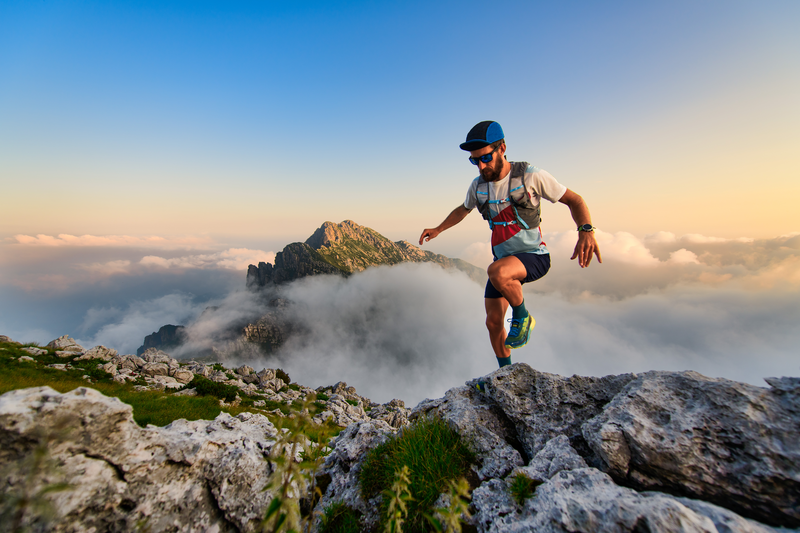
95% of researchers rate our articles as excellent or good
Learn more about the work of our research integrity team to safeguard the quality of each article we publish.
Find out more
ORIGINAL RESEARCH article
Front. Neurosci. , 01 April 2025
Sec. Neuropharmacology
Volume 19 - 2025 | https://doi.org/10.3389/fnins.2025.1466166
Nicotinic receptor signaling is influential in modulating appropriate responses to salient stimuli within a complex environment. The cholinergic neurotransmitter system drives attention to salient stimuli such as stressors, and aids in orchestrating the proper neural and behavioral responses. Dysregulation of this system, however, has been implicated in altered anxiety regulation and mood disorders. Among the multiple layers of regulation are protein modulators such as Lynx2/Lypd1, which provides negative nicotinic acetylcholine receptor regulation within anxiety-related circuits, such as the amygdala and medial prefrontal cortex, among other brain regions. Mice null for Lynx2/Lypd1 (Lynx2 KO) show elevated basal anxiety-like behavior in tests such as elevated plus maze, light-dark box and social interaction assays. Here, we queried how a line predisposed to basal anxiety-like behavior would respond to specific stressors, using validated models of experiential-based affective disorders such as fear extinction, acute and chronic social defeat stress assays. We discovered that Lynx2 KO mice demonstrate an inability to extinguish learned fear during fear extinction tests even during milder stress conditions. In social defeat studies, contrary to our predictions, the Lynx2 KO mice switched from a socially avoidant phenotype (which could be considered susceptible) before defeat to a social approach/resilient phenotype after defeat. Consistent with reports of the inverse relationship between resilience and BDNF levels, we observed reduced BDNF levels in the VTA of Lynx2 KO mice. Furthermore, we provide evidence for the functional role of α7 nicotinic receptor subtypes by phenotypic rescue of fear extinction and social defeat phenotypes by MLA antagonism of α7 nicotinic acetylcholine receptors, or by crossing with α7 nicotinic acetylcholine receptor null mutant mice. A stable physical interaction between LYNX2 and α7 nAChRs was observed by co-immunoprecipitation of complexes from mouse amygdalae extracts. Together, these data indicate that responses to specific stressors can become aberrant when baseline genetic factors predispose animals to anxiety dysregulation. These studies underscore the critical nature of well-regulated nicotinic receptor function in the adaptive response to environmental stressors.
The stress response is an adaptive suite of physiological and psychological changes that helps an individual to galvanize appropriate responses to threatening situations (Robinson et al., 2019). If not regulated properly, however, individuals can develop persistent, pervasive, and generalized anxiety or fear in the form of an affective disorder that is detrimental to quality of life (Grogans et al., 2023) and which can lead to maladaptive damage in the long-term and comorbidities such as depression (Maron and Nutt, 2017; Schneiderman et al., 2005). The capacity to moderate one’s response to stressors—to balance adaptive protection in the short term and maladaptive changes in the long term—is critical as disorders involving anxiety and fear are among the most prevalent mental disorders, affecting 33.7% of US adults at some point in their lives (McEwen, 2017; Stein et al., 2017; Grogans et al., 2023; Kessler et al., 2012). Despite the high prevalence of affective disorders, current treatments do not meet the full need, highlighting the utility of further understanding the complex underpinnings of anxiety to inform the development of effective treatments.
The cholinergic system is an important modulatory neurotransmitter system involved in a number of adaptive behaviors. Nicotinic acetylcholine receptors (nAChRs) of the cholinergic system have been shown to regulate activity in anxiety, fear, and depressive-related circuits and related behaviors in animal studies (Mineur et al., 2023; Mineur and Picciotto, 2019; Pang et al., 2016; Jiang et al., 2016; Wilson and Fadel, 2017). The use of nicotine has been demonstrated to both increase and decrease anxiety, in various contexts (Kutlu and Gould, 2015; Mineur et al., 2023; Mineur et al., 2016; Picciotto, 2003). People report self-medicating with nicotine products to alleviate anxiety (Ferraz Lima et al., 2023), and there is an association between genes of the nicotinic receptor system and anxiety (Gillentine et al., 2018; Markett et al., 2011). LYNX2, a nicotinic modulatory protein, is encoded by the Lynx2/Lypd1 gene, expressed in key regions of anxiety and fear circuitry, such as the amygdala and medial prefrontal cortex, in addition to other CNS sites (Dessaud et al., 2006; Tekinay et al., 2009; Sherafat et al., 2021), and sites outside the nervous system (Song et al., 2024; Omori et al., 2024). The LYNX2 protein binds to and reduces the activity of nAChRs (Tekinay et al., 2009; Wu et al., 2015) in response to its natural ligand, acetylcholine, as well as the drug of abuse, nicotine. The Lynx2 null mutant mouse (Lynx2KO) displays heightened fear and anxiety-like behavior across several paradigms of basal anxiety, including light–dark box, elevated plus and social interaction tests (Sherafat et al., 2021; Tekinay et al., 2009), and changes in an acoustic startle response in male Lynx2KO mice (Sherafat et al., 2021). Removal of Lynx2 leads to hyperactivity of nAChR responses, sensitizing nAChR to agonist and slowing receptor desensitization (Tekinay et al., 2009). We posited that an imbalance of nicotinic signaling is playing a role in the heightened basal anxiety-like behavior, which can influence situational or specific threats in the environment (O’Leary et al., 2013). The development of affective disorders often results from long-term adaptation to dynamic and complex conditions (such as basal anxiety and context). Therefore, we sought to probe how heighted nAChR activity in anxiety-related circuits (Calhoon and Tye, 2015; Anderson and Adolphs, 2014) might contribute to longer-term adaptive responses in Lynx2 KO mice, employing fear extinction and social defeat stress behavioral paradigms.
Neural mechanisms allow the encoding of salient events into memories by associative learning processes, and these help us respond later to cues that may predict a threat. This can be experimentally assessed by a fear conditioning paradigm that pairs an innocuous conditioned stimulus, such as a tone, with an unconditioned stimulus, such as a shock (Hegedüs et al., 2022). Since these processes employ coincidence detection to encode a suite of associated events into memories, however, not all encoded cues are predictive. New experiences which thwart conditioned expectations allow us to update learned associations that might be coincidental and not causative. Extinction of conditioned fear is an active learning process that produces a new association, integrating the conditioned association into a safety memory and is accompanied by a reduction in a conditioned fear response (Bouton et al., 2021; Lissek et al., 2004; Grillon, 2008; Craske et al., 2014; Furini et al., 2014). Cholinergic neurons respond to unexpected sensory cues that play a role in fear learning and extinction (Hegedüs et al., 2022; Herry et al., 2008; Crimmins et al., 2023). Elevations in nAChR signaling in the amygdala have been shown to inhibit fear extinction, whereas nAChR inhibition there promotes it (Jiang et al., 2016), underscoring the need of regulating nAChR activity. Fear extinction provides insight into the complex interactions that basal state of anxiety impacts on learning and responses to a specific threat.
Social stress is a pervasive form of stress experienced by most animals and is a known component of many human affective disorders such as depression and anxiety (Fiksdal et al., 2019; Krishnan et al., 2007). An etiologically relevant paradigm of conflict for the rodent species is the chronic social defeat stress test (CSDS), where test mice undergo daily defeat sessions characterized by both physical bouts and extended sensory contact with an aggressor mouse (Golden et al., 2011; Hultman et al., 2016; Bagot et al., 2016). CSDS is a highly validated assay that produces two divergent stress responses in social interaction tests, resilient, approaching the aggressor mouse, versus susceptible, avoiding it (Berton et al., 2006; Lagace et al., 2010; Golden et al., 2011; Hultman et al., 2018; Bagot et al., 2016; Hultman et al., 2016). The resilience response to CSDS has been shown to increase upon nicotine exposure via a mechanism involving BDNF, a trophic factor implicated in many neuroplasticity processes (Khalifeh et al., 2020; Mallei et al., 2019; Pagliusi et al., 2018). It has also been shown that CSDS elicits BDNF release in the ventral tegmental area (VTA) in susceptible wild-type mice (Koo Wook et al., 2016; Koo et al., 2019). While the VTA, a midbrain dopaminergic structure, is not considered a part of the primary anxiety-related circuit, it has an important role in many processes, including assessing the difference between expectation and outcome, and neuroplastic changes underling it (Anacker et al., 2016; Wang et al., 2023; Cao et al., 2010).
We probed the extent to which the dysregulation of nAChRs in anxiety-related circuits and subsequent heightened basal anxiety in Lynx2 KO mice would alter the complex behaviors of fear extinction and social defeat (Tekinay et al., 2009; Sherafat et al., 2021). Our prediction was that their heightened baseline anxiety-related phenotype would disproportionately affect their response to fear extinction and social defeat. To begin to address the mechanism, we studied the effect of Lynx2 removal on nAChR signaling and any subsequent changes in BDNF.
