- 1Department of Neurology, Clinical Medical School of Jiujiang University, Jiujiang, China
- 2Jiujiang Clinical Precision Medicine Research Center, Jiujiang, China
- 3School of Basic Medicine, Jiujiang University, Jiujiang, China
- 4Department of Neurology, University of California Irvine Medical Center, Irvine, CA, United States
Moyamoya disease (MMD) is a rare condition that affects the blood vessels of the central nervous system. This cerebrovascular disease is characterized by progressive narrowing and blockage of the internal carotid, middle cerebral, and anterior cerebral arteries, which results in the formation of a compensatory fragile vascular network. Currently, digital subtraction angiography (DSA) is considered the gold standard in diagnosing MMD. However, this diagnostic technique is invasive and may not be suitable for all patients. Hence, non-invasive imaging methods such as computed tomography angiography (CTA) and magnetic resonance angiography (MRA) are often used. However, these methods may have less reliable diagnostic results. Therefore, High-Resolution Magnetic Resonance Vessel Wall Imaging (HR-VWI) has emerged as the most accurate method for observing and analyzing arterial wall structure. It enhances the resolution of arterial walls and enables quantitative and qualitative analysis of plaque, facilitating the identification of atherosclerotic lesions, vascular entrapment, myofibrillar dysplasia, moyamoya vasculopathy, and other related conditions. Consequently, HR-VWI provides a new and more reliable evaluation criterion for diagnosing vascular lesions in patients with Moyamoya disease.
1 Introduction
Moyamoya disease (MMD) is a rare cerebral artery disease most commonly found in East Asian populations, with an annual incidence of 0. 94–4. 3 per 100,000 (Zhang et al., 2022). The typical features of MMD are the Internal carotid artery (ICA), middle cerebral artery (MCA), and anterior cerebral artery (ACA) progressive stenosis or occlusion, along with a collateral network of compensatory fragile vessels forming nearby (Kuroda et al., 2022). There are also studies claiming that these vessels of collateral circulation are actively formed (Kim et al., 2014) and that they appear “smoke-like” (called moyamoya in Japanese) in contrast (Suzuki and Takaku, 1969). The 2021 Moyamoya Research Committee’s updated criteria have revised the radiological diagnosis to specify that both bilateral and unilateral cases can be diagnosed as MMD (Kuroda et al., 2022). The diagnostic criteria for vascular disease range from DSA to MRI + MRA to HR-VWI. However, HR-VWI technology was gradually used in clinical practice after the discovery of black blood sequences to observe the structures on the vascular wall (Alexander et al., 2016; Mossa-Basha et al., 2016; Yuan and Parker, 2016; Mandell et al., 2017). For human tissues, HR-VWI is not limited to blood vessels but has been applied in bone, liver, and some other tissues (Kijowski and Fritz, 2023).
The latest research progress of HR-VWI in MMD shows that it is superior to other examination methods in MMD diagnosis, disease course detection, treatment, and prognosis evaluation (Das et al., 2023; Lu et al., 2023b; Yu et al., 2023). At present, the clinical examination items (DSA/CTA or MRA) can only detect the degree of lumen stenosis, but cannot distinguish the disease type. HR-VWI can present corresponding imaging characteristics in intracranial atherosclerosis (ICAD) (Ya et al., 2020), arterial dissection (Lee et al., 2021), vasculitis (Pfefferkorn et al., 2011; Edjlali et al., 2020), and MMD (Ya et al., 2020). HR-VWI can be used for these intracranial vascular diseases with vascular stenosis due to different etiologies.
2 Evaluation of cerebrovascular disease
Cerebrovascular diseases are evaluated based on morphology, structure, and function. The traditional way of evaluating morphology is by assessing the degree of blood vessel lumen stenosis, expressed as a percentage of the total vascular lumen, to determine disease severity and progression (Lehman et al., 2019; Ihara et al., 2022). However, there are many limitations in evaluating a single degree of stenosis (van den Wijngaard et al., 2016). In addition to reduced tube diameter, stenosis length is also associated with disease severity and progression (Mori et al., 1998). The structural evaluation assesses vascular lumen structure, and HR-VWI evaluates lumen and wall structure effectively. Luminal morphologic examination (DSA/CTA or MRA) may show normal stenosis in ischemic stroke patients. CT perfusion imaging is used for functional evaluation. Evaluating the blood supply capacity of blood vessels is crucial in ischemic stroke as stenosis or plaque rupture restricts blood flow causing cerebral infarction (Drouet, 2002). It is also necessary to evaluate the blood supply ability of blood vessels.
3 Pathologic features of MMD
MMD has six stages according to Japanese experts Suzuki et al. These stages are based on the degree of Moyamoya vasculopathy (MMV) expansion and the severity of stenosis or occlusion of major cerebral arteries as seen through MMD cerebrovascular angiography (Suzuki and Takaku, 1969). The lateral branch compensatory network develops as MMD progresses through these stages with collateral vessels such as basilar, choroidal, pericallosal, sigmoidal, and epidural (Pilgram-Pastor et al., 2022). Significant differences exist in the clinical manifestations of MMD in children and adults. MMD in children shows as recurrent transient ischemic attack (TIA) or headache (Tho-Calvi et al., 2018). Cerebral infarction and intellectual disability may occur after disease progression (Fukui et al., 2000). In adults, intracerebral, intraventricular, or subarachnoid hemorrhage often occurs. Severe disability and death may occur after intracerebral hemorrhage (ICH) (Fukui et al., 2000). In MMD patients, stenotic or occluded arteries show histopathologic features such as arterial smooth muscle cell proliferation, degeneration or death, intima-media thinning, elastic lamina fragmentation, and necrotic cellular component accumulation. This causes intimal enhancement and luminal narrowing (Guo et al., 2009). Autopsy studies show vacuolar degeneration of cerebrovascular smooth muscle cells (Lin et al., 2012). There is no evidence of significant inflammatory cell infiltration in the stenotic arteries (Fukui et al., 2000), however abnormal immune-related proteins are present (Lin et al., 2012). In contrast to ICAD, there was no significant inflammatory cell infiltration in the stenotic arteries of MMD (Zhang et al., 2019). In ICAD, the main cause of arterial stenosis is the formation of atherosclerotic plaques, which are composed mainly of a fibrous cap, foam cells cholesterol crystals, and foci of calcification (Cury et al., 2006) (Figure 1). Autoimmune diseases such as Graves disease and antiphospholipid syndrome have a close association with the progression of MMD (Chen et al., 2015; Mikami et al., 2019; Fox et al., 2021; Asselman et al., 2022). In a study by Li et al., hyperthyroidism was found in 0.9% of normal children, which is lower than the 10.5% seen in children with MMD (p = 0.003) (Li et al., 2011). In MMD, the ring finger protein 213 p.R4810K variant is strongly associated with vessel wall enhancement (VWE) and poor prognosis (Hao et al., 2024). A total of 16 million Asians living outside of Asia carry this RNF213 variant (Liu et al., 2012). Some studies have reported an independent association between the variant and recurrent stroke (Kim et al., 2020). In East Asia, MMD is tenfold more common than in European countries and the United States, at least in part because of variants (Koizumi et al., 2016). Additionally, it has been linked to a wide range of cardiovascular diseases (Okazaki et al., 2019).
