- Counselling Psychology, Faculty of Education, University of Ottawa, Ottawa, ON, Canada
Bullying victimization is a form of psychological stress that is associated with poor outcomes in the areas of mental health and learning. Although the emotional maladjustment and memory impairment following interpersonal stress are well documented, the mechanisms of complex cerebral dysfunctions have neither been outlined nor studied in depth in the context of childhood bullying victimization. As a contribution to the cross-disciplinary field of developmental psychology and neuroscience, we review the neuropathophysiology of early life stress, as well as general psychological stress to synthesize the data and clarify the versatile dynamics within neuronal networks linked to bullying victimization. The stress-induced neuropsychological cascade and associated cerebral networks with a focus on cognitive and emotional convergence are described. The main findings are that stress-evoked neuroendocrine reactivity relates to neuromodulation and limbic dysregulation that hinder emotion processing and executive functioning such as semantic cognition, cognitive flexibility, and learning. Developmental aspects and interacting neural mechanisms linked to distressed cognitive and emotional processing are pinpointed and potential theory-of-mind nuances in targets of bullying are presented. The results show that childhood stress psychopathology is associated with a complex interplay where the major role belongs to, but is not limited to, the amygdala, fusiform gyrus, insula, striatum, and prefrontal cortex. This interplay contributes to the sensitivity toward facial expressions, poor cognitive reasoning, and distress that affect behavioral modulation and emotion regulation. We integrate the data on major brain dynamics in stress neuroactivity that can be associated with childhood psychopathology to help inform future studies that are focused on the treatment and prevention of psychiatric disorders and learning problems in bullied children and adolescents.
Introduction
Bullying victimization (BV) is a form of chronic psychological stress caused by interpersonal aggression that is repeatedly directed at a person who wields less power than their abuser (Olweus, 1994). Approximately one in 10 children worldwide are bullied regularly by their peers and another 30% of children are bullied on occasion (e.g., Nansel et al., 2001; National Academies of Sciences, Engineering, and Medicine, 2016; Biswas et al., 2020). Strong and growing evidence shows that BV is an intense psychological stress–targets of BV suffer chronic emotional distress which compromises their mental health and leads to persistent physical and social dysfunction, as well as poor academic achievement (e.g., Vaillancourt and Palamarchuk, 2021). The adverse correlates of BV similarly affect children worldwide (e.g., McDougall and Vaillancourt, 2015; Jantzer et al., 2021). Moreover, BV can lead to psychological adjustment problems and persistent mental health dysfunctions that extend well into adulthood (e.g., Arseneault et al., 2010; McDougall and Vaillancourt, 2015; van Geel et al., 2016; Moore et al., 2017; Vaillancourt and Palamarchuk, 2021).
Neurocognition plays a central role in the development of psychological stress. First, psychological stress is a physiological response to a salient stimulus that is perceived as a stressor such as a challenging event or behavior that disturbs the individual emotionally and thus triggers neurocognitive reactivity to adapt. Second, it is the cognitive appraisal that assigns the severity level to a stressor (i.e., interpretation of the stressor but not the nature of a stressor per se). If the stress is extreme and the stressor is uncontrollable, as is often the case with BV, neurocognitive stress reactivity can deviate toward maladaptation and psychopathology. In other words, the main effect of psychological stress is due to the perceived severity and controllability of the stressor, as well as the timing of the stressor (i.e., novelty/acuity/chronicity, Palamarchuk and Vaillancourt, 2021). That is why we hypothesize that the impact of BV on mental health will not be that different from the impact of other forms of psychological stress, such as childhood maltreatment, although we acknowledge that unique nuances may exist. We also recognize that the presence of physical/sexual abuse can aggravate psychological harm (e.g., Lereya et al., 2015); however, this interaction is beyond the scope of this review. Our reasoning for limiting our review to BV is because most neurobiological reviews have focused on child maltreatment and not on this other notable childhood stressor that affects far more children and youth worldwide (UNICEF, 2020).
Children are particularly vulnerable to the negative effects of psychological stress because the emotional and cognitive adjustment problems occur during a time when neuronal development is taking place (e.g., Sowell et al., 2004; Zhu et al., 2011; Cowell et al., 2015; Caballero et al., 2016). Emerging evidence supports that childhood BV has a profound effect on children’s brain development. For instance, Du Plessis et al. (2019) found longitudinal links between childhood BV and structural changes in the right ventrolateral (vl) prefrontal cortex (PFC) moderated by cortisol levels in adolescent boys but not adolescent girls. High BV with low daily cortisol output and/or a steeper diurnal slope was associated with reductions in the right vlPFC surface area, and high BV with high daily cortisol output and/or a low flatter diurnal slope was associated with a larger right vlPFC surface area. These findings highlight the differential effects of BV on brain development. In particular, decreased cortisol levels in bullied adolescents might indicate stress adaptation because reduced surface area and increased cortical thinning of the PFC during adolescence are part of normal development. Quinlan et al. (2020) provided evidence that BV at age 14 was indirectly associated with generalized anxiety via reductions in volume of the left dorsal striatum (nucleus caudate and putamen) at age 19. Muetzel et al. (2019) found that 10-year-old targets of BV had increased cortical thickness in the left fusiform gyrus, yet the findings might relate to either the pre-existing differences or to the consequences of BV. Although it is important to compare the influence of BV across the lifespan, such as childhood vs. adulthood, we emphasize the integrative and inter-disciplinary research on childhood BV because the adult literature is limited. We predict that adulthood BV would differ from childhood BV mainly due to the distinct factors that hinder stress appraisal and increase stress sensitivity. Namely, the etiology of childhood BV sensitivity relates to the neurocognitive immaturity that contributes to the amygdala—PFC networking specifics, which are reviewed. Adulthood BV sensitivity likely relates to the frontrostriatal loop engaged in motivation, habits, reward-learning, and decision-making (for the nuances in general psychological stress, see Palamarchuk and Vaillancourt, 2021).
The primary focus of this review is to highlight childhood BV and psychopathology through the lens of neurocognitive functioning. The novelty is that we present BV research on executive functioning by outlining multiple levels of cognition including learning, semantic cognition, cognitive flexibility, and processing of social and emotional information that involves behavioral regulation (i.e., social cognition, Shany-Ur and Rankin, 2014). We offer an innovative approach to the topic of BV. Specifically, an integrative brain dynamics model of cognitive and emotional convergence is presented based on the neurophysiological evidence of the effects of early life and general psychological stress with the aim of helping explain why BV is likely so robustly linked to psychopathology. We also discuss the application of the results and provide examples to help with the design of future studies that are focused on the treatment and prevention of psychiatric disorders and learning problems in bullied children and adolescents. It is our cross-disciplinary contribution to cognitive neuroscience, social neuroscience, developmental neuropsychology, psychological pedagogy, and psychiatry interface, which distinguishes the innovation of this review.
Stress-Induced Neuropsychological Cascade
Neuroendocrine Reactivity Related to Psychological Neuromodulation
Psychological stressors are salient stimuli, such as events and behavior, that can trigger aversive emotions and feelings. When a negative value is assigned to the salient stimuli (cognitive appraisal), psychological stress occurs. Psychological stress is a physiological response governed by the neurocognition to stay focused on the challenge and adapt. This stress response does not principally relate to the stimulus per se, but to the perceived stress severity and controllability that depends on the stressor’s acuity (i.e., novel/unpredicted vs. homotypic/chronic). That is, the major difference between the stressor’s influence on neuromodulation is not due to the nature of the stressor (e.g., childhood maltreatment vs. BV) but rather due to the interpretation of the stressor (see detailed review by Palamarchuk and Vaillancourt, 2021).
BV is a psychologically stressful experience for children (Vaillancourt and McDougall, 2013) and the stressor experienced is far too often extreme and/or prolonged. In general, psychological stress initially activates the hypothalamic–pituitary–adrenal (HPA) axis that alters glucocorticoid levels (i.e., circulated cortisol concentration), an effect seen in bullied children (e.g., Ouellet-Morin et al., 2011a, b, 2013, 2021; Vaillancourt et al., 2011; Kliewer et al., 2019). Human and animal studies show that increased cortisol levels in response to stress can over-activate the glucocorticoid receptors that prevail in the hippocampus and PFC, areas of the brain primarily associated with memory and learning. This activation can trigger neurocognitive responses linked to memory, mood, behavior, and executive functions, which should theoretically help the individual cope with the stressor (e.g., De Kloet et al., 1998, 2018; De Kloet and Derijk, 2004; Barsegyan et al., 2010; Vogel and Schwabe, 2016; Palamarchuk and Vaillancourt, 2021). Indeed, childhood adversity does not exclusively harm cognitive functions; it can also promote developmental adaptation to resilience via the improvement of some cognitive functions (Ellis et al., 2017). Nevertheless, stress-associated outcomes differ according to the intensity/severity and controllability of the stressor, which relates to cognitive appraisal (aforementioned stressor’s interpretation), as well personal and environmental factors (e.g., Schneiderman et al., 2005; Palamarchuk and Vaillancourt, 2021). When a stressor is beyond the coping ability of the individual, impairment in cognition and metacognition (i.e., awareness of one’s own thinking/learning) can occur (e.g., Lupien et al., 2005; De Kloet et al., 2016, 2018; Palamarchuk and Vaillancourt, 2021), which is often the case with BV (e.g., Ouellet-Morin et al., 2011b; Sinclair et al., 2012; Vaillancourt et al., 2013; Liu et al., 2016; Carroll et al., 2019; Gini et al., 2019). For example, there is mounting evidence documenting that bullied children and adolescents struggle academically in comparison to their non-bullied peers (Nakamoto and Schwartz, 2010; Espelage et al., 2013). Yet despite this well-replicated literature, the prevailing view regarding the association between BV and academic achievement does not account for a neurobiological mechanism (see exception, Vaillancourt et al., 2013; Vaillancourt and Palamarchuk, 2021). Rather, this association is typically attributed to poor school attendance or poor mental health mediating the link between BV and academic achievement.
The impaired neurocognitive functioning found in bullied children might also emerge from altered cortisol concentrations (e.g., Vaillancourt et al., 2008, 2011; Ouellet-Morin et al., 2011b, 2013, 2021; Östberg et al., 2018b; Palamarchuk and Vaillancourt, 2021), that in turn, affect neurochemical mechanisms (i.e., neurotransmission) of memory, mood, and behavior. Specifically, stress can impact dopamine signaling in the PFC-striatum circuits (e.g., Gamo et al., 2015; Reneaux and Gupta, 2018), which plays an important part in neuromodulation (i.e., the capacity of a single neuron to regulate wide-ranging populations of other neurons), reward-motivated behavior, reinforcement learning (e.g., Ikemoto, 2007), and representational mentalizing (i.e., theory of mind) development (Lackner et al., 2010).
In fact, the alteration of dopamine signaling is linked to childhood adversity (e.g., De Bellis et al., 1994; Oswald et al., 2014; Egerton et al., 2016; Bloomfield et al., 2019). Dopamine signaling can be moderated by environmental and genetic factors in adolescence, a developmental period that is particularly vulnerable to the effects of stress on the PFC’s functions due to increased dopaminergic projections (Arnsten and Shansky, 2004; Oswald et al., 2014; Egerton et al., 2016). Polymorphism of the dopamine D4 receptor (DRD4) gene (i.e., shorter vs. longer alleles) was found to correlate with better representational mentalizing (Lackner et al., 2012), executive functioning, and social/emotional development in preschoolers (Pappa et al., 2015). Kretschmer et al. (2013) examined whether peer effects (negative vs. positive) on adolescent development were genetically moderated via the DRD4 polymorphism [the 7-repeat (7R) allele vs. the 4R allele]. Results indicated that the 4R allele was linked to higher susceptibility for the effects of BV and social well-being on later delinquency. Janssens et al. (2015) showed that peer rejection, a strong correlate of BV (Knack et al., 2012), was associated with rule-breaking behavior moderated by the dopamine transporter genotype (DAT1) in adolescents; in particular, the 10R-allele carriers showed more rule-breaking behavior in the context of high peer rejection, but less rule-breaking behavior in the context of low peer rejection. Cao et al. (2017) showed that functional polymorphism (TaqIA) in the dopamine receptor D2 (DRD2) gene (A2A2 genotype, i.e., no A1 allele) at age ~12 years predicted higher levels of depression at age 14 years in bullied adolescent boys, but not bullied girls. In contrast, among adolescent offenders, carriers of the A1 allele were more likely to be severely bullied compared to non-carriers of the A1 allele (Vaske et al., 2011). Thus, altered dopamine signaling may be a predisposition to BV and its pervasive psychopathological impacts, especially in adolescents who have poor executive functions, depression, and genetic risk factors.
Limbic Dysregulation and Emotion Processing
Besides the HPA axis, the principal stress response involves the locus coeruleus-norepinephrine system which relates to arousal, as well as fear supported by hyperactivation of the amygdala. This response has cognitive and behavioral effects, including arousal, attention, and cognitive flexibility (e.g., Skosnik et al., 2000; Morilak et al., 2005; Alexander et al., 2007; Valentino and Van Bockstaele, 2008). Although these effects can support careful assessment and strategic planning in unpredictable situations, they can also increase anxiety via the behavioral inhibition system associated with the lateral amygdala and the ventromedial (vm) PFC (e.g., Markett et al., 2018; Palamarchuk and Vaillancourt, 2021). In other words, behavioral inhibition ensues which involves the withdrawal from the danger, a reaction that is adaptive in this specific context. However, the continuation of inhibitory behavior is likely why we see the development of mental health problems including anxiety, depression, psychosis, psychosomatic, and eating disorders among bullied children (e.g., McDougall and Vaillancourt, 2015). For example, Weems et al. (2003) found that hyperarousal symptoms in adolescents with PTSD predicted the development of emotional numbing, which presumably relates to cognitive alterations in PTSD (see also Hayes et al., 2012; Palamarchuk and Vaillancourt, 2021).
BV is related to disturbed emotional processing and activation of the cerebral networks of social pain and monitoring (Rudolph et al., 2016; Will et al., 2016; Telzer et al., 2018, 2020; McIver et al., 2019). Within the cerebral networks of emotional processing, the essential role belongs to the amygdala, which if dysregulated, can contribute to psychiatric disorders (e.g., Monk et al., 2008; Brotman et al., 2014; Wegbreit et al., 2014). In bullied children, the amygdalar dysregulation can be seen in hyper responses to social exclusion (McIver et al., 2019), fearful faces (Swartz et al., 2019), and unexpected positive peer evaluation, in which high wariness in early childhood correlates with the severity of adolescent social anxiety (Jarcho et al., 2019). Moreover, amygdalar hyperactivity relates to the severity of BV and internalizing/externalizing symptoms, and to poor social self-esteem in bullied adolescent girls (Telzer et al., 2020). The neuronal mechanisms of anxiety are that repeated exposure to BV can facilitate the maintenance of extremely vivid aversive memories and evoke strong negative emotional responses such as acoustic perceptions or body sensations (Sansen et al., 2015). The traumatic memories can be intrusive as their activation can be triggered by even benign cues that remind the individual of the aversive event via an associative information network; this memory network can contribute to the development and maintenance of psychiatric dysfunctions such as social anxiety disorder (Iffland et al., 2014). Moreover, general anxiety levels have been shown to mediate a poor stress response in bullied children such as the negative relation between BV and cortisol levels during school lunchtime, a period of anticipated exposure to BV (Carney et al., 2010); whereas blunted cortisol responses to stress were found to be linked to increased social/behavioral problems in bullied adolescents (Ouellet-Morin et al., 2011b; 2021).
Stress-associated amygdalar dysfunction relates (but not exclusively) to the midbrain raphe nuclei and serotonergic (5-HT) pathways implicated in the downregulation of fear and anxiety (e.g., Bocchio et al., 2016; Palamarchuk and Vaillancourt, 2021) and in the enhanced active coping with inescapable stress in rodents in a time-locked manner (Nishitani et al., 2019). Uncontrollable stress is associated with the dysregulated dorsal raphe nuclei that affects 5-HT signaling (e.g., Amat et al., 2005), and alterations are linked to fear memory formation and retrieval (Sengupta and Holmes, 2019), as well as a higher risk for depression (e.g., Amat et al., 2005; Bocchio et al., 2016), greater susceptibility to future stress, and stress-induced anhedonia (Prakash et al., 2020). These serotonergic pathways can further compromise the physiology of various neuropsychological domains (e.g., Berger et al., 2009), including but not limited to those associated with BV— namely aggression (Bettencourt et al., 2013; Krygsman and Vaillancour, 2019), perception (Cole et al., 2014; Lavell et al., 2018; Östberg et al., 2018a), reward (Casement et al., 2014; Rappaport et al., 2019), attention/memory (Vaillancourt et al., 2011), appetite (Lee and Vaillancourt, 2018), and sleep (van Geel et al., 2016), with sleep problems mediating the link between delinquency and drug use in bullied girls (Sosnowski et al., 2016). In contrast, when a stressor is controllable, the vmPFC can inhibit the dorsal raphe nucleus’ stress-response, which in turn prevents learned helplessness and behavioral depression (e.g., Amat et al., 2005; Maier et al., 2006). In other words, the vmPFC plays a major role in coping with stress (i.e., strengthening of the escape response) and its deficient inhibitory control over the limbic circuits triggered by aversive events determines the uncontrollability of the stressor that affects behavior. BV can be an uncontrollable stress per its association with behavioral /psychological maladjustments in childhood (Arseneault et al., 2010; McDougall and Vaillancourt, 2015; van Geel et al., 2016; Moore et al., 2017; Vaillancourt and Palamarchuk, 2021) and interpersonal needs-hopelessness sequela that can indirectly moderate suicidal ideation in adolescents (Shin et al., 2016; also see Hong et al., 2015; Silberg et al., 2016). Hopelessness results from anticipating more negative than positive events, unachievable goals, and surrender (Marchetti, 2019; see also Palamarchuk and Vaillancourt, 2021); its high levels correlate with increased inflammatory reactivity to social stress in bullied adolescents (Giletta et al., 2018). This is a psychological defeat with an alarming global effect. For instance, BV is a high-risk factor of adolescent suicide attempts (Koyanagi et al., 2019), which is the leading cause of death among youth in high-income countries, accounting for 17.6% of all deaths (UNICEF).
Neurocognitive Effects
BV exposure has been linked to significant memory impairment in children (Vaillancourt et al., 2011). Memory impairments are driven by glucocorticoid activation (Sauro et al., 2003) and cortisol-induced genomic mechanism that develops with the stress-activated translocation of glucocorticoid receptors to the neuronal nucleus (Kino et al., 2009; Argentieri et al., 2017). Epigenetic mechanisms associated with stress primarily belong to the level of brain-specific DNA methylation, which leads to stable gene repression (e.g., Brenet et al., 2011) and has a distinguished impact in early life (e.g., Lubin et al., 2008; Szyf and Bick, 2013; Alexander et al., 2018).
Exposure to severe and/or chronic stressors can alter glucocorticoid signaling, resulting in dendritic atrophy and reduced spine density that affects synaptic plasticity (McEwen, 1999, 2000, 2001; Sousa et al., 2000; Moench and Wellman, 2015; Madalena and Lerch, 2017; Urban et al., 2019). Spine density is necessary for neuronal network formation, an essential component of cognition, including memory and learning that is promoted in the PFC and hippocampus (Amat et al., 2005; Gilabert-Juan et al., 2013). Stress can further induce cerebral morphological changes; for instance, early life adversity is associated with volume reductions in the amygdala, medial PFC, and hippocampus (in exposure to threat) and frontoparietal regions (in children exposure to deprivation; Kim et al., 2019; McLaughlin et al., 2019), as well altered white matter tracts (Kim et al., 2019), that increase the risk of developing PTSD (Yehuda, 2009) and neurodegeneration (Vyas et al., 2016). The alteration of cerebral morphology is moderated by interactions between childhood adversity and genes, such as the S allele of 5-HTTLPR (for the hippocampus), Met allele of BDNF (for the amygdala, hippocampus, PFC, and rostral ACC), and FKBP5 gene rs160780 (for white matter), which have links to depression (Kim et al., 2019). Consistent with this idea, several researchers have found links between BV and symptoms of depression and PTSD in children who are targeted by their peers (Vaillancourt et al., 2011; Idsoe et al., 2012, 2021; Nielsen et al., 2015; Lee and Vaillancourt, 2018). And, as is the case with the association between poor academic achievement and BV mentioned previously, the association between PTSD and BV likely has a strong neurobiological underpinning. We hypothesize that the PTSD and depression symptoms found in some bullied children are in fact due to neurophysiological changes that are like the ones found in maltreated children, resulting from suppressed neurogenesis, stress-associated delayed myelination, as well as distorted apoptosis (Kavanaugh et al., 2017).
Neurogenesis refers to neuronal growth and development, where key events occur in dendrites and axons when neurons differentiate and mature. In dendrites, it is seen in the morphological changes such as increases in size and branching that are necessary for signal flow between neurons. In axons, the changes involve growth cones that interact with cell adhesion molecules to anchor the tissue substrate, which are required components for primary outgoing pathways. Neurogenesis contributes to synaptogenesis and cortical thickness. Its suppression affects the amount and type of signals the neuron receives (Belsky and de Haan, 2011), whereas BV relates to the cortical thickness changes in adolescents (Muetzel et al., 2019). In a multicohort analysis of the community-based data (Brazil, Canada, and Europe), Parker et al. (2020) showed the variance in cortical thickness during maturation was linked to dendrite, spine, and myelin genes, which are also enriched in genes associated with psychopathology.