All animal procedures were conducted in accordance within the guidelines of Lehigh University IACUC to ensure the humane and ethical treatment of the animals. Animals were kept on a 12/12-h light–dark cycle with ad libitum access to food and water. Animals were weaned at 21 days of age and separated by sex into group housing ranging from 2 to 5 animals per cage. A total of 3- to 8-month-old naïve, male and female, wild-type (WT) (C57BL/6 J), Lynx2KO, and double null mutant mice for the α7 nAChR and Lynx2 (α7/Lynx2 double KO mice) were used. Breeding of the Lynx2KO mice included null mutant crosses to create knockout mice for experiments, backcrosses the C57BL/6J mice (C57) to maintain genetic diversity and avoid genetic drifts, and crosses of Lynx2 heterozygous mice from the backcrosses to other Lynx2 heterozygous mice from different pairs or knockout mice to refresh to the null mutants. The backcross was introduced every three generations. The α7/Lynx2 double KO mice were created by crossing Lynx2KO null mutant mice with α7 null mutant mice. Litters from first generation double heterozygous (Lynx2 +/− α7 +/−) mice were crossed together, and second-generation mice were genotyped to find either one allele as a null mutant (−/−) and the other allele as heterozygous (+/−) or for double mutants (D−/− or D+/−). Combinations of the possible genotypes [such as Lynx2KO (−/−), α7 heterozygous (+/−) and Lynx2 heterozygous (+/−) and α7KO (−/−)] were crossed until several double mutants were verified. The double mutants were crossed to create mice for experiments. Backcross to C57BL/6 J mice were added every three generations and added into the breeders as described before to avoid genetic drift. All mice were genotyped using a polymerase chain reaction assay from DNA that was extracted from a tail biopsy. Each tissue sample was genotyped 2–3 times before the confirmed genotype was assigned. All mice were involved in one behavioral assay.
Power analysis was conducted before data collection. For all statistical analyses, a α = 0.05 was used to establish a statistically significant result.
For fear extinction experiments, data were collected as the number of seconds spent freezing during the behavior trial on day 1 and day 2 of extinction. This was divided by the total amount of time in the chamber and multiplied by 100% to obtain the % freezing on each day. These data are represented by line graphs. A two-tailed paired Student’s t-test was performed on the set of observations on day 1 of extinction and the set of observations on day 2 of extinction in order to determine if there was a significant reduction in freezing. As a measure of fear extinction, the percentage freezing on day 2 was subtracted from the percentage freezing on day 1. Thus, the reduction in % freezing could be compared between groups such as sex and genotype. This was done using the R anova_test() function to compute one- or more-way ANOVA, depending on the number of variables being evaluated for their effect. Post-hoc Student’s t-tests were conducted when a significant result was obtained. These datasets are represented by boxplots. For the 5-day extinction trials shown in 1b, these line graphs were simply extended to have 5 days on the x-axis. A repeated measures ANOVA was performed on the set of observations to determine whether there was a significant reduction in freezing across days.
For chronic social defeat stress experiments, data were collected as seconds spent in the interaction zone before and after the novel mouse is added. Time spent in the interaction zone with a C57 stranger mouse versus the CD1 aggressor is graphed on a boxplot with individual data points. SI ratios were calculated by dividing the time in the interaction zone with a stranger present by the time in the interaction zone with no stranger present. SI ratios are shown on a boxplot with a scatter plot overlaid. Paired two-tailed Student’s t-tests were performed on the SI ratios between the C57 baseline interaction and the CD1 aggressor interaction after CSDS. Unpaired two-tailed Student’s t-tests were performed to assess the effect of genotype on SI ratios at each time point. Mice were assigned as either resilient or susceptible based on the SI ratio of a certain trial. Fisher’s exact test was performed on the distribution among these groups.
Western blots were scanned using a ChemiDoc imaging system using the stain-free gel program and analyzed using ImageJ. Molecular weight of bands was determined by dividing the location of each band on the standard by the length of the gel. This was plotted on the y-axis against the logarithm of the known molecular weights of the standards. A line of best fit could be generated from this scatter plot. Then, the quotient associated with the distance traveled along the gel of the sample bands can be fit to this line, the x-value solved for, and the exponent of this value determined. The relative intensity of BDNF in samples as compared to actin can be determined by taking the pixel intensity of each band, as well as the space directly above and below it as a control. The intensity of the BDNF band was divided by the intensity of the actin band, and these values were plotted on a bar graph, with error bars representing the standard error among the digital measurements. No statistical analysis was performed on this gel since only one replicate of the gel was run.
All major behavioral assays were performed with a minimum of three replicates or independent cohorts to ensure a biological phenomenon as opposed to a one-time result. Replicates were temporally separated tests, and each included a different set of wild-type and knockout mice. All data are given as 95% confidence intervals (CIs) and are represented as AVG ± SEM. *p < 05, **p < 0.01, and ***p < 0.001.
Fear conditioning and extinction is a 3-day experiment carried out in a conditioning hub (Coulbourn Instruments) where mice were placed inside an isolation cubicle (Coulbourn Instruments) to prevent ambient light and sound interference. The hub environment was lined with silver metallic walls, washed with isopropyl alcohol between trials, and had a mounted shock floor. On day 1, mice were placed in the cubicle and underwent a 2-min acclimation which consists of 2, 30 s sound (80 dB and 8 kHz) presentations, 2 min apart (20 WT mice, male; n = 10, female, n = 10; 19 Lynx2 KO mice, male, n = 9; female, n = 10). To induce fear conditioning, during the last 2 s of the sound, the animal received a mild (0.5 mA) foot shock, with a 30-s rest period after the last foot shock, and the animals were returned to their home cage. For the weaker fear extinction paradigm, mice received only 1 tone-shock pairing at 0.25 mA shock. This was run with a different set of mice than the 2 tone-shock pairing. Fear conditioning is a day of learning for mice, and they learn to expect that the shock will follow when a tone is heard.
After the first day of fear conditioning, day 2 and 3 fear extinction took place, with multiple measures taken to control for context. Mice were placed in a different cubicle than the one used for day 1 of fear conditioning, the walls of the hub were switched to colorful patterns, a lavender scent was introduced, and between trials, the cubicle was washed with the novel cleaning agent, ethanol. This creates a different environment for the mouse and leaves the tone as the only constant. After 30 s of acclimation in the extinction chamber, the animal was presented with 3 sound presentations at the same frequency and intensity as on the training day, each 30 s long and 2 min apart, but with no foot shocks.
The behavior of the mice was recorded using an in-hub camera, and time spent freezing was analyzed using Coulbourn FreezeFrame software. The data are collected based on freezing, which was considered to be no bout of movement in a 2-s frame. A minimum cutoff value of 50% freezing on day 1 was used to eliminate mice that did not show freezing behavior. The criteria for an extinguished fear response were a statistically significant decrease in percent freezing to tone (CS) between day 2 (Ext 1) and day 3 (Ext 2).
To confirm data, we extended the test from 2 days to 5 days following the same procedures. Mice were placed in a different cubicle than the one used for day 1 of fear conditioning, the walls of the hub were switched to colorful patterns, a lavender scent was introduced, and between trials, the cubicle was washed with the novel cleaning agent, ethanol. This creates a different environment for the mouse and leaves the tone as the only constant. After 30 s of acclimation in the extinction chamber, the animal was presented with 3 sound presentations at the same frequency and intensity as on the training day, each 30 s long and 2 min apart, but with no foot shocks.
Fear conditioning was performed as previously described but with the addition of an IP injection given to Lynx2KO mice and WT mice 1 h prior to fear extinction on days 2 and 3. Mecamylamine (Mec) (a non-specific nAChR blocker), Methyllycaconitine (MLA) (an α7 antagonist), and Dihydro-β-erythroidine (DHβE, a β2* nAChR antagonist) can cross the blood–brain barrier at several documented doses, with enough potency to produce changes to nAChR function (Turek et al., 1995; Damaj et al., 1995). Doses were chosen based upon such studies. Mec (0.3–2.5 mg/kg, Abcam, Cambridge, MA), DHβE (2 mg/kg, Tocris Bioscience, Bristol, UK), and MLA (5 mg/kg, Sigma – Aldrich, St Louis, MA) were dissolved in 0.9% saline. The control animals were injected with 0.9% saline. Successful fear extinction was defined as a decrease in percent freezing from Ext 1 to Ext 2. Ext 2 is analyzed as the final extinction time point.
The light–dark box assay was conducted in TruScan motion tracking arena (Coulbourn Instruments). A dark walled box was placed inside the back half of the arena with the front half surrounded by clear walls. An arched opening was made between the two areas. Lynx2KO or WT mice were initially placed in the light side, and their location was monitored for 10 min by the TruScan software. Mice show an increase in anxiety-like behavior when spending an extended period in the dark causing an increased latency. For pharmacological studies, mice were given an injection, IP, 1 h prior to the start of testing.
CSDS followed a method adapted from the standardized protocol (Golden et al., 2011), with the CD1 mouse strain used as aggressors (Hsieh et al., 2017). First, CD1 mice were singly housed for 7 days followed by a screen for aggression over 3 days. During each day of screening, an 8 to 20-week-old WT male mouse was added to the home cage of the CD1, and the latency of the CD1 to attack is recorded. The WT mouse was removed upon attack or after 3 min. An attack was defined as a physical altercation that included, but was not limited to, biting, shoving, rushing, jumping onto tail rattling, and kicking. CD1 aggressors must show aggression in at least 2 subsequent sessions and have an attack latency of less than 60 s to be considered as an aggressor.
We set up two groups: non-defeated and defeated mice. Non-defeated mice (WT, Lynx2KO, α7/Lynx2double KO (α7/L2 double KO) were housed with littermates after weaning. They were placed in the same room as defeated mice and not disturbed until they were used for social interaction. For defeat, test mice (WT, Lynx2KO, α7/Lynx2double KO) were exposed to a CD1 aggressor mouse for a daily bout of 10 attacks. The attacks were limited to 10 to prevent excessive harm to the test mice that was observed with a defined time window. Following the bout, the intruder mouse was physically separated from the CD1 aggressor within the CD1 home cage but kept in sensory contact for 24 h. This was repeated each day for 10 days, after which, the test mice were singly housed for 24 h to await behavioral testing.
We adapted the 10-day CSDS to test the robustness and sensitivity of the post-defeat phenotypes in this particular genetic model of anxiety-like behavior. We carried out the same procedure as described above in CSDS, but the defeat sessions occurred over 3 days. After the 3rd day of defeat, the test mice were singly housed. We found that the 3 days were sufficient to induce enough of a response in the test mice that conferred with the matching behavioral results of the 10-day protocol.
The social interaction (SI) test occurred 24 h after the defeat session ended and mice were singly housed. SI ratios were assessed in Coulbourn TruScan motion tracking arenas. The arena was calibrated with an interaction zone against the back-most wall, and a wire containment cage was built to the specifications of the protocol (Golden et al., 2011).
For each phase of social interaction test, post-defeat mice or controls (naïve WT and Lynx2KO mice that did not undergo defeat and were kept in the standard housing of 2–5 littermates), called defeated or non-defeated test mice, respectively, were placed in the center arena and tracked for 150 s. The initial phase consisted of an empty interaction zone cage. Immediately following the completion of phase 1, the test mouse was removed from the arena and returned to its home cage. During the break, a novel CD1 stranger mouse was added to the wire containment cage within the interaction zone. The same test mouse was again placed in the center of the arena and tracked for 150 s. During this second phase, sensory information could be transmitted from the stranger to the test mouse, but there was no physical contact.
