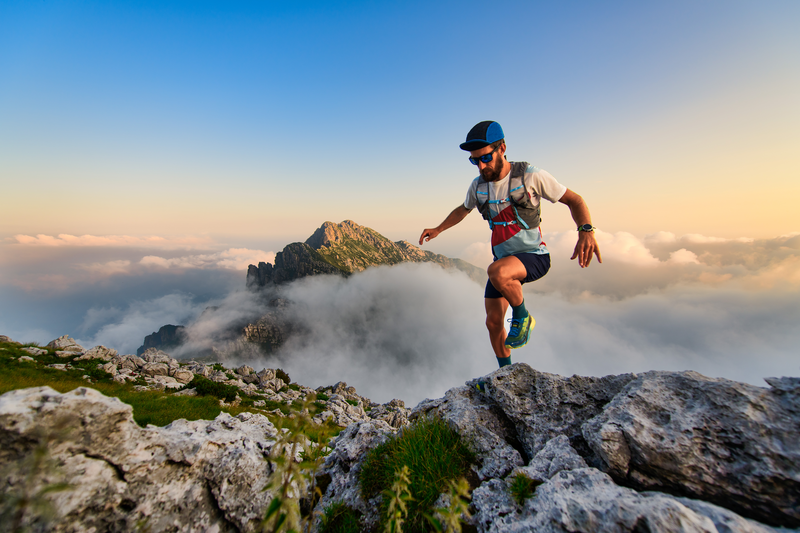
95% of researchers rate our articles as excellent or good
Learn more about the work of our research integrity team to safeguard the quality of each article we publish.
Find out more
MINI REVIEW article
Front. Neurosci. , 13 August 2024
Sec. Perception Science
Volume 18 - 2024 | https://doi.org/10.3389/fnins.2024.1459550
This article is part of the Research Topic Processing of Face and Other Animacy Cues in the Brain View all 10 articles
The ability to detect animates (as compared with inanimates) rapidly is advantageous for human survival. Due to its relevance, not only the adult human brain has evolved specific neural mechanisms to discriminate animates, but it has been proposed that selection finely tuned the human visual attention system to prioritize visual cues that signal the presence of living things. Among them, animate motion—i.e., the motion of animate entities -, is one of the most powerful cues that triggers humans’ attention. From a developmental point of view, whether such specialization is inborn or acquired through experience is a fascinating research topic. This mini-review aims to summarize and discuss recent behavioral and electrophysiological research that suggests that animate motion has an attentional advantage in the first year of life starting from birth. Specifically, the rationale underlying this paper concerns how attention deployment is affected by animate motion conveyed both by the movement of a single dot and, also, when the single dot is embedded in a complex array, named biological motion. Overall, it will highlight the importance of both inborn predispositions to pay attention preferentially to animate motion, mainly supported by subcortical structures, and the exposure to certain experiences, shortly after birth, to drive the cortical attentional visual system to become the way it is in adults.
To identify conspecifics and to differentiate them from other kinds of entities is of paramount adaptive value, because they feed us, interact (or at least they should) successfully with us, love us, and help us to hand down our genetic endowment (Bonatti et al., 2002). From an evolutionary point of view, it is plausible to hypothesize that we have inherited ancestrally-derived and highly conserved mechanisms, shared with other species (i.e., chicks, non-human primates), deputed to detect biologically relevant entities, such as prey, predators, and social companions. Indeed, it has been suggested that the human visual system is evolved to pay attention and to prioritize animates on the basis of some pictorial cues, such as the presence of a face, eye-gaze, and body structure. The way they move is another cue that attracts humans and non-humans’ visual attention toward animate entities.
Motion of living beings, here referred to “life motion” as conceptualized by Troje (2013), which includes any kind of visual stimulus that elicits the percept of something being alive, has specific features that are not shared with motion of inanimate objects, such as the capability to start from rest or to change speed and trajectory without external forces (i.e., self-propulsion). In Troje’s taxonomy, life motion is further differentiated between extrinsic and intrinsic motion. Extrinsic motion refers to animate motion perception elicited by motion of single dot or geometrical shapes (Heider and Simmel, 1944; Carey, 2009; Rutherford and Kuhlmeier, 2013; Scholl and Gao, 2013), whereas intrinsic motion refers to biological motion (BM) perception (Johansson, 1973).
Neuroscientific evidence and neuropsychological lesion studies (Battelli et al., 2003; Gilaie-Dotan et al., 2011) suggested that in the adult brain life motion detection is subserved by relatively specific set of both subcortical and cortical areas within the so called Social Neural Network (SNN) (Caramazza and Shelton, 1998; Blakemore et al., 2003; Schultz et al., 2005; Adolphs, 2009; Frith and Frith, 2010; Santos et al., 2010; Stosic et al., 2014). Not only, but it has been proposed that selection finely tuned the human visual attention system to prioritize visual cues that signal the presence of living things (New et al., 2007). Further, SNN atypicalities lead to impairments in animate (Rutherford et al., 2006) and BM motion perception (Federici et al., 2020) observed in Autism Spectrum Disorders (ASD, Sato and Uono, 2019).
Ontogenetically, whether such a level of neural and functional specialization for life motion detection found in adults is present from birth or is the result of developmental processes, is still an open question. Several behavioral studies demonstrated in human newborns the presence of inborn attentional predispositions toward visual cues of motion that trigger both animate and BM perception in adults (Simion et al., 2008; Bardi et al., 2011, 2015; Bidet-Ildei et al., 2014; Di Giorgio et al., 2017, 2021a). However, the missing piece of the puzzle concerns the experience-dependent or experience-independent nature of the mechanisms underlying such attentional biases, as well as their neural bases.
