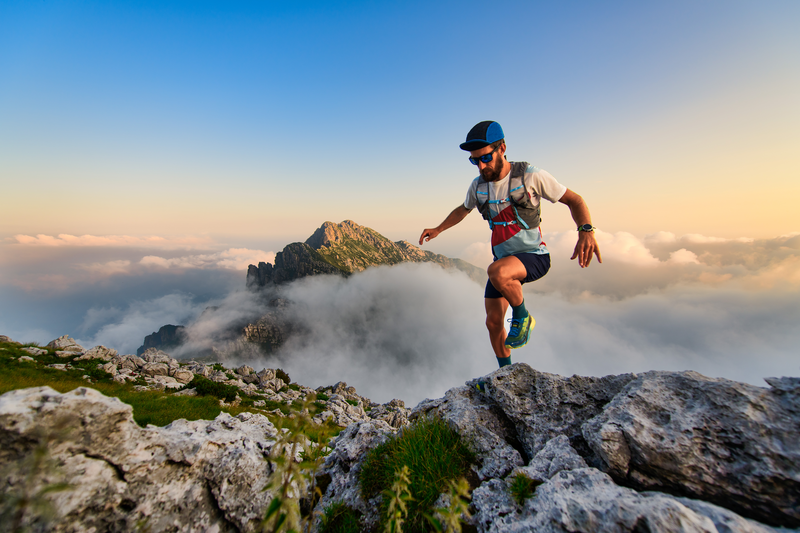
94% of researchers rate our articles as excellent or good
Learn more about the work of our research integrity team to safeguard the quality of each article we publish.
Find out more
SYSTEMATIC REVIEW article
Front. Neurosci. , 05 February 2025
Sec. Brain Imaging Methods
Volume 19 - 2025 | https://doi.org/10.3389/fnins.2025.1535288
This article is part of the Research Topic Brain Metabolic Imaging by Magnetic Resonance Imaging and Spectroscopy: Methods and Clinical Applications Volume II View all 4 articles
Objectives: Neuroimaging investigations into chronic low back pain (CLBP) have detected functional abnormalities across a spectrum of brain regions, yet the findings have often been inconsistent. In this meta-analysis, we integrated the existing data, delineating a pattern of coherent results from the encompassed studies.
Methods: A systematic search of neuroimaging studies investigating the brain activity differences between CLBP and Healthy controls (HCs) was conducted in seven databases up to December 22, 2024. An anisotropic effect-size signed differential mapping (AES-SDM)-based meta-analysis was carried out to report the results and perform a multimodal analysis.
Results: A total of 20 publications reporting on 24 experiments in this meta-analysis. The ReHo meta-analysis showed abnormal spontaneous activity of left inferior temporal gyrus (ITG), left superior frontal gyrus (SFG), right middle frontal gyrus (MFG), right precuneus, right fusiform gyrus and bilateral postcentral gyrus (PoCG) in CLBP patients. The ALFF meta-analysis demonstrated functional alterations in the right rolandic operculum (extending to the right insula and right IFG), left ITG, left middle occipital gyrus (MOG), left paracentral lobule, left PoCG and bilateral cuneus cortex in CLBP patients. The results of the functional group meta-analysis revealed that patients with CLBP displayed new decreased functional activity in the right thalamus, right precentral gyrus (PreCG) and right lingual gyrus.
Conclusion: Patients with CLBP exhibit extensive multimodal functional neuroimaging abnormalities, involving brain regions related to pain perception, emotional processing, cognitive functions, and both the visual and motor cortices. These meta-analysis findings might provide a valuable reference for the identification of potential therapeutic targets for CLBP in the brain.
Chronic low back pain (CLBP) is a pervasive musculoskeletal affliction with profound societal repercussions, ranking among the most prevalent chronic pain conditions (George et al., 2021; JOSPT, 2021). It refers to bone and muscle pain that extends from the 12th rib to the buttock fold for more than 3 months, which sometimes extends to the thighs (above the knee) (Hartvigsen et al., 2018; Shi et al., 2021). Anatomically, CLBP can arise from any innervated structures at the lumbar spine. Pain is the predominant motivator for individuals with CLBP to seek medical intervention (Mosley et al., 2017). CLBP is often closely intertwined with local pain and functional impairments, as well as with emotional and cognitive behavioral disorders, including anxiety, depression, and sleep disturbances. Globally, CLBP stands as a principal contributor to disability, with its incidence rates exhibiting an unrelenting upward trend (Global Burden of Disease Study 2013 Collaborators, 2015; Meucci et al., 2015). It also imposes a significant economic burden and leads to a substantial deterioration in the patients’ quality of life (GBD 2019 Diseases and Injuries Collaborators, 2020; Lin et al., 2022).
Recent research has yet to clarify the underlying mechanisms of CLBP, significantly impeding our comprehension of its neuropathological aspects and the development of effective therapeutic strategies. Many previous systematic reviews and clinical studies have indicated a weak or inconsistent correlation between CLBP and degenerative spinal changes, suggesting that the condition’s etiology may be more complex than initially presumed (Chou and Shekelle, 2010; Vanderstraeten et al., 2023), while increasing evidence shows that CLBP is significantly related to changes in brain function and structure (Zhang et al., 2019). Previous fMRI studies have found that patients with CLBP exhibited abnormal gray matter volume in the prefrontal cortex, the precentral gyrus and other extensive areas (Yang et al., 2017). Kregel et al. (2015) have summarized changes in the white matter of the brain specific cortical and subcortical areas in CLBP patients. Moreover, previous studies have confirmed that there are many spontaneous brain activity changes in patients with CLBP compared to Healthy Controls (HCs), such as medial prefrontal cortex (Zhou et al., 2018, 2019; Zhang et al., 2017; Sun, 2018), precuneus (Zhou et al., 2019; Chen et al., 2011; Zou et al., 2019; Hu, 2020; Zhu et al., 2020; Zhang, 2021), cerebellum posterior lobe (Zhang et al., 2017; Sun, 2018; Hu, 2020) and parahippocampal gyrus (Sun, 2018; Zhu et al., 2020). Increased ALFF and decreased ReHo in the caudate nucleus were also detected in different researches (Gao et al., 2020; Zhao, 2021). However, some studies have reported no changes in brain regions with CLBP (Li et al., 2019), and conflicting findings have also been reported in some studies (Zhou et al., 2019; Zhang et al., 2017; Hu, 2020; Gao et al., 2020; Zhao, 2016).
Resting state functional magnetic resonance imaging (rs-fMRI) is a powerful, noninvasive technique for exploring the neurological mechanisms, and has been used in many diseases, including chronic pain disorders. It can measure to neural activity by measuring changes in oxygenation concentration (BOLD signals). Common local metrics of BOLD signals include regional homogeneity (ReHo), amplitude of low-frequency fluctuation (ALFF), and its standardized variant fractional ALFF (fALFF), mean normalized low-frequency amplitude (mALFF), and functional connectivity (FC). ReHo describes the local similarity or synchronization of adjacent voxel time series, focusing on the coherence and centrality of regional activities (Ou et al., 2018), while ALFF/fALFF/mALFF depict the consistent amplitude of each signal’s time history, focusing on measuring the intensity of brain activities (Chang et al., 2021). Although FC is also a frequently utilized analytical approach, it centers on the interactions between different brain regions and typically involves the prior selection of regions of interest to investigate the connectivity patterns within brain networks. The other four methods, primarily focus on the consistency and intensity of activities within brain regions themselves. Numerous previous studies have demonstrated that ReHo and ALFF are the most commonly employed rs-fMRI data analysis techniques for capturing spontaneous neural activity within specific brain regions. Combining these two methods in the neuroimaging analytical processes can provide more comprehensive information and better explanatory power.