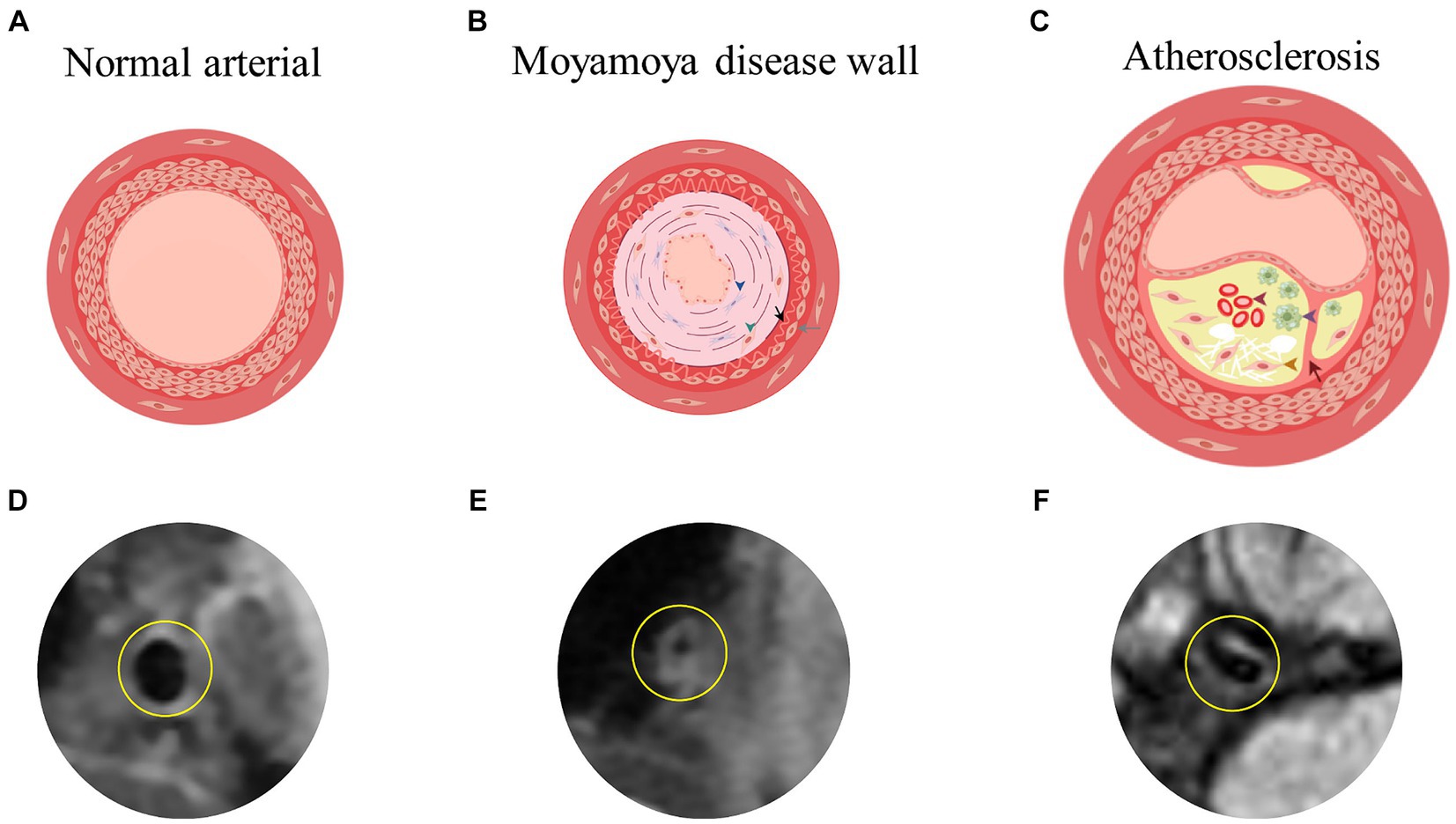
Figure 1. (A,D) Showed the simplified image of the three-layer membrane of the normal arterial wall and the actual HR-VWI of the normal artery wall. (B) Showed the damaged arterial wall in MMD, characterized by enhancement and bending of the intima and thinning of the media (blue triangles for fibroblast proliferation, green triangles for smooth muscle cell displacement and proliferation, black arrows for extremely curved and thickened intima, and gray arrows for fewer smooth muscle cells in the media). (C) Showed the atherosclerotic arterial wall, including subcutaneous lipid deposition, foam cell formation (purple triangle), smooth muscle cell displacement and proliferation (yellow triangle), and internal bleeding (red triangle) as well as rupture of the inner elastic membrane (red arrow). By Figdraw. (E,F) Showed the actual HR-VWI of patients with MMD or atherosclerosis. HR-VWI, High-Resolution Magnetic Resonance Vessel Wall Imaging; MMD, moyamoya disease.
4 Overview of HR-VWI
HR-VWI is an imaging technique that can visualize vessel lumen and detect pathological changes in arterial walls. It can identify intra-plaque lipids, calcification, and fibrous cap rupture in atherosclerotic plaques. HR-VWI is also more accurate than traditional angiographic methods (DSA/CTA or MRA) in detecting fresh hemorrhage (Yuan et al., 2001; Koo, 2015; Yu et al., 2015; Mossa-Basha et al., 2017; Young et al., 2019). HR-VWI requires a high-field-intensity nuclear magnetic resonance instrument and a high-resolution imaging coil. 3.0 T MR is widely used for detecting intracranial arterial blood walls (Li et al., 2014). At present, 7.0 T MR has been gradually applied to in vivo imaging, and there have been studies on imaging intracranial artery walls using 7.0 T MR (Deng et al., 2016). 7.0 T MR is used for in vivo imaging, but it may not help determine the Suzuki stage, ivy sign, and ICA diameter measurement (Deng et al., 2016; Dengler et al., 2016; Oh et al., 2017). However, further studies are needed to confirm this. Higher magnetic field strength leads to better image resolution or SNR. For 3.0 T MR head imaging, there are several receiving coils, including 8, 16, and 32-channel head coils, or 16, 20, 24, and 64-channel high-resolution coils for imaging the head and neck. Presently, the 32-channel high-resolution coil is commonly used (Kim et al., 2022; Liang et al., 2023). Studies have shown that, compared with 8-the channel head coil, the 32-channel head coil can increase SNR up to 3.4 times in the cortex and 1.4 times in the corpus callosum (Wiggins et al., 2006); Compared with the 12-channel head coil, the 32-channel head coil increased the SNR in the middle of the brain by about 1.5 times (Wiggins et al., 2006). For combined imaging of intracranial and extracranial arterial walls, a special head and neck joint coil is required (Hu et al., 2017). All of the above coils can only fit closely with adults, and then some scholars studied the coil that can fit closely with the head of children, and compared with the 32-channel coil sold in the market, the average SNR was 2.7 times higher (Ghotra et al., 2021) (Figure 2).
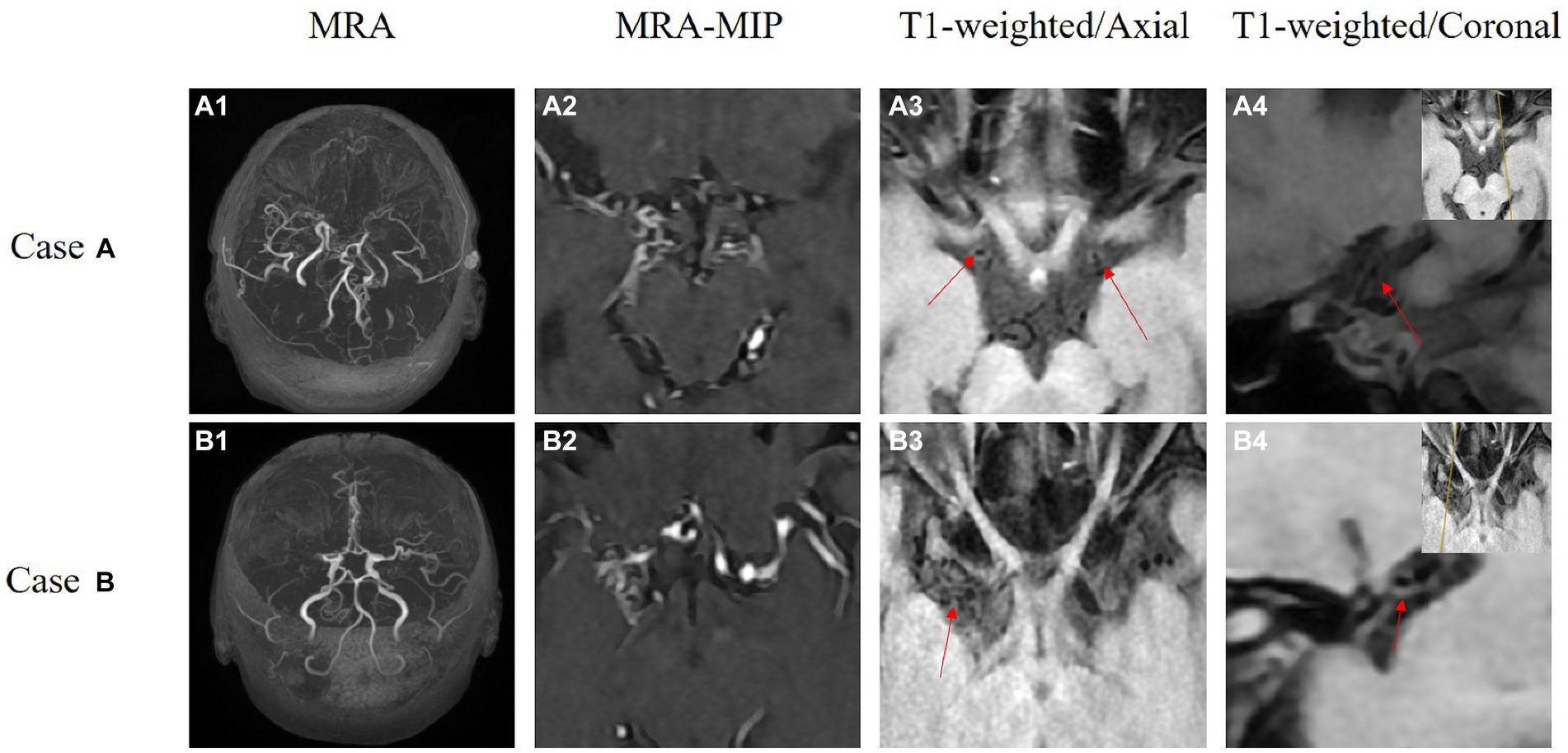
Figure 2. These are the HR-VWI of two MMD patients acquired using a 3.0 T MR scanner. Case A depicts bilateral MMD. A1,A2 show revealing occlusion of bilateral internal carotid arteries to the middle cerebral arteries. A3,A4 are high-resolution thin-slice scans of the middle cerebral arteries, where the red arrows indicate circular high signals. Case B is a patient with unilateral MMD. B1,B2 show occlusion of the right middle cerebral artery. B3,B4 present high-resolution thin-slice scans of the right middle cerebral artery, where the red arrows indicate circular high signals.