Starting from the evidence of the presence of specialized brain and attention systems in human adults, here we sought to overview and discuss the current state of the art on (i) the role of attentional predispositions in triggering visual attention toward both extrinsic (animate) and intrinsic (biological) motion at birth and in the first months of life, and (ii) the experience-independent or experience-dependent nature of the underpinning mechanisms thought to be involved in such attentional advantage.
Adults perceive animacy based on some basic visual cues of motion, such as self-propulsion, movement speed, contingency (Tremoulet and Feldman, 2000; Santos et al., 2008). Neuroimaging studies have revealed that the adult brain is equipped with a highly specialized neural network, the SNN, devoted to process such animate motion (Santos et al., 2010), that includes both subcortical—i.e., insula and amygdala—and cortical structures—i.e., the ventromedial prefrontal cortex (vmPFC), the superior temporal gyrus (STG) and sulcus (STS), the fusiform gyrus (FG) (Blakemore et al., 2003; Van Overwalle, 2009; Frith and Frith, 2010; Grill-Spector and Weiner, 2014). Similarly to what we known about non-human primates (Oram and Perrett, 1994; Tomonaga, 2001; Jellema et al., 2004; Jastorff et al., 2012), converging brain structural and connectivity evidence suggests that STS plays a key role in extrinsic and intrinsic motion processing (Schultz et al., 2005; Pavlova et al., 2006; Wheatley et al., 2007; Gobbini et al., 2011; Herrington et al., 2011; Pavlova, 2012; Sokolov et al., 2012; Yokoyama et al., 2021).
This brain specialization is coupled, at the behavioral and functional level, with an attentional advantage for stimuli that are characterized by animate motion and BM. According to the animate-monitoring hypothesis (New et al., 2007), humans’ visual attentional system has evolved to prioritize and monitor visual cues central to our survival. Despite some studies that have questioned this hypothesis (Hagen and Laeng, 2016; Cox et al., 2022), it has been shown that animates were detected more quickly and accurately, rather than inanimates, in different attentional tasks, such as attentional blink (Calvillo and Hawkins, 2016; Guerrero and Calvillo, 2016), inattentional blindness (Calvillo and Jackson, 2014), change blindness (New et al., 2007; Altman et al., 2016), and visual search (Abrams and Christ, 2003; Pratt et al., 2010).
Likewise, some evidence suggested that a similar attentional advantage is present also for BM (Chandler-Mather et al., 2020). BM elicits faster saccadic reaction (Bardi et al., 2015) and more accurate responses (Shi et al., 2010; Hirai et al., 2011) in a cueing paradigm when compared with other motion types, and it can be processed incidentally in a flanker task (Thornton and Vuong, 2004). Based on this and other results, some authors postulated the presence of an inborn mechanism sensitive to BM, namely perceptual life detector (Johnson, 2006; Troje and Westhoff, 2006).
From a developmental perspective, it is plausible to hypothesize that animate and BM perception has an early ontogenetic origin because in adults it is based on fast and automatic visual processing, which is mainly constrained by a collection of low-level visual motion cues. Whether similar attentional advantages found in adults for animate and BM are present at birth, as well as the experience-dependent or experience-independent nature of perceptual mechanisms postulated at the basis of it, are relevant and intriguing open questions.
Controversy still exists about the origin of animate motion perception (Biro and Leslie, 2007; Rakison et al., 2008). Some authors have hypothesized that infants come into the world equipped with inborn domain-specific mechanisms (Leslie, 1995), otherwise referred as core-knowledge (Spelke and Kinzler, 2007), that allow them to be sensitive to visual cues of motion that characterize animates. Others suggested that domain-general inborn predispositions to such visual cues of motion might be a sort of building blocks to the development of animacy perception through learning mechanisms (cue-based bootstrapping model, Biro and Leslie, 2007).
To disentangle the issue, developmental studies have mainly focused on few-month-old infants’ ability to attribute goals, intentions and emotions based on the objects’ motion (Poulin-Dubois et al., 1996; Luo and Baillargeon, 2005; Biro et al., 2007; Luo et al., 2009; Mahajan and Woodward, 2009), named intentional agency (Carey, 2009). However, on which visual cues infants used to infer such an abstract concept from early on, that is animate motion perception, is much a relatively unexplored topic of research.
At the behavioral level, some studies suggested that animate motion captures infants’ visual attention. For instance, Träuble et al. (2014) showed that speed and direction changes by self-propulsion induce animacy perception in 7-month-old infants. Infants’ visual attention was not only attracted by single object events, but also by objects that move contingently as in the case of chasing events. It has been demonstrated that 5-and 12-month-old infants orient their attention to and attend preferentially toward the agent that initiates an interaction within a chasing event (Galazka and Nyström, 2016). Frankenhuis et al. (2013) demonstrated an attentional bias for chasing events based on visual cues of motion (acceleration, high turning rates, and attraction) and their combination (chasing) in 4-and 10-month-old infants. Further, using a change-detection paradigm, it has been shown that 11-month-old infants are able to detect changes faster to animate compared to inanimate stimuli (Hofrichter et al., 2021).
This behavioral evidence is further corroborated by the results obtained in an ERP study with infants of 9 months. By this age infants allocate more attentional resources to an object moving inanimately than an animate object as evidenced by the increased negativity in the fronto-central Nc component, a component that indexes general attentional arousal, demonstrating differential sensitivity to animate and inanimate motion (Kaduk et al., 2013).