Myelination is the coating process of the axons that increases the speed of nerve transmission up to 100 times. Its delay has a significant impact on neuronal circuits’ functioning (Belsky and de Haan, 2011). Delayed myelination can be detected by reduced fractional anisotropy in diffusion tensor imaging. In fact, maltreated children with PTSD present with reduced fractional anisotropy in the corpus callosum, the primary white matter tract with interhemispheric projections necessary for the processing of emotional stimuli and memory functions (Jackowski et al., 2008). Apoptosis is physiologically programmed cellular death, which is elevated during psychological stress due to neurochemical changes. In particular, animal studies suggest that severe stress causes neuronal apoptosis in the hippocampus related to the neurotransmitter imbalance (between glutamate and GABA), which may explain the findings pertaining to PTSD (i.e., inhibition of the HPA axis activation, anxiety, and poor learning; Gao et al., 2014). Although not directly assessed in bullied children, we hypothesize similar neurobiological findings will be obtained, because, like child maltreatment, BV is a form of interpersonal trauma (Idsoe et al., 2021).
From a therapeutic standpoint, we foresee that some neurocognitive dysfunctions may be reversible to a certain degree if the stressor is terminated (Conrad et al., 1999) and the neural harm is not related to a permanent loss of cells but rather is related to atrophy (McEwen, 1999, 2000; Sousa et al., 2000). The prediction is supported by the fact that while myelin plasticity substantially responds to chronic psychosocial stress (Laine et al., 2018), glucocorticoid treatment can initiate and enhance post-traumatic myelin formation, which is a cellular marker of cerebral regeneration (Chan et al., 1998). At the same time, glucocorticoid-related biomarkers (e.g., bedtime salivary cortisol and plasma dehydroepiandrosterone/cortisol ratio) are associated with the treatment of PTSD symptoms, including anxiety and depression in combat veterans (Yehuda et al., 2014).
Hippocampus-Dependent Learning
The neural factors of BV likely involve a complex interplay between hyperarousal, fear conditioning, and memory processing (i.e., learning and executive functions). Memory processing can be mapped onto the anterior cerebral network between the PFC, amygdala, hippocampus, and its adjacent cortex, the parahippocampal gyrus with peri- and ento-rhinal cortical areas (e.g., Raslau et al., 2014, 2015). In this anatomical network, the stress-response of the ventral hippocampus is distressed by the amygdala’s projections that are linked to fear conditioning and affective processes (e.g., Anagnostaras et al., 2002; Cenquizca and Swanson, 2007). The amygdala plays a critical role in stress-induced memory impairment via fear conditioning and explicit/associative memory alteration (e.g., McGaugh, 2004; Rabinak and Maren, 2008; Robbins et al., 2008) that can alter hippocampus-dependent episodic memory when coupled with a stimulus that is perceived as emotionally evocative (e.g., Tulving and Markowitsch, 1998; Whitlock et al., 2006). Generally, psychological stress via fear activates the amygdala, which impacts proper hippocampal function that is needed for encoding and working memory; severe stress can also lead to hippocampal atrophy and memory deficits (e.g., Bremner and Narayan, 1998). This stress-induced effect on hippocampus-dependent learning potentially contributes to the memory deficits found in bullied children (Vaillancourt et al., 2011; Sansen et al., 2015). Lee et al. (2018) showed that being verbally abused by peers in adolescence is associated with volume reductions in the left hippocampal subfields, a finding also documented with childhood maltreatment, another form of interpersonal trauma (Teicher et al., 2012; Riem et al., 2015). Emotional childhood neglect is linked to left hippocampal white matter reductions in adult patients with major depression (Frodl et al., 2010). As well, PTSD predicts right hippocampal volumes reductions (Carrion et al., 2007). PTSD symptoms inversely correlate with hippocampal and amygdalar (left) volumes in maltreated youth with PTSD compared to those without PTSD (Morey et al., 2016), whereas reduced hippocampal and amygdalar volumes partially mediate the relation between early life stress and behavioral problems (Hanson et al., 2015). Additionally, stress-induced hyperactivation of hippocampal glucocorticoid receptors suppresses neurotrophic factor and leads to atrophic changes in the dentate gyrus (a cortical region of the hippocampal formation; Amaral et al., 2007) that impairs long-term memory and promotes depressive symptoms in chronically stressed individuals (Malberg et al., 2000; Bekinschtein et al., 2008; Surget et al., 2011; Taliaz et al., 2011). Although these effects are well documented, more work is needed to clarify specifics of the brain structure and functioning linked to poor memory in bullied children and youth.
Striatum-Dependent Learning
Under stress, the amygdala also promotes functional connectivity with the dorsal striatum that reduces its functional connectivity with the hippocampus, impacting the quantity and quality of memory; in particular, the established coupling shifts memory encoding and retrieval from hippocampus-dependent (“cognitive”) to striatum-dependent (“habit”) mode (Packard, 2009; Vogel and Schwabe, 2016; Vogel et al., 2017; Zerbes et al., 2020). The dorsal striatum relates to the automatic/habit responses and attention regulation via the PFC and parietal cortical interactions (e.g., Lago et al., 2017) and the implication of this shift is that the memory process reduces flexibility and thus becomes more reflexive. Consequently, stress-induced poor attentional set-shifting (i.e., cognitive inflexibility), which is related to the medial PFC’s dysfunction (e.g., Floresco et al., 2008; Butts et al., 2013; George et al., 2015), results in behavioral inflexibility that has been observed in bullied children. Specifically, the individual relies on old ways of responding, which might not be adaptive or appropriate because they are linked to threats from the past and not the present.
Although studies are needed to clarify the links between BV and striatum-related cognitive deficit, Telzer et al. (2020) showed that severe BV was related to hyperactivation in the amygdala, ventral striatum, fusiform gyrus, and temporoparietal junction, which was associated with increased internalizing and externalizing symptoms in adolescent girls. Of note, the ventral striatum is preferentially engaged in emotional processes and its dysregulation can lead to anxiety (Lago et al., 2017). The link between BV and anxiety is well noted in the literature and supported by meta-analytic findings (Reijntjes et al., 2010; Wu et al., 2015; Moore et al., 2017) and longitudinal studies (Takizawa et al., 2014; Sentse et al., 2017; Drazdowski et al., 2021). Silk et al. (2014) also has shown that reactivity of the ventral striatum (nucleus accumbens) and insula is moderated by depression in adolescence. Lee et al.’s (2020) findings are that BV has an indirect effect on adolescent depression via volume increases in the nucleus accumbens.
Developmental sensitization to stress differentiates children at risk for anxiety symptoms and internalizing disorders with profiles of sustained cortisol elevation (i.e., morning cortisol and during acute stress exposure; Laurent et al., 2015). Specifically, high levels of cortisol’s response to stress are distinguished by anxiety and linked to the dorsal striatum (putamen) volume reduction during a sensitive period in neuroanatomical development in childhood. Of relevance, recall that Quinlan et al. (2020) showed that chronic BV was indirectly linked to generalized anxiety via reductions in the left dorsal striatum in adolescence. Egerton et al. (2016) found that childhood adversity was linked to increased striatal dopamine activity in adulthood. Oswald et al. (2014) showed positive associations between childhood trauma and current levels of perceived stress, which related to higher ventral striatal dopamine responses to amphetamine. Accordingly, difficulties in learning and adjustments found in BV targets (Nishina and Parra, 2019; Vaillancourt et al., 2011, 2013) could be related to the striatal-dependent shift in memory processing associated with cognitive inflexibility and anxiety, especially in adolescence.
Executive Functioning: Cognitive Flexibility
Executive functioning is a cognitive control based on the analysis of environmental information and processed sensory information to assist with information encoding and cognitive flexibility (e.g., rapid attention/task-shifting and proper behavioral adjustments), which is crucial for decision making and problem-solving in adaption to the challenges presented (e.g., De Kloet et al., 1998; Dajani and Uddin, 2015; Palamarchuk and Vaillancourt, 2021). This type of cognitive control relates the dorsal and vlPFC functioning (e.g., Wager and Smith, 2003; Barbey et al., 2013), that is linked to developmental increases in the frontoparietal and parietal-dorsal ACC functional connectivity in the right hemisphere, to a stronger degree in girls compared to boys (Langeslag et al., 2013). Stress can alter executive functions, which is seen in the aforementioned cognitive inflexibility such as poor task/set-switching ability linked to the medial PFC dysfunction (Floresco et al., 2008; Butts et al., 2013; George et al., 2015) that correlates to cortisol response (e.g., Plessow et al., 2012; Goldfarb et al., 2017). Cognitive inflexibility is related to binary decision-making, which is a defensive cognitive strategy to ergonomically conquer the social challenge by integrating insecurity predictability, reasoning, learning fortification, and monitoring behavioral strategies (Collins and Koechlin, 2012). Nevertheless, poor cognitive flexibility is associated with poor mental health, for instance, depression (Gotlib and Joormann, 2010; Murphy et al., 2012; Hou et al., 2016) and BV. For example, Medeiros et al. (2016) showed that 10- and 11-year-old targets of BV had lower cognitive flexibility that was associated with poor “cold” executive functions (related to the logical-rational part of the cognition) compared to perpetrators of bullying. In another study, Jenkins and Canivez (2021) found a negative association between BV and executive functions, including cognitive flexibility, self-monitoring, emotion regulation, inhibition, and initiation in 6th Grade to 8th Grade. McQuade (2017) demonstrated that executive functions moderated the relation between BV and increases in aggression over a year in adolescents.
The neurobiological mechanisms are that psychological distress might modulate an individual’s response to stress, whereas poor stressor controllability (capacity of the PFC, discussed above) relates to exacerbated fear and anxiety that affects cerebral networks of the executive functions seen in behavioral problems (e.g., Maier et al., 2006; Maier and Watkins, 2010). For example, this mechanism can be seen in the hippocampal-related approach—avoidance conflict in young healthy adults (O’Neil et al., 2015), amygdala-related greater risk-taking behavior in bullied adolescent girls (Telzer et al., 2018), and learned helplessness and behavioral depression related to the medial PFC in rodent models (Maier et al., 2006). In childhood, there are four common behavioral responses to stress: impulsivity (i.e., acting-out or overreactive), passive-aggressiveness (i.e., overly compliant or uncooperative), dependency (i.e., passive or demanding), and repression (i.e., withdrawn or anxious; Chandler and Shermis, 1986). These responses are commonly expressed in bullied children (Reijntjes et al., 2010, 2011), which we predict are likely shaped by altered executive functioning seen in a stress-induced narrowed attention that enhances binary discrimination where the focus is placed on the aversive details (i.e., attentional bias; Cohen et al., 2009; Byrom and Murphy, 2016; Otgaar et al., 2017; Palamarchuk and Vaillancourt, 2021). Relatedly, BV is associated with goal-oriented selective attention (Carroll et al., 2019) and greater negative appraisals of peers (Troop-Gordon and Ladd, 2005).
A potential contributor to the behavioral maladjustment of bullied children is stress-induced right amygdalar hyperactivity that alters ascending projections toward the PFC. This signaling can modify executive functions related to decision making in unpredictable conditions (Maier et al., 2006; Maier and Watkins, 2010; Gupta et al., 2011; O’Neil et al., 2015), which are governed by the dorsal/lateral PFC (e.g., Wager and Smith, 2003; Barbey et al., 2013). Adolescence is a particularly vulnerable period for the development of executive dysfunctions because of the peculiarities in brain development seen in the functional organization of cognitive networks (especially in the right hemisphere; Sowell et al., 2004; Zhu et al., 2011) that parallel with attentional shifting, working memory, response inhibition, and goal-directed behavior (Luna and Sweeney, 2004; Paus, 2005; Bunge and Wright, 2007; Yurgelun-Todd, 2007; Schmithorst and Yuan, 2010). For instance, white matter microstructure was found to be correlated with the impulsive behavior in adolescents, with sex-specific differences (Silveri et al., 2006). The consequence is that learning strategies and decision-making are suboptimal at a time when an individual needs full functioning of their faculties to deal with the toxic social stressor. Relatedly, Herringa et al. (2016) showed that childhood adversity predicted hyper responses to aversive images in adolescence seen in the right amygdala hyperactivity and increased the right amygdala → bilateral PFC (BA 9, 10), bilateral hippocampi → bilateral PFC (BA 8, 9), and right hippocampus → left ACC (BA 32) functional connectivity. Moreover, right hippocampus reactivity was negatively associated with internalizing problems, while childhood adversity predicted increased right amygdala-dorsal PF connectivity specifically for negative emotional stimuli for adolescents only with lower levels of internalizing problems. This could be related to the higher ability of the PFC to regulate amygdalar responses (i.e., the PFC → amygdala inhibitory control); yet more research is needed to clarify these findings.
Aversive stimulation/stress can activate (i.e., higher 5-HT release) the dorsal raphe nucleus (and consequently the amygdala and striatum) and reduce its inhibition via diminished input from the vmPFC (Maier et al., 2006; Christianson and Greenwood, 2014); whereas inhibitory control of the vmPFC over brainstem and limbic structures determines stressor controllability and resilience (e.g., Amat et al., 2005; Maier and Watkins, 2010). In analogy to mental resilience and coping with general psychological stress (Palamarchuk and Vaillancourt, 2021), we predict that the mental health issues associated with BV in childhood are related to an escalated perception of helplessness and loss of control (hypofunctioning of the dorsomedial PFC linked to hyperactivated midbrain raphe nuclei and altered serotonergic signaling) associated with being abused (hyperactivated amygdala and norepinephrine surge) and weaker executive functioning that results in poor behavioral control and depression. In other words, the PFC, which is responsible for emotional control of impulses and behavioral responses to stimuli (i.e., inhibitory control) is hijacked by the area of the brain responsible for more primal functions like survival (i.e., the limbic system, specifically the amygdala and insula) following fear conditioning (e.g., Etkin and Wager, 2007; Figures 1–3). Vice versa, poor executive functioning is also a risk factor for BV in young children. Preliminary results by Vargas et al. (2019) showed that in youth, increased exposure to BV was independently associated with lower volumes of the right medial orbitofrontal area (BA 11, which is a part of the vmPFC). As well, clinical high-risk youth, who displayed altered gray and white matter, were more exposed to BV compared to healthy youth. It may relate to the altered social skills and inhibition problems (i.e., impulsive behavior). In particular, Verlinden et al. (2014) demonstrated that the risk for being bullied in first and second grade was associated with inhibition problems at age 4, but did not relate to working memory, shifting (i.e., cognitive flexibility), planning/organization, or emotional control. Inhibition difficulties, for instance, can be seen in the amygdalar hyperactivity and greater positive functional connectivity between the amygdala and rostral ACC (i.e., social pain network) in children with attention-deficit/hyperactivity disorder (ADHD; Hulvershorn et al., 2014). This functional pattern of the social pain network is similar to the one found in bullied girls by Rudolph et al. (2016). This might explain why children with ADHD—who also have more academic, educational, and social problems—are at higher risk for BV than non-ADHD peers (Loe and Feldman, 2007; Holmberg and Hjern, 2008; Wiener and Mak, 2009; Murray-Close et al., 2010; Taylor et al., 2010; Efron et al., 2021).
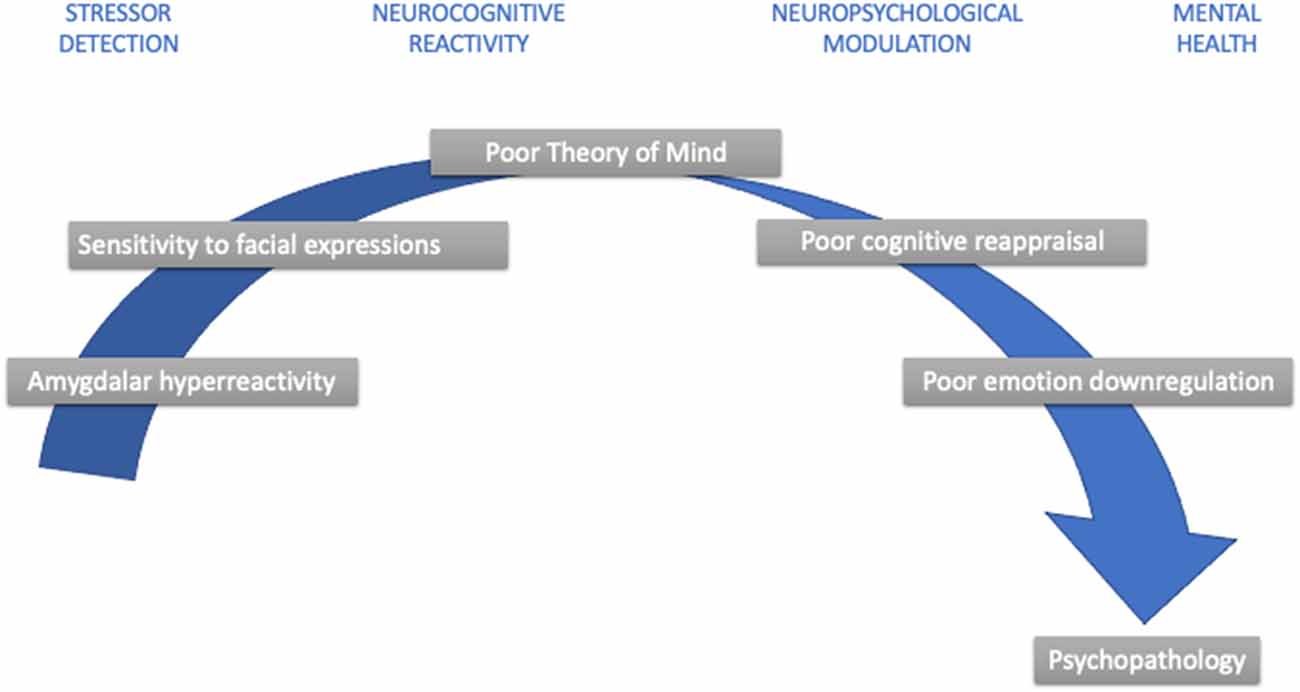
Figure 1. Developmental aspects in childhood stress psychopathology. Note: This simplified diagram summarizes developmental nuances that contribute to childhood stress sensitivity and increase psychopathology risk.
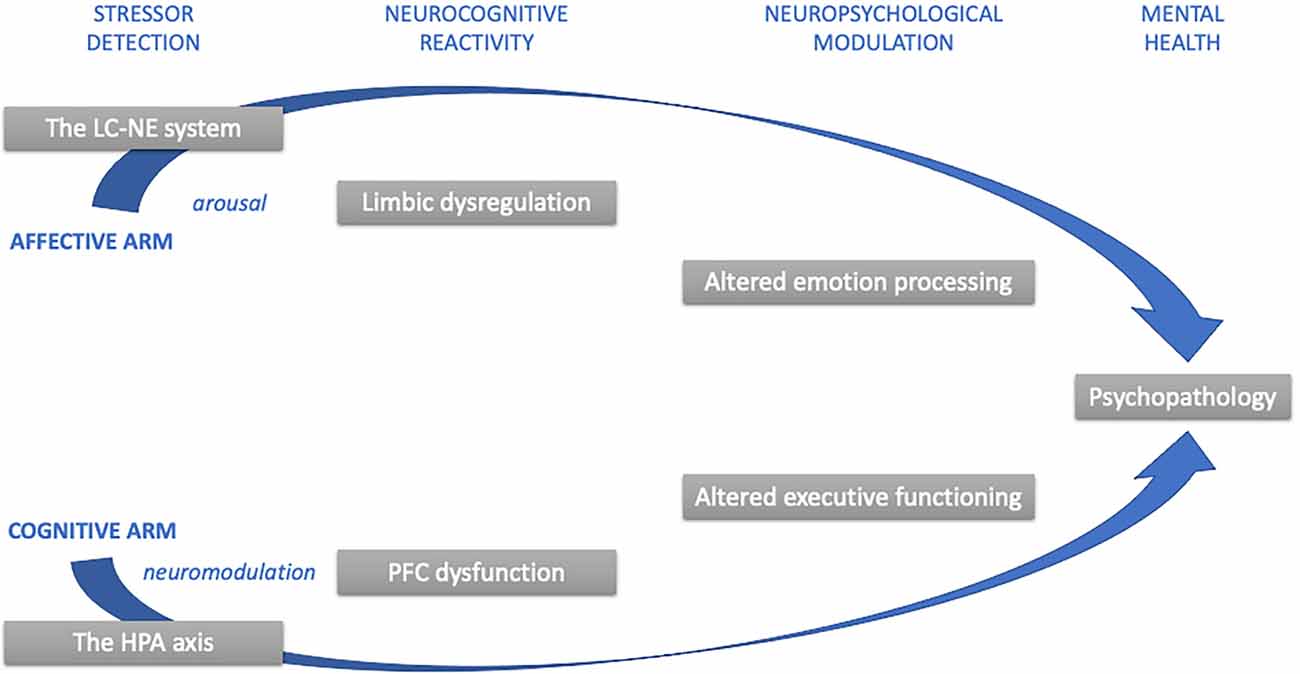
Figure 2. Cognitive and emotional convergence associated with stress psychopathology. Note: This simplified diagram summarizes the pathways of cognitive and emotional convergence in stress that can lead to psychopathology. Abbreviations: HPA, hypothalamic–pituitary–adrenal; LC-NE, locus coeruleus.