The core parameters of HR-VWI include imaging dimension, spatial resolution, and imaging sequence. The imaging dimensions include 2D and 3D (Mandell et al., 2017). The advantage of 3D imaging is that it can cover a larger imaging volume including the whole brain (Dieleman et al., 2014); 3D imaging can also be valuable for observing the morphology and distribution characteristics of intracranial arterial lesions from multiple angles (Mandell et al., 2017). Important reference for endovascular treatment such as stent implantation (Lou et al., 2014). At the same time, a large amount of image data is generated, which brings certain challenges to the analysis (Li et al., 2019). For 3D vessel wall imaging of intracranial arteries at spatial resolution, an anisotropic resolution of ≤0. 5 mm × 0. 5 mm × 0. 5 mm is recommended (Li et al., 2019). Enhance with 3D imaging, as CSF pulsation artifacts are evident as well as flow-related signals. Imaging sequences using blood and cerebrospinal fluid signal suppression sequences are commonly used today, including spin echo, double inversion recovery (DIR), and fast spin echo sequences. DIR is useful for thinner tissue layers due to its ability to suppress blood flow signals. Other techniques include spatial pre-saturation (saturation band), motion-sensitized driven-equilibrium pre-pulse (MSDE), and delay alternating with nutation for tailored excitation (DANTE) (Li et al., 2019). MSDE and DANTE can be used for thicker layers (of tissue), while DANTE is currently limited to experimental research (Li et al., 2014). The variable-angle fast spin echo sequence (VFAFSE/TSE) obtained by improvement is currently the main acquisition sequence for intracranial 3D vascular wall imaging, with advantages such as high intra-layer resolution, high SNR, and insensitivity to magnetic field inhomogeneity (Li et al., 2019). Based on the above advantages, VFAFSE/TSE has been widely used for arterial wall imaging of intracranial arteries (Huang et al., 2023), carotid arteries (Saba et al., 2023), aorta (Hu et al., 2020), and thoracic great vessels (Treitl et al., 2017). Image contrast is also included in the imaging sequence, which requires the sum of image information with multiple contrasts, mainly including the time-leap method, PDWI, T1WI, and T2WI. Among them, T1WI can fully display the location, morphology, reconstruction, and signal characteristics of intracranial arterial lesions (Dieleman et al., 2014) (such as whether the vascular wall is enhanced or not) (Lu et al., 2021). Some scholars have found that the high T2WI signal of the intracranial artery wall helps identify the nature of vascular wall lesions (Mossa-Basha et al., 2017), and can also be used to evaluate the external diameter of the vascular lesions (Kuroda et al., 2015), but further verification is needed.
5 Application of HR-VWI in MMD
A patient was diagnosed with MMD when at least two of the following three HR-VWI features were found in the affected segment of the ICA terminal segment or the MCA/ACA proximal segment: (1): No eccentric wall enhancement (Wu et al., 2021), (2): Vessel external diameter reduction (Kuroda et al., 2022), (3): uniform signal (Yang et al., 2021; Lu et al., 2023b). Eccentric wall enhancement refers to eccentric or focal wall enhancement evident on HR-VWI. The thickest part of the wall is estimated to be twice as thick as the thinnest part of the vascular lesion (Wang et al., 2017). The eccentricity index is calculated as follows: eccentricity index = (maximum vessel wall thickness − minimum vessel wall thickness) / maximum vessel wall thickness. If the eccentricity index ≥0. 5, it is considered eccentric VWE, otherwise, it is centripetal VWE (Shishikura et al., 2020). When the outer diameter of the involved artery is significantly smaller than the normal adjacent distal site or normal reference value, it indicates that the outer diameter is reduced (Kaku et al., 2012). T2WI sequences were used to assess the outer diameter of stenotic vessels at the site of minimal outer diameter (Kuroda et al., 2015). Signal heterogeneity was considered when stenotic vessel signal intensity was found to include a mixture of signals with high or low signal intensity in T1WI sequences; otherwise, signal homogeneity was considered (Lu et al., 2021). Using the above HR-VWI diagnostic criteria can improve the diagnostic rate of MMD (Figure 3).
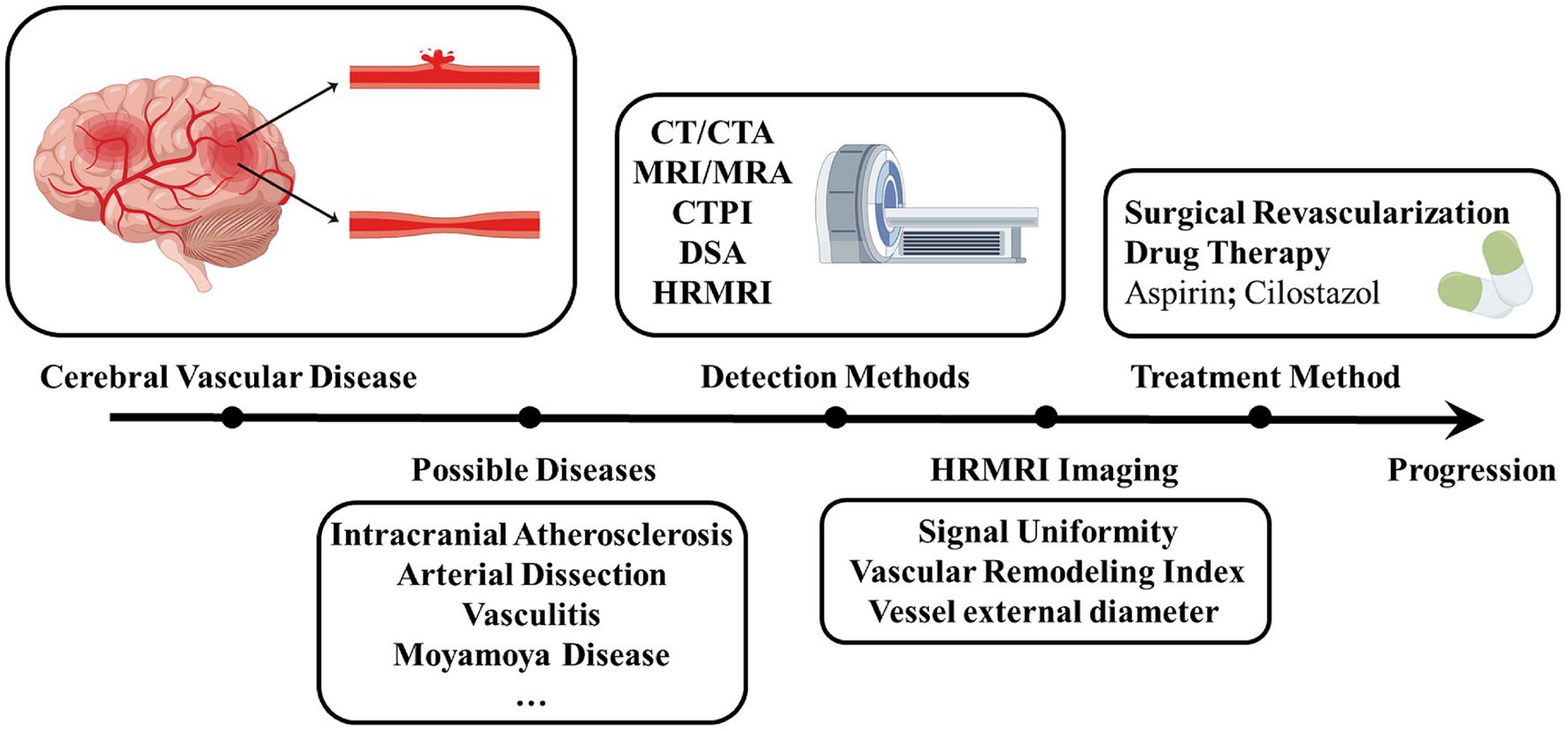
Figure 3. Progression of diagnosis and treatment of Moyamoya disease. Shows the process of diagnosing and treating a patient with a cerebrovascular disease that is eventually diagnosed as a Moyamoya disease after multiple detection methods have been performed. By figdraw. CTA, Computed tomography angiography; MRA, Magnetic resonance angiography; CTPI, Computed tomography perfusion imaging; DSA, Digital subtraction angiography; HR-VWI, High-Resolution Magnetic Resonance Vessel Wall Imaging.