All these studies concern infants of a few months with a certain degree of visual and social experience, leaving the question about the origin of such perception open. To the best of our knowledge, only two recent studies demonstrated newborns sensitive toward some low-level visual cues of motion that trigger animacy perception in adults, which are self-propulsion and speed changes without external forces. In a series of visual preference experiments, 2-day-old newborns orient their attention preferentially toward an object that started from rest (Di Giorgio et al., 2017), and changed its speed without external forces (Di Giorgio et al., 2021a), compared to an object that did not start to move or change speed by its own. Authors interpreted the presence of such inborn attentional biases as a sort of bootstrapping point to the development of animacy and agency perception found later during development, corroborating the cue-based bootstrapping model (Biro and Leslie, 2007), which emphasizes the interplay between such inborn predispositions and the role of experience and learning mechanisms during development.
As for other visual cues that indexed the presence of animate beings (i.e., faces and BM), these attentional biases are thought to be mainly guided by subcortical neural structures (the superior colliculi) which appear to contribute to the specialization of the brain cortical circuits that, during development, carry out more sophisticated social information processing. Broadly speaking, such inborn experience-independent biases not only cause newborns to orient reflexively their attention toward animate beings, but they may serve to bias voluntary cortical-mediated visual attention toward relevant stimuli over the first weeks and months of life to promote the typical progressive specialization of the SNN (Johnson, 2005; Johnson et al., 2015). It has been suggested that such a shift from reflexive-to-cortical-mediated visual mechanisms could be altered in ASD (Di Giorgio et al., 2021b), leading to a cascading effects on animate and BM perception atypicalities (Rutherford et al., 2006).
While recent findings on the currently elective animal model (domestic chicks) is very promising in shedding new light on neural and physiological mechanisms underlying inborn attentional biases (Lorenzi et al., 2017; Mayer et al., 2019; Rosa-Salva et al., 2021), in human newborns the neural signature of such biases remains almost speculative (see Buiatti et al., 2019).
As shown so far, infants’ and newborns’ attention are triggered by animate motion conveyed by the movement of a single dot. Life motion detection may also be driven by more specific types of motions cues, such as when the relative motion of many dots in a complex point light display (PLD) are organized and perceived as a particular form of vertebrates’ motion such as a human walker (Johansson, 1973; Scholl and Gao, 2013). In a complex array of dots, each single dot has self-propelled motion and follows the acceleration and deceleration motion pattern. When these cues of motion are applied to dots that composed complex arrays as in the case of BM, adults are able to extract, simply analyzing motion, a lot of social information.
Historically, the first study that investigates sensitivity toward BM during development, through use of PLD, was done by Fox and McDaniel (1982) testing 4-and 6-month-old infants. Starting from this first evidence, a growing number of studies tried to understand the origin of the sensitivity to BM.
Following the evidence coming from newly hatched chicks that demonstrates visual preference for BM rather than other motion types in this species (Vallortigara et al., 2005), Simion et al. (2008) investigates whether also in human newborn this attentional bias was present. In a series of experiments, it has been shown that that even 3-day-old human newborns have a predisposition to selectively orient their visual attention toward BM when compared with other motion types (random or rigid motion, Simion et al., 2008; Bardi et al., 2011, 2015; Bidet-Ildei et al., 2014). These results seem to support the hypothesis of the presence of a perceptual life detector which allows newborns of different species to orient preferentially their visual attention toward the local information (i.e., the motion of the limbs of an animal in locomotion) vehiculated by BM (Johnson, 2006; Troje and Westhoff, 2006). As for the inborn biases for animate motion, it has been hypothesized that attentional biases for BM at birth should be considered as a predisposed and experience-independent perceptual mechanism supported by subcortical structures (Simion et al., 2008; Chang et al., 2018).
Based on this assumption, several authors tried to better define the developmental trajectory of the sensitivity toward BM. In a series of studies, Bertenthal et al. (1984, 1987), investigated infants’ ability to organize complex arrays of dots as a coherent figure. Five-month-old infants are able to discriminate PLD walker from the same stimulus with scramble spatial relationship or with perturbed local rigidity between joints, suggesting the emergence of sensitivity toward global information level of BM. This suggests that the visual system at birth can use visual information to prefer and discriminate between coherent displays but not to integrate the individual motion information to form the global percept of a PLD. The ability to process configural relations in BM must involve, therefore, a more specialized, late-developing, higher-level processing; hence requiring more visual experience and cortical maturation (Hirai and Senju, 2020).
This evidence, coupled with the ones by Fox and McDaniel (1982), supports the hypothesis that the second mechanism that would be responsible in recognizing and identifying agents based on configural information would not be inborn, but rather an experience-dependent mechanism that becomes functioning during development (Troje and Westhoff, 2006). The extraction of relevant social information would be supported by this second mechanism (Chang and Troje, 2009), that might be used to pay voluntary attention and to interpret other intentions.
From a neural point of view, fNIRS studies showed that the selective activation of rSTS for BM processing emerges around 7–8 months (Lisboa et al., 2020a,b). The same pattern of results was found also in a neurophysiological study with 8-month-infants, where a modulation of ERP component peaking around 200–300 ms post-stimulus onset have been observed only in the right hemisphere (Reid et al., 2006). More recently, Hirai and Hakuno (2022) demonstrated that even at 6 months of age infants are able to extract a coherent human figure from PLDs, showing a larger ERP component for BM rather than scrambled motion, peaking around 500–600 ms post-stimulus onset.
Starting from this state of the art, research has attempted to investigate the functional meaning of BM walking direction processing, contemporarily probing the hypothesis according to which the walking direction of legged vertebrates may produce an attentional advantage in processing relevant information (Bardi et al., 2015; Lunghi et al., 2019, 2020). In these studies, authors tested 3-and 6-months-old infants using a cueing paradigm (Posner, 1980) with BM as central cue. Behaviorally, shorter saccadic reaction time for the target that appears in congruent position to the walking direction of BM was found. At the neural level, 3-and 6-month-old infants showed a modulation of ERPs sensory component (P1) for congruent targets even before the oculomotor response, suggesting that already at 3 months BM elicits a covert orienting of attention toward relevant information (Lunghi et al., 2019).