The location of the test mouse was tracked by the TruScan software. Social interaction ratios were determined based on time in the target zone (24 × 14 × 9 cm box surrounding the interaction zone) with and without the stranger mouse present. The social interaction (SI) ratio was used to assign the test mouse to a group: resilient or susceptible. The SI ratio is calculated by the following formula:
For these studies, an SI ratio above 1.5 was considered “resilient” or “approach” and below 1.5 “susceptible” or “avoidant” rather than the standard 1 as the Lynx2KO mice were shifted toward higher SI ratios. We choose an SI ratio of 1.5 as our cutoff to increase the criteria needed to reach the resilient phenotype. Upon separating the groups, the data are presented as the percentage of time spent in the interaction zone with the stranger mouse present.
Test mice first underwent a CD1 social interaction test with a CD1 stranger mouse on day 11. The stranger mice used in the social interaction were either naïve to the test mouse, or in some cases for the CD1, the stranger was the most remote CD1 aggressor to that test mouse, from day 1 of CSDS. Only males were used in these studies due to the difficulty of initiating attack behavior directed toward female mice (Takahashi et al., 2017).
WT, Lynx2KO, and α7KO mice were anesthetized with isoflurane and rapidly decapitated. The BLA was bilaterally dissected using visual landmarks and immediately homogenized in the bullet blender tissue homogenizer (NextAdvance, Averill Park, NY, USA), with Co-IP buffer (50 mM Tris, 150 mM NaCl, 0.75% Triton-X 100, Pierce protease inhibitor cocktail). Dynabeads A (Thermo Fisher Scientific, Waltham, MA, USA) were pre-incubated with 5 μg rabbit anti-lypd1 (LYNX2) primary antibodies (Thermo Fisher Scientific, Waltham, MA, USA) and thoroughly washed. An input sample of each homogenate was removed and kept at −80 degrees Celsius until Western blot analysis. Brain homogenates were incubated at a concentration of 13 mg/mL with the Dynabeads-antibody complex for 3 days nutating at RT. After washing, LYNX2 (LYPD1) protein complexes including interacting proteins were eluted and immediately prepared for Western blot analysis. Input (sample prior to the immunoprecipitation) and supernatant (after the immunoprecipitation) lane were to be compared to IP samples in the Western blot analysis. Co-immunoprecipitation experiments were performed in three replicates, each with one Lynx2KO mouse and two WT mice for a total of 18 technical replicates with 9 animals. The Lynx2KO mice were used as a negative control for the LYPD1 antibody. The α7KO mice were used as a negative control for the α7 antibody (data not shown).
Samples were denatured in 1x sample buffer (Thermo Fisher Scientific, Waltham, MA, USA) at 95°C and run on a 4–20% gradient SDS-PAGE gel (Bio-Rad Laboratories, Hercules, CA, USA). Gels were transferred onto activated PVDF membrane via a semi-dry transfer. The membrane was blocked with 5% milk/0.05% Tween-PBS for 1 h at 4°C followed by an incubation overnight at 4°C in mouse monoclonal anti-nicotinic acetylcholine receptor α7 Subunit (1:1000, Sigma-Aldrich, St Louis, MO) or rabbit polyclonal anti-BDNF (5 μg/mL, Millipore, Burlington, MA, USA) for the BDNF study. After thorough washing, membrane was incubated with conjugated goat anti-mouse (Abcam, Cambridge, MA, USA) at 1:10,000 for 2 h at 4°C or 1:10,000 or conjugated goat anti-rabbit (Abcam, Cambridge, MA, USA) at 1:10,000 for 2 h at 4°C for BDNF study. Membranes were incubated in ECL (Thermo Fisher Scientific, Waltham, MA, USA) and exposed to film. Loading controls were run using mouse anti-actin primary antibodies (Abcam, Cambridge, MA, USA) at 1:1000 dilution and goat anti-mouse secondary antibodies (Life Technologies, Carlsbad, CA, USA) at 1:40,000 dilution.
To discern how Lynx2KO mice responded in a paradigm that requires the updating of previously learned associations in response to new information, we tested the Lynx2KO in a fear extinction paradigm. Mice were trained by being subjected to two pairings of an innocuous tone and a foot shock and, 24 h later, were tested for freezing behavior in response to the tone alone (CS). Extinction trials were presented over 2 days, three CS trials per day. Over the course of fear extinction, Lynx2KO mice (red line) do not undergo fear extinction as demonstrated by the maintenance of freezing over the 2 extinction days. WT mice (black line), on the other hand, undergo fear extinction, measured as a reduction in freezing from extinction day 1 to day 2. A one-way repeated measures ANOVA was conducted, and there was a significant effect of genotype [p = 0.011, Wilks’ Lambda = 0.766, F(1, 34) = 7.918]. Two paired t-tests were used for post-hoc comparisons. There was significantly greater freezing in Lynx2KO (73.93 +/− 1.84%) as compared to WT mice (68.75 +/− 2.81%) on day 1 of extinction (paired samples t-test, t (35) = 35.07, p < 0.001). On day 2 of extinction, the Lynx2KO mice (73.04 +/− 1.66%) also showed greater freezing than WT (58.88 +/−2.2822%), paired samples t-test, t(35) = 32.757, p < 0001. WT n = 20, L2KO n = 19, WT 95% CI [51.548, 64.224], L2KO 95% CI [69.536, 76.310], Cohen’s d = 1.535. Two-way ANOVA for sex*genotype F = 0.743, p = 0.395 (Figure 1A).
Figure 1. Marked lack of fear extinction in Lynx2KO mice. (A) Over the course of fear extinction, Lynx2KO mice (red line) do not undergo fear extinction as demonstrated by the maintenance of the degree of freezing between extinction day 1 and day 2. WT mice (black line) do undergo fear extinction, decreasing freezing over the course of 2 extinction days (p = 0.011, one-way repeated ANOVA). The Lynx2KO mice show higher amounts of freezing on both extinction days 1 (**p < 0.01) and 2 (***p < 0.001) as compared to WT mice. Y-axis is the % freezing to tone (CS) during the test period. (B) No sex differences were observed in fear extinction tests (p = 0.0787). The reduction in freezing behavior from day 1 to day 2 was 8.334 +/− 1.180% in WT males (n = 9) and 10.031 +/− 0.852% in females (black, p = 0.078675, n = 10). In L2KO males, the reduction in freezing was 4.149+/− 0.788% (n = 8), and in females, there was an increase in freezing of −1.467 +/− 0.910 (red, p = 0.182, n = 11). Y-axis is the reduction in percentage of time freezing during the test periods. (C) Extending extinction trials to 5 days did not ameliorate the extinction deficit in Lynx2KO mice. When extending the number of extinction days from 2 to 5, WT mice (black line) display a reduction in percent freezing to tone/CS while Lynx2KO mice (red line) have an overall maintenance of fear. Lynx2KO mice continue to display elevated freezing during extinction across all days (***p < 0.001). (D) With a weaker training protocol to mitigate any differences in fear learning between WT and L2KO mice, there were no significantly different levels of freezing (%) on test day 1 (Ext 1), (p = 0.542). By the second test day (Ext 2), the Lynx2KO mice (red line) still freeze more as compared to WT mice (black line), exhibiting a lack of fear extinction behavior (p < 0.001). WT n = 10, L2KO n = 10, Y-axis is percent freezing to tone (CS).
Comparison of sex differences demonstrated comparable amounts of extinction in the WT (p = 0.182) and Lynx2KO (p = 0.183) groups (Figure 1B), two-way ANOVA for gender genotype (n = 46, p = 0.511, F = 0.743, p = 0.395). These findings indicate that sex did not significantly influence the outcomes in this study, and the males and females were grouped. In the WT group, male extinguished fear 8.32 +/−0.18% (black, p = 0.078675, males n = 9), whereas WT females reduced freezing 10.03 +/− 0.85% (n = 10). Lynx2KO males extinguished 4.149 +/− 0.788%, and females increased their fear, extinguishing −1.4672 +/− 0.9105% (red, males n = 8, females n = 11).
We found that Lynx2KO mice displayed heightened fear responses and a lack of fear extinction, as demonstrated by a maintained level of freezing over the course of extinction trials. Fear extinction is an active process that results in the formation of a new memory in which the conditioned stimulus (CS) is no longer predictive of the unconditioned stimulus (US). We sought to distinguish between lack of ability to extinguish versus a delayed or reduced sensitivity, as it is possible the Lynx2KO mice need additional input to form a safety memory that can outcompete the original fear memory. To establish the strength and longevity of the abnormal response in the fear extinction paradigm of Lynx2KO mice, we extended the number of fear extinction sessions from 2 days to 5 days.
WT mice (black line) display a reduction in percent freezing to tone/CS over the 5 extinction days, while Lynx2KO mice (red line) demonstrate a maintenance of fear. Although Lynx2KO mice show a trend in inter-trial (within extinction day) extinction after several sound presentations, the reduction in freezing from day to day is not significant resulting in a lack of extinction behavior. A one-way repeated measures ANOVA was conducted to compare the effect of genotype on freezing during extinction day 1 through extinction day 5. There was a significant effect of genotype [p = 0.006, Wilks’ Lambda = 0.410, F(4, 24) = 4.654]. Five paired t-tests were used for post-hoc comparisons, and in each day, the Lynx2KO group demonstrated significantly greater freezing than the WT one (day 1 t(28) = 50.919, p < 0.001, day 2, t(28) = 33.236, p < 0.001, day 3, t(28) = 28.178, p < 0.001, day 4, t(28) = 21.075, p < 0.001, day 5, t(28) = 20.199, p < 0.001 WT n = 12, L2KO n = 16. Day 5 95% CI: WT [53.230, 60.804], KO [67.828, 71.665], Cohen’s d = 1.089) (Figure 1C).
A possible confound in assessing extinction ability could be the heightened fear learning exhibited by Lynx2KO mice. To mitigate this, a milder training protocol used to establish the fear-based associative memory was implemented, using a single tone-shock pairing and reduced shock intensity. With this milder training protocol, there are no differences between groups in fear learning, as demonstrated by day 1 percent freezing to tone (CS). Lynx2KO mice (red line), however, continue to exhibit a lack of fear extinction behavior, whereas WT mice undergo fear extinction (black line). A one-way repeated measures ANOVA was conducted to compare the effect of genotype on freezing during extinction day 1 and extinction day 2. There was a significant effect of genotype [Wilks’ Lambda = 0.706, F(1, 17) = 7.095, p = 0.016]. Two paired t-tests were used for post-hoc comparisons. A first paired samples t-test indicated no significant effect of genotype on day 1 freezing [t(18) = 31.983, p = 0.542]. A second paired samples t-test indicated a significant effect of genotype on day 2 freezing (t(18) = 24.055, p < 0.01. WT n = 10, L2KO n = 10, WT 95% CI [44.074, 61.840], L2KO 95% CI [57.740, 67.904], Cohen’s d = 0.996) (Figure 1D).