5.1 Role of HR-VWI in the diagnosis of MMD
VWE plays a crucial role in diagnosing and monitoring MMD through HR-VWI. Studies have found that HR-VWI of MMD patients mostly have the following characteristics: concentric stenosis appears in the vessel wall at the distal ICA or the beginning of MCA, with uniform signals (Yuan et al., 2015) and VWE indicating the progression of MMD vascular lesions (Roder et al., 2019). Some studies use the vascular remodeling index to perform HR-VWI evaluation on the stenosis characteristics of MMV. In MMD patients, vascular stenosis is mainly due to negative remodeling. The VWE degree in MMD is lower than in ICAD-induced stenosis (p < 0.01) (Ya et al., 2020). HR-VWI studies show that MCA wall signal uniformity is higher in MMD patients (85.7%) than in ICAD patients (32.6%) (p < 0.001) (Yuan et al., 2015). ICAD-induced stenosis is usually characterized by eccentric and inhomogeneous enhancement of the vessel wall (Ryoo et al., 2014). Researchers evaluated MCA using HR-VWI with 3 vessel wall characteristics to differentiate between MMD and atherosclerosis-associated moyamoya vasculopathy (AS-MMV). Patients with AS-MMV had higher outer diameter, remodeling index, and pattern of VWE (all p < 0.001). The outer diameter had a better diagnostic performance compared to the remodeling index in distinguishing AS-MMV from MMD (outer diameter vs. remodeling index: 0.912 vs. 0.889, p = 0.007) (Liu et al., 2023). MMD causes the narrowing of cerebral arteries and leads to the development of side branches known as MMV. This can result in hemorrhage with a high risk of recurrence and rebleeding in hemorrhagic MMD (Funaki et al., 2019; Wang et al., 2020). This is because the pathophysiologic changes in MMD usually include the formation of abnormal collateral vessels caused by perforating arteries (Takagi et al., 2007). Branching extensions of perforating arteries can form abnormal connections known as collateral pathways. These connections increase vascular fragility and lead to microaneurysms, which can cause intracranial hemorrhage (Yamashita et al., 1983; Matsushige et al., 2016; Rhim et al., 2018). T1-weighted black blood HR-VWI shows perforating arteries in MMD patients clearly, while 3.0 T MR HR-VWI characterizes their morphological changes (Okuchi et al., 2014; Wu et al., 2021). This also suggests that identifying the side branches also helps to diagnose MMD.
5.2 Role of HR-VWI in monitoring the progress of MMD
HR-VWI can provide detailed information about the anatomy of MMD and the abnormal vascular network, which can help assess the risk of ischemia or hemorrhage in MMD (Lu et al., 2021; Zhao et al., 2021). One study studied 29 patients with MMD using 3.0 T HR-VWI intracranial vessel wall imaging and found that VWE was positively correlated with ischemic events (Kathuveetil et al., 2020). Another study showed that VWE could be used as a predictor of arterial stenosis and poor prognosis in MMD (Lu et al., 2023a). However, some studies claim that lumen and vessel outer wall diameters decrease with disease severity, but there is no significant difference in wall thickness (Cogswell et al., 2020). Some scholars have used HR-VWI to analyze symptomatic isolated MCA steno-occlusive disease (MCAD) that is not caused by atherosclerosis and found that MMD is the cause of some non-atherosclerotic lesions. Therefore, symptomatic isolated MCAD may be an early manifestation of MMD in young Asian people (Kim et al., 2016). Another study explored the correlation between choroidal anastomosis and bleeding events in 50 adult patients with advanced MMD using HR-VWI and found that choroidal anastomosis was significantly correlated with hemorrhage (Hirano et al., 2020; Wu et al., 2021). MMD patients with anterior ICH have also been analyzed, and it has been found that the coexistence of reduced lenticulostriate artery and relatively preserved lumen area of the distal ICA may be a potential predictor of anterior ICH in patients with MMD (Xu et al., 2022). In investigating the clinical significance of periventricular collateral enhancement sign (PCES) in adult patients with MMD through vascular wall imaging, it was found that PCES was the only independent factor in bleeding in patients with MMD. It can be seen that PCES can help detect major blood vessels that cause bleeding in MMD patients (Ryu et al., 2022). Using HR-VWI to predict stroke incidence in conservatively treated MMD patients, one prospective study concluded that VWE was significantly associated with an increased incidence of stroke (HR, 4.16; 95% CI, 1.55–11.15) (Zhang H. et al., 2023). However, the small number of subjects in most studies may have biased the final results. It is important to include more patients with MMD in future studies of MMD course progression.
5.3 Role of HR-VWI in the evaluation of MMD treatment
At present, surgical revascularization is the main treatment for MMD patients (Bao and Duan, 2023), however, surgical treatment may lead to perioperative complications such as ischemia or hydrocephalus (Fujimura et al., 2011). With a better understanding of the pathophysiology of MMD, non-surgical approaches targeting the pathogenesis of MMD can be used to prevent or slow the progression of the disease (Chen T. et al., 2023). In a 2018 national study in Japan, it was noted that the mechanism by which an antiplatelet agent (APT) is used to treat MMD is unknown, but it was summarized that aspirin, cilostazol, and clopidogrel are used in the course of MMD treatment, with aspirin being the most commonly used (Oki et al., 2018). An analysis of 14 years of follow-up showed that APT treatment (Aspirin HR, 0.77; 95% CI, 0.61–0.97, p = 0. 03; Clopidogrel HR, 1.08; 95% CI, 0.97–1.21, p = 0.15) can significantly improve the survival rate of MMD patients, especially cilostazol (HR, 0.64; 95% CI, 0.54–0.75, p < 0. 01), which had greater survival benefits (Seo et al., 2021). In a retrospective study in 2023, it was found that APT could reduce the mortality of patients with hemorrhagic MMD and did not increase the risk of hemorrhagic stroke, all of which indicated that APT may benefit MMD patients (Luo et al., 2023). At the same time, some studies have suggested that perfusion improvement in MMD patients, rather than antiplatelet and statins, maybe the main step in decreasing MMD-associated strokes (Chen et al., 2022). Subsequent studies using HR-VWI were able to demonstrate the specific mechanism played by cilostazol in patients with MMD. It was demonstrated that cilostazol resulted in a significant increase in the outer diameter of the diseased vessel, a significant reduction in the degree of stenosis, and positive remodeling (Kim et al., 2022). This is also the only study that used HR-VWI to analyze medical interventions for MMD. According to the analysis results, HR-VWI is a better technique to investigate the specific effects of therapeutic drugs on patients with MMD (Table 1).
HR-VWI has its advantages in MMD diagnosis, disease course monitoring, and treatment risk prediction. In the diagnosis of MMD, ICAD, and MMD are distinguished according to the characteristics of vascular wall imaging, and the characteristics of vascular imaging of MMD are summarized to provide a reference for clinical workers. In the latest research on MMD course monitoring, it can be seen that HR-VWI can more clearly investigate the related vascular lesions that may cause stroke events during MMD. In the process of treatment and prognosis of MMD, it can be used as a detection tool to timely detect the specific sites and effects of drugs or treatments in MMD, to adjust to the appropriate degree to achieve better therapeutic effects.
6 Comparison of HR-VWI with other imaging tools
Diagnostic evaluation of cerebrovascular disease includes transcranial Doppler (TCD), CTA, MRA, and DSA (Koo, 2015). DSA is currently the gold standard for the diagnosis of MMD but may capture overlapping imaging features and be more invasive (Park et al., 2017; Ihara et al., 2022; Waddle et al., 2022). A comparative analysis of studies has shown that HR-VWI improves diagnostic confidence in conditions where clinical or DSA imaging features are unclear (Park et al., 2017), and also identifies vascular remodeling, which may be clinically important but cannot be recognized by conventional imaging. TCD is a rapid, noninvasive diagnostic technique that determines the degree of stenosis based on the velocity of blood flow in the cerebral vasculature (Wan et al., 2022; Chen J. Y. et al., 2023). MRA can noninvasively identify the characteristic architecture of MMD and can intimate areas of major occlusions, reconstructions, and small-vessel collateral flow (Togao et al., 2018), but with limited resolution and poor display of fine arterioles (Li et al., 2019). HR-VWI has higher imaging clarity and precision and can visualize the subtle changes in the vessel wall. CTA scanning is fast, but requires injection of contrast medium and, a higher radiation dose, and the MMV display at the base of the skull is easily interfered with by the skull bone (Byworth et al., 2021). HR-VWI is non-invasive and radiation-free compared to the other detection methods. Given that conventional imaging techniques are more focused on the degree of luminal stenosis and are more limited, there is a tendency for misdiagnosis for a disease that manifests solely initially with luminal stenosis. This can lead to patients not being able to obtain the correct treatment in time, which further leads to a series of consequences including the decline in the survival period and overall poor prognosis (Table 2).
7 Conclusion and prospect
HR-VWI for diagnosing MMD faces challenges such as the lack of unified scanning sequences, parameters, and standardized analysis guidelines. Long MRI acquisition time increases the risk of missing the optimal window for revascularization (Bao and Duan, 2023). HR-VWI application in MMD also lacks pathologic control and pathophysiologic analysis because it is difficult to obtain arterial specimens from living patients (Yu et al., 2015), so the study of specific tissue components of intracranial vessels is still limited. Advanced intracranial wall imaging techniques are being explored for better clinical application. Further studies are needed to validate HR-VWI features and determine its predictive power for treatment and stroke (Bodle et al., 2013; Wang et al., 2021). Use HR-VWI to observe various drug therapies beyond APT, such as drugs to increase angiogenesis, anticancer drugs to reduce smooth muscle cell proliferation, retinoids, and other specific changes in diseased blood vessels of MMD patients (Guo et al., 2009; Bang et al., 2015; Lee et al., 2015). Use HR-VWI technology to investigate MMD patients in different stages and explore potential pathogenesis. Combine emerging technologies, like AI, to improve HR-VWI performance (Kim et al., 2019). A Pseudo-Three-Dimensional Residual Network has been proposed to process spatio-temporal information and combined with DSA to recognize MMD (Xu et al., 2023), and a 3D coordinate attention residual network has also been used in combination with MRA to evaluate stenotic occlusion changes in MMD (Zhang Z. et al., 2023). In the future, it is possible to use these techniques in combination with HR-VWI. Compressed sensing techniques can be used to reduce redundant imaging data acquisition (Yamamoto et al., 2018). HR-VWI can be combined with other imaging techniques for clinical decision-making.