Overall, these findings suggest that the inborn sensitivity to BM, supported by an experience-independent mechanism, should be considered as a bootstrapping point to the development of the ability of infants and adults to extract social information from motion. Behavioral results showed atypical visual orienting toward BM stimuli in newborns and 4-month-old infants at high-risk for ASD (Di Giorgio et al., 2016, 2021b), corroborating the idea of the alteration to such mechanisms early in life.
The studies reviewed here seem to corroborate the hypothesis that life motion that characterizes living beings modulates visual attention starting from birth. Humans and non-human newborns (Mascalzoni et al., 2010; Rosa-Salva et al., 2016; Lemaire et al., 2022; Rosa-Salva et al., 2023) orient their visual attention reflexively toward visual cues of motion that characterized animate motion and BM since birth.
As proposed by Frankenhuis and Barrett (2013), it is plausible that starting from birth, infants use a “coarse attentional filter that navigates their attention toward social interactions.” Such a filter is shared with other vertebrates, therefore phylogenetically old, and it is sensitive to general properties of both animate motion and BM regardless of their content. Only later during development, infants are able to infer the social meaning conveyed by such visual cues of motion, thanks to more sophisticated cognitive (and cortical-guided) mechanisms dedicated to comprehending goals and intentions.
Recent groundbreaking studies are telling us that for understanding how cognition originates and develops, and which is the role of experience, some answers can be found before birth, in fetuses (Reid, 2024). Fetuses show impressive perceptual capabilities in visual (Reid et al., 2017; Donovan et al., 2020), auditory (Vogelsang et al., 2023), and motor domains (Zoia et al., 2007), making them a promising model for future studies on the origin of knowledge.
If the presence of such bootstrapping inborn attentional biases is not questioned, the neural signatures underlying such biases remain unexplored. For instance, albeit based on indirect evidence in human newborns, such inborn attentional biases are thought to be mainly guided by a rapid and reflexive subcortical orienting mechanisms, and that, around 2–3 months of life, are being replaced by voluntary cortical-mediated visual attention mechanisms (Johnson, 2005). In line with the recent findings about the neural substrates underlying face detection in human newborns (Buiatti et al., 2019), future studies, taking advantage from non-invasive methods such as EEG or fNIRS, should investigate which are the neural pathways involved in animate and BM perception, mainly unexplored at birth as well as in the first years of life.
Finally, the study of the neural bases underlying animate and BM perception is important in typical-developing populations. But it is even more important in ASD high-risk populations, where the need for noninvasive and sensitive biomarkers reflecting altered key functional circuits is urgent. This would corroborate studies, currently controversial (Dawson et al., 2023), that suggest altered inborn attentional predispositions as potential predictive risk factors for ASD.
ML: Writing – original draft, Writing – review & editing. EDG: Writing – original draft, Writing – review & editing.
The author(s) declare that financial support was received for the research, authorship, and/or publication of this article. Open Access funding provided by Università degli Studi di Padova | University of Padua, Open Science Committee.
The authors declare that the research was conducted in the absence of any commercial or financial relationships that could be construed as a potential conflict of interest.
All claims expressed in this article are solely those of the authors and do not necessarily represent those of their affiliated organizations, or those of the publisher, the editors and the reviewers. Any product that may be evaluated in this article, or claim that may be made by its manufacturer, is not guaranteed or endorsed by the publisher.
Abrams, R. A., and Christ, S. E. (2003). Motion onset captures attention. Psychol. Sci. 14, 427–432. doi: 10.1111/1467-9280.01458
Adolphs, R. (2009). The social brain: neural basis of social knowledge. Annu. Rev. Psychol. 60, 693–716. doi: 10.1146/annurev.psych.60.110707.163514
Altman, M. N., Khislavsky, A. L., Coverdale, M. E., and Gilger, J. W. (2016). Adaptive attention: how preference for animacy impacts change detection. Evol. Hum. Behav. 37, 303–314. doi: 10.1016/j.evolhumbehav.2016.01.006
Bardi, L., Di Giorgio, E., Lunghi, M., Troje, N. F., and Simion, F. (2015). Walking direction triggers visuo-spatial orienting in 6-month-old infants and adults: an eye tracking study. Cognition 141, 112–120. doi: 10.1016/j.cognition.2015.04.014
Bardi, L., Regolin, L., and Simion, F. (2011). Biological motion preference in humans at birth: role of dynamic and configural properties. Dev. Sci. 14, 353–359. doi: 10.1111/j.1467-7687.2010.00985.x