Anisotropic effect-size signed differential mapping (AES-SDM) is a coordinate-based meta-analysis approach used to summarize and integrate neuroimaging research results that display statistically significant differences in functional activity between patients and control groups in peak-clusters (Radua et al., 2012, 2014). Despite potential influences from raw data and publication bias, it leverages and enhances many of the positive attributes identified in other analytical approaches. It not only allows for the reconstruction of effect size and statistical parameter maps that show the functional activity increases and decreases in different brain regions among multiple original studies, but it can also perform meta-regression, sensitivity and heterogeneity analysis, and bias tests by convolving isotropic kernels with peaks. So far, AES-SDM has been widely applied in neuroimaging meta-analyses in various diseases, such as migraine, post-traumatic stress disorder, anxiety disorders and so on (Li et al., 2022; Zhang et al., 2023a; Schrammen et al., 2022), due to its high sensitivity, low false positive rates, and consistency.
Hence, this study aims to perform a meta-analysis of previously published CLBP fMRI studies by using AES-SDM method, which could ultimately obtain consistent and reliable functional brain activity alterations. At the same time, to explore the potential correlation of clinical variables such as age, disease duration, and the pain intensity with these abnormal functional activities in brain regions of CLBP.
This meta-analysis has been registered in the International Prospective Register of Systematic Reviews (PROSPERO) (registration number: CRD42023428454).
A systematic search was conducted in seven electronic databases from the inception of the databases to December 22, 2024, including PubMed, EMBASE, Cochrane Central Register of Controlled Trials (CENTRAL), Web of Science, China National Knowledge Infrastructure (CNKI), Chongqing VIP (VIP), and Wanfang Database (WF). All the publications within this date were searched without any restrictions of country or article type. Briefly, the general search terms included the following words: (“low back pain” OR “dorsalgia” OR “back disorder*” OR “coccydynia” OR “sciatica” OR “back muscles”) AND (“magnetic resonance imaging” OR “MRI” OR “functional MRI” OR “fMRI” OR “voxel wise” OR “voxel” OR “regional homogeneity” OR “ReHo” OR “amplitude of low frequency fluctuations” OR “ALFF” OR “fractional amplitude of low frequency fluctuation” OR “fALFF” or “mean normalized low-frequency amplitude” OR “mALFF”) AND (“resting state”). The search strategy was transformed to adapt to Chinese electronic databases. Additionally, to identify any potential studies that were not found in our searches, the reference lists of relevant articles and reviews were consulted as additional searches.
This meta-analysis adheres to the Preferred Reporting Items for Systematic Reviews and Meta-Analyses (PRISMA) 2020 guidelines and checklist (Page et al., 2021). The inclusion criteria were as follows: (1) original research published in English or Chinese journals and peer-reviewed; (2) the differences of brain functional activity were compared between adult patients with CLBP and HCs; (3) the analysis methods used in researches was ReHo or ALFF, fALFF, mALFF or included all of them; (4) the standardized three-dimensional coordinates (x, y, z) of results were reported in Talairach space or Montreal Neurological Institute (MNI), or reported null findings; (5) magnetic strength of the MRI scanner was at least 1.5 Tesla.
The exclusion criteria were as follows: (1) researches only reported region of interest (ROI) results; (2) article types were animal studies, case reports or conference proceedings; (3) the number of participants was less than 10 in either the patient group or control group. The detailed screening process is presented in Figure 1. Article screening was performed independently by two authors (XC, PL) and supervised by another reviewer (SC).
Figure 1. The flow diagram of the studies according to the PRISMA 2020 guidelines. n, number; PRISMA, preferred reporting items for systematic reviews and meta-analyses.
If two or more studies used the same data source, only the articles with the largest sample size and more comprehensive information would be included. Besides, for longitudinal or intervention studies, we only included the baseline comparison between patients and HCs.
The information of included articles was independently extracted by two authors (XC and NC). Information of each included study was extracted and presented in Table 1, including: first author, year of publication, demographics (sample size and mean age), illness duration, visual analog scale (VAS) scores, and MRI technical details (MRI scanner, FWHM, analytic methods, correction, threshold, etc.). Statistically significant coordinates in each study were independently extracted according to the requirements of AES-SDM. If coordinates were missing or statistical values were not reported, the corresponding authors of the studies would be contacted by email. If peaks or statistics were not provided from studies reporting null findings, we recorded as none. Any disagreement was discussed by the authors’ group and the result was agreed upon.
The quality of the included studies was assessed based on a 10-point checklist which was used in many previous neuroimaging meta-analyses (Supplementary Tables S1A, S1B) (Guo et al., 2024; Yan et al., 2023). The checklist focused on sample size, demographic and clinical characteristics of the study populations, imaging specific methodology and the quality of the reported results. According to the completeness of published studies, this checklist was scored as full, partial or unmet, with each item receiving a score of 1, 0.5 or 0, respectively. The total score was 10 points. The assessment of each study was carried out independently by two researchers (XC and NC) in a standardized manner. For any differences, XC and NC gave their input and decided with consensus from the third researcher (ZL).
AES-SDM software1 was used to analyze regional changes in spontaneous brain activity between patients with CLBP and HCs (Radua et al., 2012). Z-values appeared in studies were converted into T-statistics using AES-SDM official online converter.2 Above all, the effect-size and variance maps were recreated by combining the extracted peak coordinate information with a Gaussian sliding kernel (full-width at half-maximum = 20 mm). Next, the mean random effects were generated by calculating the study maps voxel-wise based on intra-study variability, sample size, and inter-study heterogeneity (Cheng et al., 2020). The statistical significance and default threshold parameter were set as follows: voxel threshold: p ≤ 0.005, peak height threshold: peak Z ≥ 1.000, cluster extent threshold ≥10 voxels (Radua et al., 2012). Finally, the meta-analysis effect-size map was analyzed by comparing it with a null distribution created by a permutation algorithm.
We initially conducted two separate meta-analyses for the ALFF and ReHo studies using the AES-SDM software. Subsequently, we integrated the functional group data (ALFF + ReHo) to perform another meta-analysis. For all three meta-analyses, we employed a meta-regression analysis weighted by sample size and within-study and between-study variances to assess the correlation between brain changes and clinical variables (age, disease duration, VAS scores). A leave-one-out jackknife sensitivity analysis was used to test the repeatability and robustness of these three results. The approach involves conducting multiple iterations of the same meta-analysis, each time excluding one different dataset. Ultimately, we examine the frequency with which a particular result is consistently retained across these repeated iterations. If a brain region remains significant in all or most of (≥ 50%) the combinations of studies, it can be regarded as highly replicable (Radua et al., 2012; Long et al., 2022). The same statistical significance and default threshold parameter was set as in the main analysis. Egger tests were used to detect the asymmetry of funnel plots for publication bias, where p < 0.05 suggested significant publication bias. Concurrently, akin to the simple overlay of meta-analytical maps in individual meta-analyses, we utilized the multimodal meta-analysis overlap feature within the software to examine the co-localization of functional abnormalities from the first two meta-analyses, taking into account the error in p-values, in order to assess the convergence of results across different modalities (Radua et al., 2012, 2013). This multimodal meta-analysis provides a more statistically robust overlap.