We hypothesized that the Lynx2KO phenotype was due to nAChR hyperactivity from our current understanding of the mechanism of action of Lynx2 and family members such as Lynx1 (Tekinay et al., 2009; Ibañez-Tallon et al., 2002; Miwa et al., 2006; Sherafat et al., 2021; Morishita et al., 2010). We tested the ability of nAChR pharmacological treatment to restore normal extinction behavior in Lynx2 KO mice (Figure 2A). Injection of mecamylamine, a general nAChR pore blocker, prior to extinction testing rescued fear extinction behavior in the Lynx2KO mice (p = 0.000413, unpaired Student’s t-test). These doses of mecamylamine, 0.3, 1.0, and 2.5 mg/kg, have no effect on the WT mice in this paradigm. A one-way repeated measures ANOVA was conducted to compare the effect of the drug in Lynx2KO mice on extinction, demonstrating a significant effect of the drug [Wilks’ Lambda = 0.750, F(1, 56) = 6.236, p = 0.001]. Bonferroni post-hoc showed a significant effect at each mecamylamine dose; L2KO vs. L2KO 0.3 mg/kg (M = −7.154, SE = 2.568) p = 0.044, L2KO vs. L2KO 1 mg/kg (M = 11.337, SE = 2.66) p < 0.001, L2KO saline n = 20, L2KO 2.5 mg/kg n = 11, p < 0.001, L2KO 1 mg/kg n = 14, L2KO 0.3 mg/kg n = 10. 95% CI, L2KO [69.536, 76.310], L2KO 2.5 mg/kg [53.717, 69.934], L2KO 1 mg/kg [47.831, 59.615], L2KO 0.3 mg/kg [53.184, 65.183] (Figure 2B).
Figure 2. Cholinergic blockade implicates distinct nAChRs in Lynx2-mediated behaviors (A) Blocking nAChR channels with mecamylamine rescues the lack of fear extinction in Lynx2KO mice, compared to Lynx2KO mice without mecamylamine at all doses tested (red line, p = 0.001). Y-axis is percent of the time freezing to tone (CS). (B) Mecamylamine blockade of nAChRs does not have a significant effect in the WT mouse (p = 1.057). Y-axis is percent of the time freezing to tone. (C) Antagonism of α7, but not DHBE, nAChRs rescues the fear extinction phenotype in Lynx2 KO mice (red, p = 0.0000186, two-way ANOVA). Blocking α7 nAChRs with MLA (1 mg/kg) allowed Lynx2 KO mice (red) to extinguish, as seen as a reduction in freezing behavior (p = 0.000143). Each dot represents the difference in percent freezing (Ext 1 - Ext day 2) for a male (blue) or female (pink) mouse. A two-way ANOVA revealed that the difference in fear extinction among treated wild-type mice (black) was not statistically significant from naive wild-type mice. (p = 0.79) Y-axis is the reduction of freezing from day 1 to day 2.
Utilizing more specific nAChR antagonists, we found that MLA, an α7 antagonist, rescued the Lynx2KO fear extinction phenotype and demonstrated levels of extinction comparable to WT mice (naive n = 19, treated n = 14, p = 0.000143, unpaired Student’s t-test) (Figure 2C). Dihydro-β-erythroidine (DhβE), a heteromeric nAChR antagonist as opposed to the homomeric α7 nAChR subtype, did not alter extinction in Lynx2KO mice (naive n = 19, treated n = 6, p = 0.4311) (Figure 2C), suggesting that the Lynx2-based extinction phenotype is mediated by α7 nAChRs [one-way ANOVA F(7) = 10.629, p < 0.001] (Drasdo et al., 1992; Damaj et al., 1995). A two-way ANOVA was conducted on these data, assessing extinction across sexes and genetic lines. This revealed that the difference in fear extinction among treated wild-type (black) mice was not statistically significant as compared to naive WT mice (p = 0.79), whereas this effect was significant in Lynx2KO mice (red) (p = 0.0000186) (Figure 2C). In addition, a Cohen’s d-test was used to determine the effect size of these treatments on Lynx2KO mice. The effect size of mecamylamine was 1.15, the effect size of MLA was 1.22, and the effect size of DhβE was 0.277.
To investigate how an etiologically relevant stressor influences responses of mice predisposed to anxiety, we conducted chronic social defeat stress (CSDS) and assessed by a social interaction test. We first ran a control social interaction test, without a stress component, to confirm the abnormal sociability-like behavior of the Lynx2KO previously reported in (Tekinay et al. (2009) and determine whether it had been maintained over the course of generations. In social interaction tests, a test mouse was added to an arena with a restrained conspecific stranger mouse which allowed for the flow of sensory information of the stranger without physical contact. The evaluation of the phenotype was conducted by generating an SI ratio of time spent in the interaction zone with the stranger mouse vs. when there was no stranger mouse present, using a cutoff of 1.5 (see Methods, data not shown). The naive Lynx2KO mice avoided the C57Bl6 stranger mouse to a greater degree than WT mice did. These observations were consistent with previous reports (Tekinay et al., 2009), confirming the affective phenotype while allowing the CD1 mice to remain novel until the start of the CSDS sessions. (Tekinay et al., 2009).
Having confirmed the social interaction phenotype was maintained in naive Lynx2KO mice, we conducted chronic social defeat stress (CSDS) on them. We performed 10 days of CSDS, entailing daily bouts of aggressive interactions with aggressive CD1 mice, with extended sensory contact between bouts. Following the 10th day, mice were singly housed for 1 day in a home cage before undergoing social interaction (SI) with a CD1 mouse as the stranger (Figure 3A). Measuring SI ratios following CSDS, the WT mice shift to lower values, whereas Lynx2KO mice demonstrate an increase in SI ratio, indicating an overall preference toward the stranger mouse (Figure 3B). Defeated WT test mice displayed the expected divergent stress responses of the population, with individuals displaying either the resilient/approach (blue) and susceptible/avoidant phenotypes (gray) (Figure 3C). On the other hand, all the post-defeat KO mice displayed the resilient/approach phenotype—a marked difference from pre-defeat scores, even with an SI ratio cutoff that increases the criteria for resiliency (Figure 3C). There was no significant difference in the time spent in the interaction zone by the resilient subset of WT mice and the Lynx2KO mice. There were significant differences in the time spent in the interaction zone between the resilient mice and the susceptible WT mice (Figure 3D). Based upon social interaction ratios post-defeat, there are WT mice that approach and spend a greater percentage of time in the interaction zone (CSDS Resilient) as compared to WT mice that do not approach and spend less time in the interaction zone (CSDS Susceptible). Interestingly, there were no Lynx2KO mice that display a non-approach/susceptible phenotype. All Lynx2KO mice post-defeat spent a larger percentage of time in the interaction zone. One-Way ANOVA F = 14.016, p < 0.001, Bonferroni post-hoc: WT vs. L2KO p = 0.019, WT vs. CSDS L2KO p = 0.005, L2KO vs. CSDS KO p < 0.001, L2KO vs. CSDS WT Resilient p < 0.001, CSDS WT Resilient vs. CSDS WT Susceptible p = 0.016, CSDS L2KO vs. CSDS WT Susceptible p < 0.001. WT n = 17, L2 KO n = 17, CSDS L2KO n = 17, CSDS WT Resilient n = 10, CSDS WT Susceptible n = 7. 95% CI: WT [27.383, 44.147], L2KO [12.504, 26.060], CSDS Resilient WT [24.782, 44.551], CSDS Susceptible WT [27.017, 43.841], CSDS Resilient L2KO [44.495, 61.701]. Cohen’s d WT No CSDS vs. KO NO CSDS = 1.118, No CSDS L2KO vs. CSDS Approach L2KO = 1.927 (Figure 3D).
Figure 3. Shift in all social interaction behavior following CSDS in Lynx2KO mice. (A) Schematic of chronic social defeat stress timeline: Prior to CSDS, CD1 mice are screened for aggression. Mice undergo 10 days of CSDS (chronic social defeat stress), entailing daily bouts of aggressive interactions with extended sensory contact. Following the 10th day, mice are singly housed for 1 day before undergoing social interaction tests (SI). (B) After experiencing chronic social defeat stress, wild-type mice show a population split in social interaction phenotype between resilient (blue) and susceptible (gray) phenotypes in response to a CD1 mouse. All Lynx2KO mice, however, exhibit a population phenotype of resilient. (C) Prior to defeat, Lynx2KO mice display less time in the social interaction zone and exhibit social avoidance (No CSDS groups, p = 0.019). Based upon social interaction ratios post-defeat, there are WT mice that approach and spend a greater percentage of time in the interaction zone (CSDS Resilient) as well as WT mice that do not approach and spend less time in the interaction zone (CSDS Susceptible). There are no Lynx2KO mice that display a non-approach/susceptible phenotype after defeat. All Lynx2KO mice post-defeat spend a larger percentage of time in the interaction zone after defeat (p < 0.001). Y-axis is percentage of the cohort, resilient (blue) susceptible (gray). (D) Social interaction ratios (SI) change in WT (p < 0.001, n = 17) and KO mice post-defeat (p = 0.038, n = 17). Y-axis is SI ratio.
Nicotine has been shown to augment resilience in response to CSDS (Khalifeh et al., 2020) and thus would be consistent with the nAChR hyperactivity that occurs due to Lynx2 removal. The heightened basal anxiety in Lynx2KO mice could sensitize them to the defeat, in which case it might have been possible to detect both resilient and susceptible CSDS phenotypes in the KO cohort if given a reduced amount of defeat stress. Alternatively, the Lynx2KO mice could exhibit an exaggerated stress response that predisposes them to adopt a uniformly resilient phenotype independent of the amount of defeat stress. This may be analogous to the effects seen in fear extinction experiments in which the Lynx2KO mice failed to extinguish their fear, even with a lower training stimuli or an extended number of extinction days. To adapt to any potential hypersensitivity or responsiveness of Lynx2KO mice, we asked whether, by lowering the amount of stress given, some of the Lynx2KO mice would adopt a susceptible phenotype. We modified the CSDS paradigm by reducing the number of defeat days from 10 to 3, in an attempt to lower the degree of stress while retaining many of the critical features of the validated CSDS paradigm (Donahue et al., 2015; Pagliusi and Sartori, 2019; Qi et al., 2022). In this experiment, which we refer to here as acute defeat stress, mice were subjected to three trials per day on three consecutive days. In this 3-day modified CSDS paradigm, the Lynx2KO mice continued to display a resilient phenotype 100% of the time. WT mice display a divergent response including both resilience/approach and susceptible (Figure 4A).