Battelli, L., Cavanagh, P., and Thornton, I. M. (2003). Perception of biological motion in parietal patients. Neuropsychologia 41, 1808–1816. doi: 10.1016/S0028-3932(03)00182-9
Bertenthal, B. I., Proffitt, D. R., and Cutting, J. E. (1984). Infant sensitivity to figural coherence in biomechanical motions. J. Exp. Child Psychol. 37, 213–230. doi: 10.1016/0022-0965(84)90001-8
Bertenthal, B. I., Proffitt, D. R., and Kramer, S. J. (1987). Perception of biomechanical motions by infants: implementation of various processing constraints. J. Exp. Psychol. Hum. Perc. Perform 13, 577–585. doi: 10.1037/0096-1523.13.4.577
Bidet-Ildei, C., Kitromilides, E., Orliaguet, J. P., Pavlova, M., and Gentaz, E. (2014). Preference for point-light human biological motion in newborns: contribution of translational displacement. Dev. Psychol. 50, 113–120. doi: 10.1037/a0032956
Biro, S., and Leslie, A. M. (2007). Infants’ perception of goal-directed actions: development through cue-based bootstrapping. Dev. Sci. 10, 379–398. doi: 10.1111/j.1467-7687.2006.00544.x
Biro, S., Csibra, G., and Gergely, G. (2007). The role of behavioral cues in understanding goal-directed actions in infancy. Progr. Brain Res. 164, 303–322. doi: 10.1016/S0079-6123(07)64017-5
Blakemore, S. J., Boyer, P., Pachot-Clouard, M., Meltzoff, A., Segebarth, C., and Decety, J. (2003). The detection of contingency and animacy from simple animations in the human brain. Cerebr. Cortex 13, 837–844. doi: 10.1093/cercor/13.8.837
Bonatti, L., Frot, E., Zangl, R., and Mehler, J. (2002). The human first hypothesis: identification of conspecifics and individuation of objects in the young infant. Cogn. Psychol. 44, 388–426. doi: 10.1006/cogp.2002.0779
Buiatti, M., Di Giorgio, E., Piazza, M., Polloni, C., Menna, G., Taddei, F., et al. (2019). Cortical route for facelike pattern processing in human newborns. PNAS 116, 4625–4630. doi: 10.1073/pnas.1812419116
Calvillo, D. P., and Hawkins, W. C. (2016). Animate objects are detected more frequently than inanimate objects in inattentional blindness tasks independently of threat. J. Gen. Psychol. 143, 101–115. doi: 10.1080/00221309.2016.1163249
Calvillo, D. P., and Jackson, R. E. (2014). Animacy, perceptual load, and in attentional blindness. Psychon. Bull. Rev. 21, 670–675. doi: 10.3758/s13423-013-0543-8
Caramazza, A., and Shelton, J. (1998). Domain-specific knowledge systems in the brain: the animate–inanimate distinction. J. Cog. Neurosci. 10, 1–34. doi: 10.1162/089892998563752
Chandler-Mather, N., Welsh, T., Sparks, S., and Kritikos, A. (2020). Biological motion and animacy belief induce similar effects on involuntary shifts of attention. Atten. Percept. Psychophys. 82, 1099–1111. doi: 10.3758/s13414-019-01843-z
Chang, D. H., and Troje, N. F. (2009). Characterizing global and local mechanisms in biological motion perception. J. Vis. 9:8. doi: 10.1167/9.5.8
Chang, D. H., Ban, H., Ikegaya, Y., Fujita, I., and Troje, N. F. (2018). Cortical and subcortical responses to biological motion. NeuroImage 174, 87–96. doi: 10.1016/j.neuroimage.2018.03.013
Cox, J. A., Cox, T. W., and Aimola Davies, A. M. (2022). Are animates special? Exploring the effects of selective attention and animacy on visual statistical learning. Q. J. Exp. Psychol. 75, 1746–1762. doi: 10.1177/17470218221074686
Dawson, G., Rieder, A. D., and Johnson, M. H. (2023). Prediction of autism in infants: progress and challenges. Lancet Neurol. 22, 244–254. doi: 10.1016/S1474-4422(22)00407-0
Di Giorgio, E., Frasnelli, E., Rosa Salva, O., Luisa Scattoni, M., Puopolo, M., Tosoni, D., et al. (2016). Difference in visual social predispositions between newborns at low-and high-risk for autism. Sci. Rep. 6:26395. doi: 10.1038/srep26395
Di Giorgio, E., Lunghi, M., Simion, F., and Vallortigara, G. (2017). Visual cues of motion that trigger animacy perception at birth: the case of self-propulsion. Dev. Sci. 20:e12394. doi: 10.1111/desc.12394
Di Giorgio, E., Lunghi, M., Vallortigara, G., and Simion, F. (2021a). Newborns’ sensitivity to speed changes as a building block for animacy perception. Sci. Rep. 11:542. doi: 10.1038/s41598-020-79451-3
Di Giorgio, E., Rosa-Salva, O., Frasnelli, E., Calcagnì, A., Lunghi, M., Scattoni, M. L., et al. (2021b). Abnormal visual attention to simple social stimuli in 4-month-old infants at high risk for autism. Sci. Rep. 11:15785. doi: 10.1038/s41598-021-95418-4
Donovan, T., Dunn, K., Penman, A., Young, R. J., and Reid, V. M. (2020). Fetal eye movements in response to a visual stimulus. Brain Behav. 10:e01676. doi: 10.1002/brb3.1676
Federici, A., Parma, V., Vicovaro, M., Radassao, L., Casartelli, L., and Ronconi, L. (2020). Anomalous perception of biological motion in autism: a conceptual review and meta-analysis. Sci. Rep. 10:4576. doi: 10.1038/s41598-020-61252-3
Fox, R., and McDaniel, C. (1982). The perception of biological motion by human infants. Science 218, 486–487. doi: 10.1126/science.7123249
Frankenhuis, W. E., and Barrett, H. C. (2013). “Design for learning: the case of chasing” in Social perception: Detection and interpretation of animacy, agency, and intention. eds. M. D. Rutherford and V. A. Kuhlmeier (Cambridge, MA: MIT Press), 171–195.