As shown in Figure 1, a total of 1,713 articles were searched. Twenty-three potential eligible articles were screened in this study (Zhang et al., 2017, 2019; Zhou et al., 2018, 2019, 2024a,b; Sun, 2018; Chen et al., 2011; Zou et al., 2019; Hu, 2020; Zhu et al., 2020; Zhang, 2021; Gao et al., 2020; Zhao, 2021; Li et al., 2019; Zhao, 2016; Tan et al., 2019; Wen et al., 2022; Xu et al., 2023; Mei et al., 2024; Zuo, 2024; Chen, 2024; Tang et al., 2023). Due to only one literature on fALFF and two literatures on mALFF being retrieved, we did not conduct separate fALFF/ mALFF meta-analysis of CLBP studies. In addition, 5 articles conducted both ReHo and ALFF analysis (Zhang, 2021; Li et al., 2019; Xu et al., 2023; Mei et al., 2024; Zuo, 2024), but in one of the studies, multi band was extracted for the ALFF analysis (Zhang, 2021). So, we considered these five articles as 9 separate studies for the meta-analysis. At last, 20 articles were included in this meta-analysis (24 studies, 12 ReHo studies, 12 ALFF studies), including 653 patients with CLBP and 653 HCs. Only one study reported no significant between-group differences in brain regions (Li et al., 2019). There was no significant statistical difference in age or gender ratio between patients and HCs. The demographic characteristics, clinical variables, and technical details of these studies were shown in Table 1.
As illustrated in Table 2 and Figure 2, six peak foci were revealed according to the ReHo meta-analysis. Patients with CLBP had increased functional activity in the left inferior temporal gyrus (ITG, z = 2.261, p < 0.0001), left superior frontal gyrus (SFG), medial orbital (z = 1.962, p = 0.0001), right middle frontal gyrus (MFG, z = 1.603, p = 0.0014), right precuneus (z = 1.557, p = 0.0019) and decreased functional activity in the right fusiform gyrus (z = −2.162, p = 0.0001) and bilateral postcentral gyrus (PoCG, z = −1.991, p = 0.0005) compared with HCs.
Table 2. Meta‐analysis results of ReHo abnormalities in resting state fMRI between CLBP patients and HCs.
Figure 2. Resting-state functional difference between CLBP and HCs. Areas with increased resting-state functional activity value are displayed in yellow (A). L SFG, medial orbital; L ITG; R MFG; R Rolandic operculum; R precuneus; L MOG, and areas with decreased resting-state functional activity value are displayed in blue (B). Bilateral PoCG; R fusiform gyrus; R thalamus; R PreCG; R lingual gyrus; Bilateral cuneus cortex; L inferior parietal (excluding supramarginal and angular) gyri; L median cingulate / paracingulate gyri. L = left; R = right; SFG = superior frontal gyrus; ITG = inferior temporal gyrus; MFG = middle frontal gyrus; MOG = middle occipital gyrus; PoCG = postcentral gyrus; PreCG = precentral gyrus.
Patients with CLBP had increased functional activity in the right rolandic operculum (extending to the right insula and right IFG) (z = 3.135, p < 0.0001), left ITG (z = 2.138, p = 0.0008), left middle occipital gyrus (MOG, z = 1.767, p = 0.0033), as well as decreased functional activity in the left paracentral lobule (z = −1.880, p = 0.0005), left postcentral gyrus (PoCG, z = −1.790, p = 0.0008) and bilateral cuneus cortex (z = −1.890, p = 0.0004) compared with HCs (Table 3; Figure 2).
Table 3. Meta‐analysis results of ALFF abnormalities in resting state fMRI between CLBP patients and HCs.
On the basis of the above meta-analyses, we utilized the multimodal functionality of the ASE-SDM software to overlay regions with altered ReHo and ALFF, and discovered increased functional activity in the left ITG of patients with CLBP.
The results of the functional group meta-analysis (ReHo + ALFF) (Table 4; Figure 2) revealed that compared with HCs, patients with CLBP displayed new decreased functional activity in the left inferior parietal (excluding supramarginal and angular) gyri (z = −2.250, p < 0.0001), left median cingulate / paracingulate gyri (z = −1.936, p = 0.0007), right thalamus (z = −1.833, p = 0.0012), right precentral gyrus (PreCG, z = −1.876, p = 0.0010) and right lingual gyrus (z = −1.664, p = 0.0031).
Table 4. Meta‐analysis results of functional abnormalities (ReHo + ALFF) in resting state fMRI between CLBP patients and HCs.
All meta-analyses revealed significant heterogeneity in the right ITG. Egger tests demonstrated that there was no publication bias (p > 0.05) in the meta-analysis of functional data (Supplementary Figures S1A, S1B).
After reading the SDM software instructions and reviewing some meta-analyses related to neuroimaging, it became evident that a consensus regarding the threshold for sensitivity analysis to assess the robustness of the primary outcome is lacking (Long et al., 2022; Wang et al., 2020). Finally, we opted to employ a threshold of 50% in our meta-analysis (Radua et al., 2012; Zhou et al., 2017). The jackknife analysis revealed that all of the aforementioned regions exhibited stability, being reliably detected across the three meta-analyses (Supplementary Tables S2–S4).
As depicted in Tables 5–7, in meta-regression analyses, we examined the potential correlations between clinical variables (age, disease duration, and VAS scores) and these abnormal brain regions. The regression analyses based on ReHo and ALFF revealed correlations between the age of CLBP patients and specific brain regions such as the right precuneus, left PoCG, and left MOG. Consistently across all three regression analyses, a correlation was observed between the ITG and patients’ VAS scores. Furthermore, in both the standalone ALFF regression analysis and the analysis of the integrated functional dataset, correlations were identified between the disease duration of patients and several brain regions, including the PoCG, MOG, ITG, MFG, and right lingual gyrus. Since the datasets were insufficient, we did not perform meta-regression analyses on other continuous variables, such as gender, BMI, medications, and so on.
Table 5. Meta-regression analysis of the correlation between functional alterations and clinical variables in CLBP patients of ReHo studies.
Table 6. Meta-regression analysis of the correlation between functional alterations and clinical variables in CLBP patients of ALFF studies.
Table 7. Meta-regression analysis of the correlation between functional alterations and clinical variables in CLBP patients of ReHo and ALFF studies.