Figure 4. (A) In a 3-day acute social defeat stress paradigm, the WT and Lynx2KO mice continue to display the same trends of the 10-day CSDS paradigm: WT mice display a divergent response, while the Lynx2KO mice display one population response, resilience/approach. The double knockout restores the population response to that of WT levels with instances of both the susceptible and resilient phenotypes. (B) The α7/Lynx2 double knockout (green line) with a deletion of both the α7 nAChR and Lynx2 genes restores fear extinction behavior to that of WT levels (black line) as compared to Lynx2KO mice (red line). A one-way repeated measures ANOVA was conducted to compare the effect of genotype on freezing during extinction day 1 and extinction day 2. There was a significant effect of the genotype on freezing; Wilks’ Lambda = 0.836 [F(1, 49) = 4.793, p = 0.013]. Bonferroni post-hoc: WT vs. L2KO (M = 9.6752, SE = 3.095) p = 0.009, WT vs. α7L2 (M = 4.4857, SE = 3.22) p = 0.513, L2KO vs. α7L2KO (M = 8.3485, SE = 3.14) p = 0.032. WT n = 16, L2K n = 20, α7L2 n = 16. 95% CI: WT [51.548, 64.244], L2KO [69.536, 76.310], α7L2 [58.846, 69.045]. Cohen’s d L2KO vs. α7L2 = 1.050. (C) Western blot analyses showing the results of co-immunoprecipitation experiments. Lynx2KO was immunoprecipitated from homogenates of mouse amygdalae using an anti-Lynx2KO antibody. Then, a Western blot was run on these samples using anti-α7 nAChR antibodies (52 kd). These bands allow us to observe the stable complex of LYNX2 and the α7 nAChR subunit in the brain. WT animals are from different animals either of C57bl6 mice (WT 1-4) or WT littermates of the B2 nAChR-GFP mouse line (WT 5-7). WT 3 is from whole brain extract of a C57bl6 mouse, demonstrating enrichment of this complex in the amygdala. The amygdalae extracts of Lynx2KO mice were immunoprecipitated as controls (one shown here), Note that no bands are present in the Lynx2KO samples, indicating the specificity of the interaction.
α7 nAChRs have been shown to be one nAChR subtype targeted by the LYNX2 protein, although other nAChR subtypes have been shown to bind to LYNX2 (Pisapati et al., 2021; Wu et al., 2015; Tekinay et al., 2009). To confirm the pharmacological studies implicating α7 nAChRs in the fear extinction phenotype of Lynx2KO mice, we sought a genetic confirmation of α7 nAChRs involvement. The Lynx2KO mouse line was crossed to an α7 nAChR null mutant line (α7KO), to generate an independent Lynx2/α7 nAChR double KO line, and was tested using the fear extinction paradigm. The Lynx2/α7 nAChR double KO line was observed to extinguish to a similar degree as WT vs. Lynx2KO (p = 0.009), WT vs. Lynx2/α7 (p = 0.513), Lynx2KO vs. Lynx2/α7 (p = 0.032), rescuing the Lynx2KO phenotype, adding to the evidence that Lynx2-based extinction is α7-mediated. A one-way repeated measures ANOVA was conducted to compare the effect of genotype on freezing during extinction day 1 and extinction day 2. There was a significant effect of genotype on freezing; Wilks’ Lambda = 0.836 [F(1, 49) = 4.793, p = 0.013]. Bonferroni post-hoc: WT vs. L2 KO (M = 9.6752, SE = 3.095) p = 0.009, WT vs. α7L2 (M = 4.4857, SE = 3.22) p = 0.513, L2KO vs. α7L2KO (M = 8.3485, SE = 3.14) p = 0.032*. WT n = 16, L2KO n = 20, α7L2 n = 16. 95% CI: WT [51.548, 64.244], L2KO [69.536, 76.310], α7L2 [58.846, 69.045]. Cohen’s d L2KO vs. α7L2 = 1.050 (Figure 4B). The effect size of this genetic modification was calculated as a reduction in percent freezing, in a Cohen’s d-test between the Lynx2KO and α7KO/Lynx2 double KO lines. Within WT mice, we analyzed differences between males and females in line with reported observations for sex differences in fear extinction of WT mice (Clark et al., 2019). Within the entire dataset, we found a significant difference of genotype (ANOVA, p = 0.000799) and sex (p = 0.0248) but not in the sex × genotype interaction (p = 0.104). Sex differences were found in the WT group, but they were not found in the Lynx2KO or Lynx2/α7KO double KO groups. Male WT mice reduced freezing more than that of female WT mice (p = 0.01, male n = 10, female n = 13), contrasted with the Lynx2 KO (p = 0.182) or Lynx2/α7 dKO groups (p = 0.267) (graph not shown).
We tested the Lynx2/α7KO double KO double knockout line in the 3-day acute defeat protocol. The α7/Lynx2 double knockout mice displayed divergent stress responses of both susceptible and resilient phenotypes, to the same proportion as that of WT mice (Figure 4A), demonstrating that α7 nAChRs mediate these abnormal responses in Lynx2KO mice. These data support the involvement of Lynx2 and α7 nAChRs in both fear extinction and acute social defeat paradigms.
A LYNX2 protein-nAChR complex has never been detected in vivo but is a critical piece of information to interpret the functional relevance of the pharmacological and genetic results. To establish the physical interaction of LYNX2 and α7 nAChR proteins, co-immunoprecipitation experiments were performed to isolate LYNX2-nAChR stable complexes in the mouse brain. To detect specific components of the complex, we immunoprecipitated the LYNX2 protein from homogenates of the mouse amygdala using an anti-LYNX (LYPD1) antibody and performed Western blot analyses to detect the presence of α7 nAChRs using an anti-α7 nAChR antibody. Precipitated LYNX2-containing complexes from extracts of WT brain samples yielded a band corresponding to the α7 nAChRs subunit, providing evidence that LYNX2 forms a stable complex with α7 nAChRs in the mammalian brain (Figure 4C). No bands were seen in control samples prepared from Lynx2KO mice, indicating the specificity of the interaction. Taken together, our results indicate the role of Lynx2 modulation of α7 nAChRs in the abnormal fear extinction and responses to social defeat stress.
BDNF levels in the ventral tegmental area (VTA) have been shown to increase after CSDS in susceptible animals and to play a role in behavioral responses to CSDS (Koo Wook et al., 2016; Koo et al., 2019). We hypothesized that abnormalities in VTA BDNF levels might be a factor in the resilient phenotype seen in the Lynx2KO mice. We isolated the VTA from defeated mice and performed Western blots using an anti-BDNF antibody and quantitated the band intensity using Image J. There was a decreased BDNF level in Lynx2KO mice relative to WT controls, in both the naive and CDSD mice (Figure 5A). Compared to naive levels, there was an increase following defeat in both the groups but was more pronounced in the WT than in the Lynx2KO mice. Quantitation was obtained by measuring the intensity of BDNF normalized to actin of that lane, measured using ImageJ software (B2 CSDS M = 0.6213, SE = 0.0748; B2 control M = −0.0553, SE = 0.0949; Lynx2 CSDS M = 0.1177, SE = 0.0461; Lynx2 control M = −0.0477, SE = 0.0837. B2 CSDS vs. Lynx2 CSDS p = 0.0149) (Figure 5B). The bands were found to have molecular weights of approximately 41 kDa and 28 kDa, corresponding to the expected values for actin and BDNF, respectively (Figure 5C). These data are consistent with the role of VTA BDNF in susceptibility.
Figure 5. (A) BDNF levels in the VTA respond to the effects of CSDS: Western blot using anti-BDNF and actin antibodies showing the presence of BDNF in mouse VTA. (B) Quantitation of VTA BDNF levels: The relative intensities of the BDNF bands were normalized to actin, demonstrating a significant difference in BDNF protein between WT and Lynx2KO VTAs (p = 0.0149), measured using ImageJ software. The BDNF levels were significantly lowered after CSDS in WT. BDNF levels in the Lynx2KO VTAs did not change significantly. (C) The molecular weight of sample bands was determined by plotting the distance traveled by the standards against the log of their known molecular weight. A line of best fit was generated, and bands were fit to that line according to their rf. Bands were determined to have the molecular weight of approximately 41 and 28, corresponding to the expected values of actin and BDNF.
Lynx2 removal has been shown to lead to nAChR hyperactivity and heightened basal anxiety-like behavior (Tekinay et al., 2009). In this context, here we explored how augmented anxiety-like behavior may influence adaptive behaviors involving more specific threats. We found that Lynx2KO mice were unable to extinguish conditioned fear in extinction assays and showed a marked uniform resilience response in acute and chronic defeat stress. Fear extinction could be ameliorated through pharmacological blockade of α7 nAChRs, and in addition, fear extinction and acute social defeat could be restored through genetic perturbations of α7 nAChRs. Together with biochemical studies indicating a stable direct interaction of LYNX2 and α7 nAChRs in the amygdala, these data indicate that LYNX2 plays a regulatory role in α7 nAChR function for moderating fear extinction and responses to social defeat stress. Basal anxiety-like behavior in mice is thought to mirror trait anxiety in humans (O’Leary et al., 2013). There is a close relationship between trait anxiety, a person’s predisposition toward anxiety, and state anxiety, their reaction to specific cues or during specific events (Ree et al., 2008). Hence, it would be expected that a mouse model of elevated basal anxiety-like behavior may also have abnormalities in other fear and anxiety-like processes.
The cholinergic system is recognized as an important regulator of affective health (Mineur et al., 2016). There are optimal levels of ACh that can be beneficial, while excessive cholinergic signaling can be detrimental and lead to affective disorders. The cholinergic system is involved in both acquisition and extinction of fear memories (Kutlu and Gould, 2015), and acetylcholine is released to the auditory cortex in response to shock to promote fear learning (Krabbe et al., 2018). Extinction of learned fear involves plasticity mechanisms and the formation of new safety memories (Hascoët et al., 2001; Jiang et al., 2016; Kim et al., 2007; Dalton et al., 2012; Maren, 2015; Saha et al., 2017; Zhu et al., 2017) and includes, among other mechanisms, long-term depression of connections involved in the conditioned fear association, through activation of pathways coming from the medial prefrontal cortex to the amygdalar region (Cho et al., 2013; Gilman et al., 2018). mEPSCs frequency has been shown to be elevated in the prefrontal cortex of Lynx2KO mice in response to nicotine (Tekinay et al., 2009), and it would be interesting to test whether abnormalities in long-term depression are operating in Lynx2KO mice. Alterations in calcium levels by LYNX2 have been reported in vitro (Wu et al., 2015). Nicotinic receptor activation has been associated with lack of fear extinction (Kutlu and Gould, 2014), and optogenetic activation of cholinergic afferents from the medial prefrontal cortex into the amygdala can retard fear extinction, while optogenetic inhibition accelerates it (Jiang et al., 2016). The final output of fear comes from the central amygdala which integrates multiple inputs such as the prefrontal cortex. As such, increases in amygdala cholinergic tone may serve to weigh the balance in favor of maintenance of fear (Jiang et al., 2016). Lynx2 is expressed in the prefrontal cortex and amygdala, among many other brain regions, so it is not possible to know to what degree each might contribute to the extinction phenotype (Tekinay et al., 2009; Sherafat et al., 2021). A regulatory mechanism such as LYNX2 that can dampen nAChR responsiveness may be one such control mechanism that allows the individual to appropriately update conditioned fears.