Frankenhuis, W. E., House, B., Barrett, H. C., and Johnson, S. P. (2013). Infants’ perception of chasing. Cognition 126, 224–233. doi: 10.1016/j.cognition.2012.10.001
Frith, U., and Frith, C. (2010). The social brain: allowing humans to boldly go where no other species has been. Philos. Trans. R Soc. B. Biol. Sci. 365, 165–176. doi: 10.1098/rstb.2009.0160
Galazka, M., and Nyström, P. (2016). Infants’ preference for individual agents within chasing interactions. J. Exp. Child Psychol. 147, 53–70. doi: 10.1016/j.jecp.2016.02.010
Gilaie-Dotan, S., Bentin, S., Harel, M., Rees, G., and Saygin, A. P. (2011). Normal form from biological motion despite impaired ventral stream function. Neuropsychologia 49, 1033–1043. doi: 10.1016/j.neuropsychologia.2011.01.009
Gobbini, M. I., Gentili, C., Ricciardi, E., Bellucci, C., Salvini, P., Laschi, C., et al. (2011). Distinct neural systems involved in agency and animacy detection. J. Cogn. Neurosci. 23, 1911–1920. doi: 10.1162/jocn.2010.21574
Grill-Spector, K., and Weiner, K. S. (2014). The functional architecture of the ventral temporal cortex and its role in categorization. Nat. Rev. Neurosci. 15, 536–548. doi: 10.1038/nrn3747
Guerrero, G., and Calvillo, D. P. (2016). Animacy increases second target reporting in a rapid serial visual presentation task. Psychon. Bull. Rev. 23, 1832–1838. doi: 10.3758/s13423-016-1040-7
Hagen, T., and Laeng, B. (2016). The change detection advantage for animals: an effect of ancestral priorities or progeny of experimental design? i-Perception 7:2041669516651366. doi: 10.1177/2041669516651366
Heider, F., and Simmel, M. (1944). An experimental study of apparent behavior. Am. J. Psychol. 57, 243–259. doi: 10.2307/1416950
Herrington, J. D., Nymberg, C., and Schultz, R. T. (2011). Biological motion task performance predicts superior temporal sulcus activity. Brain Cog. 77, 372–381. doi: 10.1016/j.bandc.2011.09.001
Hirai, M., and Hakuno, Y. (2022). Electrophysiological evidence of global structure-from-motion processing of biological motion in 6-month-old infants. Neuropsychologia 170:108229. doi: 10.1016/j.neuropsychologia.2022.108229
Hirai, M., and Senju, A. (2020). The two-process theory of biological motion processing. Neurosci. Biobehav. Rev. 111, 114–124. doi: 10.1016/j.neubiorev.2020.01.010
Hirai, M., Saunders, D. R., and Troje, N. F. (2011). Allocation of attention to biological motion: local motion dominates global shape. J. Vis. 11:4. doi: 10.1167/11.3.4
Hofrichter, R., Siddiqui, H., Morrisey, M. N., and Rutherford, M. D. (2021). Early attention to animacy: change-detection in 11-month-olds. Evol. Psychol. 19:147470492110282. doi: 10.1177/14747049211028220
Jastorff, J., Popivanov, I. D., Vogels, R., Vanduffel, W., and Orban, G. A. (2012). Integration of shape and motion cues in biological motion processing in the monkey STS. NeuroImage 60, 911–921. doi: 10.1016/j.neuroimage.2011.12.087
Jellema, T., Maassen, G., and Perrett, D. I. (2004). Single cell integration of animate form, motion and location in the superior temporal cortex of the macaque monkey. Cereb. Cortex 14, 781–790. doi: 10.1093/cercor/bhh038
Johansson, G. (1973). Visual perception of biological motion and a model for its analysis. Percept. Psychophys. 14, 201–211. doi: 10.3758/BF03212378
Johnson, M. H. (2005). Subcortical face processing. Nat. Rev. Neurosci. 6, 766–774. doi: 10.1038/nrn1766
Johnson, M. H. (2006). Biological motion: a perceptual life detector? Curr. Bio. 16, R376–R377. doi: 10.1016/j.cub.2006.04.008
Johnson, M. H., Senju, A., and Tomalski, P. (2015). The two-process theory of face processing: modifications based on two decades of data from infants and adults. Neurosci. Biobehav. Rev. 50, 169–179. doi: 10.1016/j.neubiorev.2014.10.009
Kaduk, K., Elsner, B., and Reid, V. M. (2013). Discrimination of animate and inanimate motion in 9-month-old infants: an ERP study. Dev. Cogn. Neurosci. 6, 14–22. doi: 10.1016/j.dcn.2013.05.003
Lemaire, B. S., Rosa-Salva, O., Fraja, M., Lorenzi, E., and Vallortigara, G. (2022). Spontaneous preference for unpredictability in the temporal contingencies between agents' motion in naive domestic chicks. Proc. R. Soc. B 289:20221622. doi: 10.1098/rspb.2022.1622
Leslie, A. M. (1995). “A theory of agency” in Causal cognition: a multidisciplinary debate. eds. D. Sperber, D. Premack, and A. J. Premack (Oxford: Clarendon Press), 121–141.