This multimodal neuroimaging meta-analysis integrated information from the whole-brain ReHo and ALFF studies in CLBP by using AES-SDM software. ReHo meta-analysis showed abnormal spontaneous activity of the left ITG, left SFG, right MFG, right precuneus, right fusiform gyrus and bilateral PoCG in patients with CLBP. Meanwhile, ALFF meta-analysis demonstrated functional alterations in the right rolandic operculum, left ITG, left MOG, left paracentral lobule, left PreCG and bilateral cuneus cortex of patients with CLBP. What’s more, meta-regression analysis revealed that the age, VAS scores, duration of disease of CLBP patients were significantly associated with widespread functional abnormalities in some brain regions, such as the right precuneus, left PoCG and left ITG. The left ITG emerged as the overlapping brain region among the ReHo and ALFF studies according to the multimodal analysis. The integrated functional analysis showed the left inferior parietal (excluding supramarginal and angular) gyri, left median cingulate / paracingulate gyri, right thalamus, right PreCG and right lingual gyrus were newly identified brain regions with decreased functional activity. This study found that patients with CLBP have abnormal brain activity in pain-related pathways, which is also associated with a wide range of cognitive functions and closely related to emotional functions. These brain functional changes may be important causes of physical and mental dysfunction as well as the recurrence of clinical symptoms in patients with CLBP.
This study revealed that patients with CLBP exhibit decreased brain activity in the PoCG and thalamus when compared to HCs. Pain is perceived as an imbalance between the ascending pathway and the descending pathway (De Ridder et al., 2022). The somatosensory cortex (SCC), as expressional central of the lateral “painfulness” pathway, encodes the discriminatory/sensory components of the pain. The PoCG is part of the SCC, which primarily governs somatic perception and serves as the ultimate central hub for all sensory pathways in the parietal lobe. The thalamus is considered a crucial relay station closely associated with pain perception and the descending pain modulation pathways. The thalamus projects to the insula and periaqueductal gray matter (PAG), receiving pain signals and transmitting them to the cerebral cortex, and it releases various neurotransmitters in the descending modulation pathways that affect the transmission of pain signals in the spinal cord. Additionally, regression analysis has found a correlation between the PoCG and the duration of CLBP. When lower back pain becomes chronic, the somatic perception dominated by the SCC also undergoes changes, potentially becoming an adaptive process. A recent literature review conducted for a clinical trial targeting the affective pain circuit found that the thalamus is the preferred target for most chronic pain patients during deep brain stimulation (Keifer et al., 2014; Nüssel et al., 2022). Additionally, a study assessing the feasibility and safety of a novel neurofeedback technique based on electroencephalography (EEG ISF-NF) in retraining activity within the SCC and pregenual anterior cingulate cortex (pgACC), as well as dorsal anterior cingulate cortex (dACC), has been confirmed as a safe and effective method for treating CLBP (Adhia et al., 2023). These findings suggest that patients with CLBP exhibit sensory abnormalities in the “pain” pathways and may imply the formation of an adaptive mechanism.
Abnormalities in the prefrontal cortex (PFC), precuneus and rolandic operculum are other important findings in our results. The SFG and MFG, located in the PFC, belong to the descending pain modulatory system and are also the key node of the central executive network. The PFC not only participates in advanced cognitive and memory functions, but also responsible for emotional modulation and stress perception. The role of the PFC in pain regulation has been proved repeatedly in both clinical and pre-clinical work (Ong et al., 2019; Ma et al., 2023; Zhang et al., 2023b). This system has been clearly established to not only inhibit but also facilitate pain (Wilder-Smith, 2011). In the descending pain modulation pathway, the PFC sends descending fibers to the PAG. The functional activity variations of the PFC directly affect the PAG and play a crucial role in pain modulation. A certain review summarized the voxel-based morphometry results of 15 types of chronic pain and showed that changes in the cerebral cortex, particularly in the PFC, occur in chronic pain conditions (May, 2011). Notably, both repetitive transcranial magnetic stimulation (rTMS) and repetitive transcranial direct current stimulation (rTDS) therapies have identified the dorsolateral PFC as a therapeutic target for pain management in clinical practice (Lefaucheur et al., 2020; Li et al., 2024; Che et al., 2021). Furthermore, substantial evidence highlights the pivotal role of the PFC in the development and perpetuation of CLBP.
Precuneus is a part of both the default mode network (DMN) and a key regions of brain activity during resting states (Buckner and DiNicola, 2019; Zhang et al., 2016). Precuneus is involved in higher cognition. DMN is a collection of brain regions that are implicated in various ‘high-level’ cognitive processes. The chronic pain and suffering from CLBP lead to the formation of adaptations and are regulated through connections between the SCC and the DMN. A substantial body of evidence from previous articles indicates that the condition of CLBP leads to functional abnormalities in multiple brain regions within the DMN, further supporting the involvement of the DMN in the emotional processing and cognitive functions associated with chronic pain. Additionally, some studies have utilized the resting-state functional connectivity of the DMN as a predictive marker for assessing the response of CLBP patients to treatment regimens, thereby establishing quantifiable benchmarks for selecting appropriate therapeutic strategies (Tu et al., 2019). Regression analysis has identified a correlation between the precuneus and age, implying a potential decline in cognitive and memory functions as age advances. Studies have demonstrated that older adults with elevated superiority illusion scores tend to have greater gray matter volume in the right precuneus and enhanced FC compared to younger and middle-aged individuals (Shidei et al., 2025). The precuneus undoubtedly assumes a complex and pivotal role in the context of CLBP.
The operculum, defined as the multimodal cortex adjacent to the insula, has a widespread connectivity. Its complex functions include autonomic, cognitive processing, sensory and motor (Mălîia et al., 2018). These functions all participate in the medial pathway. The medial “suffering” pathway, consisting of the rostral to dorsal anterior cingulate cortex and anterior insular cortex, processes the affective motivational aspect of pain. The central pathway’s ability to handle pain in patients with CLBP changes and this “suffering” sensation is further modulation by the central executive network and salience network. This upward pathway overlaps with those two networks, ultimately leading to emotional disorders such as anxiety and depression. As a crucial hub for the integration of emotion and sensation, the insula participates in the transmission and modulation of nociceptive information through its extensive connections with other brain regions. Within the descending modulatory pathway, the insular cortex is connected to multiple brain areas, including the amygdala, anterior cingulate cortex, and PAG, which are key nodes in the transmission and modulation of pain signals. For instance, the connection between the insular cortex and the PAG is involved in the descending modulation of pain. Additionally, the insular cortex engages in the emotional and cognitive aspects of pain through its connection with the anterior cingulate cortex. Studies applying fMRI on patients in pain have found that the insular cortex is one of the most frequently activated brain regions (Apkarian et al., 2005). In short, the degree of pain and the regulation of negative emotions in patients with CLBP are closely related to functional changes in the insular cortex (Labrakakis, 2023).