Our data point to a regulatory role of α7-containing nAChRs within key circuits underlying social defeat as well, in line with reports tying the nicotinic receptor system to modulation of social defeat (Ortiz et al., 2022; Khalifeh et al., 2020). A relationship between responses to social defeat stress and fear extinction has been demonstrated previously, when the WT subgroup that displayed the resilient/approach response to acute social defeat also displayed a lack of fear extinction (Meduri et al., 2013). In line with this profile, Lynx2KO mice exhibit an extreme form of this relationship, a marked lack of fear extinction and a uniform resiliency/approach following CSDS (Jasnow et al., 2004; Jasnow et al., 2005), likely a consequence of unbalanced nicotinic receptor activity.
A resilient social defeat stress response has been demonstrated in nicotine exposed animals (Khalifeh et al., 2020), inducing brain-derived neurotrophic factor (BDNF), a trophic protein implicated in many neuroplasticity processes (Mallei et al., 2019; Khalifeh et al., 2020; Chou et al., 2014; Monkol et al., 2016). Other work has linked increased BDNF in the VTA to susceptible defeat outcomes (Koo Wook et al., 2016). Social defeat stress has been shown to be altered by dopaminergic activity in susceptible mice (Zhao et al., 2021; Numa et al., 2019; Krishnan et al., 2007) and to mediate mPFC activity radiating into the amygdala (Numa et al., 2019). It is unknown if increases in excitability that induce BDNF are altered in these or other brain regions of the Lynx2KO mouse due to activity-dependent acetylcholine release (Acquas et al., 1996; Letzkus et al., 2011). In our studies, α7 nAChRs have been implicated in the tests performed here, but it should be noted that the α7/Lynx2 DKO was tested with the 3-day acute defeat stress paradigm and was not tested in the standard 10-day CSDS one, so the direct test of α7 subtypes in CSDS is still an open question. Lower baseline levels of VTA BDNF or reduced BDNF induction in response to social defeat stress may explain, in part, the resilient phenotype of Lynx2KO mice. The reduction in BDNF levels post-defeat was more substantial in WT mice, but given the low starting levels of BDNF in naive mice, the modest reduction of BDNF in the Lynx2KO VTAs might be due to a floor effect. These data are consistent with the reported role of VTA BDNF in susceptibility (Letzkus et al., 2011), although more work on this line needs to be done to clarify the relationship further.
We have focused this study on the relationship of basal anxiety to other anxiety-mediated behaviors. Because of expression of Lynx2 in multiple brain regions, however, the phenotype of Lynx2KO mice is not confined to anxiety-like behavior. Interestingly, male Lynx2KO mice have been shown to have reduced acoustic startle response (Sherafat et al., 2021). Elevations in the startle response have been correlated with higher anxiety (Pantoni et al., 2020), so the reductions in acoustic startle in male Lynx2KO mice are not likely due to their increased basal anxiety-like behavior. The neural circuitry for acoustic startle response only partially overlaps that of basal anxiety, illustrating effects of Lynx2 outside of the regions we have explored here.
In humans, individuals vary in their responses to social stress and later vulnerability to psychiatric disorders (Charney and Manji, 2004; Krishnan et al., 2007). Defining the biological factors that underlie predispositions toward anxiety and how environmental stressors act to modify these biological factors can aid in the development of effective therapies. Our results are not inconsistent with studies linking variation in the human LYNX2 gene with heightened levels of anxiety in humans (Anderson et al., 2024). The Lynx2KO mouse model adds to the framework that nicotinic receptors may be an important factor in understanding affective disorders. Genetic factors which predispose an individual to heightened basal anxiety-like behavior could impair appropriate responses to more specific stressors. These results have implications for the treatment of inextinguishable anxiety and fear, such as that in generalized anxiety disorders, post-traumatic stress disorder, phobias, and other severe disorders.
Data available on request to authors.
The animal study was approved by Ethical approval to report this case series was obtained from Lehigh University’s Ethics Committee. Ethical approval to report this case series was obtained from Institutional Animal Care and Usage Committee (IACUC) (approval ID# 147). The study was conducted in accordance with the local legislation and institutional requirements.
KA: Conceptualization, Data curation, Formal Analysis, Methodology, Project administration, Supervision, Validation, Writing – original draft, Writing – review & editing. PR: Data curation, Formal Analysis, Methodology, Validation, Writing – review & editing. TP: Data curation, Formal Analysis, Validation, Writing – review & editing, Methodology. JM: Conceptualization, Data curation, Formal Analysis, Funding acquisition, Investigation, Methodology, Project administration, Resources, Software, Supervision, Validation, Visualization, Writing – original draft, Writing – review & editing.
The author(s) declare that financial support was received for the research and/or publication of this article. This study was supported by NIH NIDA R01DA043567, R21DA033831, NIH/GM GM123131, Det Frie Forskningsråd CU-29979812, PA CURE 4100068719, Lehigh University (PJR), Nemes Fellowship (KRA), Sigma Xi (KRA), BDSI/HHMI, Mountaintop Project and the Office of Creative Inquiry.
We thank Marina Picciotto for her insights and critical reading of the manuscript and Katie Hoffman for helpful discussions. The Biological Sciences department at Lehigh University, Akeive Burrows, David Geyer, Juliana Orejuela, Solomaya Schwab, Jeff Paynter, and Neel Nissen.
JMM is the founder and holds shares in Ophidion, Inc., a biotechnology company.
The remaining authors declare that the research was conducted in the absence of any commercial or financial relationships that could be construed as a potential conflict of interest.
All claims expressed in this article are solely those of the authors and do not necessarily represent those of their affiliated organizations, or those of the publisher, the editors and the reviewers. Any product that may be evaluated in this article, or claim that may be made by its manufacturer, is not guaranteed or endorsed by the publisher.
Acquas, E., Wilson, C., and Fibiger, H. C. (1996). Conditioned and unconditioned stimuli increase frontal cortical and hippocampal acetylcholine release: effects of novelty, habituation, and fear. J. Neurosci. 16, 3089–3096. doi: 10.1523/JNEUROSCI.16-09-03089.1996
Anacker, C., Scholz, J., O’Donnell, K. J., Allemang-Grand, R., Diorio, J., Bagot, R. C., et al. (2016). Neuroanatomic differences associated with stress susceptibility and resilience. Biol. Psychiatry 79, 840–849. doi: 10.1016/j.biopsych.2015.08.009
Anderson, D. J., and Adolphs, R. (2014). A framework for studying emotions across species. Cell 157, 187–200. doi: 10.1016/j.cell.2014.03.003
Anderson, K. R., Cao, W., Lee, H. S., Crenshaw, M. A., Palumbo, T. B., Fisher-Perez, E., et al. (2024). A novel anxiety-associated SNP identified in LYNX2 (LYPD1) is associated with decreased protein binding to nicotinic acetylcholine receptors. Front. Behav. Neurosci. 18:1347543. doi: 10.3389/fnbeh.2024.1347543
Bagot, R. C., Cates, H. M., Purushothaman, I., Lorsch, Z. S., Walker, D. M., Wang, J., et al. (2016). Circuit-wide transcriptional profiling reveals brain region-specific gene networks regulating depression susceptibility. Neuron 90, 969–983. doi: 10.1016/j.neuron.2016.04.015
Berton, O., McClung, C. A., Dileone, R. J., Krishnan, V., Renthal, W., Russo, S. J., et al. (2006). Essential role of BDNF in the mesolimbic dopamine pathway in social defeat stress. Science 311, 864–868. doi: 10.1126/science.1120972
Bouton, M. E., Maren, S., and McNally, G. P. (2021). Behavioral and neurobiological mechanisms of pavlovian and instrumental extinction learning.