Lisboa, I. C., Miguel, H., Sampaio, A., Mouta, S., Santos, J. A., and Pereira, A. F. (2020b). Right STS responses to biological motion in infancy–an fNIRS study using point-light walkers. Neuropsychologia 149:107668. doi: 10.1016/j.neuropsychologia.2020.107668
Lisboa, I. C., Queirós, S., Miguel, H., Sampaio, A., Santos, J. A., and Pereira, A. F. (2020a). Infants’ cortical processing of biological motion configuration–a fNIRS study. Infant Behav. Dev. 60:101450. doi: 10.1016/j.infbeh.2020.101450
Lorenzi, E., Mayer, U., Rosa-Salva, O., and Vallortigara, G. (2017). Dynamic features of animate motion activate septal and preoptic areas in visually naïve chicks (Gallus gallus). Neuroscience 354, 54–68. doi: 10.1016/j.neuroscience.2017.04.022
Lunghi, M., Di Giorgio, E., Benavides-Varela, S., and Simion, F. (2020). Covert orienting of attention in 3-month-old infants: the case of biological motion. Infant Behav. Dev. 58:101422. doi: 10.1016/j.infbeh.2020.101422
Lunghi, M., Piccardi, E. S., Richards, J. E., and Simion, F. (2019). The neural correlates of orienting to walking direction in 6-month-old infants: an ERP study. Dev. Sci. 22:e12811. doi: 10.1111/desc.12811
Luo, Y., and Baillargeon, R. (2005). Can a self-propelled box have a goal? Psychological reasoning in 5-month-old infants. Psychol. Sci. 16, 601–608. doi: 10.1111/j.1467-9280.2005.01582.x
Luo, Y., Kaufman, L., and Baillargeon, R. (2009). Young infants’ reasoning about physical events involving inert and self-propelled objects. Cogn. Psychol. 58, 441–486. doi: 10.1016/j.cogpsych.2008.11.001
Mahajan, N., and Woodward, A. L. (2009). Seven-month-old infants selectively reproduce the goals of animate but not inanimate agents. Infancy 14, 667–679. doi: 10.1080/15250000903265184
Mascalzoni, E., Regolin, L., and Vallortigara, G. (2010). Innate sensitivity for self-propelled causal agency in newly hatched chicks. PNAS 107, 4483–4485. doi: 10.1073/pnas.0908792107
Mayer, U., Rosa-Salva, O., Loveland, J. L., and Vallortigara, G. (2019). Selective response of the nucleus taeniae of the amygdala to a naturalistic social stimulus in visually naive domestic chicks. Sci. Rep. 9:9849. doi: 10.1038/s41598-019-46322-5
New, J., Cosmides, L., and Tooby, J. (2007). Category-specific attention for animals reflects ancestral priorities, not expertise. PNAS 104, 16598–16603. doi: 10.1073/pnas.0703913104
Oram, M. W., and Perrett, D. I. (1994). Responses of anterior superior temporal polysensory (STPa) neurons to ‘biological motion’ stimuli. J. Cogn. Neurosci. 6, 99–116. doi: 10.1162/jocn.1994.6.2.99
Pavlova, M. A. (2012). Biological motion processing as a hallmark of social cognition. Cereb. Cortex 22, 981–995. doi: 10.1093/cercor/bhr156
Pavlova, M., Birbaumer, N., and Sokolov, A. (2006). Attentional modulation of cortical neuromagnetic gamma response to biological movement. Cereb. Cortex 16, 321–327. doi: 10.1093/cercor/bhi108
Posner, M. I. (1980). Orienting of attention. Q. J. Exp. Psychol. 32, 3–25. doi: 10.1080/00335558008248231
Poulin-Dubois, D., Lepage, A., and Ferland, D. (1996). Infants' concept of animacy. Cogn. Dev. 11, 19–36. doi: 10.1016/S0885-2014(96)90026-X
Pratt, J., Radulescu, P. V., Guo, R. M., and Abrams, R. A. (2010). It’s alive! Animate motion captures visual attention. Psychol. Sci. 21, 1724–1730. doi: 10.1177/0956797610387440
Rakison, D. H., Lupyan, G., Oakes, L. M., and Walker-Andrews, A. S. (2008). Developing object concepts in infancy: an associative learning perspective. Monogr. Soc. Res. Child Dev. 1, 1–127.
Reid, V. M. (2024). The key to understanding core knowledge resides in the fetus. Behav. Brain Sci. 47:e139. doi: 10.1017/S0140525X23003175
Reid, V. M., Dunn, K., Young, R. J., Amu, J., Donovan, T., and Reissland, N. (2017). The human fetus preferentially engages with face-like stimuli. Curr. Bio. 27, 1825–1828. doi: 10.1016/j.cub.2017.05.044
Reid, V. M., Hoehl, S., and Striano, T. (2006). The perception of biological motion by infants: an event-related potential study. Neurosci. Lett. 395, 211–214. doi: 10.1016/j.neulet.2005.10.080
Rosa-Salva, O., Grassi, M., Lorenzi, E., Regolin, L., and Vallortigara, G. (2016). Spontaneous preference for visual cues of animacy in naïve domestic chicks: the case of speed changes. Cognition 157, 49–60. doi: 10.1016/j.cognition.2016.08.014
Rosa-Salva, O., Hernik, M., Fabbroni, M., Lorenzi, E., and Vallortigara, G. (2023). Naïve chicks do not prefer objects with stable body orientation, though they may prefer behavioural variability. Anim. Cogn. 26, 1177–1189. doi: 10.1007/s10071-023-01764-3
Rosa-Salva, O., Mayer, U., Versace, E., Hébert, M., Lemaire, B. S., and Vallortigara, G. (2021). Sensitive periods for social development: interactions between predisposed and learned mechanisms. Cognition 213:104552. doi: 10.1016/j.cognition.2020.104552
Rutherford, M. D., and Kuhlmeier, V. A. (2013). Social perception: detection and interpretation of animacy, agency, and intention. Cambridge, MA: MIT Press.
Rutherford, M. D., Pennington, B. F., and Rogers, S. J. (2006). The perception of animacy in young children with autism. J. Autism Dev. Disord. 36, 983–992. doi: 10.1007/s10803-006-0136-8
Santos, N. S., Kuzmanovic, B., David, N., Rotarska-Jagiela, A., Eickhoff, S. B., Shah, J. N., et al. (2010). Animated brain: a functional neuroimaging study on animacy experience. NeuroImage 53, 291–302. doi: 10.1016/j.neuroimage.2010.05.080
Santos, N. S., David, N., Bente, G., and Vogeley, K. (2008). Parametric induction of animacy experience. Conscious. Cogn. 17, 425–437. doi: 10.1016/j.concog.2008.03.012
Sato, W., and Uono, S. (2019). The atypical social brain network in autism: advances in structural and functional MRI studies. Curr. Opin. Neurol. 32, 617–621. doi: 10.1097/WCO.0000000000000713
Scholl, B. J., and Gao, T. (2013). “Perceiving animacy and intentionality: visual processing or higher-level judgment?” in Social perception: Detection and interpretation of animacy, agency, and intention. eds. M. D. Rutherford and V. A. Kuhlmeier (Cambridge, MA: MIT Press), 197–230.