Additionally, this meta-analysis also found that patients with CLBP have reduced functional activity in the PreCG, fusiform gyrus, precuneus, and lingual gyrus, while there is increased functional activity in the ITG and MOG. Our multimodal analysis also identified abnormalities in the ITG. The PreCG is more anatomically associated with movement and is part of the primary motor cortex (M1), serving as a higher center for human motor function. Still, some proprioceptive fibers also project to the PreCG which encodes the intensity, location, and duration of pain. A recent update on the guidelines for the therapeutic use of rTMS indicates that the application of high-frequency—rTMS to the M1 on the side of pain in patients has a definite analgesic effect (Lefaucheur et al., 2020; Olechowski et al., 2023). The fusiform gyrus is closely associated with facial, bodily, and high spatial frequency stimuli. The lingual gyrus and MOG are also located in the occipital visual cortex, and together with the ITG, they are responsible for visual memory and visual processing. These regions send sensory information through sensory afferents to pain regulation systems, such as the thalamus and amygdala. There is growing evidence that the visual cortex has been participated in the processing of pain (Bush et al., 2021; Wei et al., 2019). In all the results of the regression analysis, a significant correlation between the ITG and VAS scores was found, indicating that functional changes in the ITG play an important role in the perception of pain intensity in patients with CLBP.
This meta-analysis has certain limitations. Firstly, the sample size of the study is relatively small, which leads to insufficient available data. While Müller et al. (2018) have noted that the number of experiments required for a meta-analysis largely depends on the expected effect size, analyses based on small samples should still be approached with caution. Secondly, many differences existed between imaging processing techniques and statistical thresholds as well as the demographic characteristics of patients, publication bias, etc. Moreover, the AES-SDM analysis is based on aggregated data, specifically the coordinates from published studies, rather than original data. This approach may result in less accurate findings. All of these factors may lead to biased results. For those reason, jackknife sensitivity analysis was used to evaluate the reliability and robustness of the results. Despite conducting heterogeneity analysis and Egger’s test, with the p-value of Egger’s test indicating no significant risk of bias, the funnel plot is not symmetrical. When there are significant differences in methodology, patient population, intervention measures, and other aspects, the funnel plot may become asymmetric, even in the absence of publication bias. Greater caution should be exercised when interpreting the results. Thirdly, due to the limited availability of relevant treatment studies, we did not conduct a meta-analysis between abnormal brain regions and studies related to therapeutic effects. The research advancements in related fields are worth further exploring in the future. Finally, this study focused solely on ReHo, ALFF, fALFF and mALFF. More articles using other data analysis methods are not included. Also, the studies included in this meta-analysis were case–control studies rather than longitudinal studies, and they only provided functional data. Any existing structural differences might potentially result in functional brain differences. Our study cannot entirely elucidate that the symptoms of CLBP might either cause brain changes, or on the contrary start from pre-existing brain spontaneous abnormalities. Further integration and analysis of brain structural and functional data and longitudinal studies can be conducted in the future to get further exploration of neuropathological mechanisms of chronic pain, and clarify the causal relationships between brain function alterations and the occurrence of CLBP.
Patients with CLBP have wide multimodal functional neuroimaging abnormalities, involving brain regions related to pain perception, emotional processing, cognitive functions, as well as the visual and motor cortices. These meta-analysis findings might provide a reference for the identification of potential therapeutic targets for CLBP in the brain.
Publicly available datasets were analyzed in this study. This data can be found at: the datasets that support the findings of the current study are available from the corresponding authors upon reasonable request.
XC: Data curation, Methodology, Software, Writing – original draft. NC: Data curation, Formal analysis, Writing – original draft. PL: Investigation, Writing – original draft. YS: Investigation, Writing – original draft. JY: Resources, Supervision, Writing – review & editing. MX: Resources, Supervision, Writing – review & editing. DZ: Resources, Supervision, Writing – review & editing. FL: Resources, Supervision, Writing – review & editing. QS: Resources, Supervision, Writing – review & editing. SC: Conceptualization, Project administration, Validation, Visualization, Writing – review & editing. ZL: Funding acquisition, Supervision, Validation, Visualization, Writing – review & editing.
The author(s) declare that financial support was received for the research, authorship, and/or publication of this article. This study was supported by funds from the National Natural Science Foundation of China (No. 82474657, No. 81973958, No. 82205288), Distinguished Young Scholars Project of Science and Technology Department of Sichuan Province (No.2025NSFJQ0056), open Project of National Clinical Research Center for Chinese Medicine Acupuncture and Moxibustion (grant number NCRCOP20230011), China Postdoctoral Science Foundation (grant number 2021MD703796), Sichuan Science and Technology Program (grant number 2022NSFSC0856), and Medical Technology Project of Health Commission of Sichuan Province (grant number 21PJ110).
The authors thank all research assistants for their assistance to this article.
The authors declare that the research was conducted in the absence of any commercial or financial relationships that could be construed as a potential conflict of interest.
The authors declare that no Gen AI was used in the creation of this manuscript.
All claims expressed in this article are solely those of the authors and do not necessarily represent those of their affiliated organizations, or those of the publisher, the editors and the reviewers. Any product that may be evaluated in this article, or claim that may be made by its manufacturer, is not guaranteed or endorsed by the publisher.
The Supplementary material for this article can be found online at: https://www.frontiersin.org/articles/10.3389/fnins.2025.1535288/full#supplementary-material
Supplementary Figures S1A and S1B | Funnel plots and Egger tests of main functional studies. Abbreviations: SE, standard error; (A) Left inferior temporal gyrus: 0.38, t: 0.50, df: 22, p: 0.622; (B) Left superior frontal gyrus, medial orbital: -0.61, t: -1.04, df: 22, p: 0.310; (C) Right middle frontal gyrus: -1.59, t: -2.54, df: 22, p: 0.119; (D) Left inferior parietal (excluding supramarginal and angular) gyri: 1.32, t: 1.78, df: 22, p: 0.088; (E) Left median cingulate / paracingulate gyri: -0.18, t: -0.24, df: 22, p: 0.810; (F) Right thalamus: 0.56, t: 0.84, df: 22, p: 0.411; (G) Right postcentral gyrus: 0.50, t: 0.66, df: 22, p: 0.517; (H) Right precentral gyrus: 0.41, t: 0.54, df: 22, p: 0.597; (I) Right lingual gyrus: 0.23, t: 0.29, df: 22, p: 0.772; (J) Right cuneus cortex: 0.32, t: 0.58, df: 22, p: 0.571.
Adhia, D. B., Mani, R., Mathew, J., O’Leary, F., Smith, M., Vanneste, S., et al. (2023). Exploring electroencephalographic infraslow neurofeedback treatment for chronic low back pain: a double-blinded safety and feasibility randomized placebo-controlled trial. Sci. Rep. 13:1177. doi: 10.1038/s41598-023-28344-2
Apkarian, A. V., Bushnell, M. C., Treede, R. D., and Zubieta, J. K. (2005). Human brain mechanisms of pain perception and regulation in health and disease. Eur. J. Pain 9, 463–484. doi: 10.1016/j.ejpain.2004.11.001
Buckner, R. L., and DiNicola, L. M. (2019). The brain's default network: updated anatomy, physiology and evolving insights. Nat. Rev. Neurosci. 20, 593–608. doi: 10.1038/s41583-019-0212-7
Bush, N. J., Schneider, V., Sevel, L., Bishop, M. D., and Boissoneault, J. (2021). Associations of regional and network functional connectivity with exercise-induced low Back pain. J. Pain 22, 1606–1616. doi: 10.1016/j.jpain.2021.05.004
Chang, M., Womer, F. Y., Gong, X., Chen, X., Tang, L., Feng, R., et al. (2021). Identifying and validating subtypes within major psychiatric disorders based on frontal-posterior functional imbalance via deep learning. Mol. Psychiatry 26, 2991–3002. doi: 10.1038/s41380-020-00892-3
Che, X., Cash, R. F. H., Luo, X., Luo, H., Lu, X., Xu, F., et al. (2021). High-frequency rTMS over the dorsolateral prefrontal cortex on chronic and provoked pain: a systematic review and meta-analysis. Brain Stimul. 14, 1135–1146. doi: 10.1016/j.brs.2021.07.004
Chen, X. (2024) Study on pain mechanism of Tuina for lumbar disc herniation based on default mode network function remodeling.