Author contributions
HY: Data curation, Resources, Visualization, Writing – original draft. GH: Data curation, Writing – original draft. XL: Writing – original draft, Writing – review & editing. MW: Data curation, Writing – original draft. WZ: Writing – original draft. XY: Funding acquisition, Writing – original draft. MZ: Conceptualization, Writing – original draft, Writing – review & editing. ZC: Conceptualization, Funding acquisition, Writing – original draft, Writing – review & editing.
Funding
The author(s) declare financial support was received for the research, authorship, and/or publication of this article. This study was supported partially by the National Natural Science Foundation of China (81960221 and 82260249 to XY), and Jiangxi Provincial Health Commission Science and Technology Plan project (202311506 to ZC), Jiangxi Provincial Administration of Traditional Chinese Medicine science and technology plan project (2022A322 to ZC), Key Projects of Jiangxi Provincial Department of Education (GJJ2201902 to ZC), Youth Foundation of Natural Science Foundation of Jiangxi Province (20224BAB216045 to ZC).
Acknowledgments
We sincerely thank the staff of the Jiujiang Precision Clinical Medicine Research Center and its students Jianzhong Li, Lihong Li, Wengao Zhang, Jiaxuan Wang and Yiyang Yan.
Conflict of interest
The authors declare that the research was conducted in the absence of any commercial or financial relationships that could be construed as a potential conflict of interest.
Publisher’s note
All claims expressed in this article are solely those of the authors and do not necessarily represent those of their affiliated organizations, or those of the publisher, the editors and the reviewers. Any product that may be evaluated in this article, or claim that may be made by its manufacturer, is not guaranteed or endorsed by the publisher.
References
Alexander, M. D., Yuan, C., Rutman, A., Tirschwell, D. L., Palagallo, G., Gandhi, D., et al. (2016). High-resolution intracranial vessel wall imaging: imaging beyond the lumen. J. Neurol. Neurosurg. Psychiatry 87, 589–597. doi: 10.1136/jnnp-2015-312020
Asselman, C., Hemelsoet, D., Eggermont, D., Dermaut, B., and Impens, F. (2022). Moyamoya disease emerging as an immune-related angiopathy. Trends Mol. Med. 28, 939–950. doi: 10.1016/j.molmed.2022.08.009
Bang, O. Y., Goyal, M., and Liebeskind, D. S. (2015). Collateral circulation in ischemic stroke: assessment tools and therapeutic strategies. Stroke 46, 3302–3309. doi: 10.1161/strokeaha.115.010508
Bao, X. Y., and Duan, L. (2023). Chinese expert consensus on the treatment of MMD. Chin. Neurosurg. J. 9:5. doi: 10.1186/s41016-023-00318-3
Bodle, J. D., Feldmann, E., Swartz, R. H., Rumboldt, Z., Brown, T., and Turan, T. N. (2013). High-resolution magnetic resonance imaging: an emerging tool for evaluating intracranial arterial disease. Stroke 44, 287–292. doi: 10.1161/strokeaha.112.664680
Byworth, M. T., Moffatt, J. I., and Perera, K. S. (2021). Novel vascular anastomoses and Moyamoya disease in a woman with down syndrome. Can. J. Neurol. Sci. 48, 417–418. doi: 10.1017/cjn.2020.195
Chen, J. Y., Ding, Y. H., Li, Y., Shi, S. S., Chen, J., and Tu, X. K. (2023). Assessment of bypass patency using transcranial Doppler sonography: correlations with computerized tomography angiography findings in patients with moyamoya disease. Neurosurg. Rev. 46:64. doi: 10.1007/s10143-023-01970-0
Chen, J. B., Lei, D., He, M., Sun, H., Liu, Y., Zhang, H., et al. (2015). Clinical features and disease progression in moyamoya disease patients with graves disease. J. Neurosurg. 123, 848–855. doi: 10.3171/2014.10.Jns141140
Chen, T., Wei, W., Yu, J., Xu, S., Zhang, J., Li, X., et al. (2023). The progression of pathophysiology of Moyamoya disease. Neurosurgery 93, 502–509. doi: 10.1227/neu.0000000000002455
Chen, Z., Wu, X., Zhou, D., Shang, S., Ding, Y., Ji, X., et al. (2022). Characteristics of cerebral ischemic stroke based on moyamoya disease and atherosclerosis-associated intracranial arterial stenosis. Neurol. Sci. 43, 1087–1096. doi: 10.1007/s10072-021-05359-z
Cogswell, P. M., Lants, S. K., Davis, L. T., Juttukonda, M. R., Fusco, M. R., and Donahue, M. J. (2020). Vessel Wall and lumen features in north American Moyamoya patients. Clin. Neuroradiol. 30, 545–552. doi: 10.1007/s00062-019-00819-8
Cury, R. C., Houser, S. L., Furie, K. L., Stone, J. R., Ogilvy, C. S., Sherwood, J. B., et al. (2006). Vulnerable plaque detection by 3.0 tesla magnetic resonance imaging. Investig. Radiol. 41, 112–115. doi: 10.1097/01.rli.0000186419.55504.30
Das, S., Mossa-Basha, M., Dey, M., Hazra, A., Pandit, A., Das, G., et al. (2023). High-resolution vessel wall magnetic resonance imaging in intracranial vasculopathies: an experience from eastern India. Br. J. Radiol. 96:20230114. doi: 10.1259/bjr.20230114
Deng, X., Zhang, Z., Zhang, Y., Zhang, D., Wang, R., Ye, X., et al. (2016). Comparison of 7.0- and 3.0-T MRI and MRA in ischemic-type moyamoya disease: preliminary experience. J. Neurosurg. 124, 1716–1725. doi: 10.3171/2015.5.Jns15767
Dengler, N. F., Madai, V. I., Wuerfel, J., von Samson-Himmelstjerna, F. C., Dusek, P., Niendorf, T., et al. (2016). Moyamoya vessel pathology imaged by ultra-high-field magnetic resonance imaging at 7.0 T. J. Stroke Cerebrovasc. Dis. 25, 1544–1551. doi: 10.1016/j.jstrokecerebrovasdis.2016.01.041
Dieleman, N., van der Kolk, A. G., Zwanenburg, J. J., Harteveld, A. A., Biessels, G. J., Luijten, P. R., et al. (2014). Imaging intracranial vessel wall pathology with magnetic resonance imaging: current prospects and future directions. Circulation 130, 192–201. doi: 10.1161/circulationaha.113.006919
Drouet, L. (2002). Atherothrombosis as a systemic disease. Cerebrovasc. Dis. 13, 1–6. doi: 10.1159/000047782
Edjlali, M., Qiao, Y., Boulouis, G., Menjot, N., Saba, L., Wasserman, B. A., et al. (2020). Vessel wall MR imaging for the detection of intracranial inflammatory vasculopathies. Cardiovasc. Diagn. Ther. 10, 1108–1119. doi: 10.21037/cdt-20-324
Fox, B. M., Dorschel, K. B., Lawton, M. T., and Wanebo, J. E. (2021). Pathophysiology of vascular stenosis and remodeling in Moyamoya disease. Front. Neurol. 12:661578. doi: 10.3389/fneur.2021.661578
Fujimura, M., Shimizu, H., Inoue, T., Mugikura, S., Saito, A., and Tominaga, T. (2011). Significance of focal cerebral hyperperfusion as a cause of transient neurologic deterioration after extracranial-intracranial bypass for moyamoya disease: comparative study with non-moyamoya patients using N-isopropyl-p-[(123)I]iodoamphetamine single-photon emission computed tomography. Neurosurgery 68:957-964; discussion 964-955. doi: 10.1227/NEU.0b013e318208f1da
Fukui, M., Kono, S., Sueishi, K., and Ikezaki, K. (2000). Moyamoya disease. Neuropathology 20, 61–64. doi: 10.1046/j.1440-1789.2000.00300.x
Funaki, T., Takahashi, J. C., Houkin, K., Kuroda, S., Takeuchi, S., Fujimura, M., et al. (2019). High rebleeding risk associated with choroidal collateral vessels in hemorrhagic moyamoya disease: analysis of a nonsurgical cohort in the Japan adult Moyamoya trial. J. Neurosurg. 130, 525–530. doi: 10.3171/2017.9.Jns17576
Ghotra, A., Kosakowski, H. L., Takahashi, A., Etzel, R., May, M. W., Scholz, A., et al. (2021). A size-adaptive 32-channel array coil for awake infant neuroimaging at 3 tesla MRI. Magn. Reson. Med. 86, 1773–1785. doi: 10.1002/mrm.28791