Schultz, J., Friston, K. J., O’Doherty, J., Wolpert, D. M., and Frith, C. D. (2005). Activation in posterior superior temporal sulcus parallels parameter inducing the percept of animacy. Neuron 45, 625–635. doi: 10.1016/j.neuron.2004.12.052
Shi, J., Weng, X., He, S., and Jiang, Y. (2010). Biological motion cues trigger reflexive attentional orienting. Cognition 117, 348–354. doi: 10.1016/j.cognition.2010.09.001
Simion, F., Regolin, L., and Bulf, H. (2008). A predisposition for biological motion in the newborn baby. PNAS 105, 809–813. doi: 10.1073/pnas.0707021105
Sokolov, A. A., Erb, M., Gharabaghi, A., Grodd, W., Tatagiba, M. S., and Pavlova, M. A. (2012). Biological motion processing: the left cerebellum communicates with the right superior temporal sulcus. NeuroImage 59, 2824–2830. doi: 10.1016/j.neuroimage.2011.08.039
Spelke, E. S., and Kinzler, K. D. (2007). Core knowledge. Dev. Sci. 10, 89–96. doi: 10.1111/j.1467-7687.2007.00569.x
Stosic, M., Brass, M., Van Hoeck, N., Ma, N., and Van Overwalle, F. (2014). Brain activation related to the perception of minimal agency cues: the role of the mirror system. NeuroImage 86, 364–369. doi: 10.1016/j.neuroimage.2013.10.007
Thornton, I. M., and Vuong, Q. C. (2004). Incidental processing of biological motion. Curr. Bio. 14, 1084–1089. doi: 10.1016/j.cub.2004.06.025
Tomonaga, M. (2001). Visual search for biological motion patterns in chimpanzees (Pan troglodytes). Int. J. Psychol. Orient. 44, 46–59.
Träuble, B., Pauen, S., and Poulin-Dubois, D. (2014). Speed and direction changes induce the perception of animacy in 7-month-old infants. Front. Psychol. 5:1141. doi: 10.3389/fpsyg.2014.01141
Tremoulet, P. D., and Feldman, J. (2000). Perception of animacy from the motion of a single object. Perception 29, 943–951. doi: 10.1068/p3101
Troje, N. F. (2013). What is biological motion? Definition, stimuli and paradigms. Social perception: Detection and interpretation of animacy, agency, and intention. Cambridge, MA: MIT Press, 13–36.
Troje, N. F., and Westhoff, C. (2006). The inversion effect in biological motion perception: evidence for a “life detector”? Curr. Bio. 16, 821–824. doi: 10.1016/j.cub.2006.03.022
Vallortigara, G., Regolin, L., and Marconato, F. (2005). Visually inexperienced chicks exhibit spontaneous preference for biological motion patterns. PLoS Biol. 3:e208. doi: 10.1371/journal.pbio.0030208
Van Overwalle, F. (2009). Social cognition and the brain: a meta-analysis. Hum. Brain Map 30, 829–858. doi: 10.1002/hbm.20547
Vogelsang, M., Vogelsang, L., Diamond, S., and Sinah, P. (2023). Prenatal auditory experience and its sequelae. Dev. Sci. 26:e13278. doi: 10.1111/desc.13278
Wheatley, T., Milleville, S. C., and Martin, A. (2007). Understanding animate agents: distinct roles for the social network and mirror system. Psychol. Sci. 18, 469–474. doi: 10.1111/j.1467-9280.2007.01923.x
Yokoyama, C., Autio, J. A., Ikeda, T., Sallet, J., Mars, R. B., Van Essen, D. C., et al. (2021). Comparative connectomics of the primate social brain. NeuroImage 245:118693. doi: 10.1016/j.neuroimage.2021.118693
Keywords: visual attention, animacy, biological motion, infants, social brain
Citation: Lunghi M and Di Giorgio E (2024) I like the way you move: how animate motion affects visual attention in early human infancy. Front. Neurosci. 18:1459550. doi: 10.3389/fnins.2024.1459550
Received: 04 July 2024; Accepted: 01 August 2024;
Published: 13 August 2024.
Edited by:
Ichiro Fujita, Osaka University, JapanReviewed by:
Toshiya Matsushima, Hokkaido University, JapanCopyright © 2024 Lunghi and Di Giorgio. This is an open-access article distributed under the terms of the Creative Commons Attribution License (CC BY). The use, distribution or reproduction in other forums is permitted, provided the original author(s) and the copyright owner(s) are credited and that the original publication in this journal is cited, in accordance with accepted academic practice. No use, distribution or reproduction is permitted which does not comply with these terms.
*Correspondence: Marco Lunghi, bWFyY28ubHVuZ2hpQHVuaXBkLml0
Disclaimer: All claims expressed in this article are solely those of the authors and do not necessarily represent those of their affiliated organizations, or those of the publisher, the editors and the reviewers. Any product that may be evaluated in this article or claim that may be made by its manufacturer is not guaranteed or endorsed by the publisher.
Research integrity at Frontiers
Learn more about the work of our research integrity team to safeguard the quality of each article we publish.