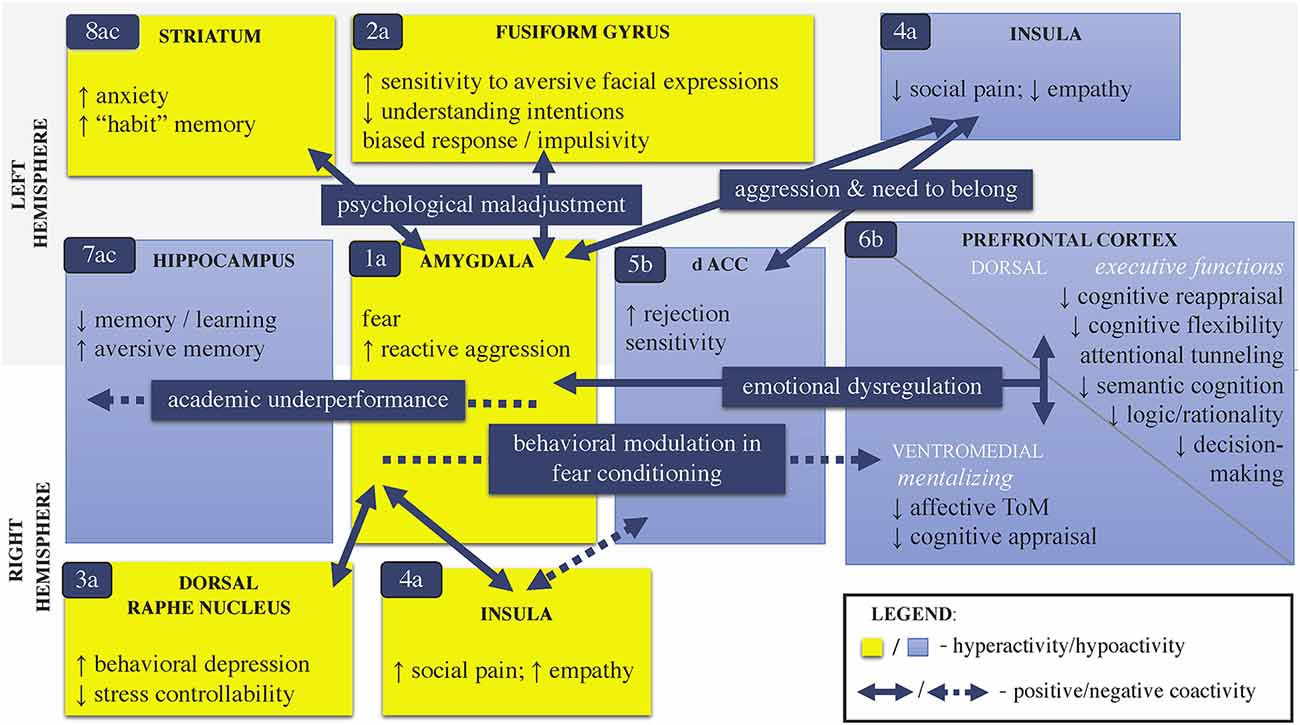
Figure 3. Major brain circuits associated with childhood stress psychopathology. Note: This schematic model integrates the major cerebral circuits (1–8) of cognitive and emotional convergence in stress neuroactivity that can be associated with childhood psychopathology. The model is a simplified syntax of the evidence in: [a] general psychological stress; [b] childhood BV; and [c] early life adversity. [a] The brain dynamics stem from (1) a hyperactivated amygdala following fear conditioning, which is a common neural response to an acute psychological stressor (i.e., “alarm-to-threat” stage, Palamarchuk and Vaillancourt, 2021). The magnitude of the amygdalar stress-reactivity can be mediated by the activity of the (2) fusiform gyrus, (3) dorsal raphe nucleus, and (4) anterior insula. [b] Hypothetically, the amygdalar overactivity can suppress the (5) dorsal/rostral ACC, which further can lead to (6) hypofunctioning of the vmPFC and thus impair mentalizing in young targets of BV. [a,c] Generally, in intense/chronic psychological stress, the amygdalar hyperactivity can (7) alter hippocampal memory encoding and (8) promote striatum-dependent memorization, which together, exacerbate compromised PFC related to executive functions. If altered PFC has poor inhibitory control over the amygdalar reactivity, a vicious cycle can occur (1 ⇄ 8). Of note, besides environmentally mediated pathways, childhood BV can also be a risk factor if the maladaptive brain dynamics pre-date the stress. For instance, genetic factors and developmental nuances of greater amygdalar sensitivity to stress and/or immature functions of the PFC (e.g., poor ToM) can increase susceptibility to stress in early life. The cerebral networks potentially display hemispheric lateralization in functions that distinguish pure targets (the right dominance) from bully-victims (the left dominance). Legend: dACC, dorsal anterior cingulate cortex; BV, bullying victimization; ToM, theory of mind; ↑, increase; ↓, decrease.
Executive Functioning: Semantic Cognition
We hypothesize that the stress children experience because of being bullied by their peers will also present as poor inhibitory control that negatively impacts semantic performance, i.e., the coherence or correspondence in comprehension and abstract thinking, which consequently impairs memory and decision-making ability. This hypothesis is based on PTSD studies demonstrating that stress-associated cognitive deficits are linked to altered memory formation due to retroactive interference (Yehuda et al., 1995). Retroactive interference relates to higher cross-categorization, which refers to altered patterning discrimination by individual cues that are present in correct and incorrect responses. The effect of retroactive interference—when a new memory interferes with the retrieval of an old memory—is more pronounced in children than in adults because children attend to too many details and cannot discern complex patterns if they are missing details or extraneous cues (e.g., Darby and Sloutsky, 2015). Consistent with this idea, Vaillancourt et al. (2011) found that altered cortisol levels in bullied adolescents predicted neurocognitive dysfunction in visual and verbal learning, as well as in executive tasks that were related to learning strategies and decision-making.
During maturation, semantic cognition capacity increases with the development of the anterior (rostral) PFC (orbitofrontal cortex, BA 10; Dumontheil, 2014), which have been found to be positively associated with levels of social anxiety in childhood and adolescence (Rosso et al., 2004). At the same time, intense stress can induce suggestive pressure or spontaneous semantic priming (e.g., interpretation of innocuous events as threats), and thus facilitate retrieval of false memories (Payne et al., 2007; Otgaar et al., 2017). Although the tendency to falsely remember events increases during normal development in human cognition due to a paradoxical complementarity effect—an increase in both accurate and false recall/recognition with age (Metzger et al., 2008; Howe et al., 2009; Brainerd et al., 2018), there are opposite developmental trends in false memory due to deficient cognitive ability to form semantic relations (i.e., semantic cognition; Ralph et al., 2017) in young children (Brainerd and Reyna, 2007). That is, false memory formation decreases with age for semantically unrelated information but increases with age for semantically related information. Besides the increase in the strength of these associative links between pieces of information (i.e., backward associative strength), its automaticity also prevents inhibition (forgetting) of false memories with age (McDermott and Roediger, 1998; Gallo and Roediger, 2002; Howe, 2005). In children, false recognition can be promoted by increased rates of similarity that leads to a gist extraction from semantically related information (i.e., fuzzy face theory; Brainerd and Reyna, 2005), as well by false identity judgment about distractors or decreased rates of non-identity judgment (Brainerd and Reyna, 1998).
These developmental nuances of false memory formation and maintenance hypothetically interplay with the stress-induced executive dysfunctions in bullied children. This complex cognitive interplay can explain the discrepancies between retrospective accounts of BV in relation to actual self-reports of BV. For example, Nishina and Parra (2019) found that students who overreported their abuse by peers had poor current (12th grade) psychosocial adjustment (i.e., higher depressive symptoms and social anxiety, and lower self-worth). Although the authors did not identify the underpinning cognitive mechanisms, they referred to an analogy with a mood-congruent memory bias (i.e., induced by emotional valence of the content/stimuli; Miranda and Kihlstrom, 2005) for over-reporters. Such biases relate to a triggered negative mood by aversive content that can distort memory; yet over-reporters presented with “naturally” negative mood (e.g., depressive symptoms) that can endorse false recall (Bookbinder and Brainerd, 2016).
We relate these types of discrepancies to false memories due to compromised semantic ability associated with psychological maladjustment following severe stress perception in over- reporters. Cognitive appraisal has a strong moderating effect on emotional dysregulation and is thus linked to psychiatric outcomes such as mood and anxiety disorders (Picó-Pérez et al., 2017; Zilverstand et al., 2017). For instance, Graham et al. (2003) showed that BV identified by self-reports but not peer-reports predicted similar psychological maladjustments as did BV identified by both self- and peer-reports for 6th grade students. Furthermore, studies that isolate functional activity uniquely to false memories (e.g., hit baseline) have shown that false memory retrieval occurs within the network of cognitive control/appraisal and emotion regulation, that includes the vmPFC (BA 24) and dorsal anterior cingulate cortex (ACC, BA 34/24, 32), as well extending to the frontoparietooccipital regions (BA 6/44, 40, 18/19) and the brainstem; whereas activity in verbal processing share same regions with semantic false memories, which includes dorsomedial (BA 32/8, 6/8) and vlPFC (BA 45/47), dorsal ACC (BA 32), and frontoparietal regions (BA 6/44, 40/7; see a voxel-wise quantitative meta-analysis by Kurkela and Dennis, 2016). Thus, verbal memory and false memory processing share a network with cognitive appraisal, which can be compromised by high rates of self-perceived stress that is associated with psychological maladjustments. Accordingly, Slattery et al. (2013) demonstrated the impact of low cognitive appraisal on poor cortisol response to a social stress test in adolescents at age 18; whereby internalizing disorders increased the links between poor verbal memory performance and poor cortisol reactivity. Woody et al. (2018) found that social stress elicited cortisol and cardiovascular responses to the speech stressor; while there were no associations between cognitive load/intelligence and stress cortisol responses (Sladek et al., 2016; Woody et al., 2018). Lastly, executive dysfunction may also explain why youth who underreported being bullied had poorer 6th grade adjustment (Nishina and Parra, 2019), which cannot be supported by a mood-congruent memory bias. Rather, the under-reporting could be due to poor post-stress recall; especially since true memories undergo retrieval-induced forgetting, which is less applicable for false memories in children (Price and Phenix, 2015). Baugerud et al. (2016) demonstrated that maltreated children had a poor true recall for both neutral and emotional information, but higher rates of false recall for aversive information compared to their non-maltreated peers. Altered post-stress recall could be a maladaptive strategy to deal with the stressor, the interpretation of that stressor, and the memory of the stressor. This hypothesis resonates with the findings that in bullied children, depression is an independent contributing factor to memory deficit including verbal domain, which is negatively correlated to the PFC’s executive functioning in addition to elevated cortisol levels (Vaillancourt et al., 2011).
Developmental Aspects and Interacting Neural Mechanisms in Childhood Stress Psychopathology
Sensitivity to Facial Expressions
Fear-conditioned memories of faces can impair the fusiform gyrus (Mueller and Pizzagalli, 2015), which functional significance is (but not limited to) in facial expression perception and face recognition (Kanwisher and Yovel, 2006; Iidaka, 2014). A recent study showed that high BV at age 8 years was associated with a “thicker cortex” of the fusiform gyrus in high-resolution structural magnetic resonance imaging (MRI) at age 10 years (Muetzel et al., 2019). Although true neural basis is unclear, in pediatric neuroimages, what appears to be larger cortical thickness, could in fact be due to delayed myelination. Myelination, the axonal insulation that facilitates signaling (action potentials) within synapses, is an essential neuronal networks component which undergoes critical developmental changes across ages 5–10 years. The increase in myelin deposition alters the contrast between gray (cortex) and white matter (which mainly contains long-range myelinated axons); thus, cerebral maturation can appear as cortical thinning after reaching peak by age ~10 years (Shaw et al., 2008; Ducharme et al., 2016; Natu et al., 2019). The reported greater thickness of the left fusiform gyrus (Muetzel et al., 2019) may in fact be related to delayed myelination in bullied children. However, more research is needed to assess the impact of potential mediators and moderators. For example, socioeconomic status was found to moderate trajectories of the cortical thickness decline, which had a curvilinear pattern (relatively steep decline in early childhood and subsequent leveling off during adolescence) for the low levels and linear pattern for the high levels, with the major effect in left superior temporal gyrus (Piccolo et al., 2016). As well, ADHD is associated with delayed maturation of the PFC (Shaw et al., 2007), whereas ADHD outcome mediates cortical thickness—the worse outcome is related to a “fixed” thinning of the left medial PFC at baseline (mean age 8.9 years) and the better outcome is related to the normalization of cortical thickness in the right parietal cortex at follow-up (mean age 5.7 years; Shaw et al., 2006).
Cortical thickness is an age-related parameter in structural MRI studies; however, the fact is that cortical thinning can relate to various factors of neurogenesis (e.g., synaptic reorganization and pruning; Shaw et al., 2008). As well, we see the interpretation during “thickening → thinning” transitioning period at age 10 years is especially challenging; conversely, longitudinal studies that define trajectories of cortical development and cortical thickness reached peaks would provide more insights into the underpinning cellular mechanisms. It cannot be explained solely by structural MRI and in vivo; an integration of other parameters is needed such as cortical pattern matching to account for gray matter variation and gyral patterning (Shaw et al., 2008; Tamnes et al., 2010). Maturation is also associated with functional connectivity transformations when cortical control within cerebral networks enhances and other cerebral regions, including the fusiform gyrus, lose centrality during the transition to adolescence period (Sato et al., 2015). Thus, we relate the reported greater cortical thickness (Muetzel et al., 2019) to delayed maturation that can be clarified with the cortical development trajectories in bullied children. The greater cortical thickness can also be a risk factor for cerebral functional connectivity due to local white matter alterations, which has to be further tested with functional MRI and fractional anisotropy.
Although Muetzel et al. (2019) do not account for the aforementioned neuroimaging specifics, that is, the delayed myelination rather than cortical hypertrophy, they did discuss that these cortical morphology changes in the fusiform gyrus could be related to a sensitivity to aggressive facial expressions developed in BV targets. Indeed, nonverbal facial expressions recognition involves emotional contagion (part of affective empathy) via brain-to-brain coupling and mimicry between individuals’ emotion systems (Prochazkova and Kret, 2017), whereas the amygdala can increase the sensitivity of the fusiform gyrus to visual response to static fearful faces accordingly to the connectivity models of fear sensitivity by Furl et al. (2013). However, we predict that this stress mechanism likely extends beyond this type of amplified emotional response to fearful/aggressive faces and involves functional connectivity (white matter architecture) that alters the fusiform gyrus input into theory-of-mind (ToM) via developmental sensitization in BV.
Theory of Mind
Theory of Mind (ToM), also known as mentalization, refers to the ability to infer the mental states of others like their thoughts/beliefs (i.e., cognitive perspective-taking or cognitive ToM) and emotions/feelings (i.e., affective perspective-taking or affective ToM) and the analysis of related behavior that is essential in social interactions and problem-solving (Dvash and Shamay-Tsoory, 2014; Healey and Grossman, 2018). Both cognitive and affective ToM engage the temporoparietal junction, precuneus, and temporal poles. Yet, cognitive ToM may engage the dorsomedial and dorsolateral PFC; whereas affective ToM may uniquely engage the vmPFC, the amygdala, and basal ganglia (that includes striatum; Healey and Grossman, 2018). The fusiform gyrus facilitates understanding intentions during ToM processing via the visual perception of others’ emotions (i.e., “reading” eye expressions or body language; Schurz et al., 2013, 2014; Thye et al., 2018; Tallarita et al., 2020). During social interactions, face processing relates to the activation of the fusiform gyrus, which is significantly influenced by emotional input from the amygdala; the latter produces fast perceptions of social–emotional feelings that are moderated by previous social experiences (i.e., subconscious threat-monitoring; Schultz et al., 2003; Duncan and Barrett, 2007; Herrington et al., 2011; Balderston et al., 2014; Frank et al., 2019). The amygdala’s hyperactivity may therefore disturb networks with the fusiform gyrus and compromise ToM processing (insufficient input from the vmPFC), which can be seen in rigid habit-like behavior during stressful social interactions due to the limbic dysregulation. In fact, Frick et al. (2013) showed that increased sensitivity of the fusiform gyrus to fearful faces was accompanied by the changes in connectivity between the fusiform gyrus and amygdala (increased) and also between the fusiform gyrus and vmPFC (decreased) in social anxiety disorder. Rudolph et al. (2016) also showed that the fusiform gyrus over responded to exclusion (relative to inclusion) together with the amygdala and dorsal ACC, the brain structures which hyper responses consequently predicted internalizing symptoms in bullied girls. In contrast, conscious awareness (the dorsomedial PFC activation in emotional downregulation) of the fearful faces can reduce the amygdala-PFC functional connectivity; whereas the connectivity is increased when information is processed non-consciously and the amygdalar responses relate to a negative bias in the subsequent evaluation of neutral faces (Lapate et al., 2016). That is, cognitive behavioral therapy that facilitates proper evaluation of the fearful faces (i.e., cognitive reappraisal) may help with overcoming explicit biases and subconsciously/“automatically” triggered social anxiety.
The need to support cognitive re-appraisal may also relate to poor executive functions in BV. Du Plessis et al. (2019) showed that childhood BV was related to a structure of the right vlPFC (i.e., thickness and surface area, moderated by cortisol levels), yet only in adolescent boys. Vice versa, stress-associated impairment of ToM likely can promote attentional tunneling that reduces cognitive flexibility (i.e., poor functioning of the medial/ventral PFC affects the dorsal/lateral PFC activity), and in turn, contribute to academic underperformance of bullied children. Clemmensen et al. (2020) showed that ToM and involvement in BV were both independently associated with academic performance in children aged 11–12 years, even after accounting for IQ and shared variance. Moreover, in this study, bullied girls had lower academic performance than non-bullied girls and boys and bullied boys.
Emotion Regulation: Prefrontal Cortical Descending Projections
The neurobiological background of ToM is that the medial PFC is critical for social cognition like judgment. Although the vmPFC is linked to understanding affective but not cognitive ToM (Shamay-Tsoory et al., 2006; Shamay-Tsoory and Aharon-Peretz, 2007), it is an anatomical key element for social, affective, and cognitive networks; and its disruption is linked to psychopathology and psychiatry (Hiser and Koenigs, 2018). Our hypothesis is that the complex interplay between altered vmPFC’s circuits linked to ToM affects the amygdalar responses to aversive emotions and fear, which relates to stress sensitization and psychiatric outcomes in bullied children.
First, the vmPFC is a reward-sensitive region that promotes social connection to others, monitors safety, and inhibits emotional-related reactions (Delgado et al., 2006). The vmPFC and left amygdala coactivity (i.e., concurrent responses during emotion processing) occurs during active regulation of negative emotions (Diekhof et al., 2011; Yang et al., 2020). The evidence for adults is that negative coactivation between the vmPFC (i.e., hyperactivity) and right amygdala (i.e., hypoactivity) influences downregulation of negative emotions, which is a cognitive “minimizing” reappraisal (Banks et al., 2007; Morawetz et al., 2017; Yang et al., 2020). Specifically, the vmPFC encodes emotional value (e.g., perceived aversiveness and fear) and further modulates it during cognitive reappraisal (e.g., distancing from feelings or reward anticipation). The successful emotion downregulation concordantly hypoactivates the left amygdala (i.e., negative coactivation that suppresses emotional value) and extends to the nucleus accumbens (the ventral striatum) to suppress reward encoding (Phan et al., 2005; Staudinger et al., 2009; Mulej Bratec et al., 2017). Thereby, we may say that successful emotional regulation relates to the pattern of top-down (i.e., cortical → subcortical) negative coactivity: the vmPFC hyperactivity → the right amygdalar hypoactivity (then mirrored by the left amygdala) → the ventral striatum hypoactivity. Of note, the cerebral activity pattern differs during the cognitive “positive” reappraisal (i.e., finding the positive meaning in negative experiences) as displays positive coactivity in this functional architecture (Doré et al., 2017), which likely contributed to Dougherty et al.’s (2015) findings of the “divergent” effect in children reappraisal (see also“self-appraisal” vs. “stressor-appraisal”; Palamarchuk and Vaillancourt, 2021).
However, BV is associated with emotional dysregulation related to the PFC dysfunction. In bullied young adults, the medial PFC-amygdalar connectivity across inclusion and exclusion was found to be positive (i.e., the medial PFC hyperactivity → the right amygdalar hyperactivity; see Figure 3) and indicated insufficient inhibitory control over the amygdala; this positive connectivity also moderated the relation between BV and depressive symptoms (McIver et al., 2019). For bullied adolescent girls, Casement et al. (2014) showed that the medial PFC and striatal hyper responses to reward anticipation mediated the link between social stressors and concurrent symptoms of depression; while Telzer et al. (2020) found that severity of BV correlated to hyperactivity in the amygdala and ventral striatum (but not limited to these structures). In bullied adolescents, it was shown that emotion regulation moderated poor cortisol reactivity to stress (Kliewer, 2016); as well, emotion regulation was associated with the emotion awareness and later on with anger regulation and lower rates of BV during the 2-years follow-up (Riley et al., 2019). In young children, Park et al. (2021) showed that chronic stress exposure can affect resting-state functional connectivity: (1) decrease it between the medial PFC and ventral tegmental area, which is essential in reward cognition such as associative learning and motivational salience; yet (2) increase it between the anterior hippocampus and left inferior frontal gyrus (BA 44), which is a part of the vlPFC implicated in language production and phonological/speech processing. These brain dynamics support the aforementioned findings that chronic BV can impair academic performance and increase the vulnerability of young targets. Similarly, mood and anxiety disorders were found to relate to less successful emotional downregulation in cognitive reappraisal (i.e., distancing strategies related to the vmPFC; Picó-Pérez et al., 2017), while the amygdalar hyper responses were linked to depression (Zilverstand et al., 2017). That is, emotional dysregulation in bullied children is likely linked to poor ToM that compromises cognitive appraisal and exhibits the pattern of top-down positive coactivity the vmPFC hyperactivity → the amygdalar hyperactivity (Figure 3).