Chen, J., Liu, B., Ye, Y., Li, X., Ge, M., Liu, X., et al. (2011). Resting state fMRI of brain in patients with chronic lumbodorsal pain. Chin. J. Med. Imag. Technol. 27, 1116–1120.
Cheng, S., Xu, G., Zhou, J., Qu, Y., Li, Z., He, Z., et al. (2020). A multimodal Meta-analysis of structural and functional changes in the brain of tinnitus. Front. Hum. Neurosci. 14:28. doi: 10.3389/fnhum.2020.00028
Chou, R., and Shekelle, P. (2010). Will this patient develop persistent disabling low back pain? JAMA 303, 1295–1302. doi: 10.1001/jama.2010.344
De Ridder, D., Vanneste, S., Smith, M., and Adhia, D. (2022). Pain and the triple network model. Front. Neurol. 13:757241. doi: 10.3389/fneur.2022.757241
Gao, Y., Zhao, L., Zhao, H., Zhu, Y., Li, J., Sang, D., et al. (2020). Alteration of low frequency fluctuation amplitude at brain-resting state in patients with chronic discogenic low back pain. Chin. J. Tissue Eng. Res. 25, 1160–1165.
GBD 2019 Diseases and Injuries Collaborators (2020). Global burden of 369 diseases and injuries in 204 countries and territories, 1990-2019: a systematic analysis for the global burden of disease study 2019. Lancet 396, 1204–1222. doi: 10.1016/S0140-6736(20)30925-9
George, S. Z., Fritz, J. M., Silfies, S. P., Schneider, M. J., Beneciuk, J. M., Lentz, T. A., et al. (2021). Interventions for the management of acute and chronic low back pain: revision 2021. J. Orthop. Sports Phys. Ther. 51:CPG1. doi: 10.2519/jospt.2021.0304
Global Burden of Disease Study 2013 Collaborators (2015). Global, regional, and national incidence, prevalence, and years lived with disability for 301 acute and chronic diseases and injuries in 188 countries, 1990-2013: a systematic analysis for the global burden of disease study 2013. Lancet 386, 743–800. doi: 10.1016/S0140-6736(15)60692-4
Guo, Z., Tang, X., Xiao, S., Yan, H., Sun, S., Yang, Z., et al. (2024). Systematic review and meta-analysis: multimodal functional and anatomical neural alterations in autism spectrum disorder. Mol. Autism. 15:16. doi: 10.1186/s13229-024-00593-6
Hartvigsen, J., Hancock, M. J., Kongsted, A., Louw, Q., Ferreira, M. L., Genevay, S., et al. (2018). What low back pain is and why we need to pay attention. Lancet 391, 2356–2367. doi: 10.1016/S0140-6736(18)30480-X
Hu, L. (2020). Regional homogeneity alterations in subjects with discogenic low back pain: a resting-state fMRI study. Integr. Tradit. Chin. West. Med. Imaging China 18, 22–25+29. doi: 10.3969/j.issn.1672-0512.2020.01.006
JOSPT (2021). Clinical guidelines to address low back pain: using the evidence to guide physical therapist practice. J. Orthop. Sports Phys. Ther. 51, 533–534. doi: 10.2519/jospt.2021.0507
Keifer, O. P. Jr., Riley, J. P., and Boulis, N. M. (2014). Deep brain stimulation for chronic pain: intracranial targets, clinical outcomes, and trial design considerations. Neurosurg. Clin. N. Am. 25, 671–692. doi: 10.1016/j.nec.2014.07.009
Kregel, J., Meeus, M., Malfliet, A., Dolphens, M., Danneels, L., Nijs, J., et al. (2015). Structural and functional brain abnormalities in chronic low back pain: a systematic review. Semin. Arthritis Rheum. 45, 229–237. doi: 10.1016/j.semarthrit.2015.05.002
Labrakakis, C. (2023). The role of the insular cortex in pain. Int. J. Mol. Sci. 24. doi: 10.3390/ijms24065736
Lefaucheur, J. P., Aleman, A., Baeken, C., Benninger, D. H., Brunelin, J., di Lazzaro, V., et al. (2020). Evidence-based guidelines on the therapeutic use of repetitive transcranial magnetic stimulation (rTMS): an update (2014-2018). Clin. Neurophysiol. 131, 474–528. doi: 10.1016/j.clinph.2019.11.002
Li, L., Qin, Y., Tian, T., Fang, J., and Zhu, W. (2019). Resting state fMRI of brain in patients with chronic low back pain. J. Clin. Radiol. 38, 768–772.