Guo, D. C., Papke, C. L., Tran-Fadulu, V., Regalado, E. S., Avidan, N., Johnson, R. J., et al. (2009). Mutations in smooth muscle alpha-actin (ACTA2) cause coronary artery disease, stroke, and Moyamoya disease, along with thoracic aortic disease. Am. J. Hum. Genet. 84, 617–627. doi: 10.1016/j.ajhg.2009.04.007
Hao, F., Han, C., Lu, M., Wang, Y., Gao, G., Wang, Q., et al. (2024). High-resolution MRI vessel wall enhancement in moyamoya disease: risk factors and clinical outcomes. Eur. Radiol. doi: 10.1007/s00330-023-10535-0
Hirano, Y., Miyawaki, S., Imai, H., Hongo, H., Ohara, K., Dofuku, S., et al. (2020). Association between the onset pattern of adult Moyamoya disease and risk factors for stroke. Stroke 51, 3124–3128. doi: 10.1161/strokeaha.120.030653
Hu, Z., Christodoulou, A. G., Wang, N., Shaw, J. L., Song, S. S., Maya, M. M., et al. (2020). Magnetic resonance multitasking for multidimensional assessment of cardiovascular system: development and feasibility study on the thoracic aorta. Magn. Reson. Med. 84, 2376–2388. doi: 10.1002/mrm.28275
Hu, X., Li, Y., Zhang, L., Zhang, X., Liu, X., and Chung, Y. C. (2017). A 32-channel coil system for MR vessel wall imaging of intracranial and extracranial arteries at 3T. Magn. Reson. Imaging 36, 86–92. doi: 10.1016/j.mri.2016.10.018
Huang, J., Jiao, S., Chen, Y., Lu, J., Song, Y., Zhang, J., et al. (2023). Efficacy of medical treatment and balloon angioplasty for severe intracranial atherosclerosis: a high-resolution MR vessel wall imaging. Eur. Radiol. 33, 2478–2488. doi: 10.1007/s00330-022-09218-z
Ihara, M., Yamamoto, Y., Hattori, Y., Liu, W., Kobayashi, H., Ishiyama, H., et al. (2022). Moyamoya disease: diagnosis and interventions. Lancet Neurol. 21, 747–758. doi: 10.1016/s1474-4422(22)00165-x
Kaku, Y., Morioka, M., Ohmori, Y., Kawano, T., Kai, Y., Fukuoka, H., et al. (2012). Outer-diameter narrowing of the internal carotid and middle cerebral arteries in moyamoya disease detected on 3D constructive interference in steady-state MR image: is arterial constrictive remodeling a major pathogenesis? Acta Neurochir. 154, 2151–2157. doi: 10.1007/s00701-012-1472-4
Kathuveetil, A., Sylaja, P. N., Senthilvelan, S., Kesavadas, C., Banerjee, M., and Jayanand Sudhir, B. (2020). Vessel Wall thickening and enhancement in high-resolution intracranial Vessel Wall imaging: A predictor of future ischemic events in Moyamoya disease. AJNR Am. J. Neuroradiol. 41, 100–105. doi: 10.3174/ajnr.A6360
Kijowski, R., and Fritz, J. (2023). Emerging Technology in Musculoskeletal MRI and CT. Radiology 306, 6–19. doi: 10.1148/radiol.220634
Kim, H. J., Choi, E. H., Chung, J. W., Kim, J. H., Kim, Y. S., Seo, W. K., et al. (2020). Luminal and wall changes in intracranial arterial lesions for predicting stroke occurrence. Stroke 51, 2495–2504. doi: 10.1161/strokeaha.120.030012
Kim, T., Heo, J., Jang, D. K., Sunwoo, L., Kim, J., Lee, K. J., et al. (2019). Machine learning for detecting moyamoya disease in plain skull radiography using a convolutional neural network. EBioMedicine 40, 636–642. doi: 10.1016/j.ebiom.2018.12.043
Kim, J. Y., Kim, H. J., Choi, E. H., Pan, K. H., Chung, J. W., Seo, W. K., et al. (2022). Vessel Wall changes on serial high-resolution MRI and the use of Cilostazol in patients with adult-onset Moyamoya disease. J. Clin. Neurol. 18, 610–618. doi: 10.3988/jcn.2022.18.6.610
Kim, Y. J., Lee, J. K., Ahn, S. H., Kim, B. J., Kang, D. W., Kim, J. S., et al. (2016). Nonatheroscleotic isolated middle cerebral artery disease may be early manifestation of Moyamoya disease. Stroke 47, 2229–2235. doi: 10.1161/strokeaha.116.012751
Kim, S. J., Son, T. O., Kim, K. H., Jeon, P., Hyun, S. H., Lee, K. H., et al. (2014). Neovascularization precedes occlusion in moyamoya disease: angiographic findings in 172 pediatric patients. Eur. Neurol. 72, 299–305. doi: 10.1159/000365286
Koizumi, A., Kobayashi, H., Hitomi, T., Harada, K. H., Habu, T., and Youssefian, S. (2016). A new horizon of moyamoya disease and associated health risks explored through RNF213. Environ. Health Prev. Med. 21, 55–70. doi: 10.1007/s12199-015-0498-7
Koo, J. (2015). The latest information on intracranial atherosclerosis: diagnosis and treatment. Interv. Neurol. 4, 48–50. doi: 10.1159/000438779
Kuroda, S., Fujimura, M., Takahashi, J., Kataoka, H., Ogasawara, K., Iwama, T., et al. (2022). Diagnostic criteria for Moyamoya disease - 2021 revised version. Neurol. Med. Chir. (Tokyo) 62, 307–312. doi: 10.2176/jns-nmc.2022-0072
Kuroda, S., Kashiwazaki, D., Akioka, N., Koh, M., Hori, E., Nishikata, M., et al. (2015). Specific shrinkage of carotid forks in Moyamoya disease: A novel key finding for diagnosis. Neurol. Med. Chir. (Tokyo) 55, 796–804. doi: 10.2176/nmc.oa.2015-0044
Lee, S. H., Kim, K. Y., and Jung, J. M. (2021). High-resolution magnetic resonance imaging for the follow-up of intracranial arterial dissections. Acta Neurol. Belg. 121, 1599–1605. doi: 10.1007/s13760-020-01432-0
Lee, J. Y., Moon, Y. J., Lee, H. O., Park, A. K., Choi, S. A., Wang, K. C., et al. (2015). Deregulation of retinaldehyde dehydrogenase 2 leads to defective angiogenic function of endothelial Colony-forming cells in pediatric Moyamoya disease. Arterioscler. Thromb. Vasc. Biol. 35, 1670–1677. doi: 10.1161/atvbaha.115.305363
Lehman, V. T., Cogswell, P. M., Rinaldo, L., Brinjikji, W., Huston, J., Klaas, J. P., et al. (2019). Contemporary and emerging magnetic resonance imaging methods for evaluation of moyamoya disease. Neurosurg. Focus. 47:E6. doi: 10.3171/2019.9.Focus19616
Li, L., Chai, J. T., Biasiolli, L., Robson, M. D., Choudhury, R. P., Handa, A. I., et al. (2014). Black-blood multicontrast imaging of carotid arteries with DANTE-prepared 2D and 3D MR imaging. Radiology 273, 560–569. doi: 10.1148/radiol.14131717
Li, J., Jin, M., Sun, X., Li, J., Liu, Y., Xi, Y., et al. (2019). Imaging of Moyamoya disease and Moyamoya syndrome: current status. J. Comput. Assist. Tomogr. 43, 257–263. doi: 10.1097/rct.0000000000000834
Li, H., Zhang, Z. S., Dong, Z. N., Ma, M. J., Yang, W. Z., Han, C., et al. (2011). Increased thyroid function and elevated thyroid autoantibodies in pediatric patients with moyamoya disease: a case-control study. Stroke 42, 1138–1139. doi: 10.1161/strokeaha.110.608471
Li, R., Chen, S., Zheng, H., Liu, X., Zhang, Z., Yang, Q., et al. (2019). Expert consensus on techniques and application of intracranial MR vessel wall imaging in China. Chin J Radiol 53:12. doi: 10.3760/cma.j.issn.1005‑1201.2019.12.006
Liang, X., Peng, F., Yao, Y., Yang, Y., Liu, A., and Chen, D. (2023). Aneurysm wall enhancement, hemodynamics, and morphology of intracranial fusiform aneurysms. Front. Aging Neurosci. 15:1145542. doi: 10.3389/fnagi.2023.1145542
Lin, R., Xie, Z., Zhang, J., Xu, H., Su, H., Tan, X., et al. (2012). Clinical and immunopathological features of Moyamoya disease. PLoS One 7:e36386. doi: 10.1371/journal.pone.0036386
Liu, W., Hitomi, T., Kobayashi, H., Harada, K. H., and Koizumi, A. (2012). Distribution of moyamoya disease susceptibility polymorphism p.R4810K in RNF213 in east and southeast Asian populations. Neurol. Med. Chir. (Tokyo) 52, 299–303. doi: 10.2176/nmc.52.299
Liu, S., Lu, M., Gao, X., Wen, C., Sun, B., Liu, Y., et al. (2023). The diagnostic performance of high-resolution magnetic resonance-vessel wall imaging in differentiating atherosclerosis-associated moyamoya vasculopathy from moyamoya disease. Eur. Radiol. 33, 6918–6926. doi: 10.1007/s00330-023-09951-z