Second, a successful “minimizing” reappraisal circuit also involves the positive coactivation of the social pain network, the vmPFC, the ACC, and the insula (Diekhof et al., 2011; Winecoff et al., 2013; Doré et al., 2017). For instance, Phan et al. (2005) showed that increases in negative emotional experiences in healthy adults were related to hyperactivity in the amygdala and hypoactivity in the dorsal ACC, which was moderated (attenuated and augmented, respectively) during cognitive reappraisal that involved activation of the dorsomedial PFC. The ACC is a behavior-monitoring and optimal decision-making region that integrates contextual socio-emotional information such as perceived fairness/unfairness (e.g., Kennerley et al., 2006; Lavin et al., 2013; Rolls, 2019). Activity in the dorsal/rostral ACC for fearful faces is negatively associated with BV (Swartz et al., 2019). Of note, a rostral part of dorsal ACC (often termed as the rostral ACC) belongs to the vmPFC (BA 32). Changes in functional coactivity within the social pain network (Figure 3) may explain heightened rejection/interpersonal sensitivity in targets compared to perpetrators; that has been shown to mediate the association between BV and mental health symptoms (Williams et al., 2017; McDonnell et al., 2018). The hypothesis resonates with Kross et al.’s (2007) findings that self-reported ratings of rejection-associated distress negatively correlated with hyperactivity in the left dorsal ACC (BA 6, which activity correlated positively with the right dorsomedial PFC, BA 8) and right insula in young healthy adults. As well, compared to high rejection sensitivity, low rejection sensitivity was associated with hyperactivity in the lateral PFC (left BA 45, 9, and right BA 6). Baird et al. (2010) demonstrated that increases in the dorsal ACC (anterior, BA 24/32 and posterior, BA 31) and bilateral dorsolateral PFC coactivity was associated with reduced sensitivity to relational aggression during an affect recognition task in bullied adolescent girls. This denotes a moderating role of the executive functions in emotion regulation. McIver et al. (2019) showed that social exclusion (compared to inclusion) increased the ACC-right insula functional connectivity in bullied and non-bullied young adults. However, positive connectivity between the left amygdala-ACC and left amygdala-right insula were attenuated in bullied adults, compared to non-bullied adults. That is, emotional dysregulation in bullied children is likely moderated by heightened rejection sensitivity with the pattern of top-down coactivity lateralized to the right: the vmPFC hyperactivity (i.e., poor affective ToM) → the dorsal ACC hypoactivity (higher rejection sensitivity) → the amygdalar hyperactivity → the right insula hyperactivity (Figure 3).
The dorsal ACC over responses to social exclusion in both BV and chronic peer rejection (Rudolph et al., 2016; Will et al., 2016), and its coactivation with anterior insula during social exclusion predicts increased internalizing symptoms and avoidance motivation in bullied girls (Rudolph et al., 2016). However, the reactivity of the left insula, as well as of the left nucleus accumbens (ventral striatum) appears to be additionally moderated by both adolescent depression (Silk et al., 2014) and bullying behavior (Perino et al., 2019). Importantly, executive functioning capacity also modulates the association between the activity in the left insula and dorsal ACC linked to aggression: low executive functioning predicts positive association and high executive functioning predicts negative association (Chester et al., 2014); whereas the dorsal ACC-insula functional connectivity correlates to target-changing behavior, which is linked to extraversion and thus, hypothetically, could facilitate increased interpersonal aggression (Takami and Haruno, 2020).
Perino et al. (2019) demonstrated that adolescents’ self-reported BV was related to hyperactivation in the medial PFC, insula, amygdala, and ventral striatum; yet Beekman et al. (2016) revealed that the need to belong can moderate the stress-responses to social exclusion. Chester et al. (2016) showed that rejection, as compared to acceptance, was not directly associated with a greater need to belong, but it was indirectly via social pain. Specifically, higher activity in the dorsal ACC and the insula predicted affiliative behavior. Thus, specifics of the dorsal ACC-insula functional coactivity, which are moderated by both executive dysfunctioning and rejection sensitivity, may differ between pure targets and bully-victims (i.e., children who are bullied and bully others; Figure 3); and because bullied children can have an anxious need to belong, which may be why they become perpetrators (Barker et al., 2008; Haltigan and Vaillancourt, 2014; Underwood and Ehrenreich, 2014). The poor outcomes for BV targets might thus be due to a socioemotional asymmetry that is characterized by a higher sensitivity to rejection vs. the higher need to belong seen in perpetrators. Perpetrators of bullying have been shown to have status goals related to dominance and prestige (Sijtsema et al., 2009) and often enjoy greater power, dominance, and popularity than BV targets (Vaillancourt et al., 2003; Pouwels et al., 2018; Faris et al., 2020).
As well, bullying behavior may relate to greater impulsivity (related to cognitive inflexibility associated with the dorsal/lateral PFC, reviewed above), which was found to be associated with the cortical volume reductions (in the medial PFC and insula) but subcortical volume increases (in the ventral striatum, hypothalamus, and anterior thalamus), whereas cortical to subcortical volume ratio partially mediated the association between early adversity and antisocial behavior (Mackey et al., 2017). That is, in bully-victims, the dorsal ACC—left insula coactivity likely has stronger functional connectivity and relates to an increased need to belong, aggression, and impulsivity but lesser rejection sensitivity compared to pure targets (Figure 3).
The dorsal ACC-insula effects on the amygdalar responses related to impulsivity and rejection sensitivity that distinguishes pure targets from bully-victims may belong to their empathic ability; and their neural network is also regulated by these structures (Völlm et al., 2006; Decety et al., 2013a, b). Empathic ability is an emotional aspect of inferring and sharing the emotional experiences of others (Dvash and Shamay-Tsoory, 2014), which entitles affective empathy (i.e., affective ToM, the vmPFC) and emotional contagion (Healey and Grossman, 2018). During social interactions, empathy levels mediate spontaneous engagement of cognitive ToM (i.e., cognitive empathy) related to the dorsomedial PFC (Pluta et al., 2011; Wagner et al., 2011). Its deterioration in the right hemisphere is associated with not understanding others’ emotions (Ratka, 2018). In mentalizing, the activation of the dorsomedial PFC is less pronounced in relation to negative social stimuli like social exclusion compared to more positive ones like social inclusion (Powers et al., 2013). This is likely an adaptive tool, a type of self-defensive mechanism that involves mental withdrawal to numb emotional pain. In fact, social exclusion is related to a higher tolerance for physical pain and “emotional numbness” such as reduced joy and empathy, which are intercorrelated (DeWall and Baumeister, 2006; Powers et al., 2013). Accordingly, we hypothesize that the aforementioned poorer ToM ability in children who are bully-victims compared to pure targets and pure perpetrators (Shakoor et al., 2012) is related to the vmPFC functioning being moderated by the dorsomedial and dorsolateral PFC activity (i.e., cognitive empathy and executive functioning; see Figure 3). This may also explain the findings that depression, anxiety, and psychosomatic symptoms were most frequently observed in bully-victims, but equally observed in pure targets and pure perpetrators (Kaltiala-Heino et al., 2000).
Behavioral Modulation: Amygdalar Ascending Projections
Meta-analytic findings of the emotional processing of fear in individuals with PTSD, social anxiety disorder, and specific phobia reveal hyperactivity in the amygdala and insula compared to healthy participants undergoing fear conditioning. Of note, the patterns of coactivation between the cortical regions of interest were observed only in individuals with PTSD (Etkin and Wager, 2007). Specifically, the patterns included: (1) negative coactivation between the right amygdala (its hyperactivity was then mirrored by left amygdala) and right dorsal/rostral ACC (its hypoactivity was then mirrored by the left cortex); and (2) positive coactivation between the latter cortex and the right (and then left) vmPFC. Conversely, extinction of conditioned fear relates to hypoactivity in the amygdala and hyperactivity in the dorsal/rostral ACC and the vmPFC (Phelps et al., 2004). Considering the amygdala-PFC connectivity, the main pathway for behavioral modulation in bullied children hypothetically can be: fear conditioning → the right amygdala hyperactivity (then mirrored by left amygdala) → hypoactivated dorsal/rostral ACC → hypoactivated vmPFC → poor affective ToM, higher rejection sensitivity, and higher distress (Figure 3).
Phobia and distress can have a larger effect on the PFC in childhood than in adulthood. ToM ability is facilitated by the inferior temporal lobe extending into the fusiform gyrus, which emerges around the ages of 3–5 years and is solidified in most children by around age 7 (Sabbagh et al., 2009; Lackner et al., 2010). Yet, ToM reasoning activity in the medial PFC shifts from ventral to dorsal only in late childhood (Moriguchi et al., 2007) and the affective component of empathy develops earlier than the cognitive component (Decety and Svetlova, 2012). The neuroanatomical background is that ToM development relates to the white matter maturation in: (1) “local structure” of (near) the medial PFC, medial parietal (precuneus), and temporoparietal regions, and (2) connectivity between the inferior frontal and temporoparietal regions (Grosse Wiesmann et al., 2017); whereas white matter tracts of the frontotemporal regions have a pattern of late maturation (Tamnes et al., 2010). The cortical functional connectivity development is also asymmetrical—earlier in the right circuits and more expressed in the anterior (dorsal frontoparietal) regions (Sowell et al., 2004; Zhu et al., 2011).
Thus, the medial PFC (i.e., anterior region) is largely influenced by the right amygdala (i.e., subcortical region). However, the medial PFC is neither capable to execute sufficient inhibitory control over the amygdala during aversive stimuli nor is supported enough by the dorsal PFC related to cognitive reasoning due to its late maturation. Not surprisingly, the pre adolescent period is a high-risk time for stress to alter the maturation and functioning of the PFC (Caballero et al., 2016). This explains why the vmPFC (especially in the right hemisphere) is susceptible to behavioral modulation imposed by the amygdala’s hyperactivation in fear conditioning/emotional face perception, which is greater in children than in adults (Monk et al., 2003; Guyer et al., 2008), and linked to psychopathology, such as major depression and bipolar disorder (Monk et al., 2008; Brotman et al., 2014; Wegbreit et al., 2014).
Barker et al. (2008) and Haltigan and Vaillancourt (2014) examined the joint trajectories of BV and perpetration across childhood and adolescence respectively and found that the evolution of bullying involvement emerged from victimization to perpetration and not the reverse. A meta-analysis by Walters (2021) further revealed that BV and perpetration are intercorrelated and have bidirectional cross-lagged longitudinal relations, i.e., perpetration ⇄ victimization. High BV seems to exacerbate poor affective reasoning and emotional control. Indeed, higher levels of BV in children are related to lower conscientiousness and higher neuroticism, which manifests as feeling angrier, blaming the perpetrator, and being less forgiving during conflicts (Bollmer et al., 2006), which could indicate ToM nuances linked to asymmetry in the PFC’s control over the amygdalar responses to anger and fear that distinguish pure targets from bully-victims. In particular, poor ToM at age 5 years was found to be related to higher levels of reactive aggression (i.e., limbic/automatic response to a real/perceived threat) at age 6 years in BV targets; whereas better ToM at age 5 years was found to be related to higher levels of proactive aggression (i.e., a non-provoked/planned behavior for personal gain) at age 6 years in perpetrators (Renouf et al., 2010). As well, the amygdala’s higher response to anger and lower response to fear predicts bullying behavior (Swartz et al., 2019). Regardless of the motivational direction (i.e., approach vs. withdrawal), higher levels of anger have been related to larger left lateralization in the PFC activity (Wacker et al., 2003; Stewart et al., 2008), while the right amygdalar hyperactivity is a frontline response to fear conditioning linked to hypoactivity of the right medial PFC (Etkin and Wager, 2007), and the latter correlates to impaired detection of deception (Stuss et al., 2001; Beekman et al., 2016). Moreover, emotional valence asymmetry in cerebral functioning is well-documented (Davidson, 1992); and the hypoactivity in the right cortex relates to poor recognizing of negative emotion such as envy, whereas hypoactivity in the left cortex relates to poor recognition of positive emotion (Shamay-Tsoory et al., 2007). We hypothesize that poor ToM is linked to the amygdala-medial PFC coactivity, which is moderated mainly by fear, and in the right hemisphere in pure targets compared to bully-victims, whose functional coactivity is moderated mainly by anger and in the left hemisphere (Figure 3).
Discussion: Stress Vulnerability Nuances and Integrative Brain Dynamics
BV is associated with a significant psychological burden that triggers a neuroendocrine cascade of stress-response activated by elevated cortisol levels and the outcomes are seen in the long-lasting deterioration of mental health, including depression, PTSD, and suicidal ideations in children and adolescents (Ttofi et al., 2011; Moore et al., 2017; Vaillancourt, 2018; Vaillancourt and Palamarchuk, 2021). BV can develop into an interpersonal trauma (Idsoe et al., 2021) where psychopathology is a by-product of a multilevel neural phenomenon with the brain dynamics bidirectionally stemming from the cortical-subcortical cerebral circuits. Given the scarcity of research that can clarify the cognitive and emotional convergence underlying psychopathology associated with childhood BV, we review and integrate the evidence on brain dynamics in: (A) general psychological stress; (B) childhood BV; and (C) early life adversity (see schematic outlining of the major cerebral circuits in Figure 3), and in doing so, emphasize the following aspects:
(A). The integral role in cognitive and emotional convergence belongs to the amygdala functioning and its circuits triggered by fear in acute stress. Fear conditioning can lead to psychiatric problems because the amygdala is the emotional hub of memory and influences the cognitive “defence” mechanism on a subconscious level, which is represented in the vm fronto-temporoparietal network (e.g., Palamarchuk and Vaillancourt, 2021).
(B). Perspective-taking abilities (i.e., ToM) play an essential role in social interactions. Both cognitive and affective ToM share the vm fronto-temporoparietal network with cognitive “defence” mechanism. Immature and/or stress-compromised ToM relates to the misperception of others’ intentions and can contribute to explicit bias and anxiety (reviewed above). This can narrow attention with a feeling of losing control over the situation. At the same time, cognitive inflexibility and insufficiency in emotion regulation aggravates stress perception and worsens psychological disturbance (e.g., Palamarchuk and Vaillancourt, 2021). Not surprisingly then, BV is linked to poor ToM.
(C). From the early life stress viewpoint, there are several major nuances that can explain why BV is reliably associated with a risk of developing psychiatric disorders in childhood. First, the higher risk of psychiatric disorders in bullied children is likely supported by developmental aspects in the anatomical ToM signature besides emotion reactivity. In particular, the higher amygdalar response to fear (seen in a norepinephrine surge) and its stronger influence on the vmPFC (seen in the negative coactivity of these brain structures) in children compared to adults could increase the risk of disorders. Second, the disturbed emotional processing due to poor inhibitory control of the vmPFC can aggravate the amygdalar hyperactivity in stress. Third, stress-induced morphological and neurochemical changes within the cerebral networks are especially harmful in early life because of undergoing neurogenesis and myelination, which is likely compromised in young targets. Lastly, the susceptibility to psychiatric dysfunctioning among bullied children and adolescents can relate to the neuroepigenetics that moderate stress reactivity (e.g., blunted cortisol response).
Although we refer to the neural mechanisms of early life and general psychological stressors, the neuropathology in childhood BV may differ and longitudinal studies are required to test the brain dynamics models—integrative vs. specific—to better understand how BV gets under the skin to confer risk in young targets. Nevertheless, psychopathology in mental stress significantly relates to the cognitive appraisal of a stressor but not to the stressor per se; while the influencing factors are stressor’s acuity, timing, and novelty (e.g., unpredicted/novel vs. homotypic, e.g., for details see review by Palamarchuk and Vaillancourt, 2021). We thus hypothesize that the brain dynamics associated with childhood stress psychopathology depend on a stress perception/comprehension to a larger extent, compared with a type of victimization (e.g., child maltreatment vs. peer victimization). The integrative brain dynamics for BV and early life adversity potentially would not be significantly distinct since many of the associations are extrapolated from research on childhood stress in the broader sense; and oftentimes, BV does not exist in a “vacuum”. Although poly victimization is out of this review scope, we would suggest that children and youth who experience multiple types of victimization across different domains (child maltreatment, community violence, physical/sex abuse, etc.) are likely to be among those with the worst neurocognitive and psychiatric outcomes due to a complex stress response.
The goal of this review is to help inform future studies that are focused on the treatment and prevention of psychiatric disorders and learning problems in bullied children and adolescents. To move the research forward in this area, we offer the following recommendations. First, research on BV, as well as on any psychological stress, must identify the exact stressor(s), perceived stress severity, and perceived controllability of the stressor. Second, psychological/clinical symptoms must be well differentiated in BV target’s anamnesis (e.g., occurred prior to a stressor/event, induced by a specific stressor, random/transient/chronic, and aggravating/relieving factors). Third, studies on cognitive re-appraisal aimed to define neural correlates in emotion regulation must consider different effects of minimizing appraisal vs. positive appraisal and self-appraisal vs. stressor-appraisal (Palamarchuk and Vaillancourt, 2021).
Our hope is that the proposed neurobiological mechanisms can be used in the prediction of risk for neurocognitive vulnerability and psychopathology following BV in childhood. For instance, consideration of dopamine-related behavioral maladjustments may facilitate proper sociomedical interventions focused on preventing children from becoming the target of BV or bully-victim. As well, a better understanding of the association between BV and academic achievement can help young BV targets with expedited sociopsychological help, including cognitive behavioral therapy focused on associative learning, cognitive flexibility, and reappraisal to develop a more appropriate reactivity to stressors and successfully cope with the stress.
Conclusion
BV is a psychological stress which can lead to pervasive interpersonal trauma that devastates far too many children worldwide. Being the target of BV places children at risk for a host of problems, most notably in the areas of mental health and learning. In our review, we delineate how exposure to this type of social pain is associated with a series of neurobiological responses that could result in psychopathology, as well as enduring structural and functional changes in the brain. We describe the complex cognitive and emotional convergence and synthesize the data in a form of integrative brain dynamics, as well as provide testable hypotheses to explain why children are more vulnerable to the adverse outcomes of BV than adults. Given how high the stakes are, the reduction of bullying must be prioritized.
Author Contributions
TV encouraged and supported ISP to investigate the impact of psychological stress on cognition in bullied children. ISP planned and carried out the project, the main conceptual ideas, developed hypotheses, and integrative model of the brain dynamics associated with bullying victimization. ISP designed the figures and wrote the manuscript with notable input from TV. Both TV and ISP provided critical feedback, helped shape the manuscript, and contributed to its final version. All authors discussed the results and agreed to be accountable for the content of the work. All authors contributed to the article and approved the submitted version.
Funding
This work was funded by the Canadian Institutes of Health Research Tier 2 and Tier 1 Canada Research Chair grants awarded to TV.
Conflict of Interest
The authors declare that the research was conducted in the absence of any commercial or financial relationships that could be construed as a potential conflict of interest.
Publisher’s Note
All claims expressed in this article are solely those of the authors and do not necessarily represent those of their affiliated organizations, or those of the publisher, the editors and the reviewers. Any product that may be evaluated in this article, or claim that may be made by its manufacturer, is not guaranteed or endorsed by the publisher.
Abbreviations
ACC, anterior cingulate cortex; BA, Brodmann area; BV, bullying victimization; HPA, hypothalamic–pituitary–adrenal; PFC, prefrontal cortexprefrontal cortex; vl, ventrolateral; vm, ventromedial.