Li, L., Zhang, Y., Zhao, Y., Li, Z., Kemp, G. J., Wu, M., et al. (2022). Cortical thickness abnormalities in patients with post-traumatic stress disorder: a vertex-based meta-analysis. Neurosci. Biobehav. Rev. 134:104519. doi: 10.1016/j.neubiorev.2021.104519
Lin, H., Wang, X., Liu, L., Fei, Y., Zhu, K., Fei, J., et al. (2022). Questionnaire of clinical questions for clinical practice guideline of acupuncture-moxibustion: non-specific low back pain. World J. Acupunct Moxibustion 32, 109–114. doi: 10.1016/j.wjam.2022.03.004
Li, X., Liu, Z., Hu, Y., et al. (2024). Analgesic effects of high-frequency rTMS on pain anticipation and perception. Commun Biol, 7:1573. doi: 10.1038/s42003-024-07129-x
Long, J., Qin, K., Wu, Y., Li, L., and Zhou, J. (2022). Gray matter abnormalities and associated familial risk endophenotype in individuals with first-episode bipolar disorder: evidence from whole-brain voxel-wise meta-analysis. Asian J. Psychiatr. 74:103179. doi: 10.1016/j.ajp.2022.103179
Ma, X., Chen, W., Fu, Y., Li, H., and Liu, C. (2023). Acupuncture for neuropathic pain: focusing on the sympathetic nerve system. Acupunct. Herbal Med. 3, 139–148. doi: 10.1097/HM9.0000000000000069
Mălîia, M. D., Donos, C., Barborica, A., Popa, I., Ciurea, J., Cinatti, S., et al. (2018). Functional mapping and effective connectivity of the human operculum. Cortex 109, 303–321. doi: 10.1016/j.cortex.2018.08.024
May, A. (2011). Structural brain imaging: a window into chronic pain. Neuroscientist 17, 209–220. doi: 10.1177/1073858410396220
Mei, Y. D., Gao, H., Chen, W. F., Zhu, W., Gu, C., Zhang, J. P., et al. (2024). Research on the multidimensional brain remodeling mechanisms at the level of brain regions, circuits, and networks in patients with chronic lower back pain caused by lumbar disk herniation. Front. Neurosci. 18:1357269. doi: 10.3389/fnins.2024.1357269
Meucci, R. D., Fassa, A. G., and Faria, N. M. (2015). Prevalence of chronic low back pain: systematic review. Rev. Saude Publica 49:1. doi: 10.1590/S0034-8910.2015049005874
Mosley, G. E., Evashwick-Rogler, T. W., Lai, A., and Iatridis, J. C. (2017). Looking beyond the intervertebral disc: the need for behavioral assays in models of discogenic pain. Ann. N. Y. Acad. Sci. 1409, 51–66. doi: 10.1111/nyas.13429
Müller, V. I., Cieslik, E. C., Laird, A. R., Fox, P. T., Radua, J., Mataix-Cols, D., et al. (2018). Ten simple rules for neuroimaging meta-analysis. Neurosci. Biobehav. Rev. 84, 151–161. doi: 10.1016/j.neubiorev.2017.11.012
Nüssel, M., Zhao, Y., Knorr, C., Regensburger, M., Stadlbauer, A., Buchfelder, M., et al. (2022). Deep brain stimulation, stereotactic radiosurgery and high-intensity focused ultrasound targeting the limbic pain matrix: a comprehensive review. Pain Ther. 11, 459–476. doi: 10.1007/s40122-022-00381-1
Olechowski, C., Gener, M., Aiyer, R., and Mischel, N. (2023). Transcranial magnetic stimulation for the treatment of chronic low back pain: a narrative review. Front. Pain Res. (Lausanne) 4:1092158. doi: 10.3389/fpain.2023.1092158
Ong, W. Y., Stohler, C. S., and Herr, D. R. (2019). Role of the prefrontal cortex in pain processing. Mol. Neurobiol. 56, 1137–1166. doi: 10.1007/s12035-018-1130-9
Ou, Y., Liu, F., Chen, J., Pan, P., Wu, R., Su, Q., et al. (2018). Increased coherence-based regional homogeneity in resting-state patients with first-episode, drug-naive somatization disorder. J. Affect. Disord. 235, 150–154. doi: 10.1016/j.jad.2018.04.036
Page, M. J., McKenzie, J. E., Bossuyt, P. M., Boutron, I., Hoffmann, T. C., Mulrow, C. D., et al. (2021). The PRISMA 2020 statement: an updated guideline for reporting systematic reviews. BMJ 372:n71. doi: 10.1136/bmj.n71
Radua, J., Mataix-Cols, D., Phillips, M. L., el-Hage, W., Kronhaus, D. M., Cardoner, N., et al. (2012). A new meta-analytic method for neuroimaging studies that combines reported peak coordinates and statistical parametric maps. Eur. Psychiatry 27, 605–611. doi: 10.1016/j.eurpsy.2011.04.001
Radua, J., Romeo, M., Mataix-Cols, D., and Fusar-Poli, P. (2013). A general approach for combining voxel-based meta-analyses conducted in different neuroimaging modalities. Curr. Med. Chem. 20, 462–466. doi: 10.2174/0929867311320030017
Radua, J., Rubia, K., Canales-Rodríguez, E. J., Pomarol-Clotet, K., Fusar-Poli, P., Mataix-Cols, D., et al. (2014). Anisotropic kernels for coordinate-based meta-analyses of neuroimaging studies. Front. Psych. 5:13. doi: 10.3389/fpsyt.2014.00013
Schrammen, E., Roesmann, K., Rosenbaum, D., Redlich, R., Harenbrock, J., Dannlowski, U., et al. (2022). Functional neural changes associated with psychotherapy in anxiety disorders – a meta-analysis of longitudinal fMRI studies. Neurosci. Biobehav. Rev. 142:104895. doi: 10.1016/j.neubiorev.2022.104895
Shi, Y., Guo, W., and Yu, L. (2021). Non-specific low back pain: interpretation of North American Spine Society (NASS) guidelines for evidence-based medicine. Zhongguo Xiu Fu Chong Jian Wai Ke Za Zhi 35, 1336–1340. doi: 10.7507/1002-1892.202103131
Shidei, D. M., Matsuyoshi, A. I., Isato, A., Sugihara, G., Takahashi, H., and Yamada, M. (2025). Ayako Isato, Superiority illusion in older adults: volume and functional connectivity of the Precuneus. Neuroscience. 4:e70046. doi: 10.1002/pcn5.70046
Sun, Y. (2018). The value of resting state functional magnetic resonance for emotion disorders in patients with chronic discogenic low Back pain, North Sichuan Medical College.
Tan, W., Wang, W., Jiang, H., Zeng, K., Yang, Y., Chen, Y., et al. (2019). Brain immediate analgesia mechanism of Tuina in low Back pain. Chin. J. Tradit. Med. Traumatol. Orthop. 27, 11–16.
Tang, C., Guo, G., Fang, S., Yao, C., Zhu, B., Kong, L., et al. (2023). Abnormal brain activity in lumbar disc herniation patients with chronic pain is associated with their clinical symptoms. Front. Neurosci. 17:1206604. doi: 10.3389/fnins.2023.1206604
Tu, Y., Ortiz, A., Gollub, R. L., Cao, J., Gerber, J., Lang, C., et al. (2019). Multivariate resting-state functional connectivity predicts responses to real and sham acupuncture treatment in chronic low back pain. Neuroimage Clin. 23:101885. doi: 10.1016/j.nicl.2019.101885
Vanderstraeten, R., Fourré, A., Demeure, I., Demoulin, C., Michielsen, J., Anthierens, S., et al. (2023). How do physiotherapists explain influencing factors to chronic low Back pain? A qualitative study using a fictive case of chronic non-specific low back pain. Int. J. Environ. Res. Public Health 20:5828. doi: 10.3390/ijerph20105828
Wang, Y., Gao, Y., Tang, S., Lu, L., Zhang, L., Bu, X., et al. (2020). Large-scale network dysfunction in the acute state compared to the remitted state of bipolar disorder: a meta-analysis of resting-state functional connectivity. EBioMedicine 54:102742. doi: 10.1016/j.ebiom.2020.102742
Wei, H. L., Zhou, X., Chen, Y. C., Yu, Y. S., Guo, X., Zhou, G. P., et al. (2019). Impaired intrinsic functional connectivity between the thalamus and visual cortex in migraine without aura. J. Headache Pain 20:116. doi: 10.1186/s10194-019-1065-1
Wen, Y., Chen, X. M., Jin, X., Ling, D. Y., Chen, S., Huang, Q., et al. (2022). A spinal manipulative therapy altered brain activity in patients with lumbar disc herniation: a resting-state functional magnetic resonance imaging study. Front. Neurosci. 16:974792. doi: 10.3389/fnins.2022.974792
Wilder-Smith, C. H. (2011). The balancing act: endogenous modulation of pain in functional gastrointestinal disorders. Gut 60, 1589–1599. doi: 10.1136/gutjnl-2011-300253
Xu, T., Wang, E., Tu, J., et al. (2023). Resting state fMRI of brain in patients with chronic nonspecific low back pain. Chin. Comput. Med. Imaging 29, 478–482. doi: 10.3969/j.issn.1006-5741.2023.05.004
Yan, H., Xiao, S., Fu, S., Gong, J., Qi, Z., Chen, G., et al. (2023). Functional and structural brain abnormalities in substance use disorder: a multimodal meta-analysis of neuroimaging studies. Acta Psychiatr. Scand. 147, 345–359. doi: 10.1111/acps.13539
Yang, Q., Wang, Z., Yang, L., Xu, Y., and Chen, L. M. (2017). Cortical thickness and functional connectivity abnormality in chronic headache and low back pain patients. Hum. Brain Mapp. 38, 1815–1832. doi: 10.1002/hbm.23484
Zhang, Y. (2021) Brain functional plasticity in patients with low back-related leg pain due to lumbar disc herniation: A multiscale frequency-based resting state fMRI study, Nanchang University.
