm. Res. 27, 930–936. doi: 10.1007/BF02975846
Plescia, F., Cirrincione, L., Martorana, D., Ledda, C., Rapisarda, V., Castelli, V., et al. (2021). Alcohol Abuse and Insomnia Disorder: focus on a Group of Night and Day Workers. Int. J. Environ. Res. Public Health 18:13196. doi: 10.3390/ijerph182413196
Reddy, S. Y., Rasmussen, N. A., Fourie, N. H., Berger, R. S., Martino, A. C., Gill, J., et al. (2014). Sleep quality, BDNF genotype and gene expression in individuals with chronic abdominal pain. BMC Med. Genomics 7:61. doi: 10.1186/s12920-014-0061-1
Riemann, D., Krone, L. B., Wulff, K., and Nissen, C. (2020). Sleep, insomnia, and depression. Neuropsychopharmacology 45, 74–89. doi: 10.1038/s41386-019-0411-y
Ru, J., Li, P., Wang, J., Zhou, W., Li, B., Huang, C., et al. (2014). TCMSP: a database of systems pharmacology for drug discovery from herbal medicines. J. Cheminform. 6:13. doi: 10.1186/1758-2946-6-13
Sejbuk, M., Mironczuk-Chodakowska, I., and Witkowska, A. M. (2022). Sleep Quality: a Narrative Review on Nutrition, Stimulants, and Physical Activity as Important Factors. Nutrients 14:1912. doi: 10.3390/nu14091912
Sharifi-Rad, J., Quispe, C., Imran, M., Rauf, A., Nadeem, M., Gondal, T. A., et al. (2021). Genistein: an Integrative Overview of Its Mode of Action, Pharmacological Properties, and Health Benefits. Oxid. Med. Cell. Longev. 2021:3268136. doi: 10.1155/2021/3268136
Shokri-Kojori, E., Wang, G. J., Wiers, C. E., Demiral, S. B., Guo, M., Kim, S. W., et al. (2018). beta-Amyloid accumulation in the human brain after one night of sleep deprivation. Proc. Natl. Acad. Sci. U. S. A. 115, 4483–4488. doi: 10.1073/pnas.1721694115
Singh, A., and Zhao, K. (2017). Treatment of Insomnia With Traditional Chinese Herbal Medicine. Int. Rev. Neurobiol. 135, 97–115. doi: 10.1016/bs.irn.2017.02.006
von Kanel, R., Princip, M., Schmid, J. P., Barth, J., Znoj, H., Schnyder, U., et al. (2018). Association of sleep problems with neuroendocrine hormones and coagulation factors in patients with acute myocardial infarction. BMC Cardiovasc. Disord. 18:213. doi: 10.1186/s12872-018-0947-5
Wang, G., Ren, X., Zhang, X., Wang, Q., Liu, T., Deng, N., et al. (2021). Proteomic Profiling Reveals the Molecular Changes of Insomnia Patients. Biomed. Res. Int. 2021:6685929. doi: 10.1155/2021/6685929
Wickwire, E. M., Vadlamani, A., Tom, S. E., Johnson, A. M., Scharf, S. M., and Albrecht, J. S. (2020). Economic aspects of insomnia medication treatment among Medicare beneficiaries. Sleep 43:zsz192. doi: 10.1093/sleep/zsz192
Xu, Y., Li, X., Man, D., and Su, X. (2020). A G. iTRAQ-based proteomics analysis on insomnia rats treated with Mongolian medical warm acupuncture. Biosci. Rep. 40:BSR20191517. doi: 10.1042/BSR20191517
Yu, G., Wang, L. G., Han, Y., and He, Q. Y. (2012). Clusterprofiler: an R package for comparing biological themes among gene clusters. OMICS 16, 284–287.
Yue, S., He, T., Li, B., Qu, Y., Peng, H., Chen, J., et al. (2019). Effectiveness of Yi-Zhi-An-Shen granules on cognition and sleep quality in older adults with amnestic mild cognitive impairment: protocol for a randomized, double-blind, placebo-controlled trial. Trials 20:518. doi: 10.1186/s13063-019-3607-x
Keywords: insomnia, protein expression, immuno-inflammation, PRM, sleep
Citation: Liu T, Wang G, Zhang X, Liu X, Liang Z, Ren X, Yan D and Zhang W (2022) B serum proteome profiles revealed dysregulated proteins and mechanisms associated with insomnia patients: A preliminary study. Front. Integr. Neurosci. 16:936955. doi: 10.3389/fnint.2022.936955
Received: 05 May 2022; Accepted: 30 June 2022;
Published: 26 July 2022.
Edited by:
Harry Pantazopoulos, University of Mississippi Medical Center, United StatesReviewed by:
Sinead M. O’Donovan, University of Toledo, United StatesLais F. Berro, University of Mississippi Medical Center, United States
Copyright © 2022 Liu, Wang, Zhang, Liu, Liang, Ren, Yan and Zhang. This is an open-access article distributed under the terms of the Creative Commons Attribution License (CC BY). The use, distribution or reproduction in other forums is permitted, provided the original author(s) and the copyright owner(s) are credited and that the original publication in this journal is cited, in accordance with accepted academic practice. No use, distribution or reproduction is permitted which does not comply with these terms.
*Correspondence: Xingping Zhang, xjmyzxp@163.com
†ORCID: Xingping Zhang, https://orcid.org/0000-0003-1642-2710