References
Alexander, J. K., Hillier, A., Smith, R. M., Tivarus, M. E., and Beversdorf, D. Q. (2007). Beta-adrenergic modulation of cognitive flexibility during stress. J. Cogn. Neurosci. 19, 468–478. doi: 10.1162/jocn.2007.19.3.468
Alexander, N., Kirschbaum, C., Wankerl, M., Stauch, B. J., Stalder, T., Steudte-Schmiedgen, S., et al. (2018). Glucocorticoid receptor gene methylation moderates the association of childhood trauma and cortisol stress reactivity. Psychoneuroendocrinology 90, 68–75. doi: 10.1016/j.psyneuen.2018.01.020
Amaral, D. G., Scharfman, H. E., and Lavenex, P. (2007). The dentate gyrus: fundamental neuroanatomical organization (dentate gyrus for dummies). Prog. Brain Res. 163, 3–22. doi: 10.1016/S0079-6123(07)63001-5
Amat, J., Baratta, M. V., Paul, E., Bland, S. T., Watkins, L. R., and Maier, S. F. (2005). Medial prefrontal cortex determines how stressor controllability affects behavior and dorsal raphe nucleus. Nat. Neurosci. 8, 365–371. doi: 10.1038/nn1399
Anagnostaras, S. G., Gale, G. D., and Fanselow, M. S. (2002). The hippocampus and Pavlovian fear conditioning: reply to Bast et al. Hippocampus 12, 561–565. doi: 10.1002/hipo.10071
Argentieri, M. A., Nagarajan, S., Seddighzadeh, B., Baccarelli, A. A., and Shields, A. E. (2017). Epigenetic pathways in human disease: the impact of DNA methylation on stress-related pathogenesis and current challenges in biomarker development. EBioMedicine 18, 327–350. doi: 10.1016/j.ebiom.2017.03.044
Arnsten, A. F., and Shansky, R. M. (2004). Adolescence: vulnerable period for stress-induced prefrontal cortical function? Introduction to part IV. Ann. N Y Acad. Sci. 1021, 143–147. doi: 10.1196/annals.1308.017
Arseneault, L., Bowes, L., and Shakoor, S. (2010). Bullying victimization in youths and mental health problems: ‘Much ado about nothing’? Psychol. Med. 40, 717–729. doi: 10.1017/S0033291709991383
Baird, A. A., Silver, S. H., and Veague, H. B. (2010). Cognitive control reduces sensitivity to relational aggression among adolescent girls. Soc. Neurosci. 5, 519–532. doi: 10.1080/17470911003747386
Balderston, N. L., Schultz, D. H., Baillet, S., and Helmstetter, F. J. (2014). Rapid amygdala responses during trace fear conditioning without awareness. PLoS One 9:e96803. doi: 10.1371/journal.pone.0096803
Banks, S. J., Eddy, K. T., Angstadt, M., Nathan, P. J., and Phan, K. L. (2007). Amygdala-frontal connectivity during emotion regulation. Soc. Cogn. Affect. Neurosci. 2, 303–312. doi: 10.1093/scan/nsm029
Barbey, A. K., Colom, R., and Grafman, J. (2013). Dorsolateral prefrontal contributions to human intelligence. Neuropsychologia 51, 1361–1369. doi: 10.1016/j.neuropsychologia.2012.05.017
Barker, E. D., Arseneault, L., Brendgen, M., Fontaine, N., and Maughan, B. (2008). Joint development of bullying and victimization in adolescence: relations to delinquency and self-harm. J. Am. Acad. Child Adolesc. Psychiatry 47, 1030–1038. doi: 10.1097/CHI.ObO13e31817eec98
Barsegyan, A., Mackenzie, S. M., Kurose, B. D., McGaugh, J. L., and Roozendaal, B. (2010). Glucocorticoids in the prefrontal cortex enhance memory consolidation and impair working memory by a common neural mechanism. Proc. Natl. Acad. Sci. U S A 107, 16655–16660. doi: 10.1073/pnas.1011975107
Baugerud, G. A., Howe, M. L., Magnussen, S., and Melinder, A. (2016). Maltreated and non-maltreated children’s true and false memories of neutral and emotional word lists in the Deese/Roediger-McDermott task. J. Exp. Child Psychol. 143, 102–110. doi: 10.1016/j.jecp.2015.10.007
Beekman, J. B., Stock, M. L., and Marcus, T. (2016). Need to Belong, not rejection sensitivity, moderates cortisol response, self-reported stress and negative affect following social exclusion. J. Soc. Psychol. 156, 131–138. doi: 10.1080/00224545.2015.1071767
Bekinschtein, P., Cammarota, M., Katche, C., Slipczuk, L., Rossato, J. I., Goldin, A., et al. (2008). BDNF is essential to promote persistence of long-term memory storage. Proc. Natl. Acad. Sci. U S A 105, 2711–2716. doi: 10.1073/pnas.0711863105
Belsky, J., and de Haan, M. (2011). Annual research review: parenting and children’s brain development: the end of the beginning. J. Child Psychol. Psychiatry 52, 409–428. doi: 10.1111/j.1469-7610.2010.02281.x
Berger, M., Gray, J. A., and Roth, B. L. (2009). The expanded biology of serotonin. Annu. Rev. Med. 60, 355–366. doi: 10.1146/annurev.med.60.042307.110802
Bettencourt, A., Farrell, A., Liu, W., and Sullivan, T. (2013). Stability and change in patterns of peer victimization and aggression during adolescence. J. Clin. Child Adolesc. Psychol. 42, 429–441. doi: 10.1080/15374416.2012.738455
Biswas, T., Scott, J. G., Munir, K., Thomas, H. J., Huda, M. M., Hasan, M. M., et al. (2020). Global variation in the prevalence of bullying victimisation amongst adolescents: role of peer and parental supports. EClinicalMedicine 20:100276. doi: 10.1016/j.eclinm.2020.100276
Bloomfield, M. A., McCutcheon, R. A., Kempton, M., Freeman, T. P., and Howes, O. (2019). The effects of psychosocial stress on dopaminergic function and the acute stress response. eLife 8:e46797. doi: 10.7554/eLife.46797
Bocchio, M., McHugh, S. B., Bannerman, D. M., Sharp, T., and Capogna, M. (2016). Serotonin, amygdala and fear: assembling the puzzle. Front. Neural Circuits 10:24. doi: 10.3389/fncir.2016.00024
Bollmer, J. M., Harris, M. J., and Milich, R. (2006). Reactions to bullying and peer victimization: narratives, physiological arousal and personality. J. Res. Personal. 40, 803–828. doi: 10.1016/j.jrp.2005.09.003
Bookbinder, S. H., and Brainerd, C. J. (2016). Emotion and false memory: the context-content paradox. Psychol. Bull. 142, 1315–1351. doi: 10.1037/bul0000077
Brainerd, C. J., and Reyna, V. F. (1998). Fuzzy-trace theory and children’s false memories. J. Exp. Child. Psychol. 71, 81–129. doi: 10.1006/jecp.1998.2464
Brainerd, C. J., and Reyna, V. F. (2005). The Science of False Memory (Vol. 38). Oxford: Oxford University Press.
Brainerd, C. J., and Reyna, V. F. (2007). Explaining developmental reversals in false memory. Psychol. Sci. 18, 442–448. doi: 10.1111/j.1467-9280.2007.01919.x
Brainerd, C. J., Reyna, V. F., and Holliday, R. E. (2018). Developmental reversals in false memory: development is complementary, not compensatory. Dev. Psychol. 54, 1773–1784. doi: 10.1037/dev0000554
Bremner, J. D., and Narayan, M. (1998). The effects of stress on memory and the hippocampus throughout the life cycle: implications for childhood development and aging. Dev. Psychopathol. 10, 871–885. doi: 10.1017/s0954579498001916
Brenet, F., Moh, M., Funk, P., Feierstein, E., Viale, A. J., Socci, N. D., et al. (2011). DNA methylation of the first exon is tightly linked to transcriptional silencing. PLoS One 6:e14524. doi: 10.1371/journal.pone.0014524
Brotman, M. A., Tseng, W. L., Olsavsky, A. K., Fromm, S. J., Muhrer, E. J., Rutenberg, J. G., et al. (2014). Fronto-limbic-striatal dysfunction in pediatric and adult patients with bipolar disorder: impact of face emotion and attentional demands. Psychol. Med. 44, 1639–1651. doi: 10.1017/S003329171300202X
Bunge, S. A., and Wright, S. B. (2007). Neurodevelopmental changes in working memory and cognitive control. Curr. Opin. Neurobiol. 17, 243–250. doi: 10.1016/j.conb.2007.02.005
Butts, K. A., Floresco, S. B., and Phillips, A. G. (2013). Acute stress impairs set-shifting but not reversal learning. Behav. Brain Res. 252, 222–229. doi: 10.1016/j.bbr.2013.06.007
Byrom, N. C., and Murphy, R. A. (2016). Individual difference in configural associative learning. J. Exp. Psychol. Anim. Learn. Cognit. 42, 325–335. doi: 10.1037/xan0000111
Caballero, A., Granberg, R., and Tseng, K. Y. (2016). Mechanisms contributing to prefrontal cortex maturation during adolescence. Neurosci. Biobehav. Rev. 70, 4–12. doi: 10.1016/j.neubiorev.2016.05.013
Cao, Y., Wang, M., Cao, C., Ji, L., and Zhang, W. (2017). The interaction between dopamine D2 receptor gene TaqIA polymorphim and peer victimization on early adolescent depression. Acta Psychol. Sin. 49, 28–39. doi: 10.3724/SP.J.1041.2017.00028
Carney, J. V., Hazler, R. J., Oh, I., Hibel, L. C., and Granger, D. A. (2010). The relations between bullying exposures in middle childhood, anxiety and adrenocortical activity. J. School Violence 9, 194–211. doi: 10.1080/15388220903479602
Carrion, V. G., Weems, C. F., and Reiss, A. L. (2007). Stress predicts brain changes in children: a pilot longitudinal study on youth stress, posttraumatic stress disorder and the hippocampus. Pediatrics 119, 509–516. doi: 10.1542/peds.2006-2028
Carroll, I. C., Planalp, E. M., Van Hulle, C. A., and Goldsmith, H. H. (2019). Peer victimization and selective attention in adolescence: evidence from a monozygotic twin difference design. J. Abnorm. Child Psychol. 47, 1303–1313. doi: 10.1007/s10802-019-00516-7
Casement, M. D., Guyer, A. E., Hipwell, A. E., McAloon, R. L., Hoffmann, A. M., Keenan, K. E., et al. (2014). Girls’ challenging social experiences in early adolescence predict neural response to rewards and depressive symptoms. Dev. Cogn. Neurosci. 8, 18–27. doi: 10.1016/j.dcn.2013.12.003
Cenquizca, L. A., and Swanson, L. W. (2007). Spatial organization of direct hippocampal field CA1 axonal projections to the rest of the cerebral cortex. Brain Res. Rev. 56, 1–26. doi: 10.1016/j.brainresrev.2007.05.002
Chan, J. R., Phillips, L. J., 2nd, and Glaser, M. (1998). Glucocorticoids and progestins signal the initiation and enhance the rate of myelin formation. Proc. Natl. Acad. Sci. U S A 95, 10459–10464. doi: 10.1073/pnas.95.18.10459
Chandler, L. A., and Shermis, M. D. (1986). Behavioral responses to stress: profile patterns of children. J. Clin. Child Psychol. 15, 317–322. doi: 10.1207/s15374424jccp1504_5
Chester, D. S., DeWall, C. N., and Pond, R. S. (2016). The push of social pain: does rejection’s sting motivate subsequent social reconnection. Cogn. Affect. Behav. Neurosci. 16, 541–550. doi: 10.3758/s13415-016-0412-9
Chester, D. S., Eisenberger, N. I., Pond, R. S., Jr, Richman, S. B., Bushman, B. J., and Dewall, C. N. (2014). The interactive effect of social pain and executive functioning on aggression: an fMRI experiment. Soc. Cogn. Affect. Neurosci. 9, 699–704. doi: 10.1093/scan/nst038
Christianson, J. P., and Greenwood, B. N. (2014). Stress-protective neural circuits: not all roads lead through the prefrontal cortex. Stress 17, 1–12. doi: 10.3109/10253890.2013.794450
Clemmensen, L., Jepsen, J. R. M., van Os, J., Blijd-Hoogewys, E. M. A., Rimvall, M. K., Olsen, E. M., et al. (2020). Are theory of mind and bullying separately associated with later academic performance among preadolescents. Br. J. Educ. Psychol. 90, 62–76. doi: 10.1111/bjep.12263
Cohen, H., Liberzon, I., and Richter-Levin, G. (2009). Exposure to extreme stress impairs contextual odour discrimination in an animal model of PTSD. Int. J. Neuropsychopharmacol. 12, 291–303. doi: 10.1017/S146114570800919X
Cole, D. A., Dukewich, T. L., Roeder, K., Sinclair, K. R., McMillan, J., Will, E., et al. (2014). Linking peer victimization to the development of depressive self-schemas in children and adolescents. J. Abnorm. Child Psychol. 42, 149–160. doi: 10.1007/s10802-013-9769-1
Collins, A., and Koechlin, E. (2012). Reasoning, learning and creativity: frontal lobe function and human decision-making. PLoS Biol. 10:e1001293. doi: 10.1371/journal.pbio.1001293
Conrad, C. D., LeDoux, J. E., Magariños, A. M., and McEwen, B. S. (1999). Repeated restraint stress facilitates fear conditioning independently of causing hippocampal CA3 dendritic atrophy. Behav. Neurosci. 113, 902–913. doi: 10.1037//0735-7044.113.5.902
Cowell, R. A., Cicchetti, D., Rogosch, F. A., and Toth, S. L. (2015). Childhood maltreatment and its effect on neurocognitive functioning: timing and chronicity matter. Dev. Psychopathol. 27, 521–533. doi: 10.1017/S0954579415000139
Dajani, D. R., and Uddin, L. Q. (2015). Demystifying cognitive flexibility: implications for clinical and developmental neuroscience. Trends Neurosci. 38, 571–578. doi: 10.1016/j.tins.2015.07.003
Darby, K. P., and Sloutsky, V. M. (2015). The cost of learning: interference effects in memory development. J. Exp. Psychol. Gen. 144, 410–431. doi: 10.1037/xge0000051
Davidson, R. J. (1992). Anterior cerebral asymmetry and the nature of emotion. Brain Cogn. 20, 125–151. doi: 10.1016/0278-2626(92)90065-t
De Bellis, M. D., Lefter, L., Trickett, P. K., Putnam, F. W., Jr (1994). Urinary catecholamine excretion in sexually abused girls. J. Am. Acad. Child Adolesc. Psychiatry 33, 320–327. doi: 10.1097/00004583-199403000-00004
De Kloet, E. R., and Derijk, R. (2004). Signaling pathways in brain involved in predisposition and pathogenesis of stress-related disease: genetic and kinetic factors affecting the MR/GR balance. Ann. N Y Acad. Sci. 1032, 14–34. doi: 10.1196/annals.1314.003
De Kloet, E. R., Meijer, O. C., de Nicola, A. F., de Rijk, R. H., and Joëls, M. (2018). Importance of the brain corticosteroid receptor balance in metaplasticity, cognitive performance and neuro-inflammation. Front. Neuroendocrinol. 49, 124–145. doi: 10.1016/j.yfrne.2018.02.003
De Kloet, E. R., Otte, C., Kumsta, R., Kok, L., Hillegers, M. H., Hasselmann, H., et al. (2016). Stress and depression: a crucial role of the mineralocorticoid receptor. J. Neuroendocrinol. 28. doi: 10.1111/jne.12379
De Kloet, E. R., Vreugdenhil, E., Oitzl, M. S., and Joëls, M. (1998). Brain corticosteroid receptor balance in health and disease. Endocr. Rev. 19, 269–301. doi: 10.1210/edrv.19.3.0331
Decety, J., Chen, C., Harenski, C., and Kiehl, K. A. (2013a). An fMRI study of affective perspective taking in individuals with psychopathy: imagining another in pain does not evoke empathy. Front. Hum. Neurosci. 7:489. doi: 10.3389/fnhum.2013.00489
Decety, J., Chenyi, C., Harenski, C., and Kiehl, K. A. (2013b). Neurological basis for lack of empathy in psychopaths. Front. Hum. Neurosci. 7:489. doi: 10.3389/fnhum.2013.00489
Decety, J., and Svetlova, M. (2012). Putting together phylogenetic and ontogenetic perspectives on empathy. Dev. Cogn. Neurosci. 2, 1–24. doi: 10.1016/j.dcn.2011.05.003
Delgado, M. R., Olsson, A., and Phelps, E. A. (2006). Extending animal models of fear conditioning to humans. Biol. Psychol. 73, 39–48. doi: 10.1016/j.biopsycho.2006.01.006
DeWall, C. N., and Baumeister, R. F. (2006). Alone but feeling no pain: effects of social exclusion on physical pain tolerance and pain threshold, affective forecasting and interpersonal empathy. J. Pers. Soc. Psychol. 91, 1–15. doi: 10.1037/0022-3514.91.1.1
Diekhof, E. K., Geier, K., Falkai, P., and Gruber, O. (2011). Fear is only as deep as the mind allows: a coordinate-based meta-analysis of neuroimaging studies on the regulation of negative affect. Neuroimage 58, 275–285. doi: 10.1016/j.neuroimage.2011.05.073
Doré, B. P., Boccagno, C., Burr, D., Hubbard, A., Long, K., Weber, J., et al. (2017). Finding positive meaning in negative experiences engages ventral striatal and ventromedial prefrontal regions associated with reward valuation. J. Cogn. Neurosci. 29, 235–244. doi: 10.17116/hirurgia202203116
Dougherty, L. R., Blankenship, S. L., Spechler, P. A., Padmala, S., and Pessoa, L. (2015). An fMRI pilot study of cognitive reappraisal in children: divergent effects on brain and behavior. J. Psychopathol. Behav. Assess. 37, 634–644. doi: 10.1007/s10862-015-9492-z
Drazdowski, T. K., Kliewer, W. L., Farrell, A., Sullivan, T., Roberson-Nay, R., and Jäggi, L. (2021). A longitudinal study of the bidirectional relations between anxiety symptoms and peer victimization in urban adolescents. J. Interpers. Violence 36, NP7183–NP7205. doi: 10.1177/0886260518824647
Du Plessis, M. R., Smeekens, S., Cillessen, A., Whittle, S., and Güroglu, B. (2019). Bullying the brain? Longitudinal links between childhood peer victimization, cortisol and adolescent brain structure. Front. Psychol. 9:2706. doi: 10.3389/fpsyg.2018.02706
Ducharme, S., Albaugh, M. D., Nguyen, T. V., Hudziak, J. J., Mateos-Pérez, J. M., Labbe, A., et al. (2016). Trajectories of cortical thickness maturation in normal brain development—the importance of quality control procedures. Neuroimage 125, 267–279. doi: 10.1016/j.neuroimage.2015.10.010
Dumontheil, I. (2014). Development of abstract thinking during childhood and adolescence: the role of rostrolateral prefrontal cortex. Dev. Cogn. Neurosci. 10, 57–76. doi: 10.1016/j.dcn.2014.07.009
Duncan, S., and Barrett, L. F. (2007). The role of the amygdala in visual awareness. Trends Cogn. Sci. 11, 190–192. doi: 10.1016/j.tics.2007.01.007
Dvash, J., and Shamay-Tsoory, S. G. (2014). Theory of mind and empathy as multidimensional constructs: neurological foundations. Top. Lang. Disord. 34, 282–295. doi: 10.1097/TLD.0000000000000040
Efron, D., Wijaya, M., Hazell, P., and Sciberras, E. (2021). Peer victimization in children with ADHD: a community-based longitudinal study. J. Attent. Disord. 25, 291–299. doi: 10.1177/1087054718796287
Egerton, A., Valmaggia, L. R., Howes, O. D., Day, F., Chaddock, C. A., Allen, P., et al. (2016). Adversity in childhood linked to elevated striatal dopamine function in adulthood. Schizophr. Res. 176, 171–176. doi: 10.1016/j.schres.2016.06.005
Ellis, B. J., Bianchi, J., Griskevicius, V., and Frankenhuis, W. E. (2017). Beyond risk and protective factors: an adaptation-based approach to resilience. Perspect. Psychol. Sci. 12, 561–587. doi: 10.1177/1745691617693054
Espelage, D. L., Hong, J. S., Rao, M. A., and Low, S. (2013). Associations between peer victimization and academic performance. Theory Pract. 52, 233–240. doi: 10.1080/00405841.2013.829724
Etkin, A., and Wager, T. D. (2007). Functional neuroimaging of anxiety: a meta-analysis of emotional processing in PTSD, social anxiety disorder and specific phobia. Am. J. Psychiatry 164, 1476–1488. doi: 10.1176/appi.ajp.2007.07030504
Faris, R., Felmlee, D., and McMillan, C. (2020). With friends like these: aggression from amity and equivalence. Am. J. Sociol. 126, 673–713. doi: 10.1086/712972
Floresco, S. B., Block, A. E., and Tse, M. T. (2008). Inactivation of the medial prefrontal cortex of the rat impairs strategy set-shifting, but not reversal learning, using a novel, automated procedure. Behav. Brain Res. 190, 85–96. doi: 10.1016/j.bbr.2008.02.008
Frank, D. W., Costa, V. D., Averbeck, B. B., and Sabatinelli, D. (2019). Directional interconnectivity of the human amygdala, fusiform gyrus and orbitofrontal cortex in emotional scene perception. J. Neurophysiol. 122, 1530–1537. doi: 10.1152/jn.00780.2018
Frick, A., Howner, K., Fischer, H., Kristiansson, M., and Furmark, T. (2013). Altered fusiform connectivity during processing of fearful faces in social anxiety disorder. Transl. Psychiatry 3:e312. doi: 10.1038/tp.2013.85
Frodl, T., Reinhold, E., Koutsouleris, N., Reiser, M., and Meisenzahl, E. M. (2010). Interaction of childhood stress with hippocampus and prefrontal cortex volume reduction in major depression. J. Psychiatr. Res. 44, 799–807. doi: 10.1016/j.jpsychires.2010.01.006
Furl, N., Henson, R. N., Friston, K. J., and Calder, A. J. (2013). Top-down control of visual responses to fear by the amygdala. J. Neurosci. 33, 17435–17443. doi: 10.1523/JNEUROSCI.2992-13.2013
Gallo, D. A., and Roediger, H. L., III (2002). Variability among word lists in eliciting memory illusions: evidence for associative activation and monitoring. J. Mem. Lang. 47, 469–497. doi: 10.1016/S0749-596X(02)00013-X
Gamo, N. J., Lur, G., Higley, M. J., Wang, M., Paspalas, C. D., Vijayraghavan, S., et al. (2015). Stress impairs prefrontal cortical function via D1 dopamine receptor interactions with hyperpolarization-activated cyclic nucleotide-gated channels. Biol. Psychiatry 78, 860–870. doi: 10.1016/j.biopsych.2015.01.009
Gao, J., Wang, H., Liu, Y., Li, Y. Y., Chen, C., Liu, L. M., et al. (2014). Glutamate and GABA imbalance promotes neuronal apoptosis in hippocampus after stress. Med. Sci. Monit. 20:499. doi: 10.12659/MSM.890589
George, S. A., Rodriguez-Santiago, M., Riley, J., Abelson, J. L., Floresco, S. B., and Liberzon, I. (2015). Alterations in cognitive flexibility in a rat model of post-traumatic stress disorder. Behav. Brain Res. 286, 256–264. doi: 10.1016/j.bbr.2015.02.051
Gilabert-Juan, J., Castillo-Gomez, E., Guirado, R., Moltó, M. D., and Nacher, J. (2013). Chronic stress alters inhibitory networks in the medial prefrontal cortex of adult mice. Brain Struct. Funct. 218, 1591–1605. doi: 10.1007/s00429-012-0479-1
Giletta, M., Slavich, G. M., Rudolph, K. D., Hastings, P. D., Nock, M. K., and Prinstein, M. J. (2018). Peer victimization predicts heightened inflammatory reactivity to social stress in cognitively vulnerable adolescents. J. Child Psychol. Psychiatry. 59, 129–139. doi: 10.1111/jcpp.12804
Gini, G., Marino, C., and Spada, M. M. (2019). The role of metacognitions and thinking styles in the negative outcomes of adolescents’ peer victimization. Violence Vict. 34, 752–769. doi: 10.1891/0886-6708.VV-D-18-00016
Goldfarb, E. V., Froböse, M. I., Cools, R., and Phelps, E. A. (2017). Stress and cognitive flexibility: cortisol increases are associated with enhanced updating but impaired switching. J. Cogn. Neurosci. 29, 14–24. doi: 10.1162/jocn_a_01029
Gotlib, I. H., and Joormann, J. (2010). Cognition and depression: current status and future directions. Ann. Rev. Clin. Psychol. 6, 285–312. doi: 10.1146/annurev.clinpsy.121208.131305
Graham, S., Bellmore, A., and Juvonen, J. (2003). Peer victimization in middle school: when self-and peer views diverge. J. Appl. School Psychol. 19, 117–137. doi: 10.1300/J008v19n02_08