Calhoon, G. G., and Tye, K. M. (2015). Resolving the neural circuits of anxiety. Nat. Neurosci. 18, 1394–1404. doi: 10.1038/nn.4101
Cao, J., Covington, H. E., Friedman, A. K., Wilkinson, M. B., Walsh, J. J., Cooper, D. C., et al. (2010). Mesolimbic dopamine neurons in the brain reward circuit mediate susceptibility to social defeat and antidepressant action. J. Neurosci. 30, 16453–16458. doi: 10.1523/JNEUROSCI.3177-10.2010
Charney, D. S., and Manji, H. K. (2004). Life stress, genes, and depression: multiple pathways lead to increased risk and new opportunities for intervention. Sci. STKE 2004:re5. doi: 10.1126/stke.2252004re5
Cho, J. H., Deisseroth, K., and Bolshakov, V. Y. (2013). Synaptic encoding of fear extinction in mPFC-amygdala circuits. Neuron 80, 1491–1507. doi: 10.1016/j.neuron.2013.09.025
Chou, D., Huang, C. C., and Hsu, K. (2014). Brain-derived neurotrophic factor in the amygdala mediates susceptibility to fear conditioning. Exp. Neurol. 255, 19–29. doi: 10.1016/j.expneurol.2014.02.016
Clark, J. W., Drummond, S. P. A., Hoyer, D., and Jacobson, L. H. (2019). Sex differences in mouse models of fear inhibition: fear extinction, safety learning, and fear-safety discrimination. Br. J. Pharmacol. 176, 4149–4158. doi: 10.1111/bph.14600
Craske, M. G., Treanor, M., Conway, C. C., Zbozinek, T., and Vervliet, B. (2014). Maximizing exposure therapy: an inhibitory learning approach. Behav. Res. Ther. 58, 10–23. doi: 10.1016/j.brat.2014.04.006
Crimmins, B. E., Lingawi, N. W., Chieng, B. C., Leung, B. K., Maren, S., and Laurent, V. (2023). Basal forebrain cholinergic signaling in the basolateral amygdala promotes strength and durability of fear memories. Neuropsychopharmacology 48, 605–614. doi: 10.1038/s41386-022-01427-w
Dalton, G. L., Chuan, D., Yu, T., Wang, Y. T., Florescobc, S. B., and Phillips, A. G. (2012). NMDA GluN2A and GluN2B receptors play separate roles in the induction of LTP and LTD in the amygdala and in the acquisition and extinction of conditioned fear. Neuropharmacology 62, 797–806. doi: 10.1016/j.neuropharm.2011.09.001
Damaj, M. I., Welch, S. P., and Martin, B. R. (1995). In vivo pharmacological effects of dihydro-beta-erythroidine, a nicotinic antagonist, in mice. Psychopharmacology 117, 67–73. doi: 10.1007/BF02245100
Dessaud, E., Salaün, D., Gayet, O., Chabbert, M., and deLapeyrière, O. (2006). Identification of lynx2, a novel member of the ly-6/neurotoxin superfamily, expressed in neuronal subpopulations during mouse development. Mol. Cell. Neurosci. 31, 232–242. doi: 10.1016/j.mcn.2005.09.010
Donahue, R. J., Landino, S. M., Golden, S. A., Carroll, F. I., Russo, S. J., and Carlezon, W. A. Jr. (2015). Effects of acute and chronic social defeat stress are differentially mediated by the dynorphin/kappa-opioid receptor system. Behav. Pharmacol. 26, 654–663. doi: 10.1097/FBP.0000000000000155
Drasdo, A., Caulfield, M., Bertrand, D., Bertrand, S., and Wonnacott, S. (1992). Methyl lycaconitine: a novel nicotinic antagonist. Mol. Cellular Neurosci. 3, 237–243. doi: 10.1016/1044-7431(92)90043-2
Ferraz Lima, M., Castaldelli-Maia, J. M., Apter, G., and Leopoldo, K. (2023). Neurobiological associations between smoking and internalizing disorders. Int. Rev. Psychiatry 35, 486–495. doi: 10.1080/09540261.2023.2252907
Fiksdal, A., Hanlin, L., Kuras, Y., Gianferante, D., Chen, X., Thoma, M. V., et al. (2019). Associations between symptoms of depression and anxiety and cortisol responses to and recovery from acute stress. Psychoneuroendocrinology 102, 44–52. doi: 10.1016/j.psyneuen.2018.11.035
Furini, C., Myskiw, J., and Izquierdo, I. (2014). The learning of fear extinction. Neurosci. Biobehav. Rev. 47, 670–683. doi: 10.1016/j.neubiorev.2014.10.016
Gillentine, M., Lozoya, R., Yin, J., Grochowski, C., White, J., Schaaf, C., et al. (2018). a7 nAChRs have been linked to anxiety and depression, and CHRNA7 copy number gains have been found enriched in adolescents with major depressive and anxiety disorders. J. Affect. Disord. 239, 247–252. doi: 10.1016/j.jad.2018.07.017
Gilman, T. L., Dutta, S., Adkins, J. M., Cecil, C. A., and Jasnow, A. M. (2018). Basolateral amygdala Thy1-expressing neurons facilitate the inhibition of contextual fear during consolidation, reconsolidation, and extinction. Neurobiol. Learn. Mem. 155, 498–507. doi: 10.1016/j.nlm.2018.09.010
Golden, S. A., Covington, H. E., Berton, O., and Russo, S. J. (2011). A standardized protocol for repeated social defeat stress in mice. Nat. Protoc. 6, 1183–1191. doi: 10.1038/nprot.2011.361
Grillon, C. (2008). Models and mechanisms of anxiety: evidence from startle studies. Psychopharmacology 199, 421–437. doi: 10.1007/s00213-007-1019-1
Grogans, S. E., Bliss-Moreau, E., Buss, K. A., Clark, L. A., Fox, A. S., Keltner, D., et al. (2023). (2023) the nature and neurobiology of fear and anxiety: state of the science and opportunities for accelerating discovery. Neurosci. Biobehav. Rev. 151:105237. doi: 10.1016/j.neubiorev.2023.105237
Hascoët, M., Bourin, M., and Nic Dhonnchadha, B. A. (2001). The mouse light-dark paradigm: a review. Prog. Neuro-Psychopharmacol. Biol. Psychiatry 25, 141–166. doi: 10.1016/S0278-5846(00)00151-2
Hegedüs, P., Sviatkó, K., Király, B., Martínez-Bellver, S., and Hangya, B. (2022). Cholinergic activity reflects reward expectations and predicts behavioral responses. iScience. 26:105814. doi: 10.1016/j.isci.2022.105814
Herry, C., Ciocchi, S., Demou, S. V., Müller, C., and Lüthi, A. (2008). Switching on and off fear by distinct neuronal circuits. Nature 454, 600–606. doi: 10.1038/nature07166
Hsieh, L. S., Wen, J. H., Miyares, L., Lombroso, P. J., and Bordey, A. (2017). Outbred CD1 mice are as suitable as inbred C57BL/6J mice in performing social tasks. Neurosci. Lett. 637, 142–147. doi: 10.1016/j.neulet.2016.11.035
Hultman, R., Mague, S. D., Li, Q., Katz, B. M., Michel, N., Lin, L., et al. (2016). Dysregulation of prefrontal cortex-mediated slow-evolving limbic dynamics drives stress-induced emotional pathology. Neuron 91, 439–452. doi: 10.1016/j.neuron.2016.05.038
Hultman, R., Ulrich, K., Sachs, B. D., Blount, C., Carlson, D. E., Ndubuizu, N., et al. (2018). Brain-wide electrical spatiotemporal dynamics encode depression vulnerability. Cell 173, 166–180. doi: 10.1016/j.cell.2018.02.012
Ibañez-Tallon, I., Miwa, J. M., Wang, H. L., Adams, N. C., Crabtree, G. W., Sine, S. M., et al. (2002). Novel modulation of neuronal nicotinic acetylcholine receptors by association with the endogenous prototoxin lynx1. Neuron 33, 893–903. doi: 10.1016/s0896-6273(02)00632-3
Jasnow, A. M., Chanjun, S., Jeris, I., Michael, D., and Huhman, K. L. (2005). Memory of social defeat is facilitated by cAMP response element-binding protein overexpression in the amygdala. Behav. Neurosci. 119, 1125–1130. doi: 10.1037/0735-7044.119.4.1125
Jasnow, A. M., Cooper, M. A., and Huhman, K. L. (2004). N-methyl-d-aspartate receptors in the amygdala are necessary for the acquisition and expression of conditioned defeat. Neuroscience 123, 625–634. doi: 10.1016/j.neuroscience.2003.10.015
Jiang, L., Kundu, S., Lederman, J. D., López-Hernández, G. Y., Ballinger, E. C., Wang, S., et al. (2016). Cholinergic signaling controls conditioned fear behaviors and enhances plasticity of cortical-amygdala circuits. Neuron 90, 1057–1070. doi: 10.1016/j.neuron.2016.04.028
Kessler, R. C., Petukhova, M., Sampson, N. A., Zaslavsky, A. M., and Wittchen, H. U. (2012). Twelve-month and lifetime prevalence and lifetime morbid risk of anxiety and mood disorders in the United States. Int. J. Methods Psychiatr. Res. 21, 169–184. doi: 10.1002/mpr.1359
Khalifeh, M., Hobeika, R., El Hayek, L., Saad, J., Eid, F., El-Khoury, R., et al. (2020). Nicotine induces resilience to chronic social defeat stress in a mouse model of water pipe tobacco exposure by activating BDNF signaling. Behav. Brain Res. 382:112499. doi: 10.1016/j.bbr.2020.112499
Kim, J., Lee, S., Park, K., Hong, I., Song, B., Son, G., et al. (2007). Amygdala depotentiation and fear extinction. Proc. Nat. Acad. Sci. U. S. A. 104, 20955–20960. doi: 10.1073/pnas.0710548105
Koo, J. W., Chaudhury, D., Han, M. H., and Nestler, E. J. (2019). Role of mesolimbic brain-derived neurotrophic factor in depression. Biol. Psychiatry 86, 738–748. doi: 10.1016/j.biopsych.2019.05.020
Koo Wook, J. W., Benoit Labonté, B., Olivia Engmann, O., Erin, S., Calipari, E. S., Barbara Juarez, B., et al. (2016). Essential Role of mesolimbic brain-derived neurotrophic factor in chronic social stress–induced depressive behaviors. Biol. Psychiatry 80, 469–478. doi: 10.1016/j.biopsych.2015.12.009
Krabbe, S., Gründemann, J., and Lüthi, A. (2018). Amygdala inhibitory circuits regulate associative fear conditioning. Biol. Psychiatry 83, 800–809. doi: 10.1016/j.biopsych.2017.10.006
Krishnan, V., Han, M. H., Graham, D. L., Berton, O., Renthal, W., Russo, S. J., et al. (2007). Molecular adaptations underlying susceptibility and resistance to social defeat in brain reward regions. Cell 131, 391–404. doi: 10.1016/j.cell.2007.09.018
Kutlu, M. G., and Gould, T. J. (2014). Acute nicotine delays extinction of contextual fear in mice. Behav. Brain Res. 263, 133–137. doi: 10.1016/j.bbr.2014.01.031
Kutlu, M. G., and Gould, T. J. (2015). Nicotine modulation of fear memories and anxiety: implications for learning and anxiety disorders. Biochem. Pharmacol. 97, 498–511. doi: 10.1016/j.bcp.2015.07.029
Lagace, D. C., Donovan, M. H., DeCarolis, N. A., Farnbauch, L. A., Malhotra, S., Berton, O., et al. (2010). Adult hippocampal neurogenesis is functionally important for stress-induced social avoidance. PNAS 107, 4436–4441. doi: 10.1073/pnas.0910072107
Letzkus, J. J., Wolff, S. B. E., Meyer, E. M., Tovote, P., Courtin, J., Herry, C., et al. (2011). A disinhibitory microcircuit for associative fear learning in the auditory cortex. Nature 480, 331–335. doi: 10.1038/nature10674
Lissek, S., Powersb, A. S., McMlurea, E. B., Phelosc, E. A., Woldehawariata, G., Frillona, C., et al. (2004). Classical fear conditioning in the anxiety disorders: a meta-analysis. Behav. Res. Ther. 43, 1391–1424. doi: 10.1016/j.brat.2004.10.007