Lou, X., Ma, N., Shen, H., Shi, K., Jiang, W., and Ma, L. (2014). Noninvasive visualization of the basilar artery wall and branch ostia with high-resolution three-dimensional black-blood sequence at 3 tesla. J. Magn. Reson. Imaging 39, 911–916. doi: 10.1002/jmri.24222
Lu, M., Zhang, H., Liu, D., Hao, F., Zhang, L., Peng, P., et al. (2023a). Vessel wall enhancement as a predictor of arterial stenosis progression and poor outcomes in moyamoya disease. Eur. Radiol. 33, 2489–2499. doi: 10.1007/s00330-022-09223-2
Lu, M., Zhang, H., Liu, S., Liu, D., Peng, P., Hao, F., et al. (2023b). Long-term outcomes of moyamoya disease versus atherosclerosis-associated moyamoya vasculopathy using high-resolution MR vessel wall imaging. J. Neurol. Neurosurg. Psychiatry 94, 567–574. doi: 10.1136/jnnp-2022-330542
Lu, M., Zhang, H., Liu, D., Liu, X., Zhang, L., Peng, P., et al. (2021). Association of intracranial vessel wall enhancement and cerebral hemorrhage in moyamoya disease: a high-resolution magnetic resonance imaging study. J. Neurol. 268, 4768–4777. doi: 10.1007/s00415-021-10587-6
Luo, Y., Cao, Z., Ye, H., Wu, S., and Sun, X. (2023). Antiplatelet therapy may improve the prognosis of patients with moyamoya disease: a 12-year retrospective study. J. Neurol. 270, 3876–3884. doi: 10.1007/s00415-023-11702-5
Mandell, D. M., Mossa-Basha, M., Qiao, Y., Hess, C. P., Hui, F., Matouk, C., et al. (2017). Intracranial Vessel Wall MRI: principles and expert consensus recommendations of the American Society of Neuroradiology. AJNR Am. J. Neuroradiol. 38, 218–229. doi: 10.3174/ajnr.A4893
Matsushige, T., Kraemer, M., Schlamann, M., Berlit, P., Forsting, M., Ladd, M. E., et al. (2016). Ventricular microaneurysms in Moyamoya Angiopathy visualized with 7T MR angiography. AJNR Am. J. Neuroradiol. 37, 1669–1672. doi: 10.3174/ajnr.A4786
Mikami, T., Suzuki, H., Komatsu, K., and Mikuni, N. (2019). Influence of inflammatory disease on the pathophysiology of Moyamoya disease and quasi-moyamoya disease. Neurol. Med. Chir. (Tokyo) 59, 361–370. doi: 10.2176/nmc.ra.2019-0059
Mori, T., Fukuoka, M., Kazita, K., and Mori, K. (1998). Follow-up study after intracranial percutaneous transluminal cerebral balloon angioplasty. AJNR Am. J. Neuroradiol. 19, 1525–1533.
Mossa-Basha, M., Alexander, M., Gaddikeri, S., Yuan, C., and Gandhi, D. (2016). Vessel wall imaging for intracranial vascular disease evaluation. J. Neurointerv. Surg. 8, 1154–1159. doi: 10.1136/neurintsurg-2015-012127
Mossa-Basha, M., Shibata, D. K., Hallam, D. K., de Havenon, A., Hippe, D. S., Becker, K. J., et al. (2017). Added value of vessel wall magnetic resonance imaging for differentiation of nonocclusive intracranial vasculopathies. Stroke 48, 3026–3033. doi: 10.1161/strokeaha.117.018227
Oh, B. H., Moon, H. C., Baek, H. M., Lee, Y. J., Kim, S. W., Jeon, Y. J., et al. (2017). Comparison of 7T and 3T MRI in patients with moyamoya disease. Magn. Reson. Imaging 37, 134–138. doi: 10.1016/j.mri.2016.11.019
Okazaki, S., Morimoto, T., Kamatani, Y., Kamimura, T., Kobayashi, H., Harada, K., et al. (2019). Moyamoya disease susceptibility variant RNF213 p.R4810K increases the risk of ischemic stroke attributable to large-artery atherosclerosis. Circulation 139, 295–298. doi: 10.1161/circulationaha.118.038439
Oki, K., Katsumata, M., Izawa, Y., Takahashi, S., Suzuki, N., and Houkin, K. (2018). Trends of antiplatelet therapy for the management of Moyamoya disease in Japan: results of a nationwide survey. J. Stroke Cerebrovasc. Dis. 27, 3605–3612. doi: 10.1016/j.jstrokecerebrovasdis.2018.08.030
Okuchi, S., Okada, T., Fujimoto, K., Fushimi, Y., Kido, A., Yamamoto, A., et al. (2014). Visualization of lenticulostriate arteries at 3T: optimization of slice-selective off-resonance sinc pulse-prepared TOF-MRA and its comparison with flow-sensitive black-blood MRA. Acad. Radiol. 21, 812–816. doi: 10.1016/j.acra.2014.03.007
Park, J. E., Jung, S. C., Lee, S. H., Jeon, J. Y., Lee, J. Y., Kim, H. S., et al. (2017). Comparison of 3D magnetic resonance imaging and digital subtraction angiography for intracranial artery stenosis. Eur. Radiol. 27, 4737–4746. doi: 10.1007/s00330-017-4860-6
Pfefferkorn, T., Saam, T., Rominger, A., Habs, M., Gerdes, L. A., Schmidt, C., et al. (2011). Vessel wall inflammation in spontaneous cervical artery dissection: a prospective, observational positron emission tomography, computed tomography, and magnetic resonance imaging study. Stroke 42, 1563–1568. doi: 10.1161/strokeaha.110.599548
Pilgram-Pastor, S., Chapot, R., and Kraemer, M. (2022). The angiographic presentation of European Moyamoya angiopathy. J. Neurol. 269, 997–1006. doi: 10.1007/s00415-021-10684-6
Rhim, J. K., Cho, Y. D., Jeon, J. P., Yoo, D. H., Cho, W. S., Kang, H. S., et al. (2018). Ruptured aneurysms of collateral vessels in adult onset Moyamoya disease with hemorrhagic presentation. Clin. Neuroradiol. 28, 191–199. doi: 10.1007/s00062-016-0554-8
Roder, C., Hauser, T. K., Ernemann, U., Tatagiba, M., Khan, N., and Bender, B. (2019). Arterial wall contrast enhancement in progressive moyamoya disease. J. Neurosurg. 132, 1845–1853. doi: 10.3171/2019.2.Jns19106
Ryoo, S., Cha, J., Kim, S. J., Choi, J. W., Ki, C. S., Kim, K. H., et al. (2014). High-resolution magnetic resonance wall imaging findings of Moyamoya disease. Stroke 45, 2457–2460. doi: 10.1161/strokeaha.114.004761
Ryu, J., Lee, K. M., Woo, H. G., Chung, Y., and Choi, S. K. (2022). Clinical significance of "periventricular collateral enhancement sign" in symptomatic moyamoya disease: a vessel wall imaging study. Eur. J. Radiol. 157:110599. doi: 10.1016/j.ejrad.2022.110599
Saba, L., Loewe, C., Weikert, T., Williams, M. C., Galea, N., Budde, R. P. J., et al. (2023). State-of-the-art CT and MR imaging and assessment of atherosclerotic carotid artery disease: standardization of scanning protocols and measurements-a consensus document by the European Society of Cardiovascular Radiology (ESCR). Eur. Radiol. 33, 1063–1087. doi: 10.1007/s00330-022-09024-7
Seo, W. K., Kim, J. Y., Choi, E. H., Kim, Y. S., Chung, J. W., Saver, J. L., et al. (2021). Association of Antiplatelet Therapy, including Cilostazol, with improved survival in patients with Moyamoya disease in a nationwide study. J. Am. Heart Assoc. 10:e017701. doi: 10.1161/jaha.120.017701
Shishikura, D., Kataoka, Y., Di Giovanni, G., Takata, K., Scherer, D. J., Andrews, J., et al. (2020). Progression of ultrasound plaque attenuation and low echogenicity associates with major adverse cardiovascular events. Eur. Heart J. 41, 2965–2973. doi: 10.1093/eurheartj/ehaa173
Suzuki, J., and Takaku, A. (1969). Cerebrovascular "moyamoya" disease. Disease showing abnormal net-like vessels in base of brain. Arch. Neurol. 20, 288–299. doi: 10.1001/archneur.1969.00480090076012
Takagi, Y., Kikuta, K., Nozaki, K., Fujimoto, M., Hayashi, J., Imamura, H., et al. (2007). Expression of hypoxia-inducing factor-1 alpha and endoglin in intimal hyperplasia of the middle cerebral artery of patients with Moyamoya disease. Neurosurgery 60:338-345; discussion 345. doi: 10.1227/01.Neu.0000249275.87310.Ff
Tho-Calvi, S. C., Thompson, D., Saunders, D., Agrawal, S., Basu, A., Chitre, M., et al. (2018). Clinical features, course, and outcomes of a UK cohort of pediatric moyamoya. Neurology 90, e763–e770. doi: 10.1212/wnl.0000000000005026