Grosse Wiesmann, C., Schreiber, J., Singer, T., Steinbeis, N., and Friederici, A. D. (2017). White matter maturation is associated with the emergence of theory of mind in early childhood. Nat. Commun. 8:14692. doi: 10.1038/ncomms14692
Gupta, R., Koscik, T. R., Bechara, A., and Tranel, D. (2011). The amygdala and decision-making. Neuropsychologia 49, 760–766. doi: 10.1016/j.neuropsychologia.2010.09.029
Guyer, A. E., Monk, C. S., McClure-Tone, E. B., Nelson, E. E., Roberson-Nay, R., Adler, A. D., et al. (2008). A developmental examination of amygdala response to facial expressions. J. Cogn. Neurosci. 20, 1565–1582. doi: 10.1162/jocn.2008.20114
Haltigan, J. D., and Vaillancourt, T. (2014). Joint trajectories of bullying and peer victimization across elementary and middle school and associations with symptoms of psychopathology. Dev. Psychol. 50, 2426–2436. doi: 10.1037/a0038030
Hanson, J. L., Nacewicz, B. M., Sutterer, M. J., Cayo, A. A., Schaefer, S. M., Rudolph, K. D., et al. (2015). Behavioral problems after early life stress: contributions of the hippocampus and amygdala. Biol. Psychiatry 77, 314–323. doi: 10.1016/j.biopsych.2014.04.020
Hayes, J. P., VanElzakker, M. B., and Shin, L. M. (2012). Emotion and cognition interactions in PTSD: a review of neurocognitive and neuroimaging studies. Front. Integrat. Neurosci. 6:89. doi: 10.3389/fnint.2012.00089
Healey, M. L., and Grossman, M. (2018). Cognitive and affective perspective-taking: evidence for shared and dissociable anatomical substrates. Front. Neurol. 9:491. doi: 10.3389/fneur.2018.00491
Herringa, R. J., Burghy, C. A., Stodola, D. E., Fox, M. E., Davidson, R. J., and Essex, M. J. (2016). Enhanced prefrontal-amygdala connectivity following childhood adversity as a protective mechanism against internalizing in adolescence. Biol. Psychiatry. Cogn. Neurosci. Neuroimaging 1, 326–334. doi: 10.1016/j.bpsc.2016.03.003
Herrington, J. D., Taylor, J. M., Grupe, D. W., Curby, K. M., and Schultz, R. T. (2011). Bidirectional communication between amygdala and fusiform gyrus during facial recognition. Neuroimage 56, 2348–2355. doi: 10.1016/j.neuroimage.2011.03.072
Hiser, J., and Koenigs, M. (2018). The multifaceted role of the ventromedial prefrontal cortex in emotion, decision making, social cognition and psychopathology. Biol. Psychiatry 83, 638–647. doi: 10.1016/j.biopsych.2017.10.030
Holmberg, K., and Hjern, A. (2008). Bullying and attention-deficit-hyperactivity disorder in 10-year-olds in a Swedish community. Dev. Med. Child Neurol. 50, 134–138. doi: 10.1111/j.1469-8749.2007.02019.x
Hong, J. S., Kral, M. J., and Sterzing, P. R. (2015). Pathways from bullying perpetration, victimization and bully victimization to suicidality among school-aged youth: a review of the potential mediators and a call for further investigation. Trauma Violence Abuse 16, 379–390. doi: 10.1177/1524838014537904
Hou, Z., Sui, Y., Song, X., and Yuan, Y. (2016). Disrupted interhemispheric synchrony in default mode network underlying the impairment of cognitive flexibility in late-onset depression. Front. Aging Neurosci. 8:230. doi: 10.3389/fnagi.2016.00230
Howe, M. L. (2005). Children (but not adults) can inhibit false memories. Psychol. Sci. 16, 927–931. doi: 10.1111/j.1467-9280.2005.01638.x
Howe, M. L., Wimmer, M. C., and Blease, K. (2009). The role of associative strength in children’s false memory illusions. Memory 17, 8–16. doi: 10.1080/09658210802438474
Hulvershorn, L. A., Mennes, M., Castellanos, F. X., Di Martino, A., Milham, M. P., Hummer, T. A., et al. (2014). Abnormal amygdala functional connectivity associated with emotional lability in children with attention-deficit/hyperactivity disorder. J. Am. Acad. Child Adolesc. Psychiatry 53, 351–361.e1. doi: 10.1016/j.jaac.2013.11.012
Idsoe, T., Dyregrov, A., and Idsoe, E. C. (2012). Bullying and PTSD symptoms. J. Abnorm. Child Psychol. 40, 901–911. doi: 10.1007/s10802-012-9620-0
Idsoe, T., Vaillancourt, T., Dyregrov, A., Hagen, K. A., Ogden, T., and Nærde, A. (2021). Bullying victimization and trauma. Front. Psychiatry 11:480353. doi: 10.3389/fpsyt.2020.480353
Iffland, B., Sansen, L. M., Catani, C., and Neuner, F. (2014). The trauma of peer abuse: effects of relational peer victimization and social anxiety disorder on physiological and affective reactions to social exclusion. Front. Psychiatry 5:26. doi: 10.3389/fpsyt.2014.00026
Iidaka, T. (2014). Role of the fusiform gyrus and superior temporal sulcus in face perception and recognition: an empirical review. Jpn. Psychol. Res. 56, 33–45. doi: 10.1111/jpr.12018
Ikemoto, S. (2007). Dopamine reward circuitry: two projection systems from the ventral midbrain to the nucleus accumbens-olfactory tubercle complex. Brain Res. Rev. 56, 27–78. doi: 10.1016/j.brainresrev.2007.05.004
Jackowski, A. P., Douglas-Palumberi, H., Jackowski, M., Win, L., Schultz, R. T., Staib, L. W., et al. (2008). Corpus callosum in maltreated children with posttraumatic stress disorder: a diffusion tensor imaging study. Psychiatry Res. 162, 256–261. doi: 10.1016/j.pscychresns.2007.08.006
Janssens, A., Van Den Noortgate, W., Goossens, L., Verschueren, K., Colpin, H., De Laet, S., et al. (2015). Externalizing problem behavior in adolescence: dopaminergic genes in interaction with peer acceptance and rejection. J. Youth Adolesc. 44, 1441–1456. doi: 10.1007/s10964-015-0304-2
Jantzer, V., Ossa, F. C., Eppelmann, L., Parzer, P., Resch, F., and Kaess, M. (2021). Under the skin: does psychiatric outcome of bullying victimization in school persist over time? A prospective intervention study. J. Child Psychol. Psychiatry. [Online ahead of print] doi: 10.1111/jcpp.13502
Jarcho, J. M., Grossman, H. Y., Guyer, A. E., Quarmley, M., Smith, A. R., Fox, N. A., et al. (2019). Connecting childhood wariness to adolescent social anxiety through the brain and peer experiences. J. Abnorm. Child Psychol. 47, 1153–1164. doi: 10.1007/s10802-019-00543-4
Jenkins, L. N., and Canivez, G. L. (2021). Hierarchical factor structure of the Bullying Participant Behavior Questionnaire with a middle school sample. Int. J. School Educ. Psychol. 9, 55–72. doi: 10.1080/21683603.2019.1636734
Kaltiala-Heino, R., Rimpelä, M., Rantanen, P., and Rimpelä, A. (2000). Bullying at school—an indicator of adolescents at risk for mental disorders. J. Adolesc. 23, 661–674. doi: 10.1006/jado.2000.0351
Kanwisher, N., and Yovel, G. (2006). The fusiform face area: a cortical region specialized for the perception of faces. Philos. Trans. R. Soc. Lond. B Biol. Sci. 361, 2109–2128. doi: 10.1098/rstb.2006.1934
Kavanaugh, B. C., Dupont-Frechette, J. A., Jerskey, B. A., and Holler, K. A. (2017). Neurocognitive deficits in children and adolescents following maltreatment: neurodevelopmental consequences and neuropsychological implications of traumatic stress. Appl. Neuropsychol. Child 6, 64–78. doi: 10.1080/21622965.2015.1079712
Kennerley, S. W., Walton, M. E., Behrens, T. E., Buckley, M. J., and Rushworth, M. F. (2006). Optimal decision making and the anterior cingulate cortex. Nat. Neurosci. 9, 940–947. doi: 10.1038/nn1724
Kim, Y. K., Ham, B. J., and Han, K. M. (2019). Interactive effects of genetic polymorphisms and childhood adversity on brain morphologic changes in depression. Prog. Neuropsychopharmacol. Biol. Psychiatry 91, 4–13. doi: 10.1016/j.pnpbp.2018.03.009
Kino, T., Su, Y. A., and Chrousos, G. P. (2009). Human glucocorticoid receptor isoform beta: recent understanding of its potential implications in physiology and pathophysiology. Cell. Mol. Life Sci. 66, 3435–3448. doi: 10.1007/s00018-009-0098-z
Kliewer, W. (2016). Victimization and biological stress responses in urban adolescents: emotion regulation as a moderator. J. Youth Adolesc. 45, 1812–1823. doi: 10.1007/s10964-015-0398-6
Kliewer, W., Sosnowski, D. W., Noh, H., McGuire, K., and Wright, A. W. (2019). Peer victimization and cortisol production in children and adolescents: a systematic review. J. Appl. Biobehav. Res. 24:e12172. doi: 10.1111/jabr.12172
Knack, J. M., Tsar, V., Vaillancourt, T., Hymel, S., and McDougall, P. (2012). What protects rejected adolescents from also being bullied by their peers? The moderating role of peer-valued characteristics. J. Res. Adolesc. 22, 467–479. doi: 10.1111/j.1532-7795.2012.00792.x
Koyanagi, A., Oh, H., Carvalho, A. F., Smith, L., Haro, J. M., Vancampfort, D., et al. (2019). Bullying victimization and suicide attempt among adolescents aged 12-15 years from 48 countries. J. Am. Acad. Child Adolesc. Psychiatry 58, 907–918.e4. doi: 10.1016/j.jaac.2018.10.018
Kretschmer, T., Dijkstra, J. K., Ormel, J., Verhulst, F. C., and Veenstra, R. (2013). Dopamine receptor D4 gene moderates the effect of positive and negative peer experiences on later delinquency: the tracking adolescents’ individual lives survey study. Dev. Psychopathol. 25, 1107–1117. doi: 10.1017/S0954579413000400
Kross, E., Egner, T., Ochsner, K., Hirsch, J., and Downey, G. (2007). Neural dynamics of rejection sensitivity. J. Cogn. Neurosci. 19, 945–956. doi: 10.1162/jocn.2007.19.6.945
Krygsman, A., and Vaillancourt, T. (2019). Peer victimization, aggression, and depression symptoms in preschoolers. Early Child. Res. Q. 47, 62–73. doi: 10.1016/j.ecresq.2018.09.006
Kurkela, K. A., and Dennis, N. A. (2016). Event-related fMRI studies of false memory: an activation likelihood estimation meta-analysis. Neuropsychologia 81, 149–167. doi: 10.1016/j.neuropsychologia.2015.12.006
Lackner, C. L., Bowman, L. C., and Sabbagh, M. A. (2010). Dopaminergic functioning and preschoolers’ theory of mind. Neuropsychologia 48, 1767–1774. doi: 10.1016/j.neuropsychologia.2010.02.027
Lackner, C., Sabbagh, M. A., Hallinan, E., Liu, X., and Holden, J. J. (2012). Dopamine receptor D4 gene variation predicts preschoolers’ developing theory of mind. Dev. Sci. 15, 272–280. doi: 10.1111/j.1467-7687.2011.01124.x
Lago, T., Davis, A., Grillon, C., and Ernst, M. (2017). Striatum on the anxiety map: small detours into adolescence. Brain Res. 1654, 177–184. doi: 10.1016/j.brainres.2016.06.006
Laine, M. A., Trontti, K., Misiewicz, Z., Sokolowska, E., Kulesskaya, N., Heikkinen, A., et al. (2018). Genetic control of myelin plasticity after chronic psychosocial stress. eNeuro 5:ENEURO.0166-18.2018. doi: 10.1523/ENEURO.0166-18.2018
Langeslag, S. J., Schmidt, M., Ghassabian, A., Jaddoe, V. W., Hofman, A., van der Lugt, A., et al. (2013). Functional connectivity between parietal and frontal brain regions and intelligence in young children: the generation R study. Hum. Brain Mapp. 34, 3299–3307. doi: 10.1002/hbm.22143
Lapate, R. C., Rokers, B., Tromp, D. P., Orfali, N. S., Oler, J. A., Doran, S. T., et al. (2016). Awareness of emotional stimuli determines the behavioral consequences of amygdala activation and amygdala-prefrontal connectivity. Sci. Rep. 6:25826. doi: 10.1038/srep25826
Laurent, H. K., Gilliam, K. S., Wright, D. B., and Fisher, P. A. (2015). Child anxiety symptoms related to longitudinal cortisol trajectories and acute stress responses: evidence of developmental stress sensitization. J. Abnorm. Psychol. 124, 68–79. doi: 10.1037/abn0000009
Lavell, C. H., Webb, H. J., Zimmer-Gembeck, M. J., and Farrell, L. J. (2018). A prospective study of adolescents’ body dysmorphic symptoms: peer victimization and the direct and protective roles of emotion regulation and mindfulness. Body Image 24, 17–25. doi: 10.1016/j.bodyim.2017.11.006
Lavin, C., Melis, C., Mikulan, E. P., Gelormini, C., Huepe, D., and Ibañez, A. (2013). The anterior cingulate cortex: an integrative hub for human socially-driven interactions. Front. Neurosci. 7:64. doi: 10.3389/fnins.2013.00064
Lee, K. S., and Vaillancourt, T. (2018). Developmental pathways between peer victimization, psychological functioning, disordered eating behavior and body mass index: a review and theoretical model. Aggress. Violent Behav. 39, 15–24. doi: 10.1016/j.avb.2018.01.004
Lee, S. W., Yoo, J. H., Kim, K. W., Kim, D., Park, H., Choi, J., et al. (2018). Hippocampal subfields volume reduction in high schoolers with previous verbal abuse experiences. Clin. Psychopharmacol. Neurosci. 16, 46–56. doi: 10.9758/cpn.2018.16.1.46
Lee, K. H., Yoo, J. H., Lee, J., Kim, S. H., Han, J. Y., Hong, S. B., et al. (2020). The indirect effect of peer problems on adolescent depression through nucleus accumbens volume alteration. Sci. Rep. 10:12870. doi: 10.1038/s41598-020-69769-3
Lereya, S. T., Copeland, W. E., Costello, E. J., and Wolke, D. (2015). Adult mental health consequences of peer bullying and maltreatment in childhood: two cohorts in two countries. Lancet Psychiatry 2, 524–531. doi: 10.1016/S2215-0366(15)00165-0
Liu, J., Cao, F., Li, P., Lou, F., and Lavebratt, C. (2016). 5-HTTLPR, victimization and ecological executive function of adolescents. Psychiatry Res. 237, 55–59. doi: 10.1016/j.psychres.2016.01.059
Loe, I. M., and Feldman, H. M. (2007). Academic and educational outcomes of children with ADHD. Ambul. Pediatr. 7, 82–90. doi: 10.1016/j.ambp.2006.05.005
Lubin, F. D., Roth, T. L., and Sweatt, J. D. (2008). Epigenetic regulation of BDNF gene transcription in the consolidation of fear memory. J. Neurosci. 28, 10576–10586. doi: 10.1523/JNEUROSCI.1786-08.2008
Luna, B., and Sweeney, J. A. (2004). The emergence of collaborative brain function: FMRI studies of the development of response inhibition. Ann. N Y Acad. Sci. 1021, 296–309. doi: 10.1196/annals.1308.035
Lupien, S. J., Buss, C., Schramek, T. E., Maheu, F., and Pruessner, J. (2005). Hormetic influence of glucocorticoids on human memory. Nonlinearity Biol. Toxicol. Med. 3, 23–56. doi: 10.2201/nonlin.003.01.003
Mackey, S., Chaarani, B., Kan, K. J., Spechler, P. A., Orr, C., Banaschewski, T., et al. (2017). Brain regions related to impulsivity mediate the effects of early adversity on antisocial behavior. Biol. Psychiatry 82, 275–282. doi: 10.1016/j.biopsych.2015.12.027
Madalena, K. M., and Lerch, J. K. (2017). The effect of glucocorticoid and glucocorticoid receptor interactions on brain, spinal cord and glial cell plasticity. Neural Plast. 2017:8640970. doi: 10.1155/2017/8640970
Maier, S. F., Amat, J., Baratta, M. V., Paul, E., and Watkins, L. R. (2006). Behavioral control, the medial prefrontal cortex and resilience. Dialogues Clin. Neurosci. 8, 397–406. doi: 10.31887/DCNS.2006.8.4/smaier
Maier, S. F., and Watkins, L. R. (2010). Role of the medial prefrontal cortex in coping and resilience. Brain Res. 1355, 52–60. doi: 10.1016/j.brainres.2010.08.039
Malberg, J. E., Eisch, A. J., Nestler, E. J., and Duman, R. S. (2000). Chronic antidepressant treatment increases neurogenesis in adult rat hippocampus. J. Neurosci. 20, 9104–9110. doi: 10.1523/JNEUROSCI.20-24-09104.2000
Marchetti, I. (2019). Hopelessness: a network analysis. Cogn. Ther. Res. 43, 611–619. doi: 10.1007/s10608-018-9981-y
Markett, S., Wudarczyk, O. A., Biswal, B. B., Jawinski, P., and Montag, C. (2018). Affective network neuroscience. Front. Neurosci. 12:895. doi: 10.3389/fnins.2018.00895
McDermott, K. B., and Roediger, H. L., III (1998). Attempting to avoid illusory memories: robust false recognition of associates persists under conditions of explicit warnings and immediate testing. J. Mem. Lang. 39, 508–520. doi: 10.1006/jmla.1998.2582
McDonnell, J., Stahl, D., Day, F., McGuire, P., and Valmaggia, L. R. (2018). Interpersonal sensitivity in those at clinical high risk for psychosis mediates the association between childhood bullying victimisation and paranoid ideation: a virtual reality study. Schizophr. Res. 192, 89–95. doi: 10.1016/j.schres.2017.04.029
McDougall, P., and Vaillancourt, T. (2015). Long-term adult outcomes of peer victimization in childhood and adolescence: pathways to adjustment and maladjustment. Am. Psychol. 70, 300–310. doi: 10.1037/a0039174
McEwen, B. S. (1999). Stress and hippocampal plasticity. Annu. Rev. Neurosci. 22, 105–122. doi: 10.1146/annurev.neuro.22.1.105
McEwen, B. S. (2000). Effects of adverse experiences for brain structure and function. Biol. Psychiatry 48, 721–731. doi: 10.1016/s0006-3223(00)00964-1
McEwen, B. S. (2001). Plasticity of the hippocampus: adaptation to chronic stress and allostatic load. Ann. N Y Acad. Sci. 933, 265–277. doi: 10.1111/j.1749-6632.2001.tb05830.x
McGaugh, J. L. (2004). The amygdala modulates the consolidation of memories of emotionally arousing experiences. Annu. Rev. Neurosci. 27, 1–28. doi: 10.1146/annurev.neuro.27.070203.144157
McIver, T. A., Bosma, R. L., Goegan, S., Sandre, A., Klassen, J., Chiarella, J., et al. (2019). Functional connectivity across social inclusion and exclusion is related to peer victimization and depressive symptoms in young adults. J. Affect. Disord. 253, 366–375. doi: 10.1016/j.jad.2019.04.085
McLaughlin, K. A., Weissman, D., and Bitrán, D. (2019). Childhood adversity and neural development: a systematic review. Annu. Rev. Dev. Psychol. 1, 277–312. doi: 10.1146/annurev-devpsych-121318-084950
McQuade, J. D. (2017). Peer victimization and changes in physical and relational aggression: the moderating role of executive functioning abilities. Aggress. Behav. 43, 503–512. doi: 10.1002/ab.21708
Medeiros, W., Torro-Alves, N., Malloy-Diniz, L. F., and Minervino, C. M. (2016). Executive functions in children who experience bullying situations. Front. Psychol. 7:1197. doi: 10.3389/fpsyg.2016.01197
Metzger, R. L., Warren, A. R., Shelton, J. T., Price, J., Reed, A. W., and Williams, D. (2008). Do children “DRM” like adults? False memory production in children. Dev. Psychol. 44, 169–181. doi: 10.1037/0012-1649.44.1.169
Miranda, R., and Kihlstrom, J. (2005). Mood congruence in childhood and recent autobiographical memory. Cogn. Emot. 19, 981–998. doi: 10.1080/02699930500202967
Moench, K. M., and Wellman, C. L. (2015). Stress-induced alterations in prefrontal dendritic spines: implications for post-traumatic stress disorder. Neurosci. Lett. 601, 41–45. doi: 10.1016/j.neulet.2014.12.035
Monk, C. S., Klein, R. G., Telzer, E. H., Schroth, E. A., Mannuzza, S., Moulton, J. L., et al. (2008). Amygdala and nucleus accumbens activation to emotional facial expressions in children and adolescents at risk for major depression. Am. J. Psychiatry 165, 90–98. doi: 10.1176/appi.ajp.2007.06111917
Monk, C. S., McClure, E. B., Nelson, E. E., Zarahn, E., Bilder, R. M., Leibenluft, E., et al. (2003). Adolescent immaturity in attention-related brain engagement to emotional facial expressions. Neuroimage 20, 420–428. doi: 10.1016/s1053-8119(03)00355-0
Moore, S. E., Norman, R. E., Suetani, S., Thomas, H. J., Sly, P. D., and Scott, J. G. (2017). Consequences of bullying victimization in childhood and adolescence: a systematic review and meta-analysis. World J. Psychiatry 7, 60–76. doi: 10.5498/wjp.v7.i1.60
Morawetz, C., Bode, S., Baudewig, J., and Heekeren, H. R. (2017). Effective amygdala-prefrontal connectivity predicts individual differences in successful emotion regulation. Soc. Cogn. Affect. Neurosci. 12, 569–585. doi: 10.1093/scan/nsw169
Morey, R. A., Haswell, C. C., Hooper, S. R., and De Bellis, M. D. (2016). Amygdala, hippocampus and ventral medial prefrontal cortex volumes differ in maltreated youth with and without chronic posttraumatic stress disorder. Neuropsychopharmacology 41, 791–801. doi: 10.1038/npp.2015.205
Moriguchi, Y., Ohnishi, T., Mori, T., Matsuda, H., and Komaki, G. (2007). Changes of brain activity in the neural substrates for theory of mind during childhood and adolescence. Psychiatry Clin. Neurosci. 61, 355–363. doi: 10.1111/j.1440-1819.2007.01687.x
Morilak, D. A., Barrera, G., Echevarria, D. J., Garcia, A. S., Hernandez, A., Ma, S., et al. (2005). Role of brain norepinephrine in the behavioral response to stress. Prog. Neuropsychopharmacol. Biol. Psychiatry 29, 1214–1224. doi: 10.1016/j.pnpbp.2005.08.007
Mueller, E. M., and Pizzagalli, D. A. (2015). One-year-old fear memories rapidly activate human fusiform gyrus. Soc. Cogn. Affect. Neurosci. 11, 308–316. doi: 10.1093/scan/nsv122
Muetzel, R. L., Mulder, R. H., Lamballais, S., Cortes Hidalgo, A. P., Jansen, P., Güroğlu, B., et al. (2019). Frequent bullying involvement and brain morphology in children. Front. Psychiatry 10:696. doi: 10.3389/fpsyt.2019.00696
Mulej Bratec, S., Xie, X., Wang, Y., Schilbach, L., Zimmer, C., Wohlschläger, A. M., et al. (2017). Cognitive emotion regulation modulates the balance of competing influences on ventral striatal aversive prediction error signals. Neuroimage 147, 650–657. doi: 10.1016/j.neuroimage.2016.12.078
Murphy, F. C., Michael, A., and Sahakian, B. J. (2012). Emotion modulates cognitive flexibility in patients with major depression. Psychol. Med. 42, 1373–1382. doi: 10.1017/S0033291711002418
Murray-Close, D., Hoza, B., Hinshaw, S. P., Arnold, L. E., Swanson, J., Jensen, P. S., et al. (2010). Developmental processes in peer problems of children with attention-deficit/hyperactivity disorder in The Multimodal Treatment Study of Children With ADHD: developmental cascades and vicious cycles. Dev. Psychopathol. 22, 785–802. doi: 10.1017/S0954579410000465
Nakamoto, J., and Schwartz, D. (2010). Is peer victimization associated with academic achievement? A meta-analytic review. Soc. Dev. 19, 221–242. doi: 10.1111/j.1467-9507.2009.00539.x
Nansel, T. R., Overpeck, M., Pilla, R. S., Ruan, W. J., Simons-Morton, B., and Scheidt, P. (2001). Bullying behaviors among US youth: prevalence and association with psychosocial adjustment. JAMA 285, 2094–2100. doi: 10.1001/jama.285.16.2094
National Academies of Sciences, Engineering, and Medicine (2016). Preventing Bullying Through Science, Policy and Practice. Washington, DC: The National Academies Press.