Mallei, A., Ieraci, A., and Popoli, M. (2019). Chronic social defeat stress differentially regulates the expression of BDNF transcripts and epigenetic modifying enzymes in susceptible and resilient mice. World J. Biol. Psychiatr. Offi. Federation Soc. Biol. Psychiatry 20, 555–566. doi: 10.1080/15622975.2018.1500029
Maren, S. (2015). Out with the old and in with the new: synaptic mechanisms of extinction in the amygdala. Brain Res. 1621, 231–238. doi: 10.1016/j.brainres.2014.10.010
Markett, S., Montag, C., and Reuter, M. (2011). The nicotinic acetylcholine receptor gene CHRNA4 is associated with negative emotionality. Emotion 11, 450–455. doi: 10.1037/a0021784
Maron, E., and Nutt, D. (2017). Biological markers of generalized anxiety disorder. Dialogues Clin. Neurosci. 19, 147–158. doi: 10.31887/DCNS.2017.19.2/dnutt
McEwen, B. S. (2017). Neurobiological and systemic effects of chronic stress. Chronic Stress 1, 1–11. doi: 10.1177/2470547017692328
Meduri, J. D., Farnbauch, L. A., and Jasnow, A. M. (2013). Paradoxical enhancement of fear expression and extinction deficits in mice resilient to social defeat. Behav. Brain Res. 256, 580–590. doi: 10.1016/j.bbr.2013.09.009
Mineur, Y. S., Fote, G. M., Blakeman, S., Cahuzac, E. L. M., Newbold, S. A., and Picciotto, M. R. (2016). Multiple nicotinic acetylcholine receptor subtypes in the mouse amygdala regulate affective behaviors and response to social stress. Neuropsychopharmacology 41, 1579–1587. doi: 10.1038/npp.2015.316
Mineur, Y. S., and Picciotto, M. R. (2019). The Role of acetylcholine in negative encoding Bias: too much of a good thing? Eur. J. Neurosci. 53, 114–125. doi: 10.1111/ejn.14641
Mineur, Y. S., Soares, A. R., Etherington, I. M., Abdulla, Z. I., and Picciotto, M. R. (2023). Pathophysiology of nAChRs: Limbic circuits and related disorders. Pharmacological research, 191:106745. doi: 10.1016/j.phrs.2023.106745
Miwa, J. M., Stevens, T. R., King, S. L., Caldarone, B. J., Ibanez-Tallon, I., Xiao, C., et al. (2006). The prototoxin lynx1 acts on nicotinic acetylcholine receptors to balance neuronal activity and survival in vivo. Neuron 51, 587–600. doi: 10.1016/j.neuron.2006.07.025
Monkol, L., Karczewski, K. J., Minikel, E. V., Samocha, K. E., Banks, E., Fennell, T., et al. (2016). Analysis of protein-coding genetic variation in 60,706 humans. Nature 536, 285–291. doi: 10.1038/nature19057
Morishita, H., Miwa, J. M., Heintz, N., and Hensch, T. K. (2010). Lynx1, a cholinergic brake limits plasticity in adult visual cortex: (a cure for amblyopia through nicotinic receptor signaling). Science 330, 1238–1240. doi: 10.1126/science.1195320
Numa, C., Nagai, H., Taniguchi, M., Nagai, M., and Furuyashiki, T. (2019). (2019) social defeat stress-specific increase in c-Fos expression in the extended amygdala in mice: involvement of dopamine D1 receptor in the medial prefrontal cortex. Sci. Rep. 9:16670. doi: 10.1038/s41598-019-52997-7
O’Leary, T. P., Gunn, R. K., and Brown, R. E. (2013). What are we measuring when we test strain differences in anxiety in mice? Behav. Genet. 43, 34–50. doi: 10.1007/s10519-012-9572-8
Omori, S. A., Iwasawa, O., Saito, H., Nakajima, H., Matsuno, A., Sato, S., et al. (2024). Association of serum Ly6/PLAUR domain-containing protein 1 levels with skin sclerosis in systemic sclerosis. Sci. Rep. 14:5572. doi: 10.1038/s41598-024-56221-z
Ortiz, V., Costa Campos, R., Fofo, H., Fernandez, S. P., and Barik, J. (2022). Nicotinic receptors promote susceptibility to social stress in female mice linked with neuroadaptations within VTA dopamine neurons. Neuropsychopharmacology 47, 1587–1596. doi: 10.1038/s41386-022-01314-4
Pagliusi, M. O. F., Bonet, I. J. M., Dias, E. V., Vieira, A. S., Tambeli, C. H., Parada, C. A., et al. (2018). Social defeat stress induces hyperalgesia and increases truncated BDNF isoforms in the nucleus accumbens regardless of the depressive-like behavior induction in mice. Eur. J. Neurosci. 48, 1635–1646. doi: 10.1111/ejn.13994
Pagliusi, M. O. F., and Sartori, C. R. (2019). Social defeat stress (SDS) in mice: using Swiss mice as resident. Bio-protocol 9:e3197. doi: 10.21769/BioProtoc.3197
Pang, X., Liu, L., Ngolab, J., Zhao-Shea, R., McIntosh, J. M., Gardner, P. D., et al. (2016). Habenula cholinergic neurons regulate anxiety during nicotine withdrawal via nicotinic acetylcholine receptors. Neuropharmacology 107, 294–304. doi: 10.1016/j.neuropharm.2016.03.039
Pantoni, M. M., Herrera, G. M., Van Alstyne, K. R., and Anagnostaras, S. G. (2020). Quantifying the acoustic startle response in mice using standard digital video. Front. Behav. Neurosci. 14:83. doi: 10.3389/fnbeh.2020.00083
Picciotto, M. R. (2003). Nicotine as a modulator of behavior: beyond the inverted U. Trends Pharmacol. Sci. 24, 493–499. doi: 10.1016/S0165-6147(03)00230-X
Pisapati, A. V., Cao, W., Anderson, K. R., Jones, G., Holick, K. H., Whiteaker, P., et al. (2021). Biophysical characterization of lynx-nicotinic receptor interactions using atomic force microscopy. FASEB Bio Adva. 3, 1034–1042. doi: 10.1096/fba.2021-00012
Qi, G., Zhang, P., Li, T., Li, M., Zhang, Q., He, F., et al. (2022). NAc-VTA circuit underlies emotional stress-induced anxiety-like behavior in the three-chamber vicarious social defeat stress mouse model. Nat. Commun. 13:577. doi: 10.1038/s41467-022-28190-2
Ree, M. J., French, D., MacLeod, C., and Locke, V. (2008). State–Trait Inventory for Cognitive and Somatic Anxiety (STICSA) [Database record]. APA PsycTests. 24:1184 doi: 10.1037/t01184-000
Robinson, O. J., Pike, A. C., Cornwell, B., and Grillon, C. (2019). The translational neural circuitry of anxiety. J. Neurol. Neurosurg. Psychiatry 90:jnnp-2019-321400. doi: 10.1136/jnnp-2019-321400
Saha, R., Knapp, S., Chakraborty, D., Horovitz, O., Albrecht, A., Kriebel, M., et al. (2017). GABAergic synapses at the axon initial segment of basolateral amygdala projection neurons modulate fear extinction. Neuropsychopharmacology 42, 473–484. doi: 10.1038/npp.2016.205
Schneiderman, N., Ironson, G., and Siegel, S. (2005). Stress and health: psychological, behavioral, and biological determinants. Annu. Rev. Clin. Psychol. 1, 607–628. doi: 10.1146/annurev.clinpsy.1.102803.144141
Sherafat, Y., Chen, E., Lallai, V., Bautista, M., Fowler, J. P., Chen, Y. C., et al. (2021). Differential expression patterns of Lynx proteins and involvement of Lynx1 in Prepulse inhibition. Front. Behav. Neurosci. 15:703748. doi: 10.3389/fnbeh.2021.703748
Song, Z., Gui, S., Xiao, S., Rao, X., Cong, N., Deng, H., et al. (2024). A novel anoikis-related gene signature identifies LYPD1 as a novel therapy target for bladder cancer. Sci. Rep. 14:3198. doi: 10.1038/s41598-024-53272-0
Stein, D. J., Scott, K. M., de Jonge, P., and Kessler, R. C. (2017, 2017). Epidemiology of anxiety disorders: from surveys to nosology and back. Dialogues Clin. Neurosci. 19, 127–136. doi: 10.31887/DCNS.2017.19.2/dstein
Takahashi, A., Chung, J.-R., Zhang, S., Zhang, H., Grossman, Y., Aleyasin, H., et al. (2017). Establishment of a repeated social defeat stress model in female mice. Sci. Rep. 7:12838. doi: 10.1038/s41598-017-12811-8
Tekinay, A. B., Nong, Y., Miwa, J. M., Lieberam, I., Ibanez-Tallon, I., Greengard, P., and Heintz, N. (2009). A role for LYNX2 in anxiety-related behavior. Proc. Nat. Acad. Sci. U. S. A., 106, 4477–4482. doi: 10.1073/pnas.0813109106
Turek, F. W., Penev, P., Zhang, Y., van Reeth, O., and Zee, P. (1995). Effects of age on the circadian system. Neurosci. Biobehav. Rev. 19, 53–58. doi: 10.1016/0149-7634(94)00030-5
Wang, J., Min, S., Zhang, D., Zhang, L., Niu, C., Li, C., et al. (2023). The cation channel mechanisms of subthreshold inward depolarizing currents in the VTA dopaminergic neurons and their roles in the chronic-stress-induced depression-like behavior. Life 12:RP88319. doi: 10.7554/eLife.88319.3
Wilson, M. A., and Fadel, J. R. (2017). Cholinergic regulation of fear learning and extinction. J. Neurosci. Res. 95, 836–852. doi: 10.1002/jnr.23840
Wu, M., Puddifoot, C. A., Taylor, P., and Joiner, W. J. (2015). Mechanisms of inhibition and potentiation of α4β2 nicotinic acetylcholine receptors by members of the Ly6 protein family. J. Biol. Chem. 290, 24509–24518. doi: 10.1074/jbc.M115.647248
Zhao, T., Gao, X., and Huang, G. B. (2021). (2021) effects of chronic social defeat stress on behavior and dopamine receptors in adolescent mice with 6-Hydroxydopamine lesions of the medial prefrontal cortex. Front. Behav. Neurosci. 15:731373. doi: 10.3389/fnbeh.2021.731373
Keywords: nAChR, Lynx2, LYPD1, social interaction, anxiety behavior, nicotinic acetylcholine receptors, CSDS, defeat stress
Citation: Anderson KR, Rogu PJ, Palumbo TB and Miwa JM (2025) Abnormal response to chronic social defeat stress and fear extinction in a mouse model of Lynx2-based cholinergic dysregulation. Front. Neurosci. 19:1466166. doi: 10.3389/fnins.2025.1466166
Received: 17 July 2024; Accepted: 27 January 2025;
Published: 01 April 2025.
Edited by:
Jacob Raber, Oregon Health and Science University, United StatesReviewed by:
Hossein Aleyasin, Icahn School of Medicine at Mount Sinai, United StatesCopyright © 2025 Anderson, Rogu, Palumbo and Miwa. This is an open-access article distributed under the terms of the Creative Commons Attribution License (CC BY). The use, distribution or reproduction in other forums is permitted, provided the original author(s) and the copyright owner(s) are credited and that the original publication in this journal is cited, in accordance with accepted academic practice. No use, distribution or reproduction is permitted which does not comply with these terms.
*Correspondence: Julie M. Miwa, am1tMzEyQGxlaGlnaC5lZHU=
†Present address: Kristin R. Anderson, Department of Biological and Chemical Science, University of Galway, Galway, Ireland
Peter J. Rogu, New York Institute of Technology College of Osteopathic Medicine, Old Westbury, NY, United States
Disclaimer: All claims expressed in this article are solely those of the authors and do not necessarily represent those of their affiliated organizations, or those of the publisher, the editors and the reviewers. Any product that may be evaluated in this article or claim that may be made by its manufacturer is not guaranteed or endorsed by the publisher.
Research integrity at Frontiers
Learn more about the work of our research integrity team to safeguard the quality of each article we publish.