Togao, O., Hiwatashi, A., Obara, M., Yamashita, K., Momosaka, D., Nishimura, A., et al. (2018). 4D ASL-based MR angiography for visualization of distal arteries and leptomeningeal collateral vessels in moyamoya disease: a comparison of techniques. Eur. Radiol. 28, 4871–4881. doi: 10.1007/s00330-018-5462-7
Treitl, K. M., Maurus, S., Sommer, N. N., Kooijman-Kurfuerst, H., Coppenrath, E., Treitl, M., et al. (2017). 3D-black-blood 3T-MRI for the diagnosis of thoracic large vessel vasculitis: a feasibility study. Eur. Radiol. 27, 2119–2128. doi: 10.1007/s00330-016-4525-x
van den Wijngaard, I. R., Holswilder, G., van Walderveen, M. A., Algra, A., Wermer, M. J., Zaidat, O. O., et al. (2016). Treatment and imaging of intracranial atherosclerotic stenosis: current perspectives and future directions. Brain Behav. 6:e00536. doi: 10.1002/brb3.536
Waddle, S. L., Garza, M., Davis, L. T., Chitale, R. V., Fusco, M. R., Lee, C. A., et al. (2022). Presurgical magnetic resonance imaging indicators of revascularization response in adults with Moyamoya vasculopathy. J. Magn. Reson. Imaging 56, 983–994. doi: 10.1002/jmri.28156
Wan, Y., Teng, X., Li, S., and Yang, Y. (2022). Application of transcranial Doppler in cerebrovascular diseases. Front. Aging Neurosci. 14:1035086. doi: 10.3389/fnagi.2022.1035086
Wang, X., Wang, Y., Nie, F., Li, Q., Zhang, K., Liu, M., et al. (2020). Association of genetic variants with moyamoya disease in 13000 individuals: a meta-analysis. Stroke 51, 1647–1655. doi: 10.1161/strokeaha.120.029527
Wang, M., Yang, Y., Zhou, F., Li, M., Liu, R., Guan, M., et al. (2017). The contrast enhancement of intracranial arterial wall on high-resolution MRI and its clinical relevance in patients with Moyamoya vasculopathy. Sci. Rep. 7:44264. doi: 10.1038/srep44264
Wang, J., Zhang, S., Lu, J., Qi, P., Hu, S., Yang, X., et al. (2021). High-resolution MR for follow-up of intracranial Steno-occlusive disease treated by endovascular treatment. Front. Neurol. 12:706645. doi: 10.3389/fneur.2021.706645
Wiggins, G. C., Triantafyllou, C., Potthast, A., Reykowski, A., Nittka, M., and Wald, L. L. (2006). 32-channel 3 tesla receive-only phased-array head coil with soccer-ball element geometry. Magn. Reson. Med. 56, 216–223. doi: 10.1002/mrm.20925
Wu, F., Han, C., Liu, Y., Liu, Z., Yang, X., Wu, Y., et al. (2021). Validation of choroidal anastomosis on high-resolution magnetic resonance imaging as an imaging biomarker in hemorrhagic moyamoya disease. Eur. Radiol. 31, 4548–4556. doi: 10.1007/s00330-020-07479-0
Xu, J., Rajah, G. B., Zhang, H., Han, C., Shen, X., Li, B., et al. (2022). Imaging features of adult moyamoya disease patients with anterior intracerebral hemorrhage based on high-resolution magnetic resonance imaging. J. Cereb. Blood Flow Metab. 42, 2123–2133. doi: 10.1177/0271678x221111082
Xu, J., Wu, J., Lei, Y., and Gu, Y. (2023). Application of Pseudo-three-dimensional residual network to classify the stages of Moyamoya disease. Brain Sci. 13:742. doi: 10.3390/brainsci13050742
Ya, J., Zhou, D., Ding, J., Ding, Y., Ji, X., Yang, Q., et al. (2020). High-resolution combined arterial spin labeling MR for identifying cerebral arterial stenosis induced by moyamoya disease or atherosclerosis. Ann. Transl. Med. 8:87. doi: 10.21037/atm.2019.12.140
Yamamoto, T., Okada, T., Fushimi, Y., Yamamoto, A., Fujimoto, K., Okuchi, S., et al. (2018). Magnetic resonance angiography with compressed sensing: an evaluation of moyamoya disease. PLoS One 13:e0189493. doi: 10.1371/journal.pone.0189493
Yamashita, M., Oka, K., and Tanaka, K. (1983). Histopathology of the brain vascular network in moyamoya disease. Stroke 14, 50–58. doi: 10.1161/01.str.14.1.50
Yang, S., Wang, X., Liao, W., Li, L., Tan, Z., Zhu, L., et al. (2021). High-resolution MRI of the vessel wall helps to distinguish moyamoya disease from atherosclerotic moyamoya syndrome. Clin. Radiol. 76, 392.e11–392.e19. doi: 10.1016/j.crad.2020.12.023
Young, C. C., Bonow, R. H., Barros, G., Mossa-Basha, M., Kim, L. J., and Levitt, M. R. (2019). Magnetic resonance vessel wall imaging in cerebrovascular diseases. Neurosurg. Focus. 47:E4. doi: 10.3171/2019.9.Focus19599
Yu, L. B., Shen, M., Zhang, Q., and Zhang, D. (2023). A vessel wall MRI investigation in patients with Moyamoya or quasi-Moyamoya disease: diagnosis, features, and outcomes. J. Craniofac. Surg. 35, e24–e28. doi: 10.1097/scs.0000000000009681
Yu, L. B., Zhang, Q., Shi, Z. Y., Wang, M. Q., and Zhang, D. (2015). High-resolution magnetic resonance imaging of Moyamoya disease. Chin. Med. J. 128, 3231–3237. doi: 10.4103/0366-6999.170257
Yuan, M., Liu, Z. Q., Wang, Z. Q., Li, B., Xu, L. J., and Xiao, X. L. (2015). High-resolution MR imaging of the arterial wall in moyamoya disease. Neurosci. Lett. 584, 77–82. doi: 10.1016/j.neulet.2014.10.021
Yuan, C., Mitsumori, L. M., Ferguson, M. S., Polissar, N. L., Echelard, D., Ortiz, G., et al. (2001). In vivo accuracy of multispectral magnetic resonance imaging for identifying lipid-rich necrotic cores and intraplaque hemorrhage in advanced human carotid plaques. Circulation 104, 2051–2056. doi: 10.1161/hc4201.097839
Yuan, C., and Parker, D. L. (2016). Three-dimensional carotid plaque MR imaging. Neuroimaging Clin. N. Am. 26, 1–12. doi: 10.1016/j.nic.2015.09.001
Zhang, D., Huang, L., Huang, Z., Zhou, Q., Yang, X., Gu, H., et al. (2022). Epidemiology of Moyamoya disease in China: a nationwide hospital-based study. Lancet Reg. Health West Pac. 18:100331. doi: 10.1016/j.lanwpc.2021.100331
Zhang, H., Lu, M., Liu, S., Liu, D., Liu, X., Shen, X., et al. (2023). Predictors of stroke outcomes in conservatively treated patients with Moyamoya disease: a follow-up MRI study. J. Magn. Reson. Imaging 59, 1456–1463. doi: 10.1002/jmri.28880
Zhang, Z., Wang, Y., Zhou, S., Li, Z., Peng, Y., Gao, S., et al. (2023). The automatic evaluation of steno-occlusive changes in time-of-flight magnetic resonance angiography of moyamoya patients using a 3D coordinate attention residual network. Quant. Imaging Med. Surg. 13, 1009–1022. doi: 10.21037/qims-22-799
Zhang, H., Zheng, L., and Feng, L. (2019). Epidemiology, diagnosis and treatment of moyamoya disease. Exp. Ther. Med. 17, 1977–1984. doi: 10.3892/etm.2019.7198
Glossary
Keywords:
Citation: Yang H, Huang G, Li X, Wu M, Zhou W, Yin X, Zhang M and Chen Z (2024) High-resolution magnetic resonance vessel wall imaging provides new insights into Moyamoya disease. Front. Neurosci. 18:1375645. doi: 10.3389/fnins.2024.1375645
Edited by:
Lirong Yan, University of Southern California, United StatesReviewed by:
Han Cong, Fifth Medical Center of the PLA General Hospital, ChinaJianming Cai, People's Liberation Army General Hospital, China
Copyright © 2024 Yang, Huang, Li, Wu, Zhou, Yin, Zhang and Chen. This is an open-access article distributed under the terms of the Creative Commons Attribution License (CC BY). The use, distribution or reproduction in other forums is permitted, provided the original author(s) and the copyright owner(s) are credited and that the original publication in this journal is cited, in accordance with accepted academic practice. No use, distribution or reproduction is permitted which does not comply with these terms.
*Correspondence: Zhiying Chen, zychenjj@jju.edu.cn; Manqing Zhang, zmqzhang328@163.com
†These authors have contributed equally to this work