Natu, V. S., Gomez, J., Barnett, M., Jeska, B., Kirilina, E., Jaeger, C., et al. (2019). Apparent thinning of human visual cortex during childhood is associated with myelination. Proc. Natl. Acad. Sci. U S A 116, 20750–20759. doi: 10.1073/pnas.1904931116
Nielsen, M. B., Tangen, T., Idsoe, T., Matthiesen, S. B., and Magerøy, N. (2015). Post-traumatic stress disorder as a consequence of bullying at work and at school. A literature review and meta-analysis. Aggress. Violent Behav. 21, 17–24. doi: 10.1016/j.avb.2015.01.001
Nishina, A., and Parra, L. A. (2019). Discrepancies between retrospective and actual self-reports of peer victimization six years earlier. J. Res. Adolesc. 29, 724–735. doi: 10.1111/jora.12410
Nishitani, N., Nagayasu, K., Asaoka, N., Yamashiro, M., Andoh, C., Nagai, Y., et al. (2019). Manipulation of dorsal raphe serotonergic neurons modulates active coping to inescapable stress and anxiety-related behaviors in mice and rats. Neuropsychopharmacology 44, 721–732. doi: 10.1038/s41386-018-0254-y
Olweus, D. (1994). Bullying at school: basic facts and effects of a school based intervention program. J. Child Psychol. Psychiatry 35, 1171–1190. doi: 10.1111/j.1469-7610.1994.tb01229.x
O’Neil, E. B., Newsome, R. N., Li, I. H., Thavabalasingam, S., Ito, R., and Lee, A. C. (2015). Examining the role of the human hippocampus in approach-avoidance decision making using a novel conflict paradigm and multivariate functional magnetic resonance Imaging. J. Neurosci. 35, 15039–15049. doi: 10.1523/JNEUROSCI.1915-15.2015
Östberg, V., Låftman, S. B., Modin, B., and Lindfors, P. (2018b). Bullying as a stressor in mid-adolescent girls and boys-associations with perceived stress, recurrent pain and salivary cortisol. Int. J. Environ. Res. Public Health 15:364. doi: 10.3390/ijerph15020364
Östberg, V., Modin, B., and Låftman, S. B. (2018a). Exposure to school bullying and psychological health in young adulthood: a prospective 10-year follow-up study. J. School Violence 17, 194–209. doi: 10.1080/15388220.2017.1296770
Oswald, L. M., Wand, G. S., Kuwabara, H., Wong, D. F., Zhu, S., and Brasic, J. R. (2014). History of childhood adversity is positively associated with ventral striatal dopamine responses to amphetamine. Psychopharmacology (Berl) 231, 2417–2433. doi: 10.1007/s00213-013-3407-z
Otgaar, H., Muris, P., Howe, M. L., and Merckelbach, H. (2017). What drives false memories in psychopathology? A case for associative activation. Clin. Psychol. Sci. 5, 1048–1069. doi: 10.1177/2167702617724424
Ouellet-Morin, I., Cantave, C., Paquin, S., Geoffroy, M. C., Brendgen, M., Vitaro, F., et al. (2021). Associations between developmental trajectories of peer victimization, hair cortisol and depressive symptoms: a longitudinal study. J. Child Psychol. Psychiatry 62, 19–27. doi: 10.1111/jcpp.13228
Ouellet-Morin, I., Danese, A., Bowes, L., Shakoor, S., Ambler, A., Pariante, C. M., et al. (2011a). A discordant monozygotic twin design shows blunted cortisol reactivity among bullied children. J. Am. Acad. Child Adolesc. Psychiatry 50, 574–582.e3. doi: 10.1016/j.jaac.2011.02.015
Ouellet-Morin, I., Odgers, C. L., Danese, A., Bowes, L., Shakoor, S., Papadopoulos, A. S., et al. (2011b). Blunted cortisol responses to stress signal social and behavioral problems among maltreated/bullied 12-year-old children. Biol. Psychiatry 70, 1016–1023. doi: 10.1016/j.biopsych.2011.06.017
Ouellet-Morin, I., Wong, C. C., Danese, A., Pariante, C. M., Papadopoulos, A. S., Mill, J., et al. (2013). Increased serotonin transporter gene (SERT) DNA methylation is associated with bullying victimization and blunted cortisol response to stress in childhood: a longitudinal study of discordant monozygotic twins. Psychol. Med. 43, 1813–1823. doi: 10.1017/S0033291712002784
Packard, M. G. (2009). Anxiety, cognition and habit: a multiple memory systems perspective. Brain Res. 1293, 121–128. doi: 10.1016/j.brainres.2009.03.029
Palamarchuk, I. S., and Vaillancourt, T. (2021). Mental resilience and coping with stress: a comprehensive, multi-level model of cognitive processing, decision making and behaviour. Front. Behav. Neurosci. 15:719674. doi: 10.3389/fnbeh.2021.719674
Pappa, I., Mileva-Seitz, V. R., Bakermans-Kranenburg, M. J., Tiemeier, H., and van IJzendoorn, M. H. (2015). The magnificent seven: a quantitative review of dopamine receptor d4 and its association with child behavior. Neurosci. Biobehav. Rev. 57, 175–186. doi: 10.1016/j.neubiorev.2015.08.009
Park, A. T., Tooley, U. A., Leonard, J. A., Boroshok, A. L., McDermott, C. L., Tisdall, M. D., et al. (2021). Early childhood stress is associated with blunted development of area functional connectivity. Dev. Cogn. Neurosci. 47:100909. doi: 10.1016/j.dcn.2020.100909
Parker, N., Patel, Y., Jackowski, A. P., Pan, P. M., Salum, G. A., Pausova, Z., et al. (2020). Assessment of neurobiological mechanisms of cortical thinning during childhood and adolescence and their implications for psychiatric disorders. JAMA Psychiatry 77, 1127–1136. doi: 10.1001/jamapsychiatry.2020.1495
Paus, T. (2005). Mapping brain maturation and cognitive development during adolescence. Trends Cogn. Sci. 9, 60–68. doi: 10.1016/j.tics.2004.12.008
Payne, J. D., Jackson, E. D., Hoscheidt, S., Ryan, L., Jacobs, W. J., and Nadel, L. (2007). Stress administered prior to encoding impairs neutral but enhances emotional long-term episodic memories. Learn. Mem. 14, 861–868. doi: 10.1101/lm.743507
Perino, M. T., Moreira, J. F. G., and Telzer, E. H. (2019). Links between adolescent bullying and neural activation to viewing social exclusion. Cogn. Affect. Behav. Neurosci. 19, 1467–1478. doi: 10.3758/s13415-019-00739-7
Phan, K. L., Fitzgerald, D. A., Nathan, P. J., Moore, G. J., Uhde, T. W., and Tancer, M. E. (2005). Neural substrates for voluntary suppression of negative affect: a functional magnetic resonance imaging study. Biol. Psychiatry 57, 210–219. doi: 10.1016/j.biopsych.2004.10.030
Phelps, E. A., Delgado, M. R., Nearing, K. I., and LeDoux, J. E. (2004). Extinction learning in humans: role of the amygdala and vmPFC. Neuron 43, 897–905. doi: 10.1016/j.neuron.2004.08.042
Piccolo, L. R., Merz, E. C., He, X., Sowell, E. R., Noble, K. G., and Pediatric Imaging. (2016). Age-related differences in cortical thickness vary by socioeconomic status. PLoS One 11:e0162511. doi: 10.1371/journal.pone.0162511
Picó-Pérez, M., Radua, J., Steward, T., Menchón, J. M., and Soriano-Mas, C. (2017). Emotion regulation in mood and anxiety disorders: a meta-analysis of fMRI cognitive reappraisal studies. Prog. Neuropsychopharmacol. Biol. Psychiatry 79, 96–104. doi: 10.1016/j.pnpbp.2017.06.001
Plessow, F., Kiesel, A., and Kirschbaum, C. (2012). The stressed prefrontal cortex and goal-directed behaviour: acute psychosocial stress impairs the flexible implementation of task goals. Exp. Brain Res. 216, 397–408. doi: 10.1007/s00221-011-2943-1
Pluta, R., Jolkkonen, J., Cuzzocrea, S., Pedata, F., Cechetto, D., and Popa-Wagner, A. (2011). Cognitive impairment with vascular impairment and degeneration. Curr. Neurovasc. Res. 8, 342–350. doi: 10.2174/156720211798120981
Pouwels, J. L., Lansu, T. A., and Cillessen, A. H. (2018). A developmental perspective on popularity and the group process of bullying. Aggress. Violent Behav. 43, 64–70. doi: 10.1016/j.avb.2018.10.003
Powers, K. E., Wagner, D. D., Norris, C. J., and Heatherton, T. F. (2013). Socially excluded individuals fail to recruit medial prefrontal cortex for negative social scenes. Soc. Cogn. Affect. Neurosci. 8, 151–157. doi: 10.1093/scan/nsr079
Prakash, N., Stark, C. J., Keisler, M. N., Luo, L., Der-Avakian, A., and Dulcis, D. (2020). Serotonergic plasticity in the dorsal raphe nucleus characterizes susceptibility and resilience to anhedonia. J. Neurosci. 40, 569–584. doi: 10.1523/JNEUROSCI.1802-19.2019
Price, H. L., and Phenix, T. L. (2015). True (but not false) memories are subject to retrieval-induced forgetting in children. J. Exp. Child Psychol. 133, 1–15. doi: 10.1016/j.jecp.2015.01.009
Prochazkova, E., and Kret, M. E. (2017). Connecting minds and sharing emotions through mimicry: a neurocognitive model of emotional contagion. Neurosci. Biobehav. Rev. 80, 99–114. doi: 10.1016/j.neubiorev.2017.05.013
Quinlan, E. B., Barker, E. D., Luo, Q., Banaschewski, T., Bokde, A., Bromberg, U., et al. (2020). Peer victimization and its impact on adolescent brain development and psychopathology. Mol. Psychiatry 25, 3066–3076. doi: 10.1038/s41380-018-0297-9
Rabinak, C. A., and Maren, S. (2008). Associative structure of fear memory after basolateral amygdala lesions in rats. Behav. Neurosci. 122, 1284–1294. doi: 10.1037/a0012903
Ralph, M. A., Jefferies, E., Patterson, K., and Rogers, T. T. (2017). The neural and computational bases of semantic cognition. Nat. Rev. Neurosci. 18, 42–55. doi: 10.1038/nrn.2016.150
Rappaport, B. I., Hennefield, L., Kujawa, A., Arfer, K. B., Kelly, D., Kappenman, E. S., et al. (2019). Peer victimization and dysfunctional reward processing: ERP and behavioral responses to social and monetary rewards. Front. Behav. Neurosci. 13:120. doi: 10.3389/fnbeh.2019.00120
Raslau, F. D., Klein, A. P., Ulmer, J. L., Mathews, V., and Mark, L. P. (2014). Memory part 1: overview. Am. J. Neuroradiol. 35, 2058–2060. doi: 10.3174/ajnr.A4059
Raslau, F. D., Mark, I. T., Klein, A. P., Ulmer, J. L., Mathews, V., and Mark, L. P. (2015). Memory part 2: the role of the medial temporal lobe. Am. J. Neuroradiol. 36, 846–849. doi: 10.3174/ajnr.A4169
Ratka, A. (2018). Empathy and the development of affective skills. Am. J. Pharm. Educ. 82:7192. doi: 10.5688/ajpe7192
Reijntjes, A., Kamphuis, J. H., Prinzie, P., Boelen, P. A., Van der Schoot, M., and Telch, M. J. (2011). Prospective linkages between peer victimization and externalizing problems in children: a meta-analysis. Aggress. Behav. 37, 215–222. doi: 10.1002/ab.20374
Reijntjes, A., Kamphuis, J. H., Prinzie, P., and Telch, M. J. (2010). Peer victimization and internalizing problems in children: a meta-analysis of longitudinal studies. Child Abuse Negl. 34, 244–252. doi: 10.1016/j.chiabu.2009.07.009
Reneaux, M., and Gupta, R. (2018). Prefronto-cortical dopamine D1 receptor sensitivity can critically influence working memory maintenance during delayed response tasks. PLoS One 13:e0198136. doi: 10.1371/journal.pone.0198136
Renouf, A., Brendgen, M., Parent, S., Vitaro, F., David Zelazo, P., Boivin, M., et al. (2010). Relations between theory of mind and indirect and physical aggression in kindergarten: evidence of the moderating role of prosocial behaviors. Soc. Dev. 19, 535–555. doi: 10.1111/j.1467-9507.2009.00552.x
Riem, M. M., Alink, L. R., Out, D., Van Ijzendoorn, M. H., and Bakermans-Kranenburg, M. J. (2015). Beating the brain about abuse: empirical and meta-analytic studies of the association between maltreatment and hippocampal volume across childhood and adolescence. Dev. Psychopathol. 27, 507–520. doi: 10.1017/S0954579415000127
Riley, T. N., Sullivan, T. N., Hinton, T. S., and Kliewer, W. (2019). Longitudinal relations between emotional awareness and expression, emotion regulation and peer victimization among urban adolescents. J. Adolesc. 72, 42–51. doi: 10.1016/j.adolescence.2019.02.005
Robbins, T. W., Ersche, K. D., and Everitt, B. J. (2008). Drug addiction and the memory systems of the brain. Ann. N Y Acad. Sci. 1141, 1–21. doi: 10.1196/annals.1441.020
Rolls, E. T. (2019). The cingulate cortex and limbic systems for emotion, action and memory. Brain Struct. Funct. 224, 3001–3018. doi: 10.1007/s00429-019-01945-2
Rosso, I. M., Young, A. D., Femia, L. A., and Yurgelun-Todd, D. A. (2004). Cognitive and emotional components of frontal lobe functioning in childhood and adolescence. Ann. N Y Acad. Sci. 1021, 355–362. doi: 10.1196/annals.1308.045
Rudolph, K. D., Miernicki, M. E., Troop-Gordon, W., Davis, M. M., and Telzer, E. H. (2016). Adding insult to injury: neural sensitivity to social exclusion is associated with internalizing symptoms in chronically peer-victimized girls. Soc. Cogn. Affect. Neurosci. 11, 829–842. doi: 10.1093/scan/nsw021
Sabbagh, M. A., Bowman, L. C., Evraire, L. E., and Ito, J. M. (2009). Neurodevelopmental correlates of theory of mind in preschool children. Child Dev. 80, 1147–1162. doi: 10.1111/j.1467-8624.2009.01322.x
Sansen, L. M., Iffland, B., and Neuner, F. (2015). The trauma of peer victimization: psychophysiological and emotional characteristics of memory imagery in subjects with social anxiety disorder. Psychophysiology 52, 107–116. doi: 10.1111/psyp.12291
Sato, J. R., Salum, G. A., Gadelha, A., Vieira, G., Zugman, A., Picon, F. A., et al. (2015). Decreased centrality of subcortical regions during the transition to adolescence: a functional connectivity study. Neuroimage 104, 44–51. doi: 10.1016/j.neuroimage.2014.09.063
Sauro, M. D., Jorgensen, R. S., and Pedlow, C. T. (2003). Stress, glucocorticoids and memory: a meta-analytic review. Stress 6, 235–245. doi: 10.1080/10253890310001616482
Schmithorst, V. J., and Yuan, W. (2010). White matter development during adolescence as shown by diffusion MRI. Brain Cogn. 72, 16–25. doi: 10.1016/j.bandc.2009.06.005
Schneiderman, N., Ironson, G., and Siegel, S. D. (2005). Stress and health: psychological, behavioral and biological determinants. Annu. Rev. Clin. Psychol. 1, 607–628. doi: 10.1146/annurev.clinpsy.1.102803.144141
Schultz, R. T., Grelotti, D. J., Klin, A., Kleinman, J., Van der Gaag, C., Marois, R., et al. (2003). The role of the fusiform face area in social cognition: implications for the pathobiology of autism. Philos. Trans. R. Soc. Lond. B Biol. Sci. 358, 415–427. doi: 10.1098/rstb.2002.1208
Schurz, M., Aichhorn, M., Martin, A., and Perner, J. (2013). Common brain areas engaged in false belief reasoning and visual perspective taking: a meta-analysis of functional brain imaging studies. Front. Hum. Neurosci. 7:712. doi: 10.3389/fnhum.2013.00712
Schurz, M., Radua, J., Aichhorn, M., Richlan, F., and Perner, J. (2014). Fractionating theory of mind: a meta-analysis of functional brain imaging studies. Neurosci. Biobehav. Rev. 42, 9–34. doi: 10.1016/j.neubiorev.2014.01.009
Sengupta, A., and Holmes, A. (2019). A discrete dorsal raphe to basal amygdala 5-HT circuit calibrates aversive memory. Neuron 103, 489–505.e7. doi: 10.1016/j.neuron.2019.05.029
Sentse, M., Prinzie, P., and Salmivalli, C. (2017). Testing the direction of longitudinal paths between victimization, peer rejection and different types of internalizing problems in adolescence. J. Abnorm. Child Psychol. 45, 1013–1023. doi: 10.1007/s10802-016-0216-y
Shakoor, S., Jaffee, S. R., Bowes, L., Ouellet-Morin, I., Andreou, P., Happé, F., et al. (2012). A prospective longitudinal study of children’s theory of mind and adolescent involvement in bullying. J. Child Psychol. Psychiatry 53, 254–261. doi: 10.1111/j.1469-7610.2011.02488.x
Shamay-Tsoory, S. G., and Aharon-Peretz, J. (2007). Dissociable prefrontal networks for cognitive and affective theory of mind: a lesion study. Neuropsychologia 45, 3054–3067. doi: 10.1016/j.neuropsychologia.2007.05.021
Shamay-Tsoory, S. G., Tibi-Elhanany, Y., and Aharon-Peretz, J. (2006). The ventromedial prefrontal cortex is involved in understanding affective but not cognitive theory of mind stories. Soc. Neurosci. 1, 149–166. doi: 10.1080/17470910600985589
Shamay-Tsoory, S. G., Tibi-Elhanany, Y., and Aharon-Peretz, J. (2007). The green-eyed monster and malicious joy: the neuroanatomical bases of envy and gloating (schadenfreude). Brain 130, 1663–1678. doi: 10.1093/brain/awm093
Shany-Ur, T., and Rankin, K. P. (2014). “Cognition, social,” in Encyclopedia of the Neurological Sciences, 2nd Edn, eds M. J. Aminoff, and R. B. Daroff (Academic Press), 814–817. doi: 10.1016/B978-0-12-385157-4.00461-9
CrossRef Full Text | Google Scholar. Available online at: https://www.sciencedirect.com/science/article/pii/B9780123851574004619.