Zhang, C., Hu, H., Wang, X., Li, B., Yang, H., Xu, X., et al. (2017). Study on functional magnetic resonance imaging at resting state based on ALFF for lumbar disc herniation. J. Med. Imaging 27, 2371–2376.
Zhang, B., Jung, M., Tu, Y., Gollub, R., Lang, C., Ortiz, A., et al. (2019). Identifying brain regions associated with the neuropathology of chronic low back pain: a resting-state amplitude of low-frequency fluctuation study. Br. J. Anaesth. 123, e303–e311. doi: 10.1016/j.bja.2019.02.021
Zhang, J., Su, J., Wang, M., Zhao, Y., Yao, Q., Zhang, Q., et al. (2016). Increased default mode network connectivity and increased regional homogeneity in migraineurs without aura. J. Headache Pain 17:98. doi: 10.1186/s10194-016-0692-z
Zhang, X., Zhou, J., Guo, M., Cheng, S., Chen, Y., Jiang, N., et al. (2023a). A systematic review and meta-analysis of voxel-based morphometric studies of migraine. J. Neurol. 270, 152–170. doi: 10.1007/s00415-022-11363-w
Zhang, Z., Zhong, W., Zhang, K., and Li, M. (2023b). The dose-effect relationship of electroacupuncture analgesia and its stimulus parameters: Progress in the last 3 decades. World Journal of Acupuncture-Moxibustion, 33, 12–19. doi: 10.1016/j.wjam.2022.09.003
Zhao, J. (2016) Effects of patients with low back pain treated by spinal manual therapy: A fMRI study based on amplitude of frequency fluctuation, Guangzhou University of Chinese Medicine.
Zhao, Y. (2021) A resting state functional magnetic resonance imaging study of chronic non-specific low Back pain with depression, Shanghai University of Sport.
Zhou, F., Gu, L., Hong, S., Liu, J., Jiang, J., Huang, M., et al. (2018). Altered low-frequency oscillation amplitude of resting state-fMRI in patients with discogenic low-back and leg pain. J. Pain Res. 11, 165–176. doi: 10.2147/JPR.S151562
Zhou, M., Hu, X., Lu, L., Zhang, L., Chen, L., Gong, Q., et al. (2017). Intrinsic cerebral activity at resting state in adults with major depressive disorder: a meta-analysis. Prog. Neuro-Psychopharmacol. Biol. Psychiatry 75, 157–164. doi: 10.1016/j.pnpbp.2017.02.001
Zhou, X., Lv, Z., Huang, Y., Wu, S., Hong, Z., Lv, L., et al. (2024a). Study on the immediate brain network effect of lever positioning manipulation in the treatment of lumbar disc herniation based on resting state functional magnetic resonance imaging. Chin. J. Integr. Tradit. West. Med. 44, 49–55. doi: 10.7661/j.cjim.20230731.285
Zhou, X., Lv, Z., Wu, S., Tian, Y., Hu, H., Wang, K., et al. (2024b). Immediate functional brain networks in patients with lumbar disc herniation using "PressEmergence sensation" at pressure pleasantly-sensitive Weizhong point(BL 40). Chin. J. Integr. Tradit. West. Med. 44, 814–820. doi: 10.7661/j.cjim.20240416.076
Zhou, F., Wu, L., Guo, L., Zhang, Y., and Zeng, X. (2019). Local connectivity of the resting brain connectome in patients with low back-related leg pain: a multiscale frequency-related Kendall's coefficient of concordance and coherence-regional homogeneity study. Neuroimage Clin. 21:101661. doi: 10.1016/j.nicl.2019.101661
Zhu, Y., Zhao, H., Gao, Y., Li, J., and Sang, D.. (2020). Changes of regional homogeneity in brain in patients with chronic low back pain due to lumbar disc herniation. J. Xinxiang Med. Univ. 37, 777–782. doi: 10.7683/xxyxyxb.2020.08.018
Zou, Y., Tang, W., Wang, S., Huang, J., Li, J., et al. (2019). Objective evaluation on brain network imaging of "treating same disease with different methods" effect of acupuncture for chronic low back pain(cLBP). Fudan Univ. J. Med. Sci. 46, 167–173. doi: 10.3969/j.issn.1672-8467.2019.02.004
Keywords: chronic low back pain, functional magnetic resonance imaging, spontaneous brain activity, meta-analysis, resting-state
Citation: Chen X, Chen N, Lai P, Sun Y, Yu J, Xin M, Zhu D, Liang F, Song Q, Cheng S and Li Z (2025) Multimodal abnormalities of brain function in chronic low back pain: a systematic review and meta-analysis of neuroimaging studies. Front. Neurosci. 19:1535288. doi: 10.3389/fnins.2025.1535288
Received: 27 November 2024; Accepted: 07 January 2025;
Published: 05 February 2025.
Edited by:
Liangjie Lin, Philips Healthcare (China), ChinaReviewed by:
Ning Zheng, Clinical & Technical Support, Philips Healthcare, ChinaCopyright © 2025 Chen, Chen, Lai, Sun, Yu, Xin, Zhu, Liang, Song, Cheng and Li. This is an open-access article distributed under the terms of the Creative Commons Attribution License (CC BY). The use, distribution or reproduction in other forums is permitted, provided the original author(s) and the copyright owner(s) are credited and that the original publication in this journal is cited, in accordance with accepted academic practice. No use, distribution or reproduction is permitted which does not comply with these terms.
*Correspondence: Shirui Cheng, Y2hlbmdzaGlydWlAY2R1dGNtLmVkdS5jbg==; Zhengjie Li, bHpqYmVuamFtaW5AMTYzLmNvbQ==
†These authors have contributed equally to this work
Disclaimer: All claims expressed in this article are solely those of the authors and do not necessarily represent those of their affiliated organizations, or those of the publisher, the editors and the reviewers. Any product that may be evaluated in this article or claim that may be made by its manufacturer is not guaranteed or endorsed by the publisher.
Research integrity at Frontiers
Learn more about the work of our research integrity team to safeguard the quality of each article we publish.