Shaw, P., Eckstrand, K., Sharp, W., Blumenthal, J., Lerch, J. P., Greenstein, D., et al. (2007). Attention-deficit/hyperactivity disorder is characterized by a delay in cortical maturation. Proc. Natl. Acad. Sci. U S A 104, 19649–19654. doi: 10.1073/pnas.0707741104
Shaw, P., Kabani, N. J., Lerch, J. P., Eckstrand, K., Lenroot, R., Gogtay, N., et al. (2008). Neurodevelopmental trajectories of the human cerebral cortex. J. Neurosci. 28, 3586–3594. doi: 10.1523/JNEUROSCI.5309-07.2008
Shaw, P., Lerch, J., Greenstein, D., Sharp, W., Clasen, L., Evans, A., et al. (2006). Longitudinal mapping of cortical thickness and clinical outcome in children and adolescents with attention-deficit/hyperactivity disorder. Arch. Gen. Psychiatry 63, 540–549. doi: 10.1001/archpsyc.63.5.540
Shin, H., Lee, D. H., Yu, K., and Ham, K. (2016). The relationship between parental bonding and peer victimization: examining child stress and hopelessness as mediators. Asia Pacific Educ. Rev. 17, 637–650. doi: 10.1007/s12564-016-9434-9
Sijtsema, J. J., Veenstra, R., Lindenberg, S., and Salmivalli, C. (2009). Empirical test of bullies’ status goals: assessing direct goals, aggression, and prestige. Aggress. Behav. 35, 57–67. doi: 10.1002/ab.20282
Silberg, J. L., Copeland, W., Linker, J., Moore, A. A., Roberson-Nay, R., and York, T. P. (2016). Psychiatric outcomes of bullying victimization: a study of discordant monozygotic twins. Psychol. Med. 46, 1875–1883. doi: 10.1017/S0033291716000362
Silk, J. S., Siegle, G. J., Lee, K. H., Nelson, E. E., Stroud, L. R., and Dahl, R. E. (2014). Increased neural response to peer rejection associated with adolescent depression and pubertal development. Soc. Cogn. Affect. Neurosci. 9, 1798–1807. doi: 10.1093/scan/nst175
Silveri, M. M., Rohan, M. L., Pimentel, P. J., Gruber, S. A., Rosso, I. M., and Yurgelun-Todd, D. A. (2006). Sex differences in the relationship between white matter microstructure and impulsivity in adolescents. Magn. Reson. Imaging 24, 833–841. doi: 10.1016/j.mri.2006.03.012
Sinclair, K. R., Cole, D. A., Dukewich, T., Felton, J., Weitlauf, A. S., Maxwell, M. A., et al. (2012). Impact of physical and relational peer victimization on depressive cognitions in children and adolescents. J. Clin. Child Adolesc. Psychol. 41, 570–583. doi: 10.1080/15374416.2012.704841
Skosnik, P. D., Chatterton Jr, R. T., Swisher, T., and Park, S. (2000). Modulation of attentional inhibition by norepinephrine and cortisol after psychological stress. Int. J. Psychophysiol. 36, 59–68. doi: 10.1016/s0167-8760(99)00100-2
Sladek, M. R., Doane, L. D., Luecken, L. J., and Eisenberg, N. (2016). Perceived stress, coping and cortisol reactivity in daily life: a study of adolescents during the first year of college. Biol. Psychol. 117, 8–15. doi: 10.1016/j.biopsycho.2016.02.003
Slattery, M. J., Grieve, A. J., Ames, M. E., Armstrong, J. M., and Essex, M. J. (2013). Neurocognitive function and state cognitive stress appraisal predict cortisol reactivity to an acute psychosocial stressor in adolescents. Psychoneuroendocrinology 38, 1318–1327. doi: 10.1016/j.psyneuen.2012.11.017
Sosnowski, D. W., Kliewer, W., and Lepore, S. J. (2016). the role of sleep in the relationship between victimization and externalizing problems in adolescents. J. Youth Adolesc. 45, 1744–1754. doi: 10.1007/s10964-016-0506-2
Sousa, N., Lukoyanov, N. V., Madeira, M. D., Almeida, O. F. X., and Paula-Barbosa, M. M. (2000). Reorganization of the morphology of hippocampal neurites and synapses after stress-induced damage correlates with behavioral improvement. Neuroscience 97, 253–266. doi: 10.1016/s0306-4522(00)00050-6
Sowell, E. R., Thompson, P. M., and Toga, A. W. (2004). Mapping changes in the human cortex throughout the span of life. Neuroscientist 10, 372–392. doi: 10.1177/1073858404263960
Staudinger, M. R., Erk, S., Abler, B., and Walter, H. (2009). Cognitive reappraisal modulates expected value and prediction error encoding in the ventral striatum. NeuroImage 47, 713–721. doi: 10.1016/j.neuroimage.2009.04.095
Stewart, J. L., Levin-Silton, R., Sass, S. M., Heller, W., and Miller, G. A. (2008). Anger style, psychopathology and regional brain activity. Emotion 8, 701–713. doi: 10.1037/a0013447
Stuss, D. T., Gallup, G. G., Jr., and Alexander, M. P. (2001). The frontal lobes are necessary for ‘theory of mind’. Brain 124, 279–286. doi: 10.1093/brain/124.2.279
Surget, A., Tanti, A., Leonardo, E. D., Laugeray, A., Rainer, Q., Touma, C., et al. (2011). Antidepressants recruit new neurons to improve stress response regulation. Mol. Psychiatry 16, 1177–1188. doi: 10.1038/mp.2011.48
Swartz, J. R., Carranza, A. F., and Knodt, A. R. (2019). Amygdala activity to angry and fearful faces relates to bullying and victimization in adolescents. Soc. Cogn. Affect. Neurosci. 14, 1027–1035. doi: 10.1093/scan/nsz084
Szyf, M., and Bick, J. (2013). DNA methylation: a mechanism for embedding early life experiences in the genome. Child Dev. 84, 49–57. doi: 10.1111/j.1467-8624.2012.01793.x
Takami, K., and Haruno, M. (2020). Dissociable behavioral and neural correlates for target-changing and conforming behaviors in interpersonal aggression. eNeuro 7:ENEURO.0273-19.2020. doi: 10.1523/ENEURO.0273-19.2020
Takizawa, R., Maughan, B., and Arseneault, L. (2014). Adult health outcomes of childhood bullying victimization: evidence from a five-decade longitudinal British birth cohort. Am. J. Psychiatry 171, 777–784. doi: 10.1176/appi.ajp.2014.13101401
Taliaz, D., Loya, A., Gersner, R., Haramati, S., Chen, A., and Zangen, A. (2011). Resilience to chronic stress is mediated by hippocampal brain-derived neurotrophic factor. J. Neurosci. 31, 4475–4483. doi: 10.1523/JNEUROSCI.5725-10.2011
Tallarita, G. M., Parente, A., Didato, G., and Giovagnoli, A. R. (2020). The fusiform gyrus and theory of mind: a case study. Acta Neurol. Belg. 120, 973–975. doi: 10.1007/s13760-019-01235-y
Tamnes, C. K., Østby, Y., Fjell, A. M., Westlye, L. T., Due-Tønnessen, P., and Walhovd, K. B. (2010). Brain maturation in adolescence and young adulthood: regional age-related changes in cortical thickness and white matter volume and microstructure. Cereb. Cortex 20, 534–548. doi: 10.1093/cercor/bhp118
Taylor, L. A., Saylor, C., Twyman, K., and Macias, M. (2010). Adding insult to injury: bullying experiences of youth with attention deficit hyperactivity disorder. Child. Health Care 39, 59–72. doi: 10.1080/02739610903455152
Teicher, M. H., Anderson, C. M., and Polcari, A. (2012). Childhood maltreatment is associated with reduced volume in the hippocampal subfields CA3, dentate gyrus and subiculum. Proc. Natl. Acad. Sci. U S A 109, E563–E572. doi: 10.1073/pnas.1115396109
Telzer, E. H., Fowler, C. H., Davis, M. M., and Rudolph, K. D. (2020). Hungry for inclusion: exposure to peer victimization and heightened social monitoring in adolescent girls. Dev. Psychopathol. 32, 1495–1508. doi: 10.1017/S0954579419001433
Telzer, E. H., Miernicki, M. E., and Rudolph, K. D. (2018). Chronic peer victimization heightens neural sensitivity to risk taking. Dev. Psychopathol. 30, 13–26. doi: 10.1017/S0954579417000438
Thye, M. D., Murdaugh, D. L., and Kana, R. K. (2018). Brain mechanisms underlying reading the mind from eyes, voice and actions. Neuroscience 374, 172–186. doi: 10.1016/j.neuroscience.2018.01.045
Troop-Gordon, W., and Ladd, G. W. (2005). Trajectories of peer victimization and perceptions of the self and schoolmates: precursors to internalizing and externalizing problems. Child Dev. 76, 1072–1091. doi: 10.1111/j.1467-8624.2005.00898.x
Ttofi, M. M., Farrington, D. P., Lösel, F., and Loeber, R. (2011). Do the victims of school bullies tend to become depressed later in life? A systematic review and meta-analysis of longitudinal studies. J. Aggress. Confl. Peace Res. 3, 63–73. doi: 10.1108/17596591111132873
Tulving, E., and Markowitsch, H. J. (1998). Episodic and declarative memory: role of the hippocampus. Hippocampus 8, 198–204. doi: 10.1002/(SICI)1098-1063(1998)8:3<98::AID-HIPO2>3.0.CO;2-G
Underwood, M. K., and Ehrenreich, S. E. (2014). Bullying may be fueled by the desperate need to belong. Theory Pract. 53, 265–270. doi: 10.1080/00405841.2014.947217
UNICEF. (2020). Report Card 16. Available online at: https://www.unicef.ca/en/unicef-report-card-16.
Urban, K. R., Geng, E., Bhatnagar, S., and Valentino, R. J. (2019). Age- and sex-dependent impact of repeated social stress on morphology of rat prefrontal cortex pyramidal neurons. Neurobiol. Stress 10:100165. doi: 10.1016/j.ynstr.2019.100165
Vaillancourt, T. (2018). Introduction to the special issue: the neurobiology of peer victimization. Merrill-Palmer Q. 64, 1–11. doi: 10.13110/merrpalmquar1982.64.1.0001
Vaillancourt, T., Brittain, H. L., McDougall, P., and Duku, E. (2013). Longitudinal links between childhood peer victimization, internalizing and externalizing problems and academic functioning: developmental cascades. J. Abnorm. Child Psychlol. 41, 1203–1215. doi: 10.1007/s10802-013-9781-5
Vaillancourt, T., Duku, E., Becker, S., Schmidt, L. A., Nicol, J., Muir, C., et al. (2011). Peer victimization, depressive symptoms and high salivary cortisol predict poorer memory in children. Brain Cogn. 77, 191–199. doi: 10.1016/j.bandc.2011.06.012
Vaillancourt, T., Hymel, S., and McDougall, P. (2003). Bullying is power: implications for school-based intervention strategies. J. Appl. School Psychol. 19, 157–176. doi: 10.1300/J008v19n02_10
Vaillancourt, T., and McDougall, P. (2013). The link between childhood exposure to violence and academic achievement: complex pathways. J. Abnorm. Child Psychlol. 41, 1177–1178. doi: 10.1007/s10802-013-9803-3
Vaillancourt, T., McDougall, P., Hymel, S., Krygsman, A., Miller, J., Stiver, K., et al. (2008). Bullying: are researchers and children/youth talking about the same thing? Int. J. Behav. Dev. 32, 486–495. doi: 10.1177/0165025408095553
Vaillancourt, T., and Palamarchuk, I. S. (2021). “Neurobiological factors of bullying victimization,” in The Wiley Blackwell Handbook of Bullying, eds P. K. Smith, J. O’Higgins Norman (Oxford: Blackwell Publishing), 390–405. doi: 10.1002/9781118482650.ch22
Valentino, R. J., and Van Bockstaele, E. (2008). Convergent regulation of locus coeruleus activity as an adaptive response to stress. Eur. J. Ppharmacol. 583, 194–203. doi: 10.1016/j.ejphar.2007.11.062
van Geel, M., Goemans, A., and Vedder, P. H. (2016). The relation between peer victimization and sleeping problems: a meta-analysis. Sleep Med. Rev. 27, 89–95. doi: 10.1016/j.smrv.2015.05.004
Vargas, T., Damme, K., and Mittal, V. A. (2019). Bullying victimization in typically developing and clinical high risk (CHR) adolescents: a multimodal imaging study. Schizophr. Res. 213, 40–47. doi: 10.1016/j.schres.2018.11.017
Vaske, J., Wright, J. P., and Beaver, K. M. (2011). A dopamine gene (DRD2) distinguishes between offenders who have and have not been violently victimized. Int. J. Offender Ther. Comp. Criminol. 55, 251–267. doi: 10.1177/0306624X10361583
Verlinden, M., Veenstra, R., Ghassabian, A., Jansen, P. W., Hofman, A., Jaddoe, V. W., et al. (2014). Executive functioning and non-verbal intelligence as predictors of bullying in early elementary school. J. Abnorm. Child Psychol. 42, 953–966. doi: 10.1007/s10802-013-9832-y
Vogel, S., Klumpers, F., Schröder, T. N., Oplaat, K. T., Krugers, H. J., Oitzl, M. S., et al. (2017). Stress induces a shift towards striatum-dependent stimulus-response learning via the mineralocorticoid receptor. Neuropsychopharmacology 42, 1262–1271. doi: 10.1038/npp.2016.262
Vogel, S., and Schwabe, L. (2016). Learning and memory under stress: implications for the classroom. NPJ Sci. Learn. 1:16011. doi: 10.1038/npjscilearn.2016.11
Völlm, B. A., Taylor, A. N., Richardson, P., Corcoran, R., Stirling, J., McKie, S., et al. (2006). Neuronal correlates of theory of mind and empathy: a functional magnetic resonance imaging study in a nonverbal task. NeuroImage 29, 90–98. doi: 10.1016/j.neuroimage.2005.07.022
Vyas, S., Rodrigues, A. J., Silva, J. M., Tronche, F., Almeida, O. F., Sousa, N., et al. (2016). Chronic stress and glucocorticoids: from neuronal plasticity to neurodegeneration. Neural Plast. 2016:6391686. doi: 10.1155/2016/6391686
Wacker, J., Heldmann, M., and Stemmler, G. (2003). Separating emotion and motivational direction in fear and anger: effects on frontal asymmetry. Emotion 3, 167–193. doi: 10.1037/1528-3542.3.2.167
Wagner, D. D., Kelley, W. M., and Heatherton, T. F. (2011). Individual differences in the spontaneous recruitment of brain regions supporting mental state understanding when viewing natural social scenes. Cereb. Cortex 21, 2788–2796. doi: 10.1093/cercor/bhr074
Wager, T. D., and Smith, E. E. (2003). Neuroimaging studies of working memory: a meta-analysis. Cogn. Affect. Behav. Neurosci. 3, 255–274. doi: 10.3758/cabn.3.4.255
Walters, G. D. (2021). School-age bullying victimization and perpetration: a meta-analysis of prospective studies and research. Trauma Violence Abuse 22, 1129–1139. doi: 10.1177/1524838020906513
Weems, C. F., Saltzman, K. M., Reiss, A. L., and Carrion, V. G. (2003). A prospective test of the association between hyperarousal and emotional numbing in youth with a history of traumatic stress. J. Clin. Child Adolesc. Psychol. 32, 166–171. doi: 10.1207/S15374424JCCP3201_15
Wegbreit, E., Cushman, G. K., Puzia, M. E., Weissman, A. B., Kim, K. L., Laird, A. R., et al. (2014). Developmental meta-analyses of the functional neural correlates of bipolar disorder. JAMA Psychiatry 71, 926–935. doi: 10.1001/jamapsychiatry.2014.660
Whitlock, J. R., Heynen, A. J., Shuler, M. G., and Bear, M. F. (2006). Learning induces long-term potentiation in the hippocampus. Science 313, 1093–1097. doi: 10.1126/science.1128134
Wiener, J., and Mak, M. (2009). Peer victimization in children with attention-deficit/hyperactivity disorder. Psychol. Schools 46, 116–131. doi: 10.1002/pits.20358
Will, G. J., van Lier, P. A., Crone, E. A., and Güroğlu, B. (2016). Chronic childhood peer rejection is associated with heightened neural responses to social exclusion during adolescence. J. Abnorm. Child Psychol. 44, 43–55. doi: 10.1007/s10802-015-9983-0
Williams, C. A., Doorley, J. D., and Esposito-Smythers, C. (2017). Interpersonal rejection sensitivity mediates the associations between peer victimization and two high-risk outcomes. Clin. Child Psychol. Psychiatry 22, 649–663. doi: 10.1177/1359104517712041
Winecoff, A., Clithero, J. A., Carter, R. M., Bergman, S. R., Wang, L., and Huettel, S. A. (2013). Ventromedial prefrontal cortex encodes emotional value. J. Neurosci. 33, 11032–11039. doi: 10.1523/JNEUROSCI.4317-12.2013
Woody, A., Hooker, E. D., Zoccola, P. M., and Dickerson, S. S. (2018). Social-evaluative threat, cognitive load and the cortisol and cardiovascular stress response. Psychoneuroendocrinology 97, 149–155. doi: 10.1016/j.psyneuen.2018.07.009
Wu, L., Zhang, D., Su, Z., and Hu, T. (2015). Peer victimization among children and adolescents: a meta-analytic review of links to emotional maladjustment. Clin. Pediatr. (Phila) 54, 941–955. doi: 10.1177/0009922814567873
Yang, M., Tsai, S. J., and Li, C. R. (2020). Concurrent amygdalar and ventromedial prefrontal cortical responses during emotion processing: a meta-analysis of the effects of valence of emotion and passive exposure versus active regulation. Brain Struct. Funct. 225, 345–363. doi: 10.1007/s00429-019-02007-3
Yehuda, R. (2009). Status of glucocorticoid alterations in post-traumatic stress disorder. Ann. N Y Acad. Sci. 1179, 56–69. doi: 10.1111/j.1749-6632.2009.04979.x
Yehuda, R., Keefe, R. S., Harvey, P. D., Levengood, R. A., Gerber, D. K., Geni, J., et al. (1995). Learning and memory in combat veterans with posttraumatic stress disorder. Am. J. Psychiatry 152, 137–139. doi: 10.1176/ajp.152.1.137
Yehuda, R., Pratchett, L. C., Elmes, M. W., Lehrner, A., Daskalakis, N. P., Koch, E., et al. (2014). Glucocorticoid-related predictors and correlates of post-traumatic stress disorder treatment response in combat veterans. Interface Focus 4:20140048. doi: 10.1098/rsfs.2014.0048
Yurgelun-Todd, D. (2007). Emotional and cognitive changes during adolescence. Curr. Opin. Neurobiol. 17, 251–257. doi: 10.1016/j.conb.2007.03.009
Zerbes, G., Kausche, F. M., and Schwabe, L. (2020). Stress-induced cortisol modulates the control of memory retrieval towards the dorsal striatum. Eur. J. Neurosci. . [Online ahead of print]. doi: 10.1111/ejn.14942
Zhu, C., Guo, X., Jin, Z., Sun, J., Qiu, Y., Zhu, Y., et al. (2011). Influences of brain development and ageing on cortical interactive networks. Clin. Neurophysiol. 122, 278–283. doi: 10.1016/j.clinph.2010.06.016
Keywords: bullying victimization, cerebral functional activity, executive functions, memory, psychopathology, stress, theory of mind
Citation: Palamarchuk IS and Vaillancourt T (2022) Integrative Brain Dynamics in Childhood Bullying Victimization: Cognitive and Emotional Convergence Associated With Stress Psychopathology. Front. Integr. Neurosci. 16:782154. doi: 10.3389/fnint.2022.782154
Received: 23 September 2021; Accepted: 15 March 2022;
Published: 27 April 2022.
Edited by:
Elizabeth B. Torres, Rutgers, The State University of New Jersey, United StatesReviewed by:
Angela Maia, University of Minho, PortugalMatteo Angelo Fabris, University of Turin, Italy
Copyright © 2022 Palamarchuk and Vaillancourt. This is an open-access article distributed under the terms of the Creative Commons Attribution License (CC BY). The use, distribution or reproduction in other forums is permitted, provided the original author(s) and the copyright owner(s) are credited and that the original publication in this journal is cited, in accordance with accepted academic practice. No use, distribution or reproduction is permitted which does not comply with these terms.
*Correspondence: Tracy Vaillancourt, tracy.vaillancourt@uottawa.ca
† ORCID: Iryna S. Palamarchuk, orcid.org/0000-0002-9121-405X; Tracy Vaillancourt, orcid.org/0000-0002-9058-7276